Abstract
1. Single muscle fibres were dissected from the toe muscles of Xenopus laevis and microinjected with Fura-2 to measure myoplasmic calcium concentration ([Ca2+]i). Injected fibres were illuminated at 340 and 380 nm and the ratio of the resulting fluorescence at 505 nm (the Fura-2 ratio) was taken as a measure of [Ca2+]i. Fibres were fatigued at 21 degrees C by repeated tetani until developed tension had fallen to 50% of control. 2. Tetanic tension declined monotonically during fatiguing stimulation, whereas the tetanic Fura-2 ratio first increased and then declined. At the 10th tetanus, tension was 87% of control whereas the Fura-2 ratio was 106% of control. At the end of fatiguing stimulation, where tension was around 50% of control, the tetanic Fura-2 ratio was reduced to 71%. The rate of decline of both tension and the Fura-2 ratio after a tetanus slowed during fatigue. During recovery, the tension and the tetanic Fura-2 ratio recovered in parallel. 3. The resting Fura-2 ratio increased throughout fatigue reaching 237% of control when tension had declined to 50%. There was a rapid phase of recovery, complete within 1 min, by which time the resting Fura-2 ratio was 198% of control. Subsequent recovery was slower and took 20-30 min to reach a stable level which was 121% of control. 4. The resting Fura-2 ratio towards the end of fatiguing stimulation was greater than the tetanic