Abstract
The development of miniature end-plate currents (m.e.p.c.s) was studied in the superior oblique and interhyoideus muscles of Xenopus laevis. An analysis of m.e.p.c. decays shows that each muscle possesses its own characteristic programme of end-plate current development. In the superior oblique, the exponential decay constants of m.e.p.c.s were initially about 3 ms; they declined within half a day to 1 ms and remained at that value for six weeks. They then gradually became longer, reaching a mean value of 1.7 ms at late metamorphosis. In the interhyoideus, m.e.p.c. decay constants were initially about 6 ms. They declined in less than one day to a mean value of 2.6 ms and remained there for the following seven weeks. Upon completion of metamorphosis, the decay constants underwent a further decrease to about 1 ms. In both muscles, the changes in m.e.p.c. decays were correlated with developmental changes in muscle contraction speeds, as measured by maximum twitch frequencies. The above changes in end-plate currents in the superior oblique and interhyoideus muscles are discussed in terms of the development of acetylcholine receptor channel gating and acetylcholinesterase activity.
Full text
PDF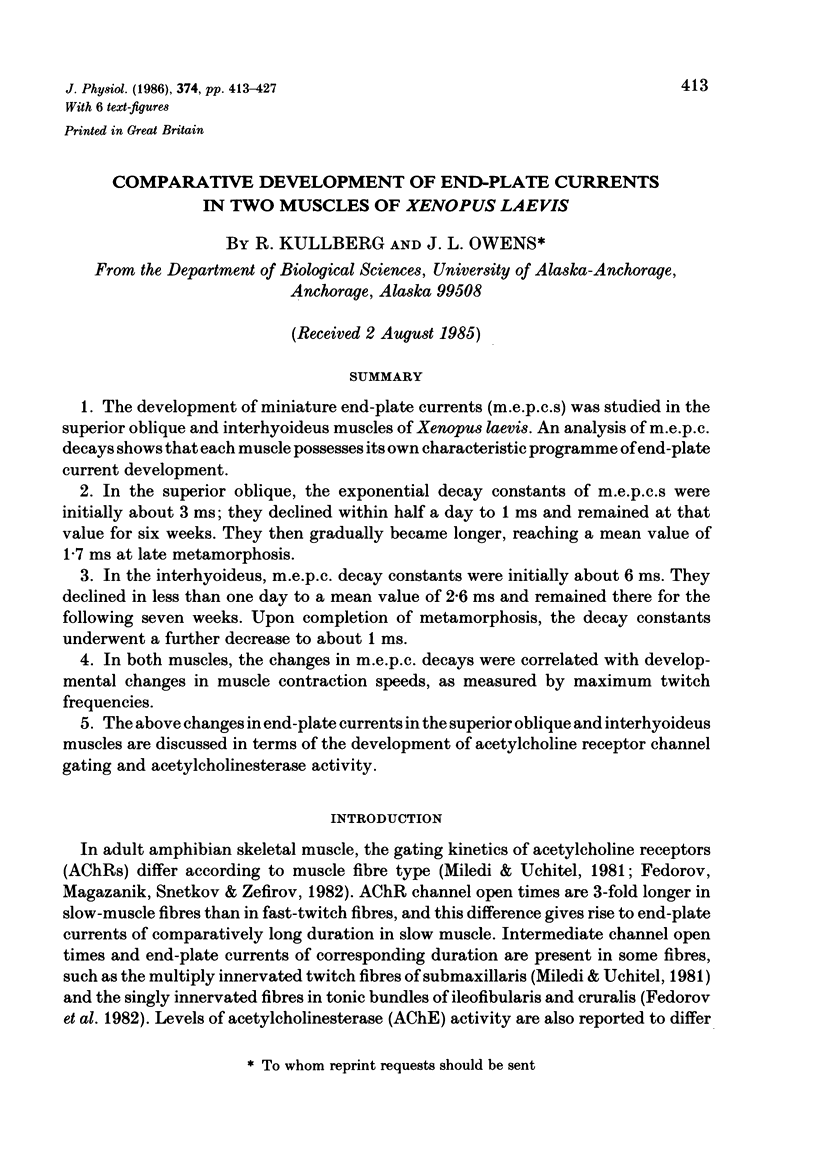
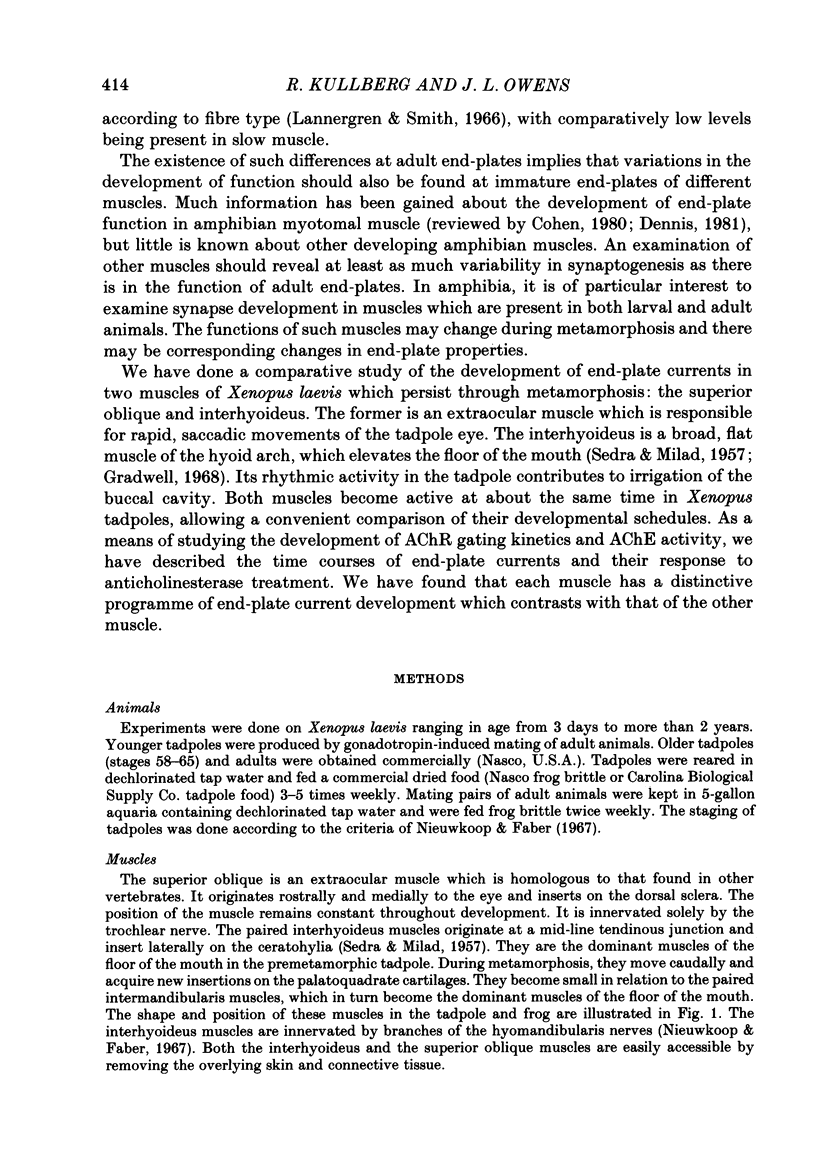
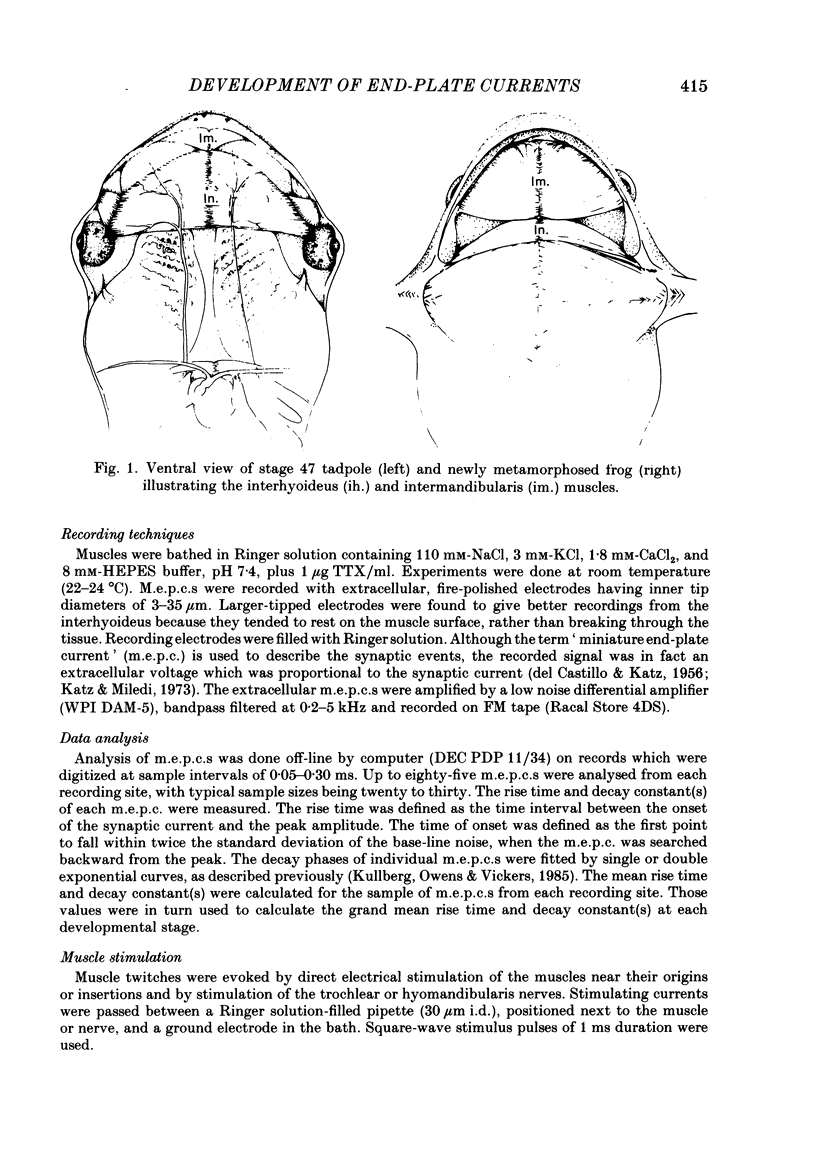
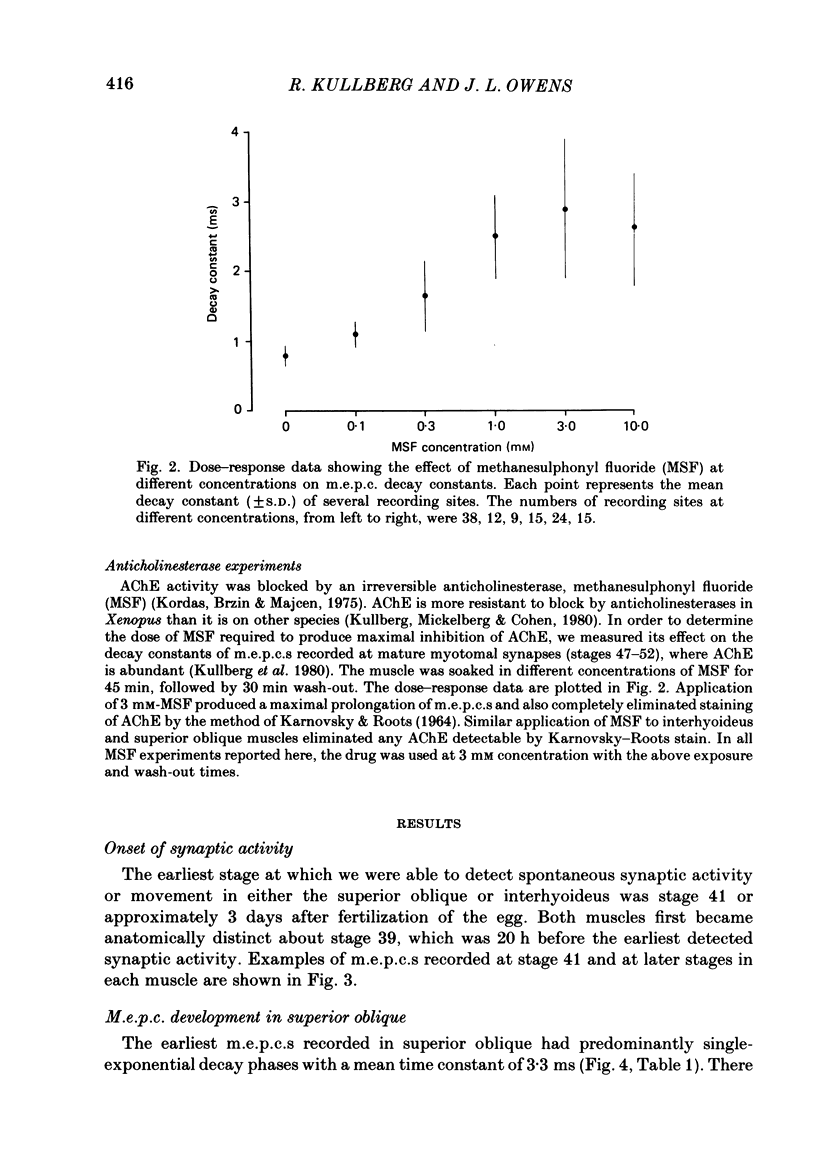
