Abstract
1. Stable N-methyl-D-aspartic acid (NMDA) receptor-mediated currents in cultured mouse hippocampal neurons were evoked by 20 ms pressure pulse applications of L-aspartate, repeatedly applied at 30 or 40 s intervals, to the cell body region of the neurone. We have characterized the voltage- and use-dependent blockade of the currents by three dissociative anaesthetics: ketamine, phencyclidine (PCP) and MK-801 in mouse hippocampal neurones grown in dissociated tissue culture. 2. We have used a simple model of the blockade, based on the 'guarded receptor hypothesis' to interpret our data. The model assumes that receptors are maximally activated at the peak of the response with an open probability (Po) approaching 1, that there is no desensitization and that the blocking drug only associates with, or dissociates from, receptor channels which have been activated by agonist (e.g. open channels). 3. The model allows us to estimate forward and reverse rate constants for binding of the blockers to open channels from measurements of the steady-state level of blockade and the rate of change of the current amplitude per pulse during onset and offset of blockade. As predicted by the model, the estimated reverse rate was independent of blocker concentration while the forward rate increased with concentration. Changing the level of positively charged ketamine (pKa 7.5) tenfold by changing pH from 6.5 to 8.5 caused a corresponding change in the forward rate while having no effect on the reverse rate. Most of the voltage dependence of the blockade could be accounted for by reduction of the reverse rate by depolarization. 