Abstract
We examine how the structure and function of indirect flight muscle (IFM) and the entire flight system of Drosophila melanogaster are affected by phosphorylation of the myosin regulatory light chain (MLC2). This integrated study uses site-directed mutagenesis to examine the relationship between removal of the myosin light chain kinase (MLCK) phosphorylation site, in vivo function of the flight system (flight tests, wing kinematics, metabolism, power output), isolated IFM fiber mechanics, MLC2 isoform pattern, and sarcomeric ultrastructure. The MLC2 mutants exhibit graded impairment of flight ability that correlates with a reduction in both IFM and flight system power output and a reduction in the constitutive level of MLC2 phosphorylation. The MLC2 mutants have wild-type IFM sarcomere and cross-bridge structures, ruling out obvious changes in the ultrastructure as the cause of the reduced performance. We describe a viscoelastic model of cross-bridge dynamics based on sinusoidal length perturbation analysis (Nyquist plots) of skinned IFM fibers. The sinusoidal analysis suggests the high power output of Drosophila IFM required for flight results from a phosphorylation-dependent recruitment of power-generating cross-bridges rather than a change in kinetics of the power generating step. The reduction in cross-bridge number appears to affect the way mutant flies generate flight forces of sufficient magnitude to keep them airborne. In two MLC2 mutant strains that exhibit a reduced IFM power output, flies appear to compensate by lowering wingbeat frequency and by elevating wingstroke amplitude (and presumably muscle strain). This behavioral