Abstract
Thin strips of guinea pig tenia cecum were loaded with the Mg2+ indicator furaptra, and the indicator fluorescence signals measured in Ca2+-free condition were converted to cytoplasmic-free Mg2+ concentration ([Mg2+]i). Lowering the extracellular Na+ concentration ([Na+]o) caused a reversible increase in [Mg2+]i, consistent with the inhibition of Na+ gradient-dependent extrusion of cellular Mg2+ (Na+-Mg2+ exchange). Curve-fitting analysis indicated that the relation between [Na+]o and the rate of rise in [Mg2+], had a Hill coefficient of approximately 3, a [Na+]o at the half-maximal rate of rise of approximately 30 mM, and a maximal rate of 0.16 +/- 0.01 microM/s (mean +/- SE, n = 6). Depolarization with 56 mM K+ shifted the curve slightly toward higher [Na+]o without significantly changing the maximal rate, suggesting that the Na+-Mg2+ exchange was inhibited by depolarization. The maximal rate would correspond to a flux of 0.15-0.4 pmol/cm2/s, if cytoplasmic Mg2+ buffering power (defined as the ratio of the changes in total Mg2+ and free Mg2+ concentrations) is assumed to be 2-5. Ouabain (1-5 microM) increased the intracellular Na+ concentration, as assessed with fluorescence of SBFI (sodium-binding benzofuran isophthalate, a