Abstract
The effects of replacement of external and internal K+ ions by Rb+ ions on the two fast components (gf1 and gf2) and slow component (gs) of the K+ conductance (gK) in frog nodes of Ranvier were investigated under voltage- and current-clamp conditions. Fast and slow components of gK were separated by double exponential fits to tail currents following long depolarizing pre-pulses, or by the use of short pre-pulses which activate little gs X gs was also isolated by 1 mM-4-aminopyridine (4-AP). gf1 and gf2 were distinguished in the fast conductance-voltage curve by their different voltage dependences, gf1 activating at more negative potentials. Reversal potential measurements indicated that Rb+ is less permeant than K+, and measurements in 4-AP indicated that the slow component has a lower Rb+ permeability than the fast. In a 50% K+, 50% Rb+ mixture PRb/PK was less than that in 100% Rb+ suggesting that PRb/PK is mole-fraction dependent. With external Rb+ the current-voltage relation was shifted by ca.-10 mV compared to that in K+, an effect on gf ( = gf1 + gf2). The slow conductance (gs) and, under similar conditions, the Na+ current-voltage relation were not shifted. gf, calculated from inward tail currents, was reduced with external Rb+ at potentials where gf2 was activated. Instantaneous current-voltage relations following pre-pulses which activate different components of gf confirmed these observations. In K+ the instantaneous current-voltage relation showed some inward rectification which was largely abolished with Rb+. Comparison of gf calculated from outward (go) and inward (gi) currents confirmed this, and showed