Abstract
We studied the mechanism of the lamellar-to-inverted hexagonal (L alpha/H[II]) phase transition, using time-resolved cryotransmission electron microscopy (TRC-TEM), 31P-NMR, and differential scanning calorimetry. The transition was initiated in dispersions of large unilamellar vesicles of dipalmitoleoyl phosphatidylethanolamine (DiPoPE). We present evidence that the transition proceeds in three steps. First, many small connections form between apposed membranes. Second, the connections aggregate within the planes of the bilayers, forming arrays with hexagonal order in some projections. Third, these quasihexagonal structures elongate into small domains of H(II) phase, acquiring lipid molecules by diffusion from contiguous bilayers. A previously proposed membrane fusion mechanism rationalizes these results. The modified stalk theory predicts that the L alpha/H(II) phase transition involves some of the same intermediate structures as membrane fusion. The small interbilayer connections observed via TRC-TEM are compatible with the structure of a critical intermediate in the modified stalk mechanism: the trans monolayer contact (TMC). The theory predicts that 1) TMCs should form starting at tens of degrees below TH; 2) when TMCs become sufficiently numerous, they should aggregate into transient arrays like the quasihexagonal arrays observed here by TRC-TEM; and 3) these quasihexagonal arrays can then elongate directly into H(II) phase domains. These predictions rationalize the principal features of our data, which are incompatible with the