Abstract
1. In frog a variety of treatments have been shown to increase quantal size at the neuromuscular junction (NMJ), apparently by releasing more acetylcholine (ACh) per quantum. The present experiments were undertaken to see whether similar changes occur at the mouse NMJ. 2. None of the hormones tested, adrenaline, insulin or corticosteroids, significantly increased quantal size at the mouse NMJ. 3. Soaking diaphragms in hypertonic solution (about 475 mosmol/kg) for 15-30 min roughly doubled the size of miniature endplate potentials (MEPPs), miniature endplate currents (MEPCs), and uniquantal endplate potentials (EPPs). We will refer to these as 'large quanta'. Note that all of the measurements were made after returning the preparation to normal (Tyrode) solution. 4. In frog hypertonic solution made with sodium gluconate replacing NaCl increases quantal size four- rather than two-fold. In mouse there was little difference in the effects of solutions made with the two anions. In Cl(-)-free hypertonic solution, made with sodium gluconate and SO4(2-) solutions, quantal size increase is somewhat less, so there may be a role for Cl- in enlarging quantal size. 5. After hypertonic treatment, quantal size remained elevated for at least 1 h and then gradually declined back to usual levels. The data suggest a gradual decrease in mean quantal size, rather than the appearance of a new subpopulation of smaller quanta. 6. Hypertonic treatment did not change the endplate depolarization in response to ionophoretically applied ACh. This suggests that the increased quantal size is not due to a postsynaptic change. Large MEPP's disappear