4. Estimated forward rate constants for ketamine, PCP and MK-801 were similar to one another when measured under similar conditions and were 3 x 10(4) - 3 x 10(5) M-1 S-1. Most of the differences in potency of the three blockers could be accounted for by differences in the reverse rate constants which were approximately 0.2, 0.03 and 0.003 s-1 for ketamine, PCP and MK-801, respectively. The estimated rate constants actually are the product of the rate constants and 1/Po. Suggestions that maximum Po is much less than 1 for NMDA channels imply that both forward and reverse rate constants of blockade may in fact be larger than we have calculated. However, their magnitudes, relative to one another, are unaffected by this consideration. 5. The reverse rate constant of blockade increased at positive potentials. This increase was prevented when the neurone was loaded with N-methyl-D-glucamine, an impermeant cation which prevented outward currents.(ABSTRACT TRUNCATED AT 400 WORDS)
Full text
PDF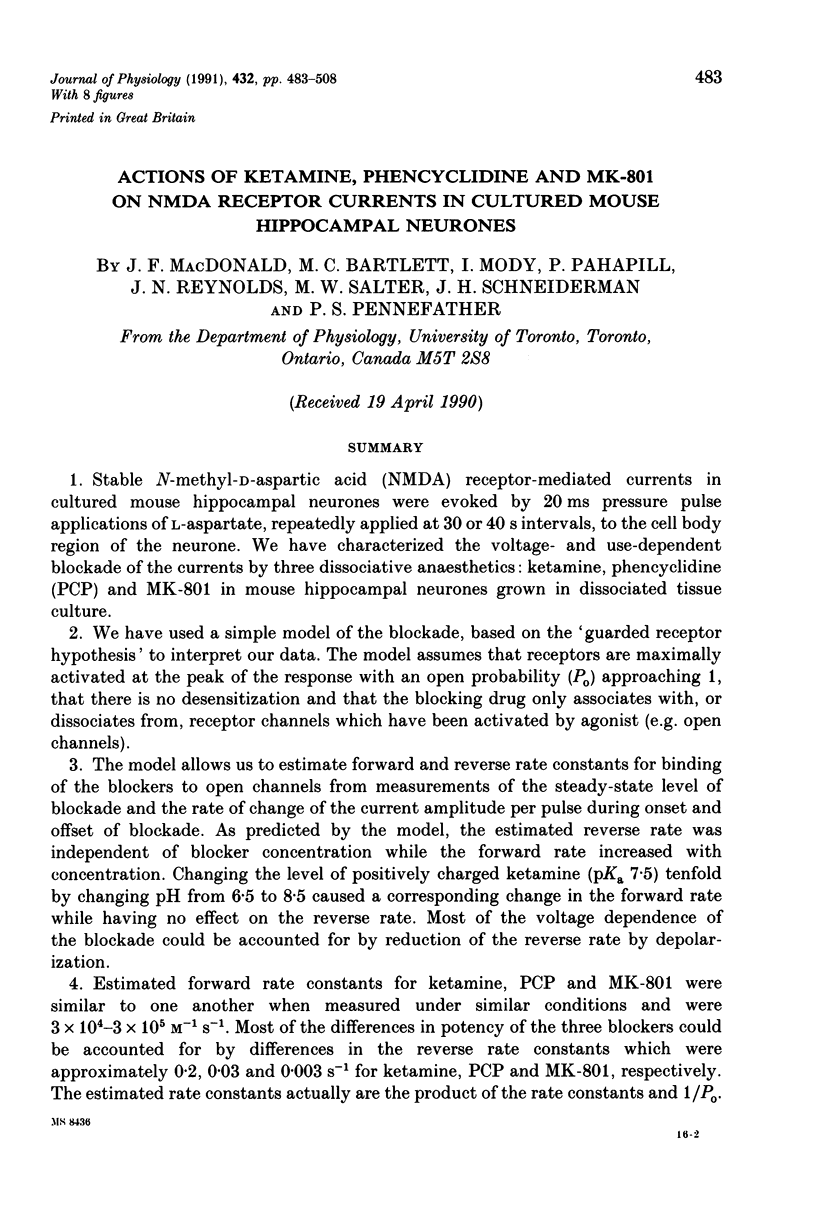
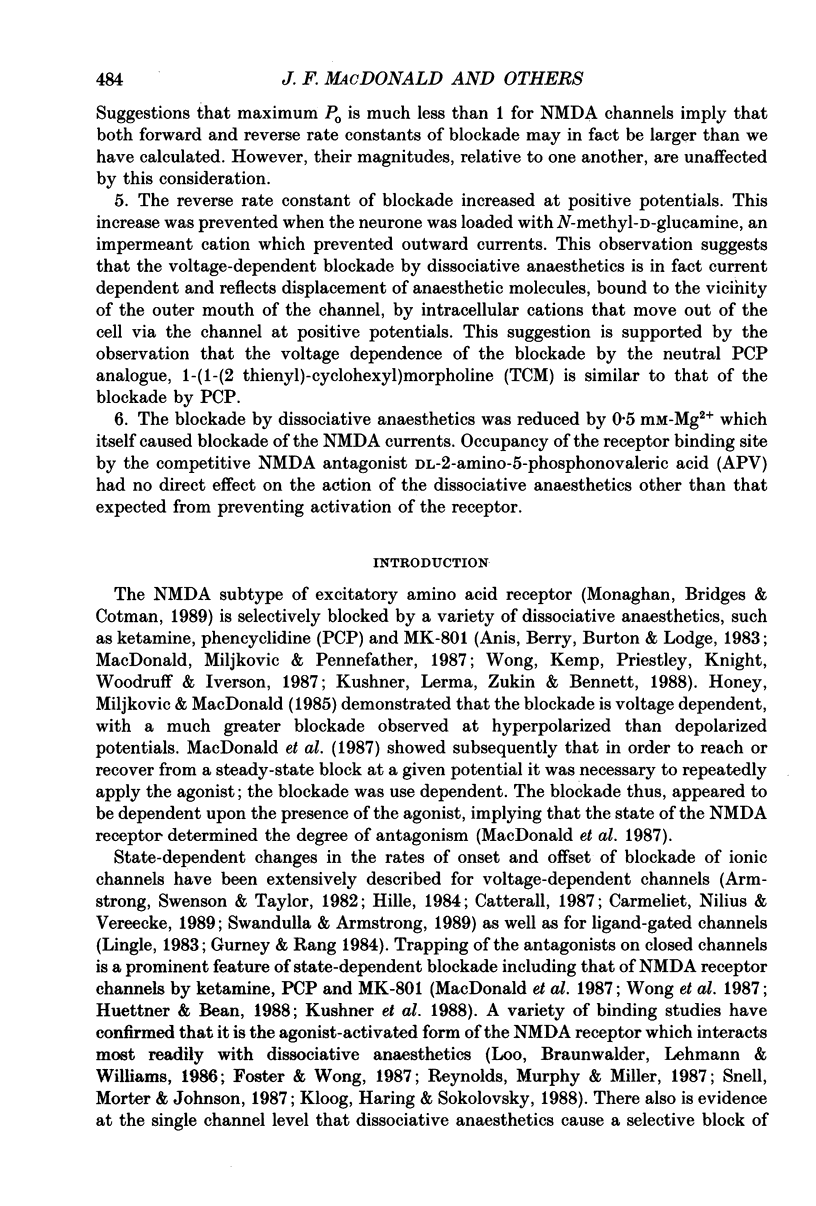
