Abstract
1. The responses of megakaryocytes to adenosine diphosphate (ADP) were studied using whole-cell patch electrodes and a Ca2(+)-sensitive fluorescent dye, Fura-2. Megakaryocytes (diameter, 17-42 microns) were mechanically dissociated from the bone marrow of adult guinea-pigs and ADP (1-10 microM) was pressure-applied to megakaryocytes under recording. 2. In megakaryocytes immersed in standard saline, ADP evoked an obvious outward current at a membrane potential of -63 mV. The current was identified as a K(+)-carried current, since the reversal potential depended distinctly on the external K+ concentration, but it showed no changes after removal of external Na+. The amplitude of evoked K+ currents showed considerable intercell variation, which is presumably due to differences of current density in the membrane. 3. During application of ADP, the evoked K+ current was not sustained but slowly decayed to become negligible within 10-20 s, suggesting the appearance of desensitization. The response of the megakaryocyte to ADP recovered slowly and returned to an original level after 4-5 min of continuous washing. 4. When the intracellular free Ca2+ concentration ([Ca2+]i) was measured using the Ca2(+)-sensitive fluorescent dye, Fura-2, application of 10 microM-ADP induced an increase of [Ca2+]i by about 5-fold, which was followed by a gradual decay to the original level within 30-50 s. Roles of internal Ca2+ for activating the K+ current were confirmed by observing (1) enhancement of evoked currents by the use of internal saline containing no Ca2+ chelators and (2) generation of prolonged K+ current by application of a Ca2+ ionophore, A23187, to the megakaryocyte. 