Na+ indicator), and elevated [Mg2+]i. In ouabain-treated preparations, removal of extracellular Na+ rapidly increased [Mg2+]i, with an initial rate of rise roughly proportional to the degree of the Mg2+ load, and, probably, to the Na+ load caused by ouabain. The enhanced rate of rise in [Mg2+]i (up to approximately 1 microM/s) could be attributed to the Mg2+ influx as a result of the reversed Na+-Mg2+ exchange. Our results support the presence of a reversible and possibly electrogenic Na+-Mg2+ exchange in the smooth muscle cells of tenia cecum.
Full text
PDF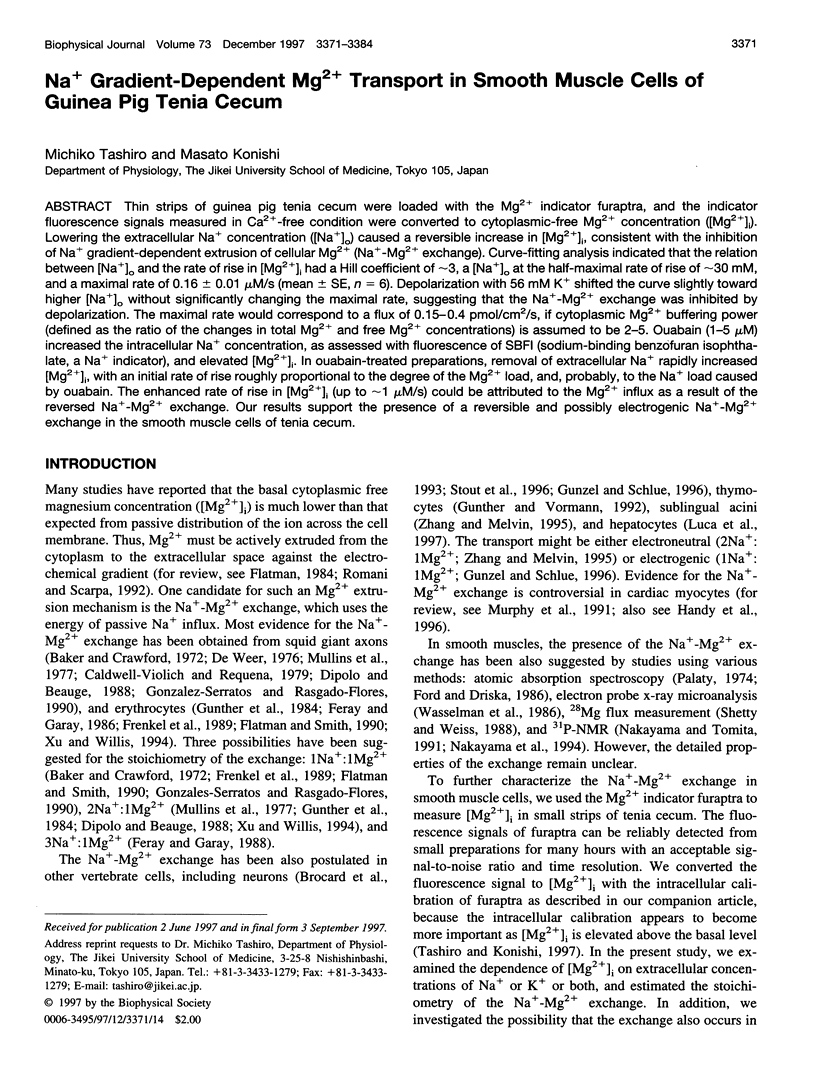
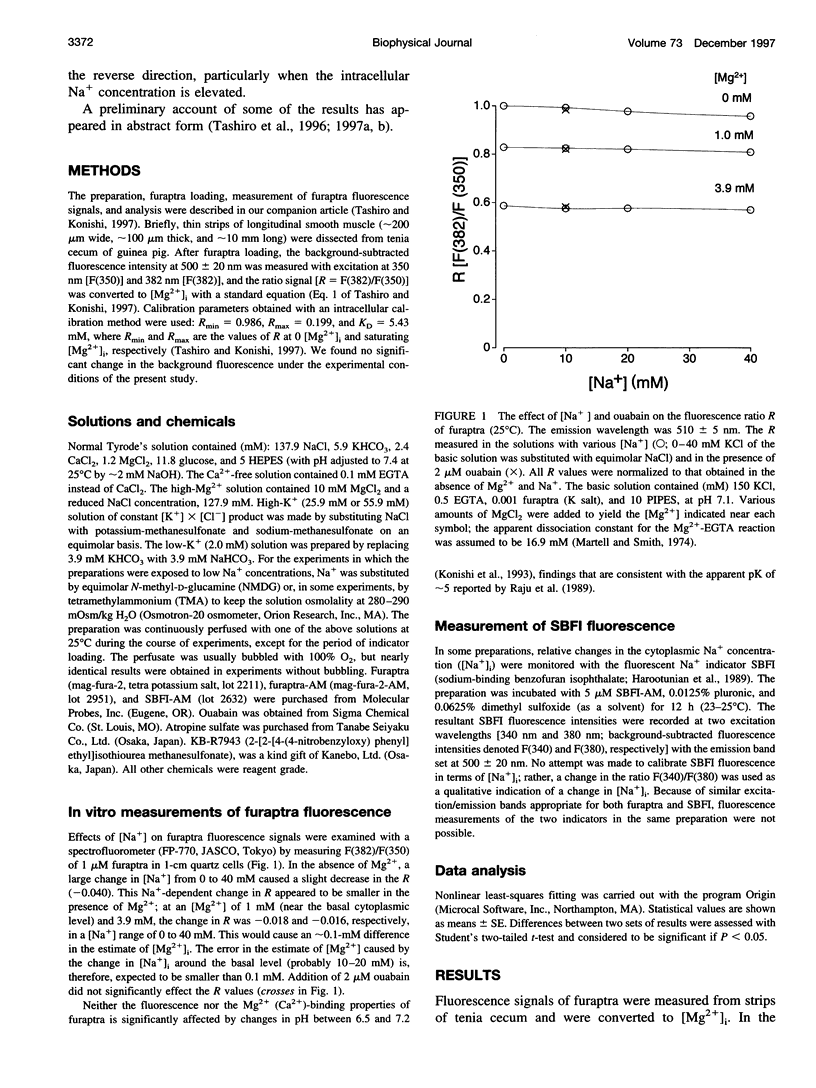
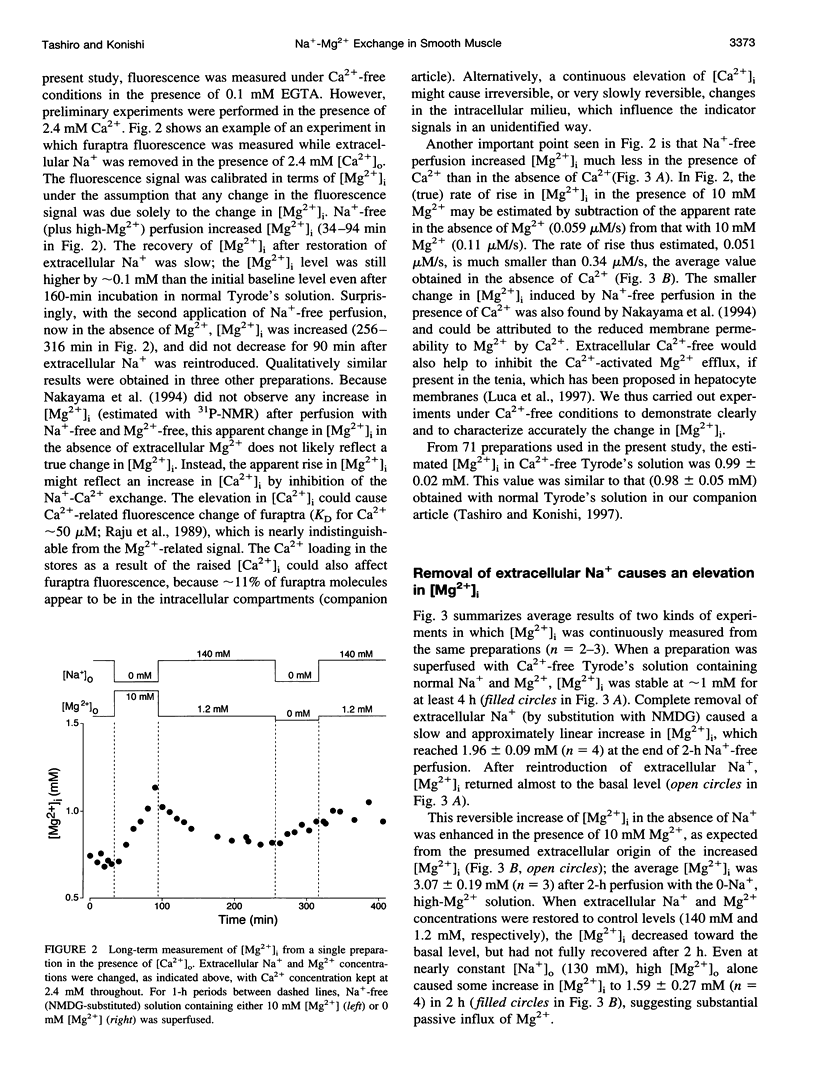
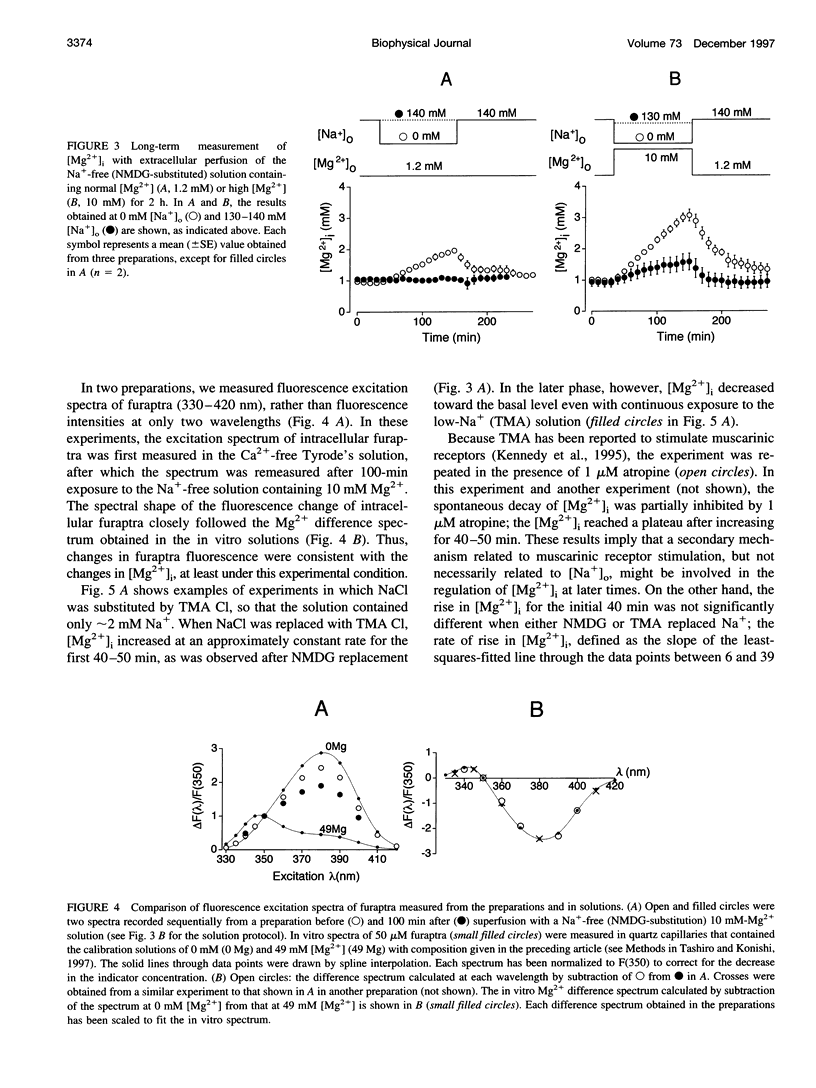
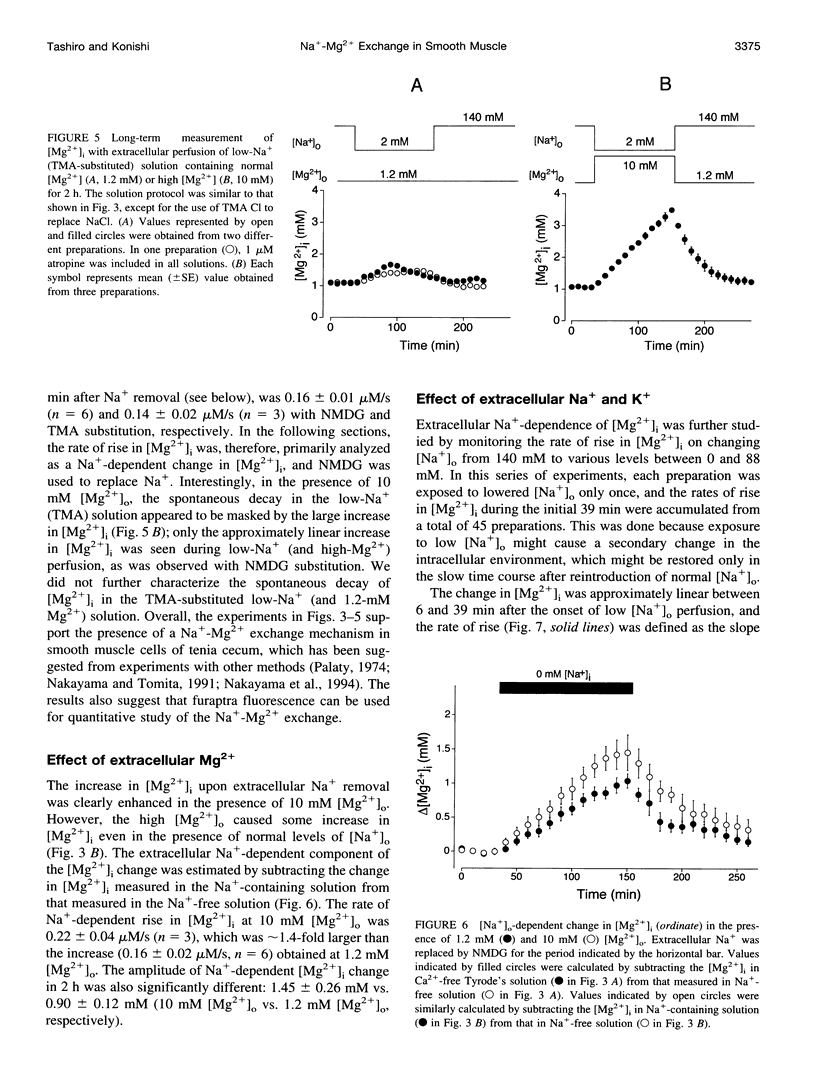
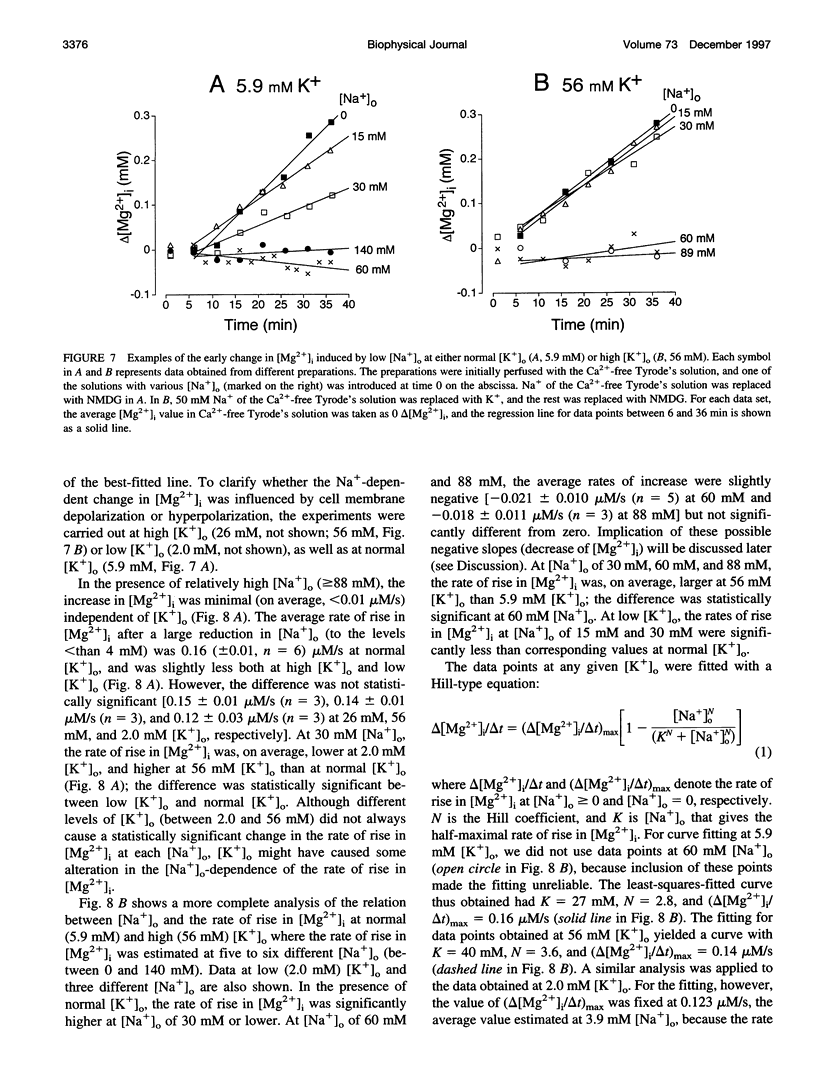
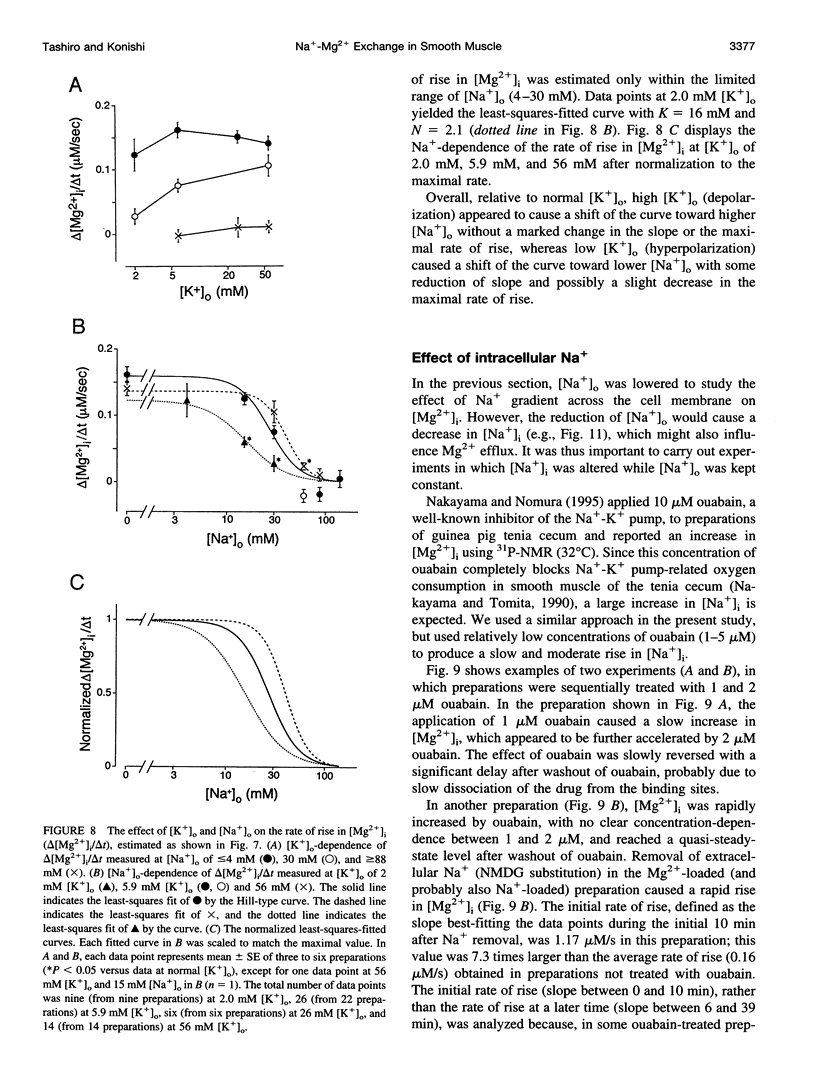
