Abstract
1. The pH dependence of the Ca2+ sensitivities of isometric tension and stiffness was investigated at 10 and 15 degrees C in skinned single fibres from rat and rabbit fast- and slow-twitch skeletal muscles. Stiffness was determined by recording the tension responses to sinusoidal length changes (3.3 kHz); peak-to-peak amplitude of the length change was monitored by laser diffraction and averaged approximately 1.3 nm (half-sarcomere)-1. We have assumed that stiffness provides a measure of the number of cross-bridge attachments to actin. 2. At maximal Ca2+ activation, stiffness was depressed by 22 +/- 2% (mean +/- S.E.M.) in fast-twitch fibres but was unchanged in slow-twitch fibres when pH was lowered from 7.00 to 6.20. As reported previously, maximum tension was depressed by 20-45% at low pH, with the effect being greater in fast-twitch compared to slow-twitch fibres. 3. In fast-twitch fibres at 10 and 15 degrees C the Ca2+ concentrations for half-maximal activation of tension and stiffness were increased at low pH. In slow-twitch fibres, similar effects were observed at 15 degrees C, but at 10 degrees C there were no changes in the Ca2+ sensitivities of tension and stiffness when pH was lowered. 4. Linear relationships between relative tension and relative stiffness were obtained at all temperatures, with slopes of 1.04 +/- 0.01 at pH 7.00 and 0.76 +/- 0.01 at pH 6.20 in fast- and slow-twitch fibres, indicating that force per cross-bridge attachment is similarly reduced at low pH in both types of fibres. 5. In both fast- and slow-twitch fibres, rigor tension (no added ATP or creatine phosphate; pCa 9.0) was depressed by 35 +/- 7% and stiffness by 12 +/- 4% when pH was reduced from 7.00 to 6.20. Since cross-bridge cycling is inhibited in rigor the effect of low pH to depress rigor tension suggests that pH directly modulates the strength of the bond formed between actin and myosin. 6. The effect of low pH to reduce the apparent number of cross-bridge attachments during maximal Ca2+ activation in fast- but not slow-twitch fibres could account for the greater H(+)-induced depression of maximum force in fast-twitch fibres. In both fibre types, the decrease in the number of cross-bridge attachments at submaximal concentrations of Ca2+ may in part account for the loss in Ca2+ sensitivity of tension at low pH, due perhaps to a reduction in co-operative activation of the thin filament by bound cross-bridges.
Full text
PDF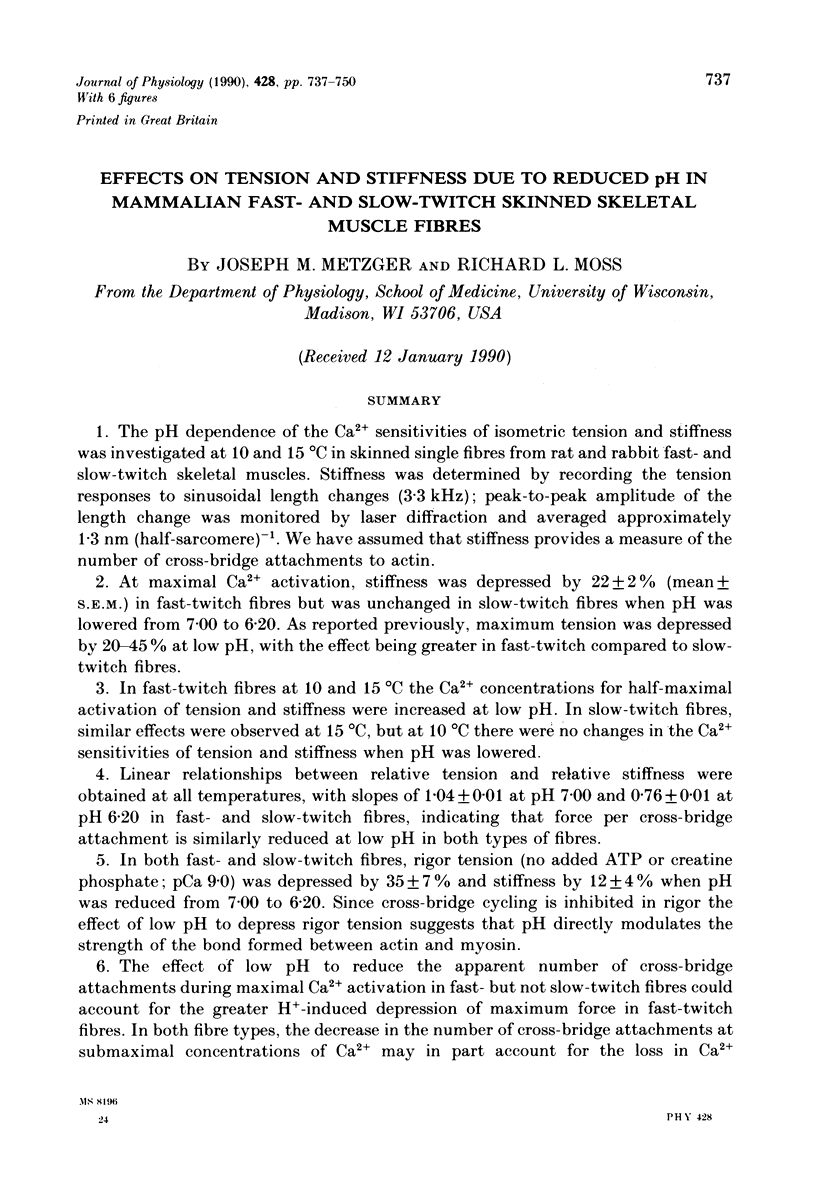
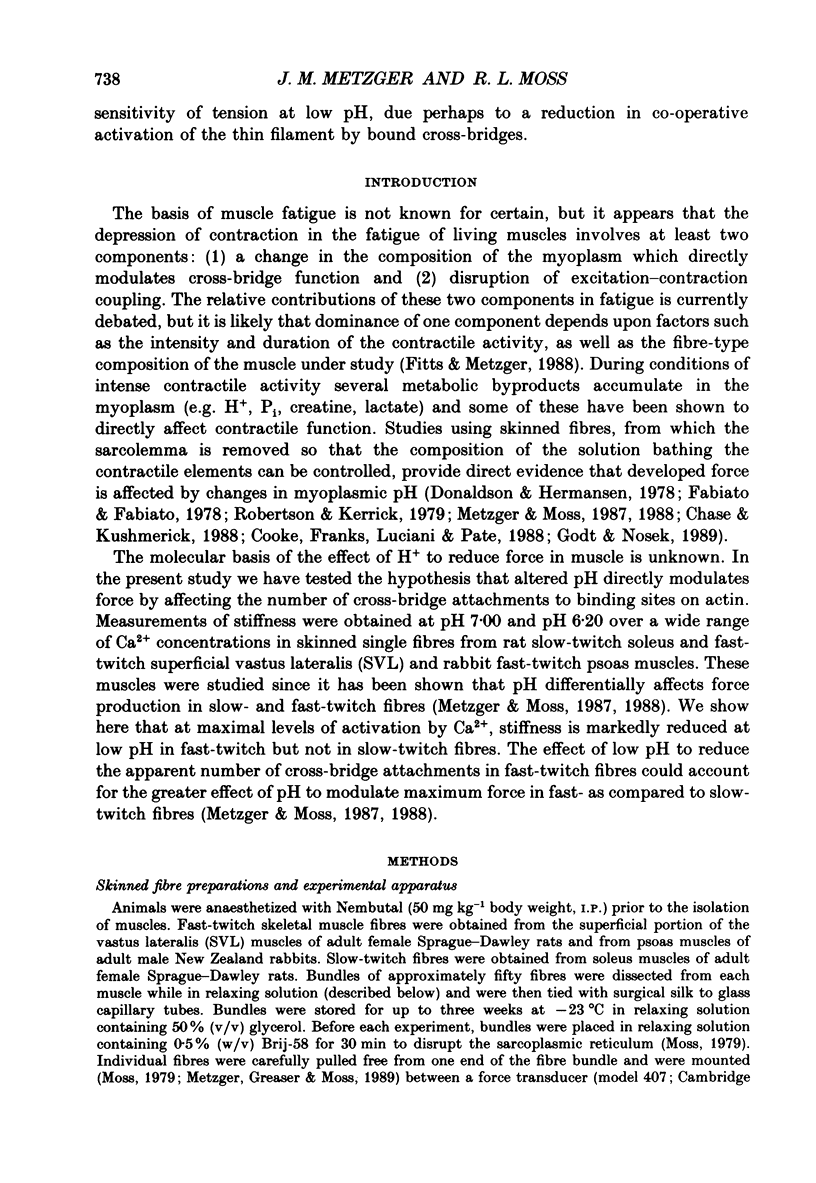
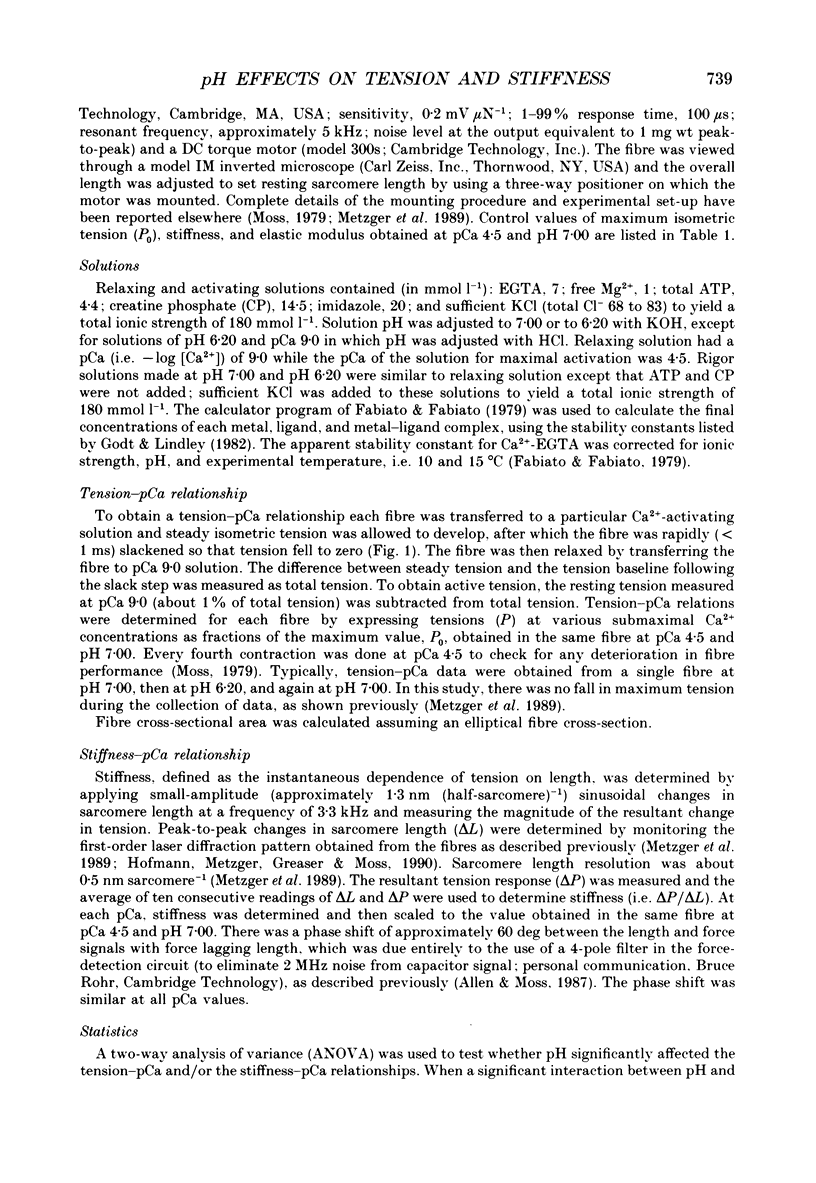
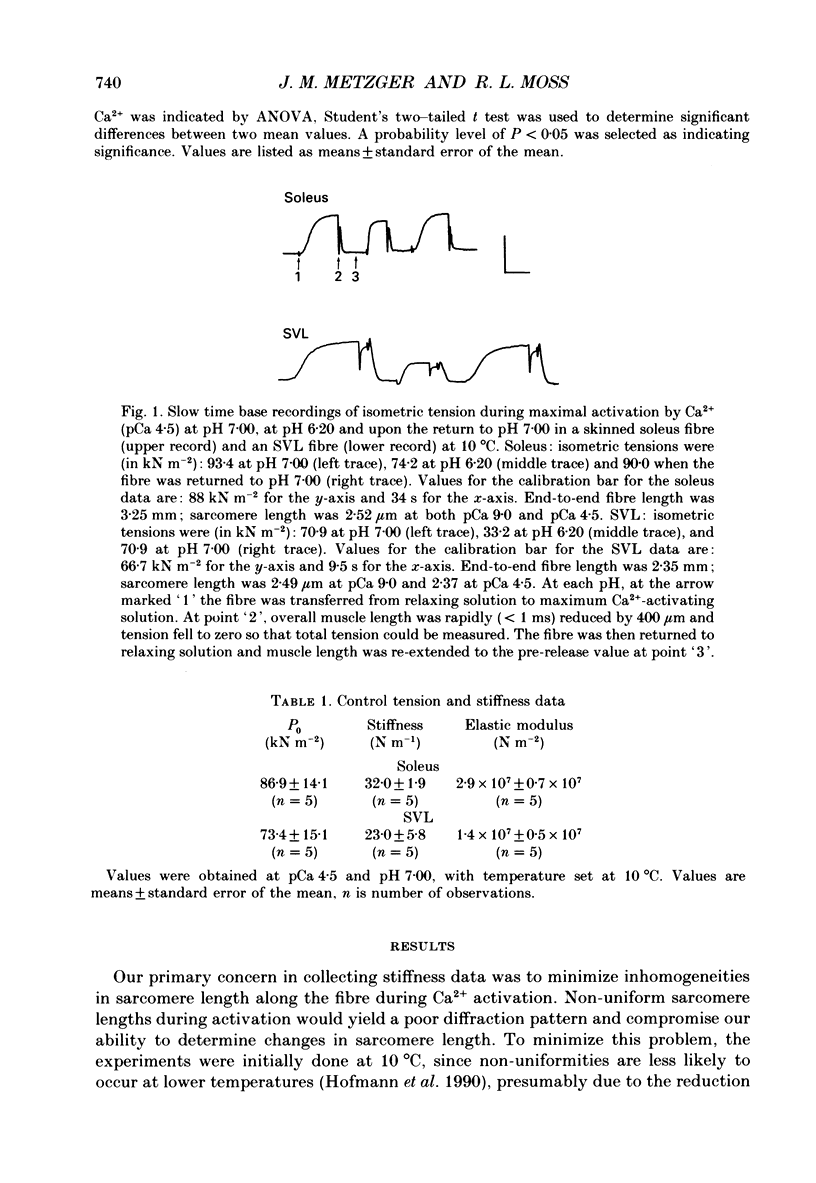
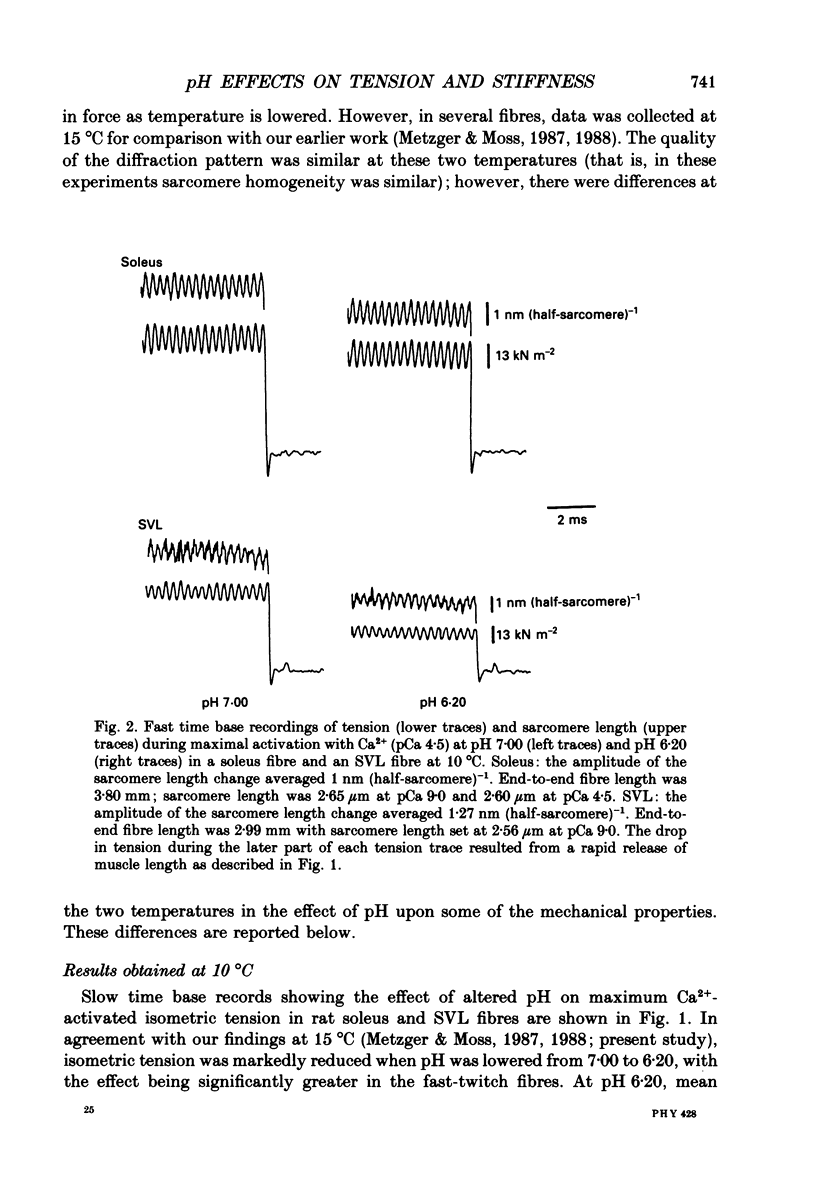
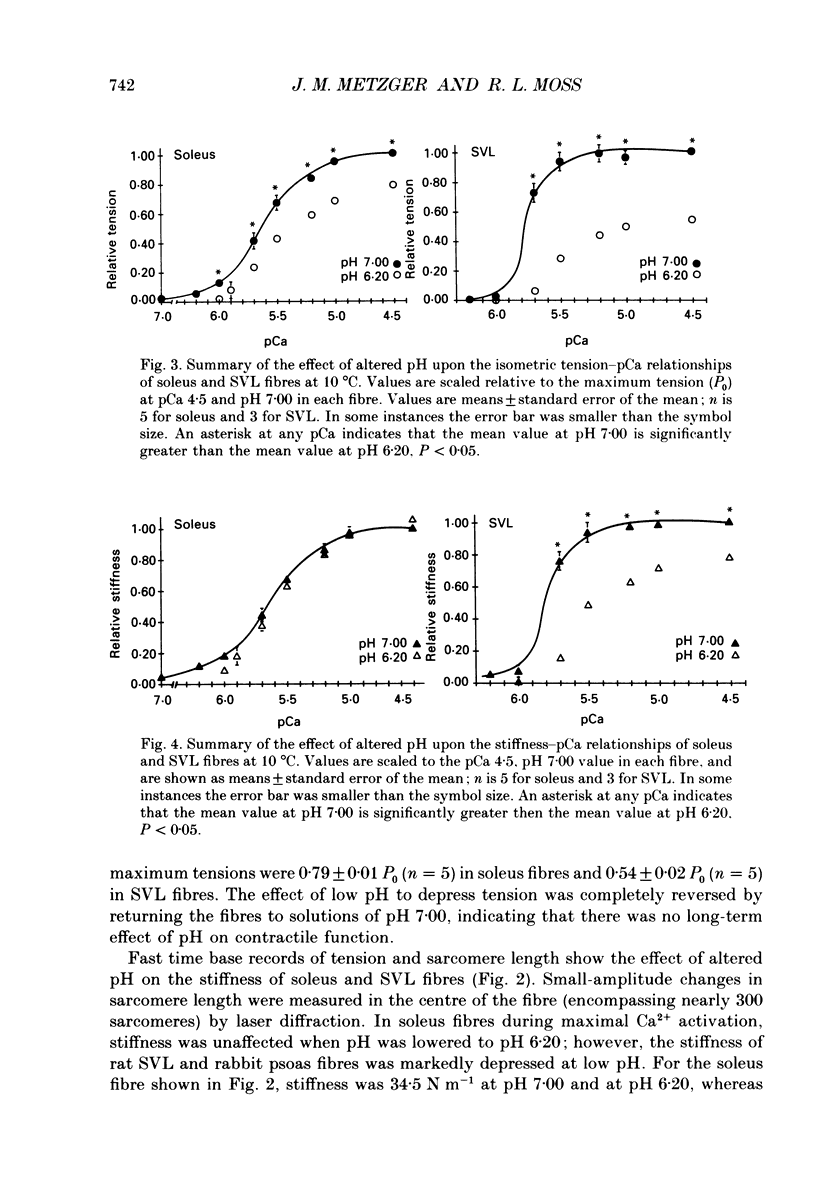