5. In a fraction of the megakaryocytes, spontaneous hyperpolarization of the resting membrane potential was observed. The hyperpolarization seemed to result from the activation of K+ channels in the membrane, which was caused by spontaneous release of Ca2+ from the internal storage site. 6. It was concluded that megakaryocytes of the guinea-pig can respond to external ADP by increasing [Ca2+]i and consequently by activating Ca2(+)-dependent K+ channels in the membrane.
Full text
PDF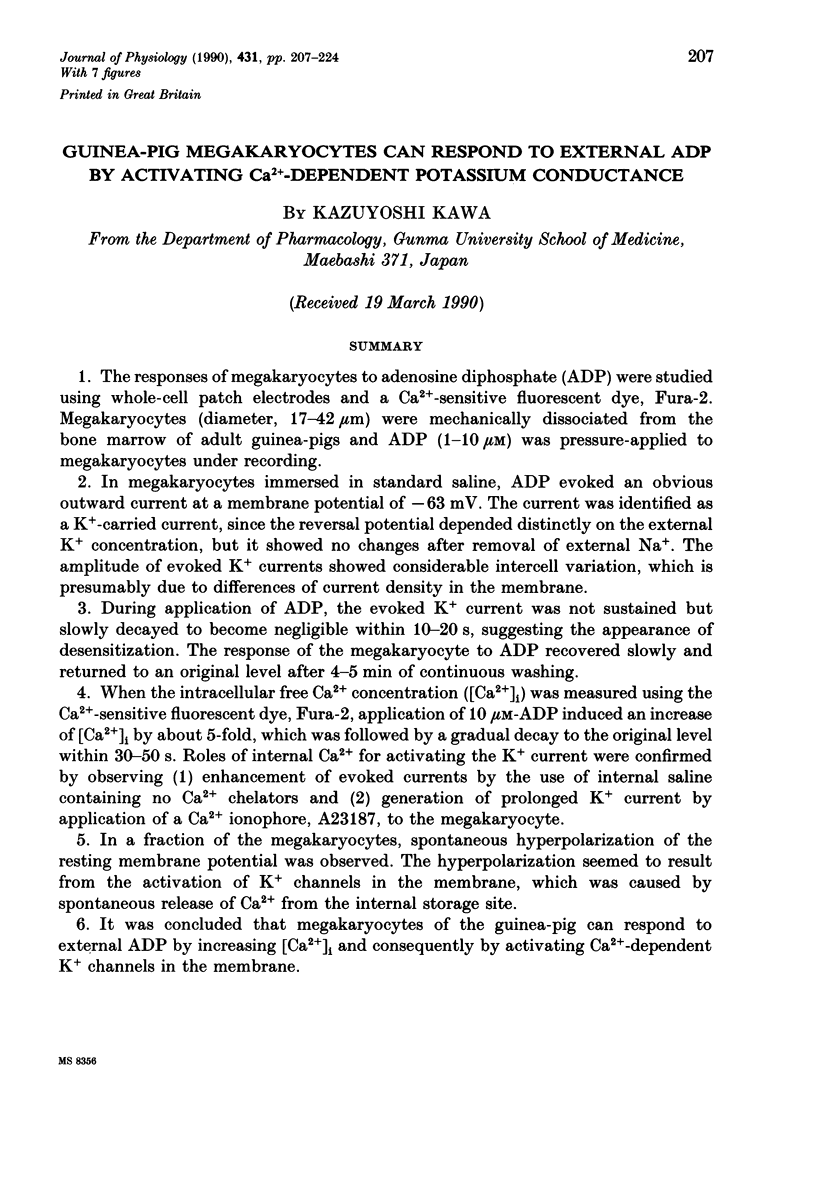
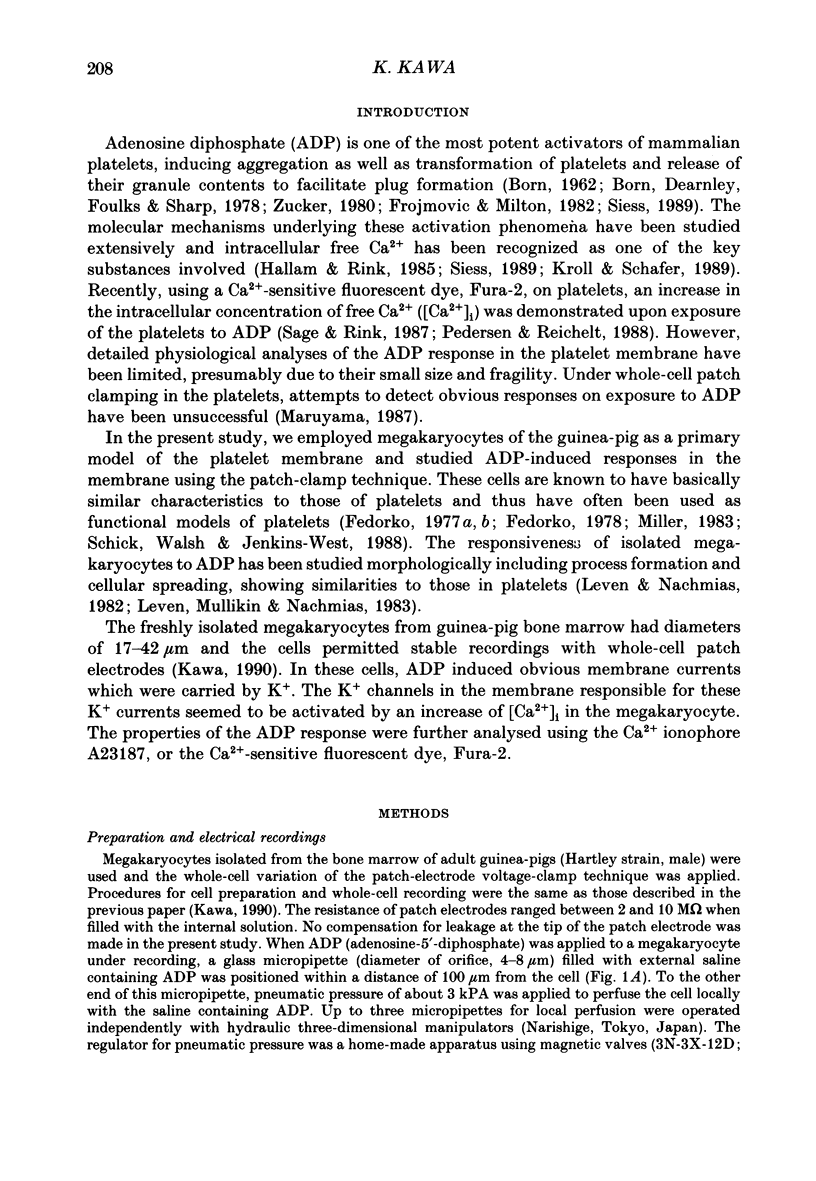
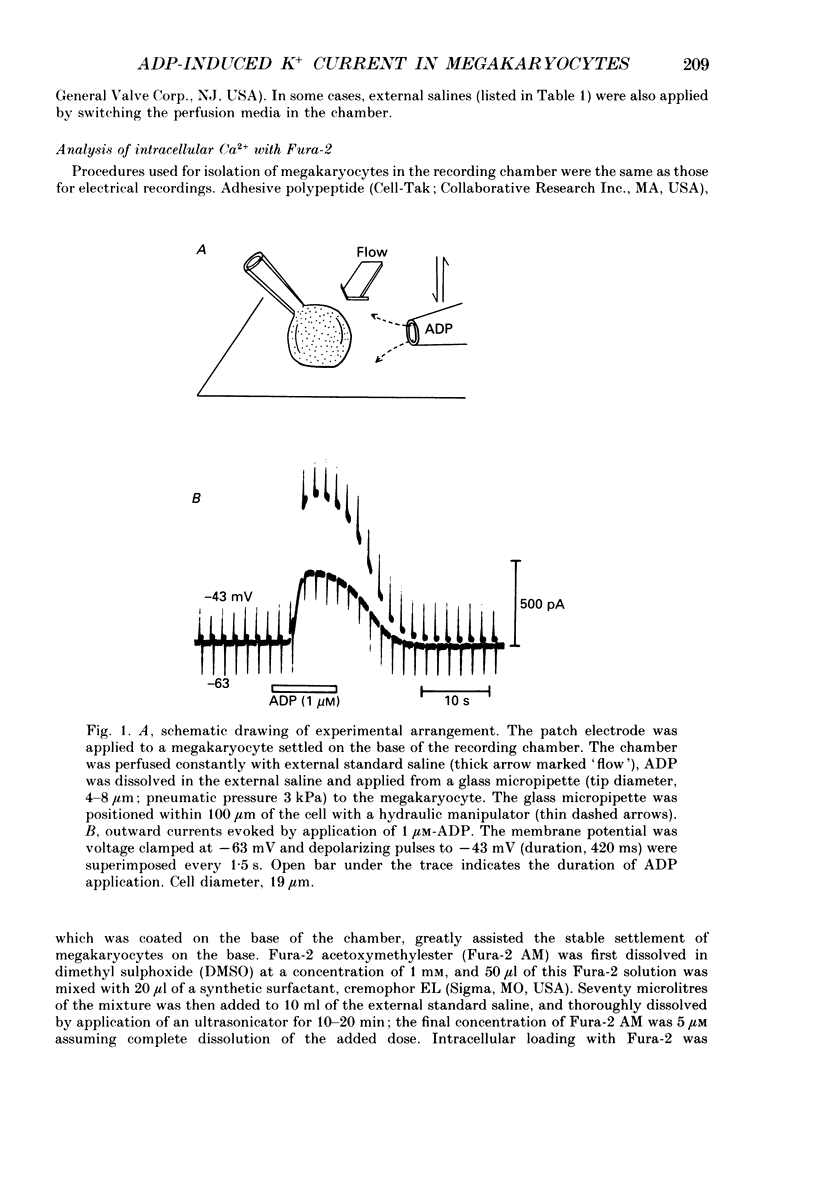
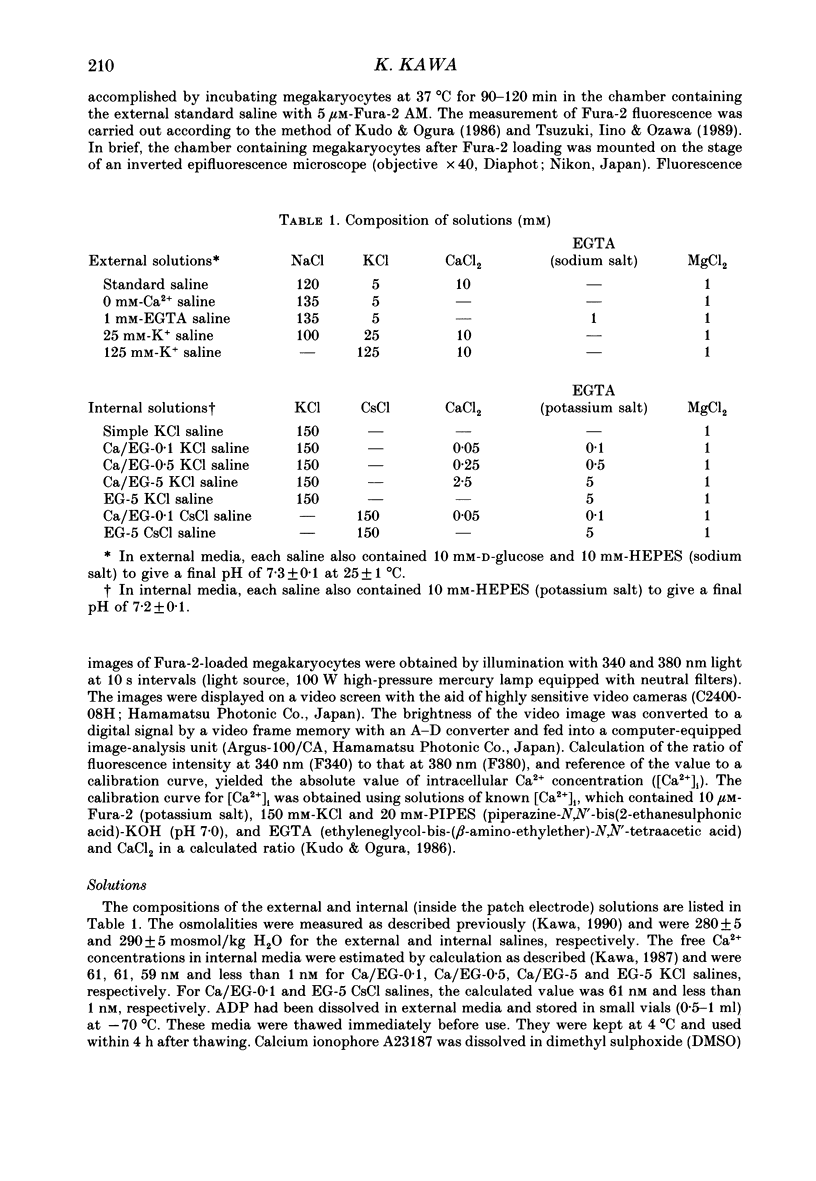
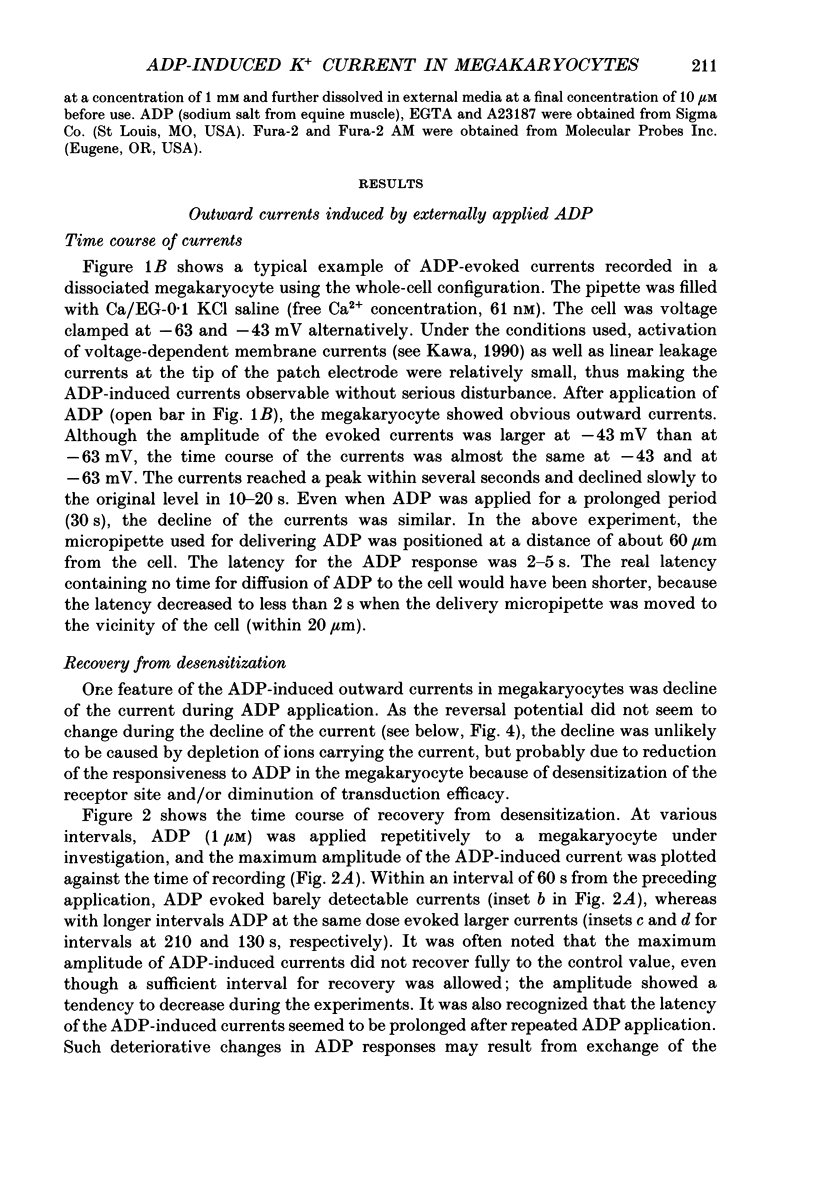
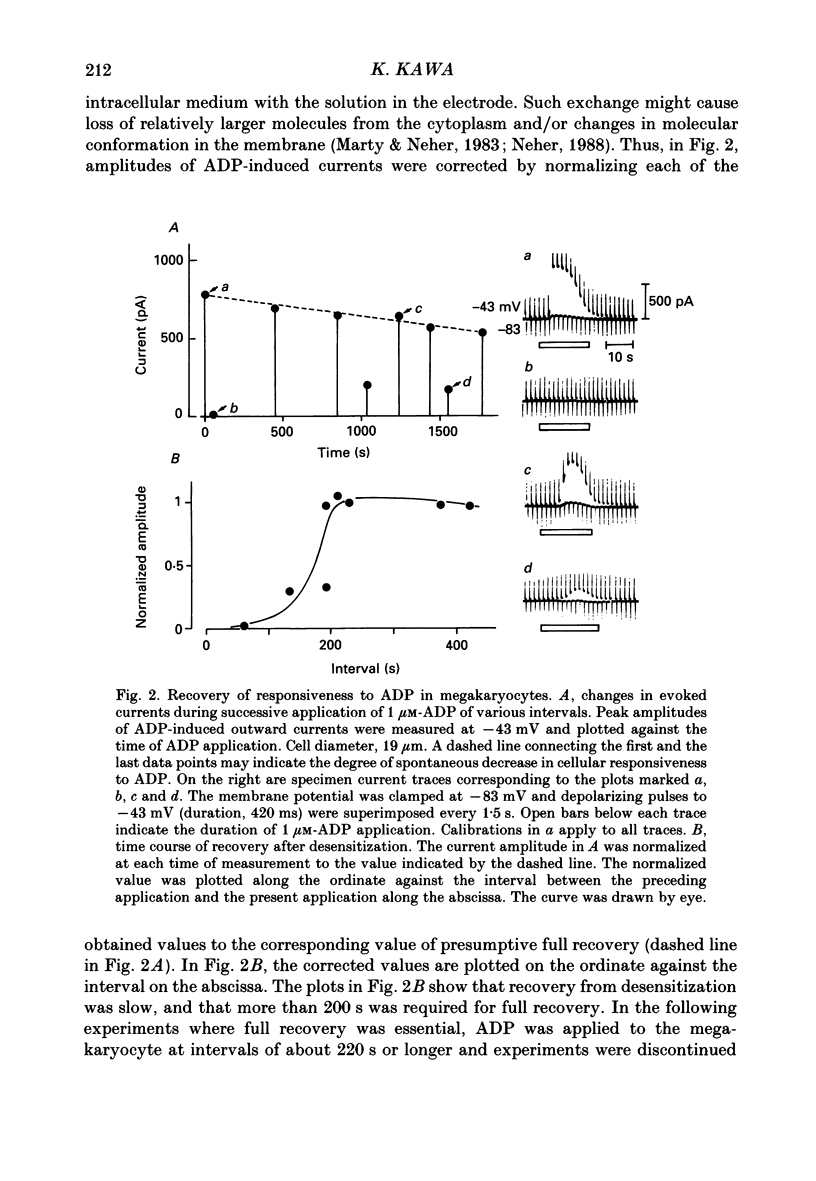
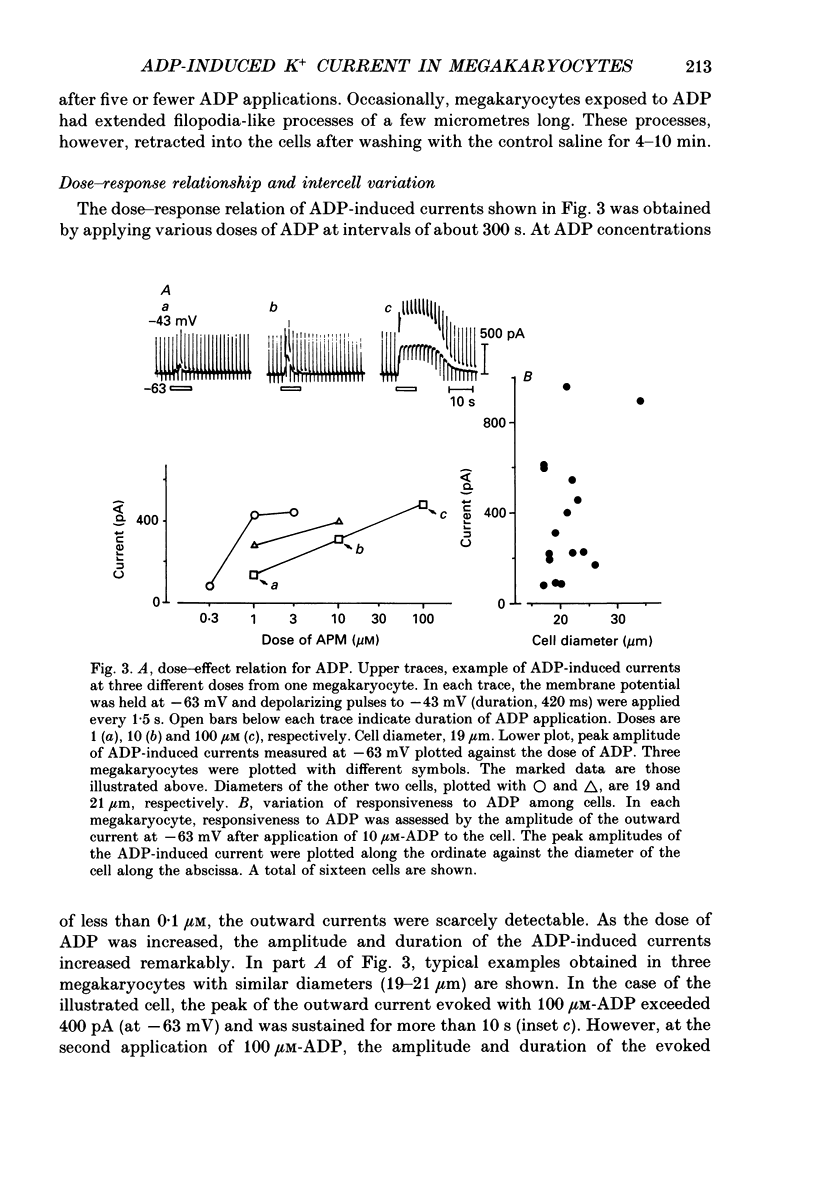
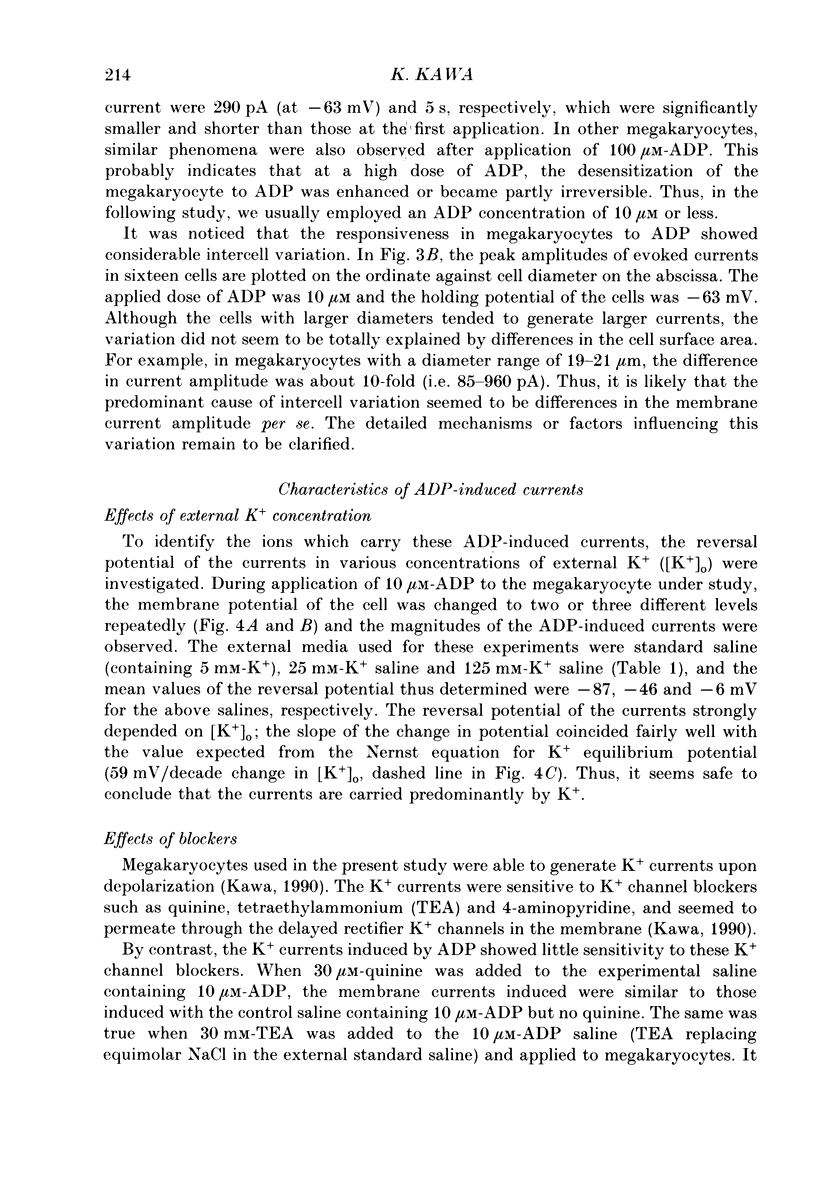
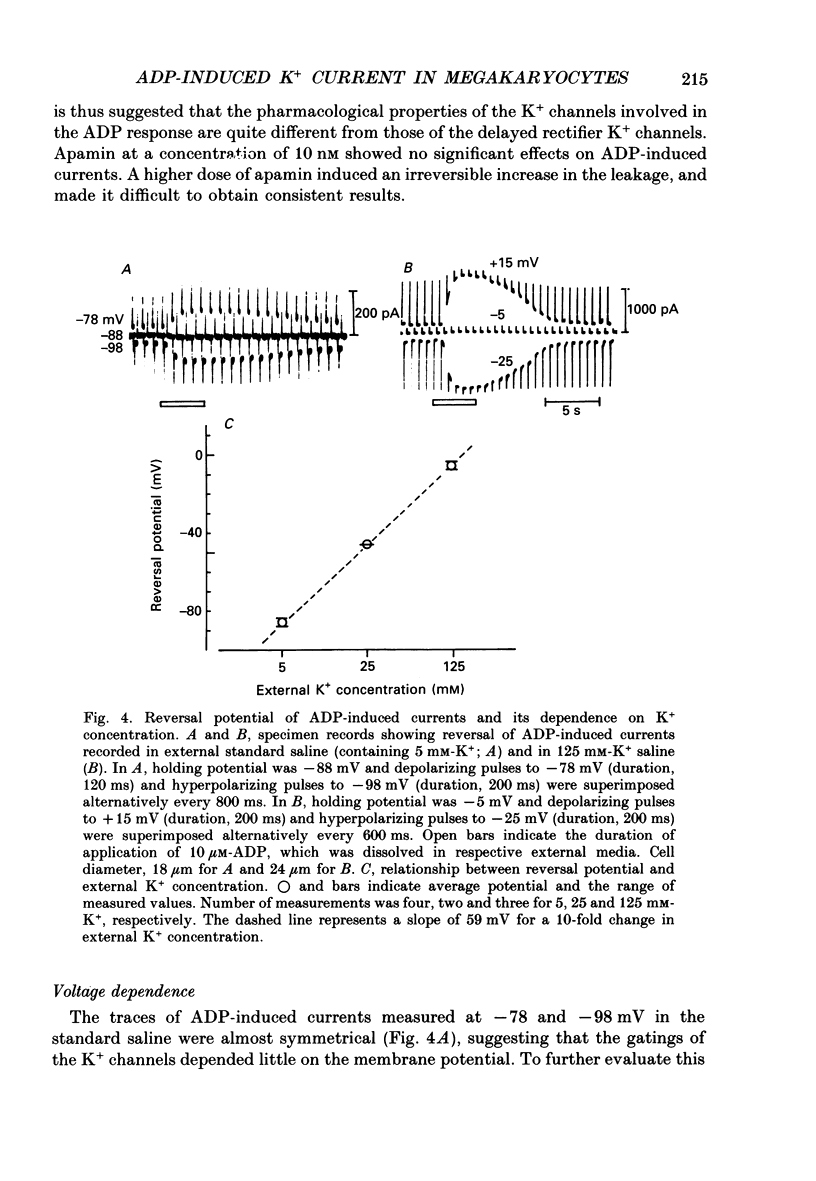
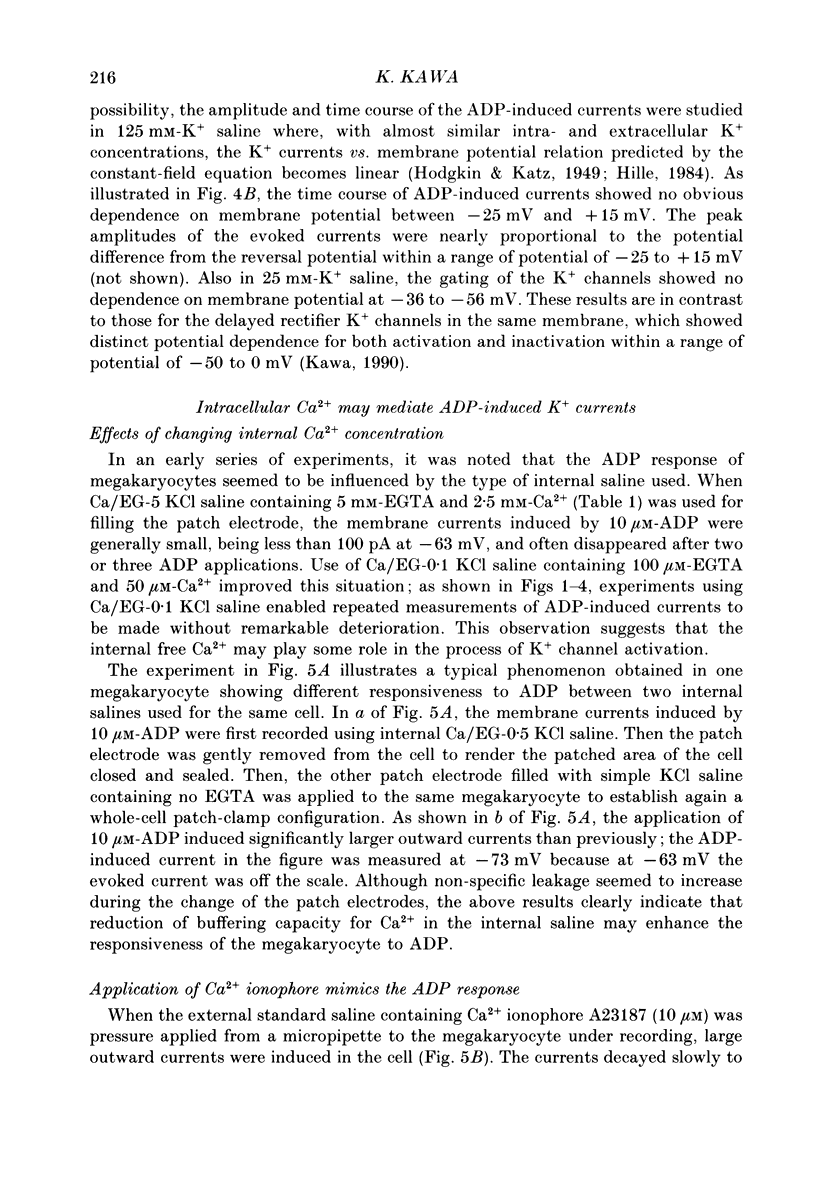