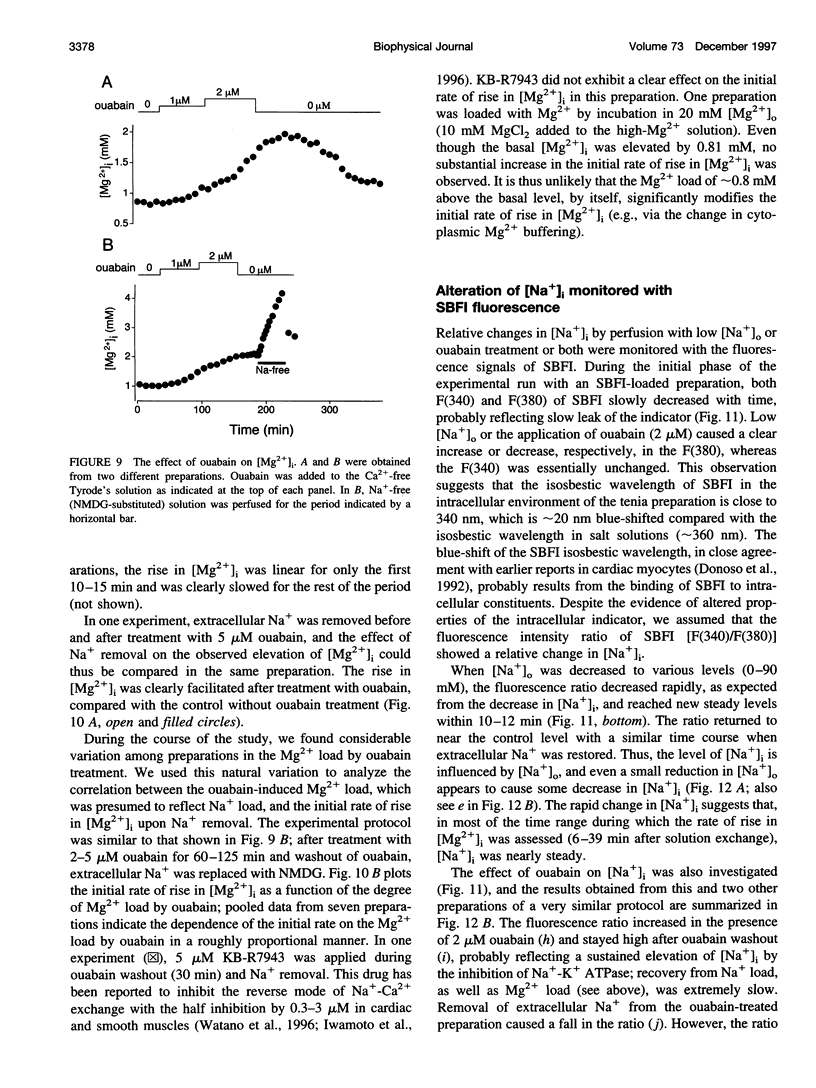
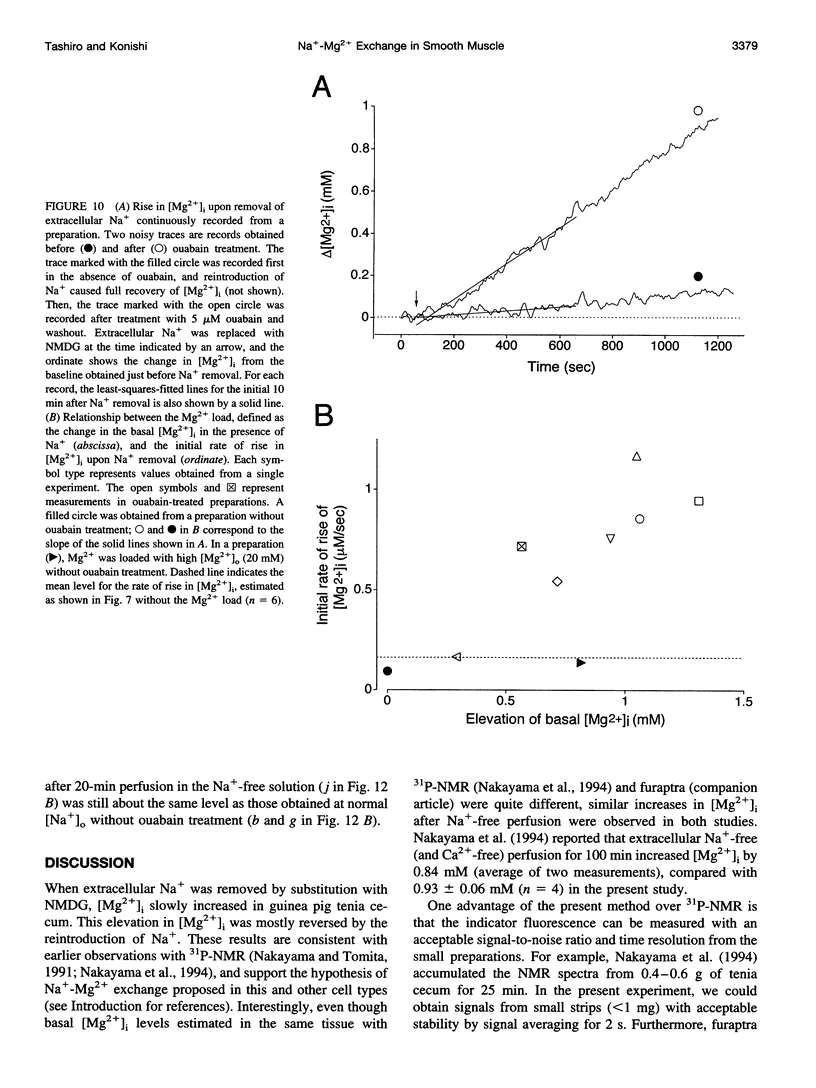
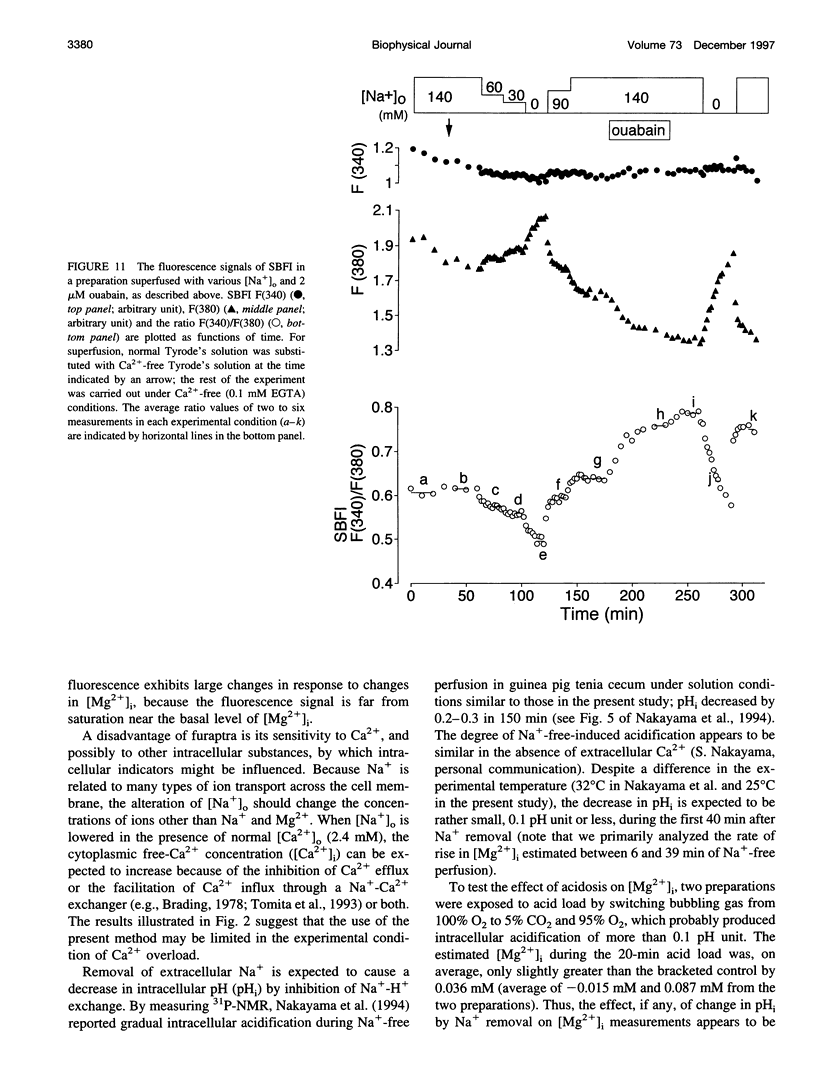
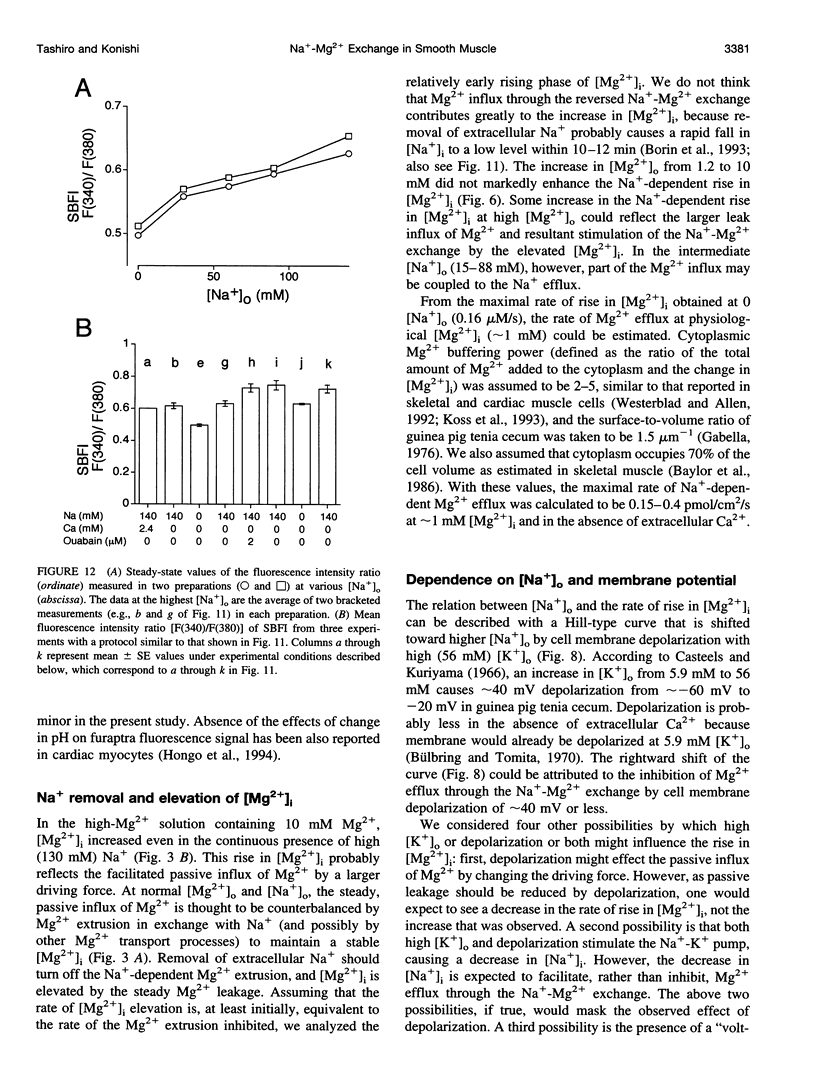
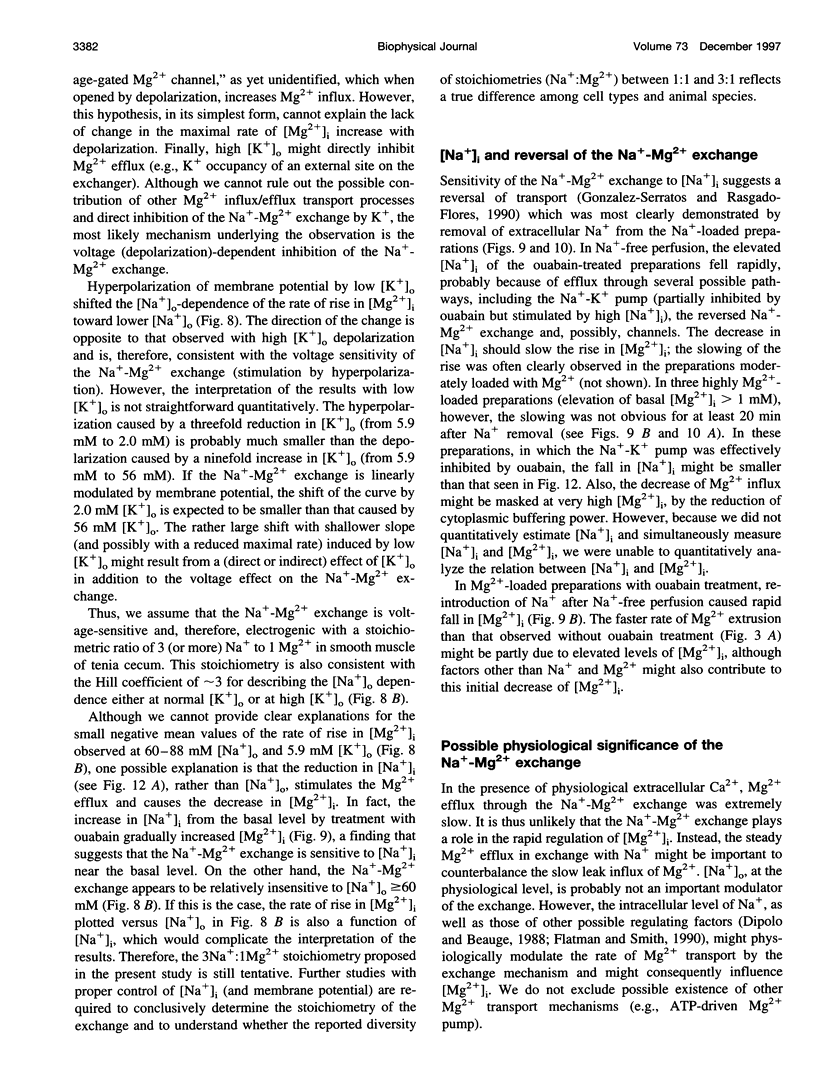
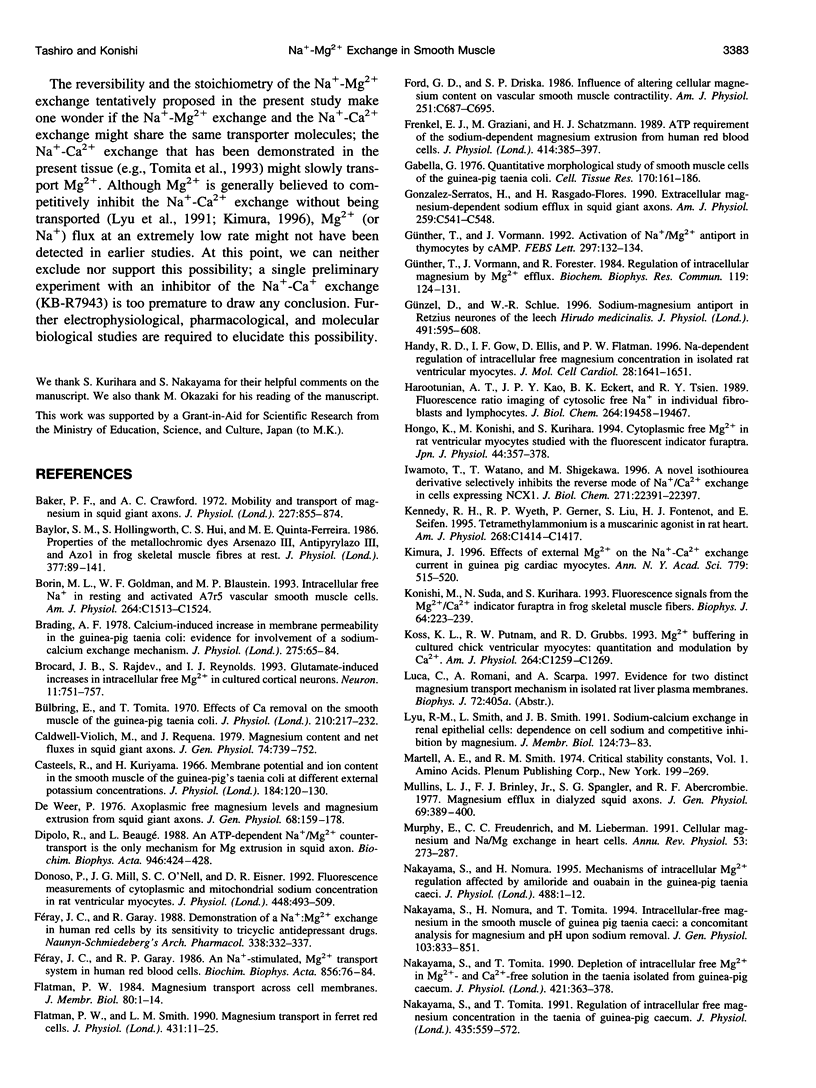
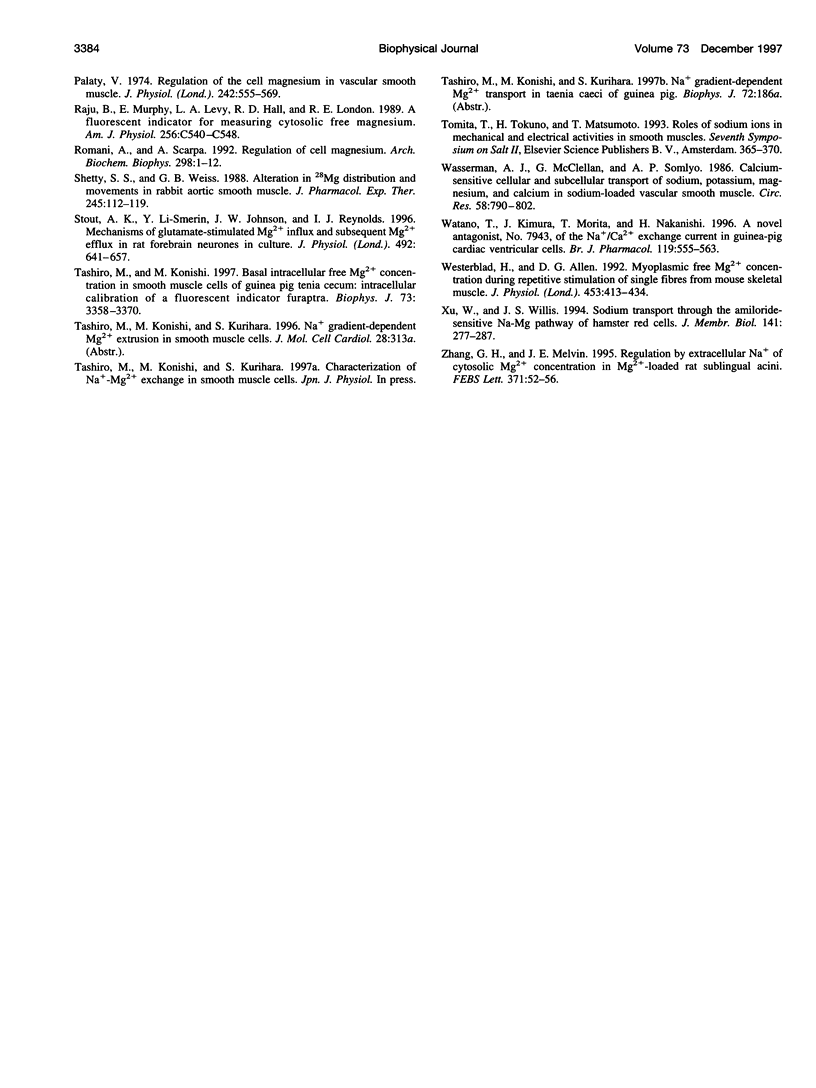
Selected References
These references are in PubMed. This may not be the complete list of references from this article.