other transition mechanisms proposed to date. Thus these results support the modified stalk mechanism for both membrane fusion and the L alpha/H(II) phase transition. We also discuss some implications of the modified stalk theory for fusion in protein-containing systems. Specifically, we point out that recent data on the effects of hydrophobic peptides and viral fusion peptides on lipid phase behavior are consistent with an effect of the peptides on TMC stability.
Full text
PDF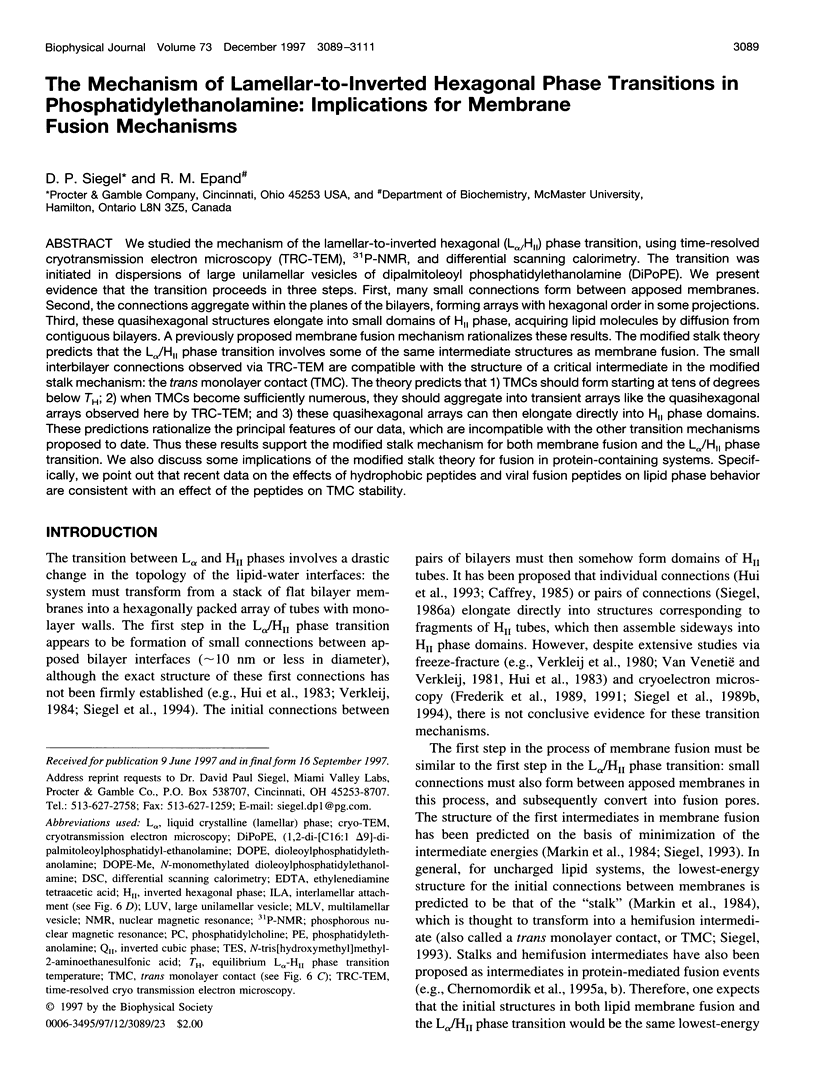