alteration is not seen in another mutant strain in which the power output and estimated number of recruited cross-bridges is similar to that of wild type.
Full text
PDF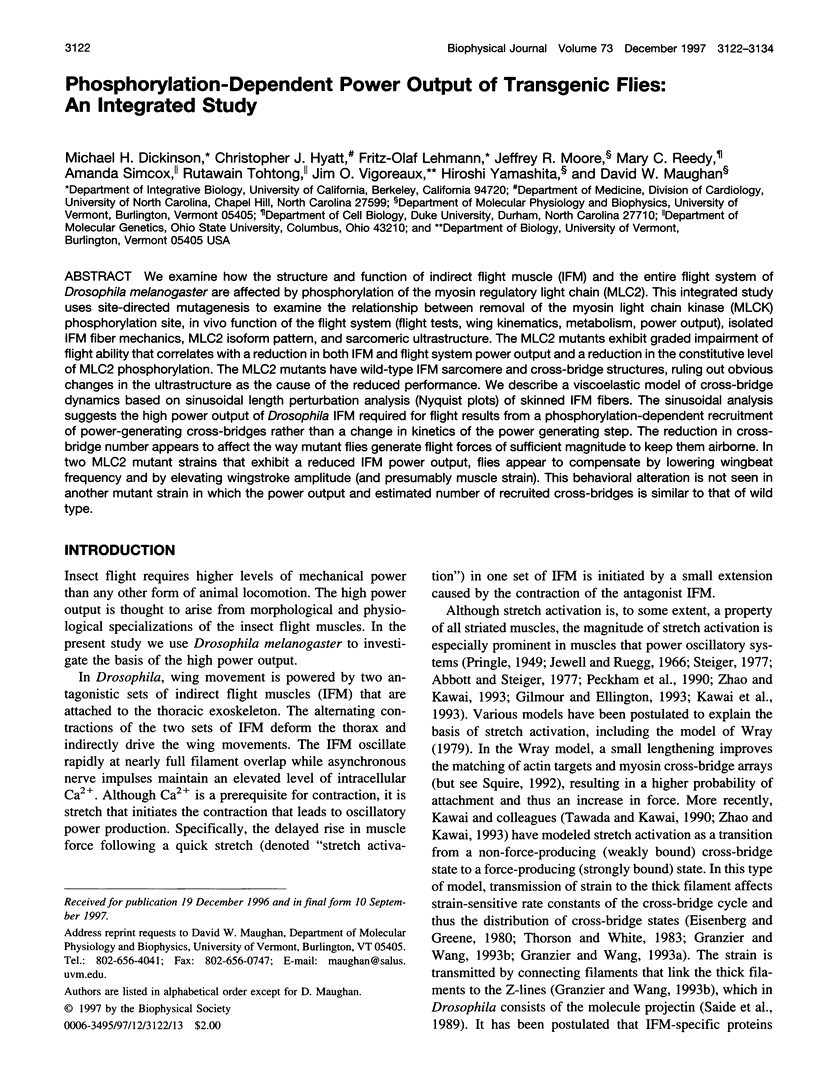
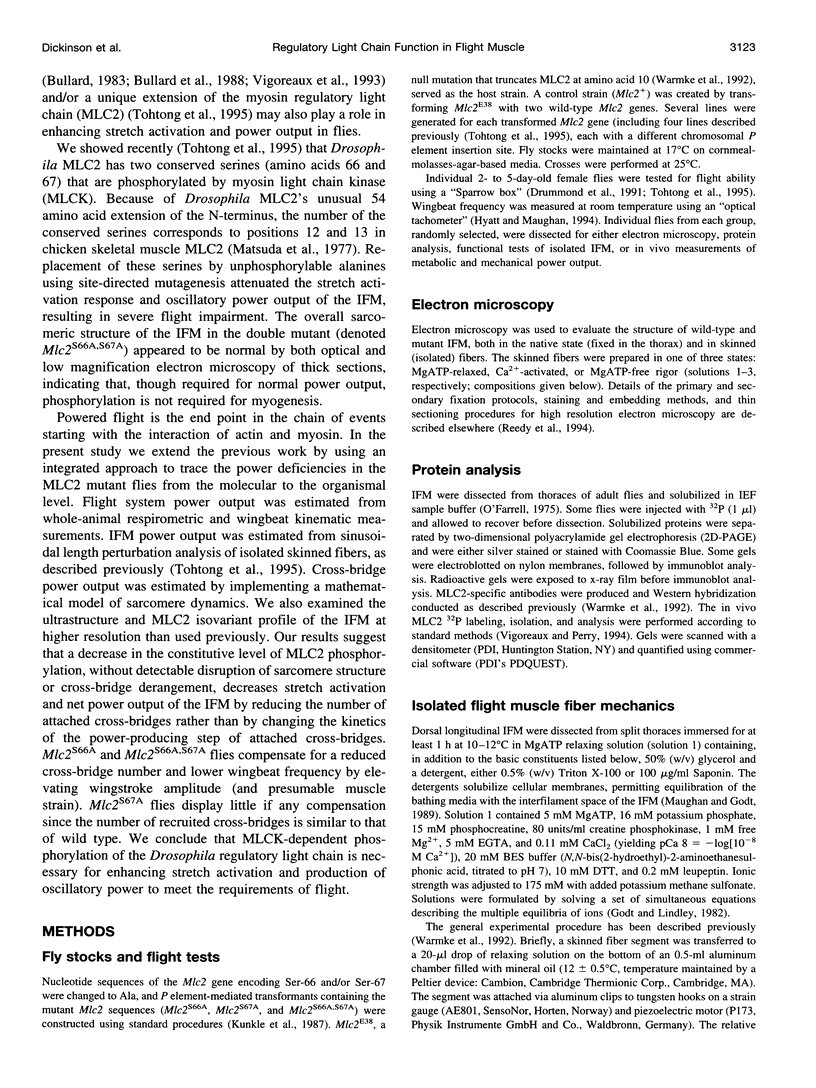
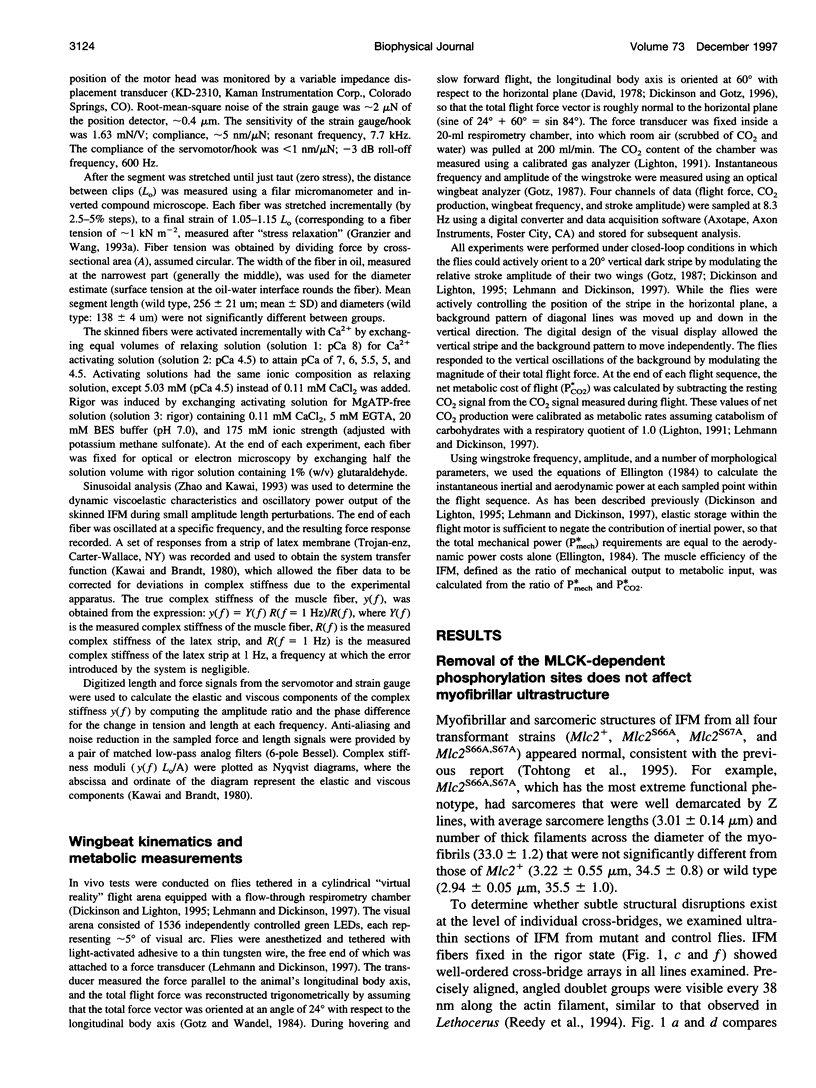
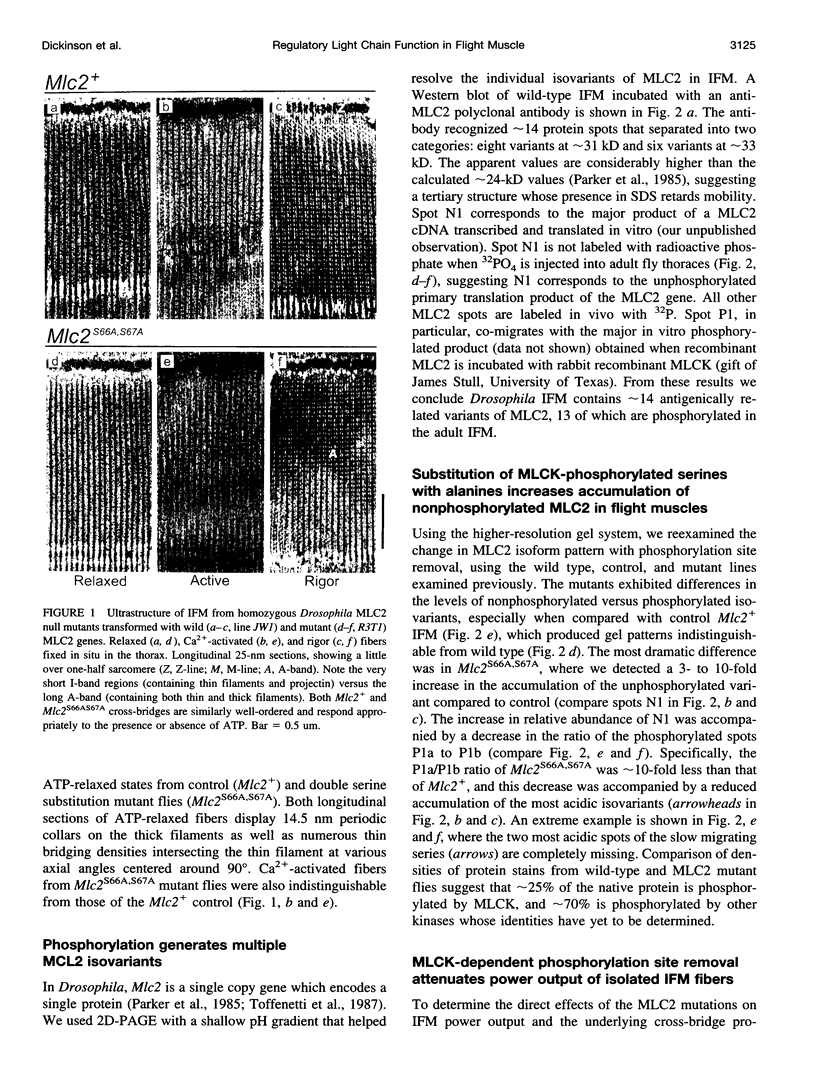
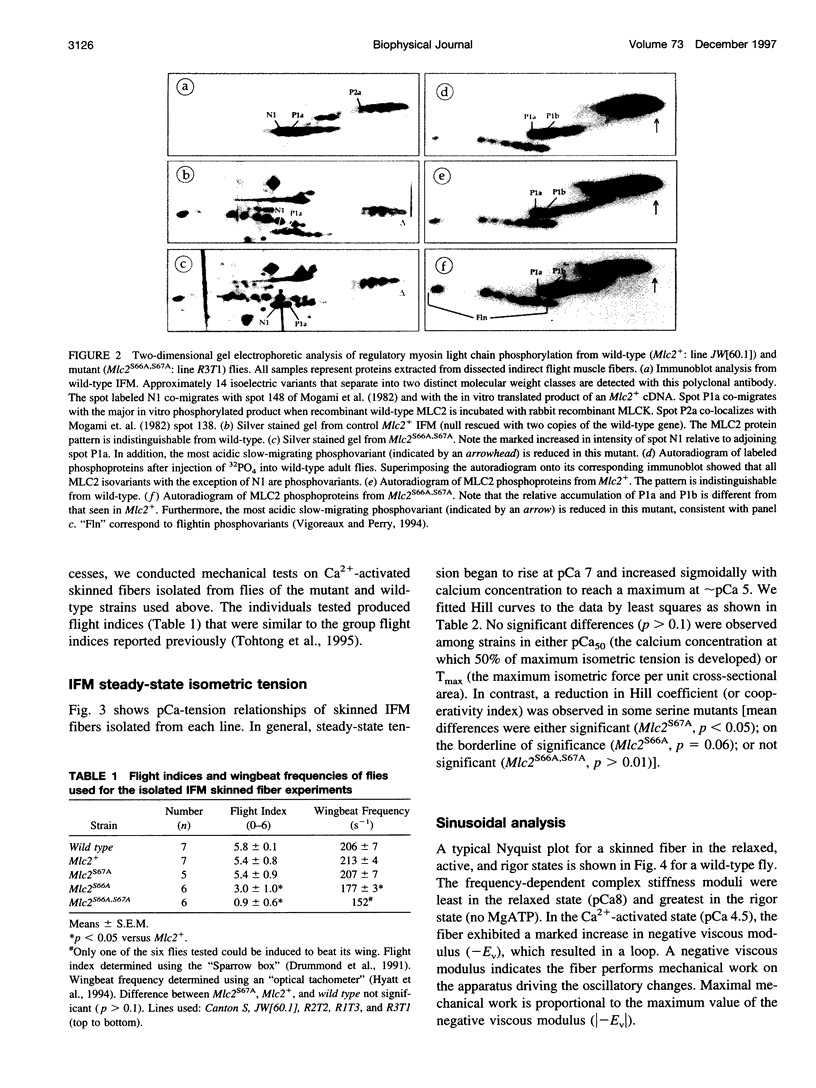
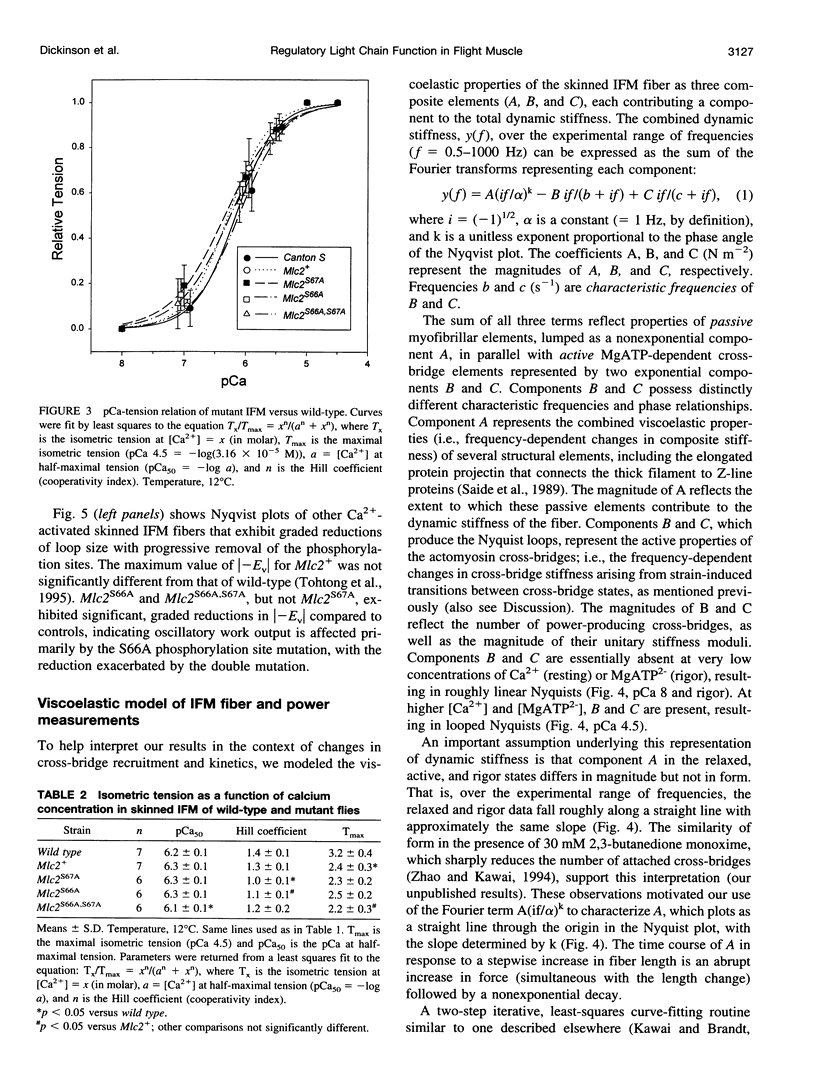