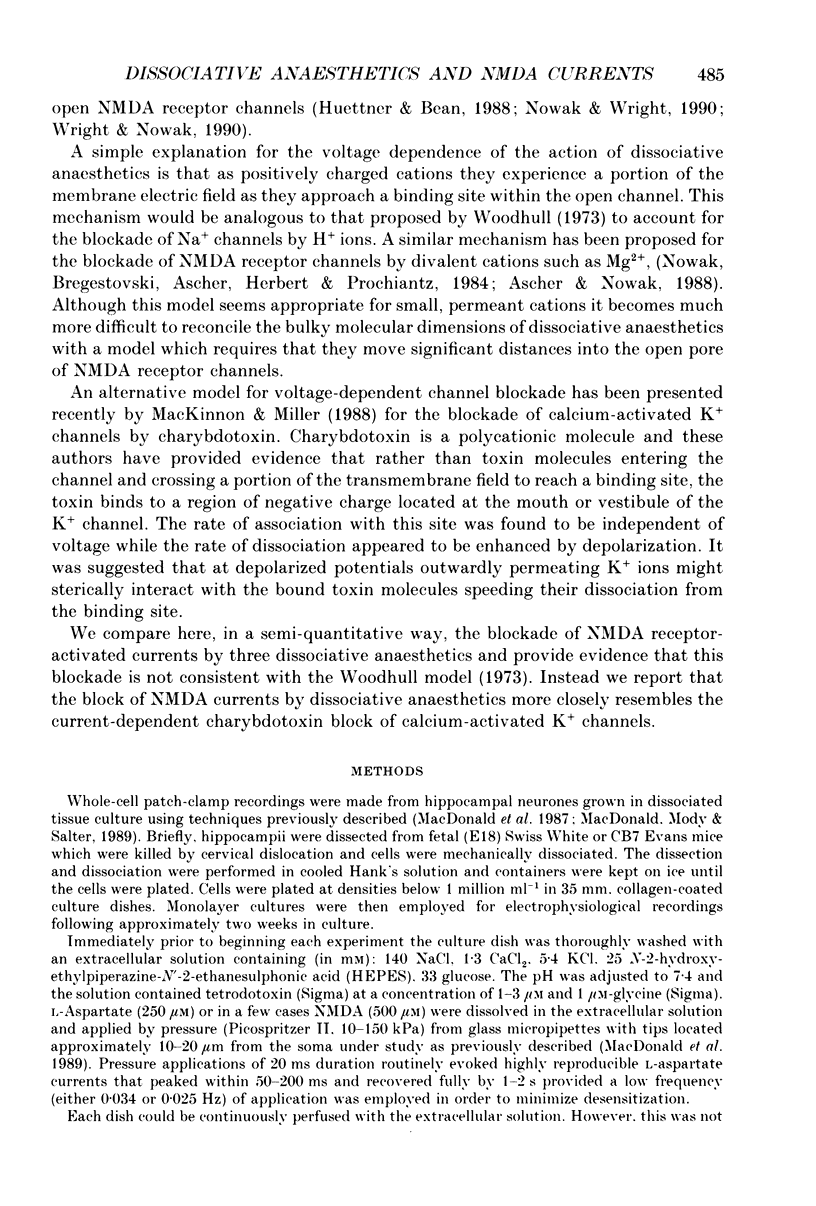
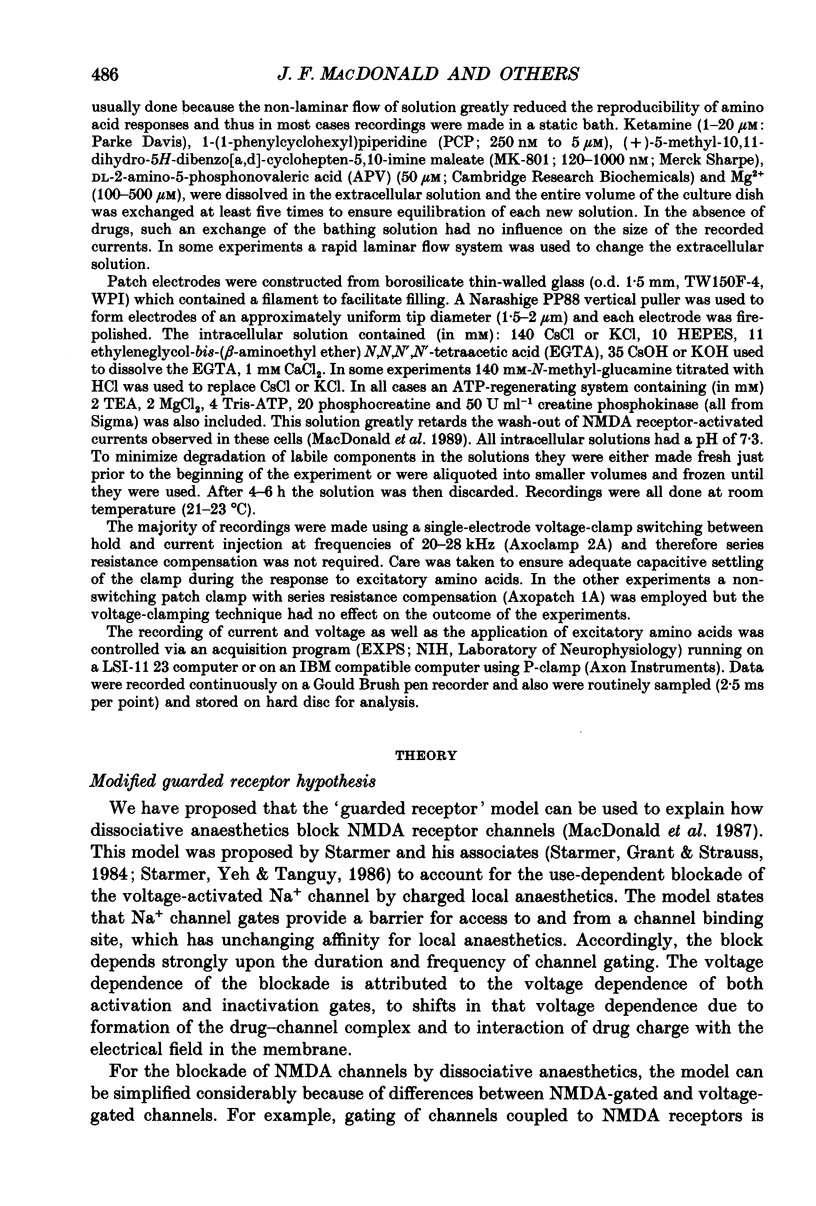
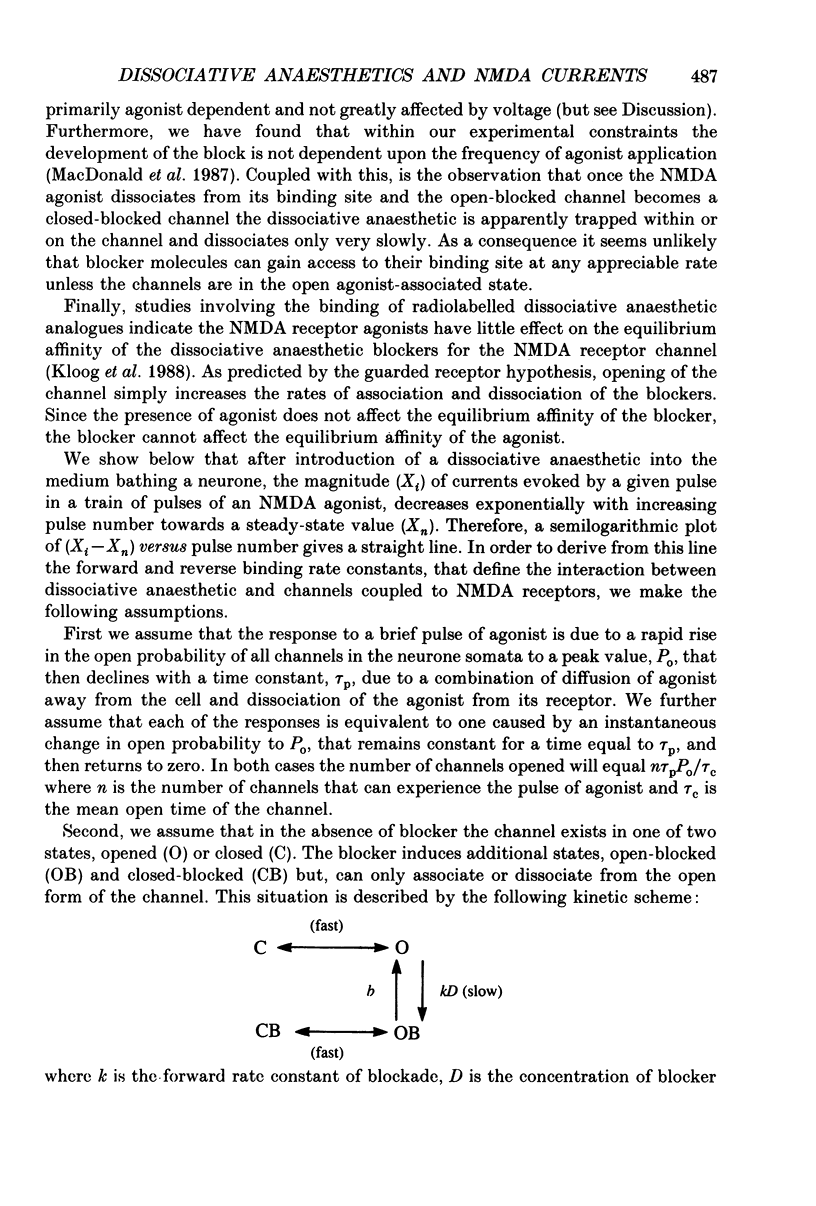
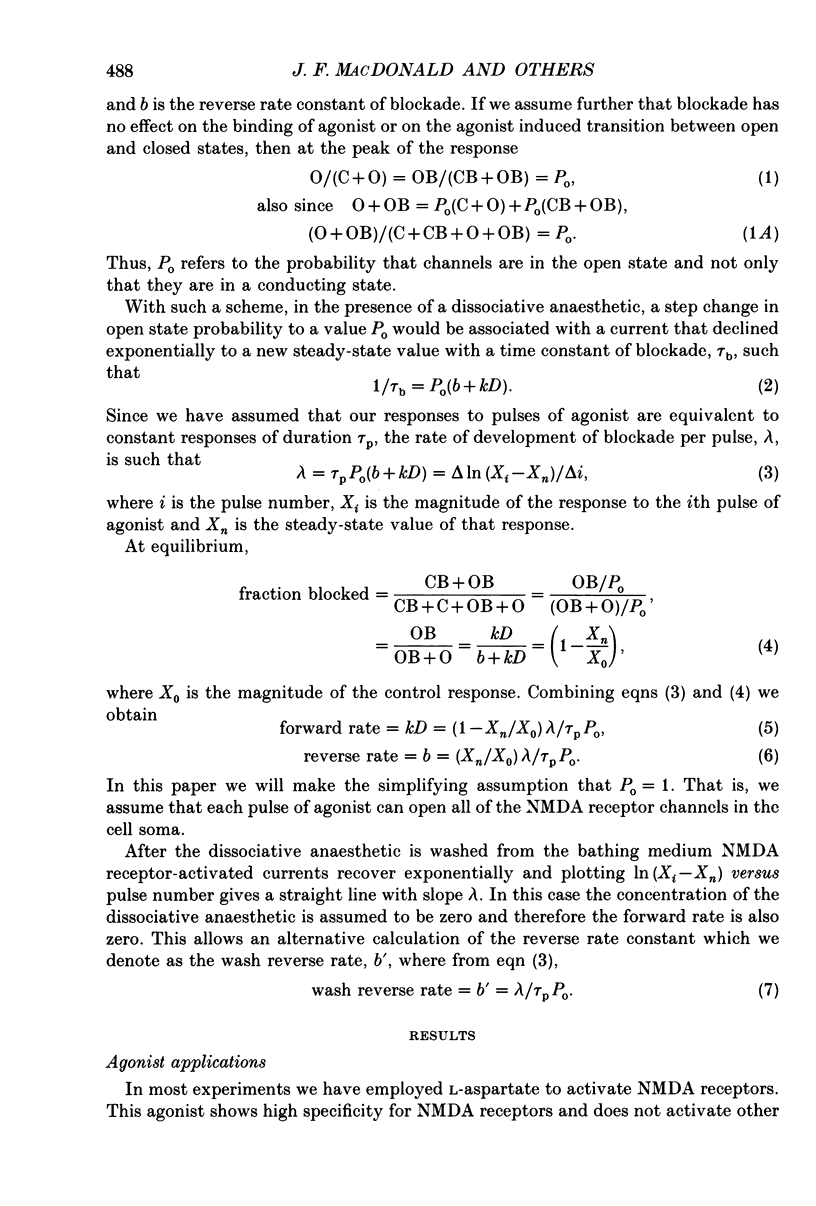
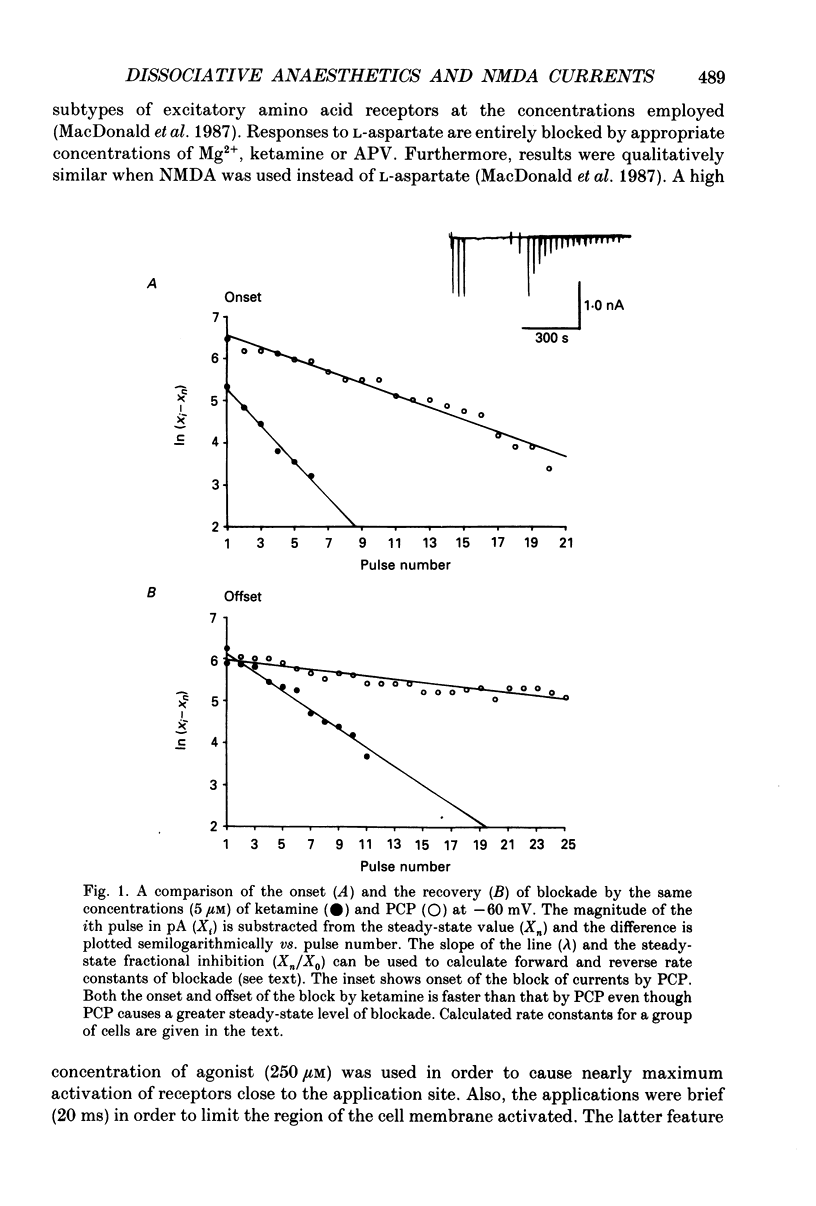
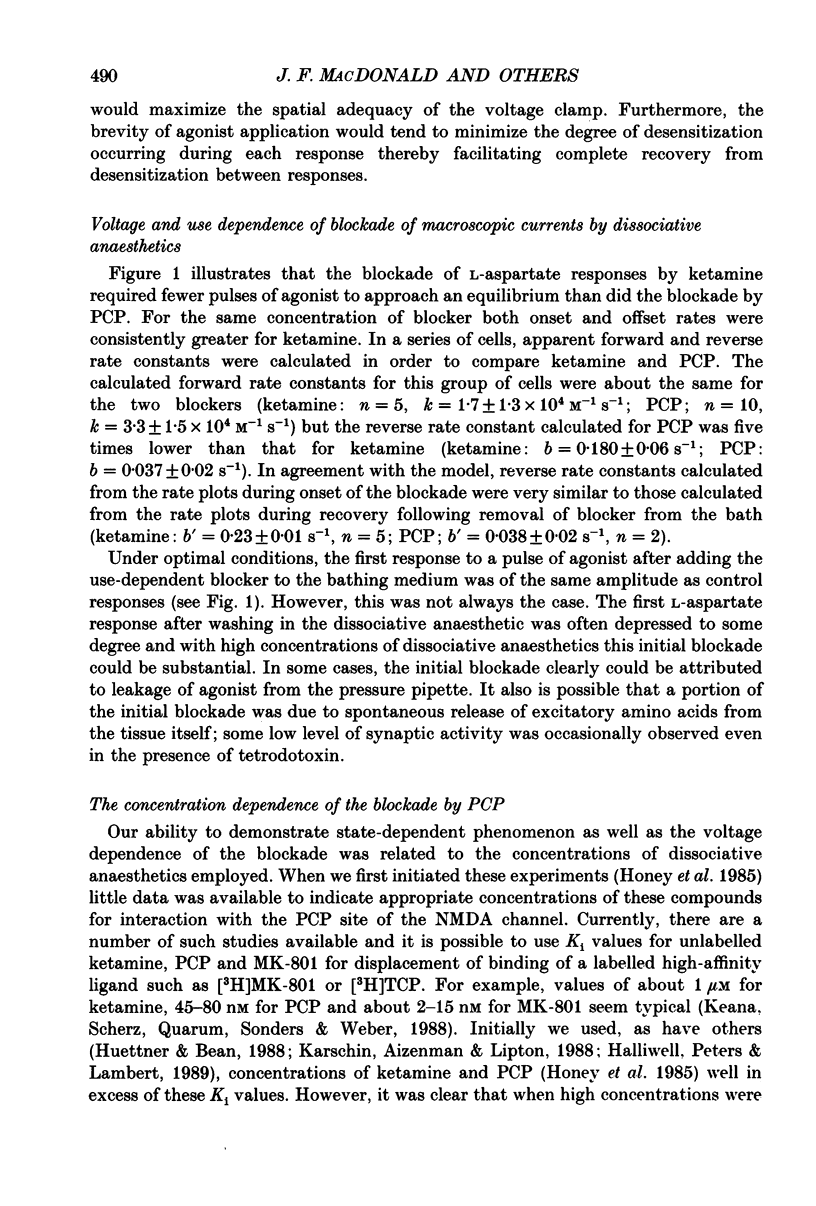
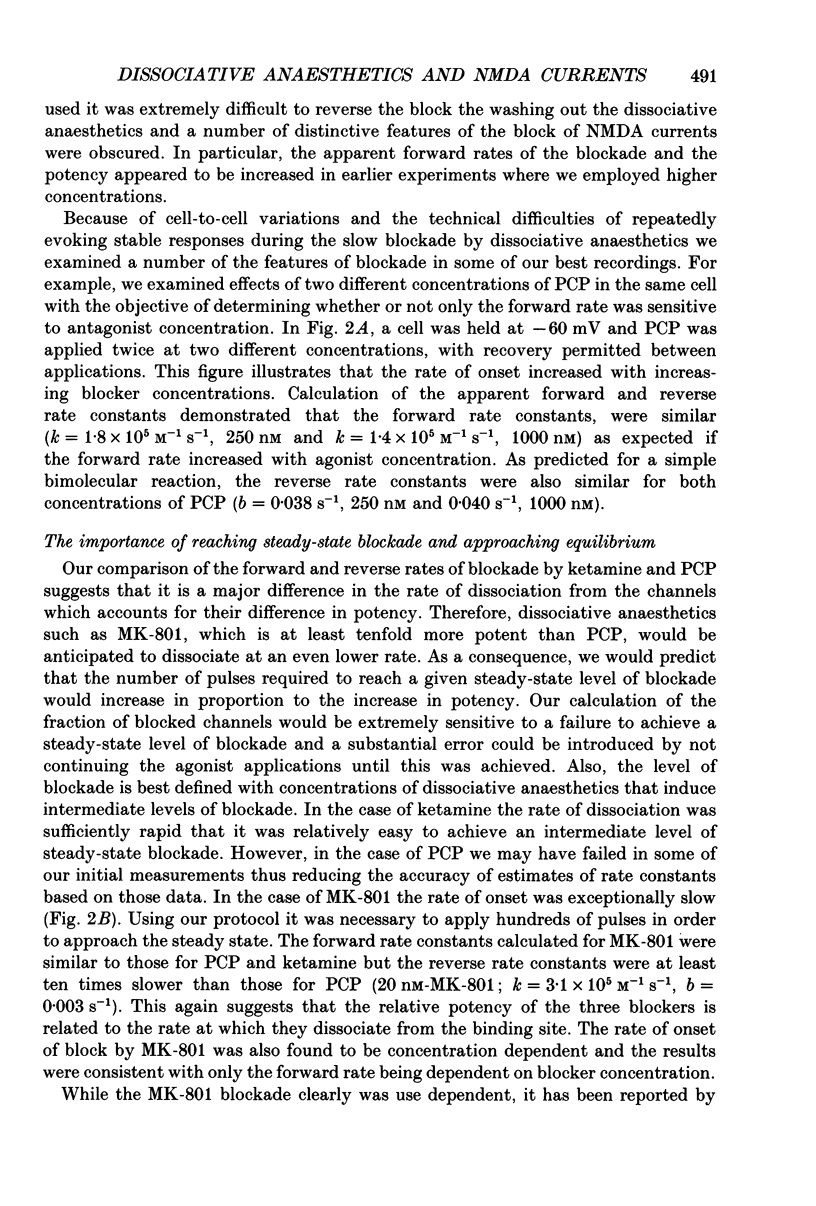
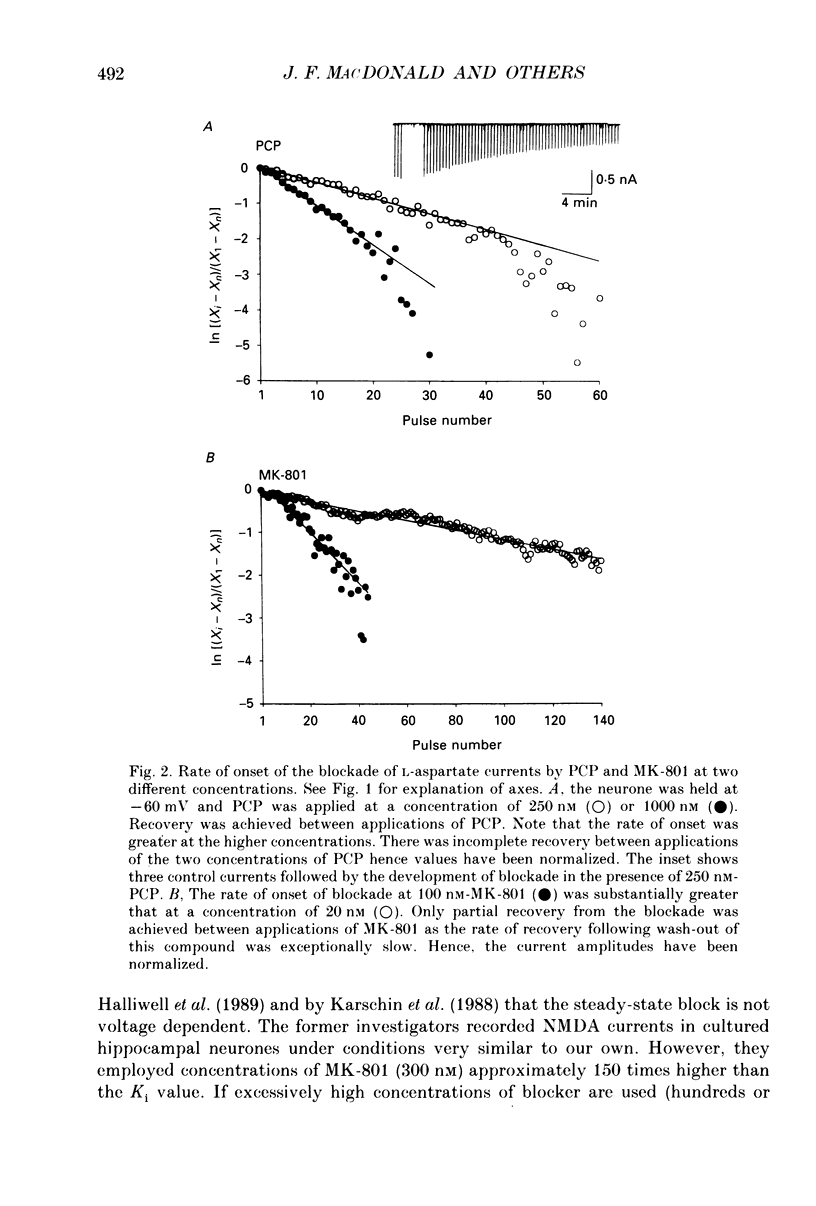
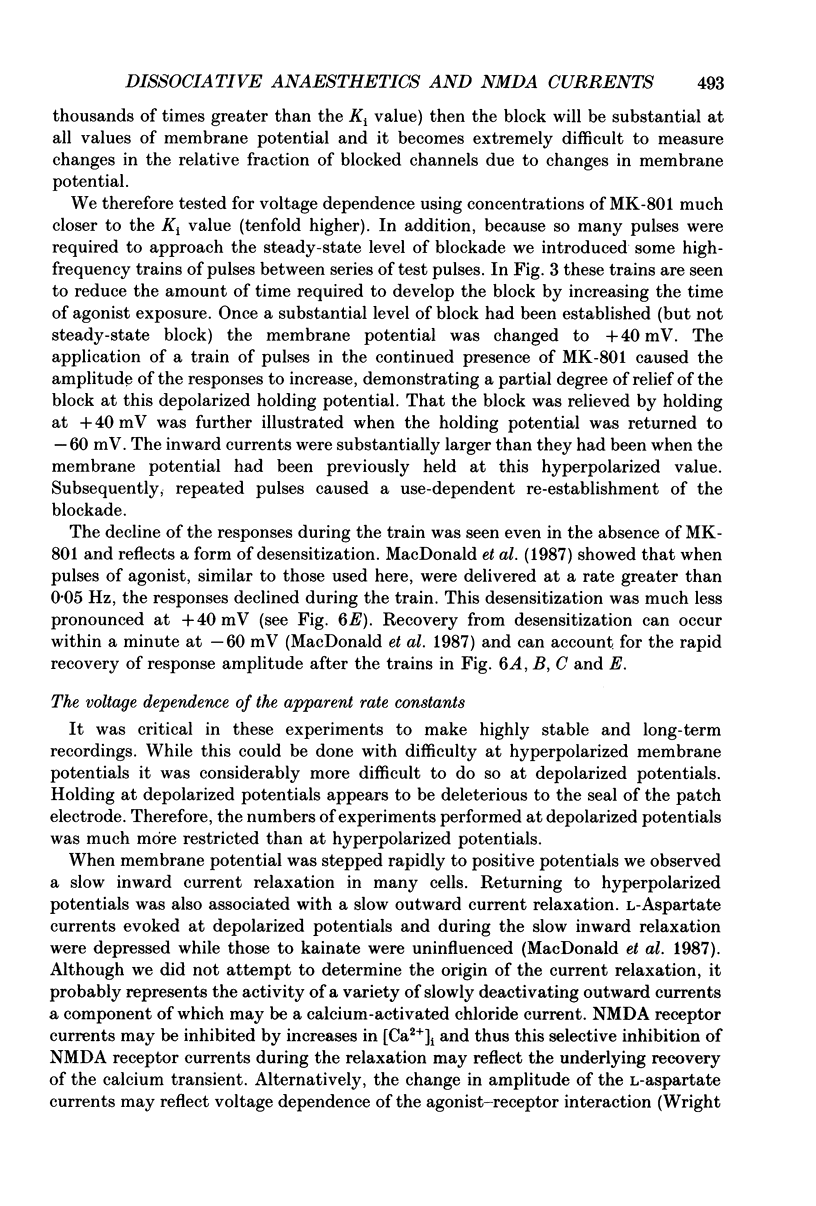
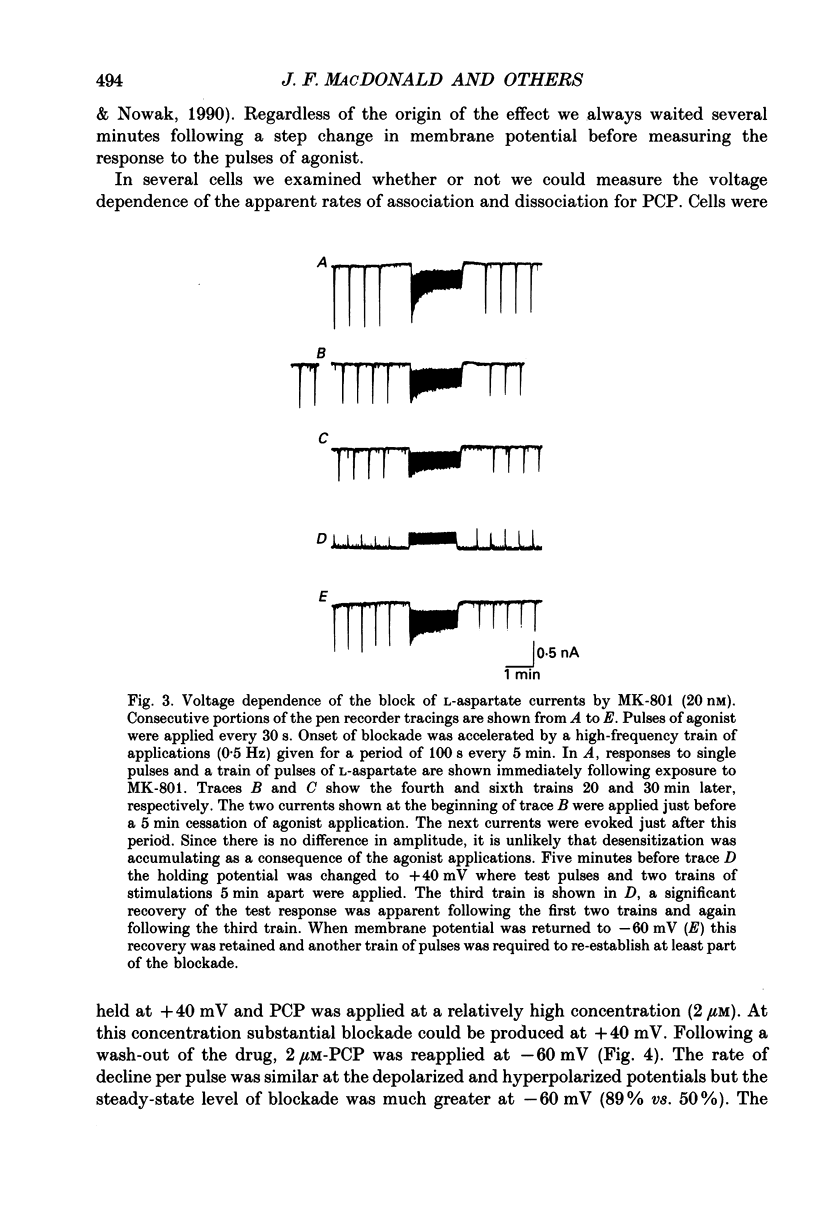
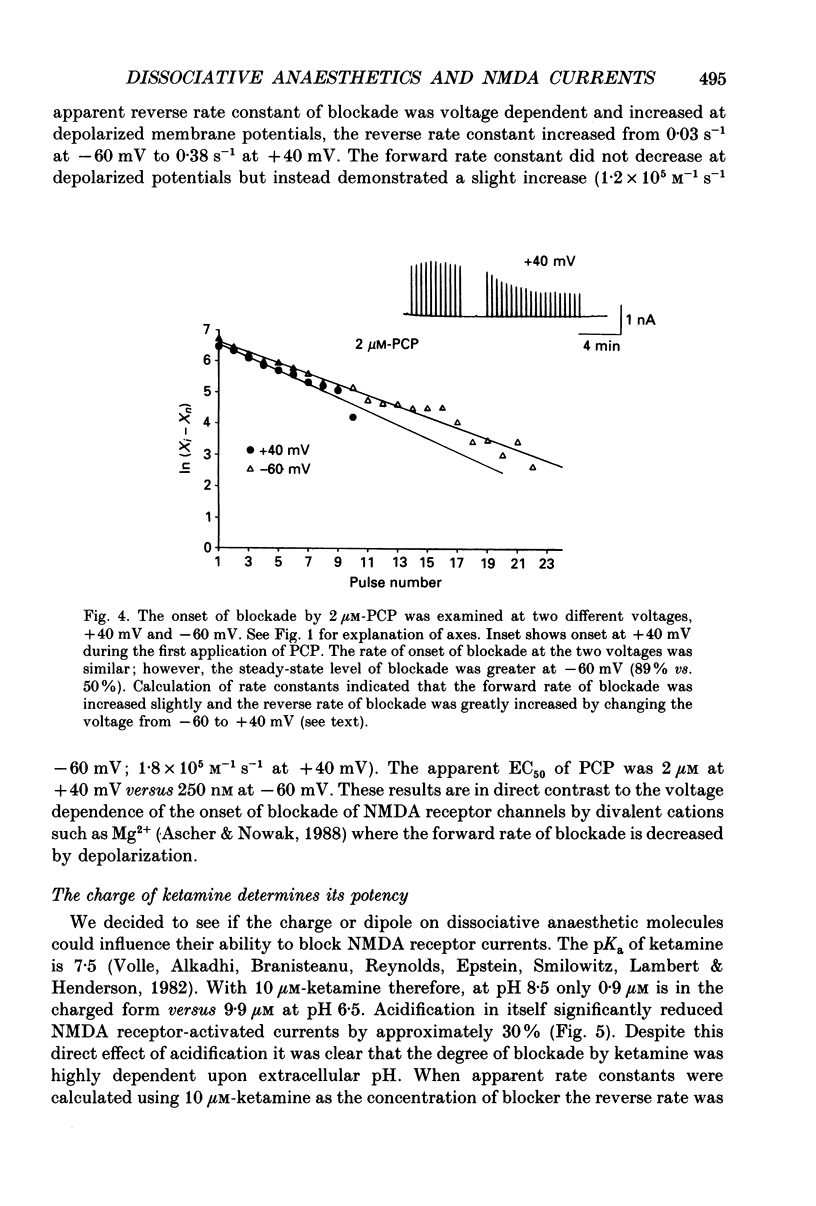
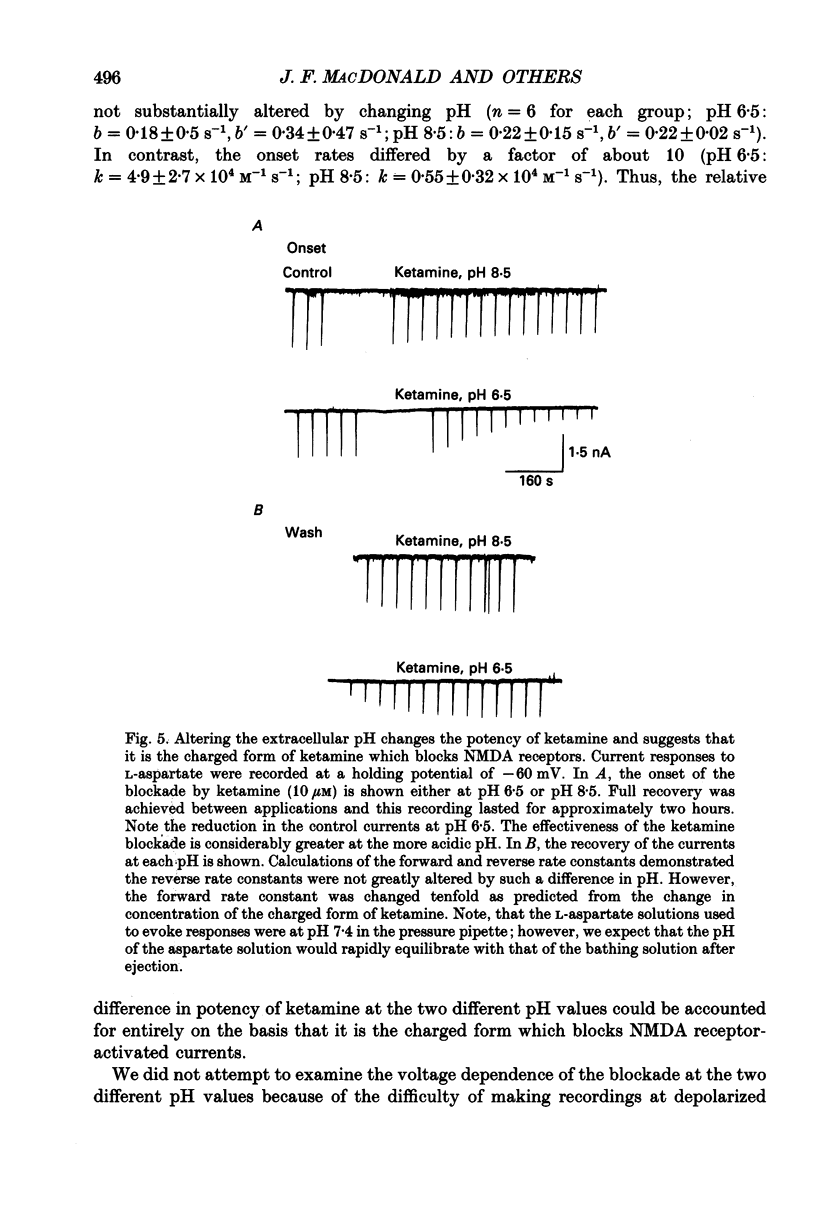
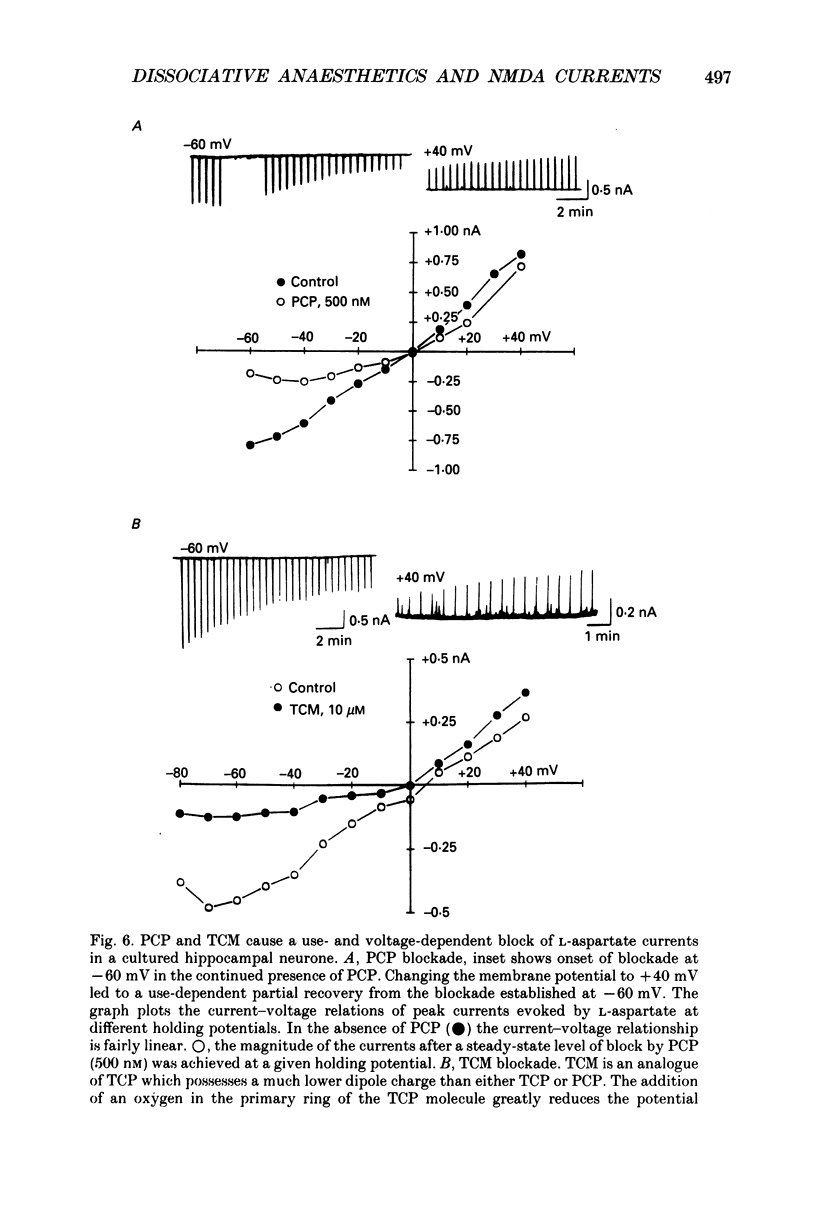
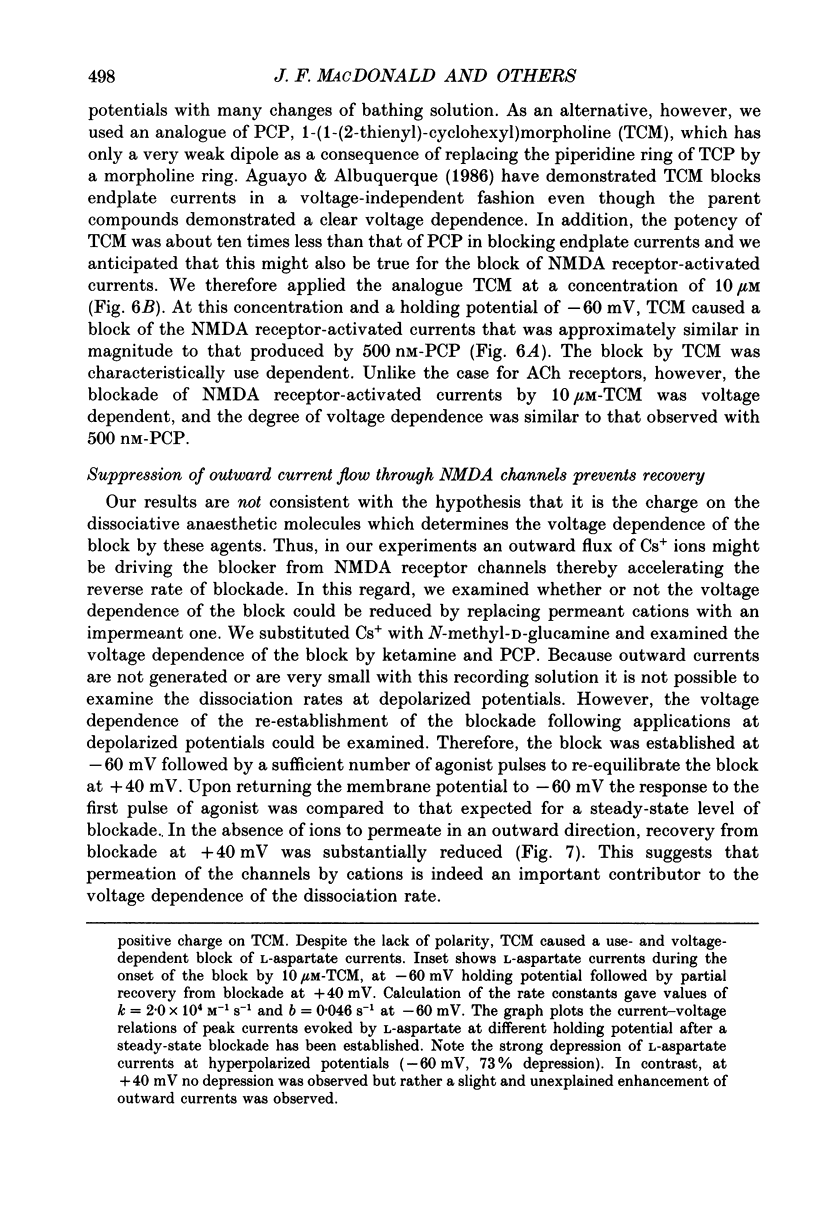