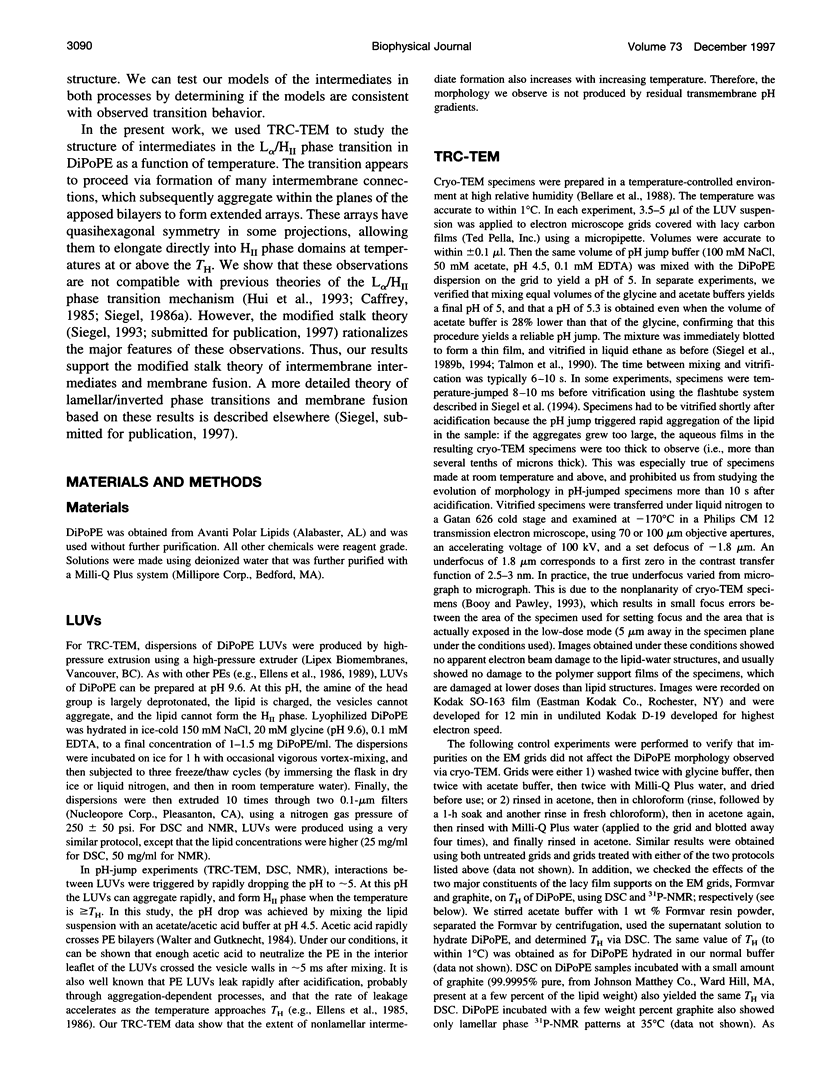
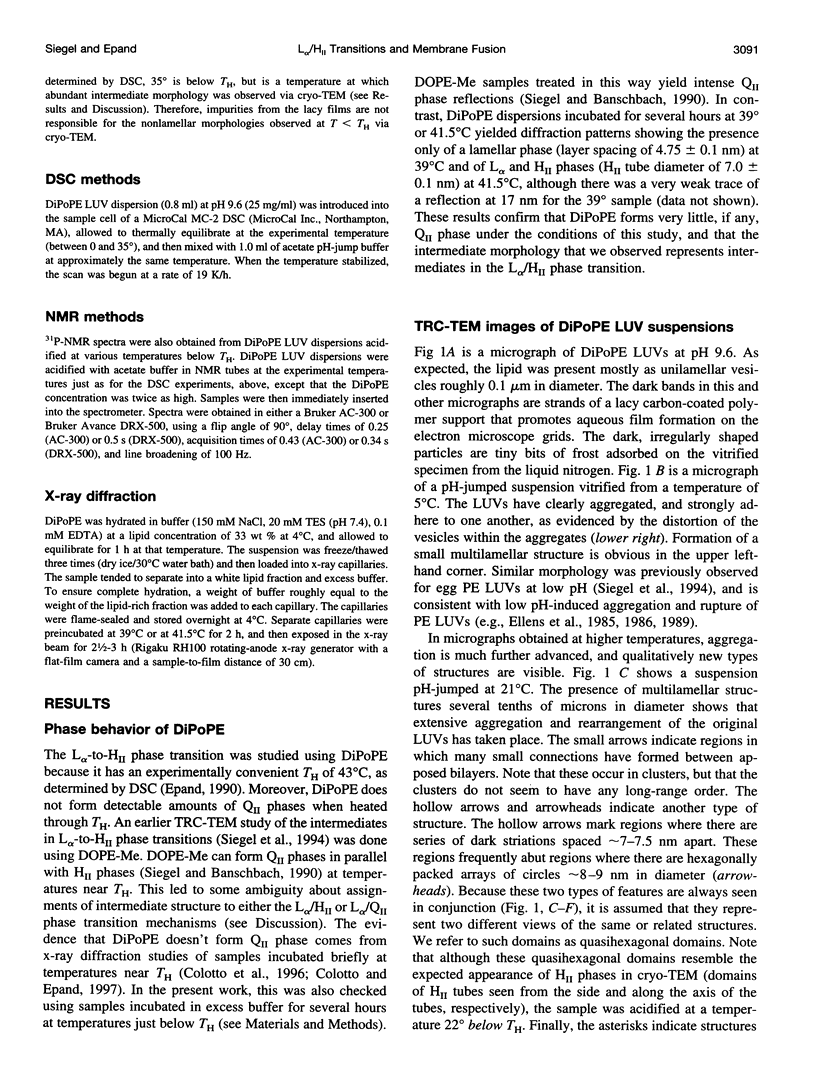
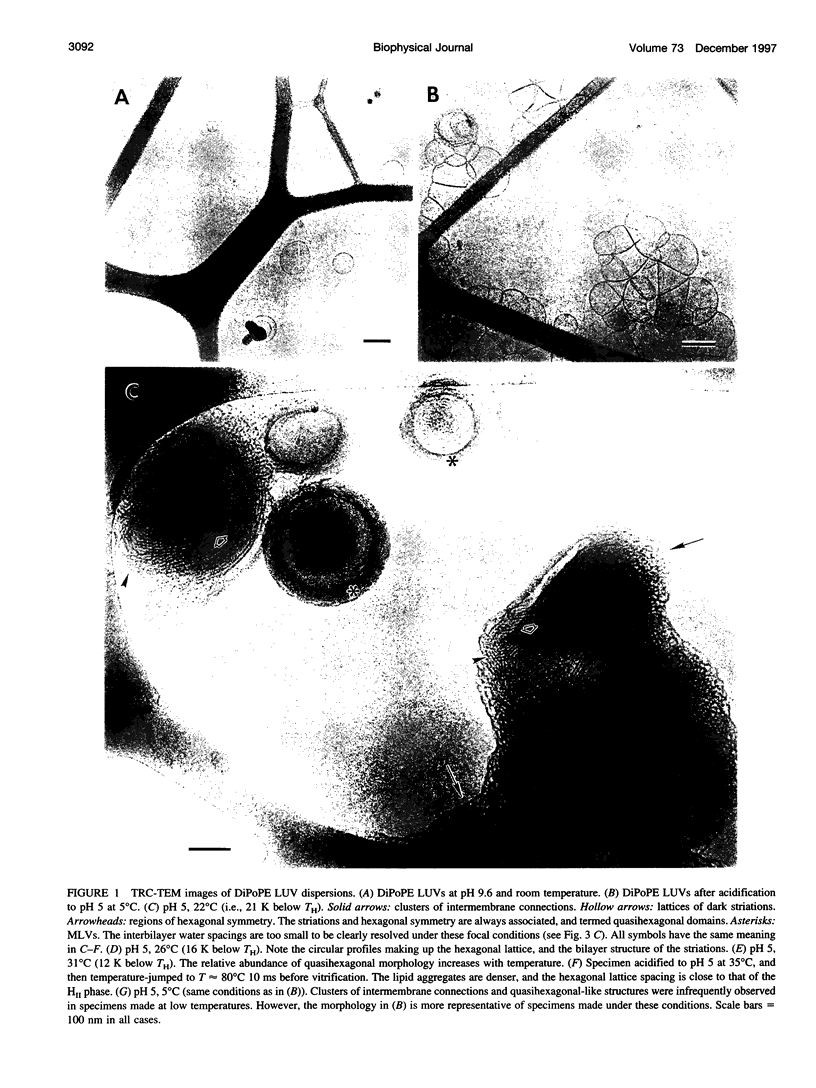
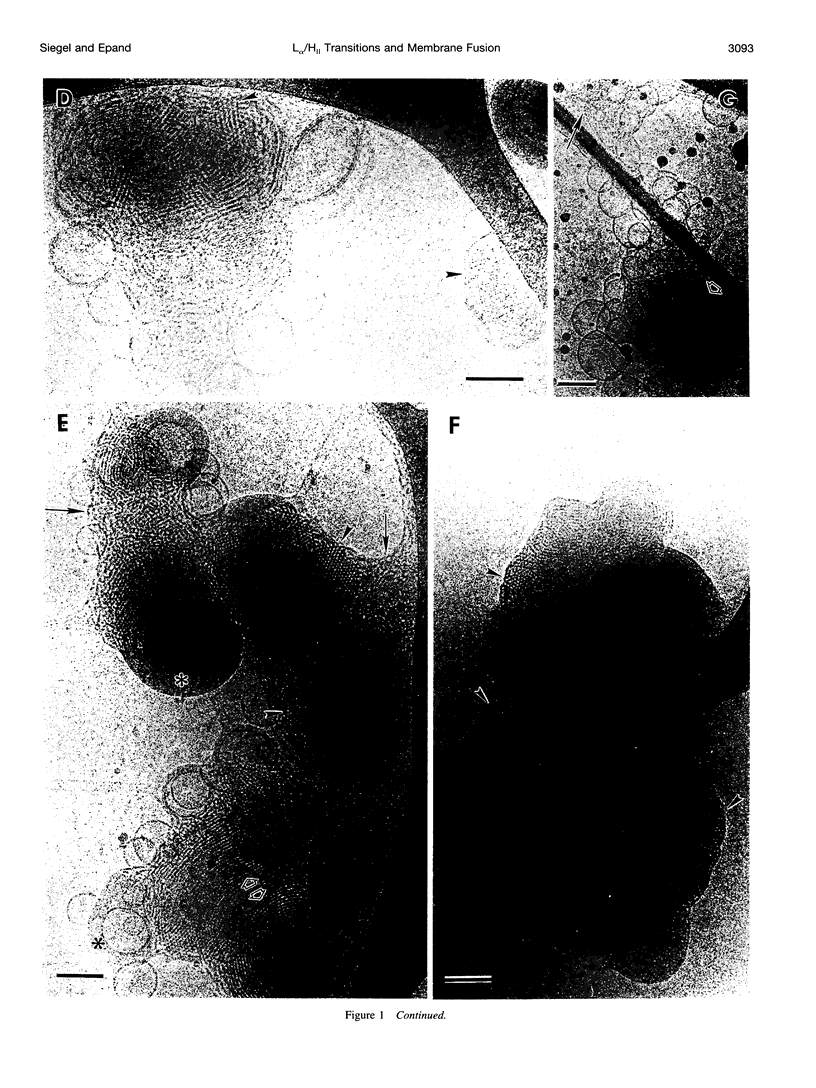
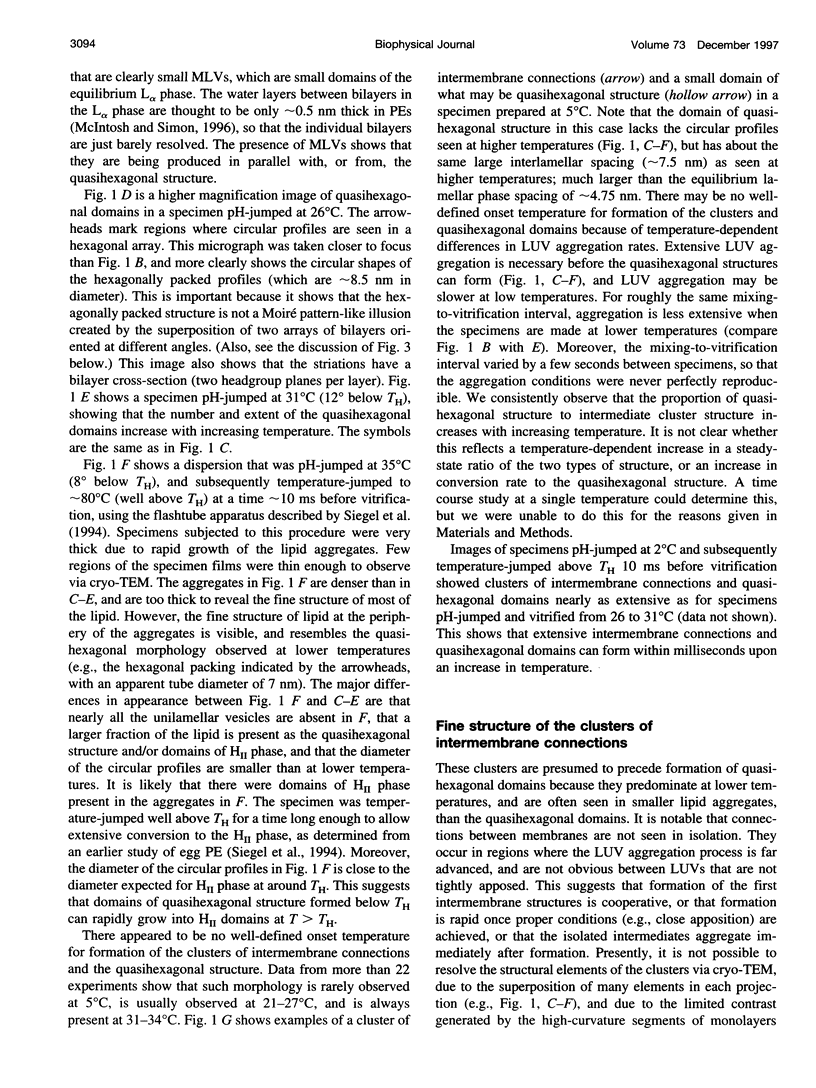
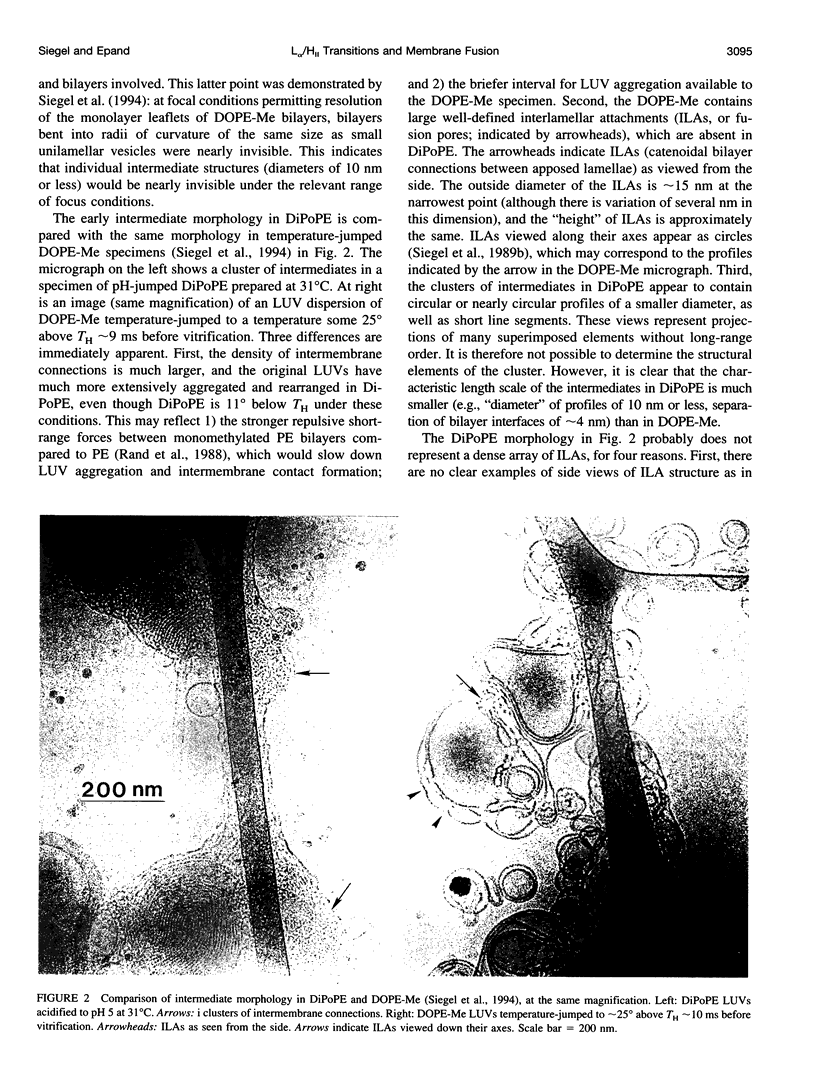
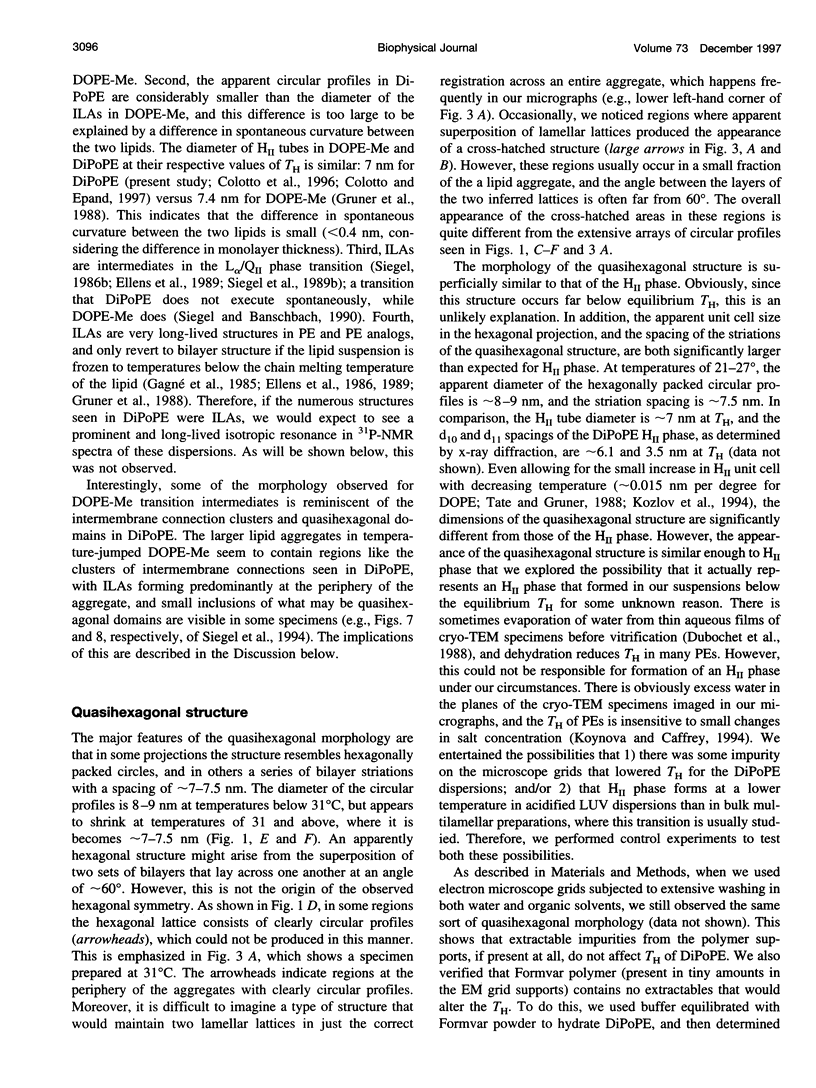
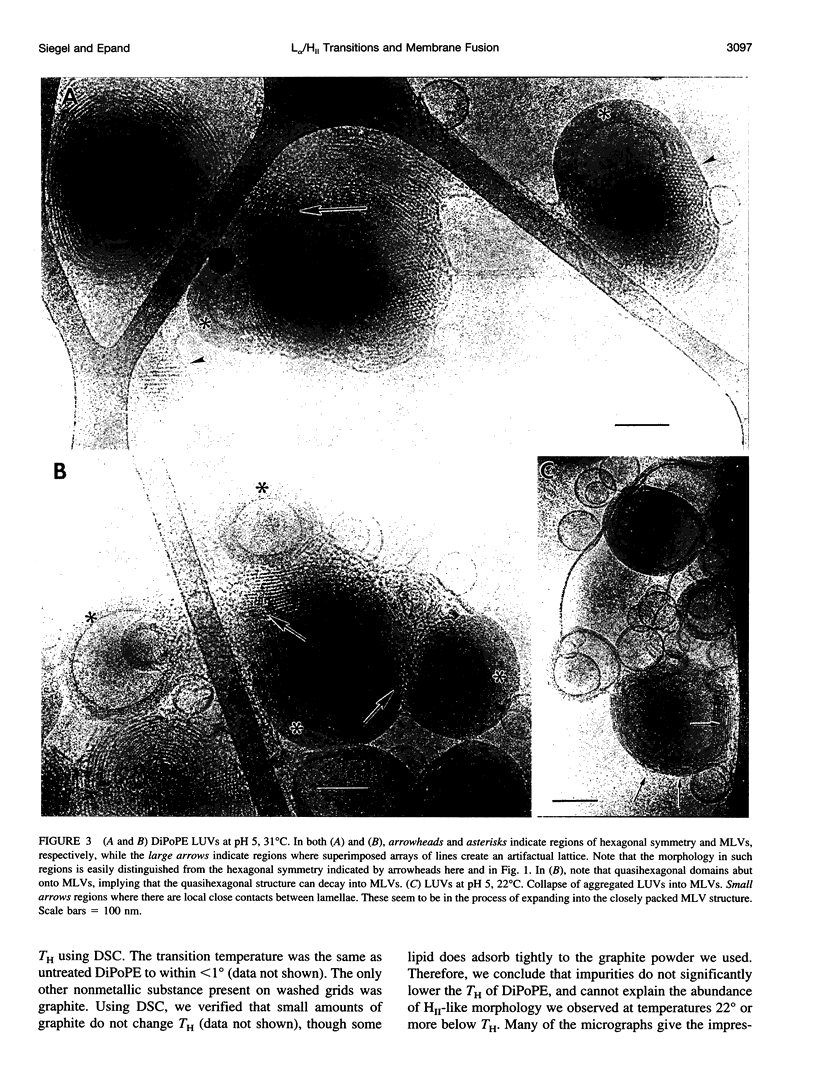
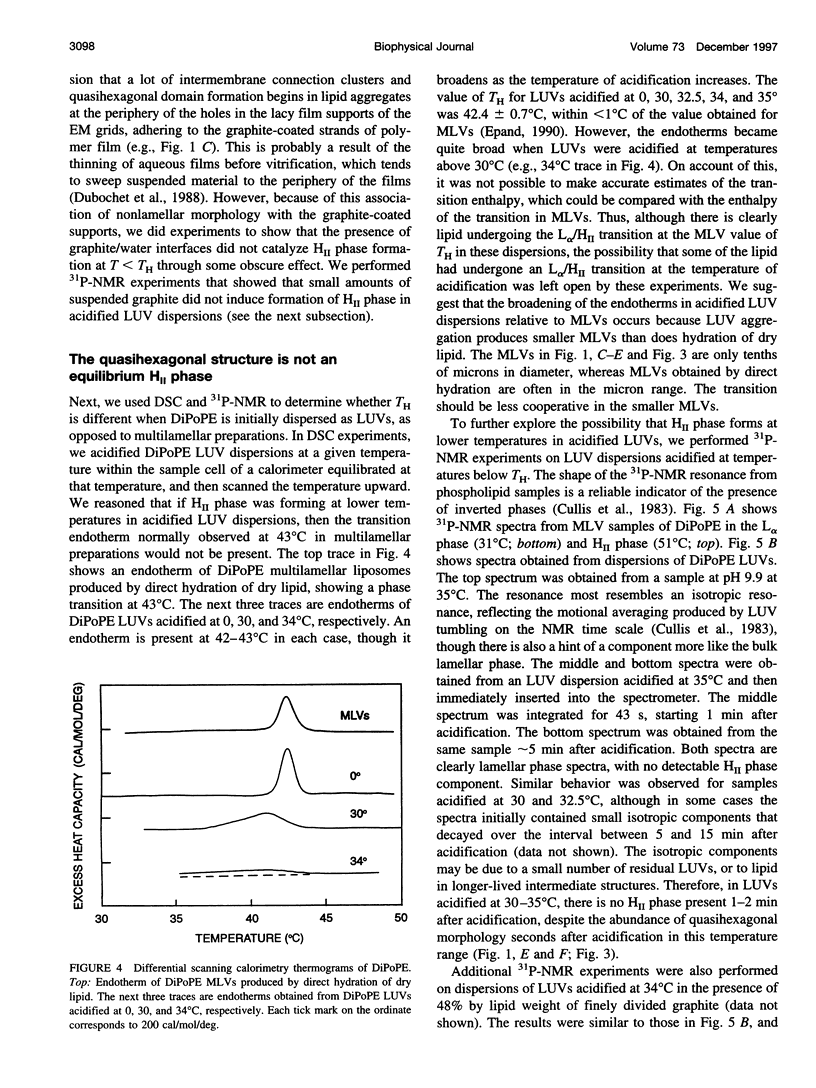
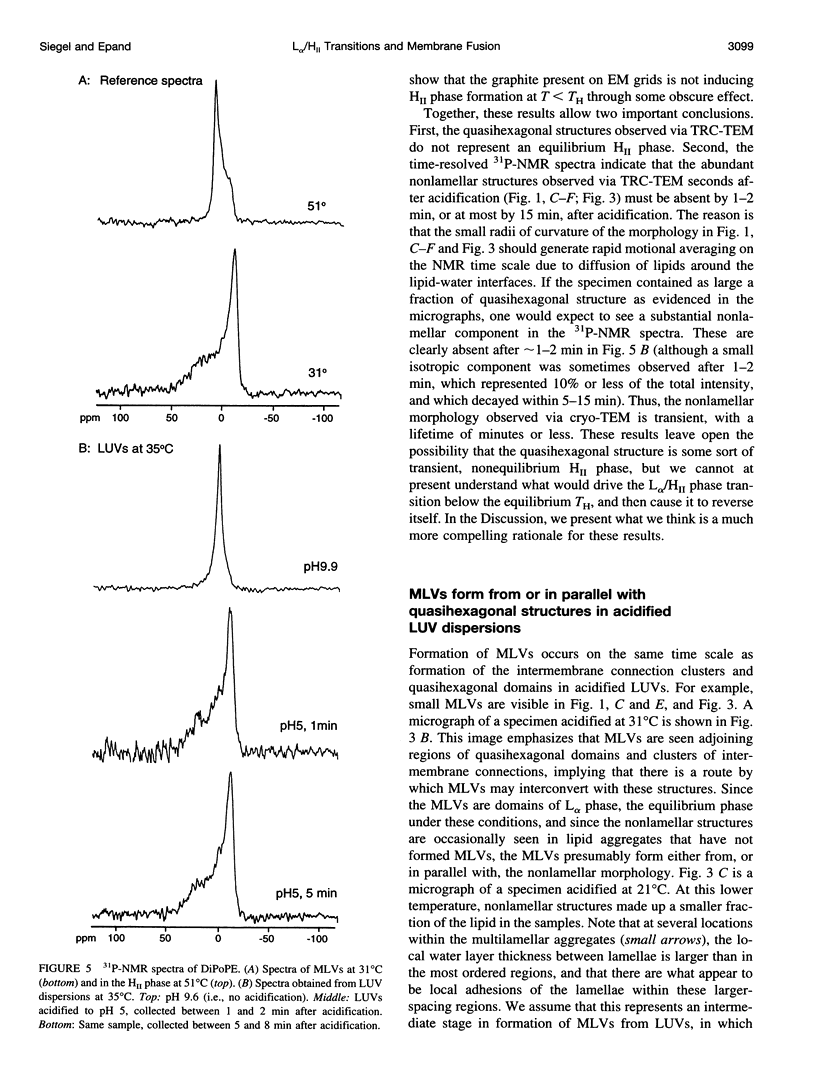
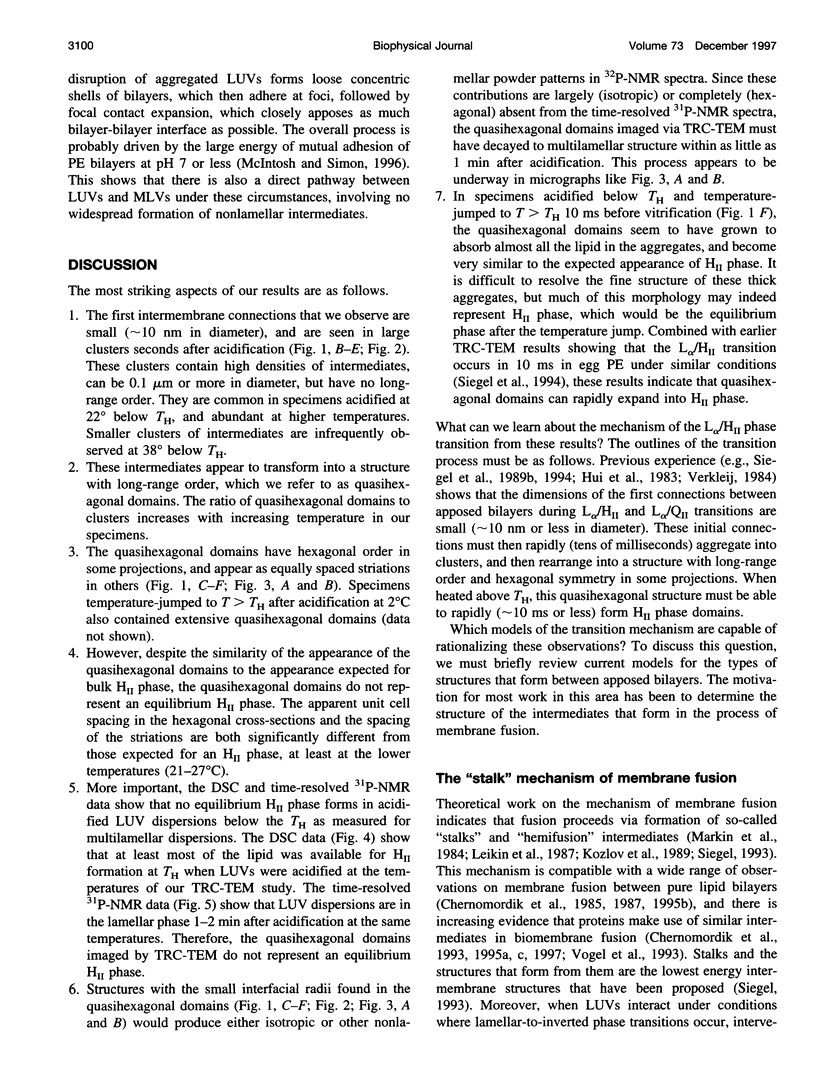
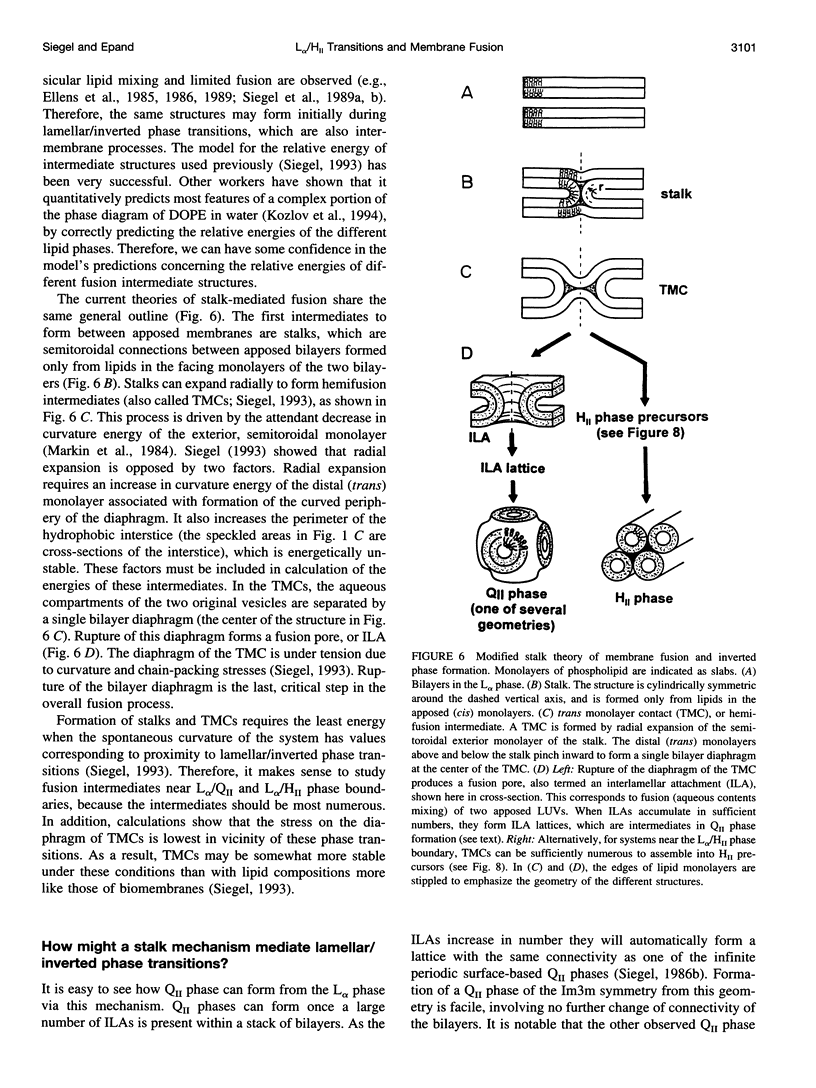
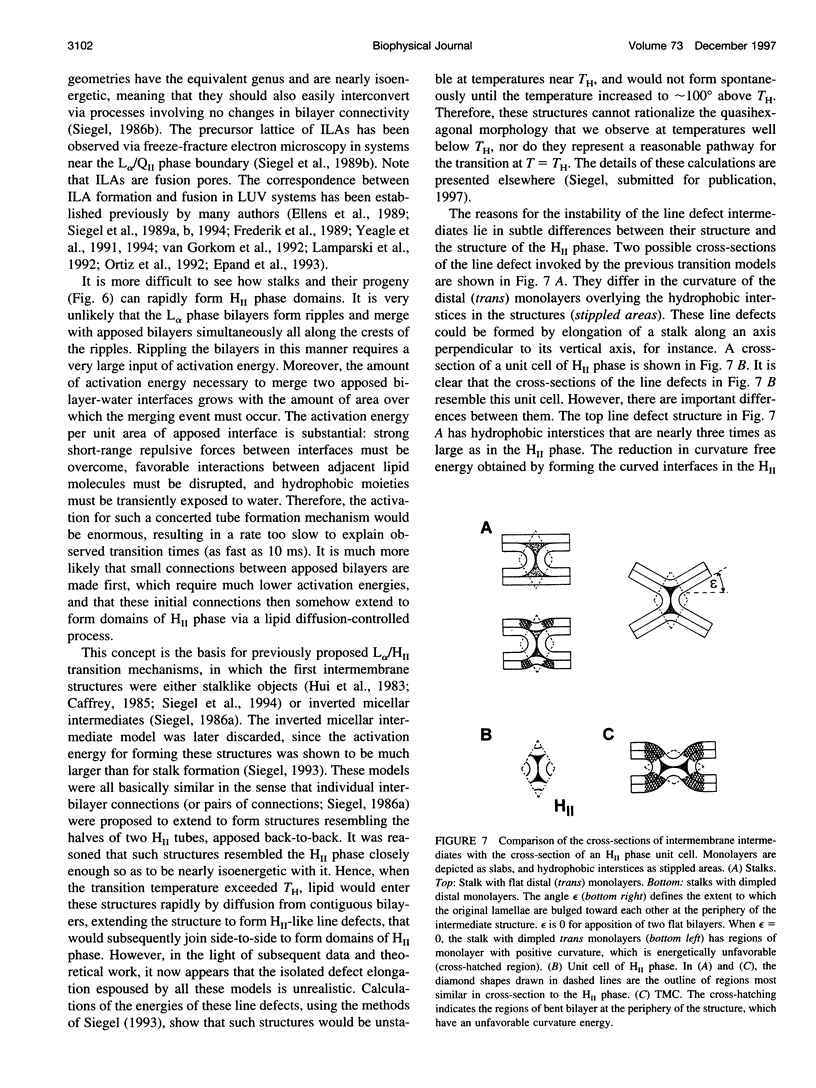