that inward gf2 was reduced with Rb+ such that go = gi. Outward currents were little affected by external Rb+. External Rb+ slowed the fast inward tail current following all pre-pulses which activate gf, but had no effect on the time course of the slow component of the tail current. Regenerative responses, which occur in high [K+] (+300 nM-tetrodotoxin) solutions in current clamp did not repolarize in Rb+. Voltage-clamp experiments showed that inactivation of inward currents is slowed when Rb+ is the charge carrier. Replacement of internal K+, by application of Rb+ to the cut ends of the fibre, shifted the reversal potential to more positive potentials but had no effect on the conductance or kinetics. External Rb+ has a large number of effects on inward currents, but little effect on outward currents. Internal Rb+ had little effect on outward or inward currents.(ABSTRACT TRUNCATED AT 400 WORDS)
Full text
PDF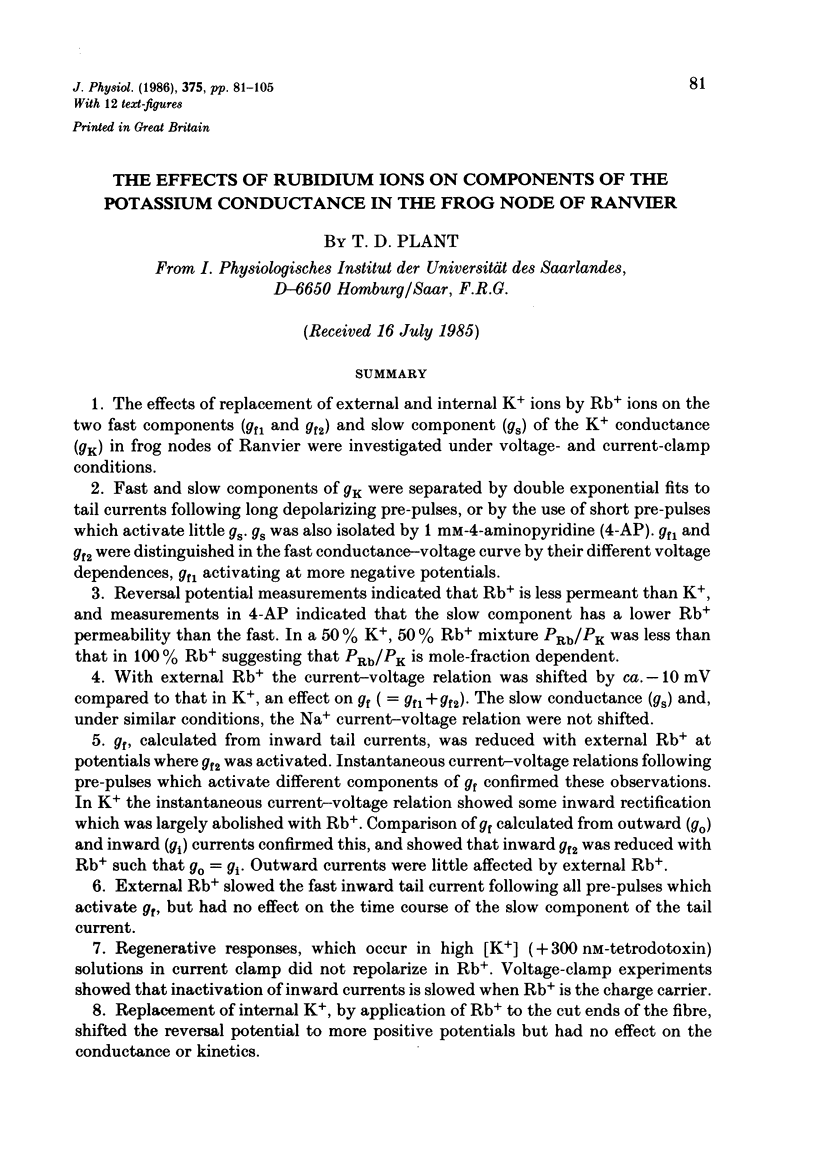
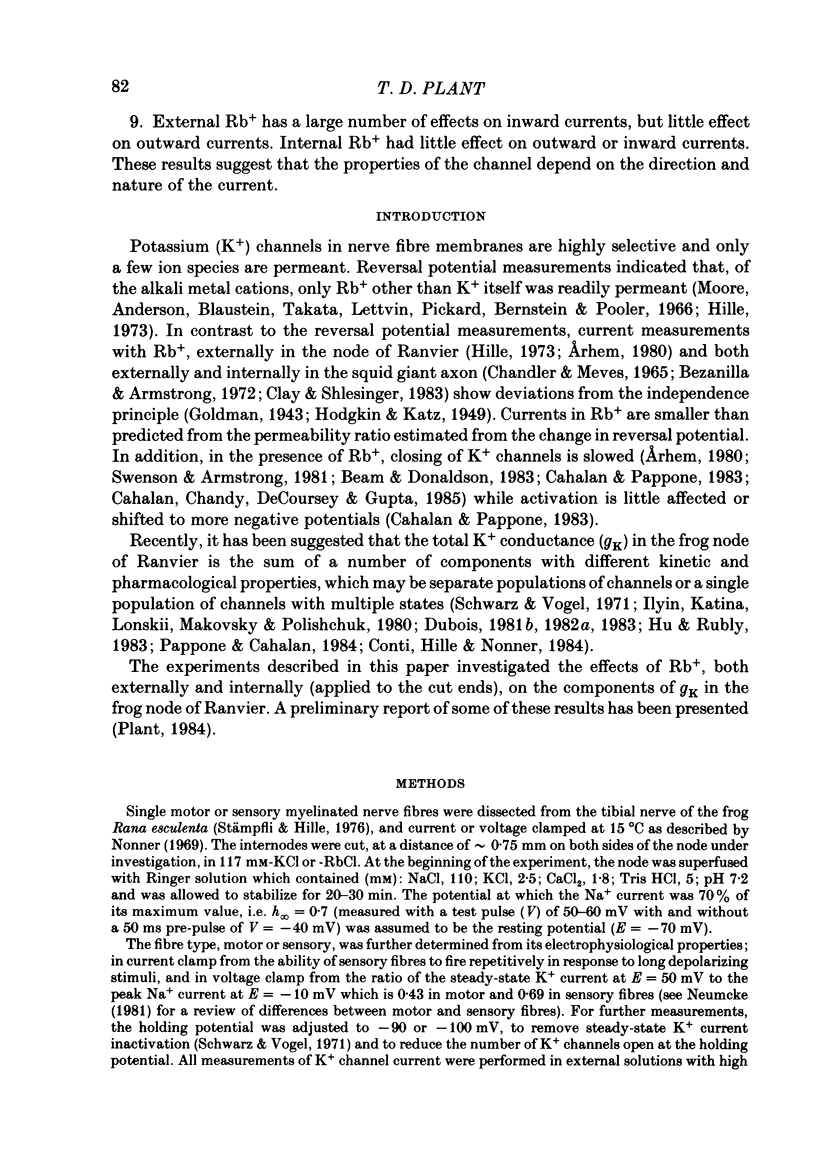
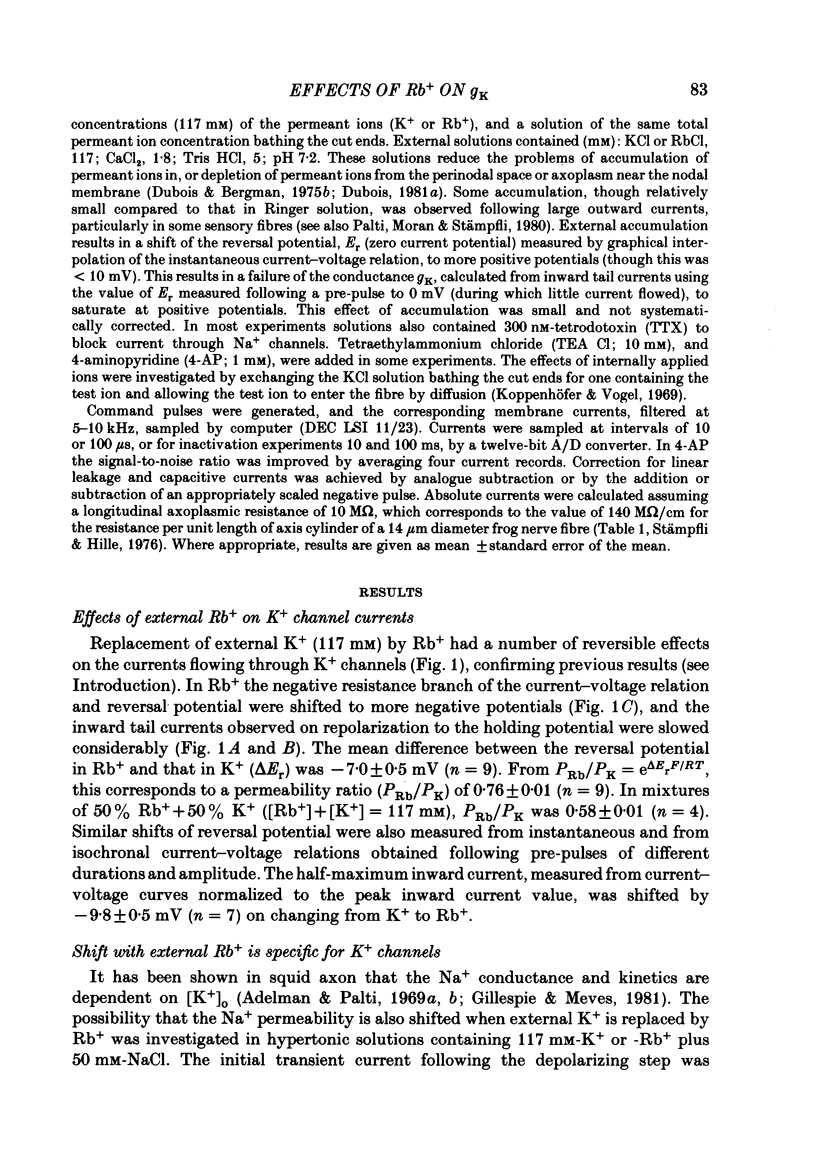
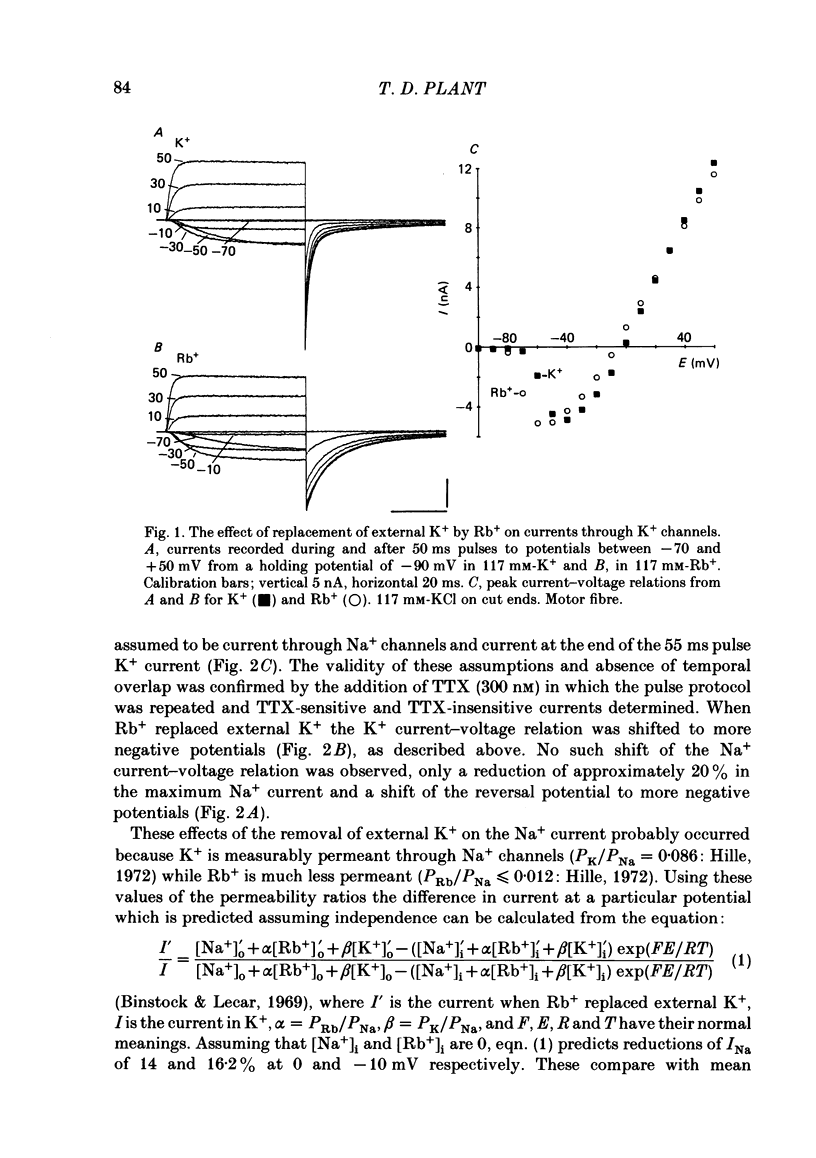
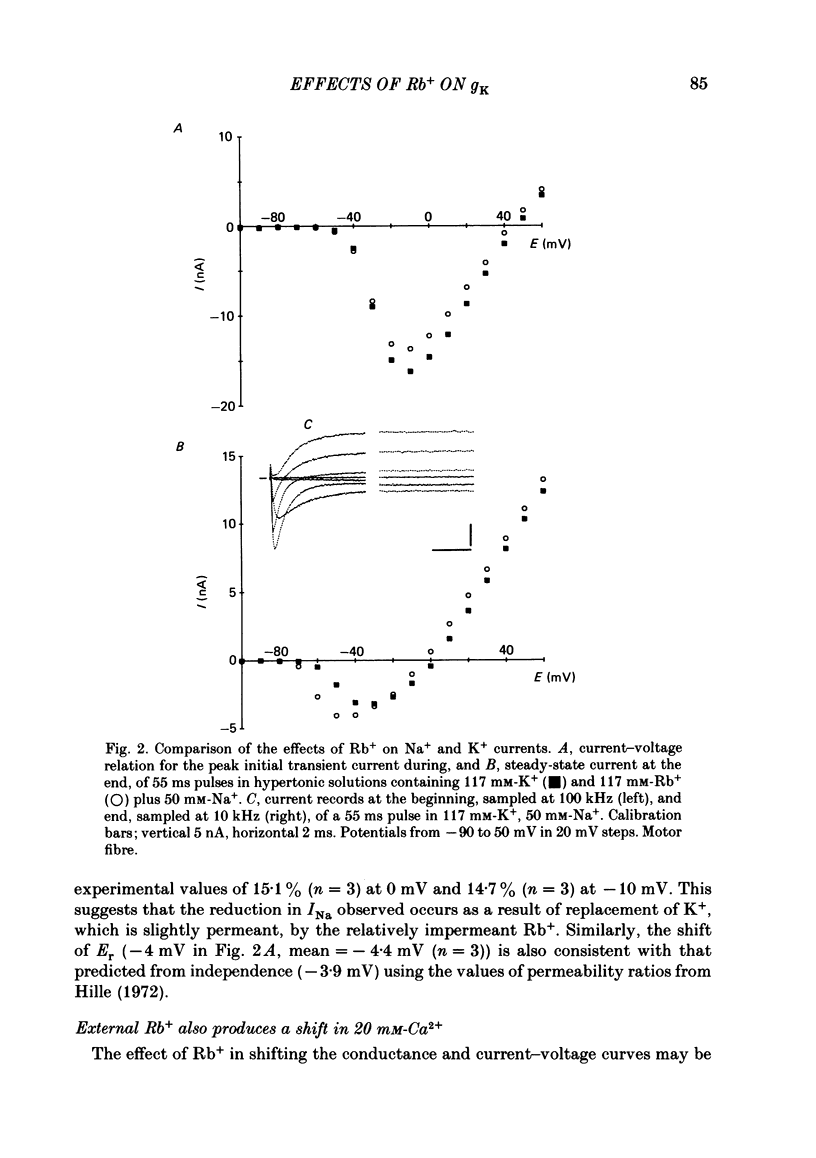
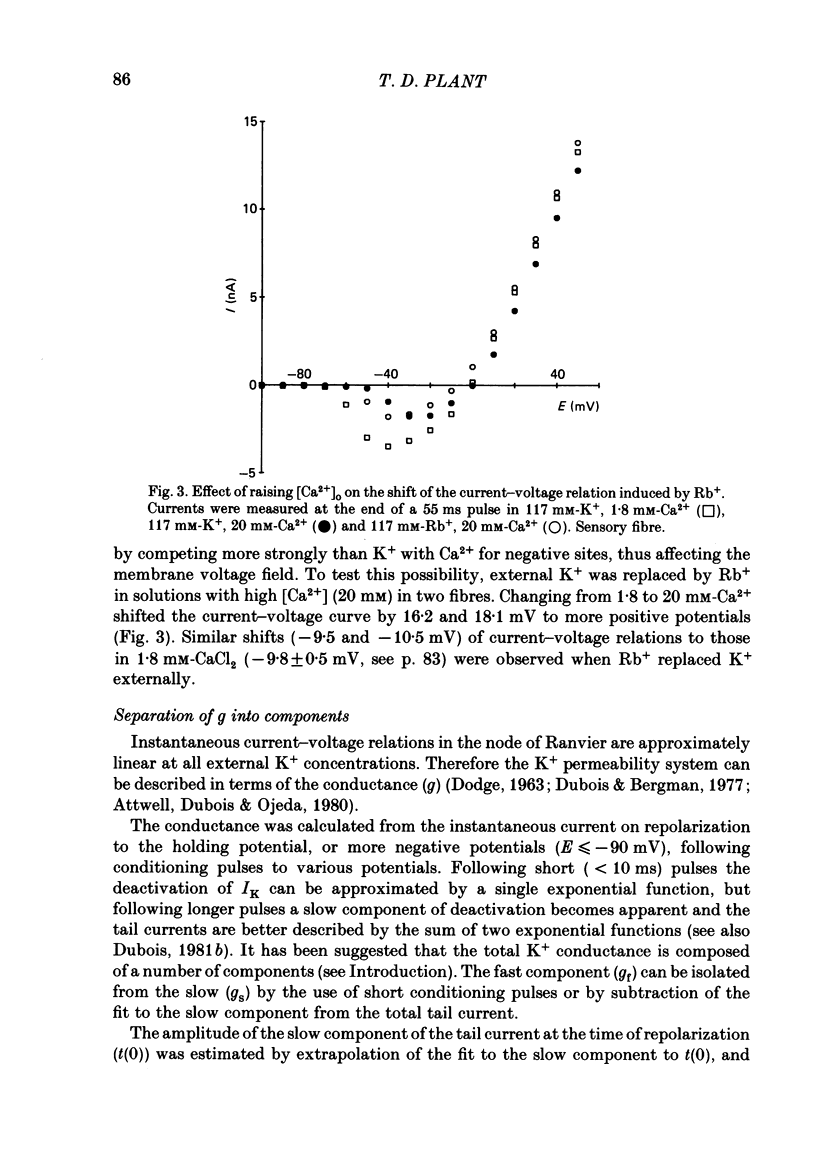
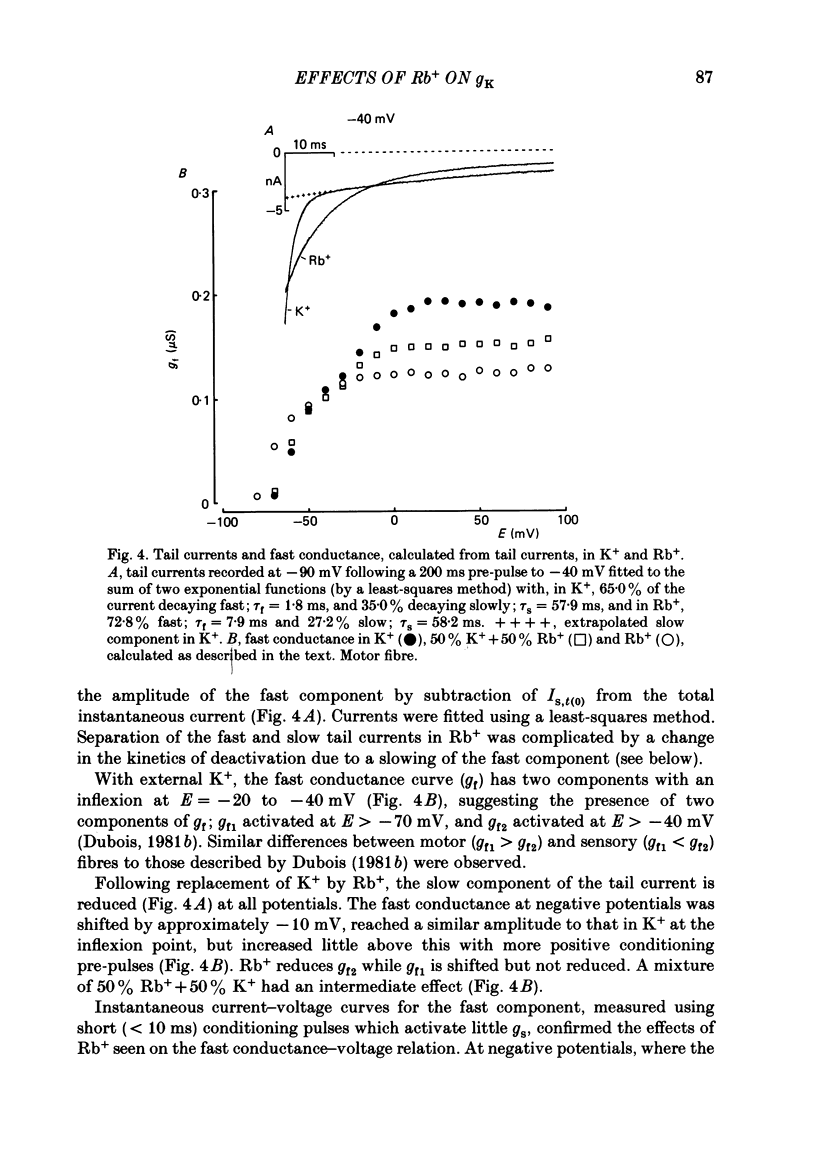
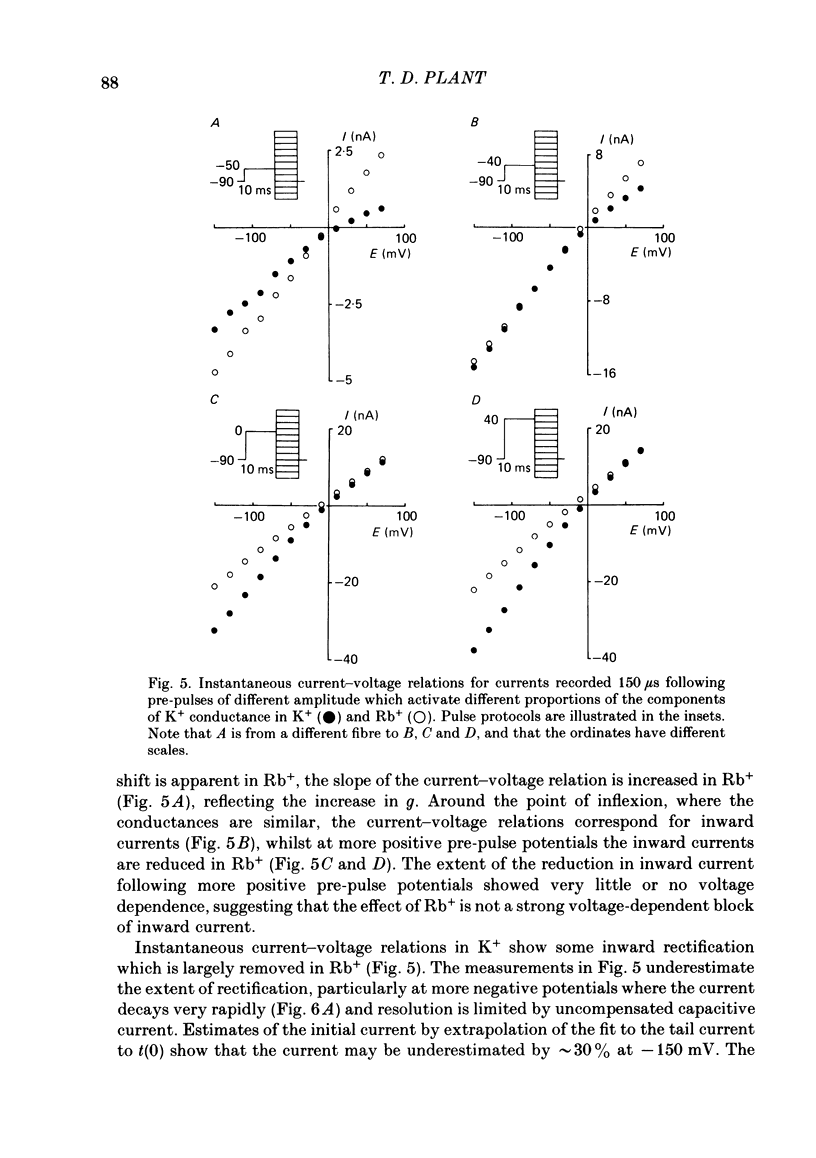