Fura-2 ratio in the early part of recovery although there was no detectable increase of resting tension during fatiguing stimulation. This observation suggests that the Ca2+ sensitivity of the contractile proteins was reduced at the end of fatiguing stimulation. 5. Plots of the tetanic tension against tetanic Fura-2 ratios throughout fatiguing stimulation and recovery also suggested that Ca2+ sensitivity was reduced during fatiguing stimulation when compared to recovery. 6. The increases in resting [Ca2+]i caused by raised [K+]o (from 2.5 to 10 mM) and/or by application of 15% CO2 were much less than those produced by fatiguing stimulation. Much of the elevated [Ca2+]i in fatigue could be reversed by application of dantrolene (25 microM). 7. The results suggest that both reduced tetanic [Ca2+]i and reduced Ca2+ sensitivity contribute to the decline of tension during fatigue.(ABSTRACT TRUNCATED AT 400 WORDS)
Full text
PDF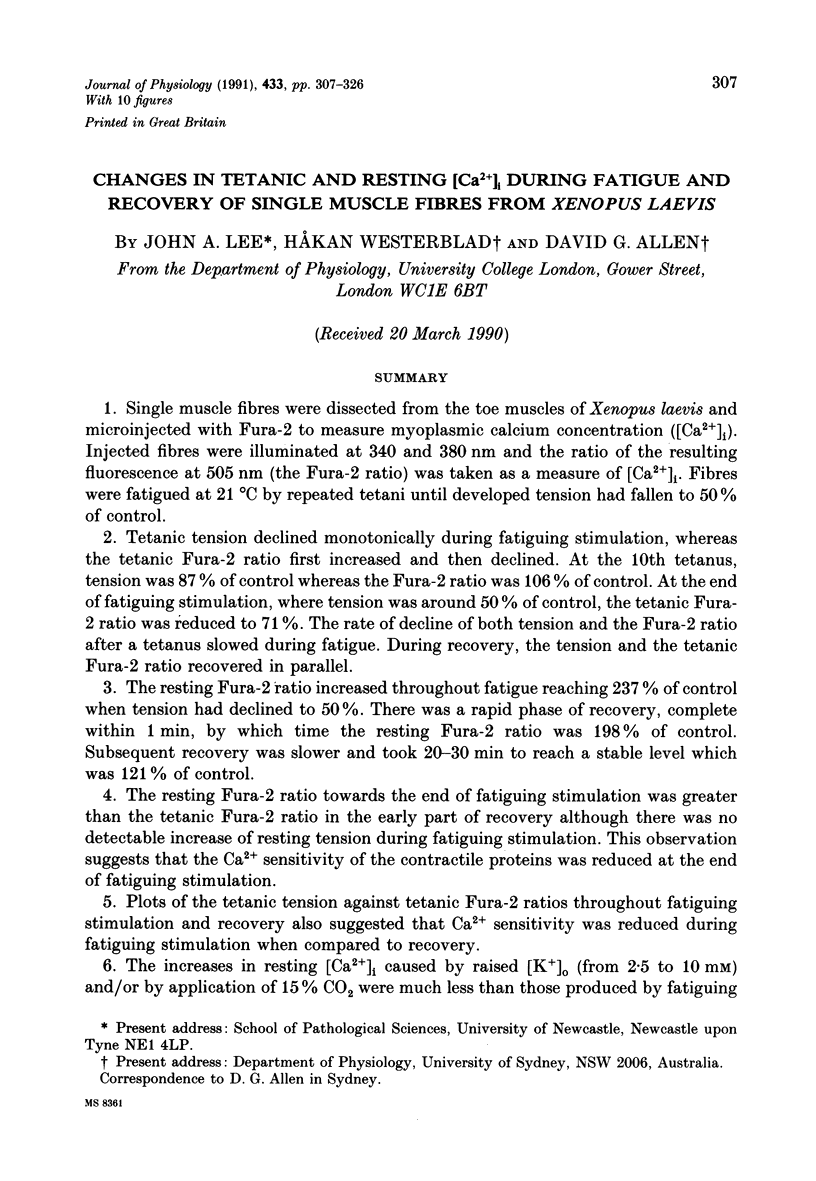
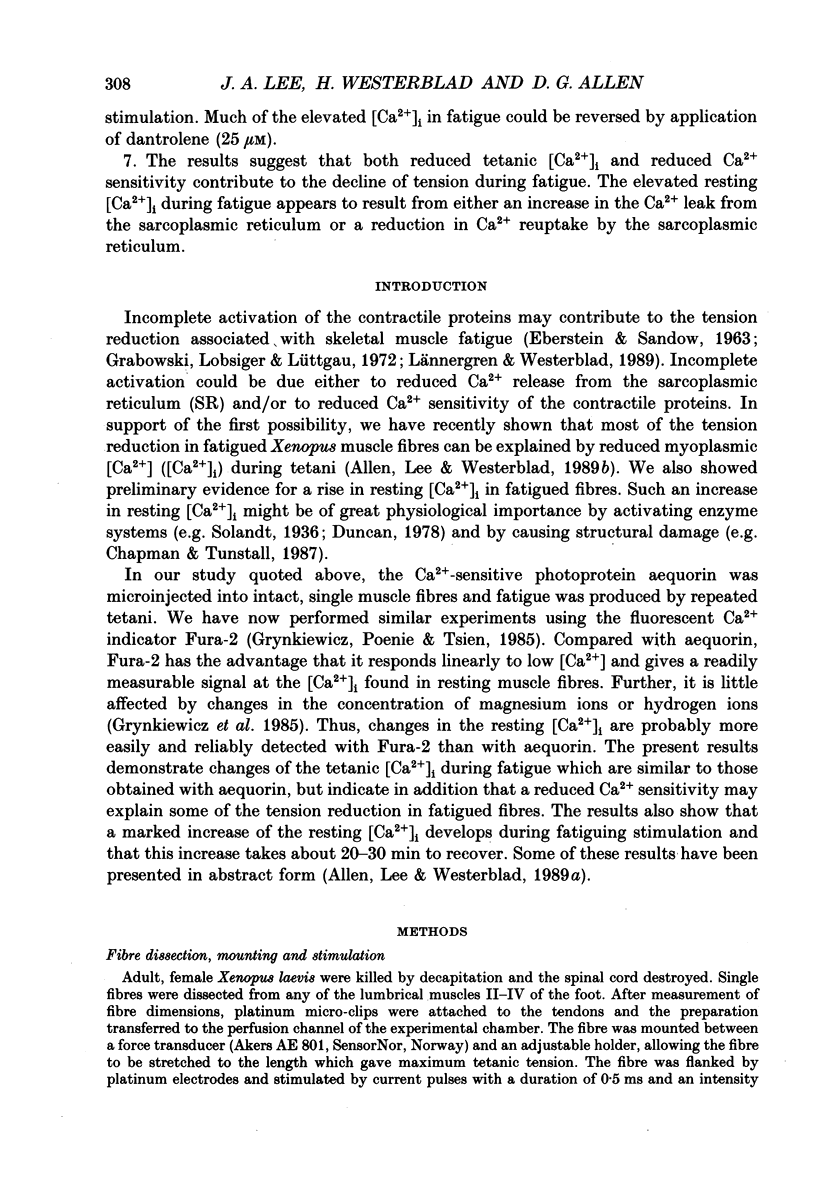
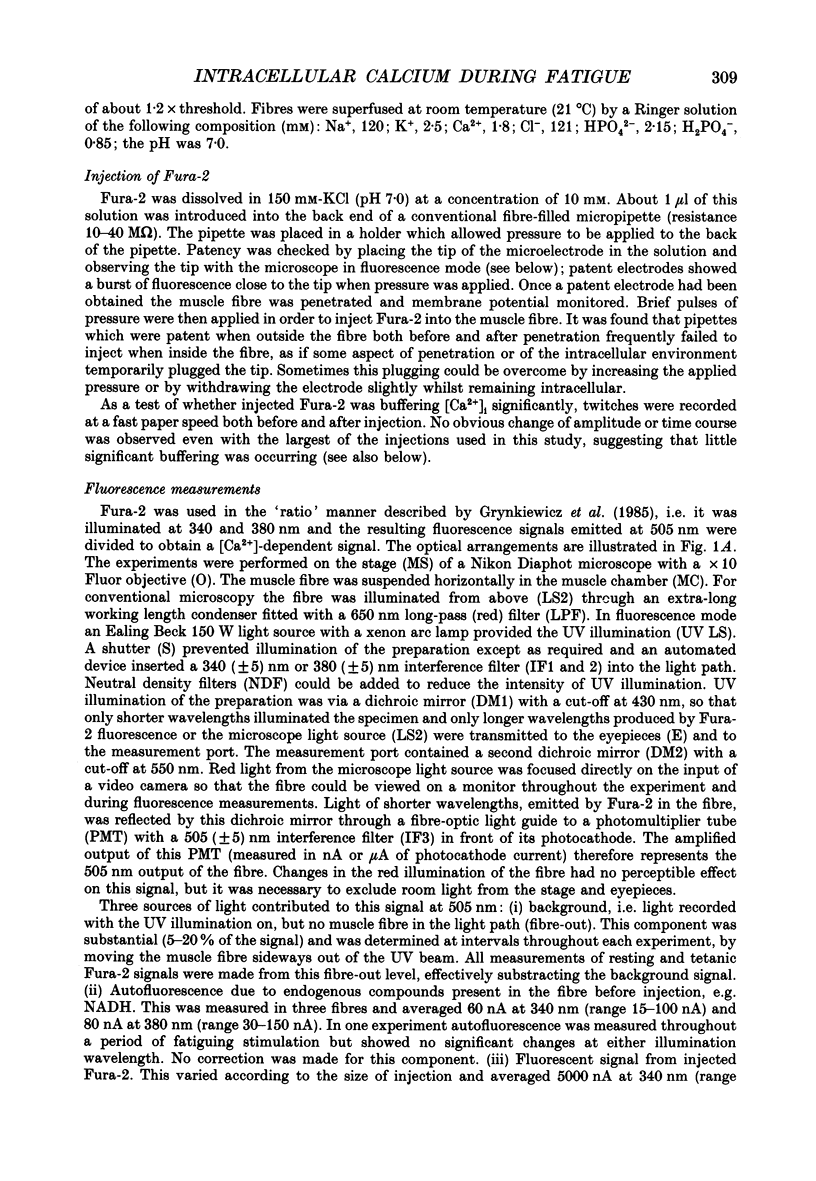
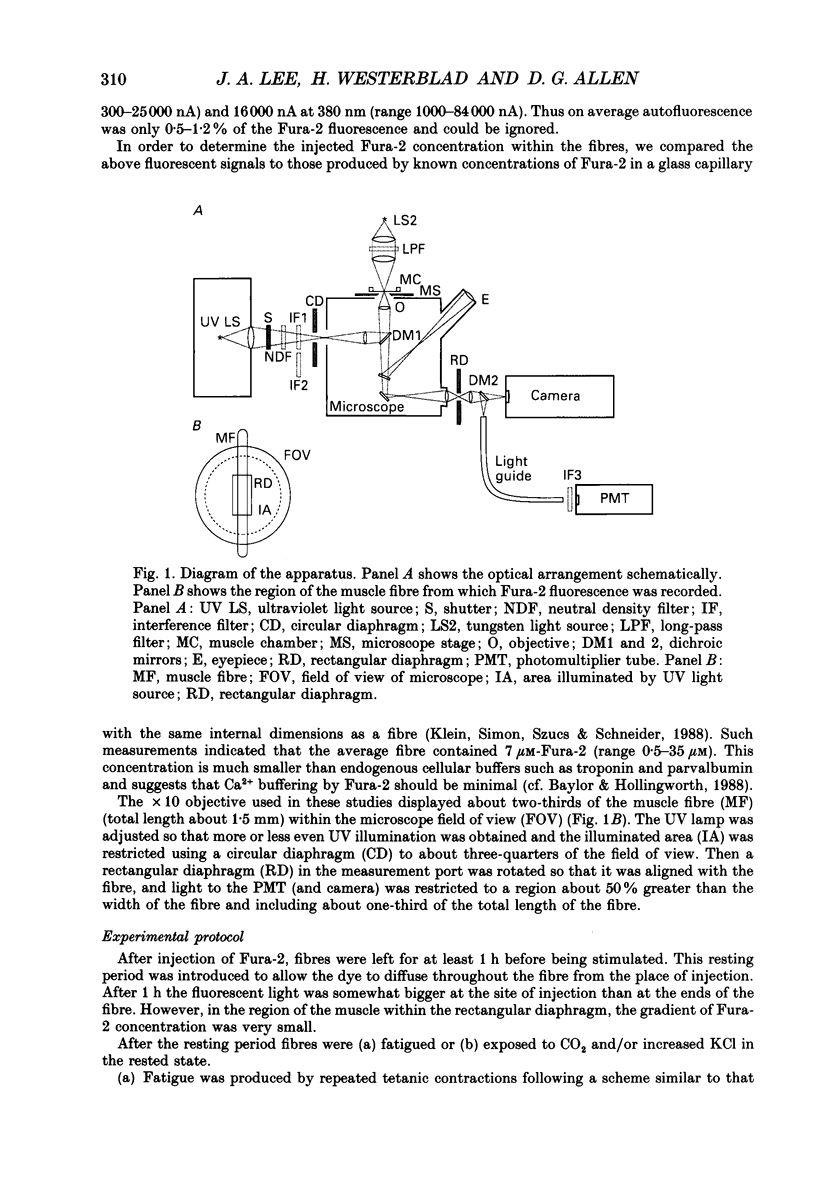
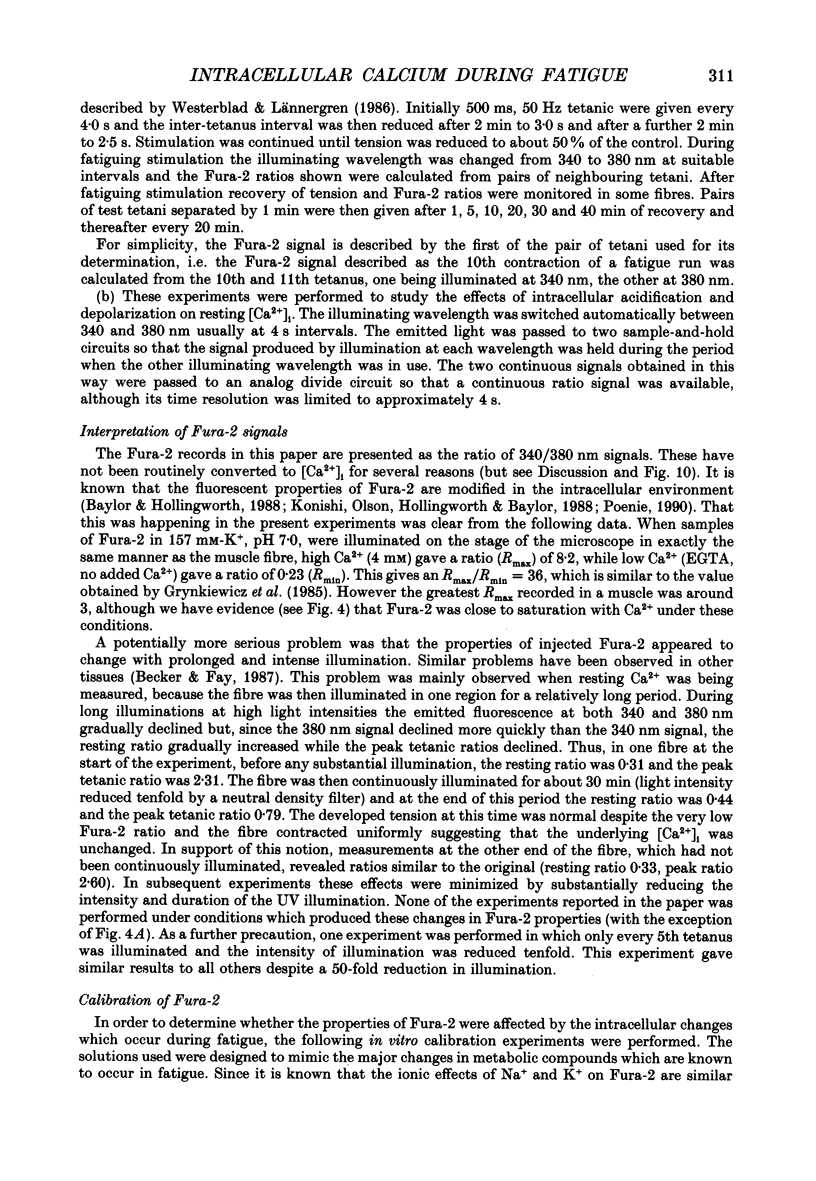
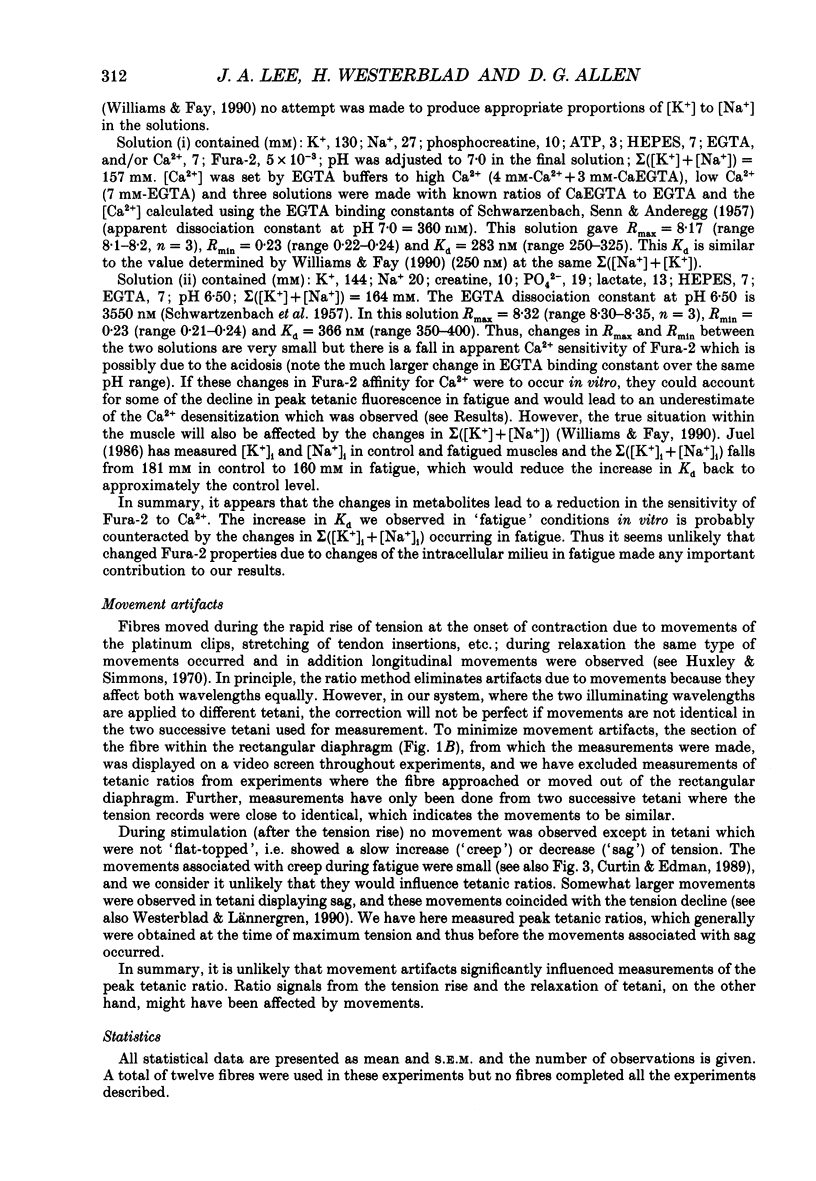
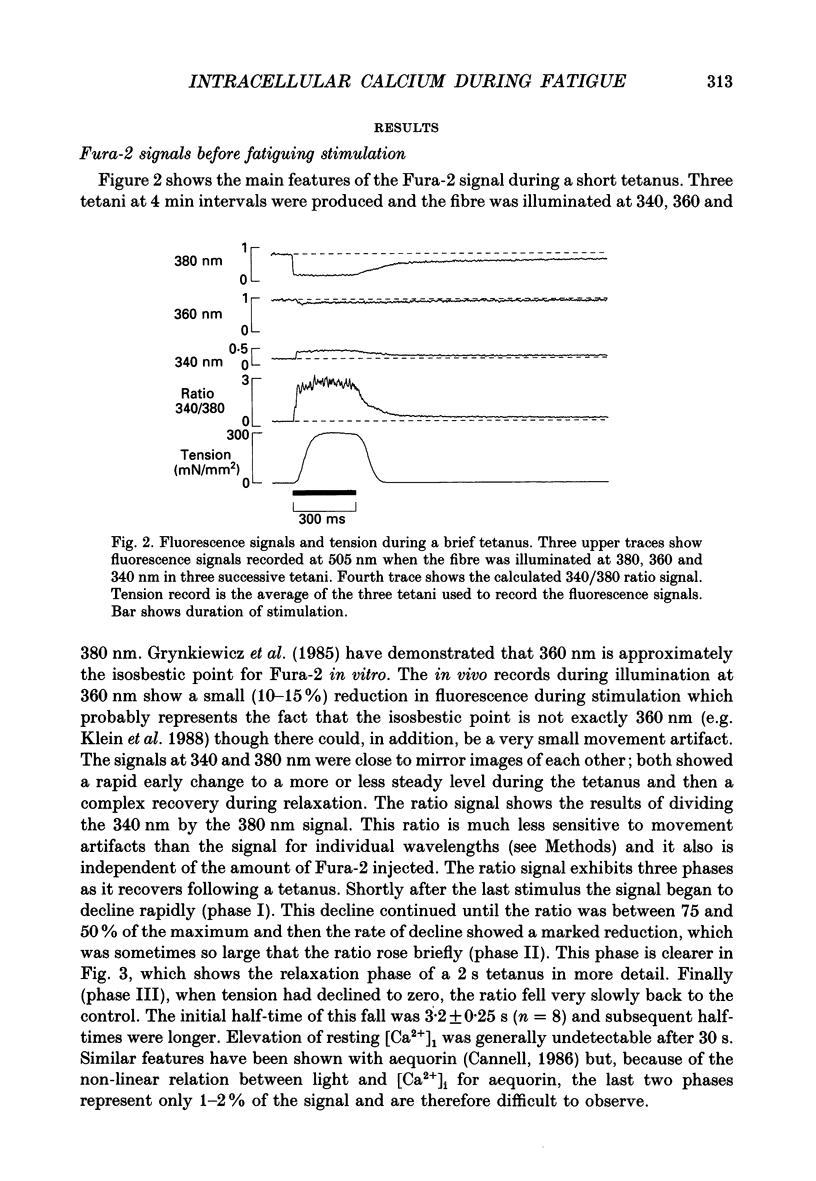
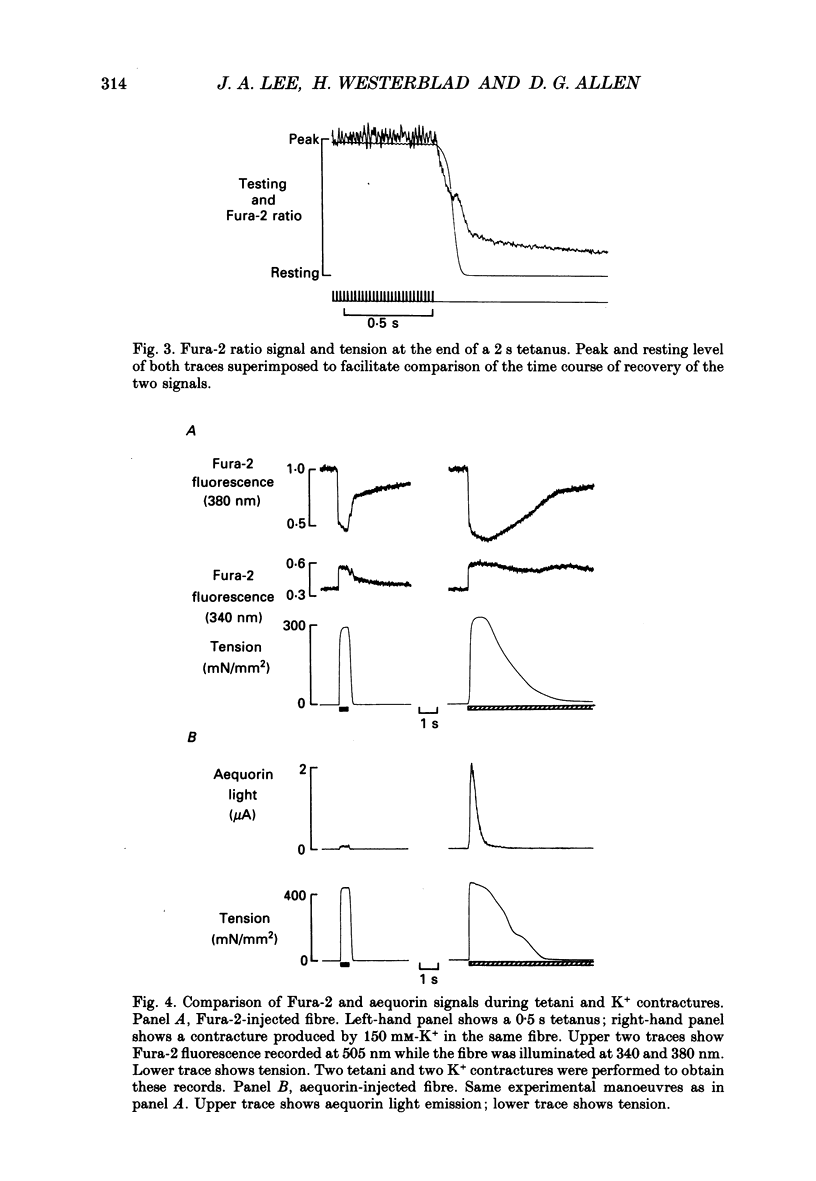
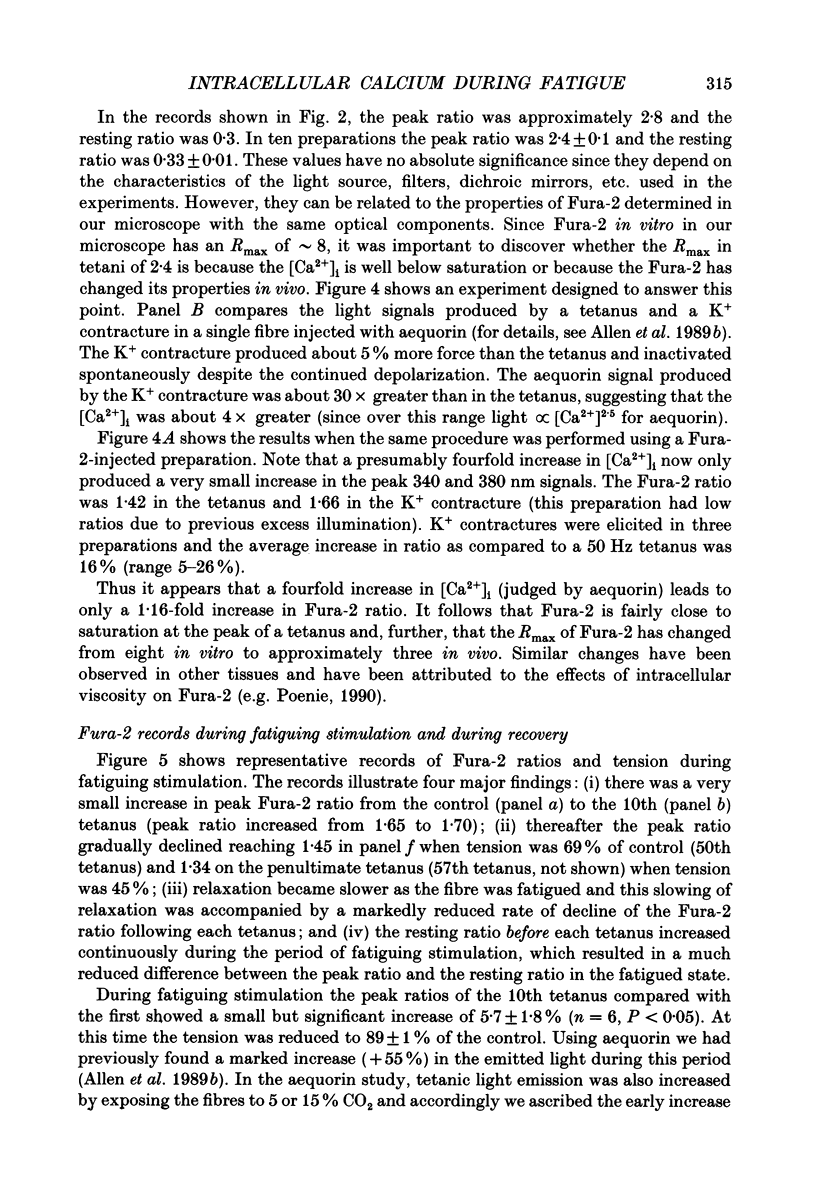
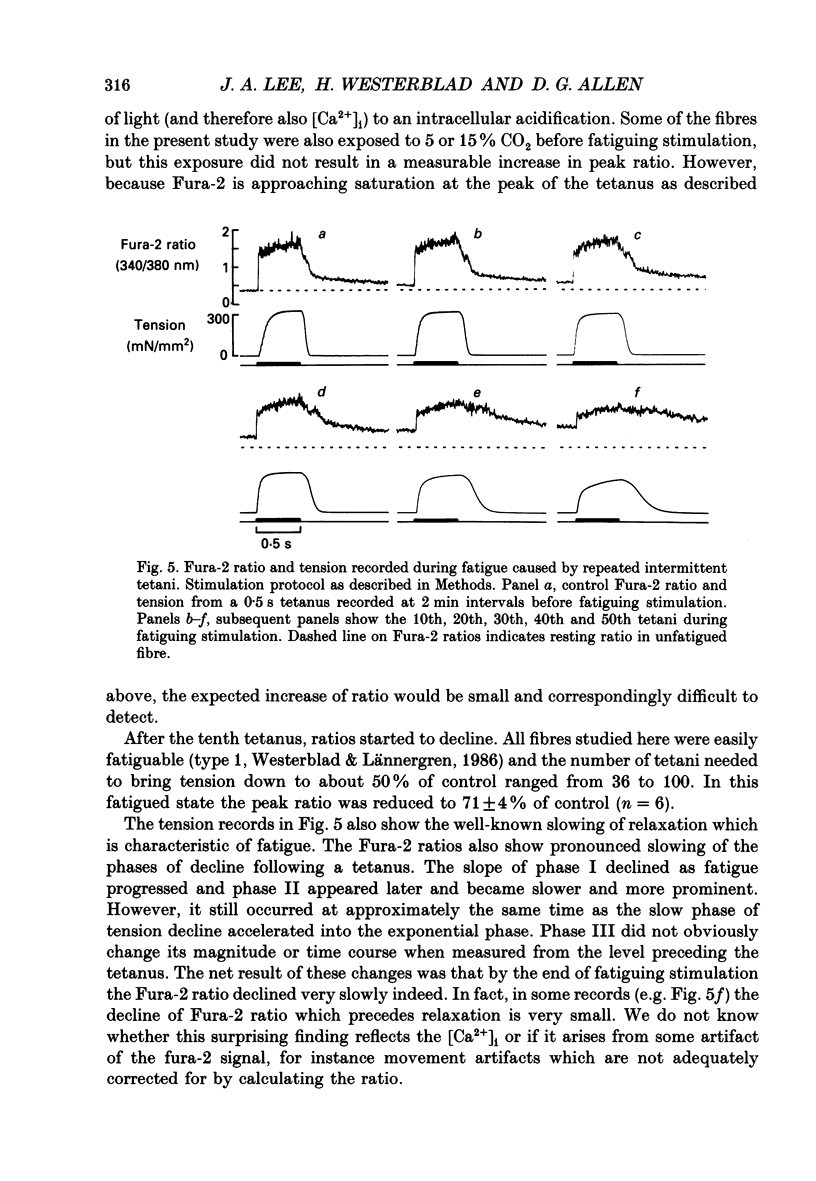
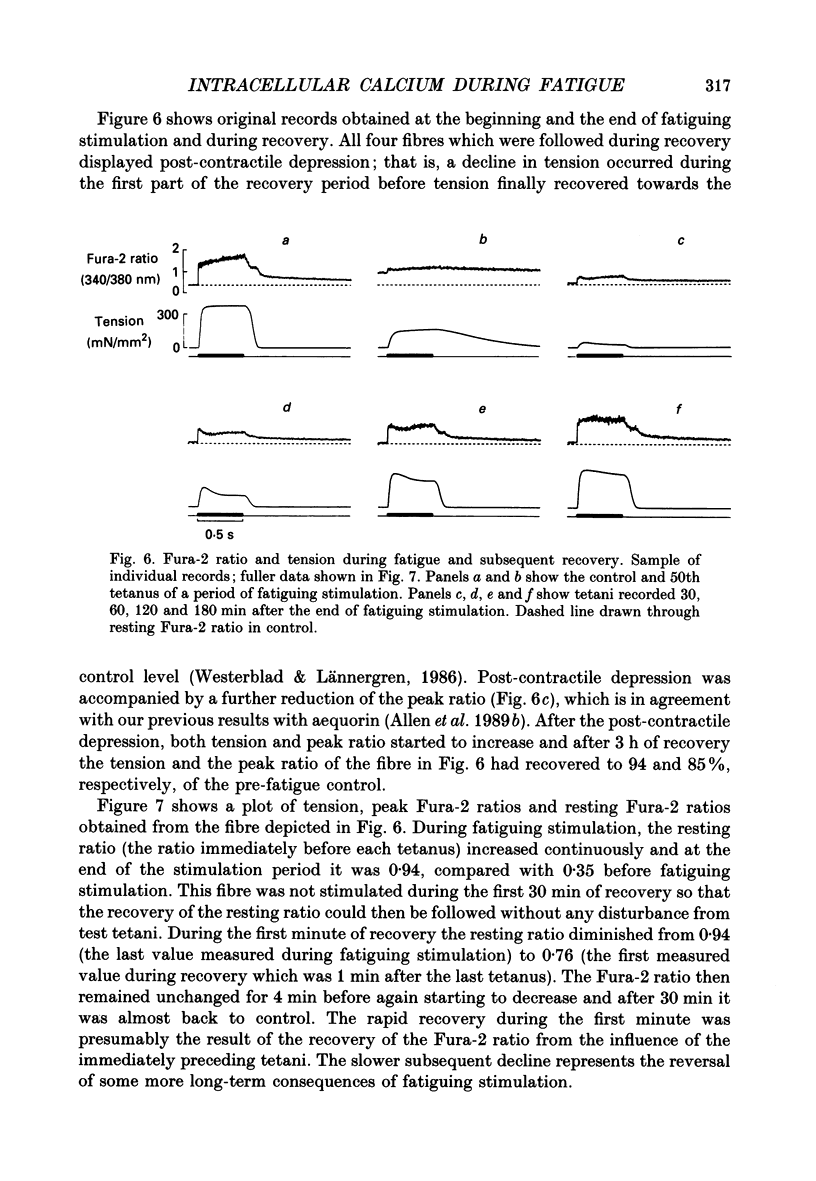