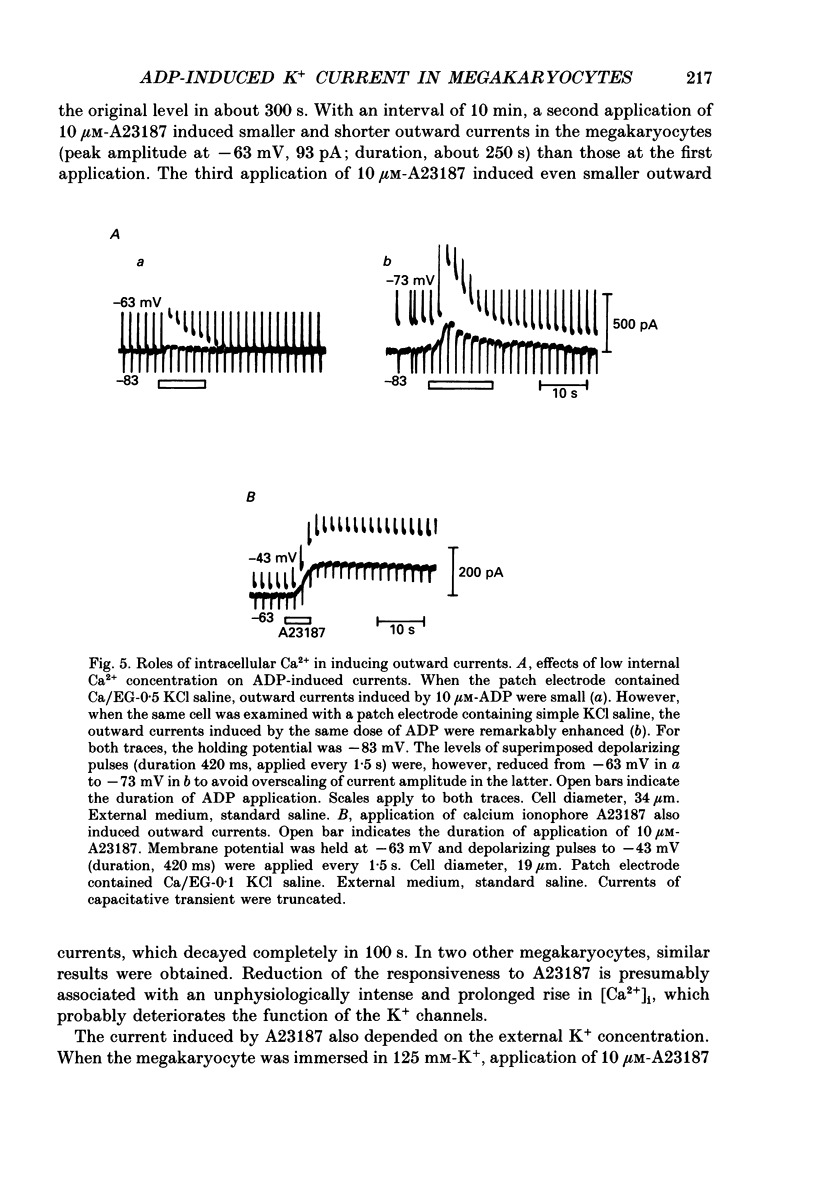
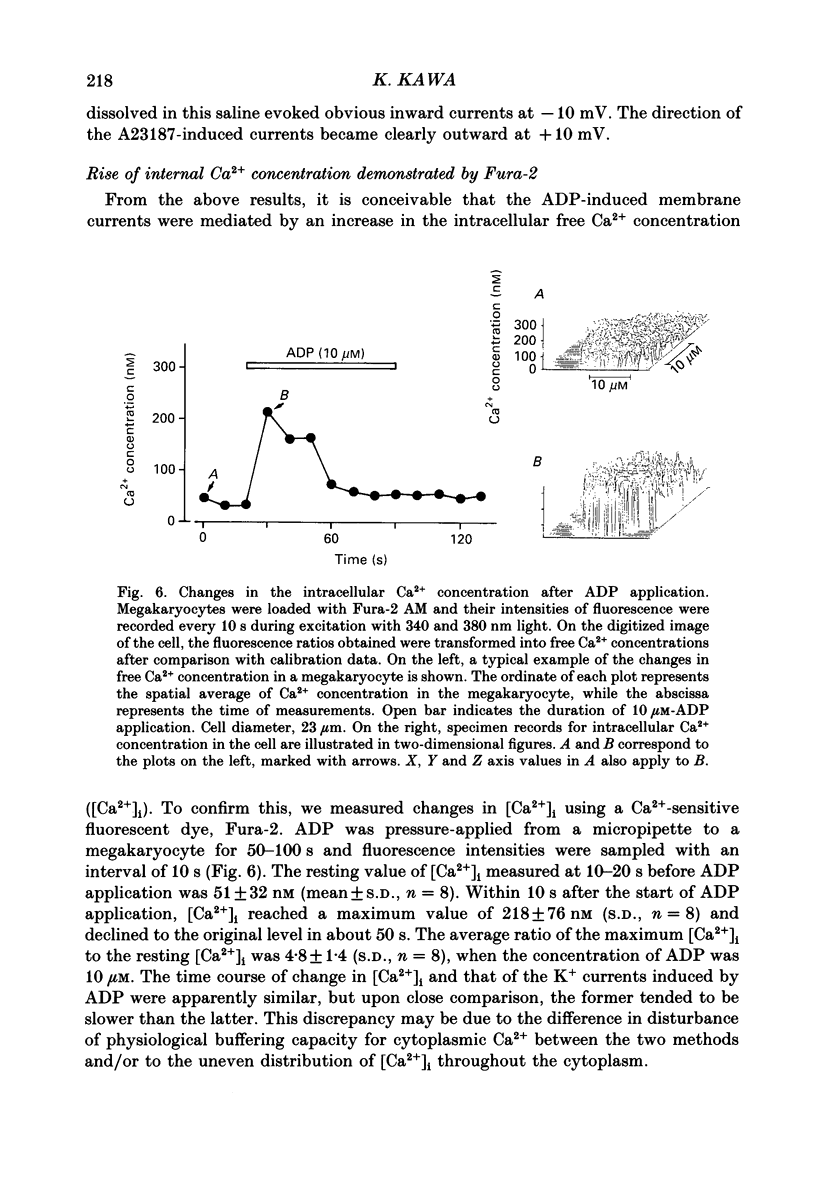
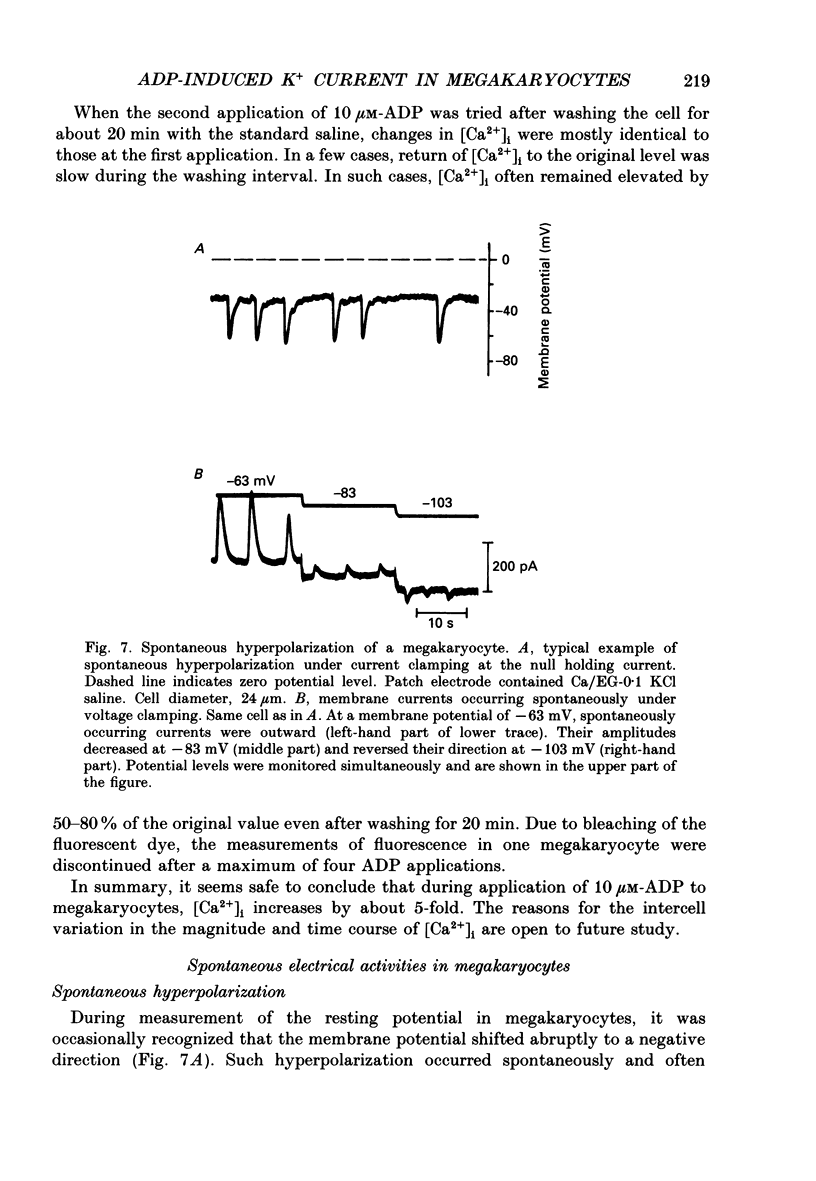
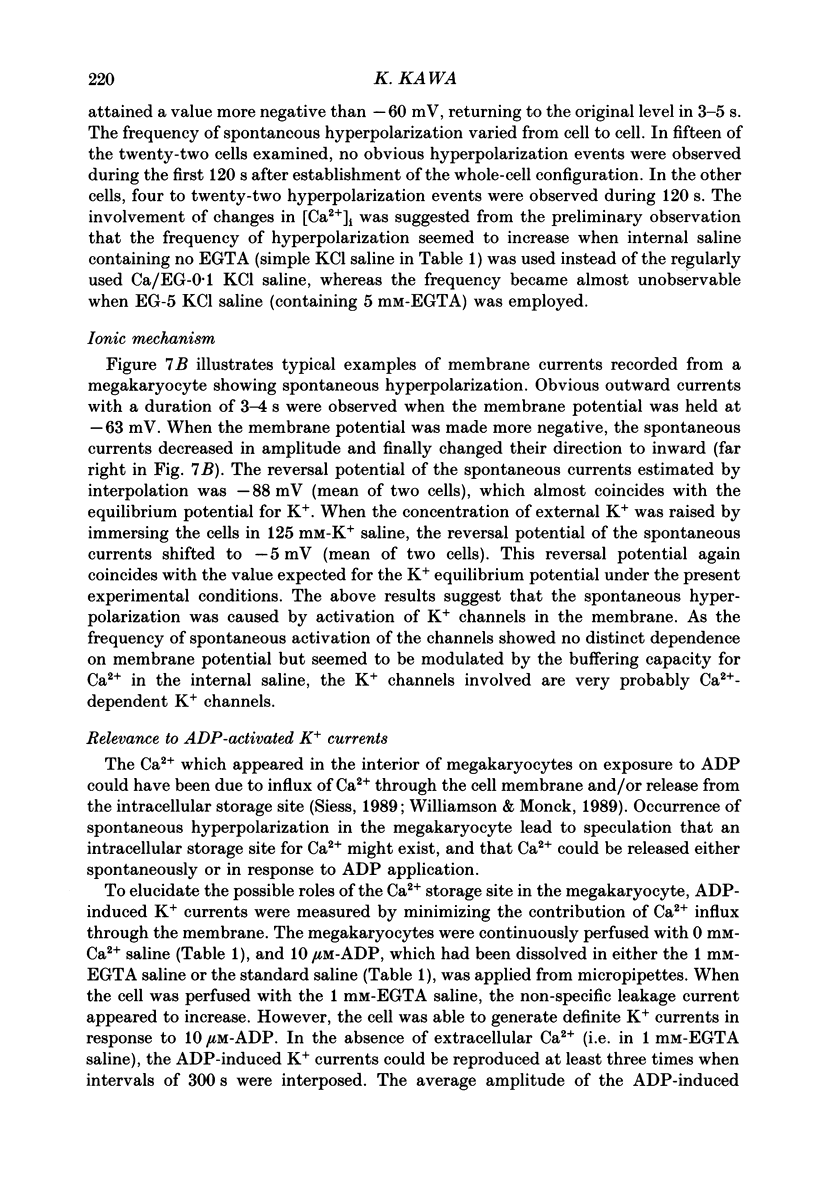
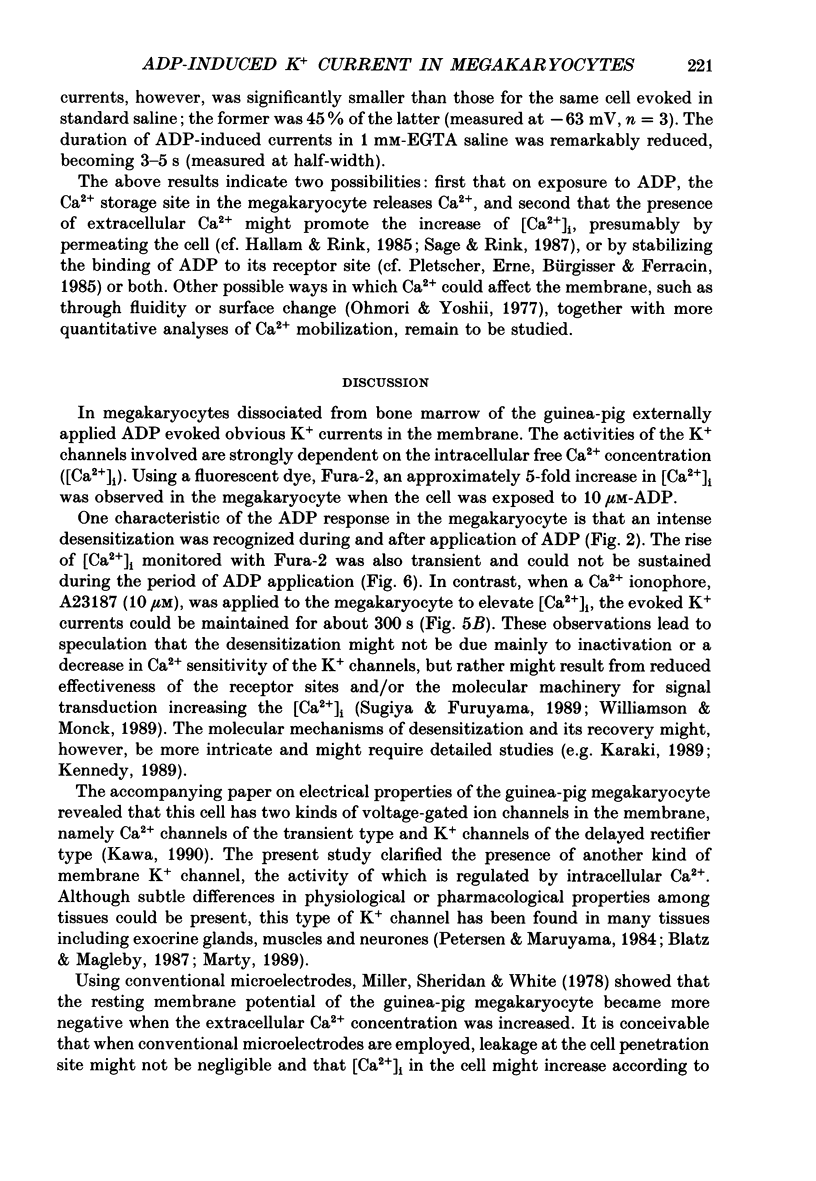
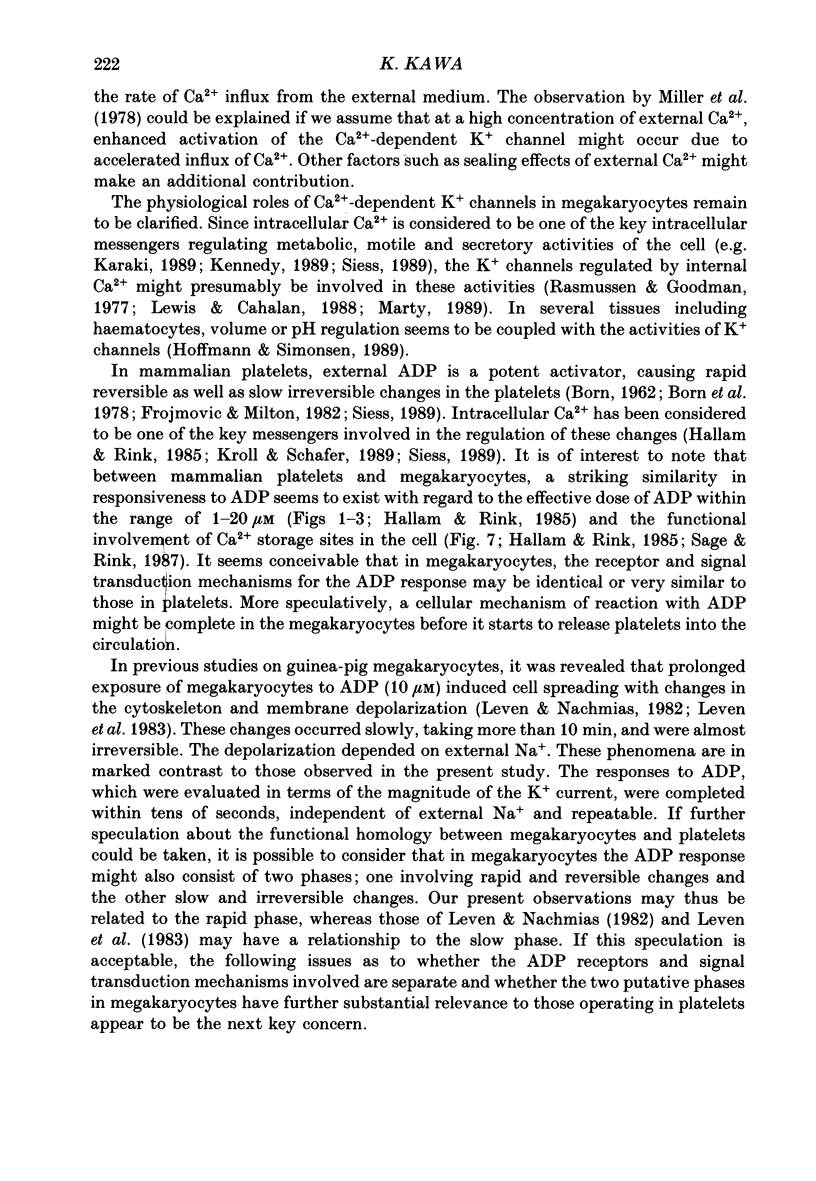
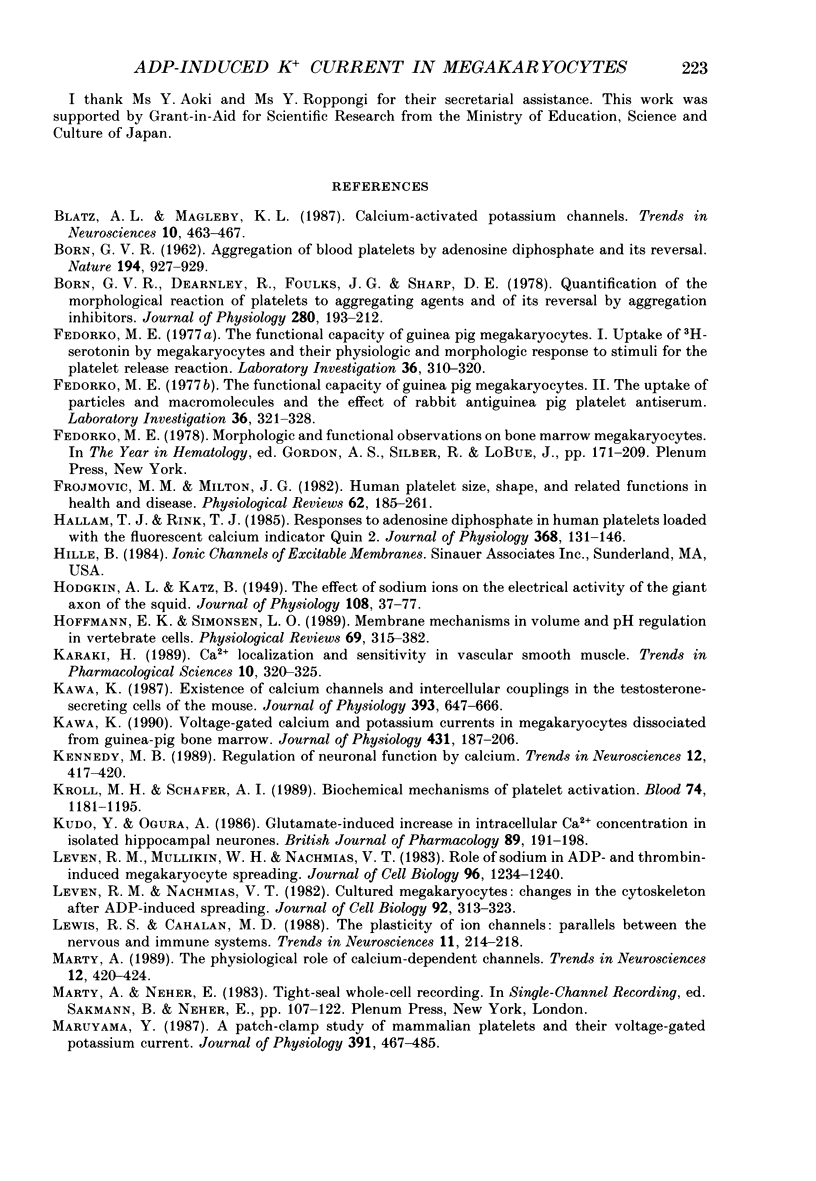
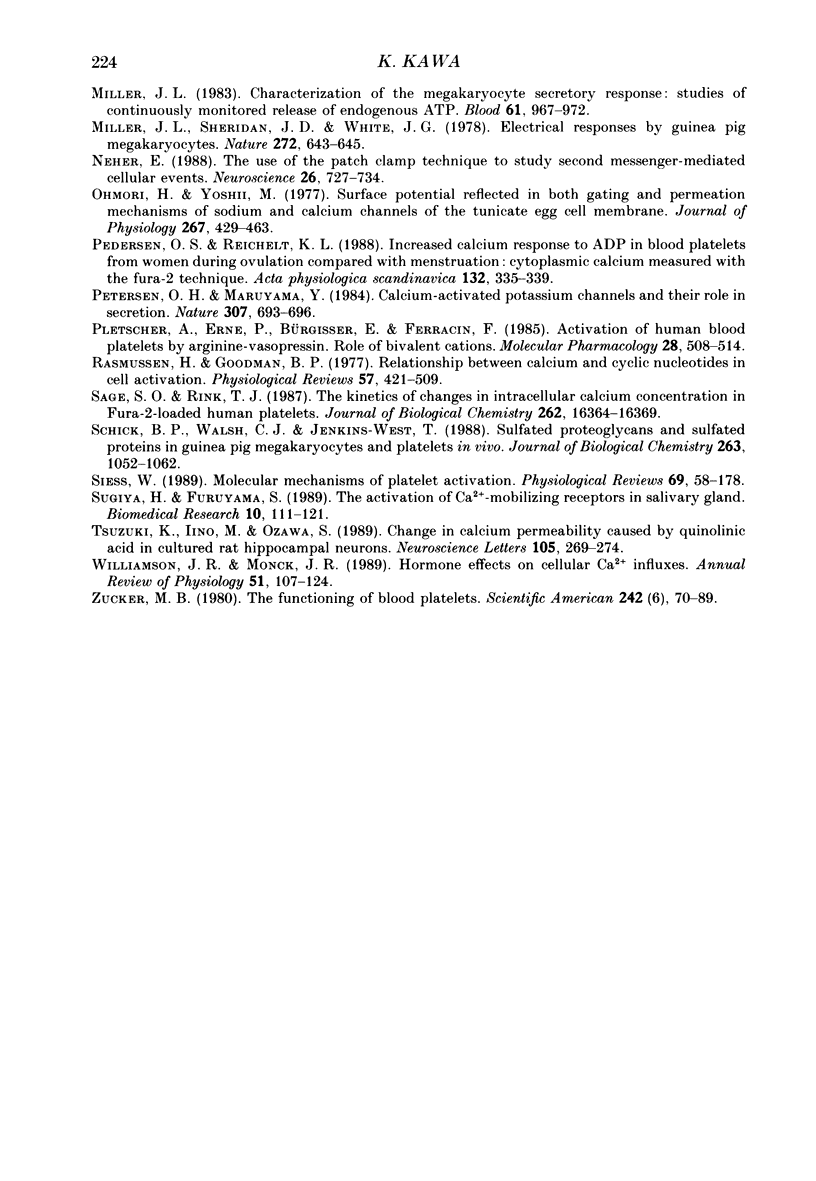
Selected References
These references are in PubMed. This may not be the complete list of references from this article.