- Baker P. F., Crawford A. C. Mobility and transport of magnesium in squid giant axons. J Physiol. 1972 Dec;227(3):855–874. doi: 10.1113/jphysiol.1972.sp010062. [DOI] [PMC free article] [PubMed] [Google Scholar]
- Baylor S. M., Hollingworth S., Hui C. S., Quinta-Ferreira M. E. Properties of the metallochromic dyes Arsenazo III, Antipyrylazo III and Azo1 in frog skeletal muscle fibres at rest. J Physiol. 1986 Aug;377:89–141. doi: 10.1113/jphysiol.1986.sp016178. [DOI] [PMC free article] [PubMed] [Google Scholar]
- Borin M. L., Goldman W. F., Blaustein M. P. Intracellular free Na+ in resting and activated A7r5 vascular smooth muscle cells. Am J Physiol. 1993 Jun;264(6 Pt 1):C1513–C1524. doi: 10.1152/ajpcell.1993.264.6.C1513. [DOI] [PubMed] [Google Scholar]
- Brading A. F. Calcium-induced increase in membrane permeability in the guinea-pig taenia coli: evidence for involvement of a sodium-calcium exchange mechanism. J Physiol. 1978 Feb;275:65–84. doi: 10.1113/jphysiol.1978.sp012178. [DOI] [PMC free article] [PubMed] [Google Scholar]
- Brocard J. B., Rajdev S., Reynolds I. J. Glutamate-induced increases in intracellular free Mg2+ in cultured cortical neurons. Neuron. 1993 Oct;11(4):751–757. doi: 10.1016/0896-6273(93)90084-5. [DOI] [PubMed] [Google Scholar]
- Bülbring E., Tomita T. Effects of Ca removal on the smooth muscle of the guinea-pig taenia coli. J Physiol. 1970 Sep;210(1):217–232. doi: 10.1113/jphysiol.1970.sp009205. [DOI] [PMC free article] [PubMed] [Google Scholar]
- Caldwell-Violich M., Requena J. Magnesium content and net fluxes in squid giant axons. J Gen Physiol. 1979 Dec;74(6):739–752. doi: 10.1085/jgp.74.6.739. [DOI] [PMC free article] [PubMed] [Google Scholar]
- Casteels R., Kuriyama H. Membrane potential and ion content in the smooth muscle of the guinea-pig's taenia coli at different external potassium concentrations. J Physiol. 1966 May;184(1):120–130. doi: 10.1113/jphysiol.1966.sp007906. [DOI] [PMC free article] [PubMed] [Google Scholar]
- De Weer P. Axoplasmic free magnesium levels and magnesium extrusion from squid giant axons. J Gen Physiol. 1976 Aug;68(2):159–178. doi: 10.1085/jgp.68.2.159. [DOI] [PMC free article] [PubMed] [Google Scholar]
- DiPolo R., Beaugé L. An ATP-dependent Na+/Mg2+ countertransport is the only mechanism for Mg extrusion in squid axons. Biochim Biophys Acta. 1988 Dec 22;946(2):424–428. doi: 10.1016/0005-2736(88)90418-x. [DOI] [PubMed] [Google Scholar]
- Donoso P., Mill J. G., O'Neill S. C., Eisner D. A. Fluorescence measurements of cytoplasmic and mitochondrial sodium concentration in rat ventricular myocytes. J Physiol. 1992 Mar;448:493–509. doi: 10.1113/jphysiol.1992.sp019053. [DOI] [PMC free article] [PubMed] [Google Scholar]
- Flatman P. W. Magnesium transport across cell membranes. J Membr Biol. 1984;80(1):1–14. doi: 10.1007/BF01868686. [DOI] [PubMed] [Google Scholar]
- Flatman P. W., Smith L. M. Magnesium transport in ferret red cells. J Physiol. 1990 Dec;431:11–25. doi: 10.1113/jphysiol.1990.sp018318. [DOI] [PMC free article] [PubMed] [Google Scholar]
- Ford G. D., Driska S. P. Influence of altering cellular magnesium content on vascular smooth muscle contractility. Am J Physiol. 1986 Nov;251(5 Pt 1):C687–C695. doi: 10.1152/ajpcell.1986.251.5.C687. [DOI] [PubMed] [Google Scholar]
- Frenkel E. J., Graziani M., Schatzmann H. J. ATP requirement of the sodium-dependent magnesium extrusion from human red blood cells. J Physiol. 1989 Jul;414:385–397. doi: 10.1113/jphysiol.1989.sp017694. [DOI] [PMC free article] [PubMed] [Google Scholar]
- Féray J. C., Garay R. An Na+-stimulated Mg2+-transport system in human red blood cells. Biochim Biophys Acta. 1986 Mar 27;856(1):76–84. doi: 10.1016/0005-2736(86)90012-x. [DOI] [PubMed] [Google Scholar]
- Féray J. C., Garay R. Demonstration of a Na+: Mg2+ exchange in human red cells by its sensitivity to tricyclic antidepressant drugs. Naunyn Schmiedebergs Arch Pharmacol. 1988 Sep;338(3):332–337. doi: 10.1007/BF00173409. [DOI] [PubMed] [Google Scholar]
- Gabella G. Quantitative morphological study of smooth muscle cells of the guinea-pig taenia coli. Cell Tissue Res. 1976 Jul 26;170(2):161–186. doi: 10.1007/BF00224297. [DOI] [PubMed] [Google Scholar]
- Gonzalez-Serratos H., Rasgado-Flores H. Extracellular magnesium-dependent sodium efflux in squid giant axons. Am J Physiol. 1990 Oct;259(4 Pt 1):C541–C548. doi: 10.1152/ajpcell.1990.259.4.C541. [DOI] [PubMed] [Google Scholar]
- Günther T., Vormann J. Activation of Na+/Mg2+ antiport in thymocytes by cAMP. FEBS Lett. 1992 Feb 3;297(1-2):132–134. doi: 10.1016/0014-5793(92)80343-f. [DOI] [PubMed] [Google Scholar]
- Günzel D., Schlue W. R. Sodium-magnesium antiport in Retzius neurones of the leech Hirudo medicinalis. J Physiol. 1996 Mar 15;491(Pt 3):595–608. doi: 10.1113/jphysiol.1996.sp021242. [DOI] [PMC free article] [PubMed] [Google Scholar]
- Güther T., Vormann J., Förster R. Regulation of intracellular magnesium by Mg2+ efflux. Biochem Biophys Res Commun. 1984 Feb 29;119(1):124–131. doi: 10.1016/0006-291x(84)91627-9. [DOI] [PubMed] [Google Scholar]
- Handy R. D., Gow I. F., Ellis D., Flatman P. W. Na-dependent regulation of intracellular free magnesium concentration in isolated rat ventricular myocytes. J Mol Cell Cardiol. 1996 Aug;28(8):1641–1651. doi: 10.1006/jmcc.1996.0154. [DOI] [PubMed] [Google Scholar]
- Harootunian A. T., Kao J. P., Eckert B. K., Tsien R. Y. Fluorescence ratio imaging of cytosolic free Na+ in individual fibroblasts and lymphocytes. J Biol Chem. 1989 Nov 15;264(32):19458–19467. [PubMed] [Google Scholar]
- Hongo K., Konishi M., Kurihara S. Cytoplasmic free Mg2+ in rat ventricular myocytes studied with the fluorescent indicator furaptra. Jpn J Physiol. 1994;44(4):357–378. doi: 10.2170/jjphysiol.44.357. [DOI] [PubMed] [Google Scholar]