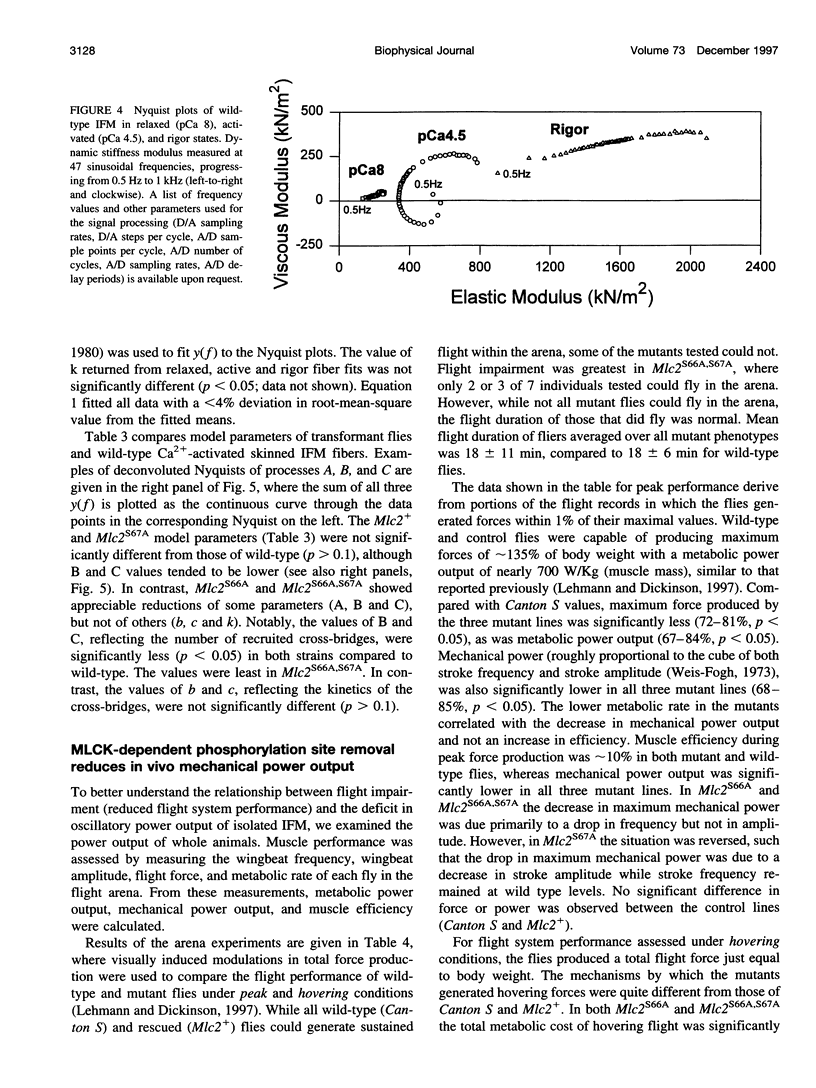
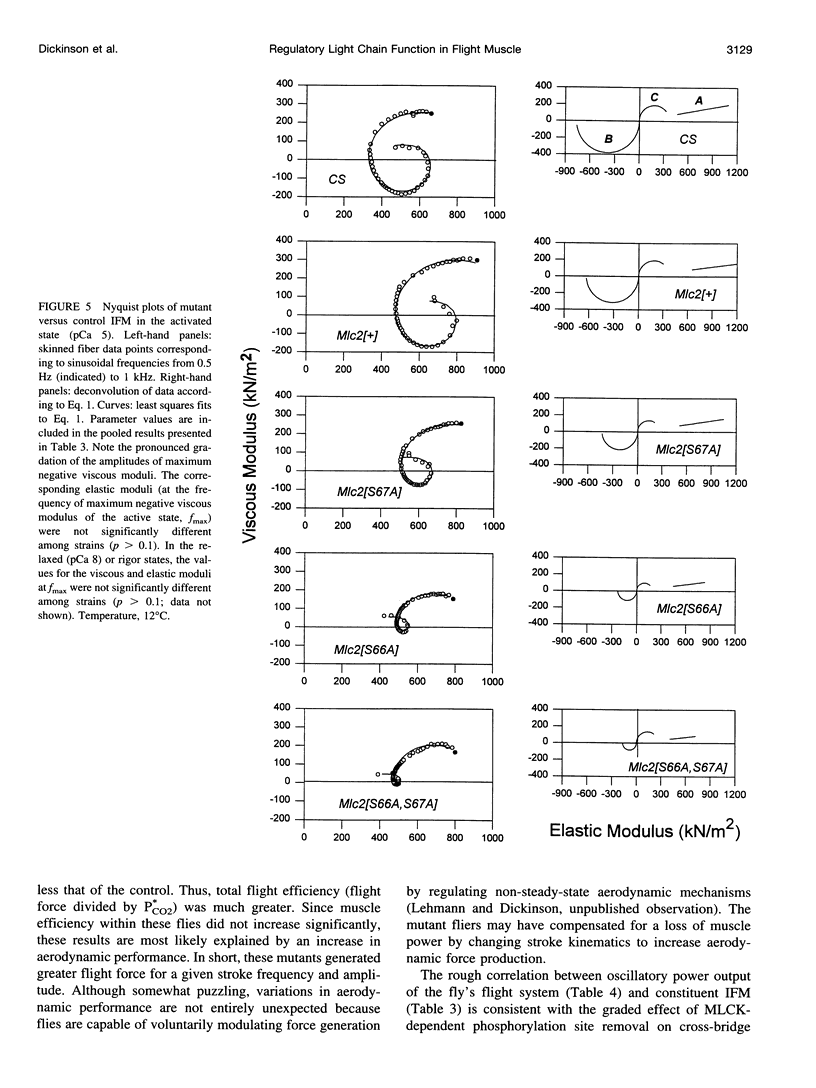
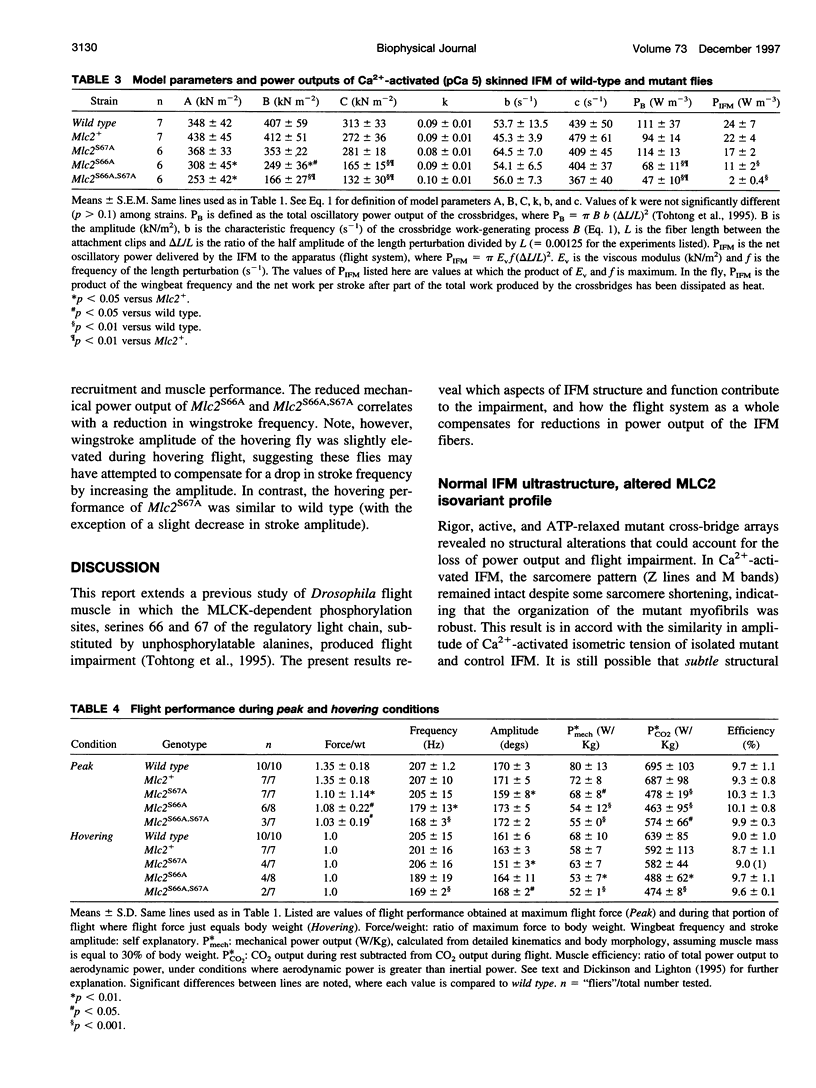
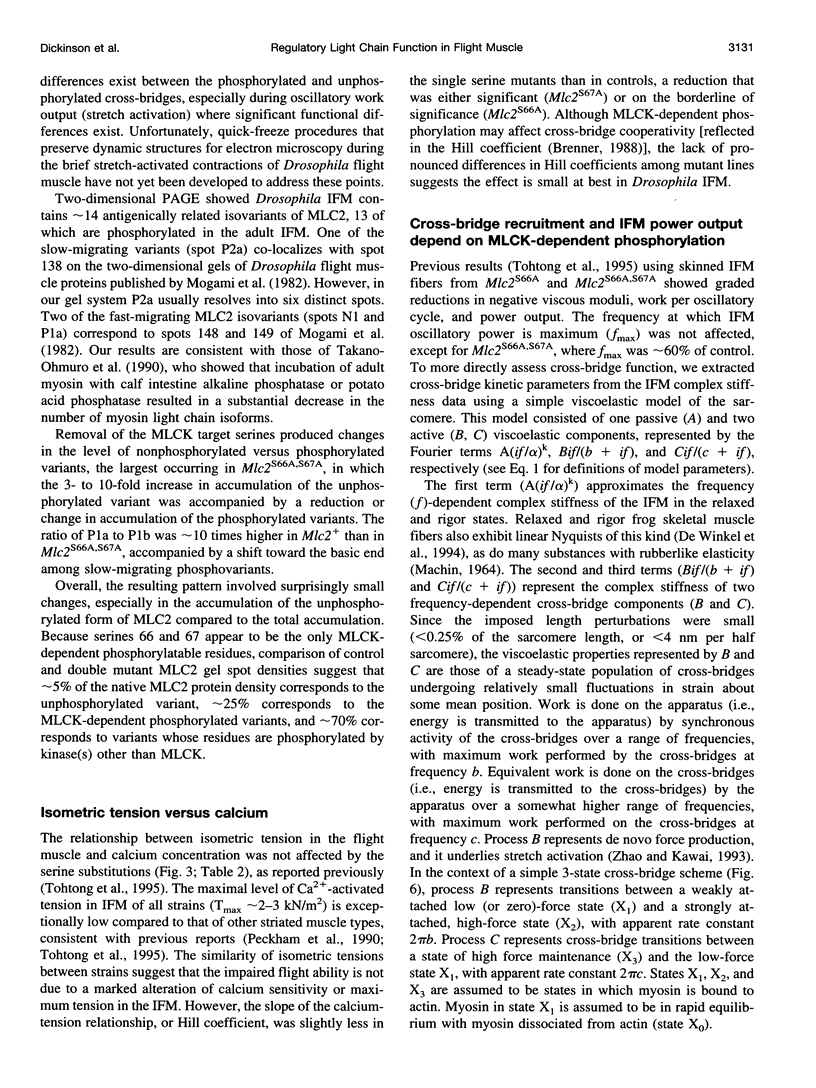
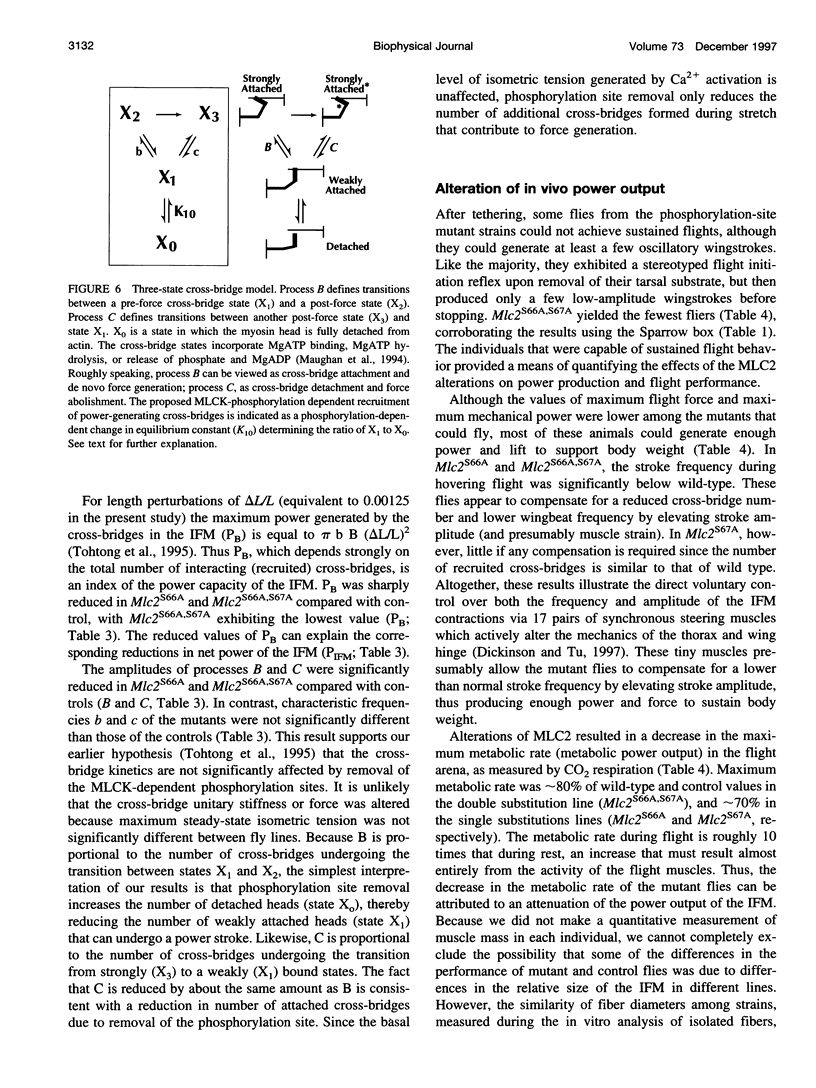
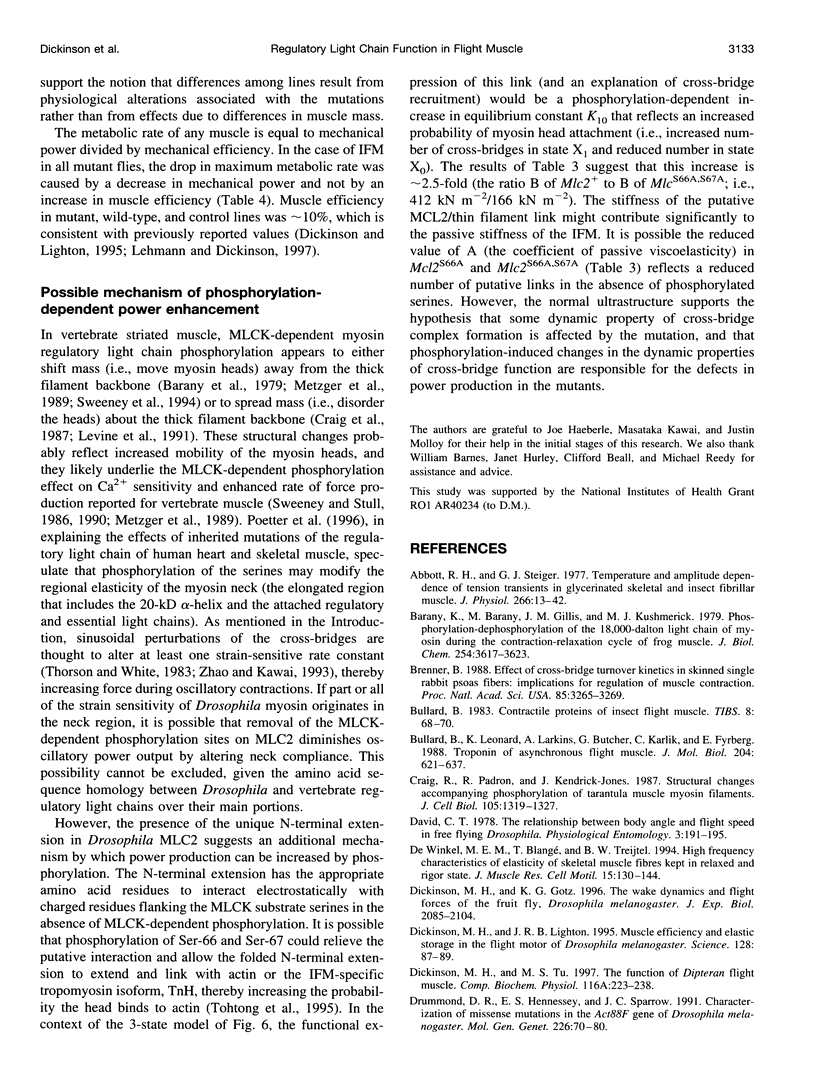
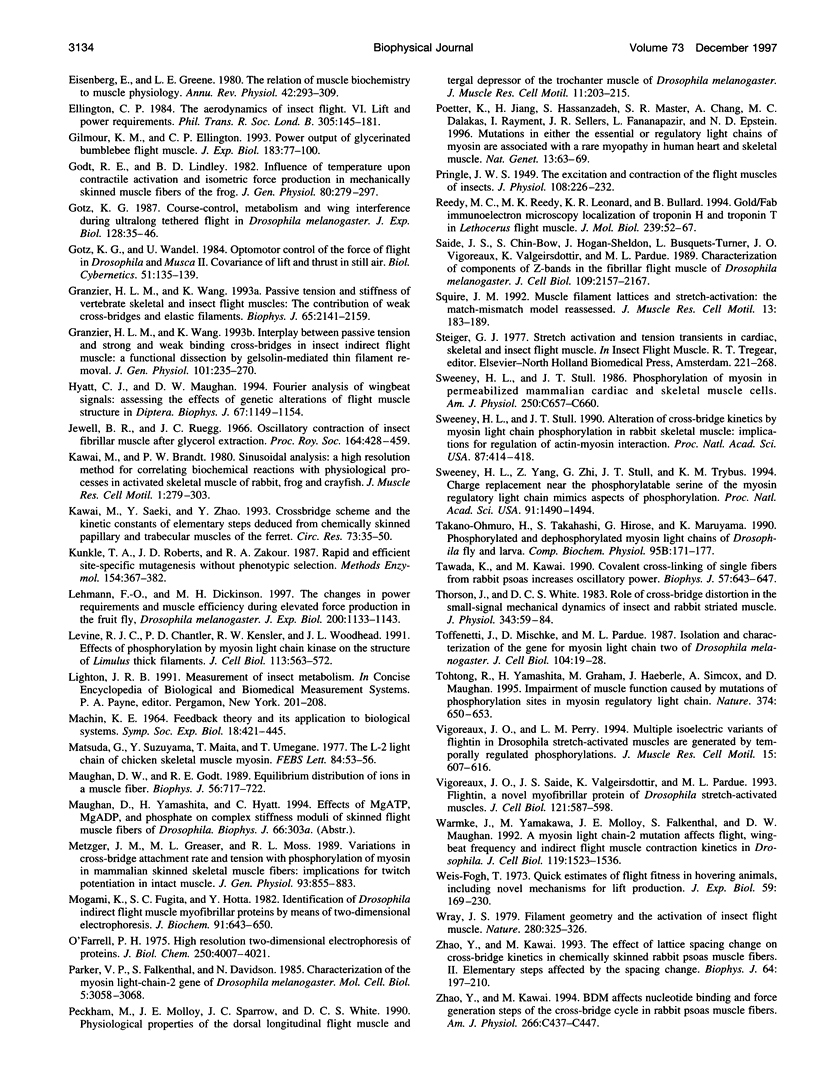
Images in this article
Selected References
These references are in PubMed. This may not be the complete list of references from this article.