in the presence of tubocurarine and reappear when the drug is washed away. 7. Vesamicol is an inhibitor of active ACh uptake into isolated synaptic vesicles. 5 microM-vesamicol has no detectable postjunctional effect. However, when vesamicol was included in the Tyrode and the hypertonic solutions the increase in quantal size was blocked. This is further evidence that the large quanta are produced by the release of more ACh per quantum. 8. Even when added after the hypertonic treatment, vesamicol soon decreased quantal size back to the normal level. Two other inhibitors of active ACh uptake into vesicles, tetraphenylboron (TPB) and hexanitrodiphenylamine (HNPA) also decreased quantal size after hypertonic treatment, apparently by a presynaptic action. This suggests that the additional ACh that produces large miniatures may be incorporated into the quanta shortly before release.(ABSTRACT TRUNCATED AT 400 WORDS)
Full text
PDF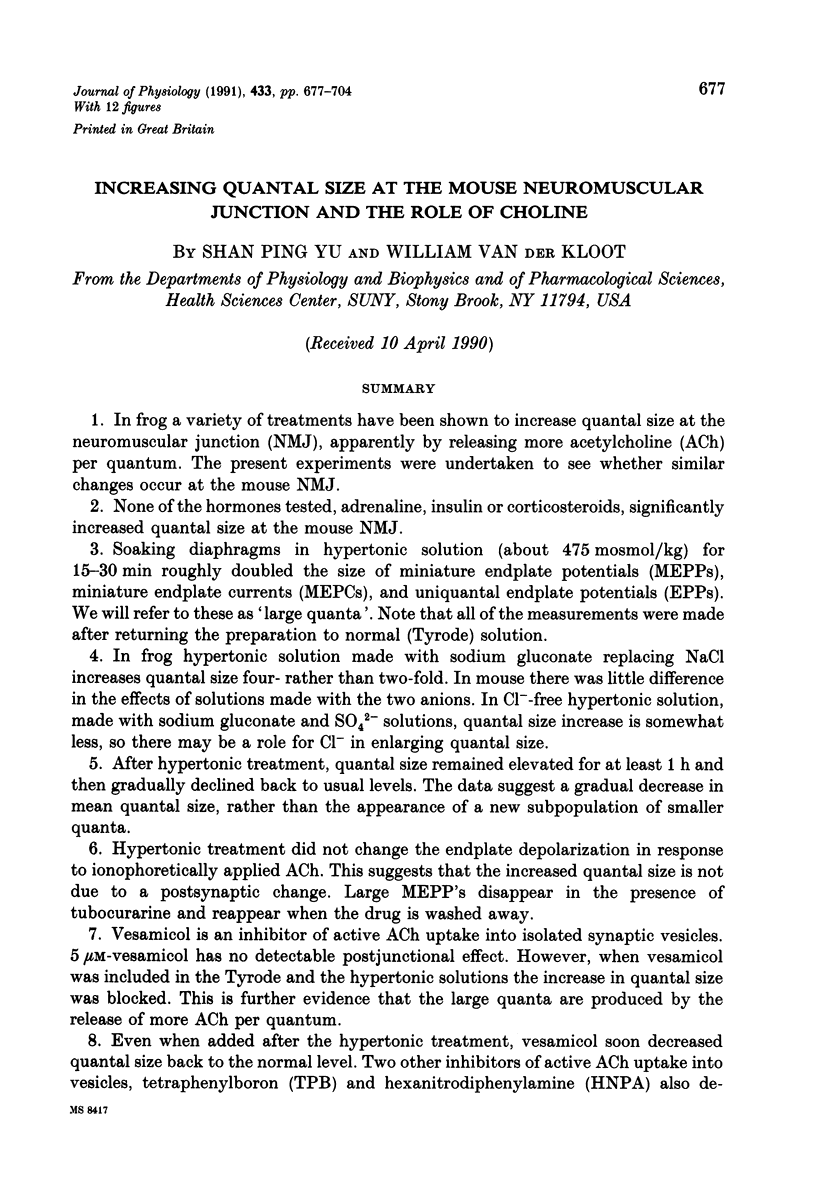
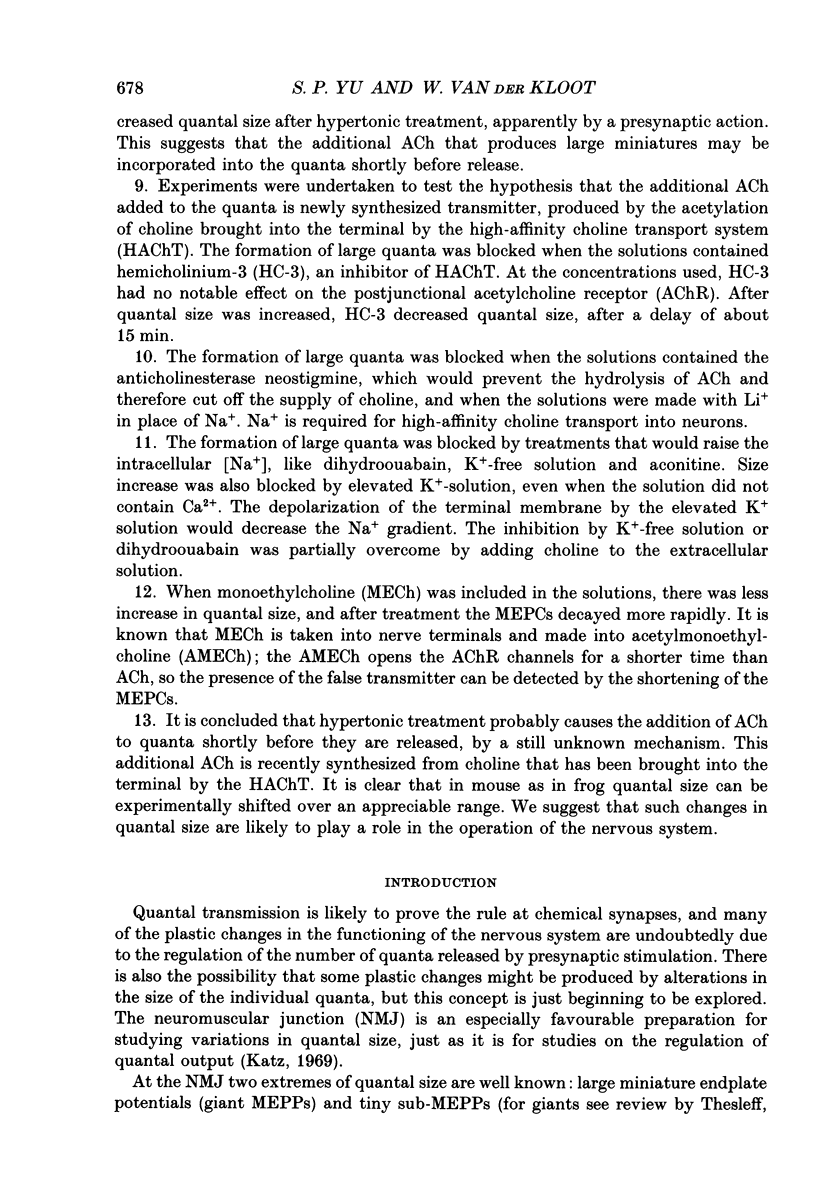
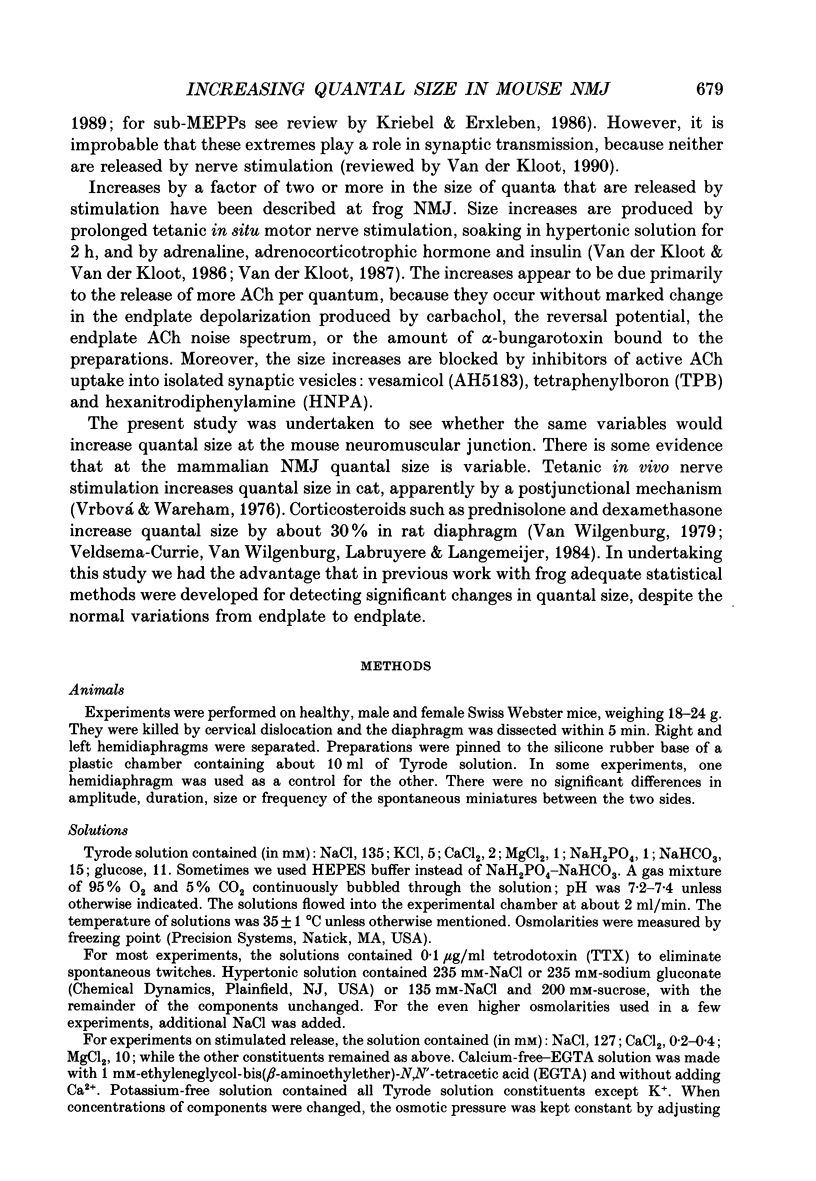
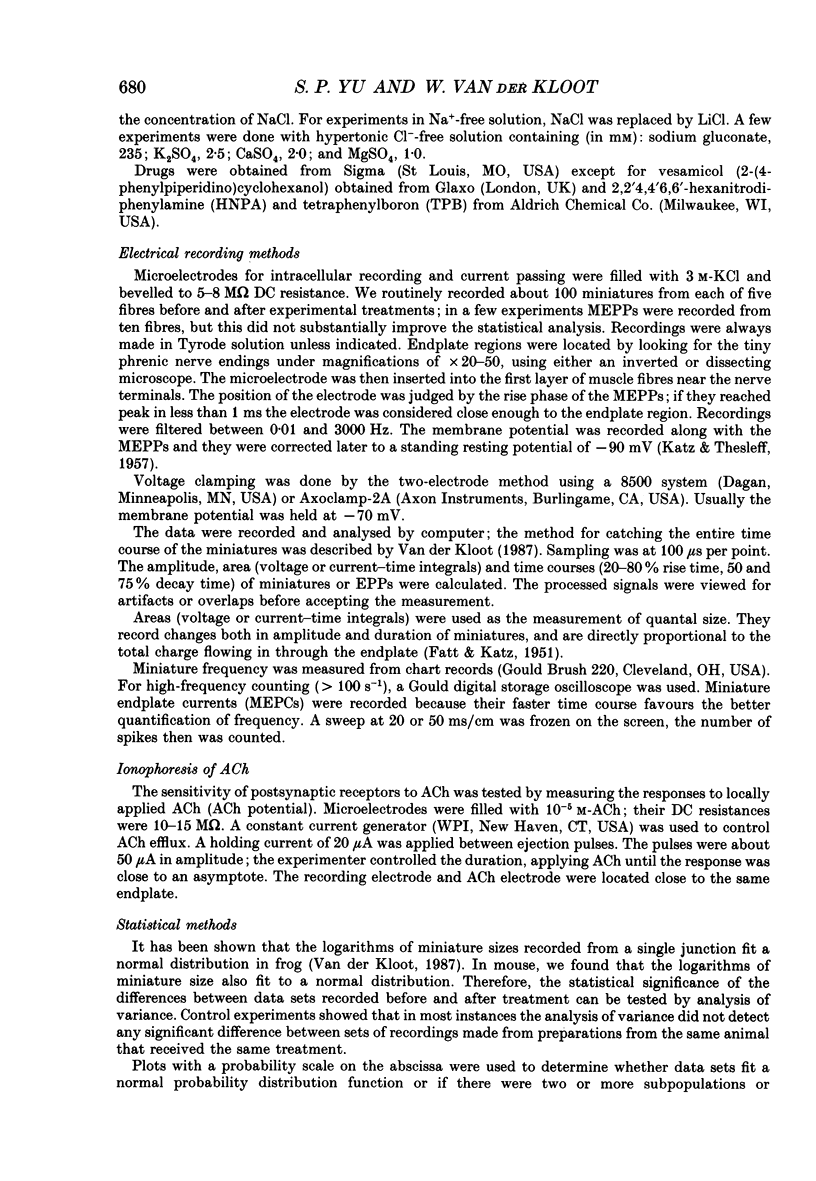