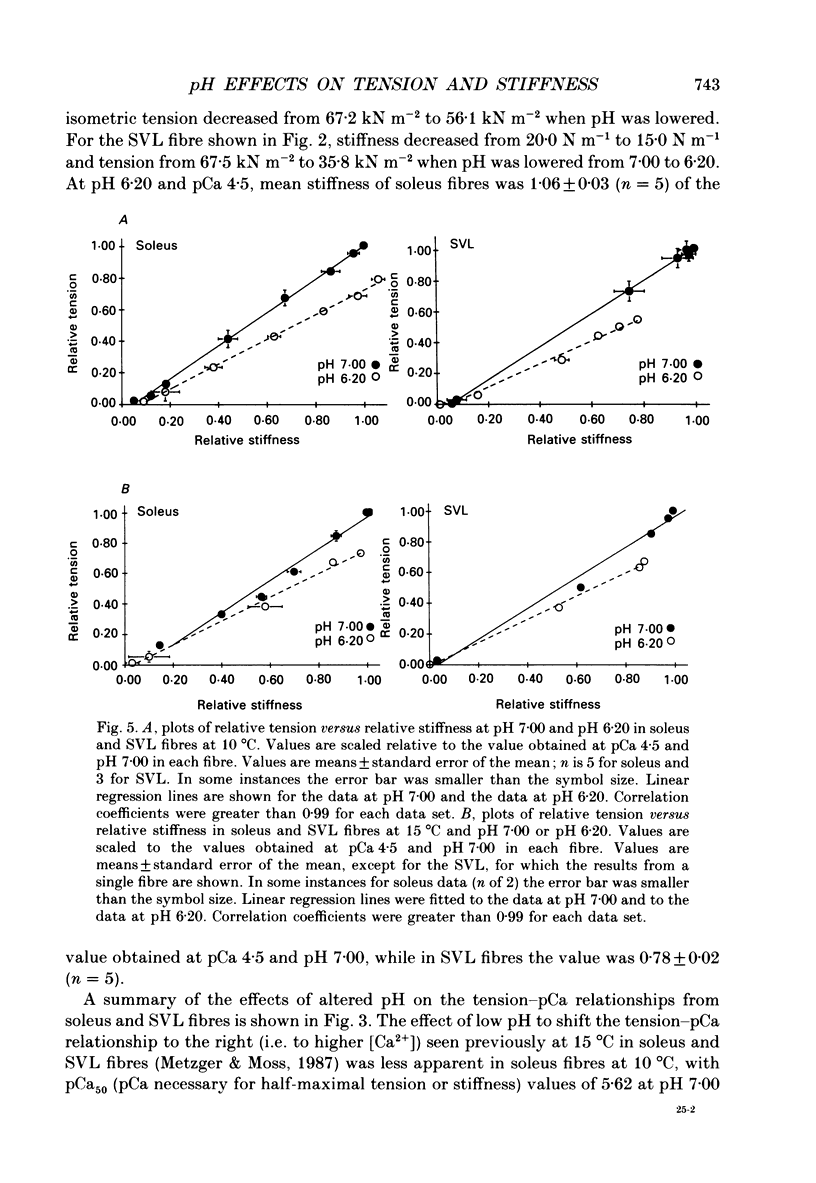
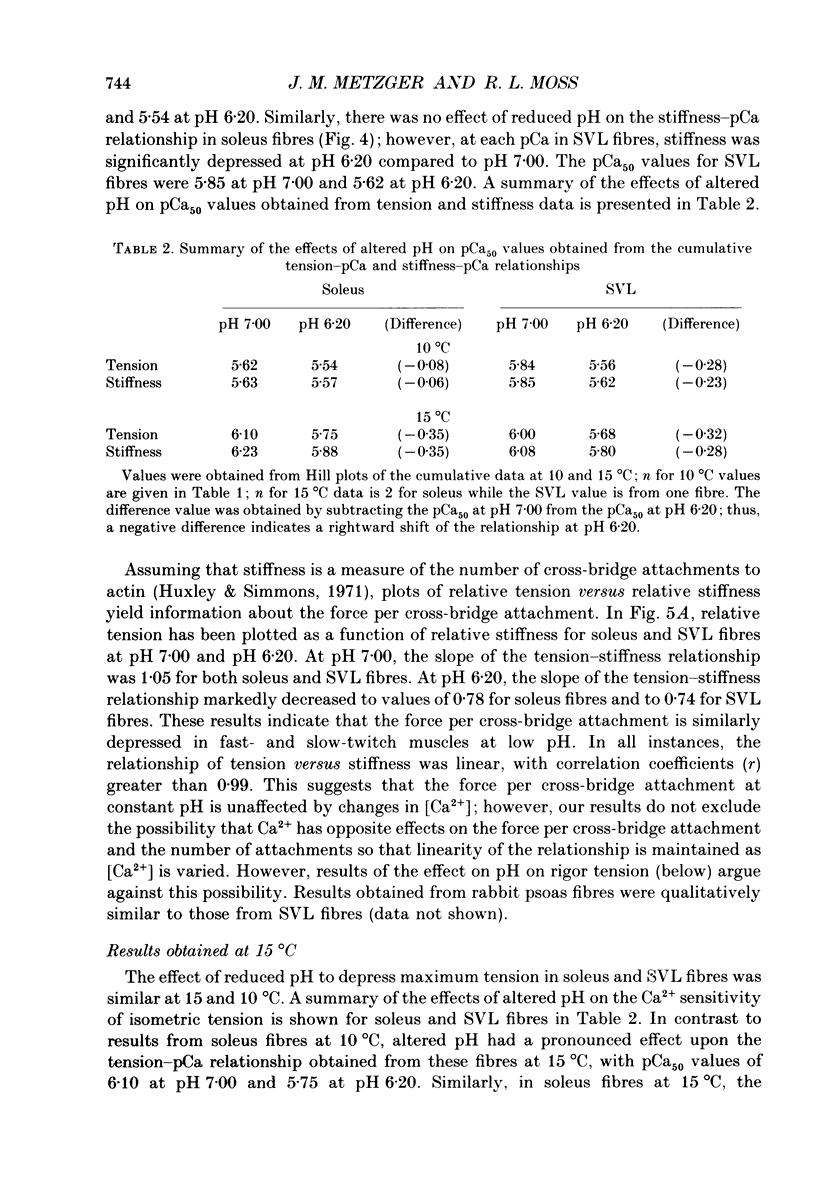
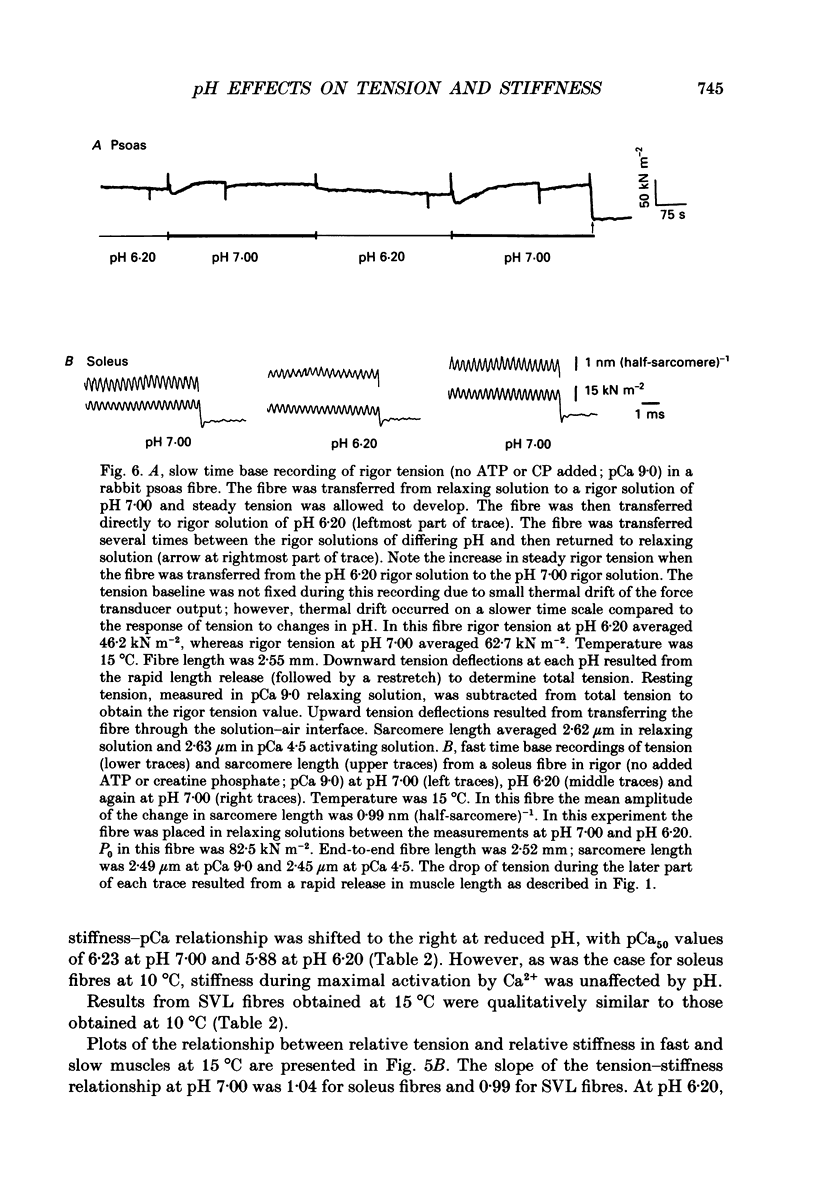
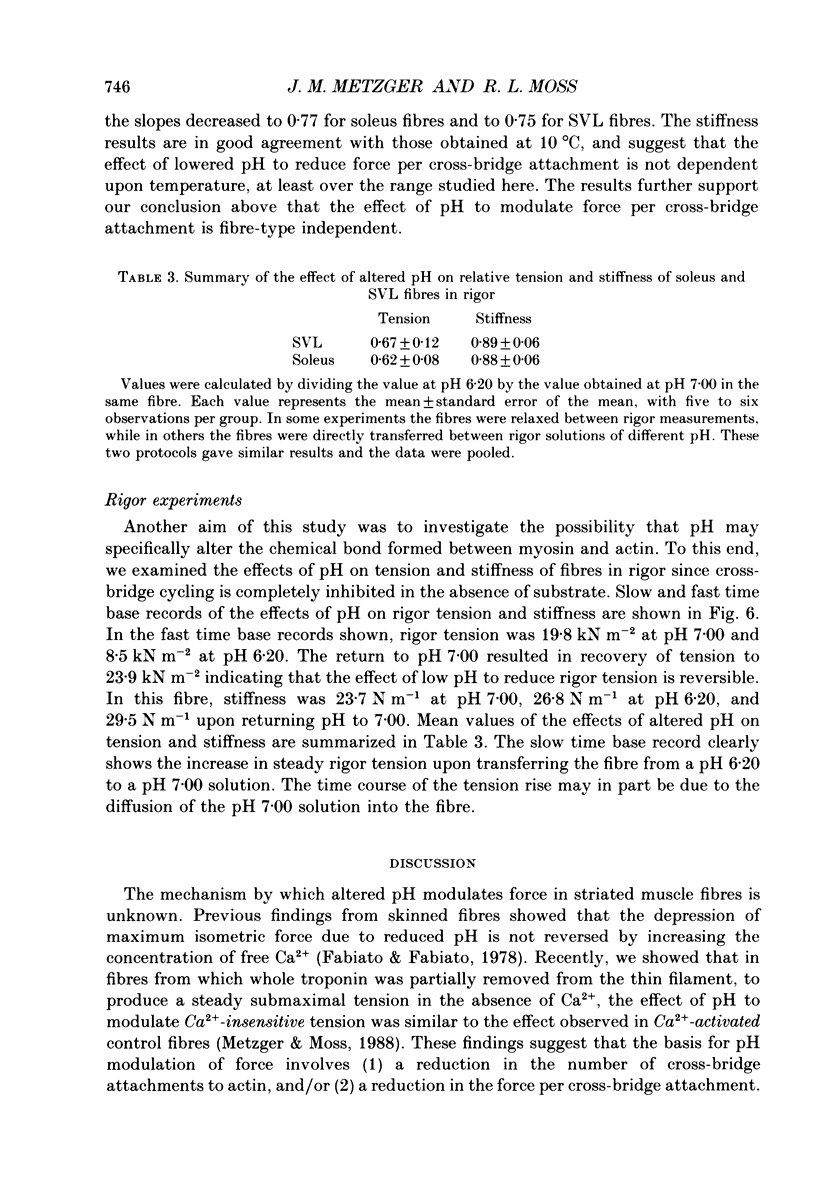
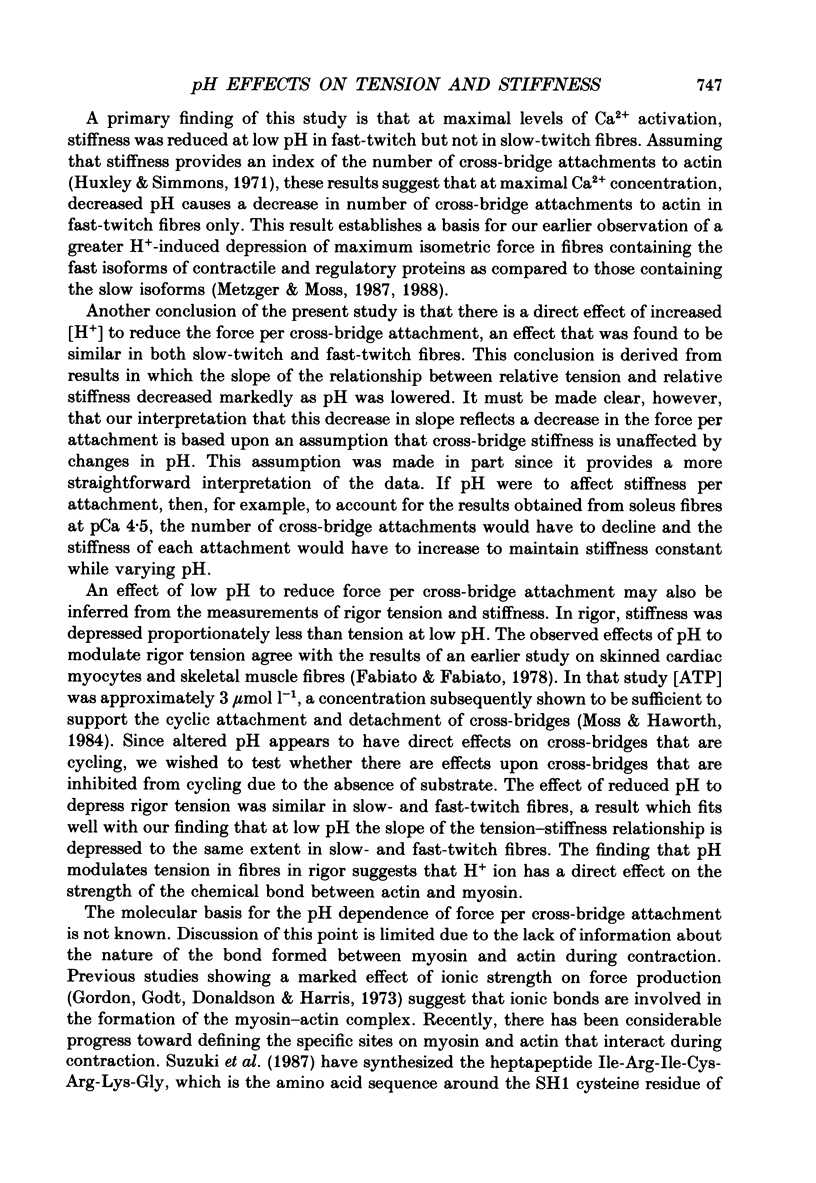
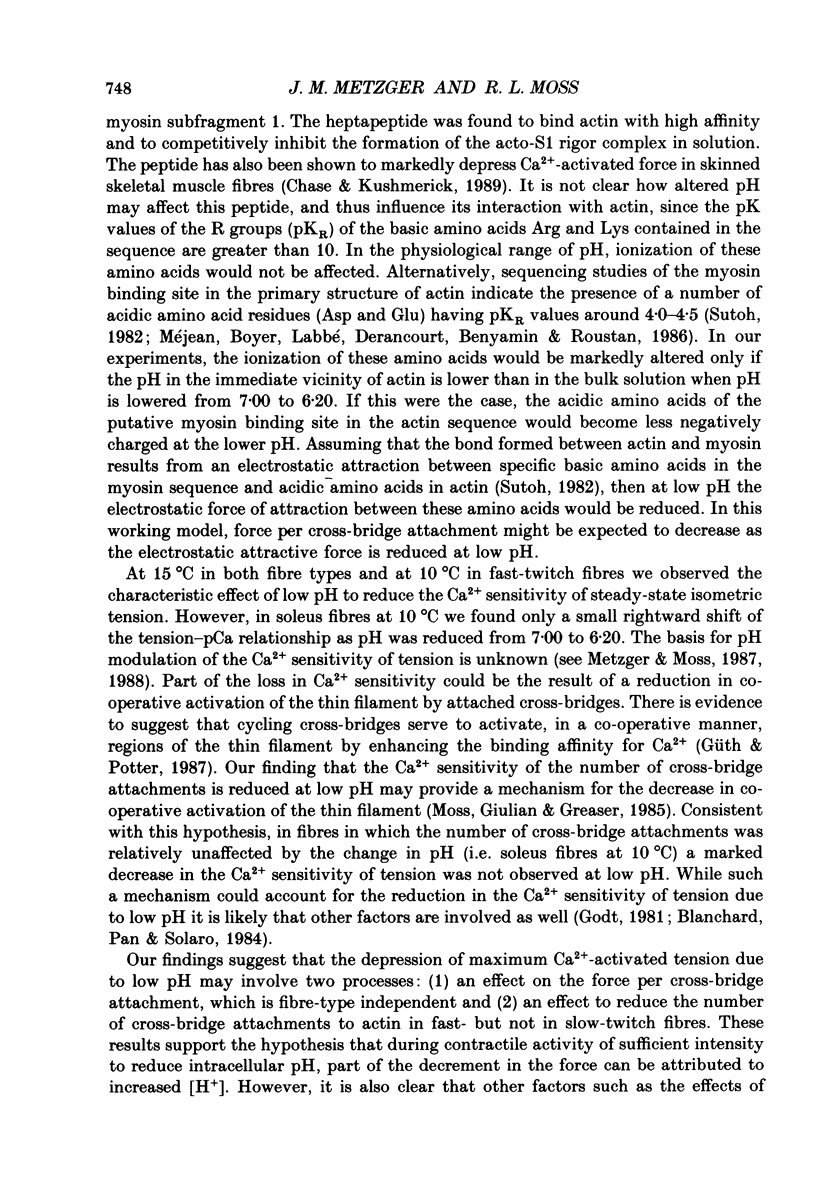
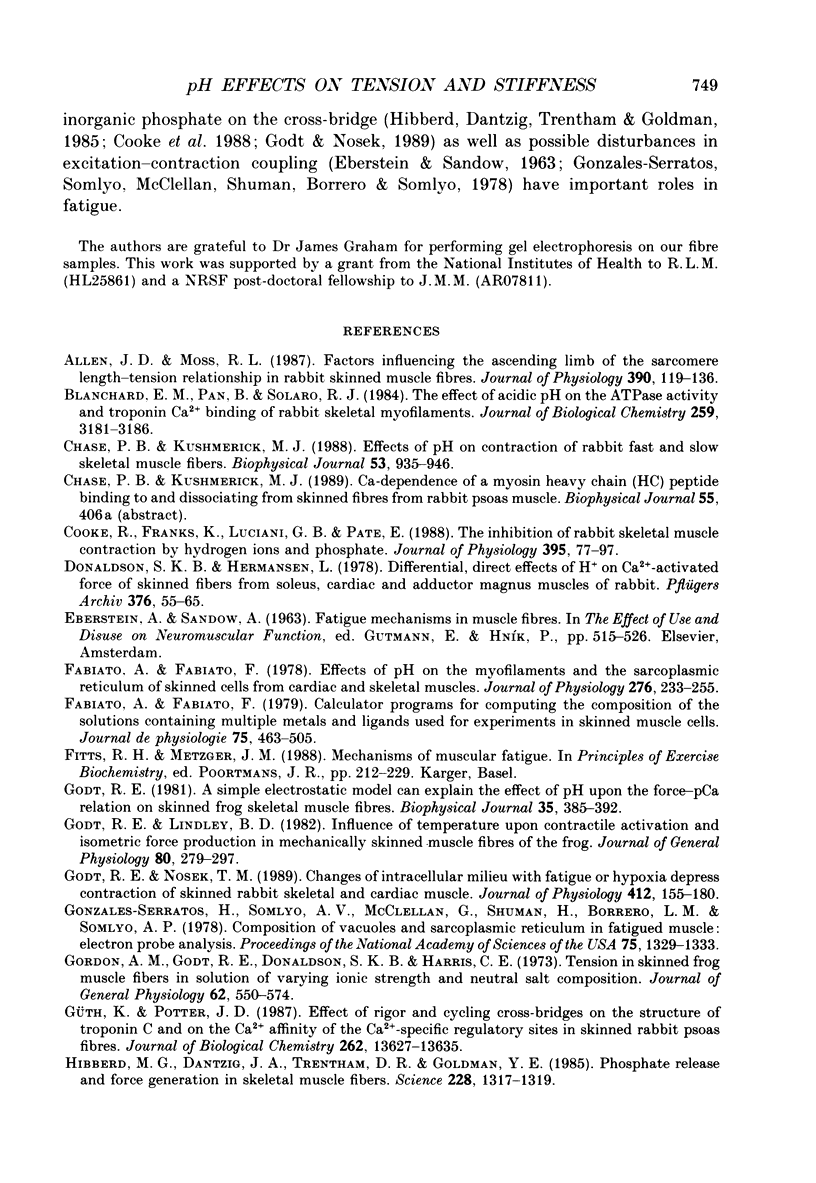
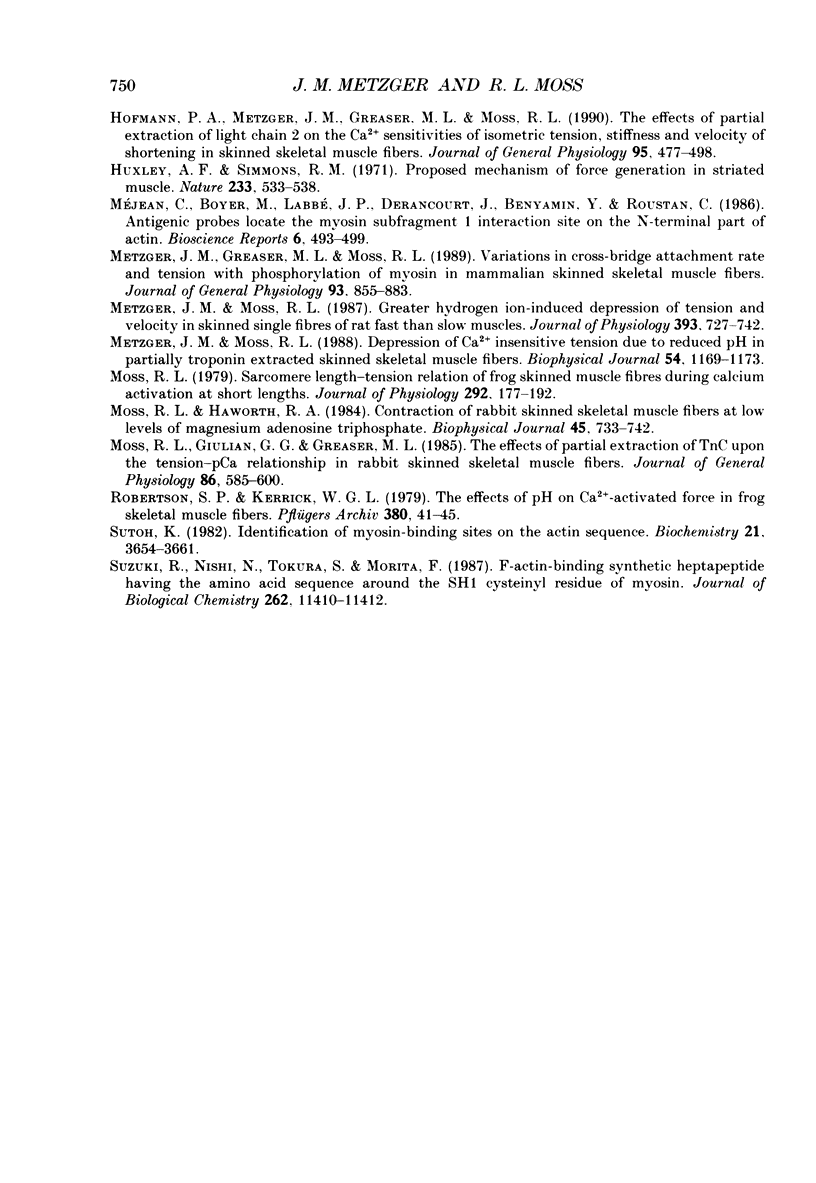
Selected References
These references are in PubMed. This may not be the complete list of references from this article.