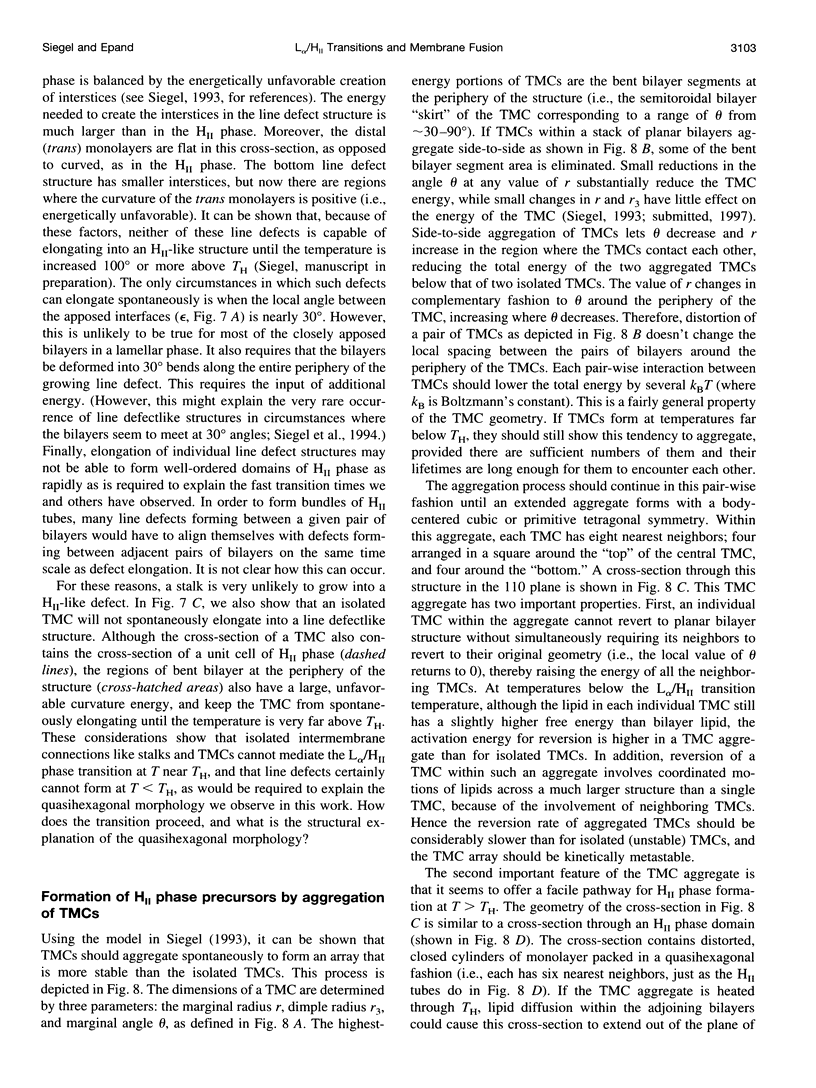
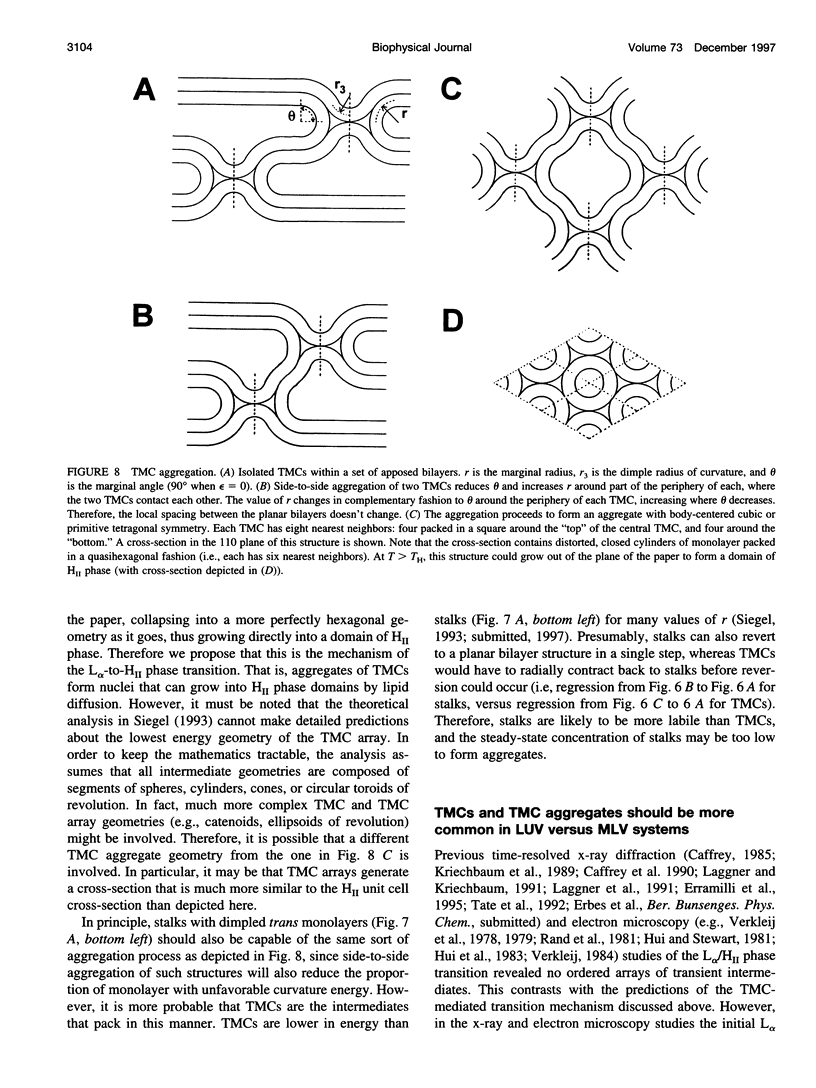
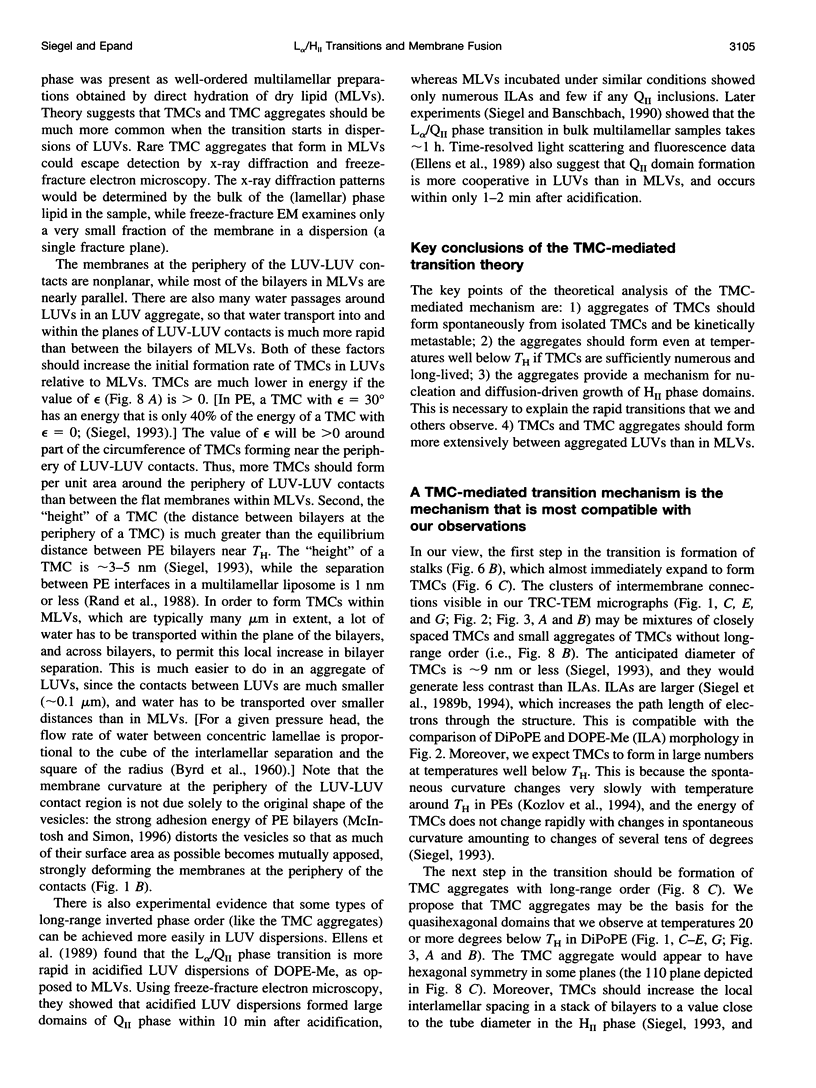
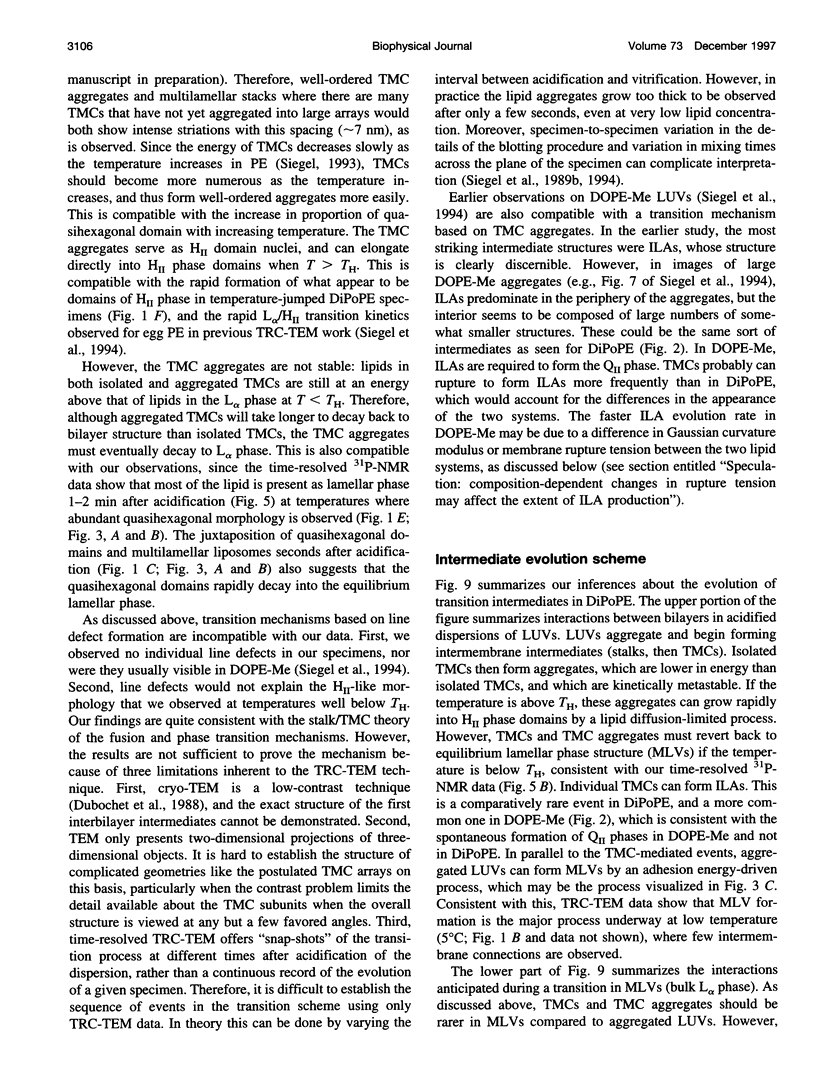
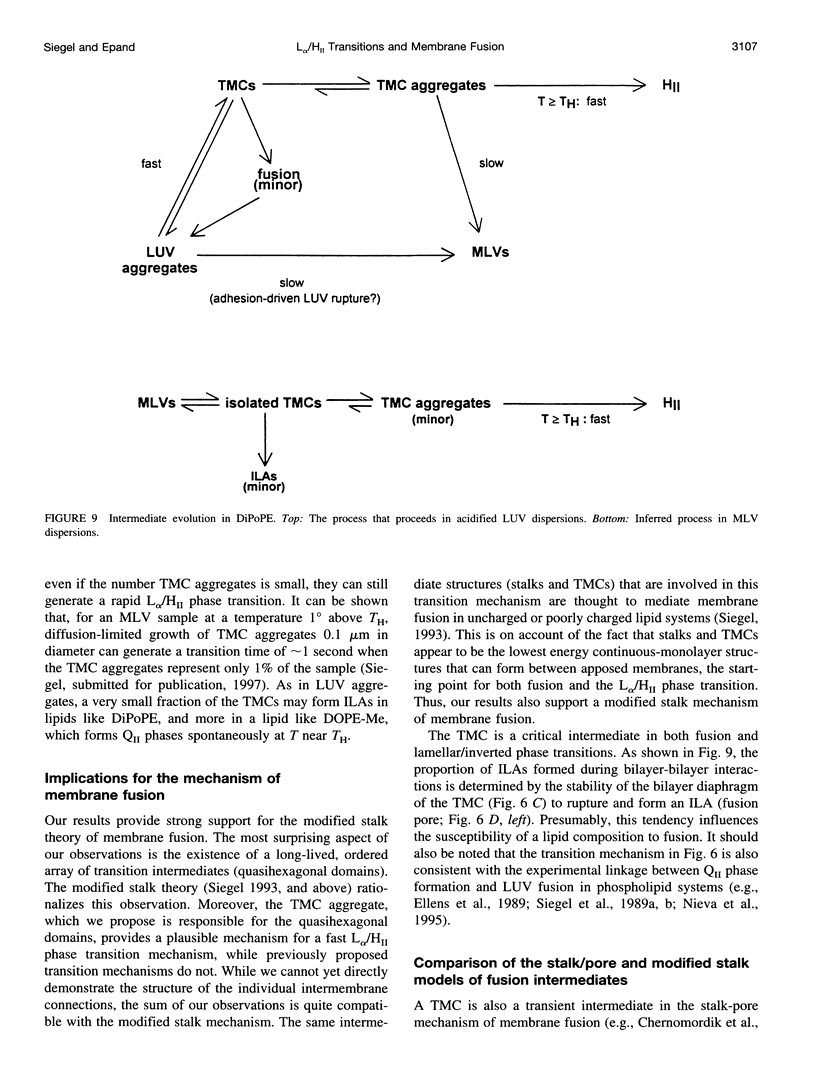
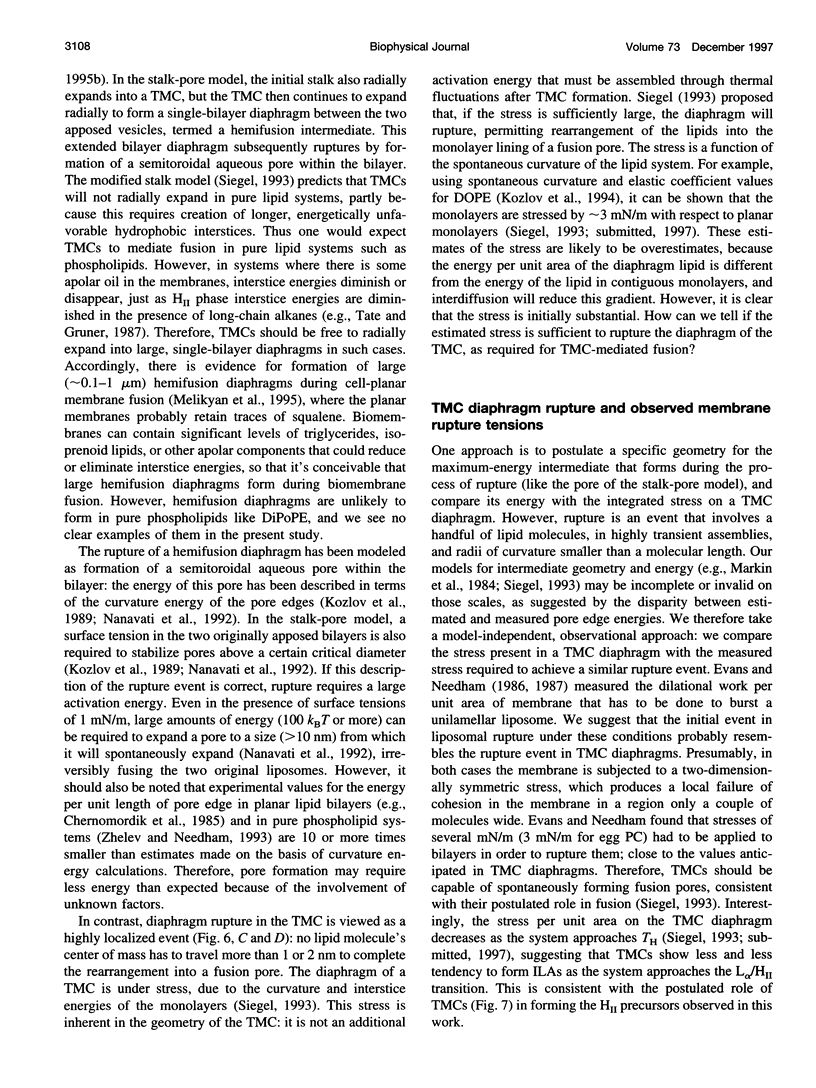
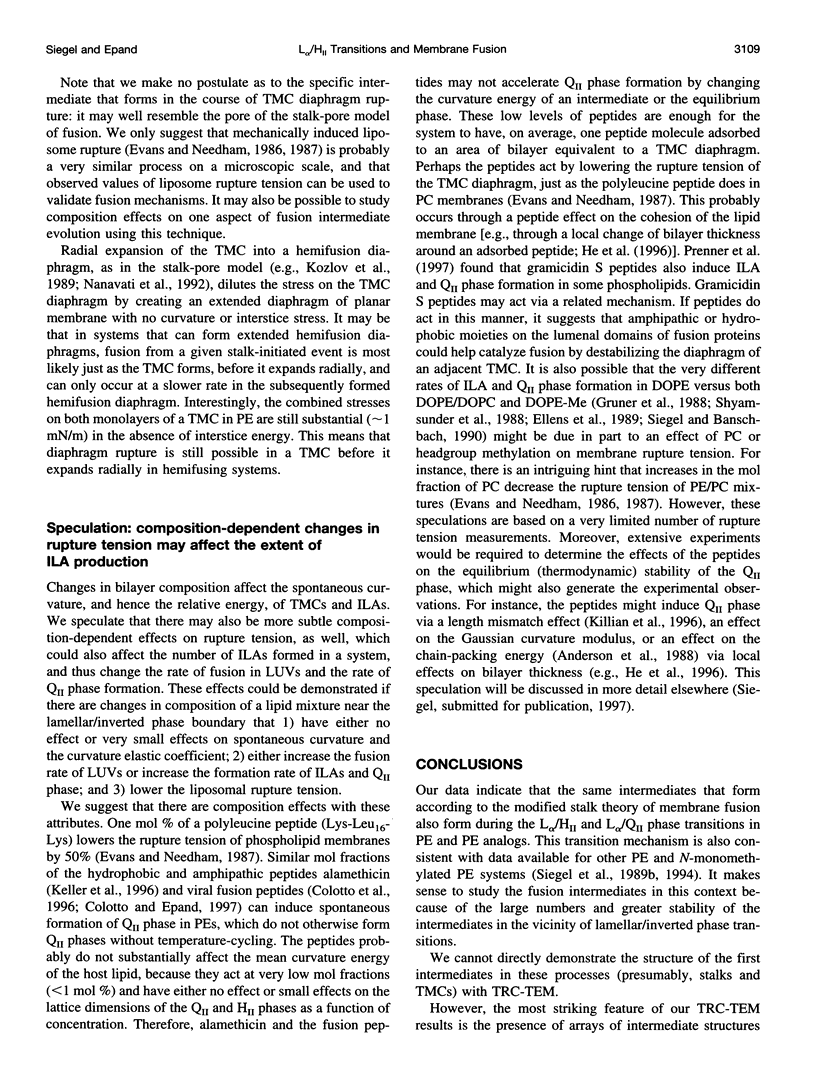
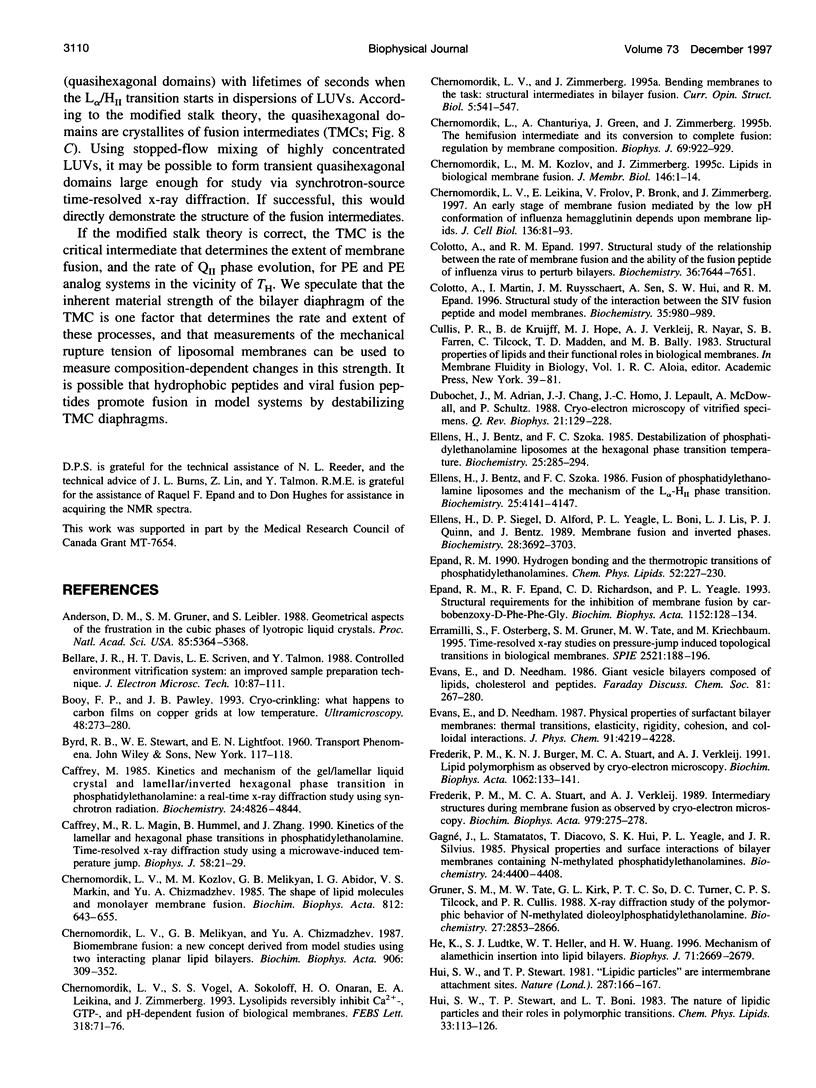
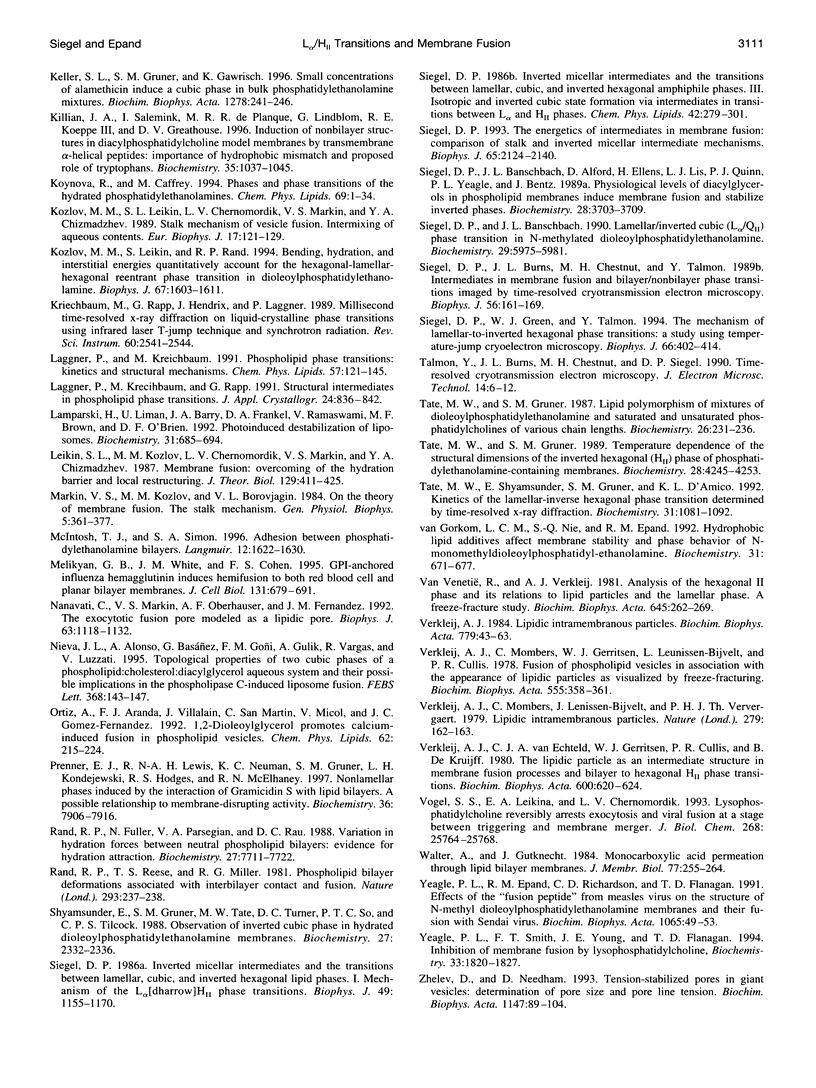
Images in this article
Selected References
These references are in PubMed. This may not be the complete list of references from this article.