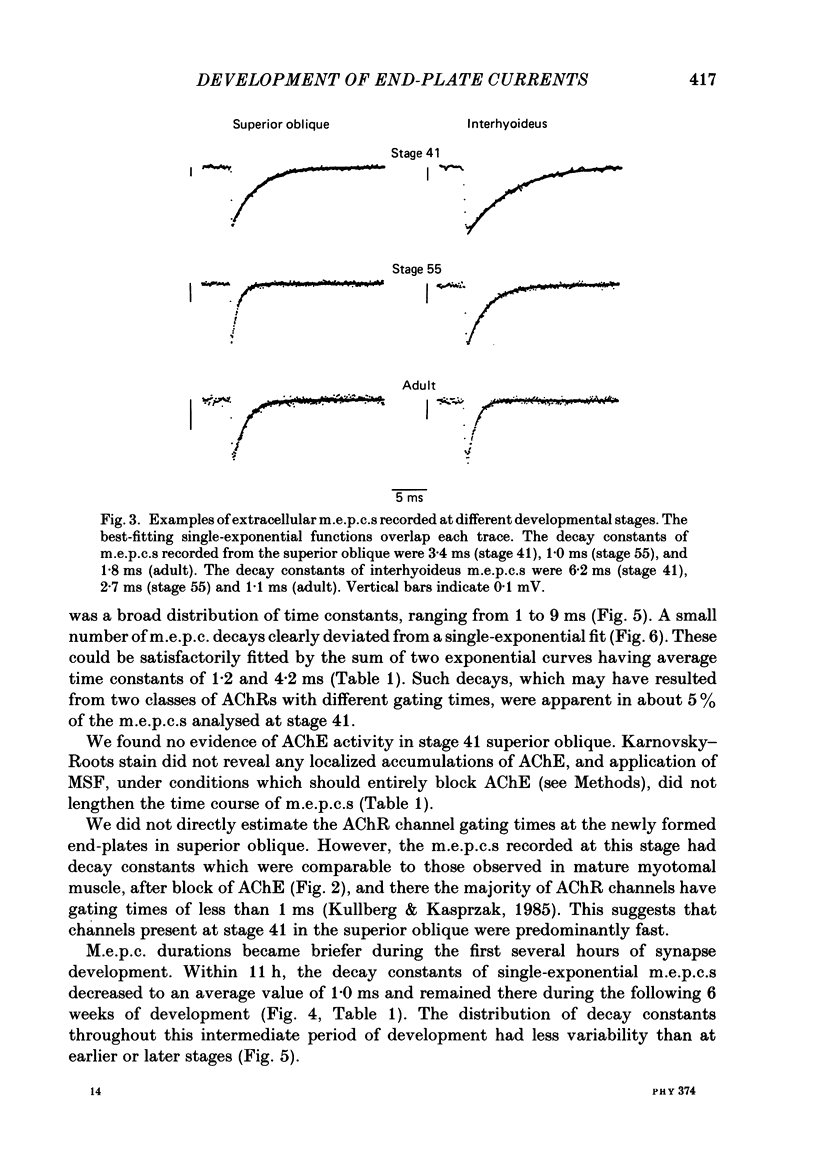
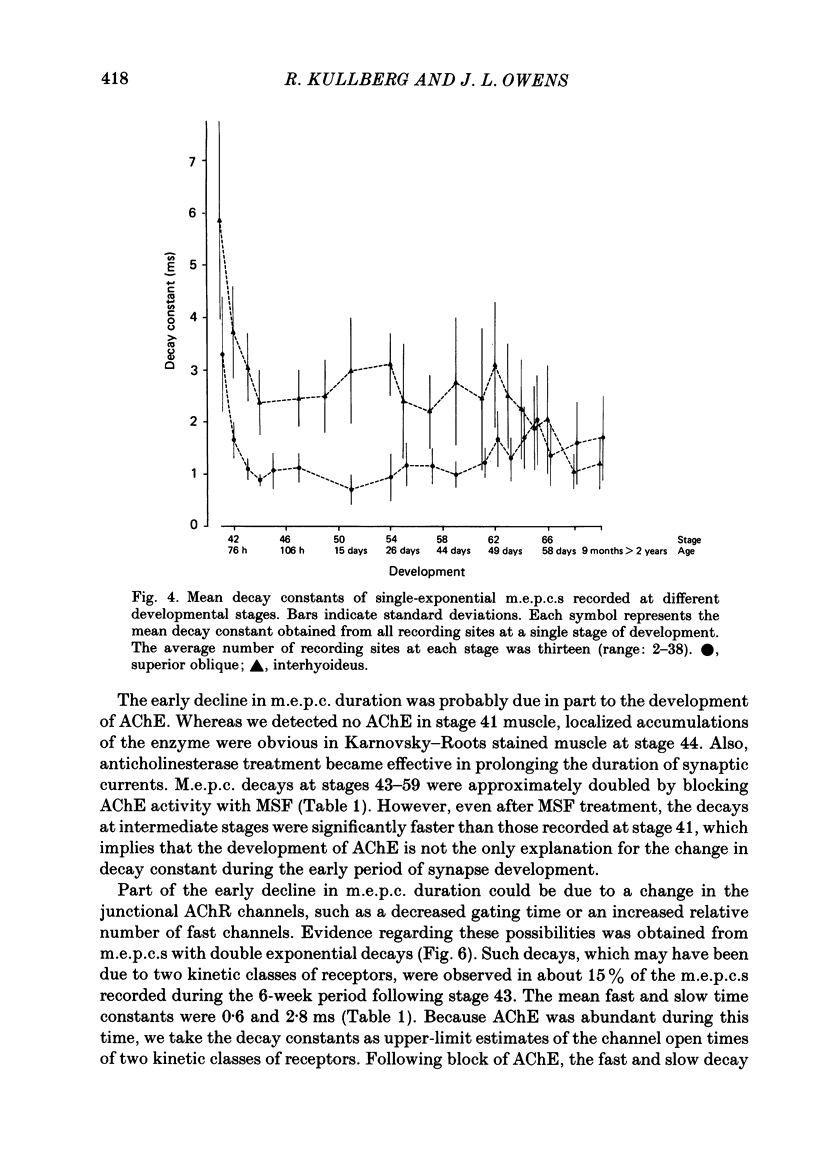
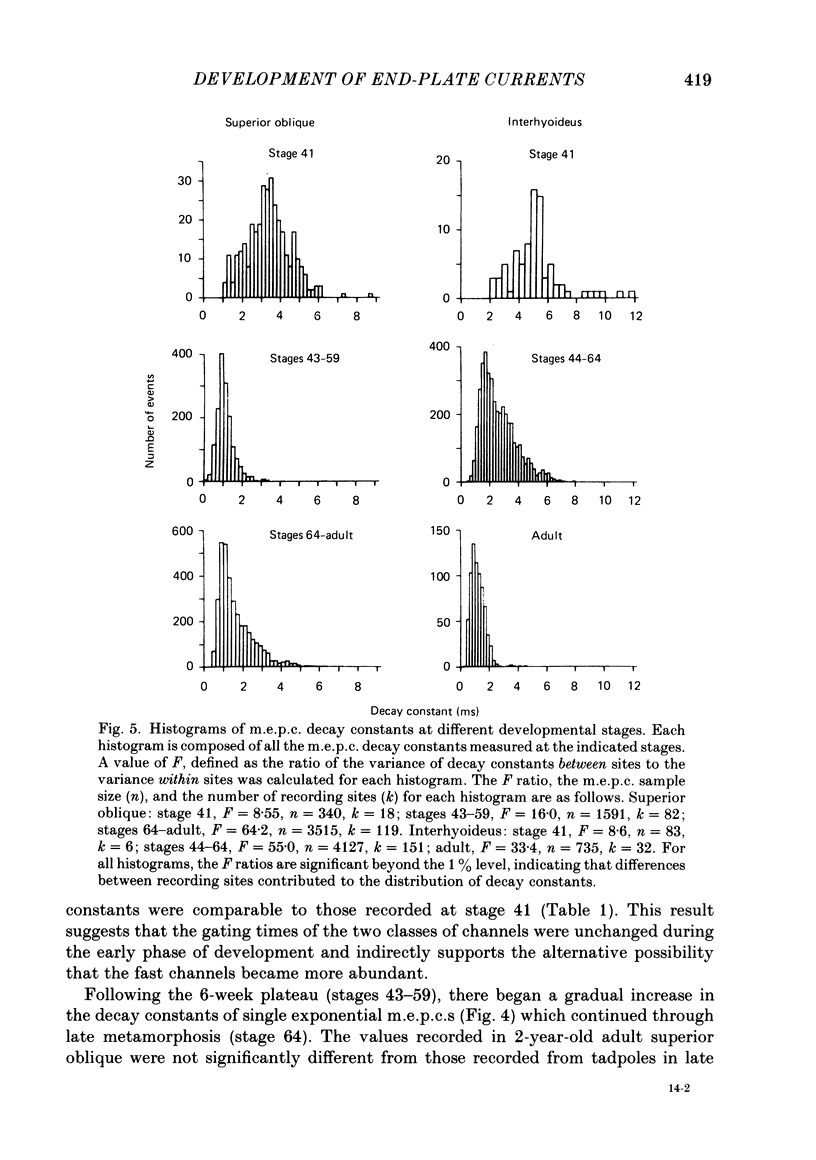
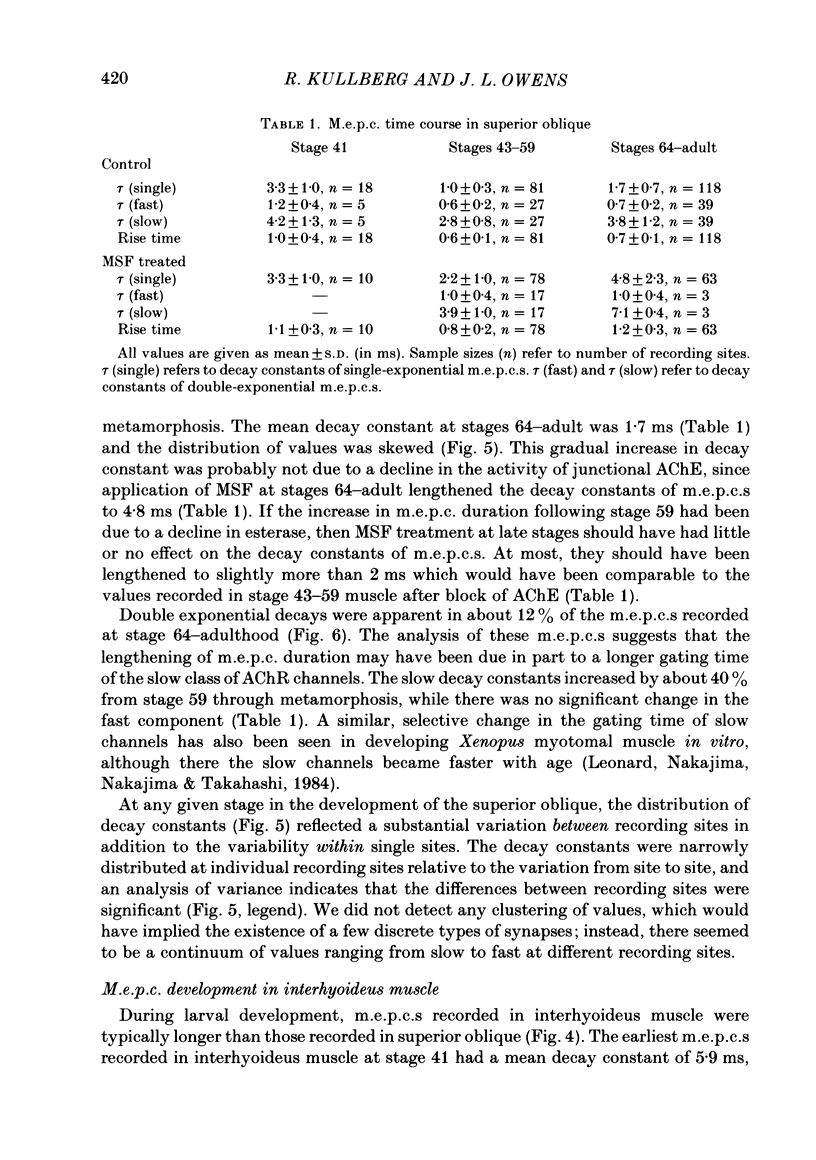
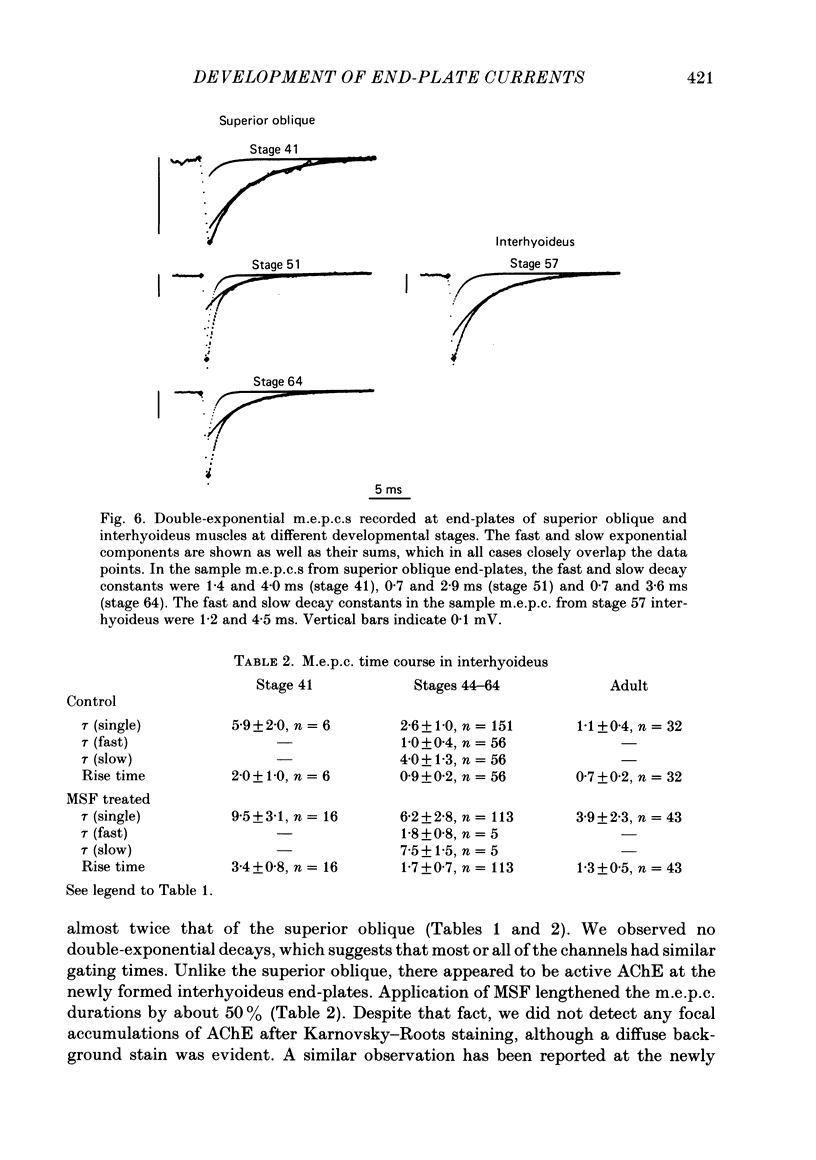
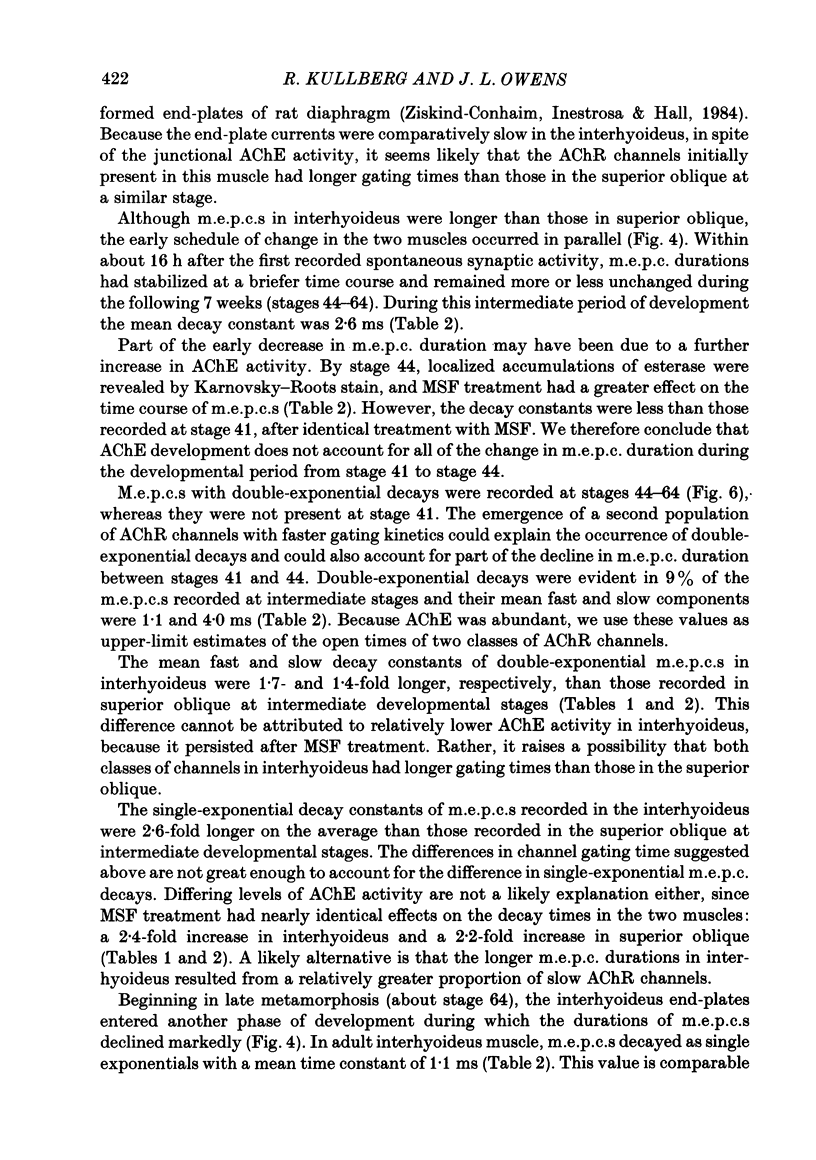
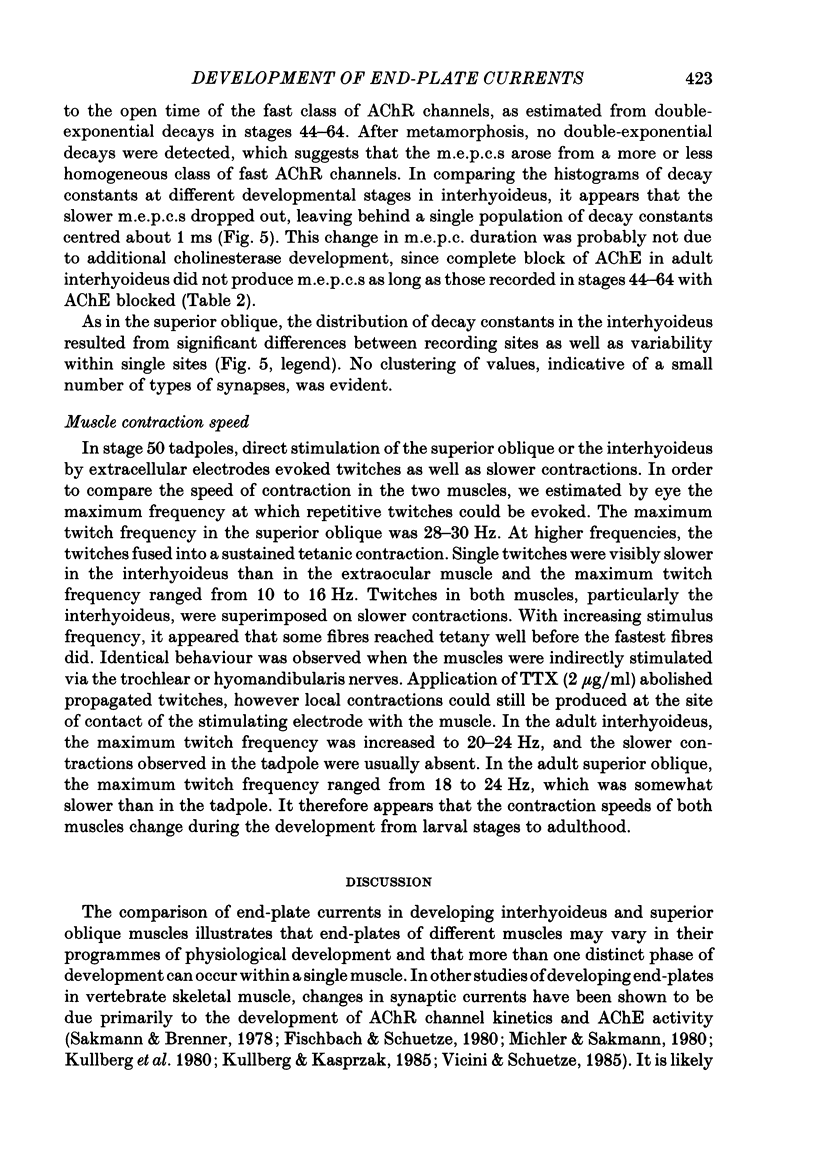