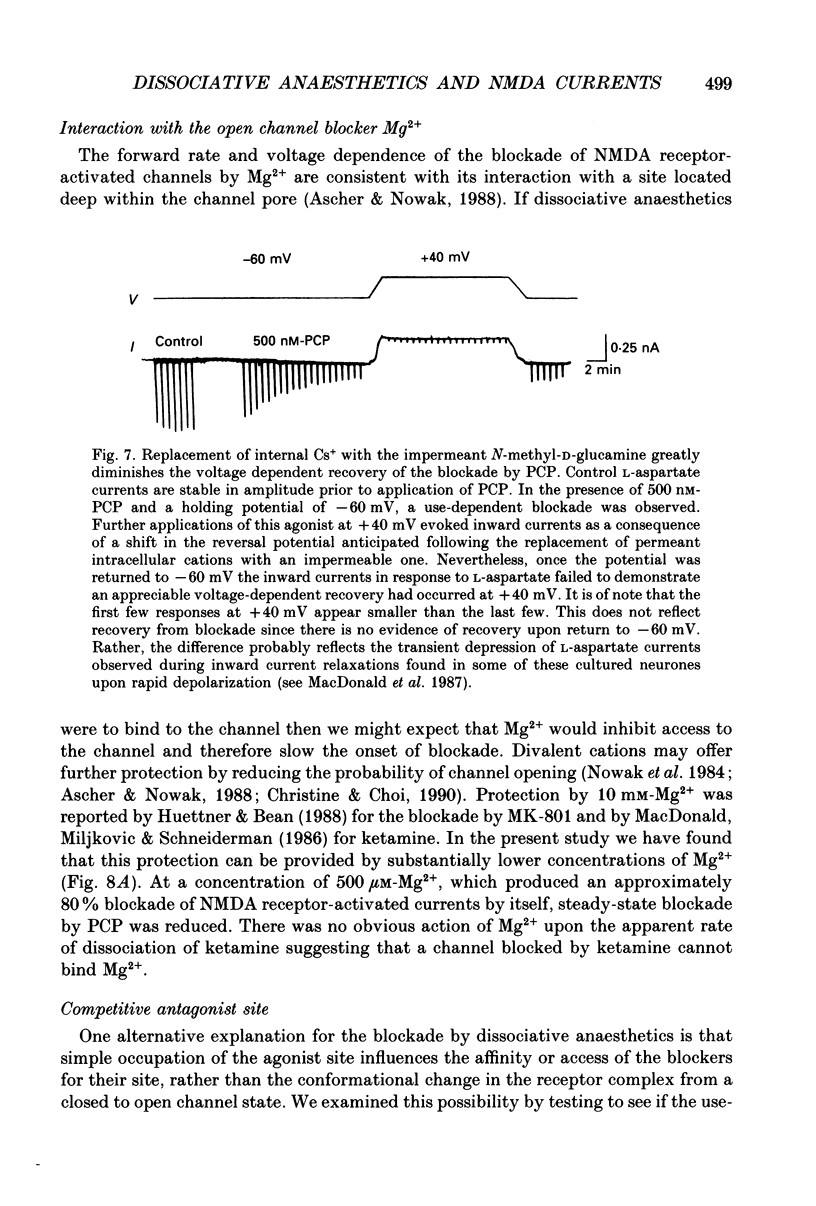
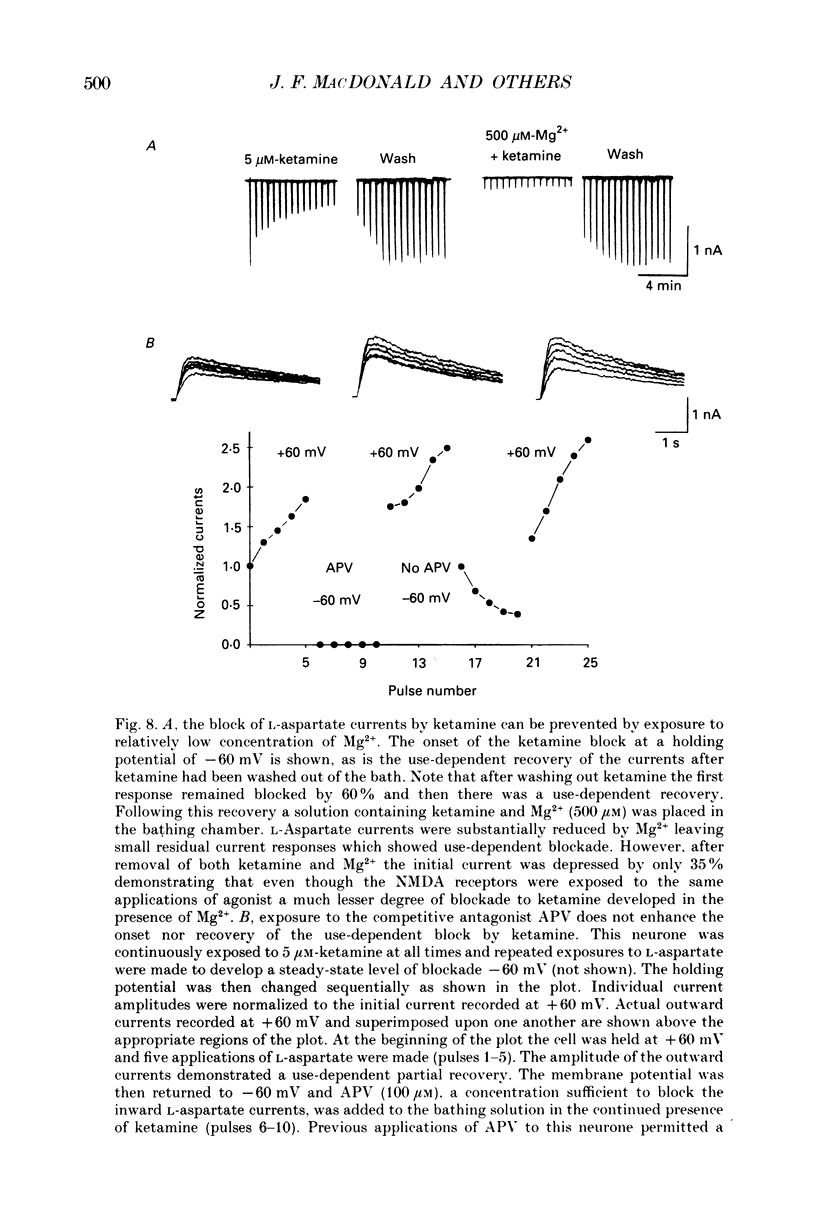
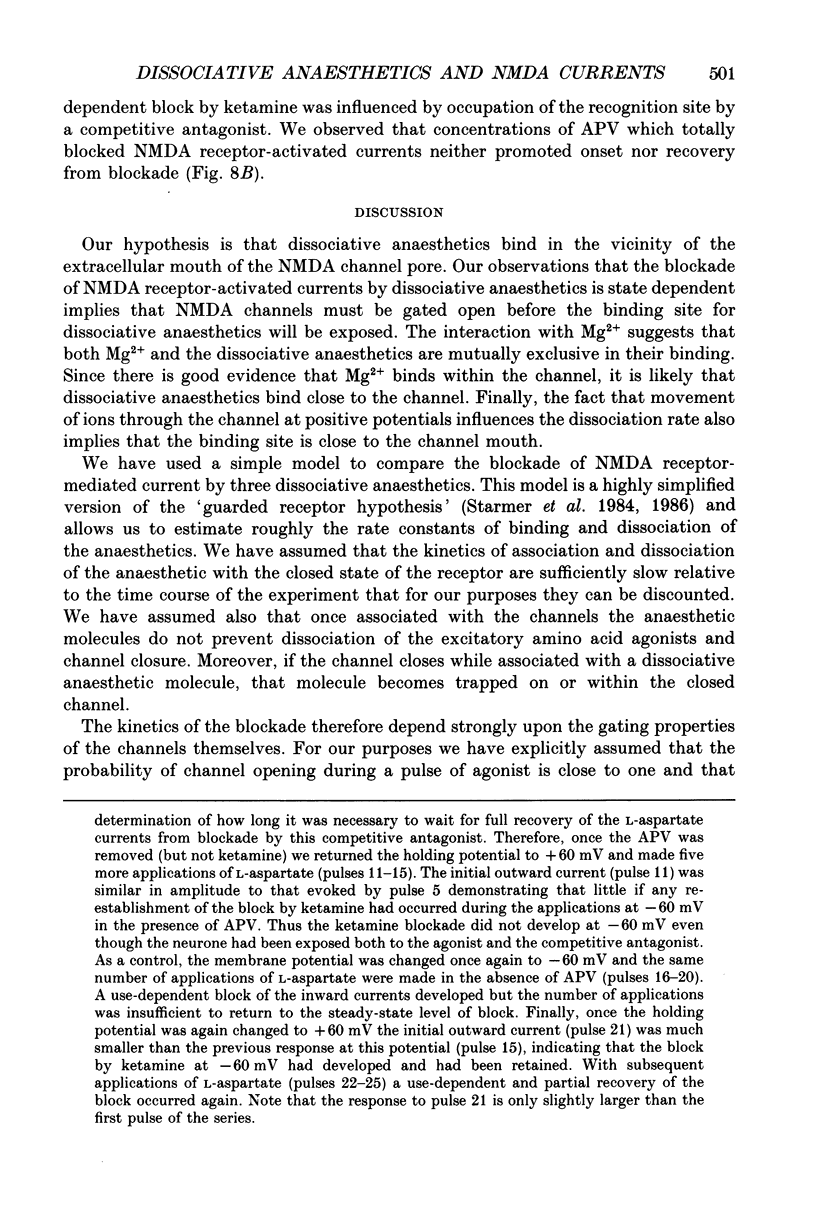
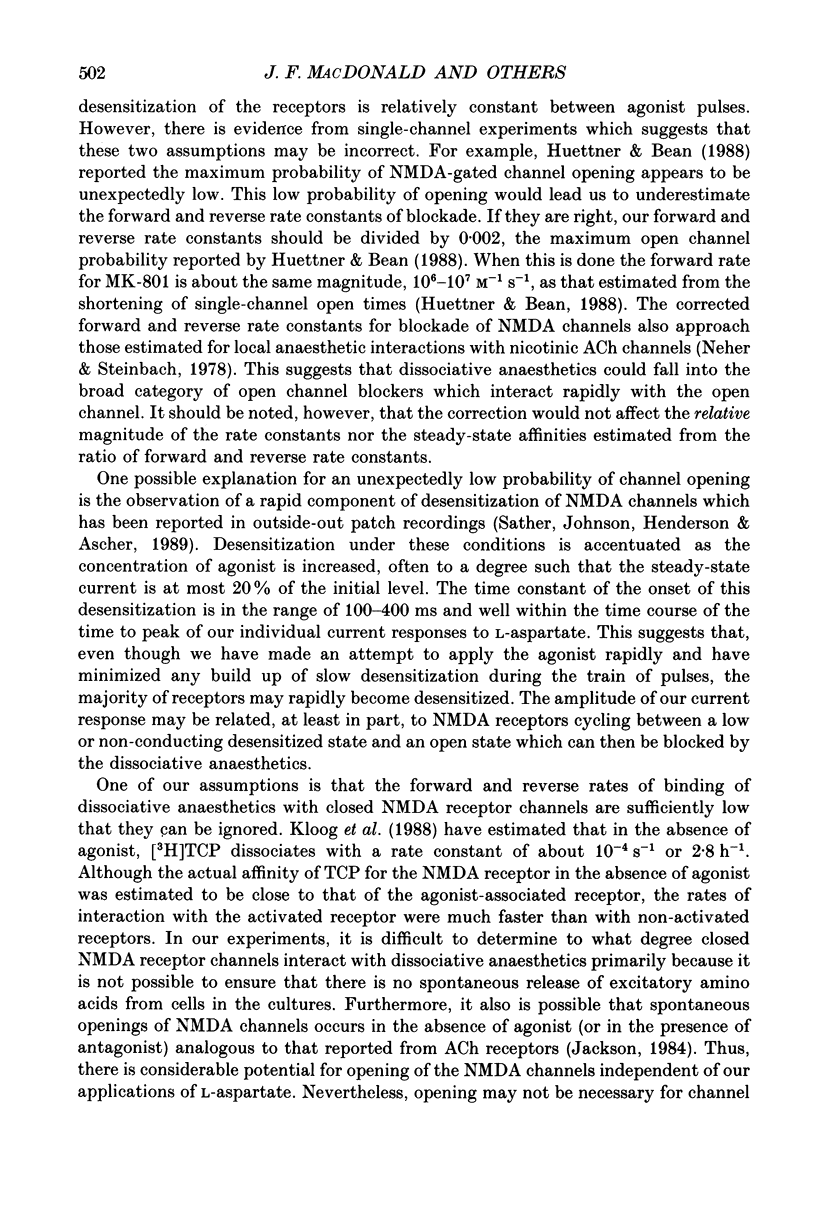
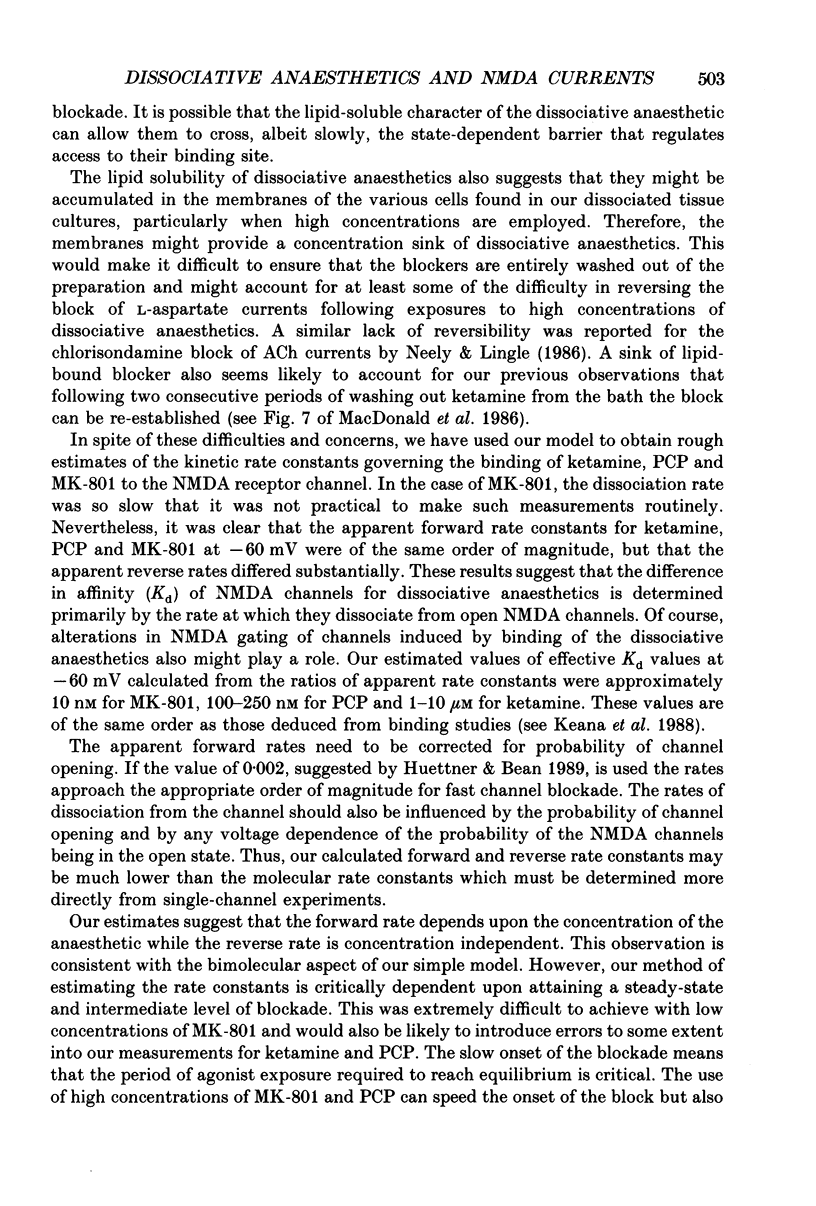
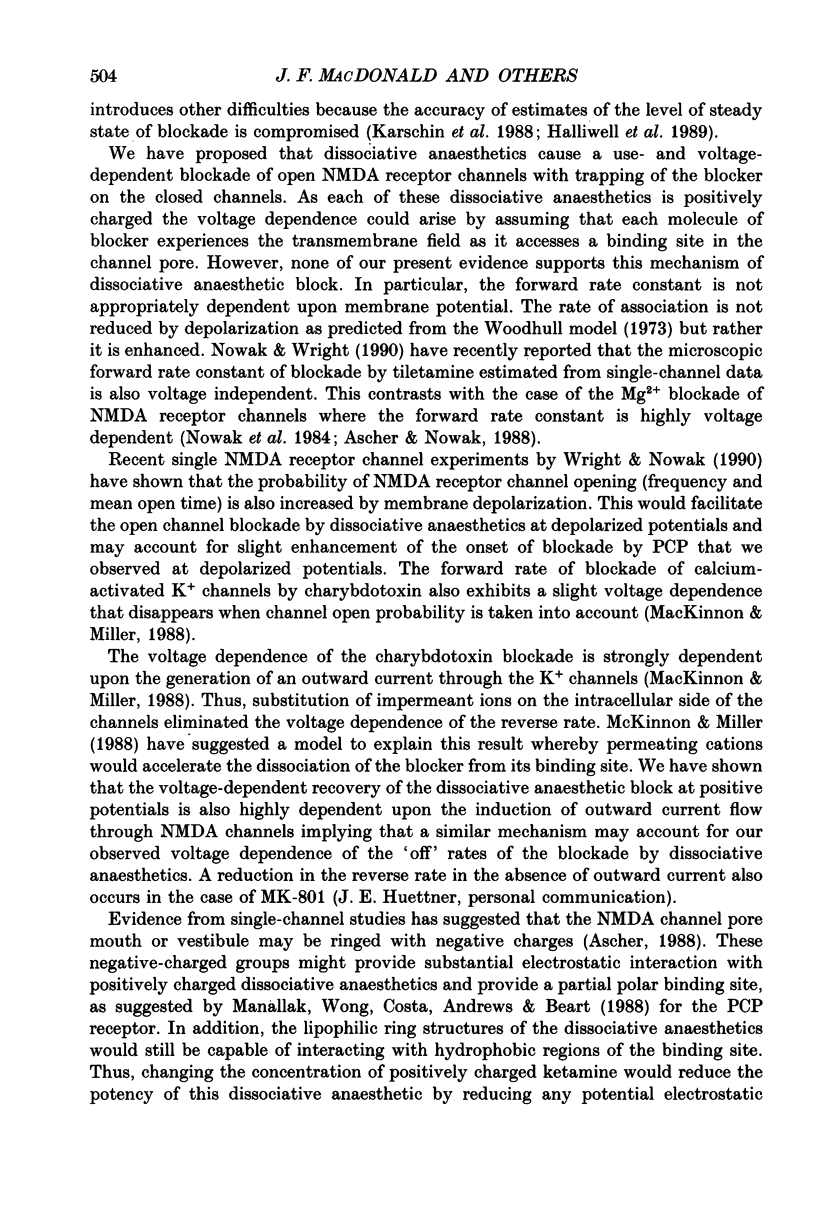
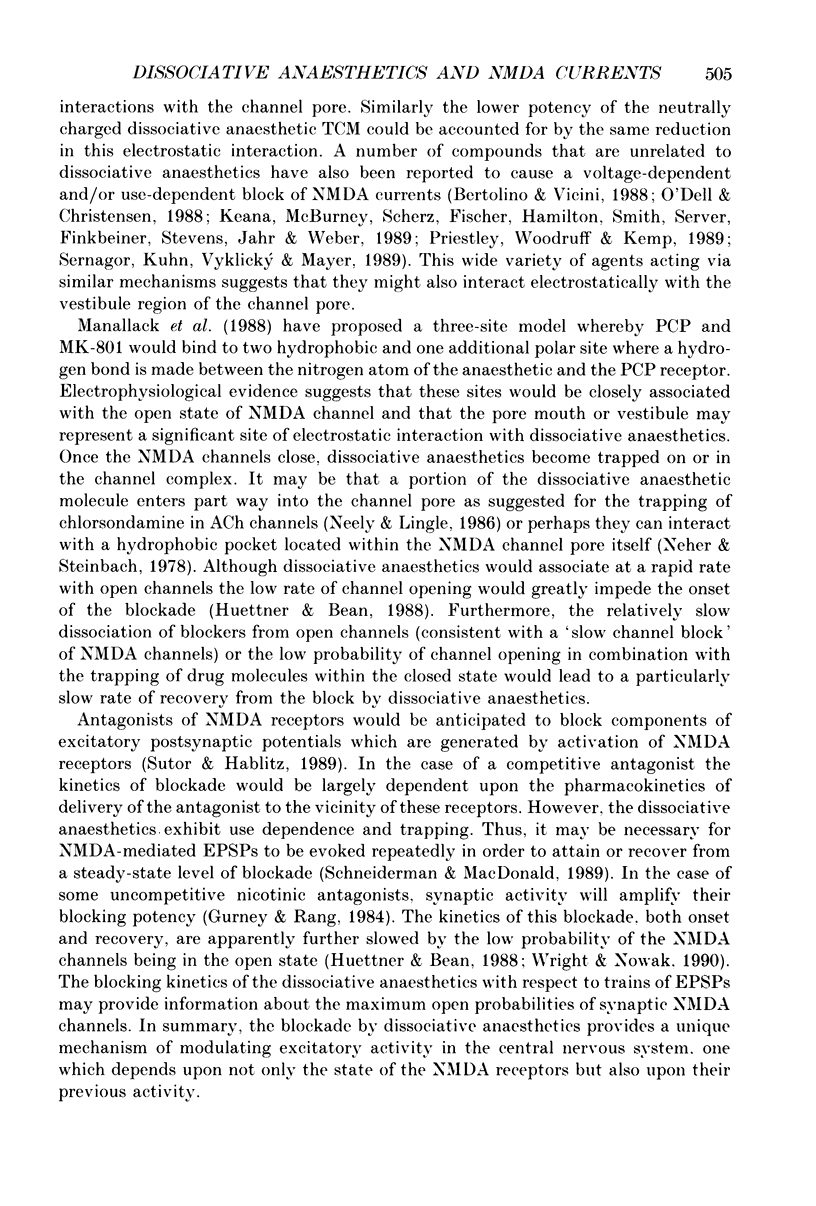
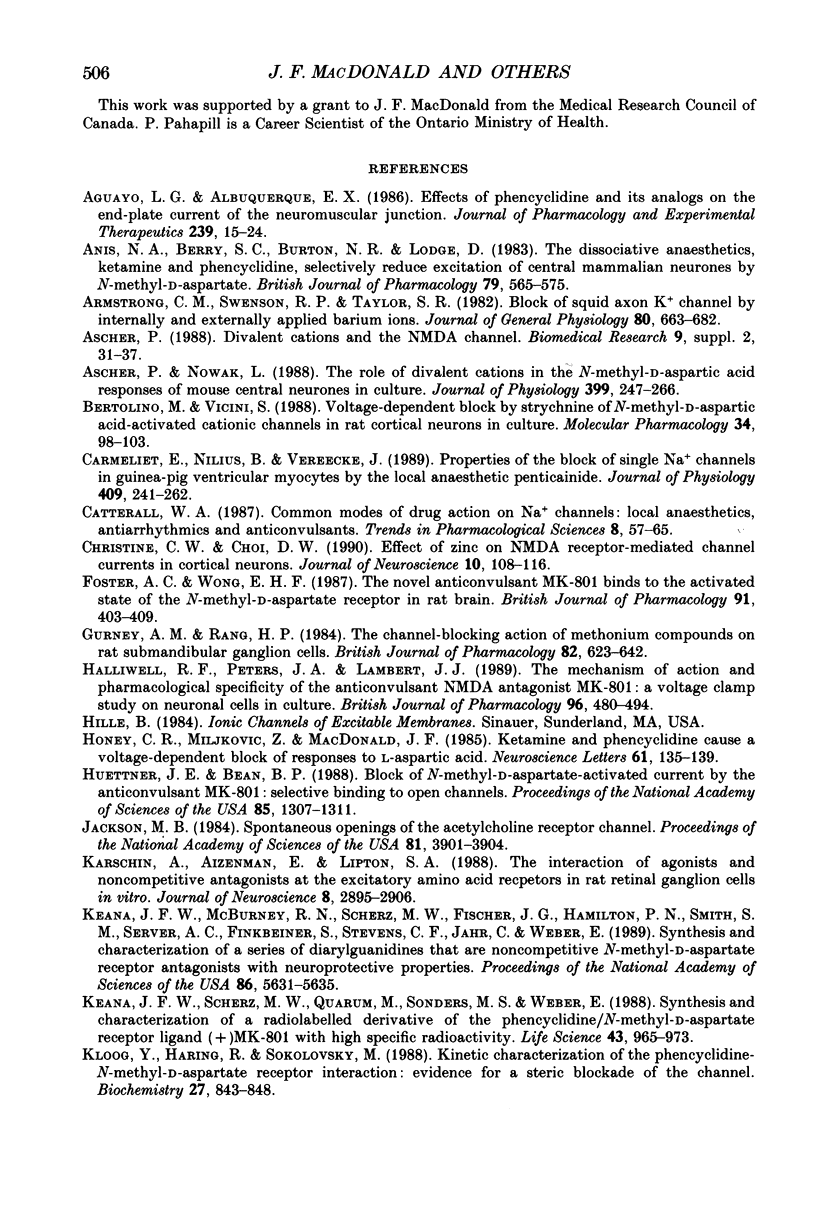
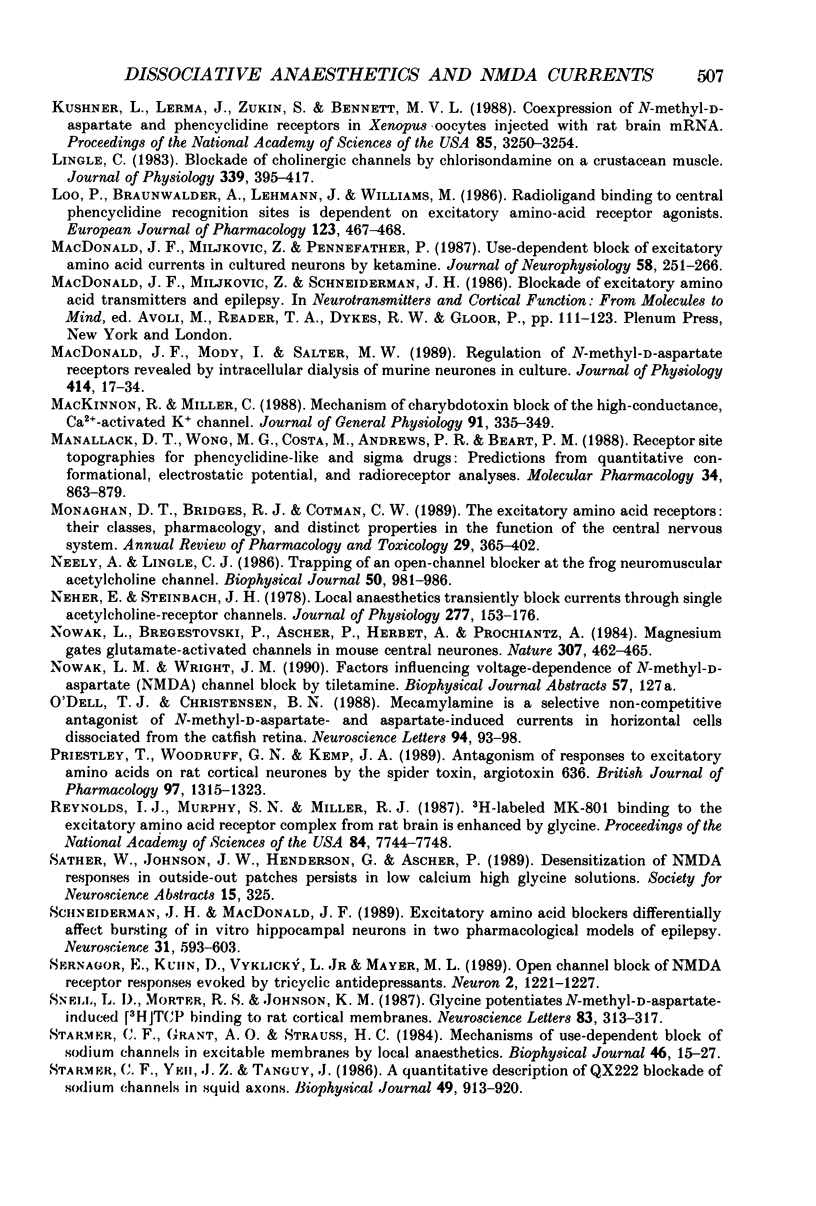
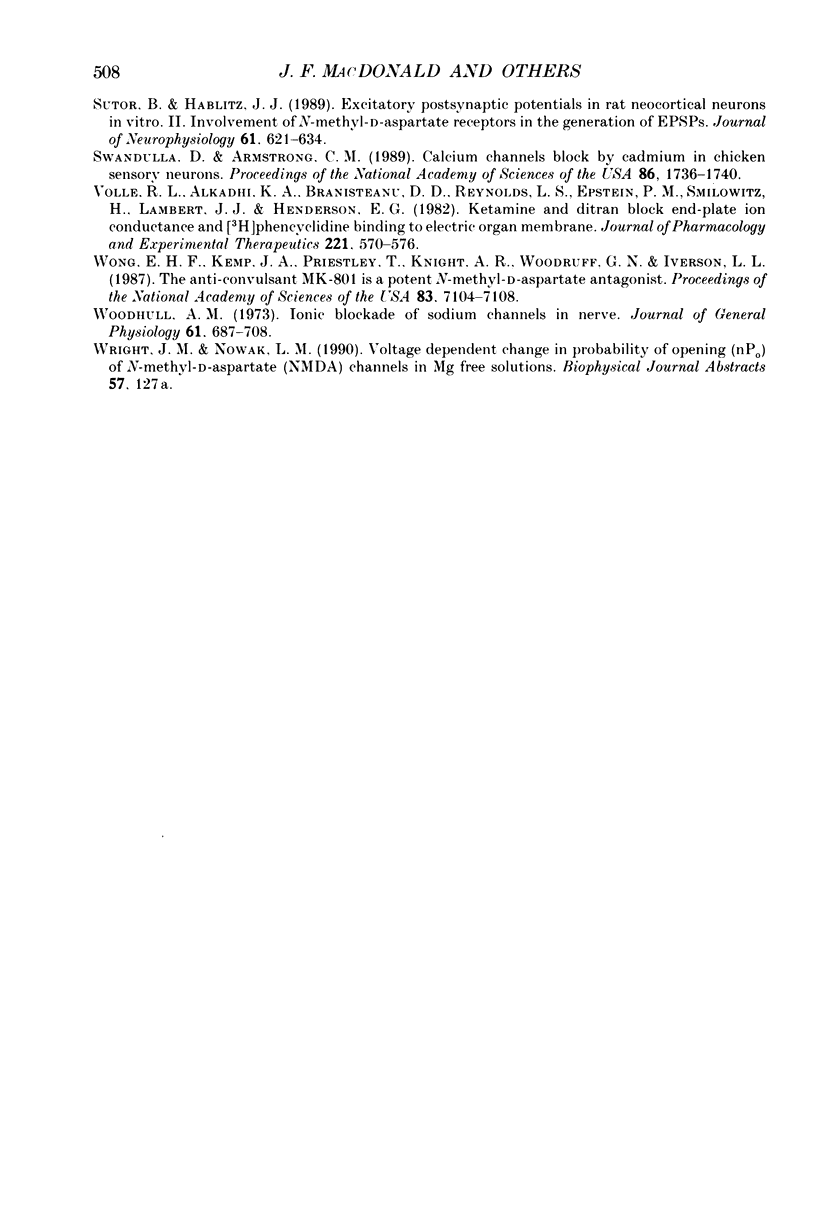
Selected References
These references are in PubMed. This may not be the complete list of references from this article.