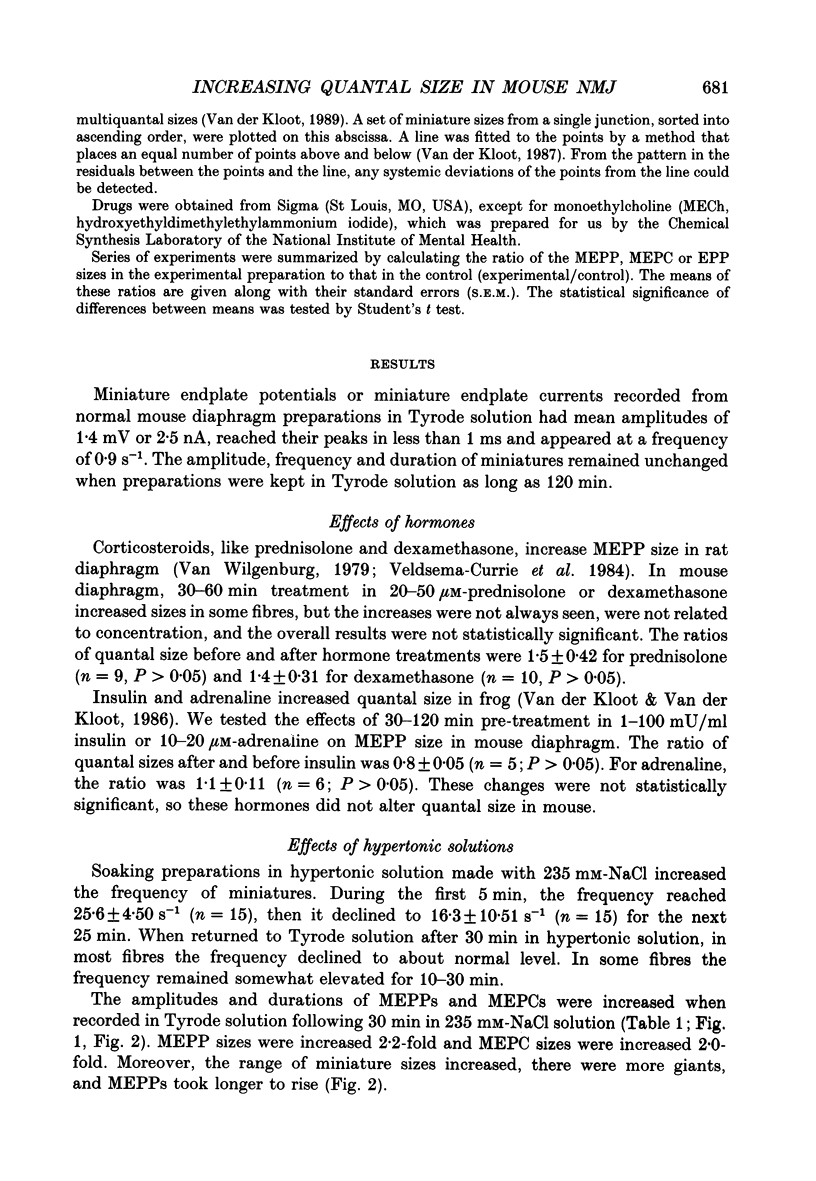
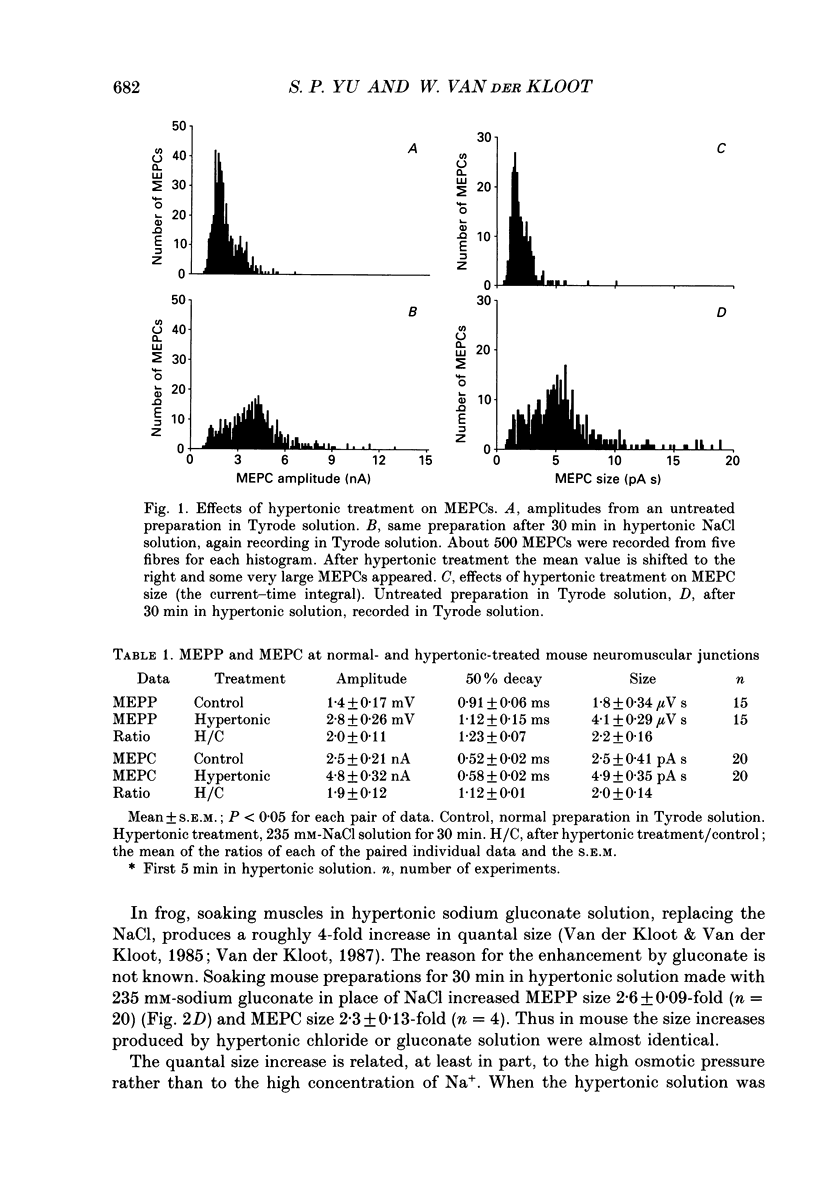
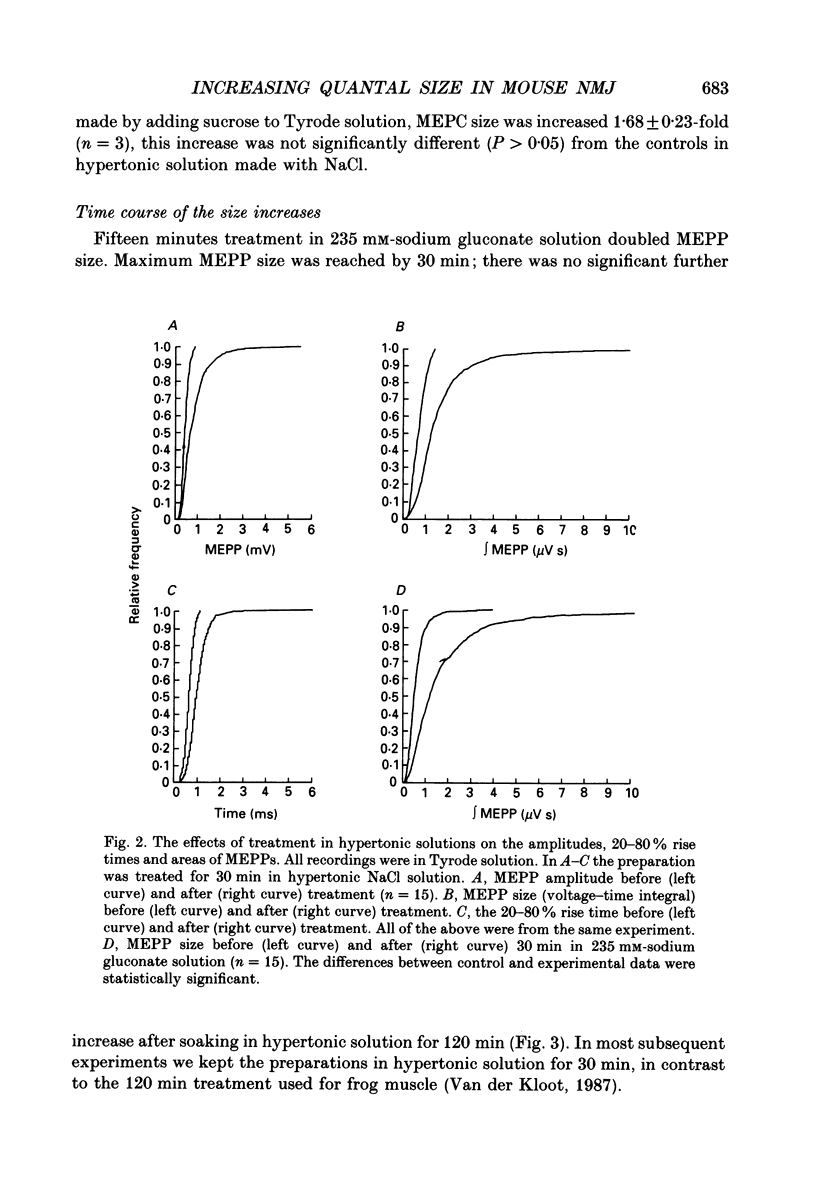
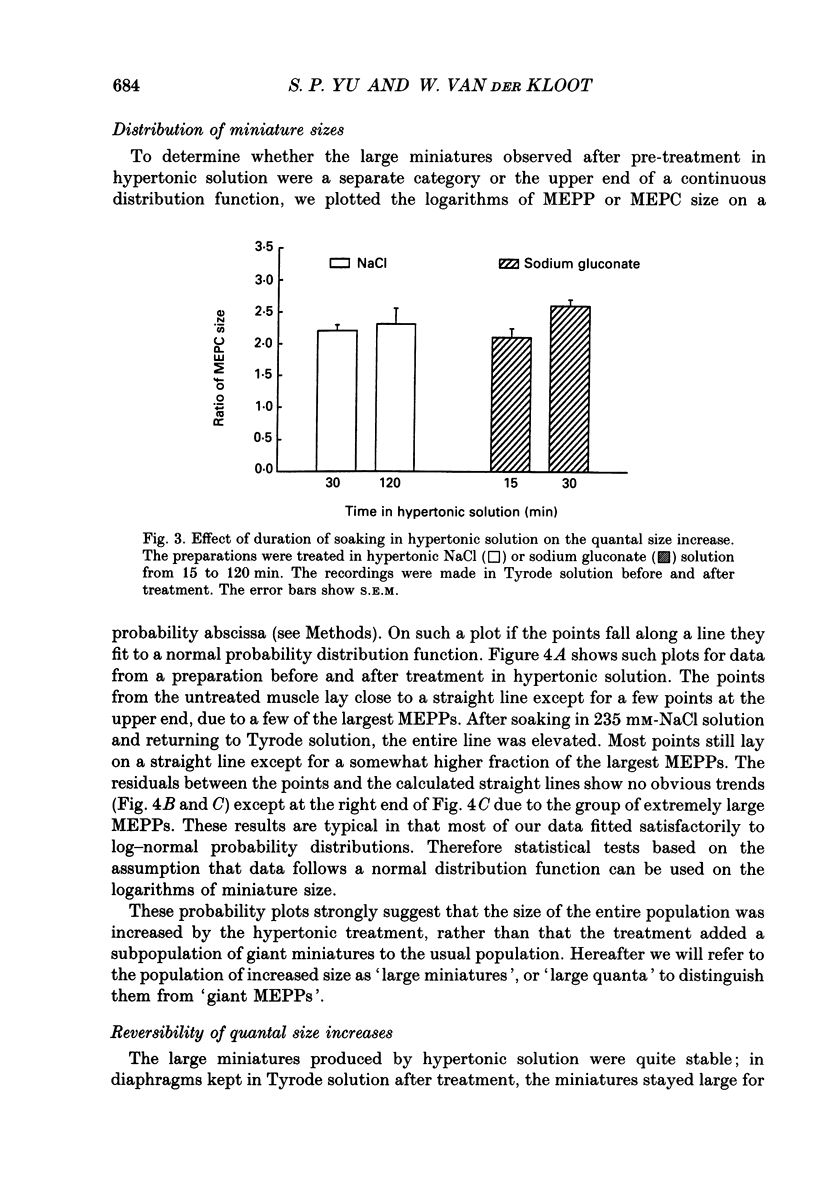
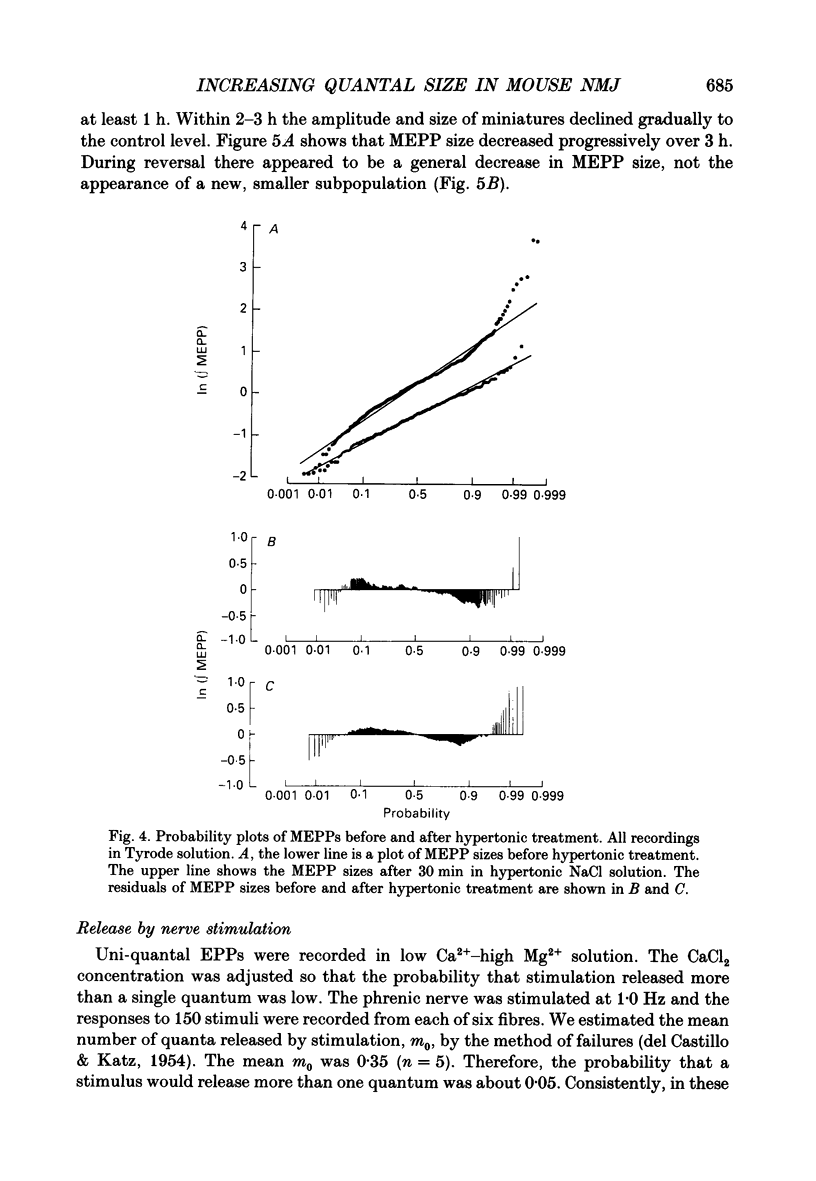
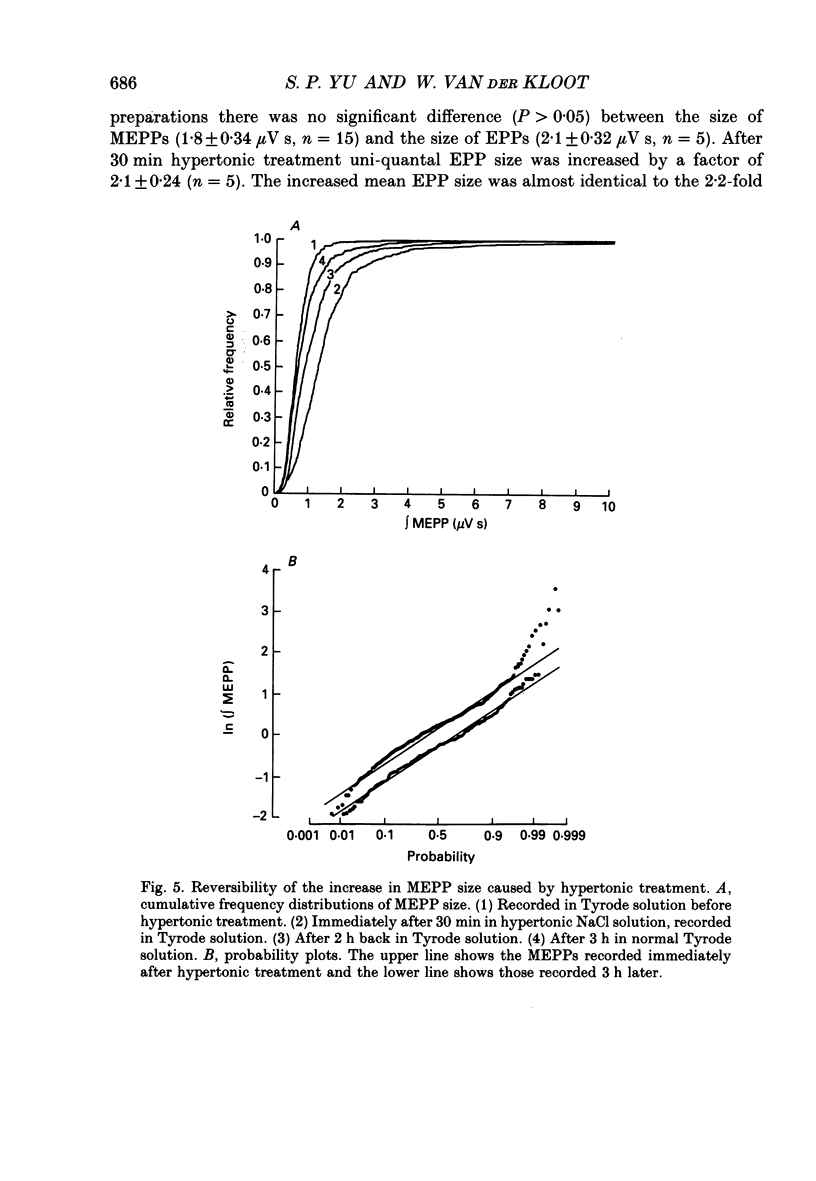
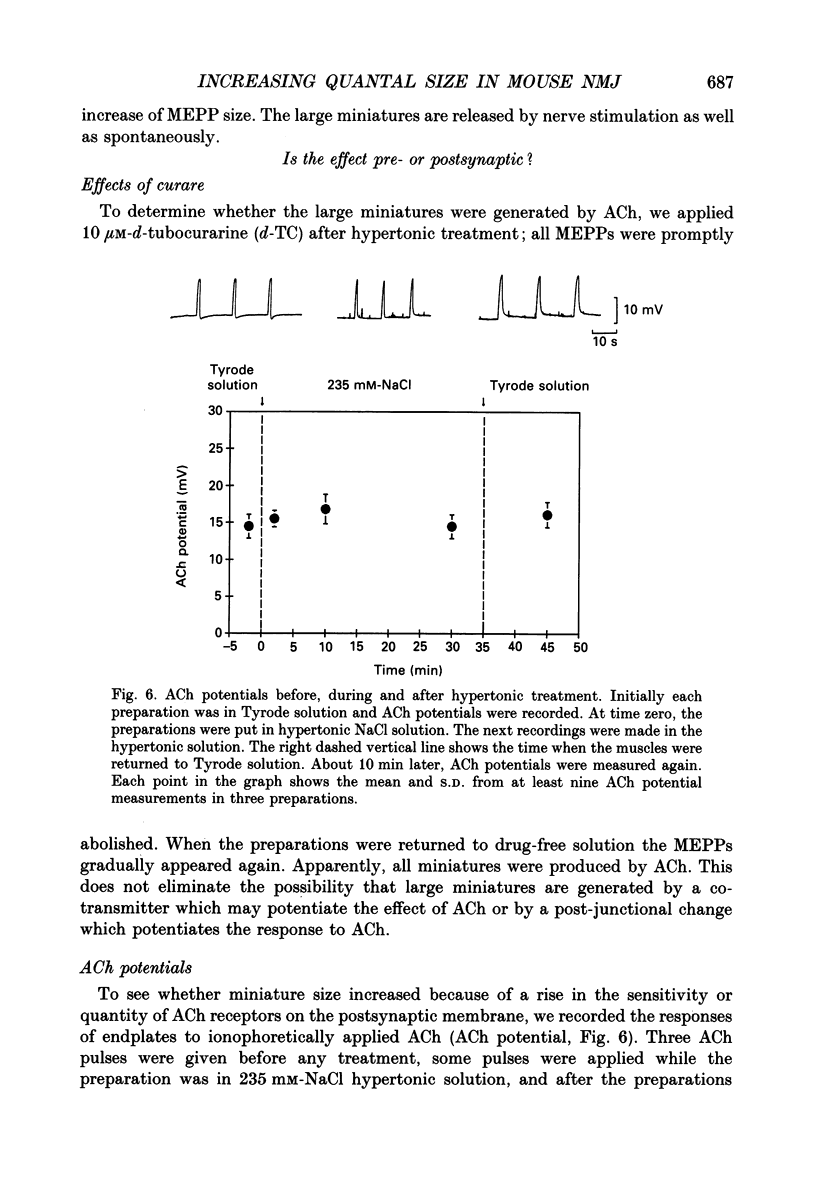
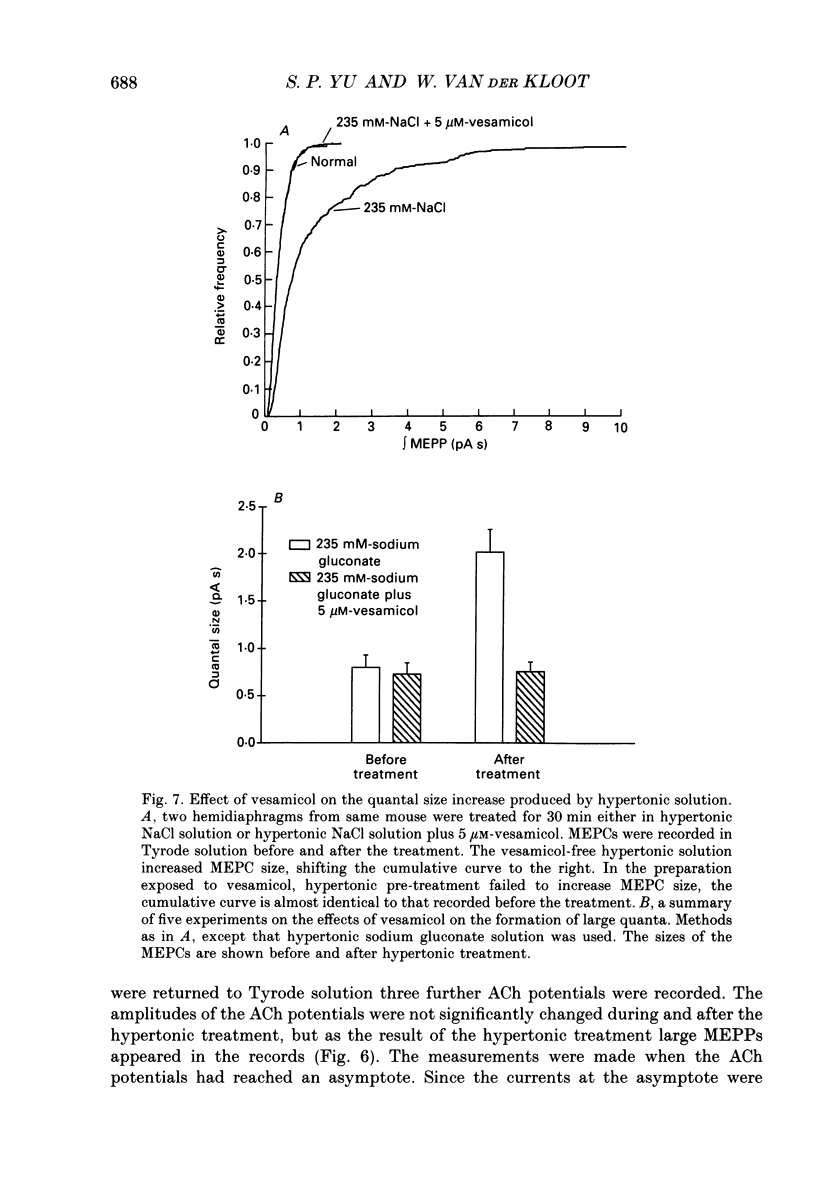
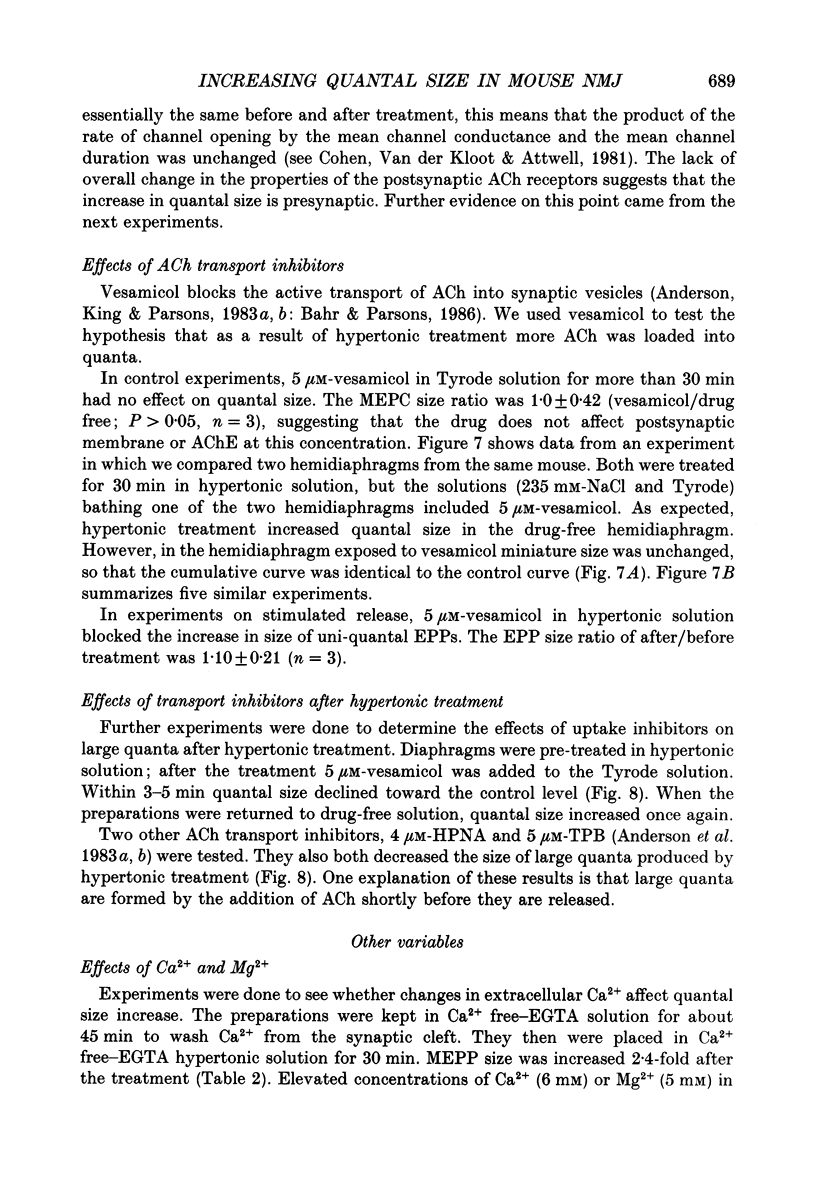
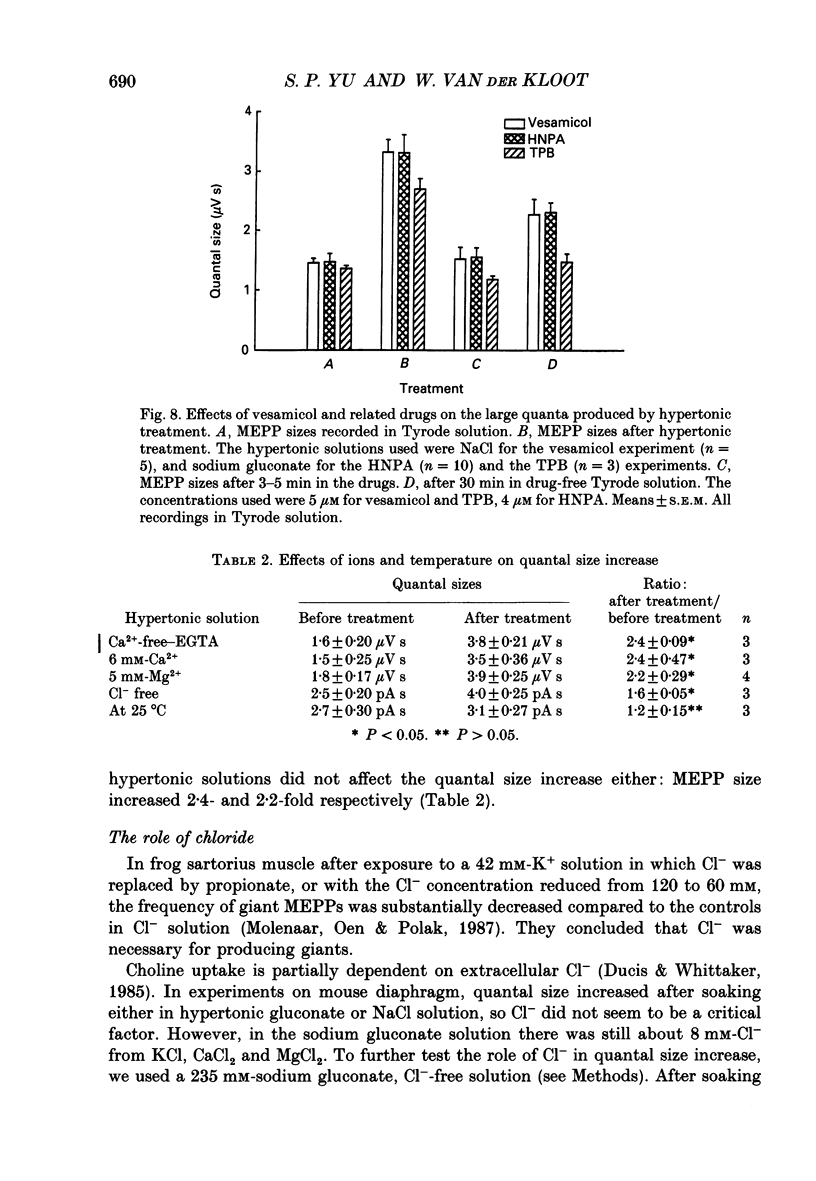
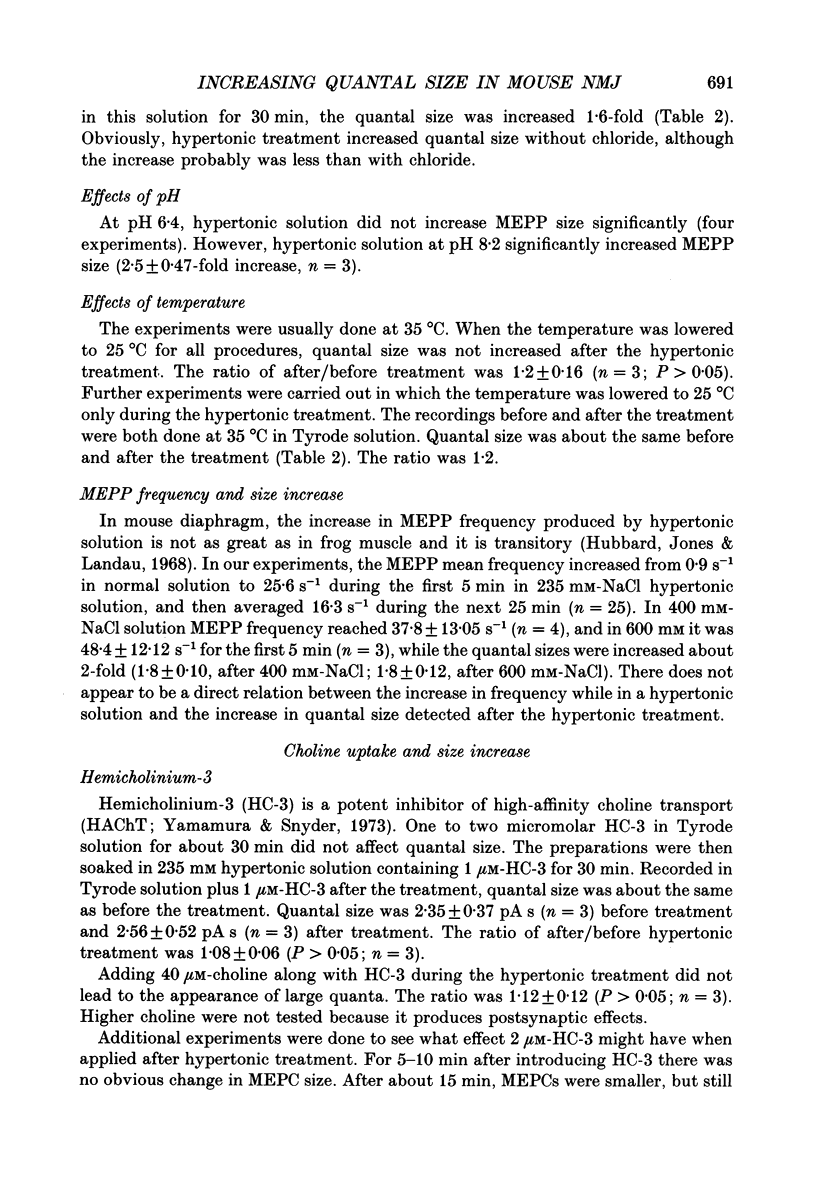
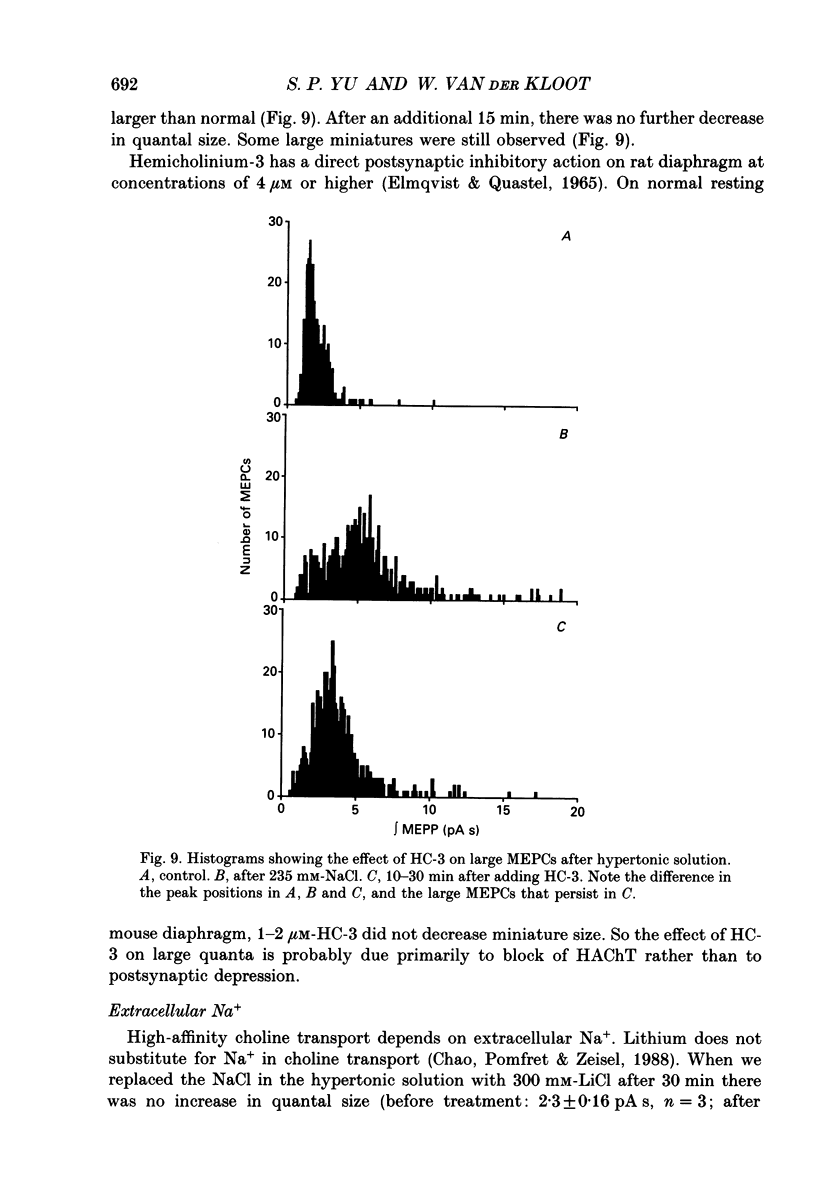
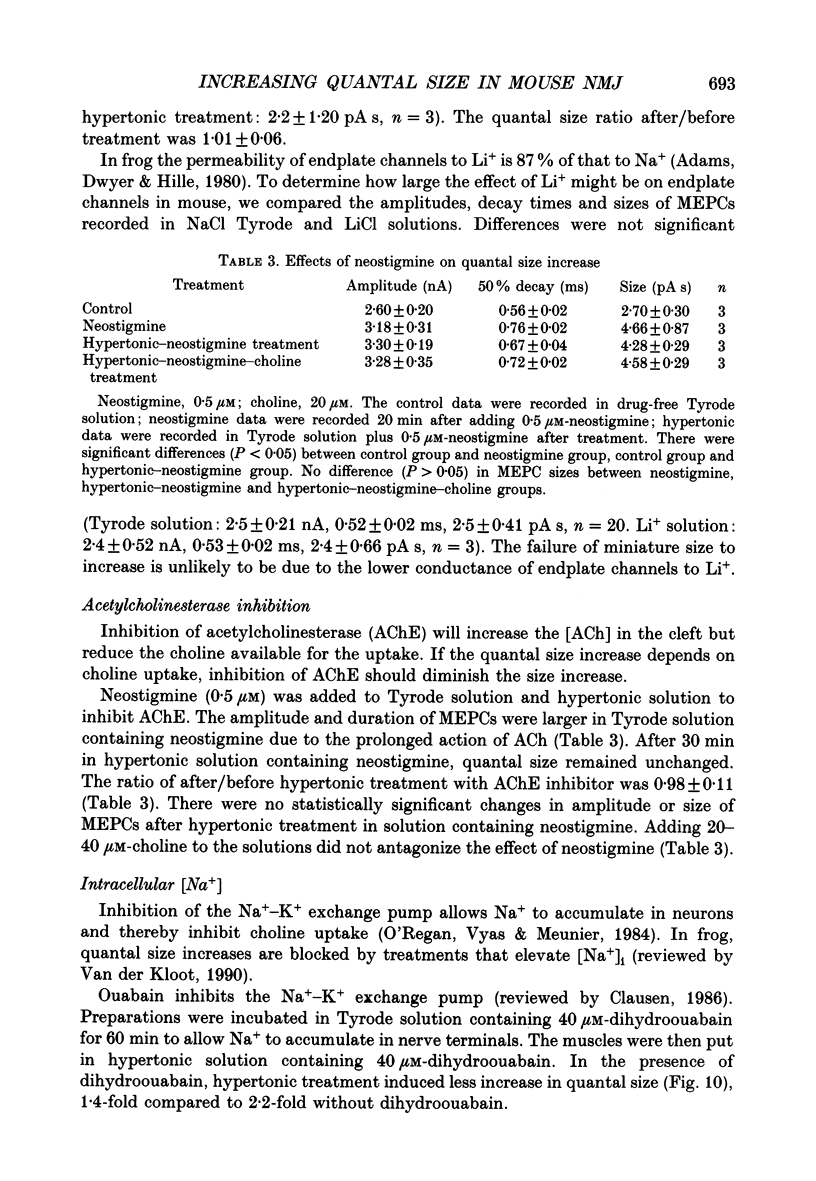