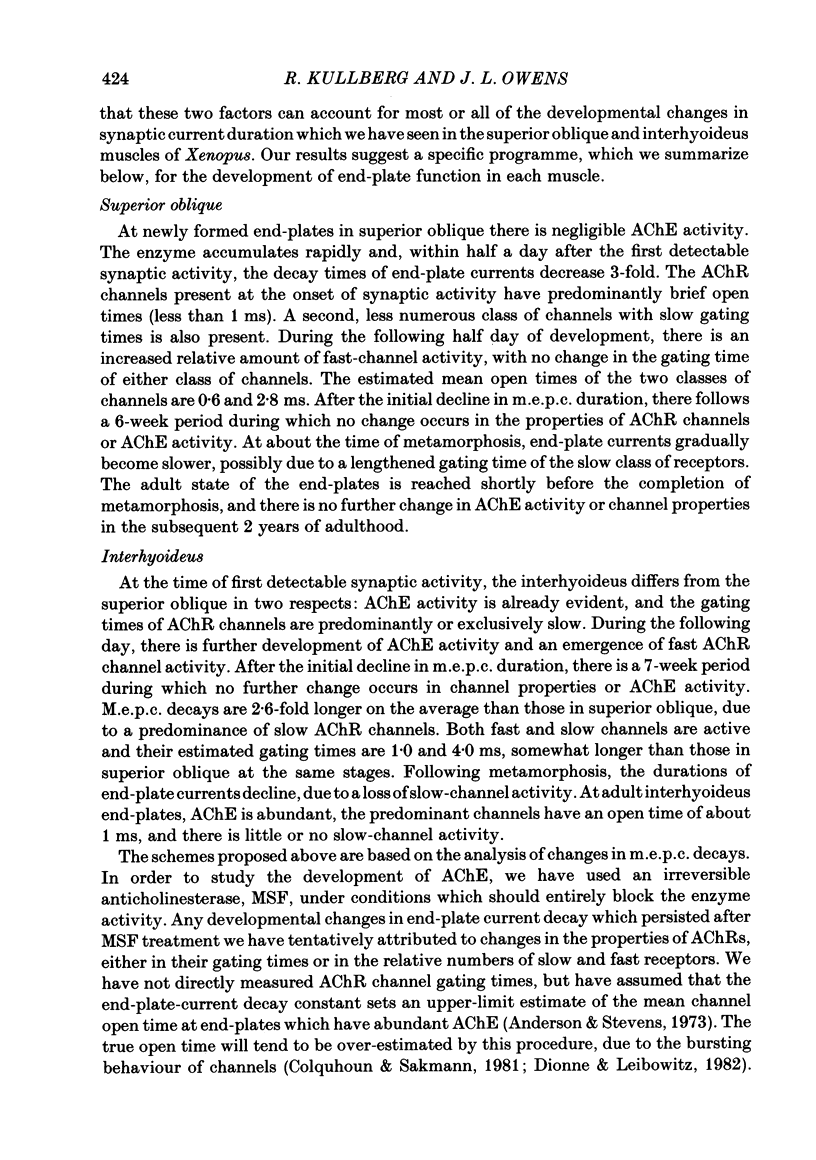
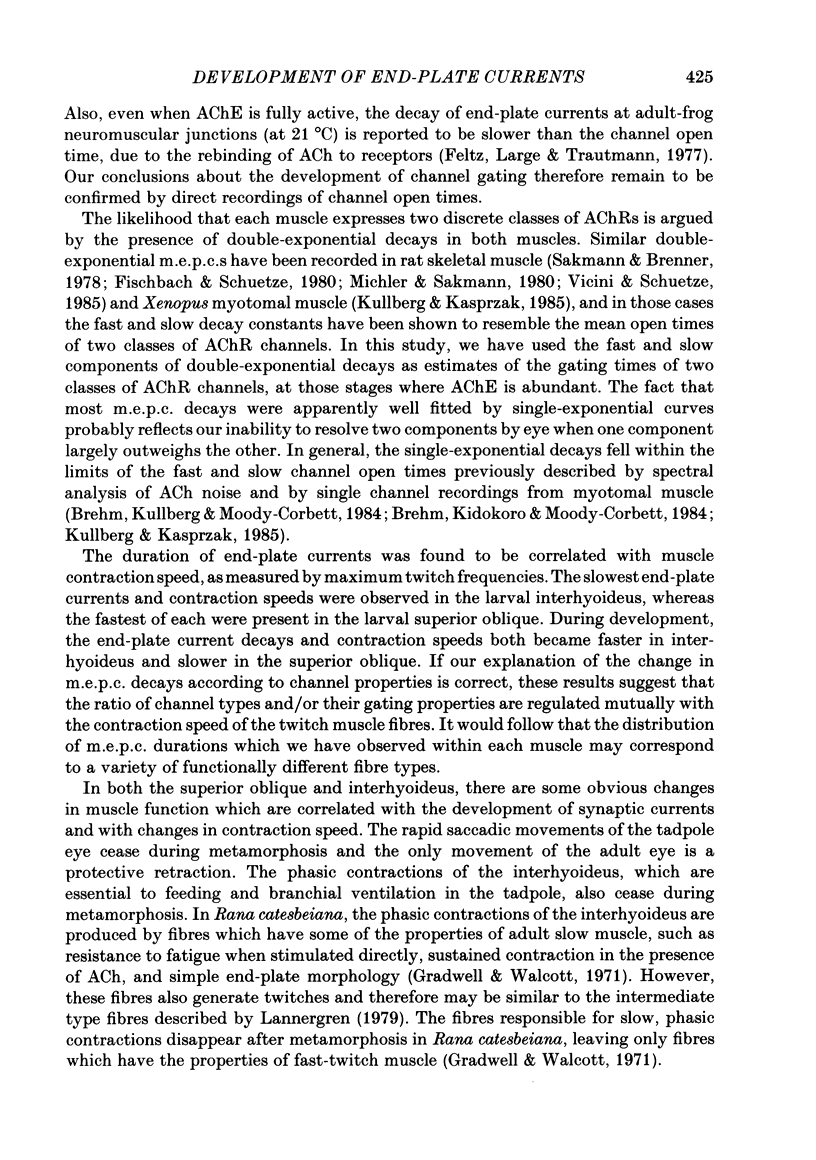
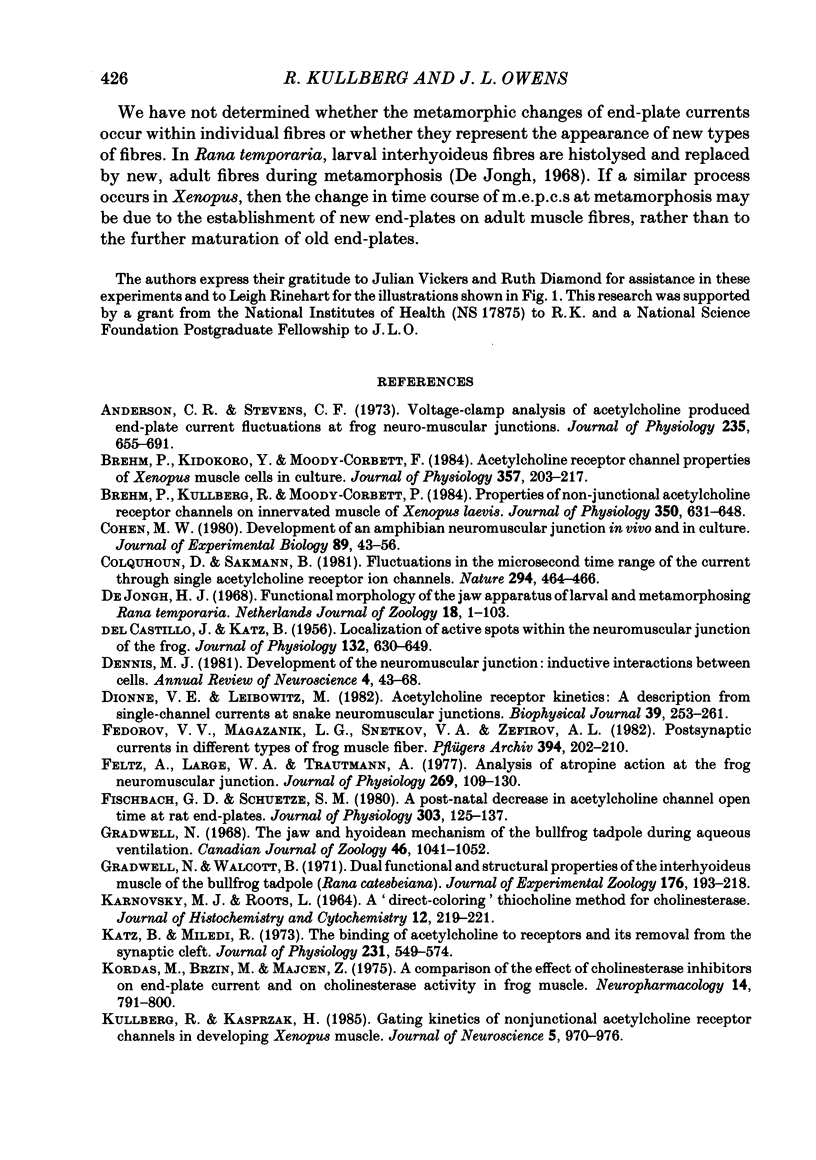
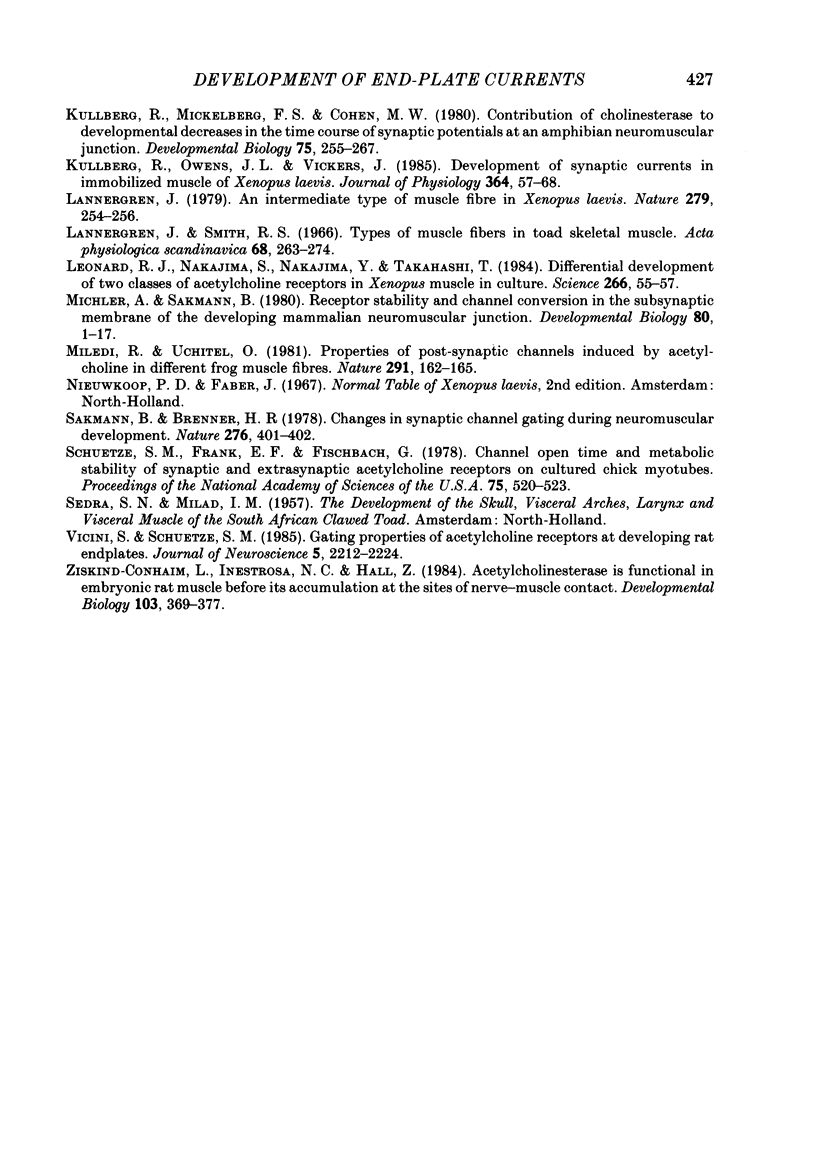
Selected References
These references are in PubMed. This may not be the complete list of references from this article.