- Aguayo L. G., Albuquerque E. X. Effects of phencyclidine and its analogs on the end-plate current of the neuromuscular junction. J Pharmacol Exp Ther. 1986 Oct;239(1):15–24. [PubMed] [Google Scholar]
- Anis N. A., Berry S. C., Burton N. R., Lodge D. The dissociative anaesthetics, ketamine and phencyclidine, selectively reduce excitation of central mammalian neurones by N-methyl-aspartate. Br J Pharmacol. 1983 Jun;79(2):565–575. doi: 10.1111/j.1476-5381.1983.tb11031.x. [DOI] [PMC free article] [PubMed] [Google Scholar]
- Armstrong C. M., Swenson R. P., Jr, Taylor S. R. Block of squid axon K channels by internally and externally applied barium ions. J Gen Physiol. 1982 Nov;80(5):663–682. doi: 10.1085/jgp.80.5.663. [DOI] [PMC free article] [PubMed] [Google Scholar]
- Ascher P., Nowak L. The role of divalent cations in the N-methyl-D-aspartate responses of mouse central neurones in culture. J Physiol. 1988 May;399:247–266. doi: 10.1113/jphysiol.1988.sp017078. [DOI] [PMC free article] [PubMed] [Google Scholar]
- Bertolino M., Vicini S. Voltage-dependent block by strychnine of N-methyl-D-aspartic acid-activated cationic channels in rat cortical neurons in culture. Mol Pharmacol. 1988 Aug;34(2):98–103. [PubMed] [Google Scholar]
- Carmeliet E., Nilius B., Vereecke J. Properties of the block of single Na+ channels in guinea-pig ventricular myocytes by the local anaesthetic penticainide. J Physiol. 1989 Feb;409:241–262. doi: 10.1113/jphysiol.1989.sp017495. [DOI] [PMC free article] [PubMed] [Google Scholar]
- Christine C. W., Choi D. W. Effect of zinc on NMDA receptor-mediated channel currents in cortical neurons. J Neurosci. 1990 Jan;10(1):108–116. doi: 10.1523/JNEUROSCI.10-01-00108.1990. [DOI] [PMC free article] [PubMed] [Google Scholar]
- Foster A. C., Wong E. H. The novel anticonvulsant MK-801 binds to the activated state of the N-methyl-D-aspartate receptor in rat brain. Br J Pharmacol. 1987 Jun;91(2):403–409. doi: 10.1111/j.1476-5381.1987.tb10295.x. [DOI] [PMC free article] [PubMed] [Google Scholar]
- Gurney A. M., Rang H. P. The channel-blocking action of methonium compounds on rat submandibular ganglion cells. Br J Pharmacol. 1984 Jul;82(3):623–642. doi: 10.1111/j.1476-5381.1984.tb10801.x. [DOI] [PMC free article] [PubMed] [Google Scholar]
- Halliwell R. F., Peters J. A., Lambert J. J. The mechanism of action and pharmacological specificity of the anticonvulsant NMDA antagonist MK-801: a voltage clamp study on neuronal cells in culture. Br J Pharmacol. 1989 Feb;96(2):480–494. doi: 10.1111/j.1476-5381.1989.tb11841.x. [DOI] [PMC free article] [PubMed] [Google Scholar]
- Honey C. R., Miljkovic Z., MacDonald J. F. Ketamine and phencyclidine cause a voltage-dependent block of responses to L-aspartic acid. Neurosci Lett. 1985 Oct 24;61(1-2):135–139. doi: 10.1016/0304-3940(85)90414-8. [DOI] [PubMed] [Google Scholar]
- Huettner J. E., Bean B. P. Block of N-methyl-D-aspartate-activated current by the anticonvulsant MK-801: selective binding to open channels. Proc Natl Acad Sci U S A. 1988 Feb;85(4):1307–1311. doi: 10.1073/pnas.85.4.1307. [DOI] [PMC free article] [PubMed] [Google Scholar]
- Jackson M. B. Spontaneous openings of the acetylcholine receptor channel. Proc Natl Acad Sci U S A. 1984 Jun;81(12):3901–3904. doi: 10.1073/pnas.81.12.3901. [DOI] [PMC free article] [PubMed] [Google Scholar]
- Karschin A., Aizenman E., Lipton S. A. The interaction of agonists and noncompetitive antagonists at the excitatory amino acid receptors in rat retinal ganglion cells in vitro. J Neurosci. 1988 Aug;8(8):2895–2906. doi: 10.1523/JNEUROSCI.08-08-02895.1988. [DOI] [PMC free article] [PubMed] [Google Scholar]
- Keana J. F., McBurney R. N., Scherz M. W., Fischer J. B., Hamilton P. N., Smith S. M., Server A. C., Finkbeiner S., Stevens C. F., Jahr C. Synthesis and characterization of a series of diarylguanidines that are noncompetitive N-methyl-D-aspartate receptor antagonists with neuroprotective properties. Proc Natl Acad Sci U S A. 1989 Jul;86(14):5631–5635. doi: 10.1073/pnas.86.14.5631. [DOI] [PMC free article] [PubMed] [Google Scholar]
- Keana J. F., Scherz M. W., Quarum M., Sonders M. S., Weber E. Synthesis and characterization of a radiolabelled derivative of the phencyclidine/N-methyl-D-aspartate receptor ligand (+) MK-801 with high specific radioactivity. Life Sci. 1988;43(12):965–973. doi: 10.1016/0024-3205(88)90541-3. [DOI] [PubMed] [Google Scholar]
- Kloog Y., Haring R., Sokolovsky M. Kinetic characterization of the phencyclidine-N-methyl-D-aspartate receptor interaction: evidence for a steric blockade of the channel. Biochemistry. 1988 Feb 9;27(3):843–848. doi: 10.1021/bi00403a001. [DOI] [PubMed] [Google Scholar]
- Kushner L., Lerma J., Zukin R. S., Bennett M. V. Coexpression of N-methyl-D-aspartate and phencyclidine receptors in Xenopus oocytes injected with rat brain mRNA. Proc Natl Acad Sci U S A. 1988 May;85(9):3250–3254. doi: 10.1073/pnas.85.9.3250. [DOI] [PMC free article] [PubMed] [Google Scholar]
- Lingle C. Blockade of cholinergic channels by chlorisondamine on a crustacean muscle. J Physiol. 1983 Jun;339:395–417. doi: 10.1113/jphysiol.1983.sp014723. [DOI] [PMC free article] [PubMed] [Google Scholar]
- Loo P., Braunwalder A., Lehmann J., Williams M. Radioligand binding to central phencyclidine recognition sites is dependent on excitatory amino acid receptor agonists. Eur J Pharmacol. 1986 Apr 29;123(3):467–468. doi: 10.1016/0014-2999(86)90726-0. [DOI] [PubMed] [Google Scholar]
- MacDonald J. F., Miljkovic Z., Pennefather P. Use-dependent block of excitatory amino acid currents in cultured neurons by ketamine. J Neurophysiol. 1987 Aug;58(2):251–266. doi: 10.1152/jn.1987.58.2.251. [DOI] [PubMed] [Google Scholar]
- MacDonald J. F., Mody I., Salter M. W. Regulation of N-methyl-D-aspartate receptors revealed by intracellular dialysis of murine neurones in culture. J Physiol. 1989 Jul;414:17–34. doi: 10.1113/jphysiol.1989.sp017674. [DOI] [PMC free article] [PubMed] [Google Scholar]
- MacKinnon R., Miller C. Mechanism of charybdotoxin block of the high-conductance, Ca2+-activated K+ channel. J Gen Physiol. 1988 Mar;91(3):335–349. doi: 10.1085/jgp.91.3.335. [DOI] [PMC free article] [PubMed] [Google Scholar]
- Manallack D. T., Wong M. G., Costa M., Andrews P. R., Beart P. M. Receptor site topographies for phencyclidine-like and sigma drugs: predictions from quantitative conformational, electrostatic potential, and radioreceptor analyses. Mol Pharmacol. 1988 Dec;34(6):863–879. [PubMed] [Google Scholar]
- Monaghan D. T., Bridges R. J., Cotman C. W. The excitatory amino acid receptors: their classes, pharmacology, and distinct properties in the function of the central nervous system. Annu Rev Pharmacol Toxicol. 1989;29:365–402. doi: 10.1146/annurev.pa.29.040189.002053. [DOI] [PubMed] [Google Scholar]
- Neely A., Lingle C. J. Trapping of an open-channel blocker at the frog neuromuscular acetylcholine channel. Biophys J. 1986 Nov;50(5):981–986. doi: 10.1016/S0006-3495(86)83538-X. [DOI] [PMC free article] [PubMed] [Google Scholar]
- Neher E., Steinbach J. H. Local anaesthetics transiently block currents through single acetylcholine-receptor channels. J Physiol. 1978 Apr;277:153–176. doi: 10.1113/jphysiol.1978.sp012267. [DOI] [PMC free article] [PubMed] [Google Scholar]
- Nowak L., Bregestovski P., Ascher P., Herbet A., Prochiantz A. Magnesium gates glutamate-activated channels in mouse central neurones. Nature. 1984 Feb 2;307(5950):462–465. doi: 10.1038/307462a0. [DOI] [PubMed] [Google Scholar]
- O'Dell T. J., Christensen B. N. Mecamylamine is a selective non-competitive antagonist of N-methyl-D-aspartate- and aspartate-induced currents in horizontal cells dissociated from the catfish retina. Neurosci Lett. 1988 Nov 22;94(1-2):93–98. doi: 10.1016/0304-3940(88)90276-5. [DOI] [PubMed] [Google Scholar]
- Priestley T., Woodruff G. N., Kemp J. A. Antagonism of responses to excitatory amino acids on rat cortical neurones by the spider toxin, argiotoxin636. Br J Pharmacol. 1989 Aug;97(4):1315–1323. doi: 10.1111/j.1476-5381.1989.tb12594.x. [DOI] [PMC free article] [PubMed] [Google Scholar]
- Reynolds I. J., Murphy S. N., Miller R. J. 3H-labeled MK-801 binding to the excitatory amino acid receptor complex from rat brain is enhanced by glycine. Proc Natl Acad Sci U S A. 1987 Nov;84(21):7744–7748. doi: 10.1073/pnas.84.21.7744. [DOI] [PMC free article] [PubMed] [Google Scholar]
- Schneiderman J. H., MacDonald J. F. Excitatory amino acid blockers differentially affect bursting of in vitro hippocampal neurons in two pharmacological models of epilepsy. Neuroscience. 1989;31(3):593–603. doi: 10.1016/0306-4522(89)90425-9. [DOI] [PubMed] [Google Scholar]
- Sernagor E., Kuhn D., Vyklicky L., Jr, Mayer M. L. Open channel block of NMDA receptor responses evoked by tricyclic antidepressants. Neuron. 1989 Mar;2(3):1221–1227. doi: 10.1016/0896-6273(89)90306-1. [DOI] [PubMed] [Google Scholar]
- Snell L. D., Morter R. S., Johnson K. M. Glycine potentiates N-methyl-D-aspartate-induced [3H]TCP binding to rat cortical membranes. Neurosci Lett. 1987 Dec 29;83(3):313–317. doi: 10.1016/0304-3940(87)90106-6. [DOI] [PubMed] [Google Scholar]
- Starmer C. F., Grant A. O., Strauss H. C. Mechanisms of use-dependent block of sodium channels in excitable membranes by local anesthetics. Biophys J. 1984 Jul;46(1):15–27. doi: 10.1016/S0006-3495(84)83994-6. [DOI] [PMC free article] [PubMed] [Google Scholar]
- Starmer C. F., Yeh J. Z., Tanguy J. A quantitative description of QX222 blockade of sodium channels in squid axons. Biophys J. 1986 Apr;49(4):913–920. doi: 10.1016/S0006-3495(86)83719-5. [DOI] [PMC free article] [PubMed] [Google Scholar]
- Sutor B., Hablitz J. J. EPSPs in rat neocortical neurons in vitro. II. Involvement of N-methyl-D-aspartate receptors in the generation of EPSPs. J Neurophysiol. 1989 Mar;61(3):621–634. doi: 10.1152/jn.1989.61.3.621. [DOI] [PubMed] [Google Scholar]
- Swandulla D., Armstrong C. M. Calcium channel block by cadmium in chicken sensory neurons. Proc Natl Acad Sci U S A. 1989 Mar;86(5):1736–1740. doi: 10.1073/pnas.86.5.1736. [DOI] [PMC free article] [PubMed] [Google Scholar]
- Volle R. L., Alkadhi K. A., Branisteanu D. D., Reynolds L. S., Epstein P. M., Smilowitz H., Lambert J. J., Henderson E. G. Ketamine and ditran block end-plate ion conductance and [3H]phencyclidine binding to electric organ membrane. J Pharmacol Exp Ther. 1982 Jun;221(3):570–576. [PubMed] [Google Scholar]
- Wong E. H., Kemp J. A., Priestley T., Knight A. R., Woodruff G. N., Iversen L. L. The anticonvulsant MK-801 is a potent N-methyl-D-aspartate antagonist. Proc Natl Acad Sci U S A. 1986 Sep;83(18):7104–7108. doi: 10.1073/pnas.83.18.7104. [DOI] [PMC free article] [PubMed] [Google Scholar]