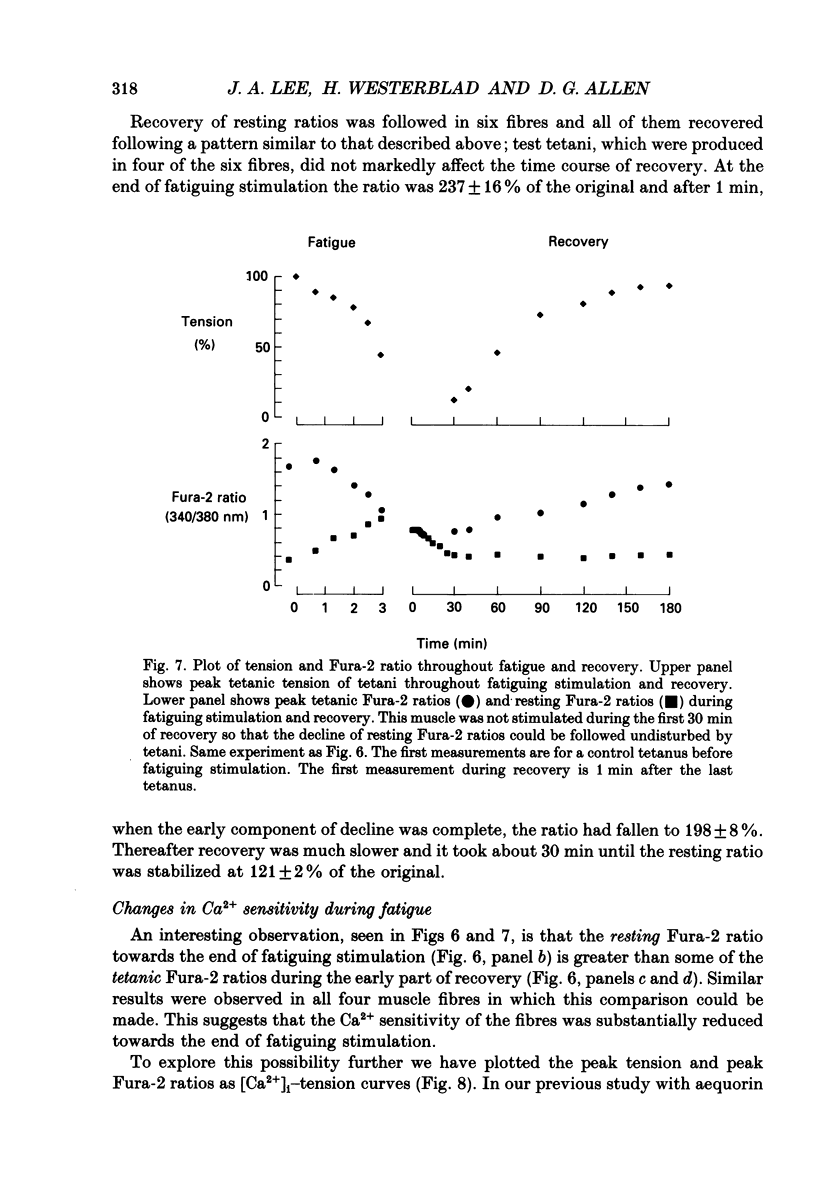
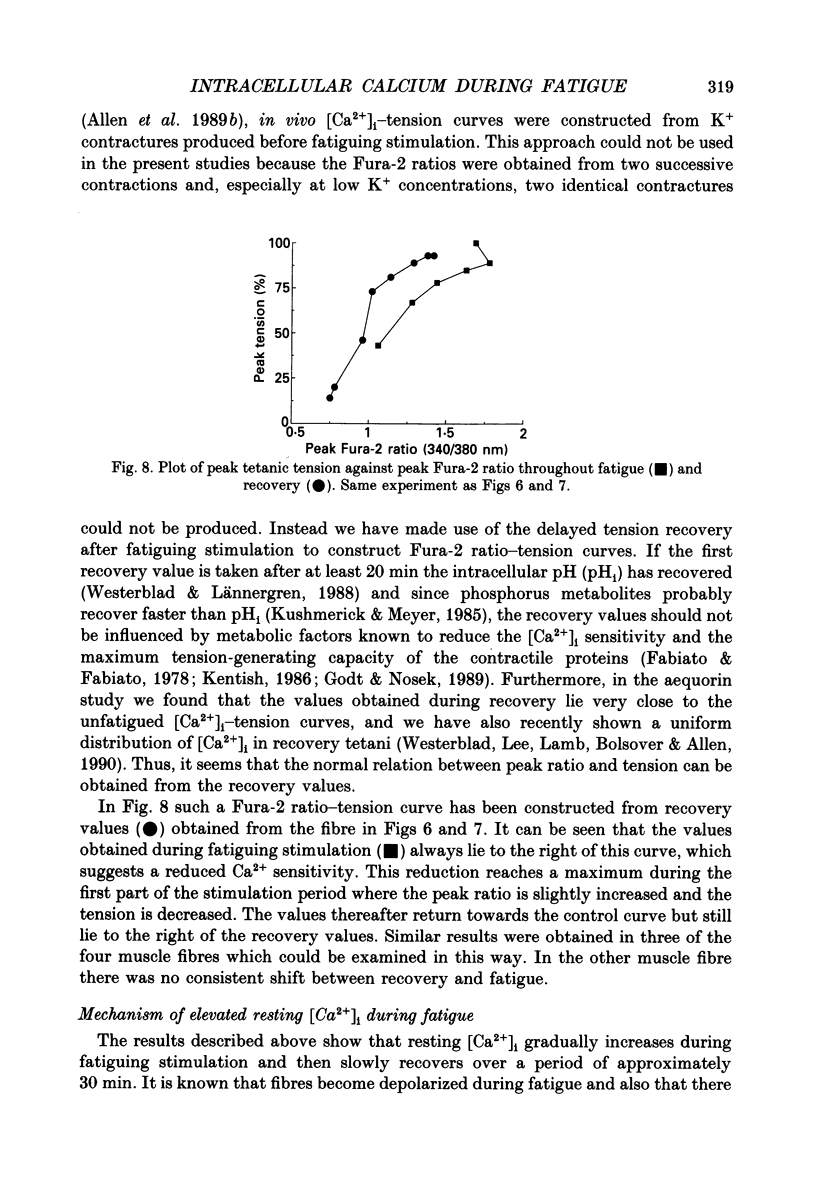
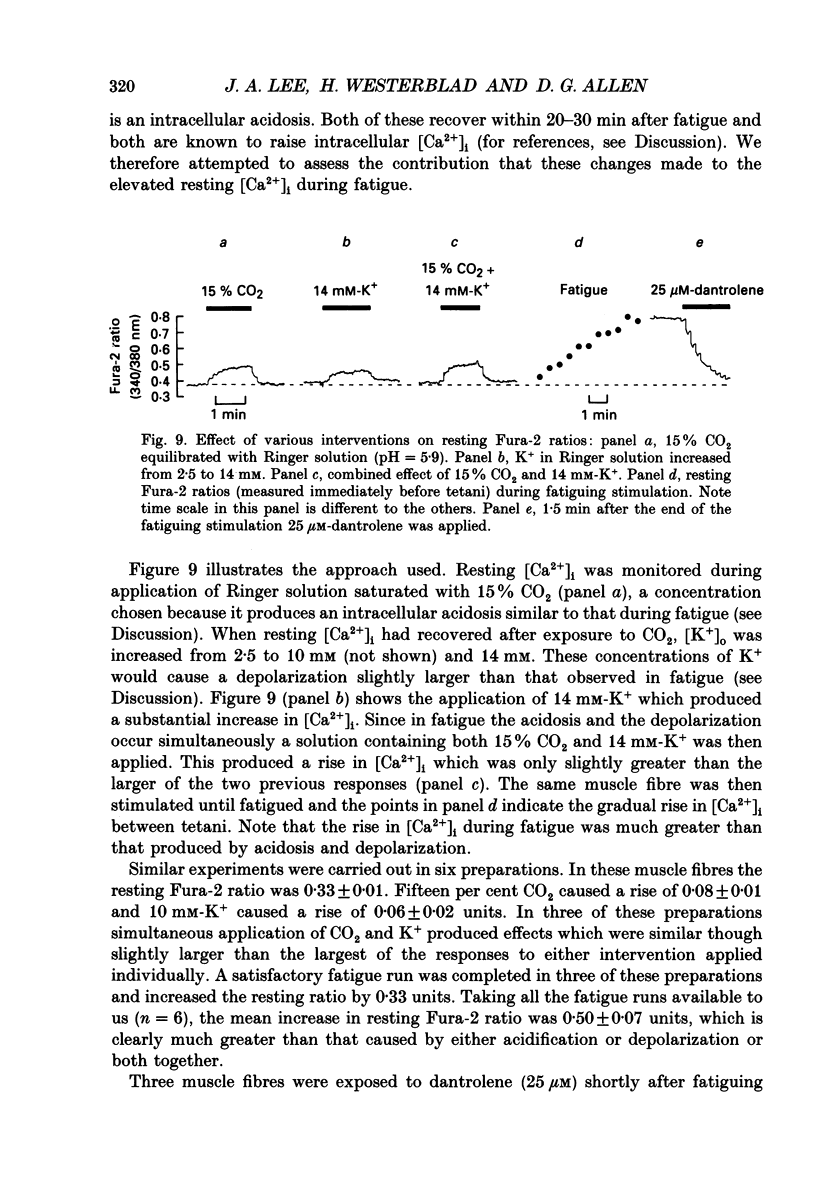
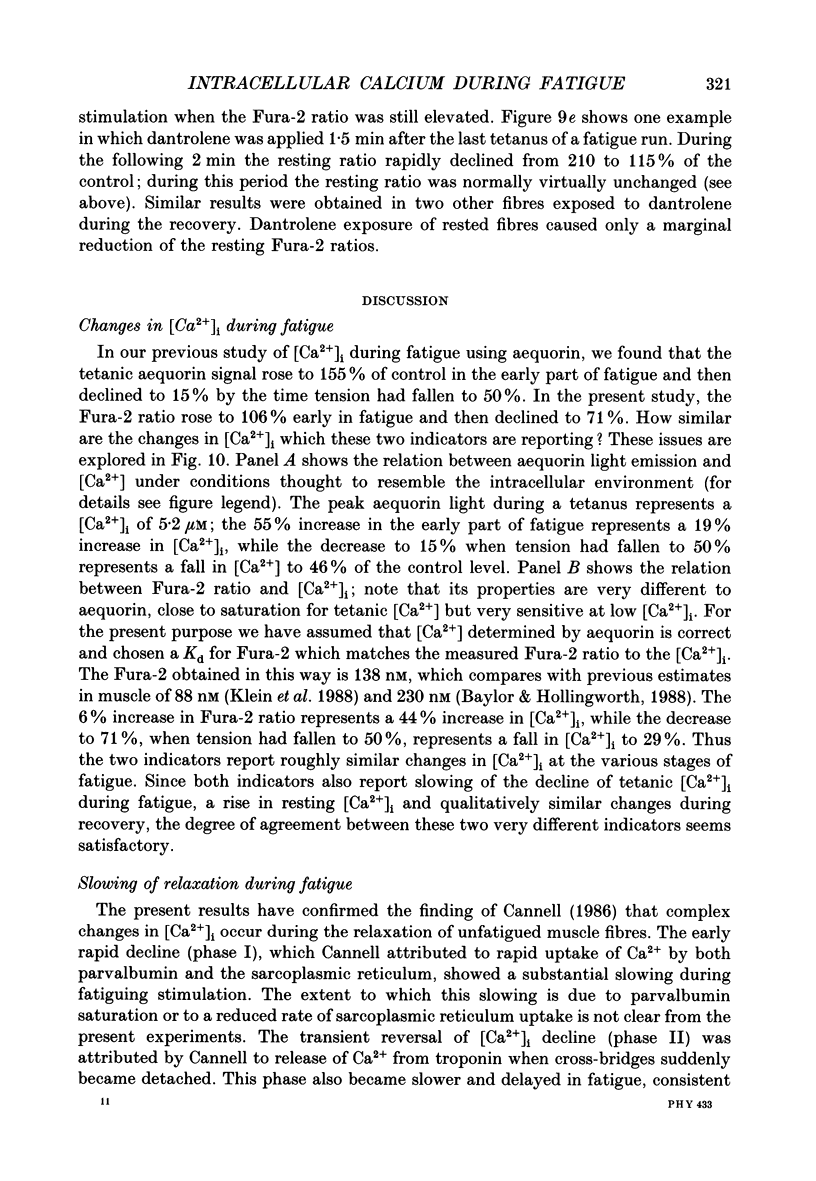
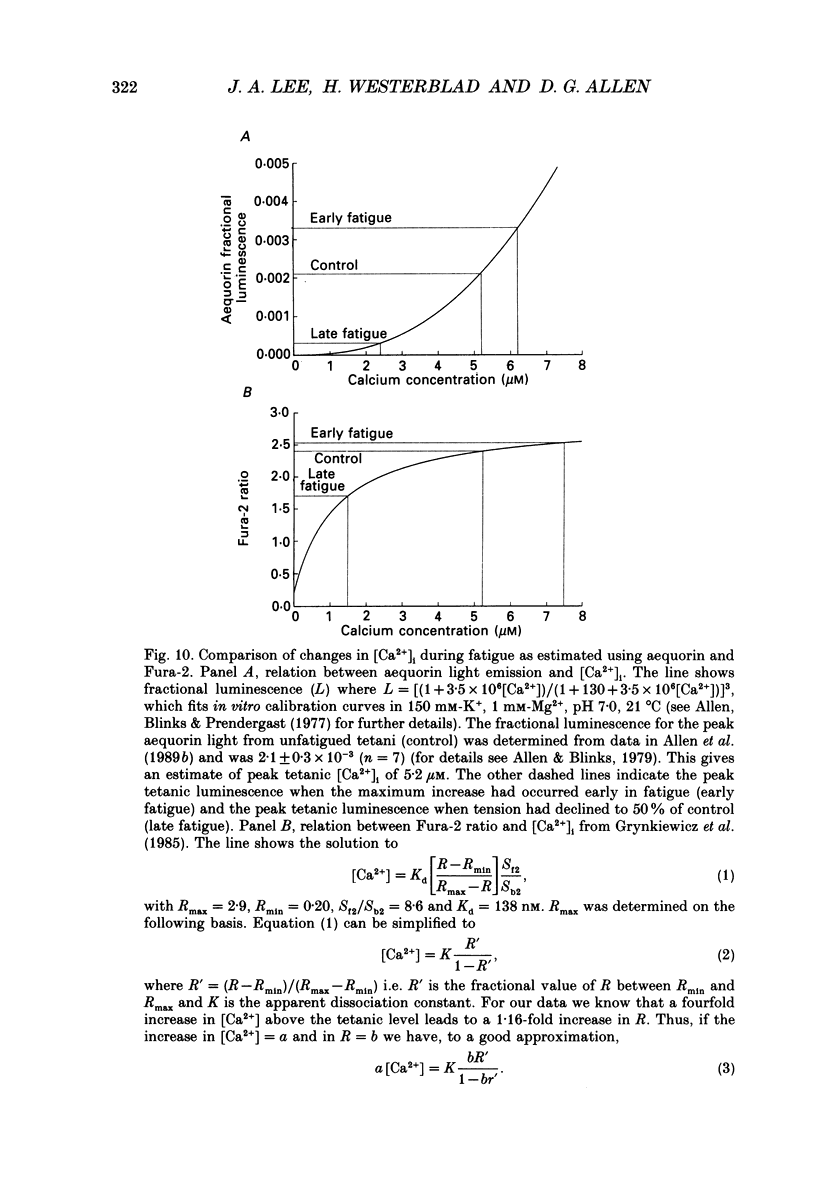
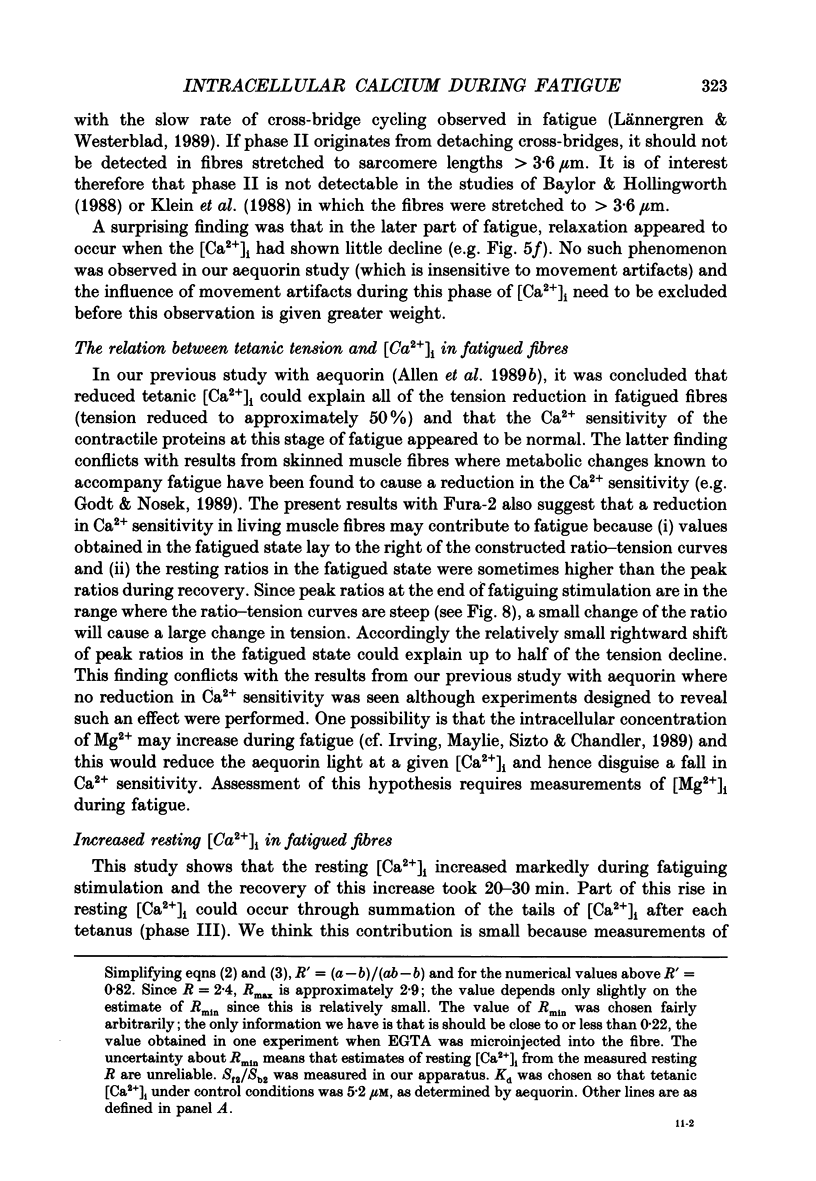
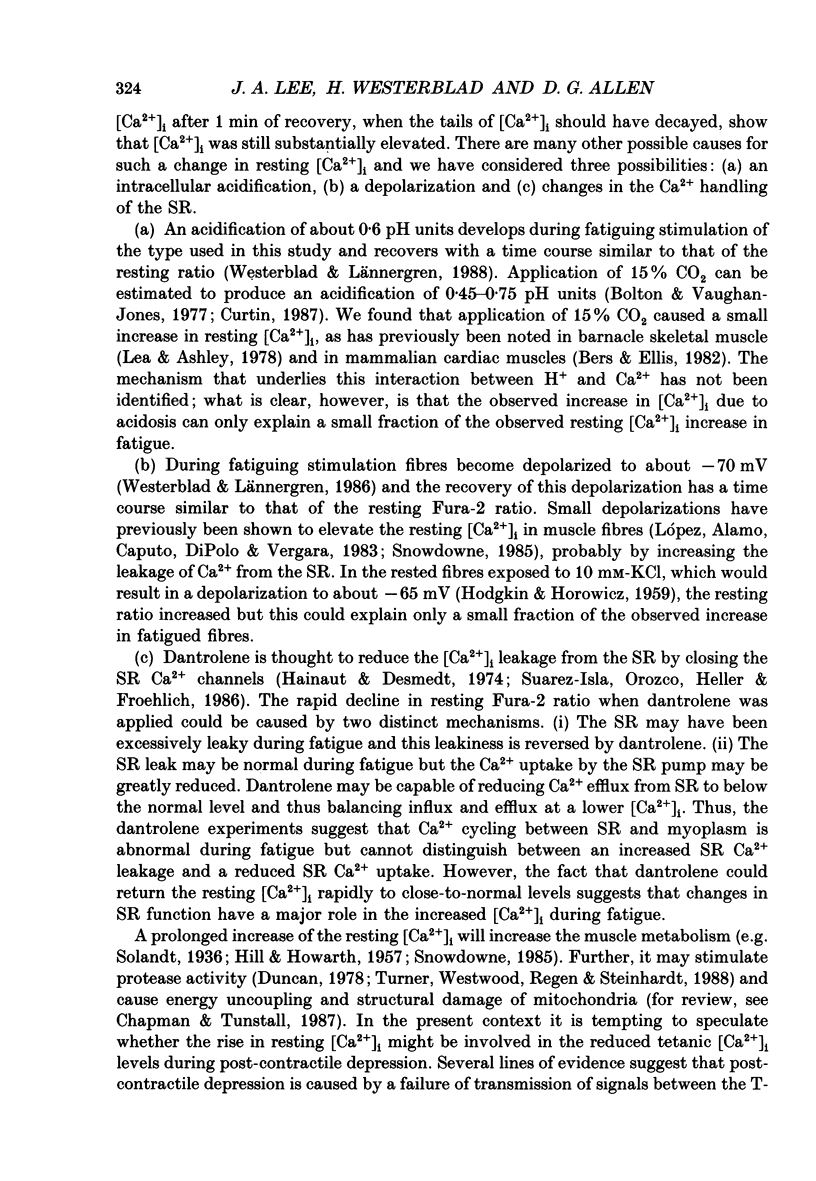
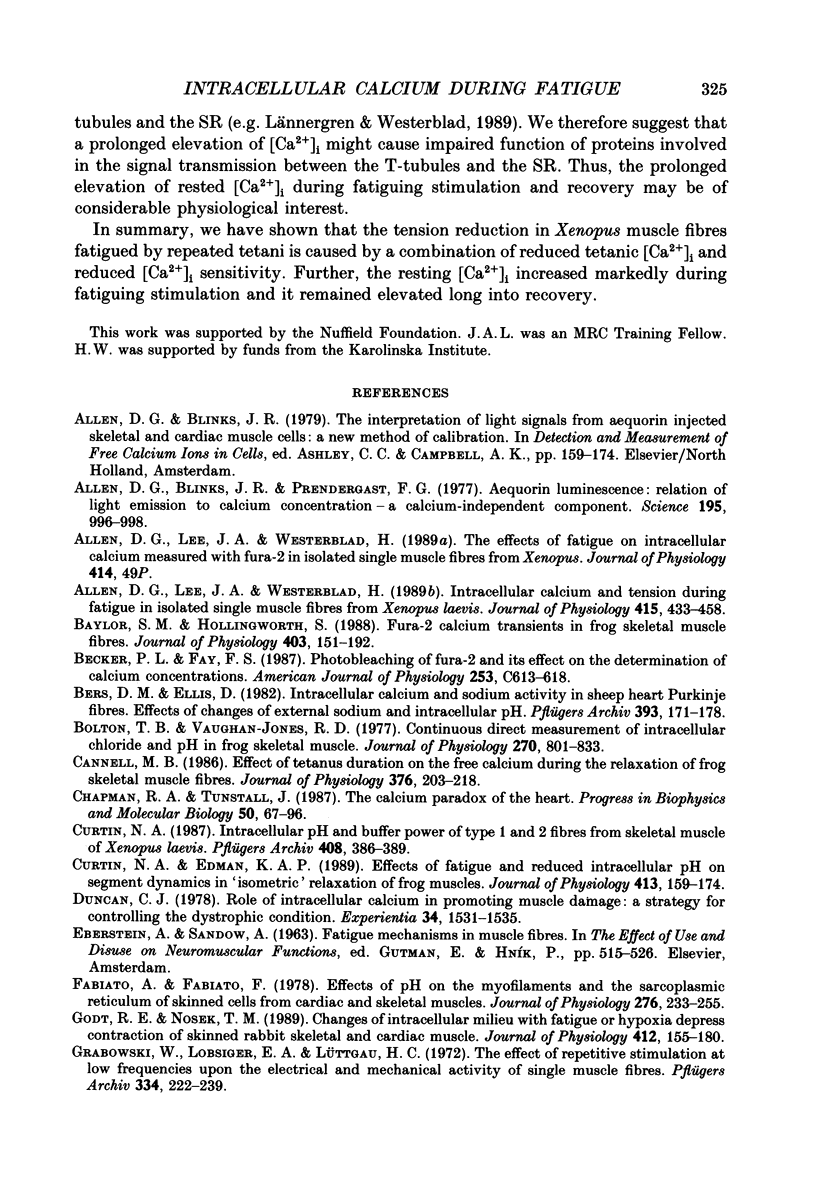
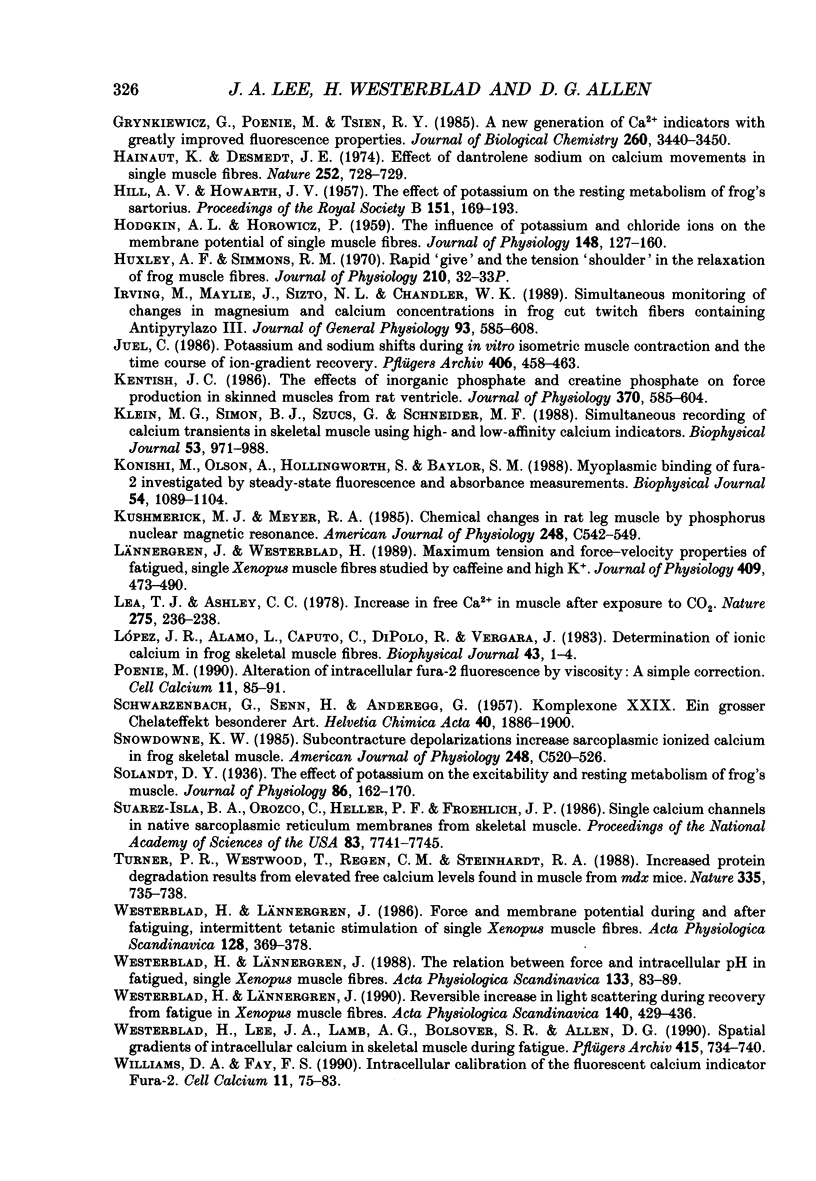
Selected References
These references are in PubMed. This may not be the complete list of references from this article.