- Anderson C. R., Stevens C. F. Voltage clamp analysis of acetylcholine produced end-plate current fluctuations at frog neuromuscular junction. J Physiol. 1973 Dec;235(3):655–691. doi: 10.1113/jphysiol.1973.sp010410. [DOI] [PMC free article] [PubMed] [Google Scholar]
- Brehm P., Kidokoro Y., Moody-Corbett F. Acetylcholine receptor channel properties during development of Xenopus muscle cells in culture. J Physiol. 1984 Dec;357:203–217. doi: 10.1113/jphysiol.1984.sp015497. [DOI] [PMC free article] [PubMed] [Google Scholar]
- Brehm P., Kullberg R., Moody-Corbett F. Properties of non-junctional acetylcholine receptor channels on innervated muscle of Xenopus laevis. J Physiol. 1984 May;350:631–648. doi: 10.1113/jphysiol.1984.sp015222. [DOI] [PMC free article] [PubMed] [Google Scholar]
- Cohen M. W. Development of an amphibian neuromuscular junction in vivo and in culture. J Exp Biol. 1980 Dec;89:43–56. doi: 10.1242/jeb.89.1.43. [DOI] [PubMed] [Google Scholar]
- Colquhoun D., Sakmann B. Fluctuations in the microsecond time range of the current through single acetylcholine receptor ion channels. Nature. 1981 Dec 3;294(5840):464–466. doi: 10.1038/294464a0. [DOI] [PubMed] [Google Scholar]
- DEL CASTILLO J., KATZ B. Localization of active spots within the neuromuscular junction of the frog. J Physiol. 1956 Jun 28;132(3):630–649. doi: 10.1113/jphysiol.1956.sp005554. [DOI] [PMC free article] [PubMed] [Google Scholar]
- Dennis M. J. Development of the neuromuscular junction: inductive interactions between cells. Annu Rev Neurosci. 1981;4:43–68. doi: 10.1146/annurev.ne.04.030181.000355. [DOI] [PubMed] [Google Scholar]
- Dionne V. E., Leibowitz M. D. Acetylcholine receptor kinetics. A description from single-channel currents at snake neuromuscular junctions. Biophys J. 1982 Sep;39(3):253–261. doi: 10.1016/S0006-3495(82)84515-3. [DOI] [PMC free article] [PubMed] [Google Scholar]
- Fedorov V. V., Magazanik L. G., Snetkov V. A., Zefirov A. L. Postsynaptic currents in different types of frog muscle fibre. Pflugers Arch. 1982 Sep;394(3):202–210. doi: 10.1007/BF00589092. [DOI] [PubMed] [Google Scholar]
- Feltz A., Large W. A., Trautmann A. Analysis of atropine action at the frog neutromuscular junction. J Physiol. 1977 Jul;269(1):109–130. doi: 10.1113/jphysiol.1977.sp011895. [DOI] [PMC free article] [PubMed] [Google Scholar]
- Fischbach G. D., Schuetze S. M. A post-natal decrease in acetylcholine channel open time at rat end-plates. J Physiol. 1980 Jun;303:125–137. doi: 10.1113/jphysiol.1980.sp013275. [DOI] [PMC free article] [PubMed] [Google Scholar]
- Gradwell N., Walcott B. Dual functional and structural properties of the interhyoideus muscle of the bullfrog tadpole (Rana catesbeiana). J Exp Zool. 1971 Feb;176(2):193–218. doi: 10.1002/jez.1401760207. [DOI] [PubMed] [Google Scholar]
- KARNOVSKY M. J., ROOTS L. A "DIRECT-COLORING" THIOCHOLINE METHOD FOR CHOLINESTERASES. J Histochem Cytochem. 1964 Mar;12:219–221. doi: 10.1177/12.3.219. [DOI] [PubMed] [Google Scholar]
- Katz B., Miledi R. The binding of acetylcholine to receptors and its removal from the synaptic cleft. J Physiol. 1973 Jun;231(3):549–574. doi: 10.1113/jphysiol.1973.sp010248. [DOI] [PMC free article] [PubMed] [Google Scholar]
- Kordás M., Brzin M., Majcen Z. A comparison of the effect of cholinesterase inhibitors on end-plate current and on cholinesterase activity in frog muscle. Neuropharmacology. 1975 Nov;14(11):791–800. doi: 10.1016/0028-3908(75)90106-9. [DOI] [PubMed] [Google Scholar]
- Kullberg R. W., Mikelberg F. S., Cohen M. W. Contribution of cholinesterase to developmental decreases in the time course of synaptic potentials at an amphibian neuromuscular junction. Dev Biol. 1980 Mar 15;75(2):255–267. doi: 10.1016/0012-1606(80)90161-x. [DOI] [PubMed] [Google Scholar]
- Kullberg R., Kasprzak H. Gating kinetics of nonjunctional acetylcholine receptor channels in developing Xenopus muscle. J Neurosci. 1985 Apr;5(4):970–976. doi: 10.1523/JNEUROSCI.05-04-00970.1985. [DOI] [PMC free article] [PubMed] [Google Scholar]
- Kullberg R., Owens J. L., Vickers J. Development of synaptic currents in immobilized muscle of Xenopus laevis. J Physiol. 1985 Jul;364:57–68. doi: 10.1113/jphysiol.1985.sp015729. [DOI] [PMC free article] [PubMed] [Google Scholar]
- Leonard R. J., Nakajima S., Nakajima Y., Takahashi T. Differential development of two classes of acetylcholine receptors in Xenopus muscle in culture. Science. 1984 Oct 5;226(4670):55–57. doi: 10.1126/science.6474189. [DOI] [PubMed] [Google Scholar]
- Lännergren J. An intermediate type of muscle fibre in Xenopus laevis. Nature. 1979 May 17;279(5710):254–256. doi: 10.1038/279254a0. [DOI] [PubMed] [Google Scholar]
- Michler A., Sakmann B. Receptor stability and channel conversion in the subsynaptic membrane of the developing mammalian neuromuscular junction. Dev Biol. 1980 Nov;80(1):1–17. doi: 10.1016/0012-1606(80)90494-7. [DOI] [PubMed] [Google Scholar]
- Miledi R., Uchitel O. D. Properties of postsynaptic channels induced by acetylcholine in different frog muscle fibres. Nature. 1981 May 14;291(5811):162–165. doi: 10.1038/291162a0. [DOI] [PubMed] [Google Scholar]
- Sakmann B., Brenner H. R. Change in synaptic channel gating during neuromuscular development. Nature. 1978 Nov 23;276(5686):401–402. doi: 10.1038/276401a0. [DOI] [PubMed] [Google Scholar]
- Schuetze S. M., Frank E. F., Fischbach G. D. Channel open time and metabolic stability of synaptic and extrasynaptic acetylcholine receptors on cultured chick myotubes. Proc Natl Acad Sci U S A. 1978 Jan;75(1):520–523. doi: 10.1073/pnas.75.1.520. [DOI] [PMC free article] [PubMed] [Google Scholar]
- Vicini S., Schuetze S. M. Gating properties of acetylcholine receptors at developing rat endplates. J Neurosci. 1985 Aug;5(8):2212–2224. doi: 10.1523/JNEUROSCI.05-08-02212.1985. [DOI] [PMC free article] [PubMed] [Google Scholar]
- Ziskind-Conhaim L., Inestrosa N. C., Hall Z. W. Acetylcholinesterase is functional in embryonic rat muscle before its accumulation at the sites of nerve-muscle contact. Dev Biol. 1984 Jun;103(2):369–377. doi: 10.1016/0012-1606(84)90325-7. [DOI] [PubMed] [Google Scholar]