- Anderson D. M., Gruner S. M., Leibler S. Geometrical aspects of the frustration in the cubic phases of lyotropic liquid crystals. Proc Natl Acad Sci U S A. 1988 Aug;85(15):5364–5368. doi: 10.1073/pnas.85.15.5364. [DOI] [PMC free article] [PubMed] [Google Scholar]
- Bellare J. R., Davis H. T., Scriven L. E., Talmon Y. Controlled environment vitrification system: an improved sample preparation technique. J Electron Microsc Tech. 1988 Sep;10(1):87–111. doi: 10.1002/jemt.1060100111. [DOI] [PubMed] [Google Scholar]
- Booy F. P., Pawley J. B. Cryo-crinkling: what happens to carbon films on copper grids at low temperature. Ultramicroscopy. 1993 Mar;48(3):273–280. doi: 10.1016/0304-3991(93)90101-3. [DOI] [PubMed] [Google Scholar]
- Caffrey M. Kinetics and mechanism of the lamellar gel/lamellar liquid-crystal and lamellar/inverted hexagonal phase transition in phosphatidylethanolamine: a real-time X-ray diffraction study using synchrotron radiation. Biochemistry. 1985 Aug 27;24(18):4826–4844. doi: 10.1021/bi00339a017. [DOI] [PubMed] [Google Scholar]
- Caffrey M., Magin R. L., Hummel B., Zhang J. Kinetics of the lamellar and hexagonal phase transitions in phosphatidylethanolamine. Time-resolved x-ray diffraction study using a microwave-induced temperature jump. Biophys J. 1990 Jul;58(1):21–29. doi: 10.1016/S0006-3495(90)82350-X. [DOI] [PMC free article] [PubMed] [Google Scholar]
- Chernomordik L. V., Leikina E., Frolov V., Bronk P., Zimmerberg J. An early stage of membrane fusion mediated by the low pH conformation of influenza hemagglutinin depends upon membrane lipids. J Cell Biol. 1997 Jan 13;136(1):81–93. doi: 10.1083/jcb.136.1.81. [DOI] [PMC free article] [PubMed] [Google Scholar]
- Chernomordik L. V., Melikyan G. B., Chizmadzhev Y. A. Biomembrane fusion: a new concept derived from model studies using two interacting planar lipid bilayers. Biochim Biophys Acta. 1987 Oct 5;906(3):309–352. doi: 10.1016/0304-4157(87)90016-5. [DOI] [PubMed] [Google Scholar]
- Chernomordik L. V., Vogel S. S., Sokoloff A., Onaran H. O., Leikina E. A., Zimmerberg J. Lysolipids reversibly inhibit Ca(2+)-, GTP- and pH-dependent fusion of biological membranes. FEBS Lett. 1993 Feb 22;318(1):71–76. doi: 10.1016/0014-5793(93)81330-3. [DOI] [PubMed] [Google Scholar]
- Chernomordik L. V., Zimmerberg J. Bending membranes to the task: structural intermediates in bilayer fusion. Curr Opin Struct Biol. 1995 Aug;5(4):541–547. doi: 10.1016/0959-440x(95)80041-7. [DOI] [PubMed] [Google Scholar]
- Chernomordik L., Chanturiya A., Green J., Zimmerberg J. The hemifusion intermediate and its conversion to complete fusion: regulation by membrane composition. Biophys J. 1995 Sep;69(3):922–929. doi: 10.1016/S0006-3495(95)79966-0. [DOI] [PMC free article] [PubMed] [Google Scholar]
- Chernomordik L., Kozlov M. M., Zimmerberg J. Lipids in biological membrane fusion. J Membr Biol. 1995 Jul;146(1):1–14. doi: 10.1007/BF00232676. [DOI] [PubMed] [Google Scholar]
- Colotto A., Epand R. M. Structural study of the relationship between the rate of membrane fusion and the ability of the fusion peptide of influenza virus to perturb bilayers. Biochemistry. 1997 Jun 24;36(25):7644–7651. doi: 10.1021/bi970382u. [DOI] [PubMed] [Google Scholar]
- Colotto A., Martin I., Ruysschaert J. M., Sen A., Hui S. W., Epand R. M. Structural study of the interaction between the SIV fusion peptide and model membranes. Biochemistry. 1996 Jan 23;35(3):980–989. doi: 10.1021/bi951991+. [DOI] [PubMed] [Google Scholar]
- Dubochet J., Adrian M., Chang J. J., Homo J. C., Lepault J., McDowall A. W., Schultz P. Cryo-electron microscopy of vitrified specimens. Q Rev Biophys. 1988 May;21(2):129–228. doi: 10.1017/s0033583500004297. [DOI] [PubMed] [Google Scholar]
- Ellens H., Bentz J., Szoka F. C. Destabilization of phosphatidylethanolamine liposomes at the hexagonal phase transition temperature. Biochemistry. 1986 Jan 28;25(2):285–294. doi: 10.1021/bi00350a001. [DOI] [PubMed] [Google Scholar]
- Ellens H., Bentz J., Szoka F. C. Fusion of phosphatidylethanolamine-containing liposomes and mechanism of the L alpha-HII phase transition. Biochemistry. 1986 Jul 15;25(14):4141–4147. doi: 10.1021/bi00362a023. [DOI] [PubMed] [Google Scholar]
- Ellens H., Siegel D. P., Alford D., Yeagle P. L., Boni L., Lis L. J., Quinn P. J., Bentz J. Membrane fusion and inverted phases. Biochemistry. 1989 May 2;28(9):3692–3703. doi: 10.1021/bi00435a011. [DOI] [PubMed] [Google Scholar]
- Epand R. M., Epand R. F., Richardson C. D., Yeagle P. L. Structural requirements for the inhibition of membrane fusion by carbobenzoxy-D-Phe-Phe-Gly. Biochim Biophys Acta. 1993 Oct 10;1152(1):128–134. doi: 10.1016/0005-2736(93)90239-v. [DOI] [PubMed] [Google Scholar]
- Evans E., Needham D. Giant vesicle bilayers composed of mixtures of lipids, cholesterol and polypeptides. Thermomechanical and (mutual) adherence properties. Faraday Discuss Chem Soc. 1986;(81):267–280. doi: 10.1039/dc9868100267. [DOI] [PubMed] [Google Scholar]
- Frederik P. M., Burger K. N., Stuart M. C., Verkleij A. J. Lipid polymorphism as observed by cryo-electron microscopy. Biochim Biophys Acta. 1991 Feb 25;1062(2):133–141. doi: 10.1016/0005-2736(91)90384-k. [DOI] [PubMed] [Google Scholar]
- Frederik P. M., Stuart M. C., Verkleij A. J. Intermediary structures during membrane fusion as observed by cryo-electron microscopy. Biochim Biophys Acta. 1989 Feb 27;979(2):275–278. doi: 10.1016/0005-2736(89)90445-8. [DOI] [PubMed] [Google Scholar]
- Gagné J., Stamatatos L., Diacovo T., Hui S. W., Yeagle P. L., Silvius J. R. Physical properties and surface interactions of bilayer membranes containing N-methylated phosphatidylethanolamines. Biochemistry. 1985 Jul 30;24(16):4400–4408. doi: 10.1021/bi00337a022. [DOI] [PubMed] [Google Scholar]
- Gruner S. M., Tate M. W., Kirk G. L., So P. T., Turner D. C., Keane D. T., Tilcock C. P., Cullis P. R. X-ray diffraction study of the polymorphic behavior of N-methylated dioleoylphosphatidylethanolamine. Biochemistry. 1988 Apr 19;27(8):2853–2866. doi: 10.1021/bi00408a029. [DOI] [PubMed] [Google Scholar]
- He K., Ludtke S. J., Heller W. T., Huang H. W. Mechanism of alamethicin insertion into lipid bilayers. Biophys J. 1996 Nov;71(5):2669–2679. doi: 10.1016/S0006-3495(96)79458-4. [DOI] [PMC free article] [PubMed] [Google Scholar]
- Hui S. W., Stewart T. P., Boni L. T. The nature of lipidic particles and their roles in polymorphic transitions. Chem Phys Lipids. 1983 Aug;33(2):113–126. doi: 10.1016/0009-3084(83)90015-4. [DOI] [PubMed] [Google Scholar]
- Keller S. L., Gruner S. M., Gawrisch K. Small concentrations of alamethicin induce a cubic phase in bulk phosphatidylethanolamine mixtures. Biochim Biophys Acta. 1996 Jan 31;1278(2):241–246. doi: 10.1016/0005-2736(95)00229-4. [DOI] [PubMed] [Google Scholar]
- Killian J. A., Salemink I., de Planque M. R., Lindblom G., Koeppe R. E., 2nd, Greathouse D. V. Induction of nonbilayer structures in diacylphosphatidylcholine model membranes by transmembrane alpha-helical peptides: importance of hydrophobic mismatch and proposed role of tryptophans. Biochemistry. 1996 Jan 23;35(3):1037–1045. doi: 10.1021/bi9519258. [DOI] [PubMed] [Google Scholar]
- Koynova R., Caffrey M. Phases and phase transitions of the hydrated phosphatidylethanolamines. Chem Phys Lipids. 1994 Jan;69(1):1–34. doi: 10.1016/0009-3084(94)90024-8. [DOI] [PubMed] [Google Scholar]
- Kozlov M. M., Leikin S. L., Chernomordik L. V., Markin V. S., Chizmadzhev Y. A. Stalk mechanism of vesicle fusion. Intermixing of aqueous contents. Eur Biophys J. 1989;17(3):121–129. doi: 10.1007/BF00254765. [DOI] [PubMed] [Google Scholar]
- Kozlov M. M., Leikin S., Rand R. P. Bending, hydration and interstitial energies quantitatively account for the hexagonal-lamellar-hexagonal reentrant phase transition in dioleoylphosphatidylethanolamine. Biophys J. 1994 Oct;67(4):1603–1611. doi: 10.1016/S0006-3495(94)80633-2. [DOI] [PMC free article] [PubMed] [Google Scholar]
- Laggner P., Kriechbaum M. Phospholipid phase transitions: kinetics and structural mechanisms. Chem Phys Lipids. 1991 Mar;57(2-3):121–145. doi: 10.1016/0009-3084(91)90072-j. [DOI] [PubMed] [Google Scholar]
- Lamparski H., Liman U., Barry J. A., Frankel D. A., Ramaswami V., Brown M. F., O'Brien D. F. Photoinduced destabilization of liposomes. Biochemistry. 1992 Jan 28;31(3):685–694. doi: 10.1021/bi00118a008. [DOI] [PubMed] [Google Scholar]