- Allen D. G., Blinks J. R., Prendergast F. G. Aequorin luminescence: relation of light emission to calcium concentration--a calcium-independent component. Science. 1977 Mar 11;195(4282):996–998. doi: 10.1126/science.841325. [DOI] [PubMed] [Google Scholar]
- Allen D. G., Lee J. A., Westerblad H. Intracellular calcium and tension during fatigue in isolated single muscle fibres from Xenopus laevis. J Physiol. 1989 Aug;415:433–458. doi: 10.1113/jphysiol.1989.sp017730. [DOI] [PMC free article] [PubMed] [Google Scholar]
- Baylor S. M., Hollingworth S. Fura-2 calcium transients in frog skeletal muscle fibres. J Physiol. 1988 Sep;403:151–192. doi: 10.1113/jphysiol.1988.sp017244. [DOI] [PMC free article] [PubMed] [Google Scholar]
- Becker P. L., Fay F. S. Photobleaching of fura-2 and its effect on determination of calcium concentrations. Am J Physiol. 1987 Oct;253(4 Pt 1):C613–C618. doi: 10.1152/ajpcell.1987.253.4.C613. [DOI] [PubMed] [Google Scholar]
- Bers D. M., Ellis D. Intracellular calcium and sodium activity in sheep heart Purkinje fibres. Effect of changes of external sodium and intracellular pH. Pflugers Arch. 1982 Apr;393(2):171–178. doi: 10.1007/BF00582941. [DOI] [PubMed] [Google Scholar]
- Bolton T. B., Vaughan-Jones R. D. Continuous direct measurement of intracellular chloride and pH in frog skeletal muscle. J Physiol. 1977 Sep;270(3):801–833. doi: 10.1113/jphysiol.1977.sp011983. [DOI] [PMC free article] [PubMed] [Google Scholar]
- Cannell M. B. Effect of tetanus duration on the free calcium during the relaxation of frog skeletal muscle fibres. J Physiol. 1986 Jul;376:203–218. doi: 10.1113/jphysiol.1986.sp016149. [DOI] [PMC free article] [PubMed] [Google Scholar]
- Chapman R. A., Tunstall J. The calcium paradox of the heart. Prog Biophys Mol Biol. 1987;50(2):67–96. doi: 10.1016/0079-6107(87)90004-6. [DOI] [PubMed] [Google Scholar]
- Curtin N. A., Edman K. A. Effects of fatigue and reduced intracellular pH on segment dynamics in 'isometric' relaxation of frog muscle fibres. J Physiol. 1989 Jun;413:159–174. doi: 10.1113/jphysiol.1989.sp017647. [DOI] [PMC free article] [PubMed] [Google Scholar]
- Curtin N. A. Intracellular pH and buffer power of type 1 and 2 fibres from skeletal muscle of Xenopus laevis. Pflugers Arch. 1987 Apr;408(4):386–389. doi: 10.1007/BF00581133. [DOI] [PubMed] [Google Scholar]
- Duncan C. J. Role of intracellular calcium in promoting muscle damage: a strategy for controlling the dystrophic condition. Experientia. 1978 Dec 15;34(12):1531–1535. doi: 10.1007/BF02034655. [DOI] [PubMed] [Google Scholar]
- Fabiato A., Fabiato F. Effects of pH on the myofilaments and the sarcoplasmic reticulum of skinned cells from cardiace and skeletal muscles. J Physiol. 1978 Mar;276:233–255. doi: 10.1113/jphysiol.1978.sp012231. [DOI] [PMC free article] [PubMed] [Google Scholar]
- Godt R. E., Nosek T. M. Changes of intracellular milieu with fatigue or hypoxia depress contraction of skinned rabbit skeletal and cardiac muscle. J Physiol. 1989 May;412:155–180. doi: 10.1113/jphysiol.1989.sp017609. [DOI] [PMC free article] [PubMed] [Google Scholar]
- Grabowski W., Lobsiger E. A., Lüttgau H. C. The effect of repetitive stimulation at low frequencies upon the electrical and mechanical activity of single muscle fibres. Pflugers Arch. 1972;334(3):222–239. doi: 10.1007/BF00626225. [DOI] [PubMed] [Google Scholar]
- Grynkiewicz G., Poenie M., Tsien R. Y. A new generation of Ca2+ indicators with greatly improved fluorescence properties. J Biol Chem. 1985 Mar 25;260(6):3440–3450. [PubMed] [Google Scholar]
- HODGKIN A. L., HOROWICZ P. The influence of potassium and chloride ions on the membrane potential of single muscle fibres. J Physiol. 1959 Oct;148:127–160. doi: 10.1113/jphysiol.1959.sp006278. [DOI] [PMC free article] [PubMed] [Google Scholar]
- Hainaut K., Desmedt J. E. Effect of dantrolene sodium on calcium movements in single muscle fibres. Nature. 1974 Dec 20;252(5485):728–730. doi: 10.1038/252728a0. [DOI] [PubMed] [Google Scholar]
- Huxley A. F., Simmons R. M. Rapid 'give' and the tension 'shoulder' in the relaxation of frog muscle fibres. J Physiol. 1970 Sep;210(1):32P–33P. [PubMed] [Google Scholar]
- Irving M., Maylie J., Sizto N. L., Chandler W. K. Simultaneous monitoring of changes in magnesium and calcium concentrations in frog cut twitch fibers containing antipyrylazo III. J Gen Physiol. 1989 Apr;93(4):585–608. doi: 10.1085/jgp.93.4.585. [DOI] [PMC free article] [PubMed] [Google Scholar]
- Juel C. Potassium and sodium shifts during in vitro isometric muscle contraction, and the time course of the ion-gradient recovery. Pflugers Arch. 1986 May;406(5):458–463. doi: 10.1007/BF00583367. [DOI] [PubMed] [Google Scholar]
- Kentish J. C. The effects of inorganic phosphate and creatine phosphate on force production in skinned muscles from rat ventricle. J Physiol. 1986 Jan;370:585–604. doi: 10.1113/jphysiol.1986.sp015952. [DOI] [PMC free article] [PubMed] [Google Scholar]
- Klein M. G., Simon B. J., Szucs G., Schneider M. F. Simultaneous recording of calcium transients in skeletal muscle using high- and low-affinity calcium indicators. Biophys J. 1988 Jun;53(6):971–988. doi: 10.1016/S0006-3495(88)83178-3. [DOI] [PMC free article] [PubMed] [Google Scholar]
- Konishi M., Olson A., Hollingworth S., Baylor S. M. Myoplasmic binding of fura-2 investigated by steady-state fluorescence and absorbance measurements. Biophys J. 1988 Dec;54(6):1089–1104. doi: 10.1016/S0006-3495(88)83045-5. [DOI] [PMC free article] [PubMed] [Google Scholar]
- Kushmerick M. J., Meyer R. A. Chemical changes in rat leg muscle by phosphorus nuclear magnetic resonance. Am J Physiol. 1985 May;248(5 Pt 1):C542–C549. doi: 10.1152/ajpcell.1985.248.5.C542. [DOI] [PubMed] [Google Scholar]
- Lea T. J., Ashley C. C. Increase in free Ca2+ in muscle after exposure to CO2. Nature. 1978 Sep 21;275(5677):236–238. doi: 10.1038/275236a0. [DOI] [PubMed] [Google Scholar]
- Lännergren J., Westerblad H. Maximum tension and force-velocity properties of fatigued, single Xenopus muscle fibres studied by caffeine and high K+. J Physiol. 1989 Feb;409:473–490. doi: 10.1113/jphysiol.1989.sp017508. [DOI] [PMC free article] [PubMed] [Google Scholar]
- López J. R., Alamo L., Caputo C., DiPolo R., Vergara S. Determination of ionic calcium in frog skeletal muscle fibers. Biophys J. 1983 Jul;43(1):1–4. doi: 10.1016/S0006-3495(83)84316-1. [DOI] [PMC free article] [PubMed] [Google Scholar]
- Poenie M. Alteration of intracellular Fura-2 fluorescence by viscosity: a simple correction. Cell Calcium. 1990 Feb-Mar;11(2-3):85–91. doi: 10.1016/0143-4160(90)90062-y. [DOI] [PubMed] [Google Scholar]
- Snowdowne K. W. Subcontracture depolarizations increase sarcoplasmic ionized calcium in frog skeletal muscle. Am J Physiol. 1985 May;248(5 Pt 1):C520–C526. doi: 10.1152/ajpcell.1985.248.5.C520. [DOI] [PubMed] [Google Scholar]
- Solandt D. Y. The effect of potassium on the excitability and resting metabolism of frog's muscle. J Physiol. 1936 Feb 8;86(2):162–170. doi: 10.1113/jphysiol.1936.sp003351. [DOI] [PMC free article] [PubMed] [Google Scholar]
- Suarez-Isla B. A., Orozco C., Heller P. F., Froehlich J. P. Single calcium channels in native sarcoplasmic reticulum membranes from skeletal muscle. Proc Natl Acad Sci U S A. 1986 Oct;83(20):7741–7745. doi: 10.1073/pnas.83.20.7741. [DOI] [PMC free article] [PubMed] [Google Scholar]
- Turner P. R., Westwood T., Regen C. M., Steinhardt R. A. Increased protein degradation results from elevated free calcium levels found in muscle from mdx mice. Nature. 1988 Oct 20;335(6192):735–738. doi: 10.1038/335735a0. [DOI] [PubMed] [Google Scholar]
- Westerblad H., Lee J. A., Lamb A. G., Bolsover S. R., Allen D. G. Spatial gradients of intracellular calcium in skeletal muscle during fatigue. Pflugers Arch. 1990 Mar;415(6):734–740. doi: 10.1007/BF02584013. [DOI] [PubMed] [Google Scholar]
- Westerblad H., Lännergren J. Force and membrane potential during and after fatiguing, intermittent tetanic stimulation of single Xenopus muscle fibres. Acta Physiol Scand. 1986 Nov;128(3):369–378. doi: 10.1111/j.1748-1716.1986.tb07990.x. [DOI] [PubMed] [Google Scholar]
- Westerblad H., Lännergren J. Reversible increase in light scattering during recovery from fatigue in Xenopus muscle fibres. Acta Physiol Scand. 1990 Nov;140(3):429–435. doi: 10.1111/j.1748-1716.1990.tb09018.x. [DOI] [PubMed] [Google Scholar]
- Westerblad H., Lännergren J. The relation between force and intracellular pH in fatigued, single Xenopus muscle fibres. Acta Physiol Scand. 1988 May;133(1):83–89. doi: 10.1111/j.1748-1716.1988.tb08383.x. [DOI] [PubMed] [Google Scholar]
- Williams D. A., Fay F. S. Intracellular calibration of the fluorescent calcium indicator Fura-2. Cell Calcium. 1990 Feb-Mar;11(2-3):75–83. doi: 10.1016/0143-4160(90)90061-x. [DOI] [PubMed] [Google Scholar]