- Abbott R. H., Steiger G. J. Temperature and amplitude dependence of tension transients in glycerinated skeletal and insect fibrillar muscle. J Physiol. 1977 Mar;266(1):13–42. doi: 10.1113/jphysiol.1977.sp011754. [DOI] [PMC free article] [PubMed] [Google Scholar]
- Brenner B. Effect of Ca2+ on cross-bridge turnover kinetics in skinned single rabbit psoas fibers: implications for regulation of muscle contraction. Proc Natl Acad Sci U S A. 1988 May;85(9):3265–3269. doi: 10.1073/pnas.85.9.3265. [DOI] [PMC free article] [PubMed] [Google Scholar]
- Bullard B., Leonard K., Larkins A., Butcher G., Karlik C., Fyrberg E. Troponin of asynchronous flight muscle. J Mol Biol. 1988 Dec 5;204(3):621–637. doi: 10.1016/0022-2836(88)90360-9. [DOI] [PubMed] [Google Scholar]
- Bárány K., Bárány M., Gillis J. M., Kushmerick M. J. Phosphorylation-dephosphorylation of the 18,000-dalton light chain of myosin during the contraction-relaxation cycle of frog muscle. J Biol Chem. 1979 May 10;254(9):3617–3623. [PubMed] [Google Scholar]
- Craig R., Padrón R., Kendrick-Jones J. Structural changes accompanying phosphorylation of tarantula muscle myosin filaments. J Cell Biol. 1987 Sep;105(3):1319–1327. doi: 10.1083/jcb.105.3.1319. [DOI] [PMC free article] [PubMed] [Google Scholar]
- De Winkel M. E., Blangé T., Treijtel B. W. High frequency characteristics of elasticity of skeletal muscle fibres kept in relaxed and rigor state. J Muscle Res Cell Motil. 1994 Apr;15(2):130–144. doi: 10.1007/BF00130424. [DOI] [PubMed] [Google Scholar]
- Dickinson M. H., Götz K. G. The wake dynamics and flight forces of the fruit fly Drosophila melanogaster. J Exp Biol. 1996 Sep;199(Pt 9):2085–2104. doi: 10.1242/jeb.199.9.2085. [DOI] [PubMed] [Google Scholar]
- Dickinson M. H., Lighton J. R. Muscle efficiency and elastic storage in the flight motor of Drosophila. Science. 1995 Apr 7;268(5207):87–90. doi: 10.1126/science.7701346. [DOI] [PubMed] [Google Scholar]
- Drummond D. R., Hennessey E. S., Sparrow J. C. Characterisation of missense mutations in the Act88F gene of Drosophila melanogaster. Mol Gen Genet. 1991 Apr;226(1-2):70–80. doi: 10.1007/BF00273589. [DOI] [PubMed] [Google Scholar]
- Eisenberg E., Greene L. E. The relation of muscle biochemistry to muscle physiology. Annu Rev Physiol. 1980;42:293–309. doi: 10.1146/annurev.ph.42.030180.001453. [DOI] [PubMed] [Google Scholar]
- Godt R. E., Lindley B. D. Influence of temperature upon contractile activation and isometric force production in mechanically skinned muscle fibers of the frog. J Gen Physiol. 1982 Aug;80(2):279–297. doi: 10.1085/jgp.80.2.279. [DOI] [PMC free article] [PubMed] [Google Scholar]
- Granzier H. L., Wang K. Interplay between passive tension and strong and weak binding cross-bridges in insect indirect flight muscle. A functional dissection by gelsolin-mediated thin filament removal. J Gen Physiol. 1993 Feb;101(2):235–270. doi: 10.1085/jgp.101.2.235. [DOI] [PMC free article] [PubMed] [Google Scholar]
- Granzier H. L., Wang K. Passive tension and stiffness of vertebrate skeletal and insect flight muscles: the contribution of weak cross-bridges and elastic filaments. Biophys J. 1993 Nov;65(5):2141–2159. doi: 10.1016/S0006-3495(93)81262-1. [DOI] [PMC free article] [PubMed] [Google Scholar]
- Hyatt C. J., Maughan D. W. Fourier analysis of wing beat signals: assessing the effects of genetic alterations of flight muscle structure in Diptera. Biophys J. 1994 Sep;67(3):1149–1154. doi: 10.1016/S0006-3495(94)80582-X. [DOI] [PMC free article] [PubMed] [Google Scholar]
- Kawai M., Brandt P. W. Sinusoidal analysis: a high resolution method for correlating biochemical reactions with physiological processes in activated skeletal muscles of rabbit, frog and crayfish. J Muscle Res Cell Motil. 1980 Sep;1(3):279–303. doi: 10.1007/BF00711932. [DOI] [PubMed] [Google Scholar]
- Kawai M., Saeki Y., Zhao Y. Crossbridge scheme and the kinetic constants of elementary steps deduced from chemically skinned papillary and trabecular muscles of the ferret. Circ Res. 1993 Jul;73(1):35–50. doi: 10.1161/01.res.73.1.35. [DOI] [PubMed] [Google Scholar]
- Kunkel T. A., Roberts J. D., Zakour R. A. Rapid and efficient site-specific mutagenesis without phenotypic selection. Methods Enzymol. 1987;154:367–382. doi: 10.1016/0076-6879(87)54085-x. [DOI] [PubMed] [Google Scholar]
- Lehmann F. O., Dickinson M. H. The changes in power requirements and muscle efficiency during elevated force production in the fruit fly Drosophila melanogaster. J Exp Biol. 1997 Apr;200(Pt 7):1133–1143. doi: 10.1242/jeb.200.7.1133. [DOI] [PubMed] [Google Scholar]
- Levine R. J., Chantler P. D., Kensler R. W., Woodhead J. L. Effects of phosphorylation by myosin light chain kinase on the structure of Limulus thick filaments. J Cell Biol. 1991 May;113(3):563–572. doi: 10.1083/jcb.113.3.563. [DOI] [PMC free article] [PubMed] [Google Scholar]
- Machin K. E. Feedback theory and its application to biological systems. Symp Soc Exp Biol. 1964;18:421–445. [PubMed] [Google Scholar]
- Matsuda G., Suzuyama Y., Maita T., Umegane T. The L-2 light chain of chicken skeletal muscle myosin. FEBS Lett. 1977 Dec 1;84(1):53–56. doi: 10.1016/0014-5793(77)81055-7. [DOI] [PubMed] [Google Scholar]
- Maughan D. W., Godt R. E. Equilibrium distribution of ions in a muscle fiber. Biophys J. 1989 Oct;56(4):717–722. doi: 10.1016/S0006-3495(89)82719-5. [DOI] [PMC free article] [PubMed] [Google Scholar]
- Metzger J. M., Greaser M. L., Moss R. L. Variations in cross-bridge attachment rate and tension with phosphorylation of myosin in mammalian skinned skeletal muscle fibers. Implications for twitch potentiation in intact muscle. J Gen Physiol. 1989 May;93(5):855–883. doi: 10.1085/jgp.93.5.855. [DOI] [PMC free article] [PubMed] [Google Scholar]