- Leikin S. L., Kozlov M. M., Chernomordik L. V., Markin V. S., Chizmadzhev Y. A. Membrane fusion: overcoming of the hydration barrier and local restructuring. J Theor Biol. 1987 Dec 21;129(4):411–425. doi: 10.1016/s0022-5193(87)80021-8. [DOI] [PubMed] [Google Scholar]
- Markin V. S., Kozlov M. M., Borovjagin V. L. On the theory of membrane fusion. The stalk mechanism. Gen Physiol Biophys. 1984 Oct;3(5):361–377. [PubMed] [Google Scholar]
- Melikyan G. B., White J. M., Cohen F. S. GPI-anchored influenza hemagglutinin induces hemifusion to both red blood cell and planar bilayer membranes. J Cell Biol. 1995 Nov;131(3):679–691. doi: 10.1083/jcb.131.3.679. [DOI] [PMC free article] [PubMed] [Google Scholar]
- Nanavati C., Markin V. S., Oberhauser A. F., Fernandez J. M. The exocytotic fusion pore modeled as a lipidic pore. Biophys J. 1992 Oct;63(4):1118–1132. doi: 10.1016/S0006-3495(92)81679-X. [DOI] [PMC free article] [PubMed] [Google Scholar]
- Nieva J. L., Alonso A., Basáez G., Goñi F. M., Gulik A., Vargas R., Luzzati V. Topological properties of two cubic phases of a phospholipid:cholesterol:diacylglycerol aqueous system and their possible implications in the phospholipase C-induced liposome fusion. FEBS Lett. 1995 Jul 10;368(1):143–147. doi: 10.1016/0014-5793(95)00631-i. [DOI] [PubMed] [Google Scholar]
- Ortiz A., Aranda F. J., Villalaín J., San Martín C., Micol V., Gómez-Fernandez J. C. 1,2-Dioleoylglycerol promotes calcium-induced fusion in phospholipid vesicles. Chem Phys Lipids. 1992 Oct;62(3):215–224. doi: 10.1016/0009-3084(92)90058-w. [DOI] [PubMed] [Google Scholar]
- Prenner E. J., Lewis R. N., Neuman K. C., Gruner S. M., Kondejewski L. H., Hodges R. S., McElhaney R. N. Nonlamellar phases induced by the interaction of gramicidin S with lipid bilayers. A possible relationship to membrane-disrupting activity. Biochemistry. 1997 Jun 24;36(25):7906–7916. doi: 10.1021/bi962785k. [DOI] [PubMed] [Google Scholar]
- Rand R. P., Fuller N., Parsegian V. A., Rau D. C. Variation in hydration forces between neutral phospholipid bilayers: evidence for hydration attraction. Biochemistry. 1988 Oct 4;27(20):7711–7722. doi: 10.1021/bi00420a021. [DOI] [PubMed] [Google Scholar]
- Rand R. P., Reese T. S., Miller R. G. Phospholipid bilayer deformations associated with interbilayer contact and fusion. Nature. 1981 Sep 17;293(5829):237–238. doi: 10.1038/293237a0. [DOI] [PubMed] [Google Scholar]
- Shyamsunder E., Gruner S. M., Tate M. W., Turner D. C., So P. T., Tilcock C. P. Observation of inverted cubic phase in hydrated dioleoylphosphatidylethanolamine membranes. Biochemistry. 1988 Apr 5;27(7):2332–2336. doi: 10.1021/bi00407a014. [DOI] [PubMed] [Google Scholar]
- Siegel D. P., Banschbach J. L. Lamellar/inverted cubic (L alpha/QII) phase transition in N-methylated dioleoylphosphatidylethanolamine. Biochemistry. 1990 Jun 26;29(25):5975–5981. doi: 10.1021/bi00477a014. [DOI] [PubMed] [Google Scholar]
- Siegel D. P., Banschbach J., Alford D., Ellens H., Lis L. J., Quinn P. J., Yeagle P. L., Bentz J. Physiological levels of diacylglycerols in phospholipid membranes induce membrane fusion and stabilize inverted phases. Biochemistry. 1989 May 2;28(9):3703–3709. doi: 10.1021/bi00435a012. [DOI] [PubMed] [Google Scholar]
- Siegel D. P., Burns J. L., Chestnut M. H., Talmon Y. Intermediates in membrane fusion and bilayer/nonbilayer phase transitions imaged by time-resolved cryo-transmission electron microscopy. Biophys J. 1989 Jul;56(1):161–169. doi: 10.1016/S0006-3495(89)82661-X. [DOI] [PMC free article] [PubMed] [Google Scholar]
- Siegel D. P. Energetics of intermediates in membrane fusion: comparison of stalk and inverted micellar intermediate mechanisms. Biophys J. 1993 Nov;65(5):2124–2140. doi: 10.1016/S0006-3495(93)81256-6. [DOI] [PMC free article] [PubMed] [Google Scholar]
- Siegel D. P., Green W. J., Talmon Y. The mechanism of lamellar-to-inverted hexagonal phase transitions: a study using temperature-jump cryo-electron microscopy. Biophys J. 1994 Feb;66(2 Pt 1):402–414. doi: 10.1016/s0006-3495(94)80790-8. [DOI] [PMC free article] [PubMed] [Google Scholar]
- Siegel D. P. Inverted micellar intermediates and the transitions between lamellar, cubic, and inverted hexagonal amphiphile phases. III. Isotropic and inverted cubic state formation via intermediates in transitions between L alpha and HII phases. Chem Phys Lipids. 1986 Dec 31;42(4):279–301. doi: 10.1016/0009-3084(86)90087-3. [DOI] [PubMed] [Google Scholar]
- Siegel D. P. Inverted micellar intermediates and the transitions between lamellar, cubic, and inverted hexagonal lipid phases. I. Mechanism of the L alpha----HII phase transitions. Biophys J. 1986 Jun;49(6):1155–1170. doi: 10.1016/S0006-3495(86)83744-4. [DOI] [PMC free article] [PubMed] [Google Scholar]
- Talmon Y., Burns J. L., Chestnut M. H., Siegel D. P. Time-resolved cryotransmission electron microscopy. J Electron Microsc Tech. 1990 Jan;14(1):6–12. doi: 10.1002/jemt.1060140103. [DOI] [PubMed] [Google Scholar]
- Tate M. W., Gruner S. M. Lipid polymorphism of mixtures of dioleoylphosphatidylethanolamine and saturated and monounsaturated phosphatidylcholines of various chain lengths. Biochemistry. 1987 Jan 13;26(1):231–236. doi: 10.1021/bi00375a031. [DOI] [PubMed] [Google Scholar]
- Tate M. W., Gruner S. M. Temperature dependence of the structural dimensions of the inverted hexagonal (HII) phase of phosphatidylethanolamine-containing membranes. Biochemistry. 1989 May 16;28(10):4245–4253. doi: 10.1021/bi00436a019. [DOI] [PubMed] [Google Scholar]
- Tate M. W., Shyamsunder E., Gruner S. M., D'Amico K. L. Kinetics of the lamellar-inverse hexagonal phase transition determined by time-resolved X-ray diffraction. Biochemistry. 1992 Feb 4;31(4):1081–1092. doi: 10.1021/bi00119a017. [DOI] [PubMed] [Google Scholar]
- Van Venetie R., Verkleij A. J. Analysis of the hexagonal II phase and its relations to lipidic particles and the lamellar phase. A freeze-fracture study. Biochim Biophys Acta. 1981 Jul 20;645(2):262–269. doi: 10.1016/0005-2736(81)90197-8. [DOI] [PubMed] [Google Scholar]
- Verkleij A. J. Lipidic intramembranous particles. Biochim Biophys Acta. 1984 Jan 27;779(1):43–63. doi: 10.1016/0304-4157(84)90003-0. [DOI] [PubMed] [Google Scholar]
- Verkleij A. J., Mombers C., Gerritsen W. J., Leunissen-Bijvelt L., Cullis P. R. Fusion of phospholipid vesicles in association with the appearance of lipidic particles as visualized by freeze fracturing. Biochim Biophys Acta. 1979 Aug 7;555(2):358–361. doi: 10.1016/0005-2736(79)90175-5. [DOI] [PubMed] [Google Scholar]
- Verkleij A. J., Momvers C., Leunissen-Bijvelt J., Ververgaert P. H. Lipidic intramembranous particles. Nature. 1979 May 10;279(5709):162–163. doi: 10.1038/279162a0. [DOI] [PubMed] [Google Scholar]
- Verkleij A. J., van Echteld C. J., Gerritsen W. J., Cullis P. R., de Kruijff B. The lipidic particle as an intermediate structure in membrane fusion processes and bilayer to hexagonal HII transitions. Biochim Biophys Acta. 1980 Aug 14;600(3):620–624. doi: 10.1016/0005-2736(80)90465-4. [DOI] [PubMed] [Google Scholar]
- Vogel S. S., Leikina E. A., Chernomordik L. V. Lysophosphatidylcholine reversibly arrests exocytosis and viral fusion at a stage between triggering and membrane merger. J Biol Chem. 1993 Dec 5;268(34):25764–25768. [PubMed] [Google Scholar]
- Walter A., Gutknecht J. Monocarboxylic acid permeation through lipid bilayer membranes. J Membr Biol. 1984;77(3):255–264. doi: 10.1007/BF01870573. [DOI] [PubMed] [Google Scholar]
- Yeagle P. L., Epand R. M., Richardson C. D., Flanagan T. D. Effects of the 'fusion peptide' from measles virus on the structure of N-methyl dioleoylphosphatidylethanolamine membranes and their fusion with Sendai virus. Biochim Biophys Acta. 1991 May 31;1065(1):49–53. doi: 10.1016/0005-2736(91)90009-w. [DOI] [PubMed] [Google Scholar]
- Yeagle P. L., Smith F. T., Young J. E., Flanagan T. D. Inhibition of membrane fusion by lysophosphatidylcholine. Biochemistry. 1994 Feb 22;33(7):1820–1827. doi: 10.1021/bi00173a027. [DOI] [PubMed] [Google Scholar]
- Zhelev D. V., Needham D. Tension-stabilized pores in giant vesicles: determination of pore size and pore line tension. Biochim Biophys Acta. 1993 Apr 8;1147(1):89–104. doi: 10.1016/0005-2736(93)90319-u. [DOI] [PubMed] [Google Scholar]
- van Gorkom L. C., Nie S. Q., Epand R. M. Hydrophobic lipid additives affect membrane stability and phase behavior of N-monomethyldioleoylphosphatidylethanolamine. Biochemistry. 1992 Jan 28;31(3):671–677. doi: 10.1021/bi00118a006. [DOI] [PubMed] [Google Scholar]