- BORN G. V. Aggregation of blood platelets by adenosine diphosphate and its reversal. Nature. 1962 Jun 9;194:927–929. doi: 10.1038/194927b0. [DOI] [PubMed] [Google Scholar]
- Born G. V., Dearnley R., Foulks J. G., Sharp D. E. Quantification of the morphological reaction of platelets to aggregating agents and of its reversal by aggregation inhibitors. J Physiol. 1978 Jul;280:193–212. doi: 10.1113/jphysiol.1978.sp012380. [DOI] [PMC free article] [PubMed] [Google Scholar]
- Fedorko M. E. The functional capacity of guinea pig megakaryocytes. I. Uptake of 3H-serotonin by megakaryocytes and their physiologic and morphologic response to stimuli for the platelet release reaction. Lab Invest. 1977 Mar;36(3):310–320. [PubMed] [Google Scholar]
- Fedorko M. E. The functional capacity of guinea pig megakaryocytes. II. The uptake of particles and macromolecules and the effect of rabbit antiguinea pig platelet antiserum. Lab Invest. 1977 Mar;36(3):321–328. [PubMed] [Google Scholar]
- Frojmovic M. M., Milton J. G. Human platelet size, shape, and related functions in health and disease. Physiol Rev. 1982 Jan;62(1):185–261. doi: 10.1152/physrev.1982.62.1.185. [DOI] [PubMed] [Google Scholar]
- HODGKIN A. L., KATZ B. The effect of sodium ions on the electrical activity of giant axon of the squid. J Physiol. 1949 Mar 1;108(1):37–77. doi: 10.1113/jphysiol.1949.sp004310. [DOI] [PMC free article] [PubMed] [Google Scholar]
- Hallam T. J., Rink T. J. Responses to adenosine diphosphate in human platelets loaded with the fluorescent calcium indicator quin2. J Physiol. 1985 Nov;368:131–146. doi: 10.1113/jphysiol.1985.sp015850. [DOI] [PMC free article] [PubMed] [Google Scholar]
- Hoffmann E. K., Simonsen L. O. Membrane mechanisms in volume and pH regulation in vertebrate cells. Physiol Rev. 1989 Apr;69(2):315–382. doi: 10.1152/physrev.1989.69.2.315. [DOI] [PubMed] [Google Scholar]
- Karaki H. Ca2+ localization and sensitivity in vascular smooth muscle. Trends Pharmacol Sci. 1989 Aug;10(8):320–325. doi: 10.1016/0165-6147(89)90066-7. [DOI] [PubMed] [Google Scholar]
- Kawa K. Existence of calcium channels and intercellular couplings in the testosterone-secreting cells of the mouse. J Physiol. 1987 Dec;393:647–666. doi: 10.1113/jphysiol.1987.sp016846. [DOI] [PMC free article] [PubMed] [Google Scholar]
- Kawa K. Voltage-gated calcium and potassium currents in megakaryocytes dissociated from guinea-pig bone marrow. J Physiol. 1990 Dec;431:187–206. doi: 10.1113/jphysiol.1990.sp018326. [DOI] [PMC free article] [PubMed] [Google Scholar]
- Kennedy M. B. Regulation of neuronal function by calcium. Trends Neurosci. 1989 Nov;12(11):417–420. doi: 10.1016/0166-2236(89)90089-1. [DOI] [PubMed] [Google Scholar]
- Kroll M. H., Schafer A. I. Biochemical mechanisms of platelet activation. Blood. 1989 Sep;74(4):1181–1195. [PubMed] [Google Scholar]
- Kudo Y., Ogura A. Glutamate-induced increase in intracellular Ca2+ concentration in isolated hippocampal neurones. Br J Pharmacol. 1986 Sep;89(1):191–198. doi: 10.1111/j.1476-5381.1986.tb11135.x. [DOI] [PMC free article] [PubMed] [Google Scholar]
- Leven R. M., Mullikin W. H., Nachmias V. T. Role of sodium in ADP- and thrombin-induced megakaryocyte spreading. J Cell Biol. 1983 May;96(5):1234–1240. doi: 10.1083/jcb.96.5.1234. [DOI] [PMC free article] [PubMed] [Google Scholar]
- Leven R. M., Nachmias V. T. Cultured megakaryocytes: changes in the cytoskeleton after ADP-induced spreading. J Cell Biol. 1982 Feb;92(2):313–323. doi: 10.1083/jcb.92.2.313. [DOI] [PMC free article] [PubMed] [Google Scholar]
- Lewis R. S., Cahalan M. D. The plasticity of ion channels: parallels between the nervous and immune systems. Trends Neurosci. 1988 May;11(5):214–218. doi: 10.1016/0166-2236(88)90129-4. [DOI] [PubMed] [Google Scholar]
- Marty A. The physiological role of calcium-dependent channels. Trends Neurosci. 1989 Nov;12(11):420–424. doi: 10.1016/0166-2236(89)90090-8. [DOI] [PubMed] [Google Scholar]
- Maruyama Y. A patch-clamp study of mammalian platelets and their voltage-gated potassium current. J Physiol. 1987 Oct;391:467–485. doi: 10.1113/jphysiol.1987.sp016750. [DOI] [PMC free article] [PubMed] [Google Scholar]
- Miller J. L. Characterization of the megakaryocyte secretory response: studies of continuously monitored release of endogenous ATP. Blood. 1983 May;61(5):967–972. [PubMed] [Google Scholar]
- Miller J. L., Sheridan J. D., White J. G. Electrical responses by guinea pig megakaryocytes. Nature. 1978 Apr 13;272(5654):643–645. doi: 10.1038/272643a0. [DOI] [PubMed] [Google Scholar]
- Neher E. The use of the patch clamp technique to study second messenger-mediated cellular events. Neuroscience. 1988 Sep;26(3):727–734. doi: 10.1016/0306-4522(88)90094-2. [DOI] [PubMed] [Google Scholar]
- Ohmori H., Yoshii M. Surface potential reflected in both gating and permeation mechanisms of sodium and calcium channels of the tunicate egg cell membrane. J Physiol. 1977 May;267(2):429–463. doi: 10.1113/jphysiol.1977.sp011821. [DOI] [PMC free article] [PubMed] [Google Scholar]
- Pedersen O. S., Reichelt K. L. Increased calcium response to ADP in blood platelets from women during ovulation compared with menstruation: cytoplasmic calcium measured with the fura-2 technique. Acta Physiol Scand. 1988 Mar;132(3):335–339. doi: 10.1111/j.1748-1716.1988.tb08337.x. [DOI] [PubMed] [Google Scholar]
- Petersen O. H., Maruyama Y. Calcium-activated potassium channels and their role in secretion. Nature. 1984 Feb 23;307(5953):693–696. doi: 10.1038/307693a0. [DOI] [PubMed] [Google Scholar]
- Pletscher A., Erne P., Bürgisser E., Ferracin F. Activation of human blood platelets by arginine-vasopressin. Role of bivalent cations. Mol Pharmacol. 1985 Dec;28(6):508–514. [PubMed] [Google Scholar]
- Rasmussen H., Goodman D. B. Relationships between calcium and cyclic nucleotides in cell activation. Physiol Rev. 1977 Jul;57(3):421–509. doi: 10.1152/physrev.1977.57.3.421. [DOI] [PubMed] [Google Scholar]
- Sage S. O., Rink T. J. The kinetics of changes in intracellular calcium concentration in fura-2-loaded human platelets. J Biol Chem. 1987 Dec 5;262(34):16364–16369. [PubMed] [Google Scholar]
- Schick B. P., Walsh C. J., Jenkins-West T. Sulfated proteoglycans and sulfated proteins in guinea pig megakaryocytes and platelets in vivo. Relevance to megakaryocyte maturation and platelet activation. J Biol Chem. 1988 Jan 15;263(2):1052–1062. [PubMed] [Google Scholar]
- Siess W. Molecular mechanisms of platelet activation. Physiol Rev. 1989 Jan;69(1):58–178. doi: 10.1152/physrev.1989.69.1.58. [DOI] [PubMed] [Google Scholar]
- Tsuzuki K., Iino M., Ozawa S. Change in calcium permeability caused by quinolinic acid in cultured rat hippocampal neurons. Neurosci Lett. 1989 Nov 6;105(3):269–274. doi: 10.1016/0304-3940(89)90632-0. [DOI] [PubMed] [Google Scholar]
- Williamson J. R., Monck J. R. Hormone effects on cellular Ca2+ fluxes. Annu Rev Physiol. 1989;51:107–124. doi: 10.1146/annurev.ph.51.030189.000543. [DOI] [PubMed] [Google Scholar]