- Iwamoto T., Watano T., Shigekawa M. A novel isothiourea derivative selectively inhibits the reverse mode of Na+/Ca2+ exchange in cells expressing NCX1. J Biol Chem. 1996 Sep 13;271(37):22391–22397. doi: 10.1074/jbc.271.37.22391. [DOI] [PubMed] [Google Scholar]
- Kennedy R. H., Wyeth R. P., Gerner P., Liu S., Fontenot H. J., Seifen E. Tetramethylammonium is a muscarinic agonist in rat heart. Am J Physiol. 1995 Jun;268(6 Pt 1):C1414–C1417. doi: 10.1152/ajpcell.1995.268.6.C1414. [DOI] [PubMed] [Google Scholar]
- Kimura J. Effects of external Mg2+ on the Na-Ca exchange current in guinea pig cardiac myocytes. Ann N Y Acad Sci. 1996 Apr 15;779:515–520. doi: 10.1111/j.1749-6632.1996.tb44825.x. [DOI] [PubMed] [Google Scholar]
- Konishi M., Suda N., Kurihara S. Fluorescence signals from the Mg2+/Ca2+ indicator furaptra in frog skeletal muscle fibers. Biophys J. 1993 Jan;64(1):223–239. doi: 10.1016/S0006-3495(93)81359-6. [DOI] [PMC free article] [PubMed] [Google Scholar]
- Koss K. L., Putnam R. W., Grubbs R. D. Mg2+ buffering in cultured chick ventricular myocytes: quantitation and modulation by Ca2+. Am J Physiol. 1993 May;264(5 Pt 1):C1259–C1269. doi: 10.1152/ajpcell.1993.264.5.C1259. [DOI] [PubMed] [Google Scholar]
- Lyu R. M., Smith L., Smith J. B. Sodium-calcium exchange in renal epithelial cells: dependence on cell sodium and competitive inhibition by magnesium. J Membr Biol. 1991 Oct;124(1):73–83. doi: 10.1007/BF01871366. [DOI] [PubMed] [Google Scholar]
- Mullins L. J., Brinley F. J., Jr, Spangler S. G., Abercrombie R. F. Magnesium efflux in dialyzed squid axons. J Gen Physiol. 1977 Apr;69(4):389–400. doi: 10.1085/jgp.69.4.389. [DOI] [PMC free article] [PubMed] [Google Scholar]
- Murphy E., Freudenrich C. C., Lieberman M. Cellular magnesium and Na/Mg exchange in heart cells. Annu Rev Physiol. 1991;53:273–287. doi: 10.1146/annurev.ph.53.030191.001421. [DOI] [PubMed] [Google Scholar]
- Nakayama S., Nomura H. Mechanisms of intracellular Mg2+ regulation affected by amiloride and ouabain in the guinea-pig taenia caeci. J Physiol. 1995 Oct 1;488(Pt 1):1–12. doi: 10.1113/jphysiol.1995.sp020941. [DOI] [PMC free article] [PubMed] [Google Scholar]
- Nakayama S., Nomura H., Tomita T. Intracellular-free magnesium in the smooth muscle of guinea pig taenia caeci: a concomitant analysis for magnesium and pH upon sodium removal. J Gen Physiol. 1994 May;103(5):833–851. doi: 10.1085/jgp.103.5.833. [DOI] [PMC free article] [PubMed] [Google Scholar]
- Nakayama S., Tomita T. Depletion of intracellular free Mg2+ in Mg2(+)- and Ca2(+)-free solution in the taenia isolated from guinea-pig caecum. J Physiol. 1990 Feb;421:363–378. doi: 10.1113/jphysiol.1990.sp017949. [DOI] [PMC free article] [PubMed] [Google Scholar]
- Nakayama S., Tomita T. Regulation of intracellular free magnesium concentration in the taenia of guinea-pig caecum. J Physiol. 1991 Apr;435:559–572. doi: 10.1113/jphysiol.1991.sp018525. [DOI] [PMC free article] [PubMed] [Google Scholar]
- Palatý V. Regulation of the cell magnesium in vascular smooth muscle. J Physiol. 1974 Oct;242(2):555–569. doi: 10.1113/jphysiol.1974.sp010723. [DOI] [PMC free article] [PubMed] [Google Scholar]
- Raju B., Murphy E., Levy L. A., Hall R. D., London R. E. A fluorescent indicator for measuring cytosolic free magnesium. Am J Physiol. 1989 Mar;256(3 Pt 1):C540–C548. doi: 10.1152/ajpcell.1989.256.3.C540. [DOI] [PubMed] [Google Scholar]
- Romani A., Scarpa A. Regulation of cell magnesium. Arch Biochem Biophys. 1992 Oct;298(1):1–12. doi: 10.1016/0003-9861(92)90086-c. [DOI] [PubMed] [Google Scholar]
- Shetty S. S., Weiss G. B. Alterations in 28Mg distribution and movements in rabbit aortic smooth muscle. J Pharmacol Exp Ther. 1988 Apr;245(1):112–119. [PubMed] [Google Scholar]
- Stout A. K., Li-Smerin Y., Johnson J. W., Reynolds I. J. Mechanisms of glutamate-stimulated Mg2+ influx and subsequent Mg2+ efflux in rat forebrain neurones in culture. J Physiol. 1996 May 1;492(Pt 3):641–657. doi: 10.1113/jphysiol.1996.sp021334. [DOI] [PMC free article] [PubMed] [Google Scholar]
- Tashiro M., Konishi M. Basal intracellular free Mg2+ concentration in smooth muscle cells of guinea pig tenia cecum: intracellular calibration of the fluorescent indicator furaptra. Biophys J. 1997 Dec;73(6):3358–3370. doi: 10.1016/S0006-3495(97)78360-7. [DOI] [PMC free article] [PubMed] [Google Scholar]
- Wasserman A. J., McClellan G., Somlyo A. P. Calcium-sensitive cellular and subcellular transport of sodium, potassium, magnesium, and calcium in sodium-loaded vascular smooth muscle. Electron probe analysis. Circ Res. 1986 Jun;58(6):790–802. doi: 10.1161/01.res.58.6.790. [DOI] [PubMed] [Google Scholar]
- Watano T., Kimura J., Morita T., Nakanishi H. A novel antagonist, No. 7943, of the Na+/Ca2+ exchange current in guinea-pig cardiac ventricular cells. Br J Pharmacol. 1996 Oct;119(3):555–563. doi: 10.1111/j.1476-5381.1996.tb15708.x. [DOI] [PMC free article] [PubMed] [Google Scholar]
- Westerblad H., Allen D. G. Myoplasmic free Mg2+ concentration during repetitive stimulation of single fibres from mouse skeletal muscle. J Physiol. 1992;453:413–434. doi: 10.1113/jphysiol.1992.sp019236. [DOI] [PMC free article] [PubMed] [Google Scholar]
- Xu W., Willis J. S. Sodium transport through the amiloride-sensitive Na-Mg pathway of hamster red cells. J Membr Biol. 1994 Sep;141(3):277–287. doi: 10.1007/BF00235137. [DOI] [PubMed] [Google Scholar]
- Zhang G. H., Melvin J. E. Regulation by extracellular Na+ of cytosolic Mg2+ concentration in Mg(2+)-loaded rat sublingual acini. FEBS Lett. 1995 Aug 28;371(1):52–56. doi: 10.1016/0014-5793(95)00869-b. [DOI] [PubMed] [Google Scholar]