- Mogami K., Fujita S. C., Hotta Y. Identification of Drosophila indirect flight muscle myofibrillar proteins by means of two-dimensional electrophoresis. J Biochem. 1982 Feb;91(2):643–650. doi: 10.1093/oxfordjournals.jbchem.a133736. [DOI] [PubMed] [Google Scholar]
- O'Farrell P. H. High resolution two-dimensional electrophoresis of proteins. J Biol Chem. 1975 May 25;250(10):4007–4021. [PMC free article] [PubMed] [Google Scholar]
- Parker V. P., Falkenthal S., Davidson N. Characterization of the myosin light-chain-2 gene of Drosophila melanogaster. Mol Cell Biol. 1985 Nov;5(11):3058–3068. doi: 10.1128/mcb.5.11.3058. [DOI] [PMC free article] [PubMed] [Google Scholar]
- Peckham M., Molloy J. E., Sparrow J. C., White D. C. Physiological properties of the dorsal longitudinal flight muscle and the tergal depressor of the trochanter muscle of Drosophila melanogaster. J Muscle Res Cell Motil. 1990 Jun;11(3):203–215. doi: 10.1007/BF01843574. [DOI] [PubMed] [Google Scholar]
- Poetter K., Jiang H., Hassanzadeh S., Master S. R., Chang A., Dalakas M. C., Rayment I., Sellers J. R., Fananapazir L., Epstein N. D. Mutations in either the essential or regulatory light chains of myosin are associated with a rare myopathy in human heart and skeletal muscle. Nat Genet. 1996 May;13(1):63–69. doi: 10.1038/ng0596-63. [DOI] [PubMed] [Google Scholar]
- Reedy M. C., Reedy M. K., Leonard K. R., Bullard B. Gold/Fab immuno electron microscopy localization of troponin H and troponin T in Lethocerus flight muscle. J Mol Biol. 1994 May 27;239(1):52–67. doi: 10.1006/jmbi.1994.1350. [DOI] [PubMed] [Google Scholar]
- Saide J. D., Chin-Bow S., Hogan-Sheldon J., Busquets-Turner L., Vigoreaux J. O., Valgeirsdottir K., Pardue M. L. Characterization of components of Z-bands in the fibrillar flight muscle of Drosophila melanogaster. J Cell Biol. 1989 Nov;109(5):2157–2167. doi: 10.1083/jcb.109.5.2157. [DOI] [PMC free article] [PubMed] [Google Scholar]
- Squire J. M. Muscle filament lattices and stretch-activation: the match-mismatch model reassessed. J Muscle Res Cell Motil. 1992 Apr;13(2):183–189. doi: 10.1007/BF01874155. [DOI] [PubMed] [Google Scholar]
- Sweeney H. L., Stull J. T. Alteration of cross-bridge kinetics by myosin light chain phosphorylation in rabbit skeletal muscle: implications for regulation of actin-myosin interaction. Proc Natl Acad Sci U S A. 1990 Jan;87(1):414–418. doi: 10.1073/pnas.87.1.414. [DOI] [PMC free article] [PubMed] [Google Scholar]
- Sweeney H. L., Stull J. T. Phosphorylation of myosin in permeabilized mammalian cardiac and skeletal muscle cells. Am J Physiol. 1986 Apr;250(4 Pt 1):C657–C660. doi: 10.1152/ajpcell.1986.250.4.C657. [DOI] [PubMed] [Google Scholar]
- Sweeney H. L., Yang Z., Zhi G., Stull J. T., Trybus K. M. Charge replacement near the phosphorylatable serine of the myosin regulatory light chain mimics aspects of phosphorylation. Proc Natl Acad Sci U S A. 1994 Feb 15;91(4):1490–1494. doi: 10.1073/pnas.91.4.1490. [DOI] [PMC free article] [PubMed] [Google Scholar]
- Takano-Ohmuro H., Takahashi S., Hirose G., Maruyama K. Phosphorylated and dephosphorylated myosin light chains of Drosophila fly and larva. Comp Biochem Physiol B. 1990;95(1):171–177. doi: 10.1016/0305-0491(90)90266-v. [DOI] [PubMed] [Google Scholar]
- Tawada K., Kawai M. Covalent cross-linking of single fibers from rabbit psoas increases oscillatory power. Biophys J. 1990 Mar;57(3):643–647. doi: 10.1016/S0006-3495(90)82582-0. [DOI] [PMC free article] [PubMed] [Google Scholar]
- Thorson J., White D. C. Role of cross-bridge distortion in the small-signal mechanical dynamics of insect and rabbit striated muscle. J Physiol. 1983 Oct;343:59–84. doi: 10.1113/jphysiol.1983.sp014881. [DOI] [PMC free article] [PubMed] [Google Scholar]
- Toffenetti J., Mischke D., Pardue M. L. Isolation and characterization of the gene for myosin light chain two of Drosophila melanogaster. J Cell Biol. 1987 Jan;104(1):19–28. doi: 10.1083/jcb.104.1.19. [DOI] [PMC free article] [PubMed] [Google Scholar]
- Tohtong R., Yamashita H., Graham M., Haeberle J., Simcox A., Maughan D. Impairment of muscle function caused by mutations of phosphorylation sites in myosin regulatory light chain. Nature. 1995 Apr 13;374(6523):650–653. doi: 10.1038/374650a0. [DOI] [PubMed] [Google Scholar]
- Vigoreaux J. O., Perry L. M. Multiple isoelectric variants of flightin in Drosophila stretch-activated muscles are generated by temporally regulated phosphorylations. J Muscle Res Cell Motil. 1994 Dec;15(6):607–616. doi: 10.1007/BF00121068. [DOI] [PubMed] [Google Scholar]
- Vigoreaux J. O., Saide J. D., Valgeirsdottir K., Pardue M. L. Flightin, a novel myofibrillar protein of Drosophila stretch-activated muscles. J Cell Biol. 1993 May;121(3):587–598. doi: 10.1083/jcb.121.3.587. [DOI] [PMC free article] [PubMed] [Google Scholar]
- Warmke J., Yamakawa M., Molloy J., Falkenthal S., Maughan D. Myosin light chain-2 mutation affects flight, wing beat frequency, and indirect flight muscle contraction kinetics in Drosophila. J Cell Biol. 1992 Dec;119(6):1523–1539. doi: 10.1083/jcb.119.6.1523. [DOI] [PMC free article] [PubMed] [Google Scholar]
- Zhao Y., Kawai M. BDM affects nucleotide binding and force generation steps of the cross-bridge cycle in rabbit psoas muscle fibers. Am J Physiol. 1994 Feb;266(2 Pt 1):C437–C447. doi: 10.1152/ajpcell.1994.266.2.C437. [DOI] [PubMed] [Google Scholar]
- Zhao Y., Kawai M. The effect of the lattice spacing change on cross-bridge kinetics in chemically skinned rabbit psoas muscle fibers. II. Elementary steps affected by the spacing change. Biophys J. 1993 Jan;64(1):197–210. doi: 10.1016/S0006-3495(93)81357-2. [DOI] [PMC free article] [PubMed] [Google Scholar]