- Allen J. D., Moss R. L. Factors influencing the ascending limb of the sarcomere length-tension relationship in rabbit skinned muscle fibres. J Physiol. 1987 Sep;390:119–136. doi: 10.1113/jphysiol.1987.sp016689. [DOI] [PMC free article] [PubMed] [Google Scholar]
- Blanchard E. M., Pan B. S., Solaro R. J. The effect of acidic pH on the ATPase activity and troponin Ca2+ binding of rabbit skeletal myofilaments. J Biol Chem. 1984 Mar 10;259(5):3181–3186. [PubMed] [Google Scholar]
- Chase P. B., Kushmerick M. J. Effects of pH on contraction of rabbit fast and slow skeletal muscle fibers. Biophys J. 1988 Jun;53(6):935–946. doi: 10.1016/S0006-3495(88)83174-6. [DOI] [PMC free article] [PubMed] [Google Scholar]
- Cooke R., Franks K., Luciani G. B., Pate E. The inhibition of rabbit skeletal muscle contraction by hydrogen ions and phosphate. J Physiol. 1988 Jan;395:77–97. doi: 10.1113/jphysiol.1988.sp016909. [DOI] [PMC free article] [PubMed] [Google Scholar]
- Donaldson S. K., Hermansen L., Bolles L. Differential, direct effects of H+ on Ca2+ -activated force of skinned fibers from the soleus, cardiac and adductor magnus muscles of rabbits. Pflugers Arch. 1978 Aug 25;376(1):55–65. doi: 10.1007/BF00585248. [DOI] [PubMed] [Google Scholar]
- Fabiato A., Fabiato F. Calculator programs for computing the composition of the solutions containing multiple metals and ligands used for experiments in skinned muscle cells. J Physiol (Paris) 1979;75(5):463–505. [PubMed] [Google Scholar]
- Fabiato A., Fabiato F. Effects of pH on the myofilaments and the sarcoplasmic reticulum of skinned cells from cardiace and skeletal muscles. J Physiol. 1978 Mar;276:233–255. doi: 10.1113/jphysiol.1978.sp012231. [DOI] [PMC free article] [PubMed] [Google Scholar]
- Godt R. E. A simple electrostatic model can explain the effect of pH upon the force-pCa relation of skinned frog skeletal muscle fibers. Biophys J. 1981 Aug;35(2):385–392. doi: 10.1016/S0006-3495(81)84797-2. [DOI] [PMC free article] [PubMed] [Google Scholar]
- Godt R. E., Lindley B. D. Influence of temperature upon contractile activation and isometric force production in mechanically skinned muscle fibers of the frog. J Gen Physiol. 1982 Aug;80(2):279–297. doi: 10.1085/jgp.80.2.279. [DOI] [PMC free article] [PubMed] [Google Scholar]
- Godt R. E., Nosek T. M. Changes of intracellular milieu with fatigue or hypoxia depress contraction of skinned rabbit skeletal and cardiac muscle. J Physiol. 1989 May;412:155–180. doi: 10.1113/jphysiol.1989.sp017609. [DOI] [PMC free article] [PubMed] [Google Scholar]
- Gonzalez-Serratos H., Somlyo A. V., McClellan G., Shuman H., Borrero L. M., Somlyo A. P. Composition of vacuoles and sarcoplasmic reticulum in fatigued muscle: electron probe analysis. Proc Natl Acad Sci U S A. 1978 Mar;75(3):1329–1333. doi: 10.1073/pnas.75.3.1329. [DOI] [PMC free article] [PubMed] [Google Scholar]
- Gordon A. M., Godt R. E., Donaldson S. K., Harris C. E. Tension in skinned frog muscle fibers in solutions of varying ionic strength and neutral salt composition. J Gen Physiol. 1973 Nov;62(5):550–574. doi: 10.1085/jgp.62.5.550. [DOI] [PMC free article] [PubMed] [Google Scholar]
- Güth K., Potter J. D. Effect of rigor and cycling cross-bridges on the structure of troponin C and on the Ca2+ affinity of the Ca2+-specific regulatory sites in skinned rabbit psoas fibers. J Biol Chem. 1987 Oct 5;262(28):13627–13635. [PubMed] [Google Scholar]
- Hibberd M. G., Dantzig J. A., Trentham D. R., Goldman Y. E. Phosphate release and force generation in skeletal muscle fibers. Science. 1985 Jun 14;228(4705):1317–1319. doi: 10.1126/science.3159090. [DOI] [PubMed] [Google Scholar]
- Hofmann P. A., Metzger J. M., Greaser M. L., Moss R. L. Effects of partial extraction of light chain 2 on the Ca2+ sensitivities of isometric tension, stiffness, and velocity of shortening in skinned skeletal muscle fibers. J Gen Physiol. 1990 Mar;95(3):477–498. doi: 10.1085/jgp.95.3.477. [DOI] [PMC free article] [PubMed] [Google Scholar]
- Huxley A. F., Simmons R. M. Proposed mechanism of force generation in striated muscle. Nature. 1971 Oct 22;233(5321):533–538. doi: 10.1038/233533a0. [DOI] [PubMed] [Google Scholar]
- Metzger J. M., Greaser M. L., Moss R. L. Variations in cross-bridge attachment rate and tension with phosphorylation of myosin in mammalian skinned skeletal muscle fibers. Implications for twitch potentiation in intact muscle. J Gen Physiol. 1989 May;93(5):855–883. doi: 10.1085/jgp.93.5.855. [DOI] [PMC free article] [PubMed] [Google Scholar]
- Metzger J. M., Moss R. L. Depression of Ca2+ insensitive tension due to reduced pH in partially troponin-extracted skinned skeletal muscle fibers. Biophys J. 1988 Dec;54(6):1169–1173. doi: 10.1016/S0006-3495(88)83052-2. [DOI] [PMC free article] [PubMed] [Google Scholar]
- Metzger J. M., Moss R. L. Greater hydrogen ion-induced depression of tension and velocity in skinned single fibres of rat fast than slow muscles. J Physiol. 1987 Dec;393:727–742. doi: 10.1113/jphysiol.1987.sp016850. [DOI] [PMC free article] [PubMed] [Google Scholar]
- Moss R. L., Giulian G. G., Greaser M. L. The effects of partial extraction of TnC upon the tension-pCa relationship in rabbit skinned skeletal muscle fibers. J Gen Physiol. 1985 Oct;86(4):585–600. doi: 10.1085/jgp.86.4.585. [DOI] [PMC free article] [PubMed] [Google Scholar]
- Moss R. L., Haworth R. A. Contraction of rabbit skinned skeletal muscle fibers at low levels of magnesium adenosine triphosphate. Biophys J. 1984 Apr;45(4):733–742. doi: 10.1016/S0006-3495(84)84216-2. [DOI] [PMC free article] [PubMed] [Google Scholar]
- Moss R. L. Sarcomere length-tension relations of frog skinned muscle fibres during calcium activation at short lengths. J Physiol. 1979 Jul;292:177–192. doi: 10.1113/jphysiol.1979.sp012845. [DOI] [PMC free article] [PubMed] [Google Scholar]
- Méjean C., Boyer M., Labbé J. P., Derancourt J., Benyamin Y., Roustan C. Antigenic probes locate the myosin subfragment 1 interaction site on the N-terminal part of actin. Biosci Rep. 1986 May;6(5):493–499. doi: 10.1007/BF01116141. [DOI] [PubMed] [Google Scholar]
- Robertson S. P., Kerrick W. G. The effects of pH on Ca2+-activated force in frog skeletal muscle fibers. Pflugers Arch. 1979 May 15;380(1):41–45. doi: 10.1007/BF00582610. [DOI] [PubMed] [Google Scholar]
- Sutoh K. Identification of myosin-binding sites on the actin sequence. Biochemistry. 1982 Jul 20;21(15):3654–3661. doi: 10.1021/bi00258a020. [DOI] [PubMed] [Google Scholar]
- Suzuki R., Nishi N., Tokura S., Morita F. F-actin-binding synthetic heptapeptide having the amino acid sequence around the SH1 cysteinyl residue of myosin. J Biol Chem. 1987 Aug 25;262(24):11410–11412. [PubMed] [Google Scholar]