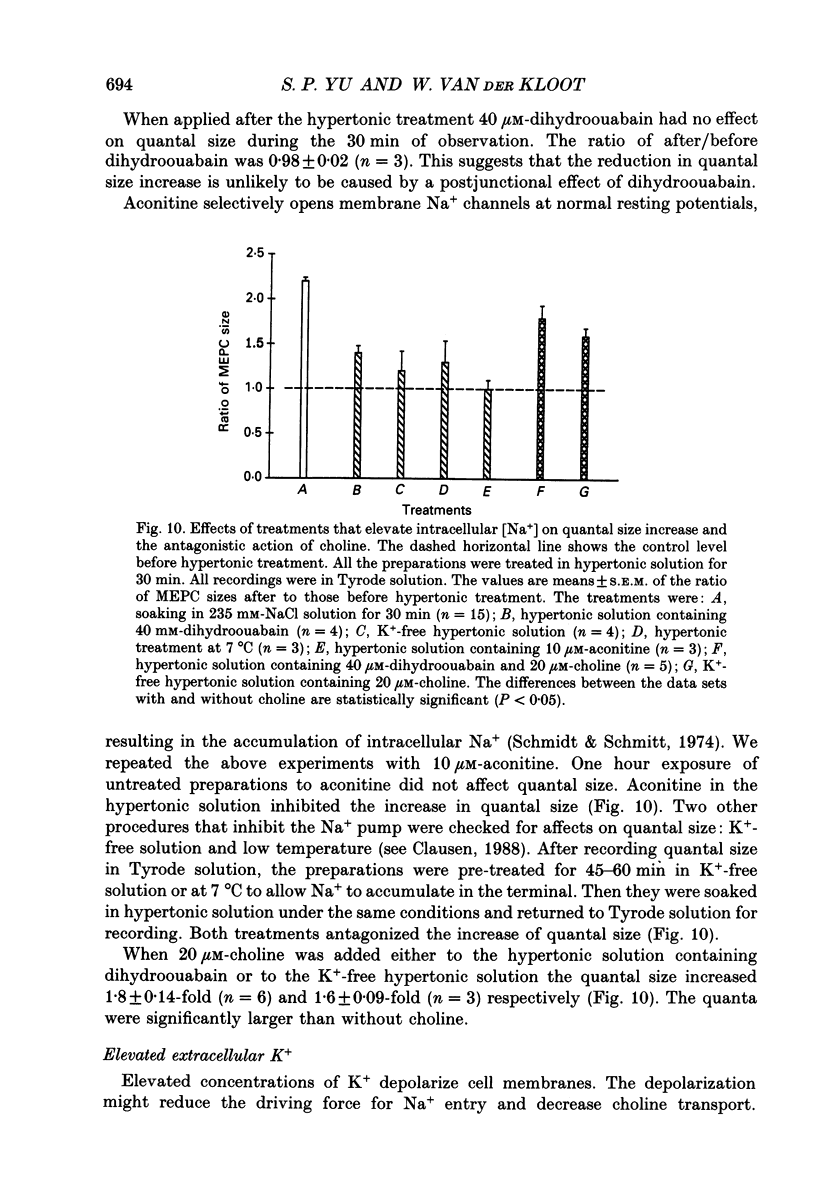
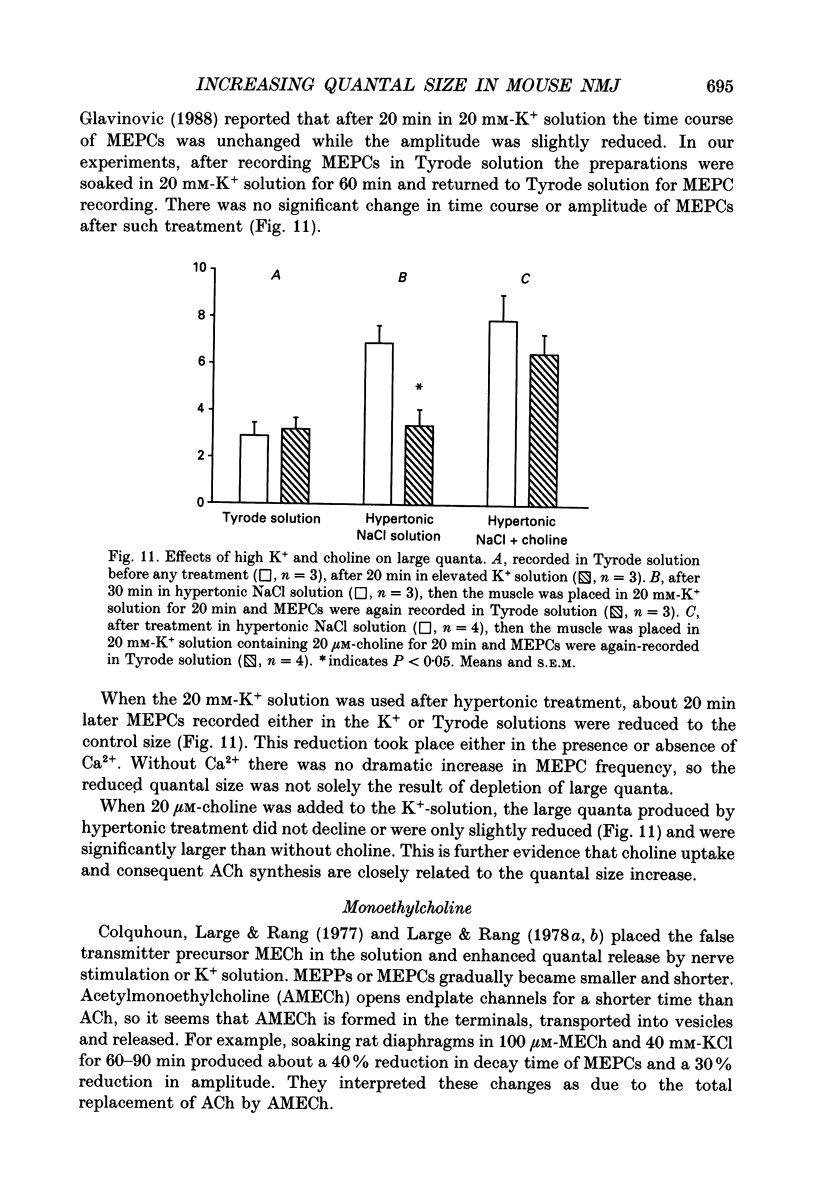
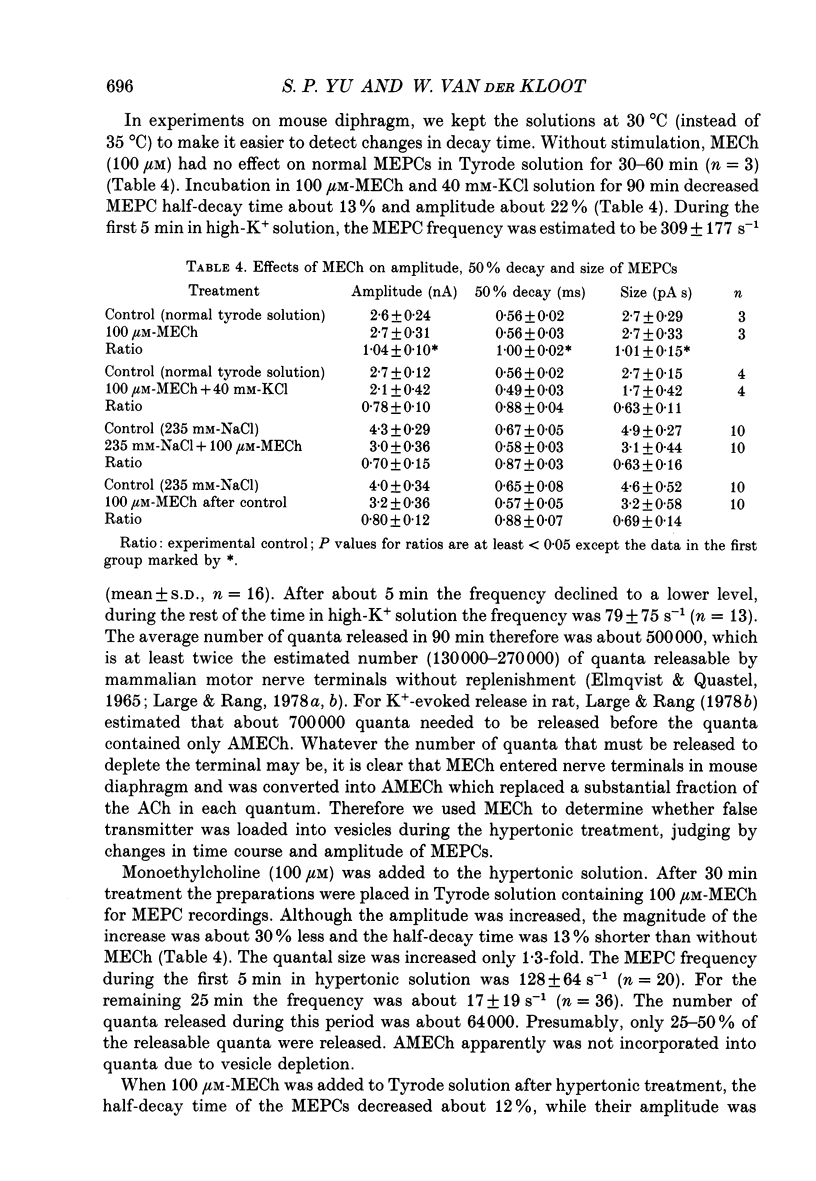
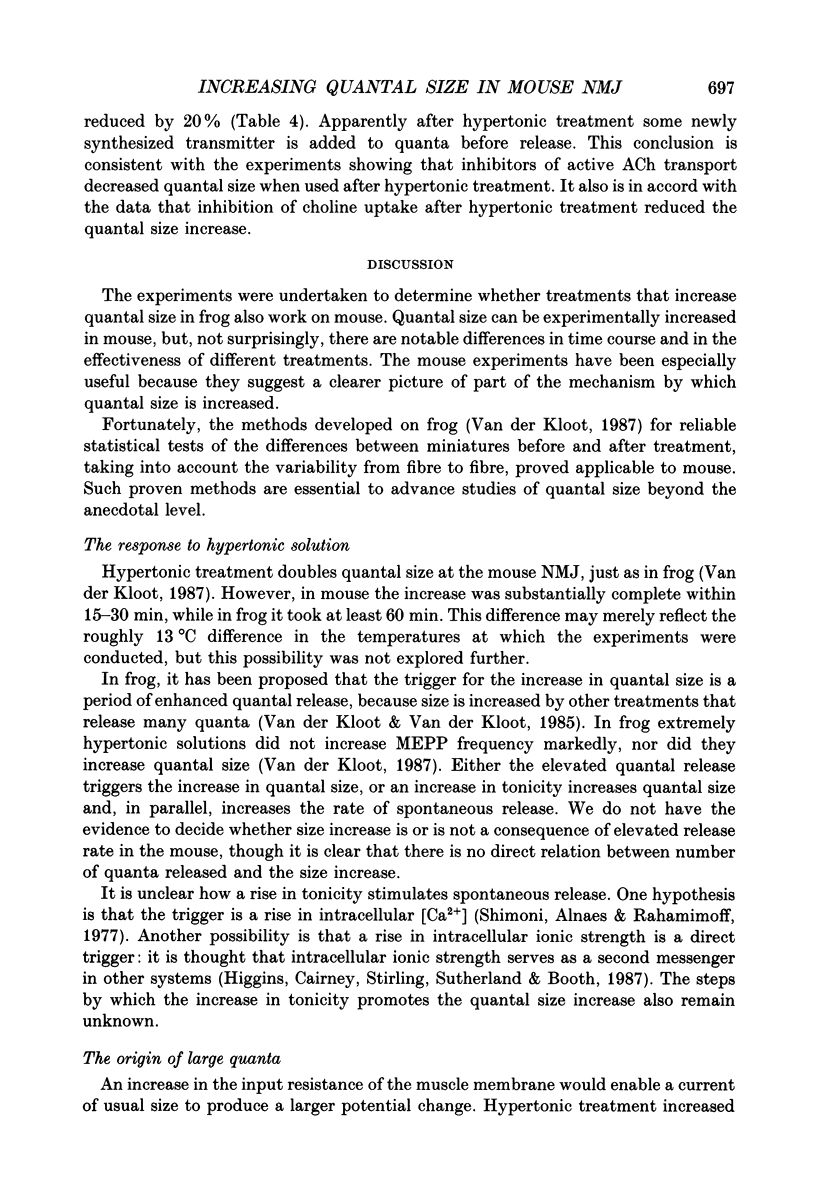
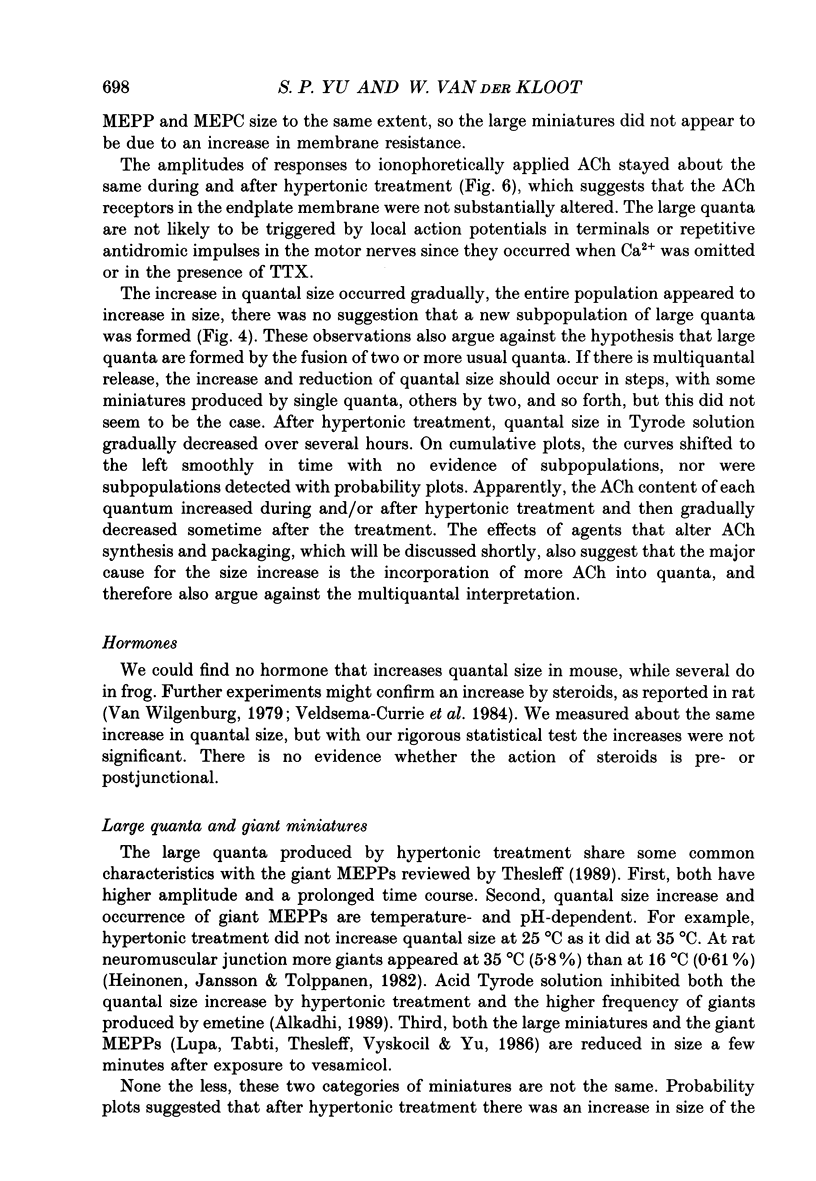
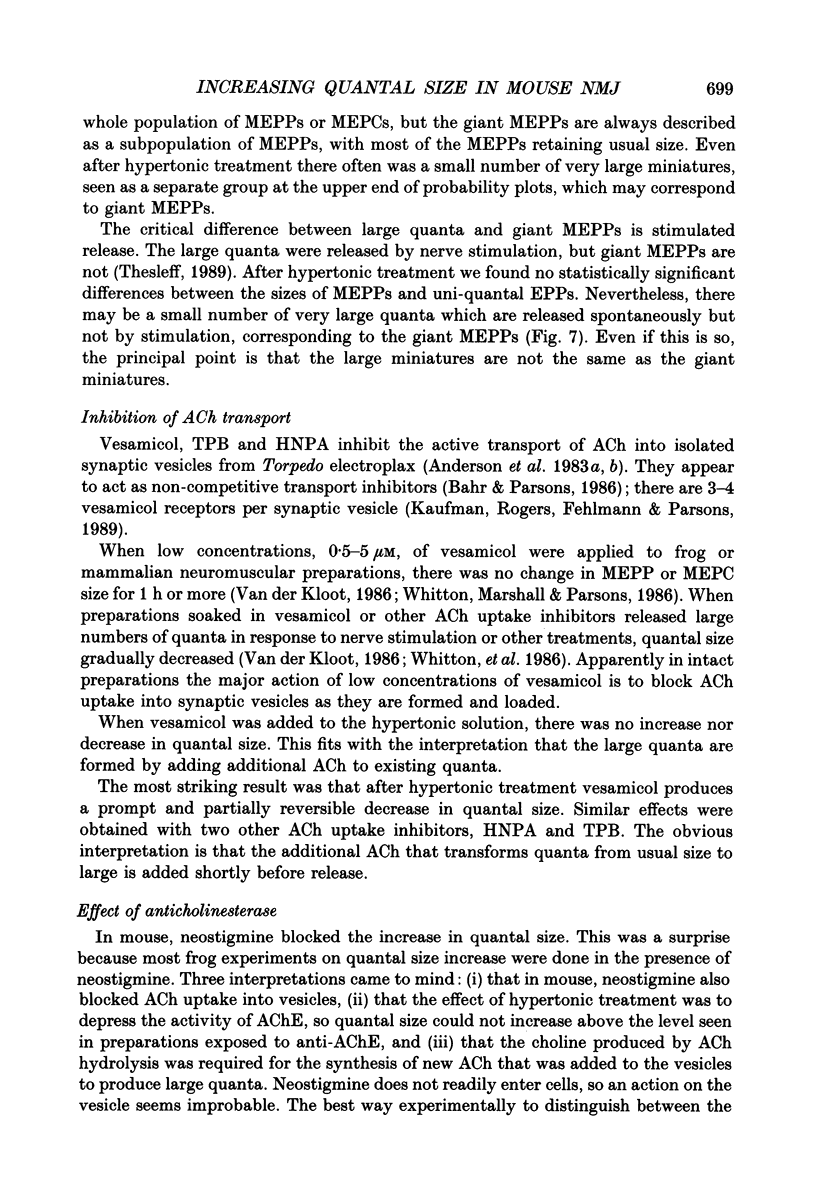
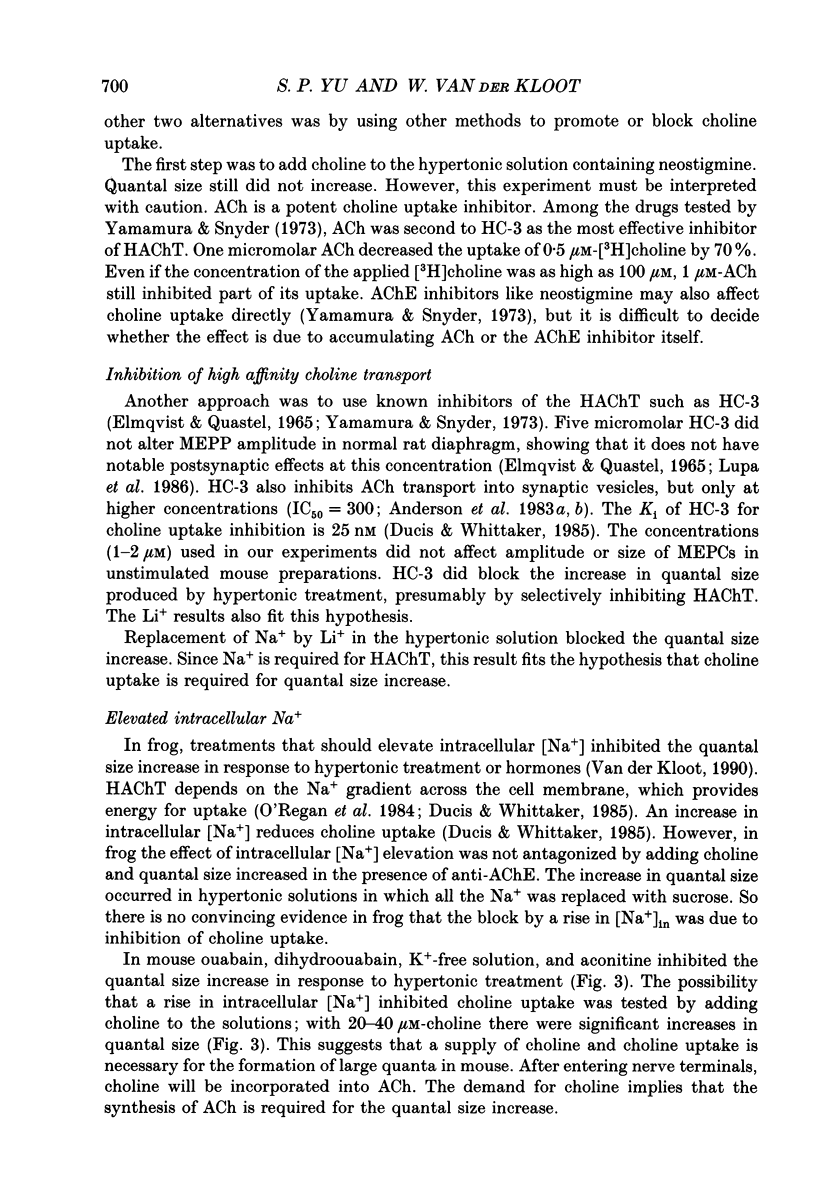
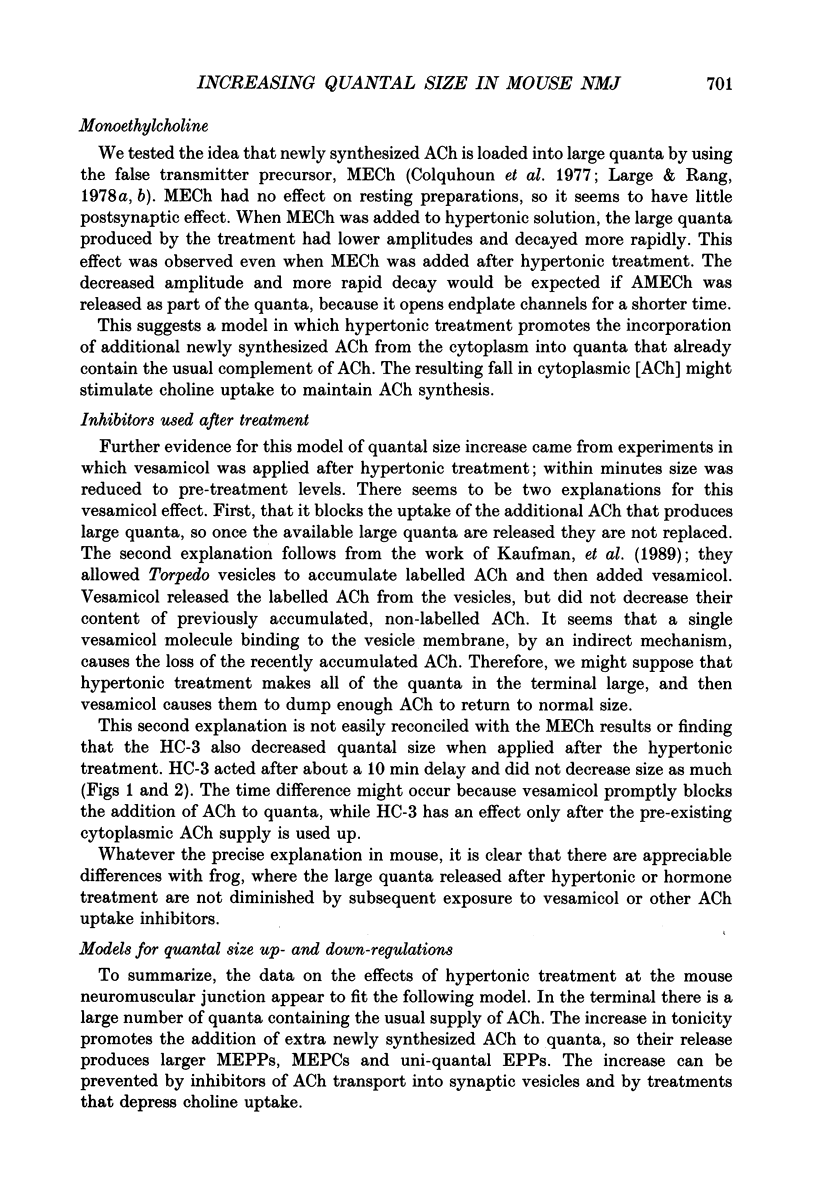
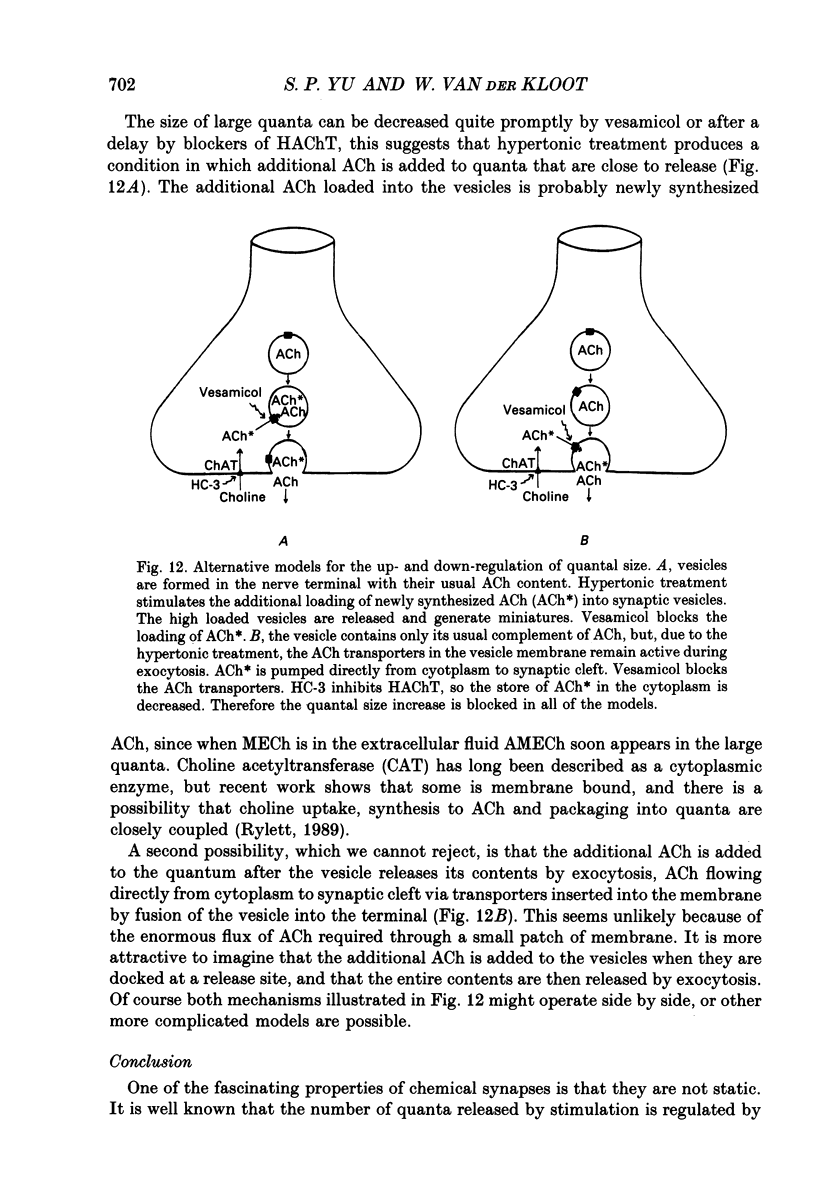
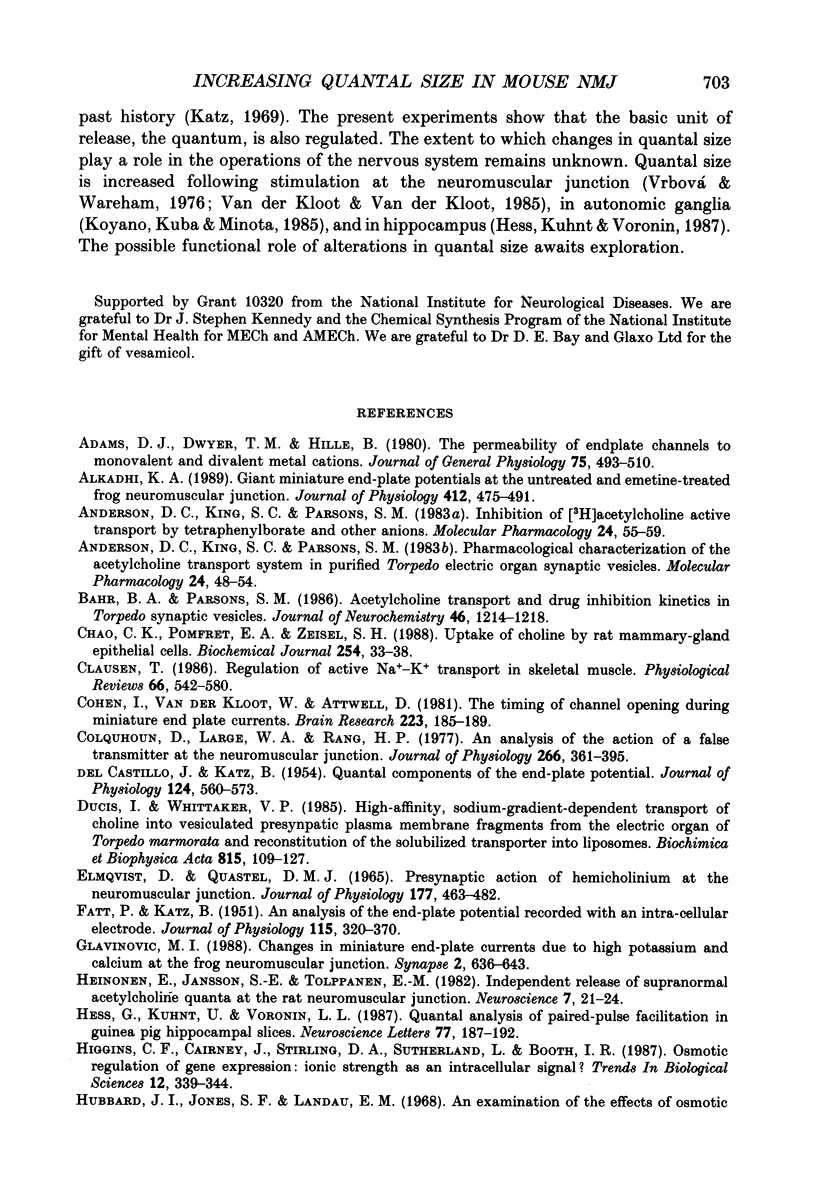
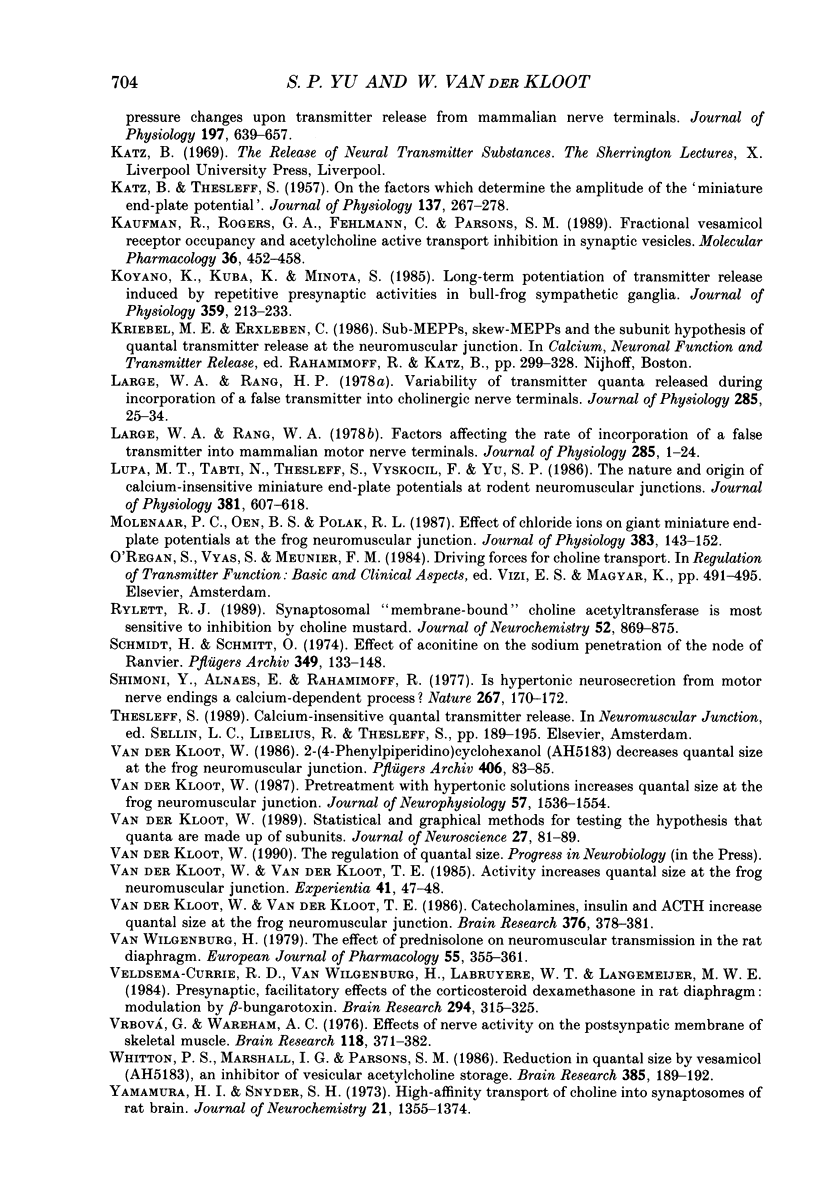
Selected References
These references are in PubMed. This may not be the complete list of references from this article.
- Adams D. J., Dwyer T. M., Hille B. The permeability of endplate channels to monovalent and divalent metal cations. J Gen Physiol. 1980 May;75(5):493–510. doi: 10.1085/jgp.75.5.493. [DOI] [PMC free article] [PubMed] [Google Scholar]
- Alkadhi K. A. Giant miniature end-plate potentials at the untreated and emetine-treated frog neuromuscular junction. J Physiol. 1989 May;412:475–491. doi: 10.1113/jphysiol.1989.sp017627. [DOI] [PMC free article] [PubMed] [Google Scholar]
- Anderson D. C., King S. C., Parsons S. M. Inhibition of [3H]acetylcholine active transport by tetraphenylborate and other anions. Mol Pharmacol. 1983 Jul;24(1):55–59. [PubMed] [Google Scholar]
- Anderson D. C., King S. C., Parsons S. M. Pharmacological characterization of the acetylcholine transport system in purified Torpedo electric organ synaptic vesicles. Mol Pharmacol. 1983 Jul;24(1):48–54. [PubMed] [Google Scholar]
- Bahr B. A., Parsons S. M. Acetylcholine transport and drug inhibition kinetics in Torpedo synaptic vesicles. J Neurochem. 1986 Apr;46(4):1214–1218. doi: 10.1111/j.1471-4159.1986.tb00640.x. [DOI] [PubMed] [Google Scholar]
- Chao C. K., Pomfret E. A., Zeisel S. H. Uptake of choline by rat mammary-gland epithelial cells. Biochem J. 1988 Aug 15;254(1):33–38. doi: 10.1042/bj2540033. [DOI] [PMC free article] [PubMed] [Google Scholar]
- Clausen T. Regulation of active Na+-K+ transport in skeletal muscle. Physiol Rev. 1986 Jul;66(3):542–580. doi: 10.1152/physrev.1986.66.3.542. [DOI] [PubMed] [Google Scholar]
- Cohen I., van der Kloot W., Attwell D. The timing of channel opening during miniature end-plate currents. Brain Res. 1981 Oct 26;223(1):185–189. doi: 10.1016/0006-8993(81)90821-0. [DOI] [PubMed] [Google Scholar]
- Colquhoun D., Large W. A., Rang H. P. An analysis of the action of a false transmitter at the neuromuscular junction. J Physiol. 1977 Apr;266(2):361–395. doi: 10.1113/jphysiol.1977.sp011772. [DOI] [PMC free article] [PubMed] [Google Scholar]
- DEL CASTILLO J., KATZ B. Quantal components of the end-plate potential. J Physiol. 1954 Jun 28;124(3):560–573. doi: 10.1113/jphysiol.1954.sp005129. [DOI] [PMC free article] [PubMed] [Google Scholar]
- Ducis I., Whittaker V. P. High-affinity, sodium-gradient-dependent transport of choline into vesiculated presynaptic plasma membrane fragments from the electric organ of Torpedo marmorata and reconstitution of the solubilized transporter into liposomes. Biochim Biophys Acta. 1985 Apr 26;815(1):109–127. doi: 10.1016/0005-2736(85)90481-x. [DOI] [PubMed] [Google Scholar]
- ELMQVIST D., QUASTEL D. M. PRESYNAPTIC ACTION OF HEMICHOLINIUM AT THE NEUROMUSCULAR JUNCTION. J Physiol. 1965 Apr;177:463–482. doi: 10.1113/jphysiol.1965.sp007605. [DOI] [PMC free article] [PubMed] [Google Scholar]
- FATT P., KATZ B. An analysis of the end-plate potential recorded with an intracellular electrode. J Physiol. 1951 Nov 28;115(3):320–370. doi: 10.1113/jphysiol.1951.sp004675. [DOI] [PMC free article] [PubMed] [Google Scholar]
- Glavinović M. I. Changes in miniature end-plate currents due to high potassium and calcium at the frog neuromuscular junction. Synapse. 1988;2(6):636–643. doi: 10.1002/syn.890020610. [DOI] [PubMed] [Google Scholar]
- Heinonen E., Jansson S. E., Tolppanen E. M. Independent release of supranormal acetylcholine quanta at the rat neuromuscular junction. Neuroscience. 1982 Jan;7(1):21–24. doi: 10.1016/0306-4522(82)90149-x. [DOI] [PubMed] [Google Scholar]
- Hess G., Kuhnt U., Voronin L. L. Quantal analysis of paired-pulse facilitation in guinea pig hippocampal slices. Neurosci Lett. 1987 Jun 15;77(2):187–192. doi: 10.1016/0304-3940(87)90584-2. [DOI] [PubMed] [Google Scholar]
- Hubbard J. I., Jones S. F., Landau E. M. An examination of the effects of osmotic pressure changes upon transmitter release from mammalian motor nerve terminals. J Physiol. 1968 Aug;197(3):639–657. doi: 10.1113/jphysiol.1968.sp008579. [DOI] [PMC free article] [PubMed] [Google Scholar]
- KATZ B., THESLEFF S. On the factors which determine the amplitude of the miniature end-plate potential. J Physiol. 1957 Jul 11;137(2):267–278. doi: 10.1113/jphysiol.1957.sp005811. [DOI] [PMC free article] [PubMed] [Google Scholar]
- Kaufman R., Rogers G. A., Fehlmann C., Parsons S. M. Fractional vesamicol receptor occupancy and acetylcholine active transport inhibition in synaptic vesicles. Mol Pharmacol. 1989 Sep;36(3):452–458. [PubMed] [Google Scholar]
- Koyano K., Kuba K., Minota S. Long-term potentiation of transmitter release induced by repetitive presynaptic activities in bull-frog sympathetic ganglia. J Physiol. 1985 Feb;359:219–233. doi: 10.1113/jphysiol.1985.sp015582. [DOI] [PMC free article] [PubMed] [Google Scholar]
- Large W. A., Rang H. P. Factors affecting the rate of incorporation of a false transmitter into mammalian motor nerve terminals. J Physiol. 1978 Dec;285:1–24. doi: 10.1113/jphysiol.1978.sp012553. [DOI] [PMC free article] [PubMed] [Google Scholar]
- Large W. A., Rang H. P. Variability of transmitter quanta released during incorporation of a false transmitter into cholinergic nerve terminals. J Physiol. 1978 Dec;285:25–34. doi: 10.1113/jphysiol.1978.sp012554. [DOI] [PMC free article] [PubMed] [Google Scholar]
- Lupa M. T., Tabti N., Thesleff S., Vyskocil F., Yu S. P. The nature and origin of calcium-insensitive miniature end-plate potentials at rodent neuromuscular junctions. J Physiol. 1986 Dec;381:607–618. doi: 10.1113/jphysiol.1986.sp016346. [DOI] [PMC free article] [PubMed] [Google Scholar]
- Molenaar P. C., Oen B. S., Polak R. L. Effect of chloride ions on giant miniature end-plate potentials at the frog neuromuscular junction. J Physiol. 1987 Feb;383:143–152. doi: 10.1113/jphysiol.1987.sp016401. [DOI] [PMC free article] [PubMed] [Google Scholar]
- Rylett R. J. Synaptosomal "membrane-bound" choline acetyltransferase is most sensitive to inhibition by choline mustard. J Neurochem. 1989 Mar;52(3):869–875. doi: 10.1111/j.1471-4159.1989.tb02535.x. [DOI] [PubMed] [Google Scholar]
- Schmidt H., Schmitt O. Effect of aconitine on the sodium permeability of the node of Ranvier. Pflugers Arch. 1974 Jun 11;349(2):133–148. doi: 10.1007/BF00586624. [DOI] [PubMed] [Google Scholar]
- Shimoni Y., Alnaes E., Rahamimoff R. Is hyperosmotic neurosecretion from motor nerve endings a calcium-dependent process? Nature. 1977 May 12;267(5607):170–172. doi: 10.1038/267170a0. [DOI] [PubMed] [Google Scholar]
- Van Wilgenburg H. The effect of prednisolone on neuromuscular transmission in the rat diaphragm. Eur J Pharmacol. 1979 May 15;55(4):355–361. doi: 10.1016/0014-2999(79)90109-2. [DOI] [PubMed] [Google Scholar]
- Van der Kloot W. 2-(4-phenylpiperidino) cyclohexanol (AH5183) decreases quantal size at the frog neuromuscular junction. Pflugers Arch. 1986 Jan;406(1):83–85. doi: 10.1007/BF00582958. [DOI] [PubMed] [Google Scholar]
- Van der Kloot W. Pretreatment with hypertonic solutions increases quantal size at the frog neuromuscular junction. J Neurophysiol. 1987 May;57(5):1536–1554. doi: 10.1152/jn.1987.57.5.1536. [DOI] [PubMed] [Google Scholar]
- Van der Kloot W. Statistical and graphical methods for testing the hypothesis that quanta are made up of subunits. J Neurosci Methods. 1989 Feb;27(1):81–89. doi: 10.1016/0165-0270(89)90054-x. [DOI] [PubMed] [Google Scholar]
- Van der Kloot W., Van der Kloot T. E. Activity increases quantal size at the frog neuromuscular junction. Experientia. 1985 Jan 15;41(1):47–48. doi: 10.1007/BF02005866. [DOI] [PubMed] [Google Scholar]
- Veldsema-Currie R. D., Van Wilgenburg H., Labruyère W. T., Langemeijer M. W. Presynaptic, facilitatory effects of the corticosteroid dexamethasone in rat diaphragm: modulation by beta-bungarotoxin. Brain Res. 1984 Mar 5;294(2):315–325. doi: 10.1016/0006-8993(84)91043-6. [DOI] [PubMed] [Google Scholar]
- Vrbová G., Wareham A. C. Effects of nerve activity on the postsynaptic membrane of skeletal muscle. Brain Res. 1976 Dec 24;118(3):371–382. doi: 10.1016/0006-8993(76)90305-x. [DOI] [PubMed] [Google Scholar]
- Whitton P. S., Marshall I. G., Parsons S. M. Reduction of quantal size by vesamicol (AH5183), an inhibitor of vesicular acetylcholine storage. Brain Res. 1986 Oct 15;385(1):189–192. doi: 10.1016/0006-8993(86)91565-9. [DOI] [PubMed] [Google Scholar]
- Yamamura H. I., Snyder S. H. High affinity transport of choline into synaptosomes of rat brain. J Neurochem. 1973 Dec;21(6):1355–1374. doi: 10.1111/j.1471-4159.1973.tb06022.x. [DOI] [PubMed] [Google Scholar]
- van der Kloot W., van der Kloot T. E. Catecholamines, insulin and ACTH increase quantal size at the frog neuromuscular junction. Brain Res. 1986 Jun 25;376(2):378–381. doi: 10.1016/0006-8993(86)90203-9. [DOI] [PubMed] [Google Scholar]