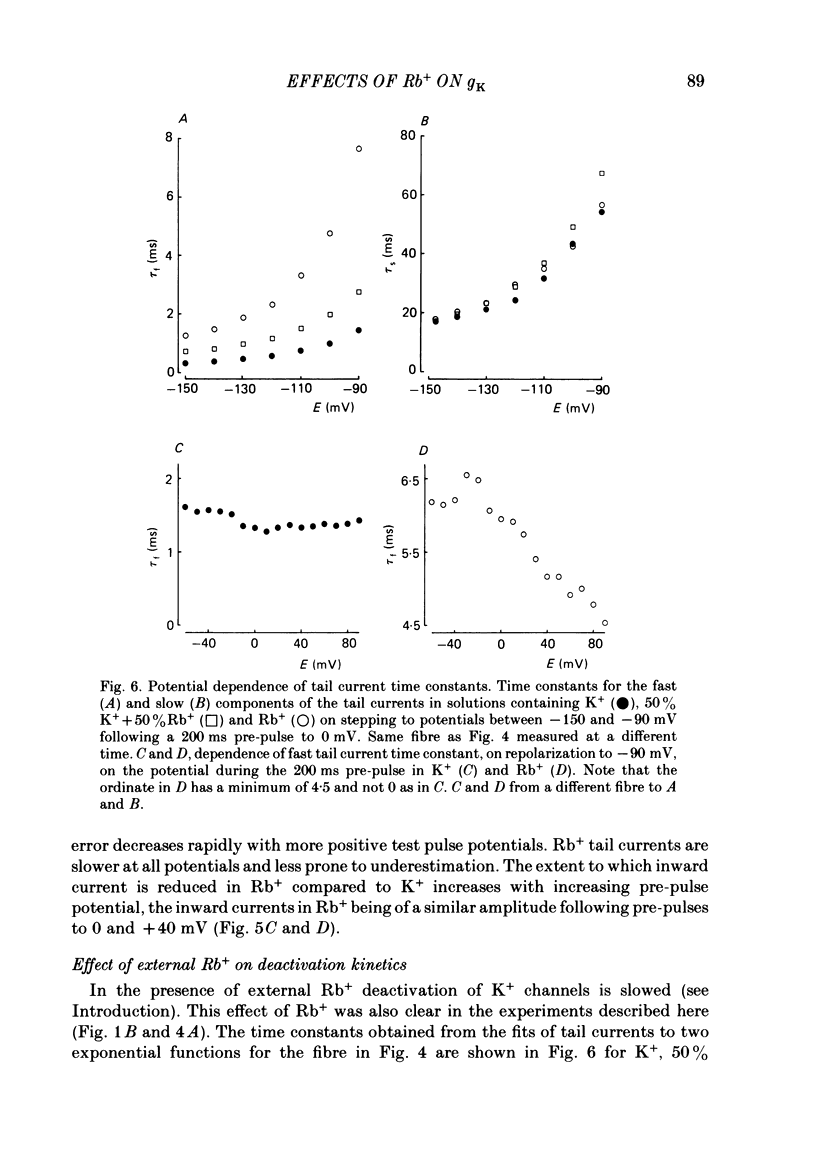
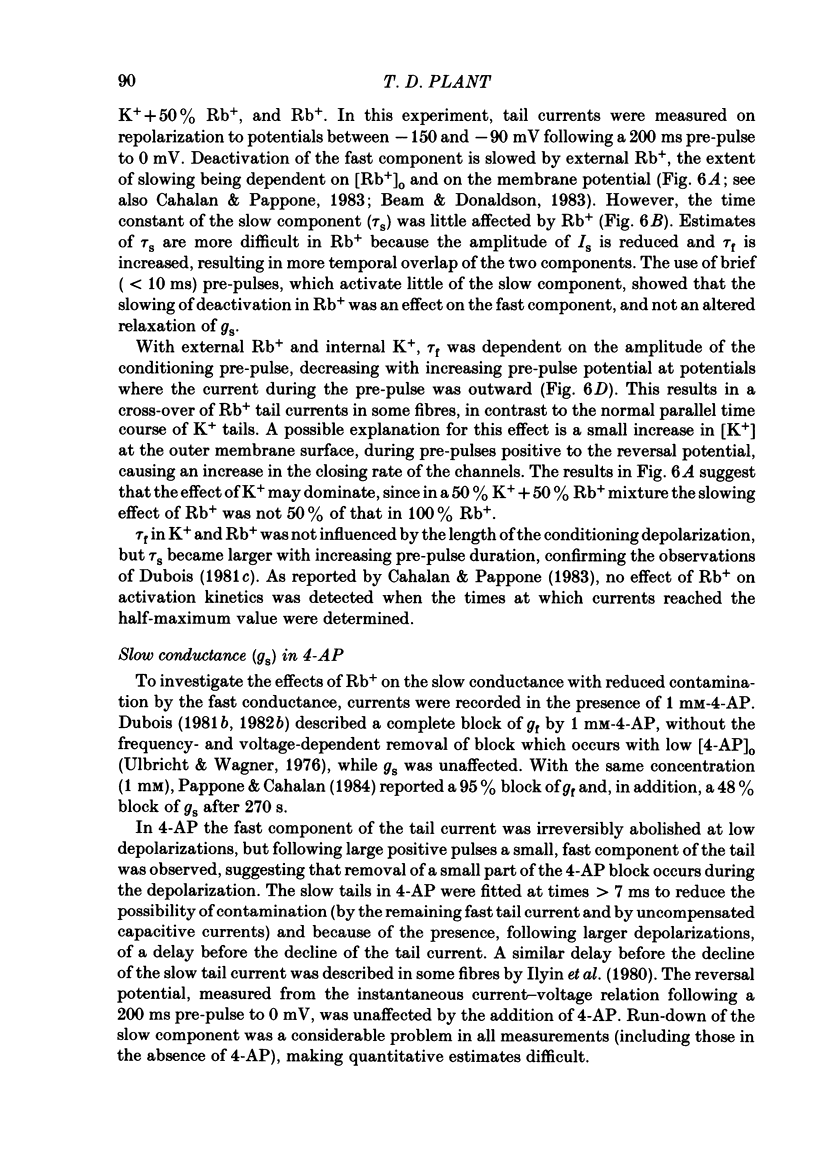
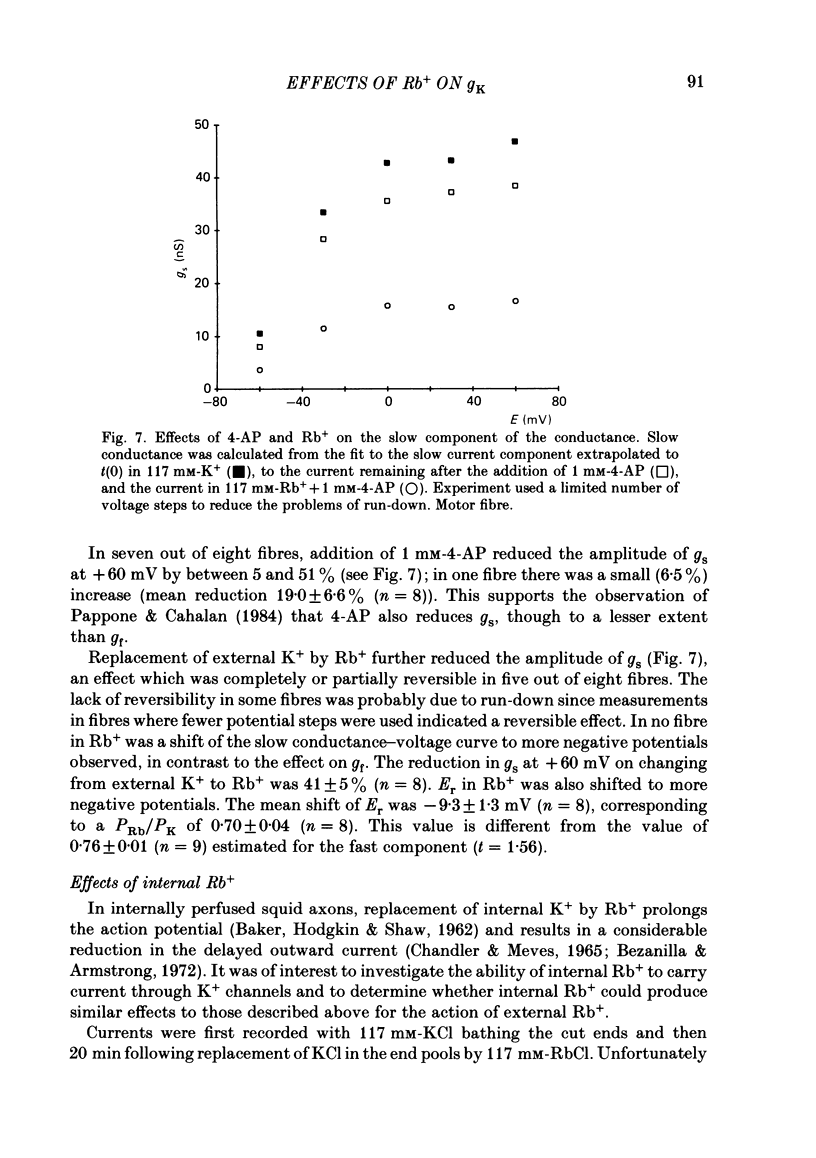
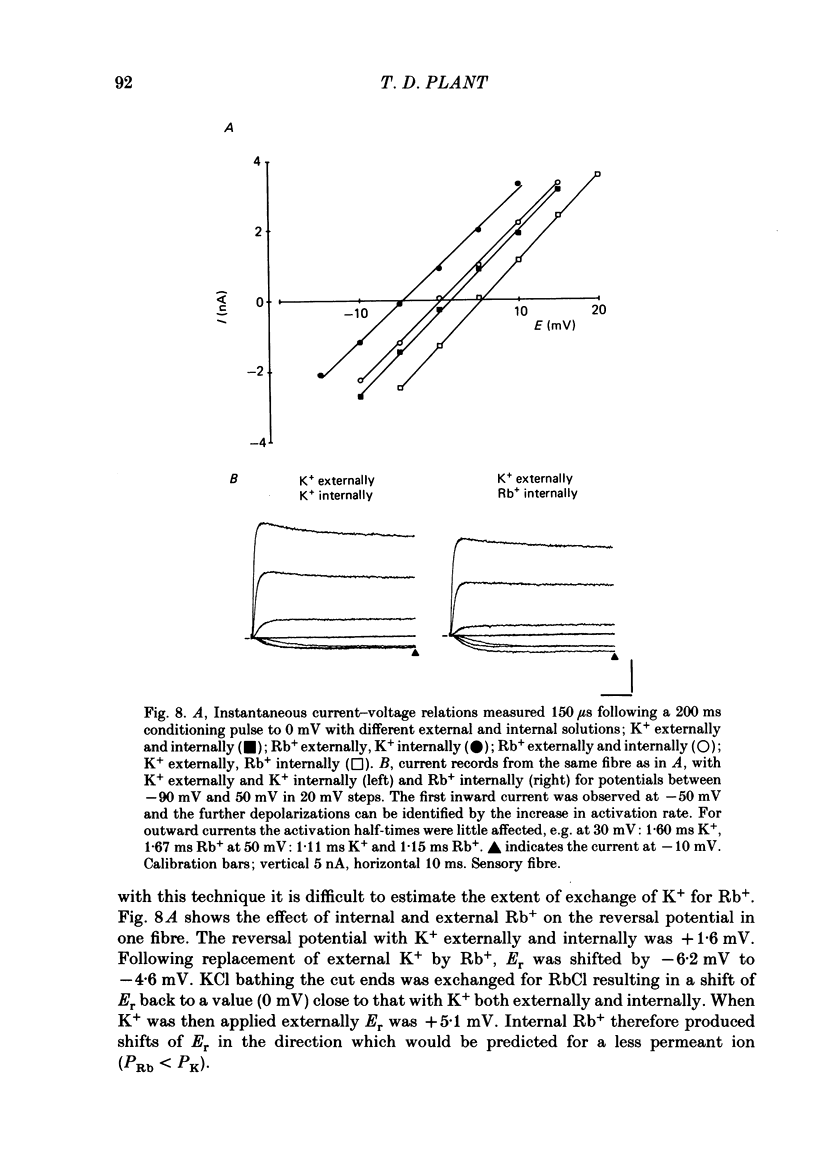
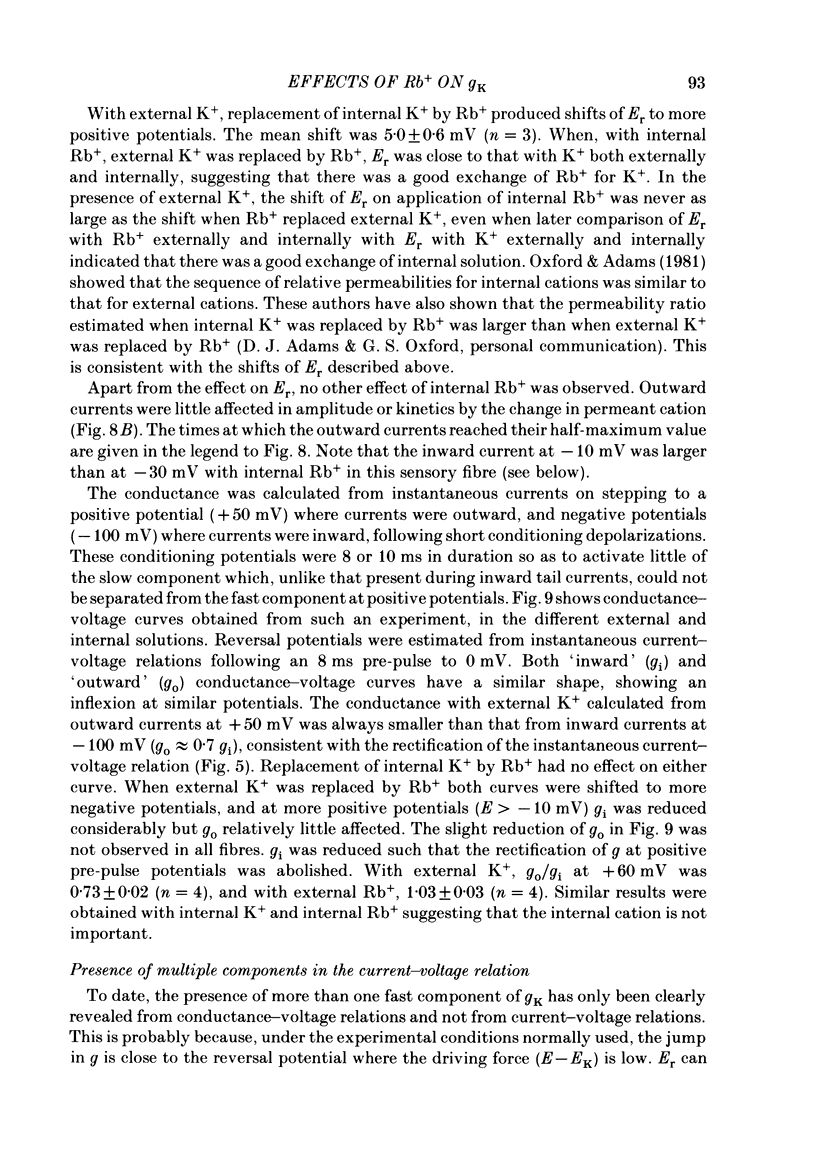
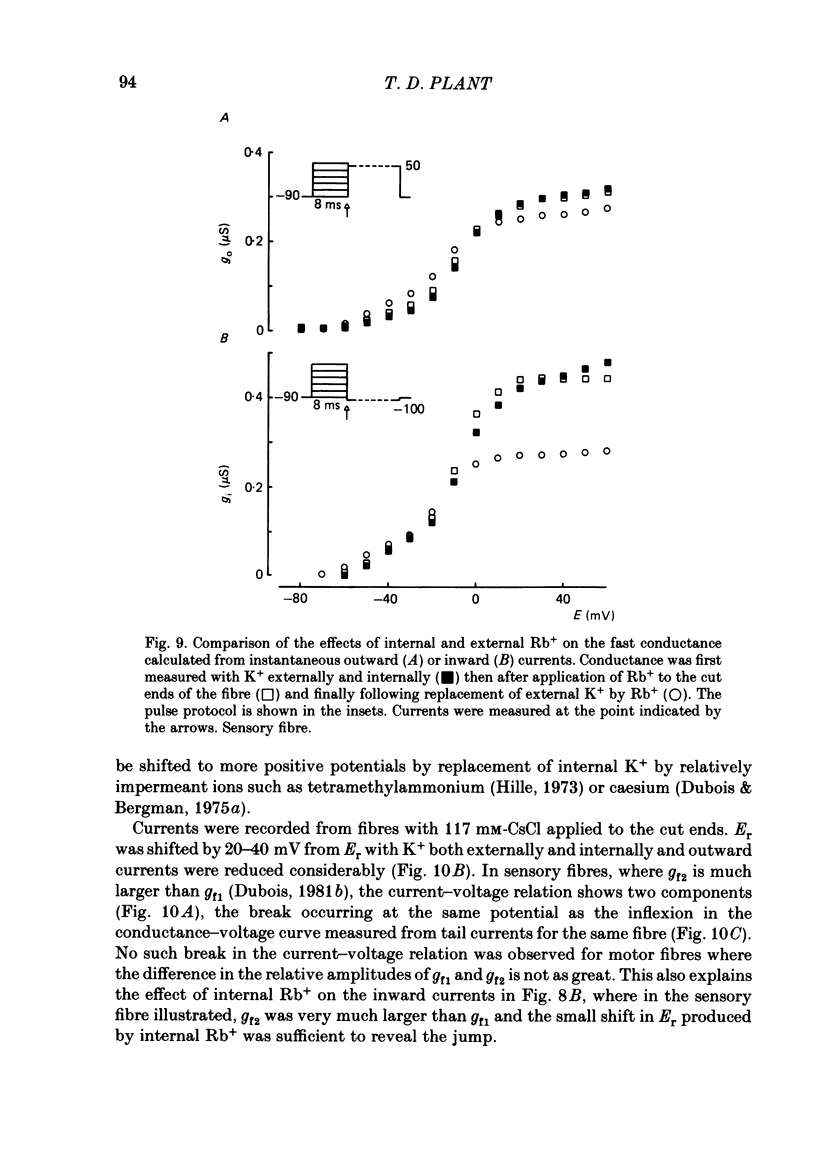
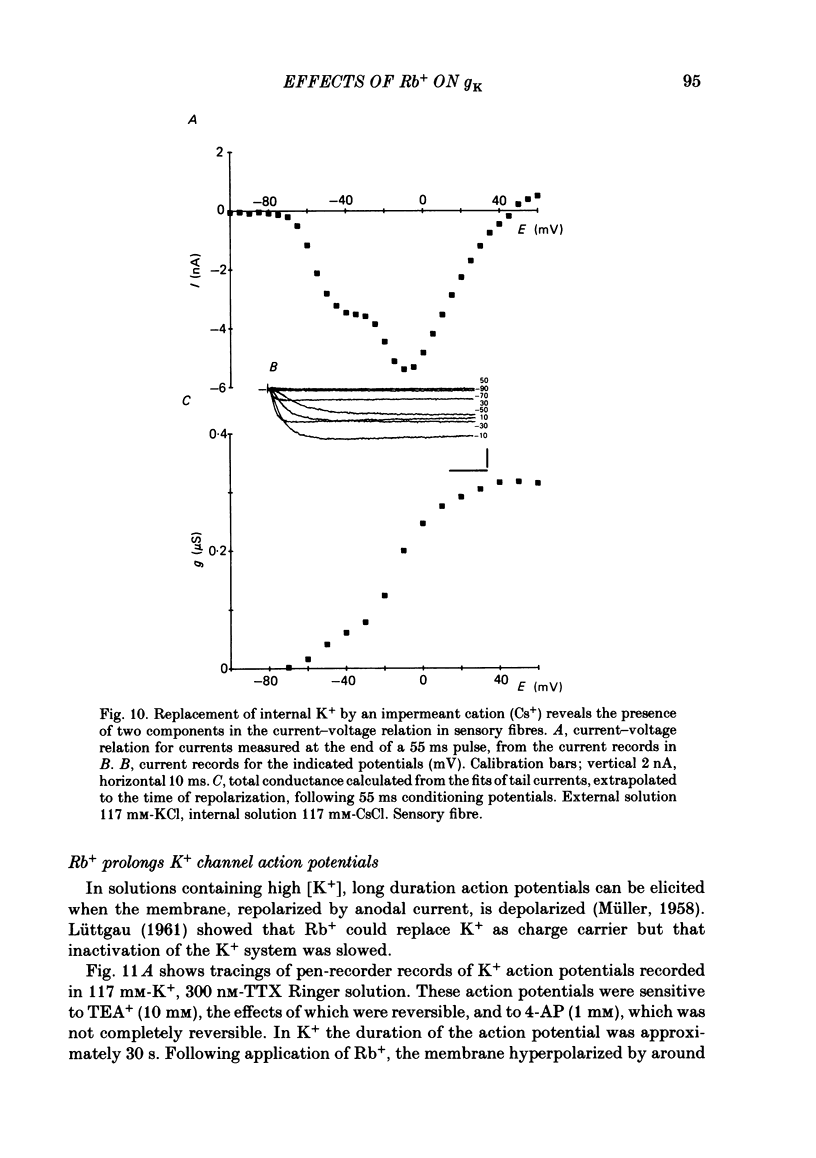
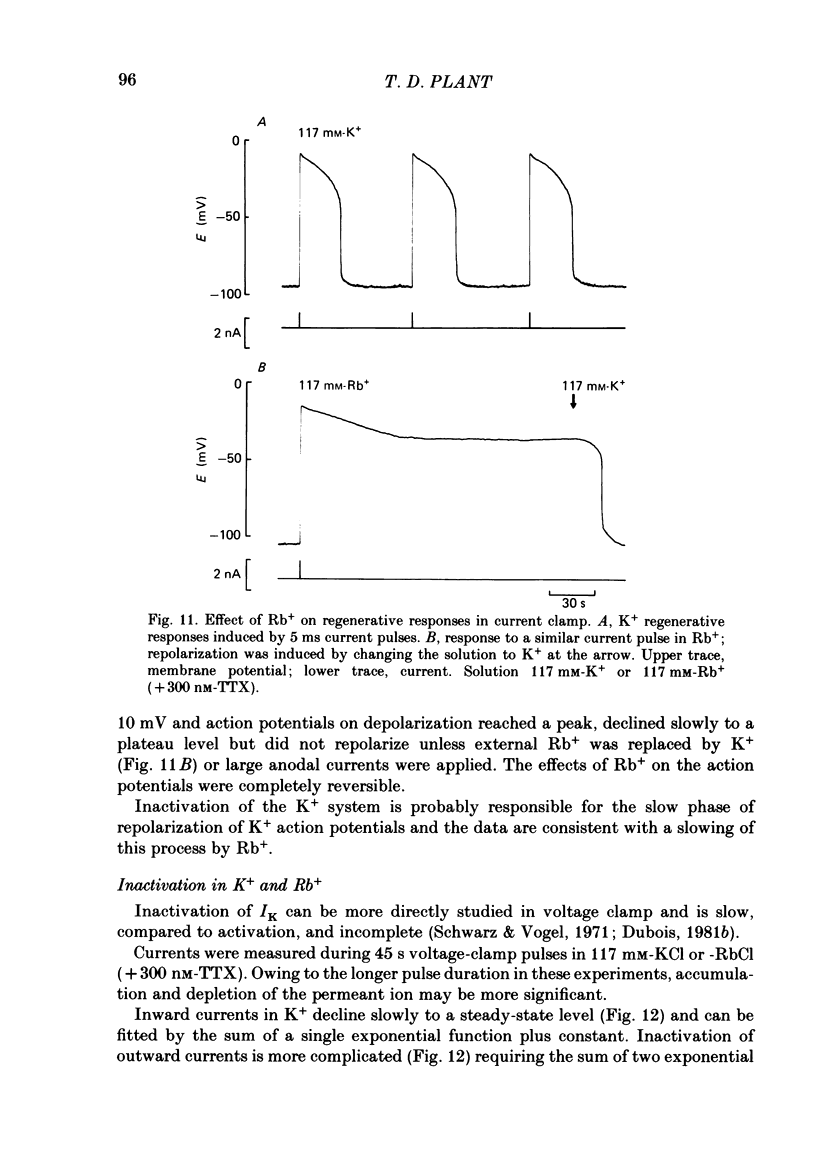
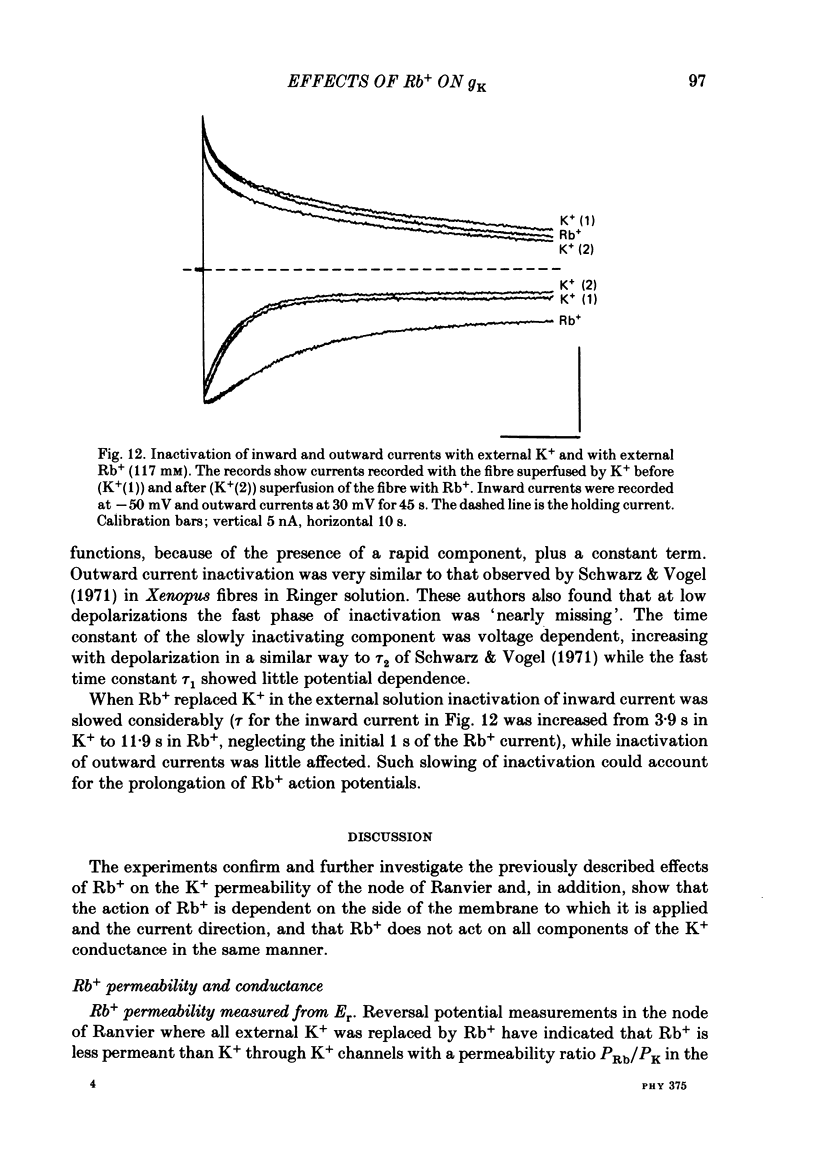
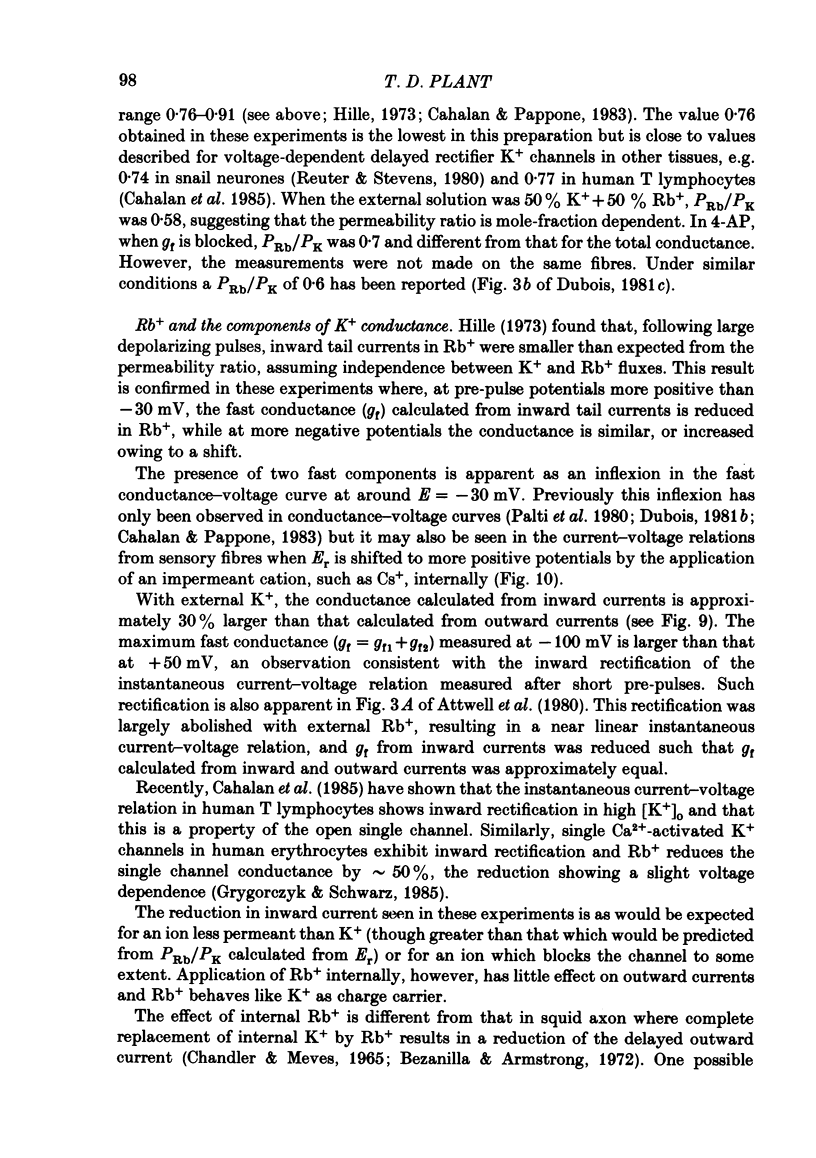
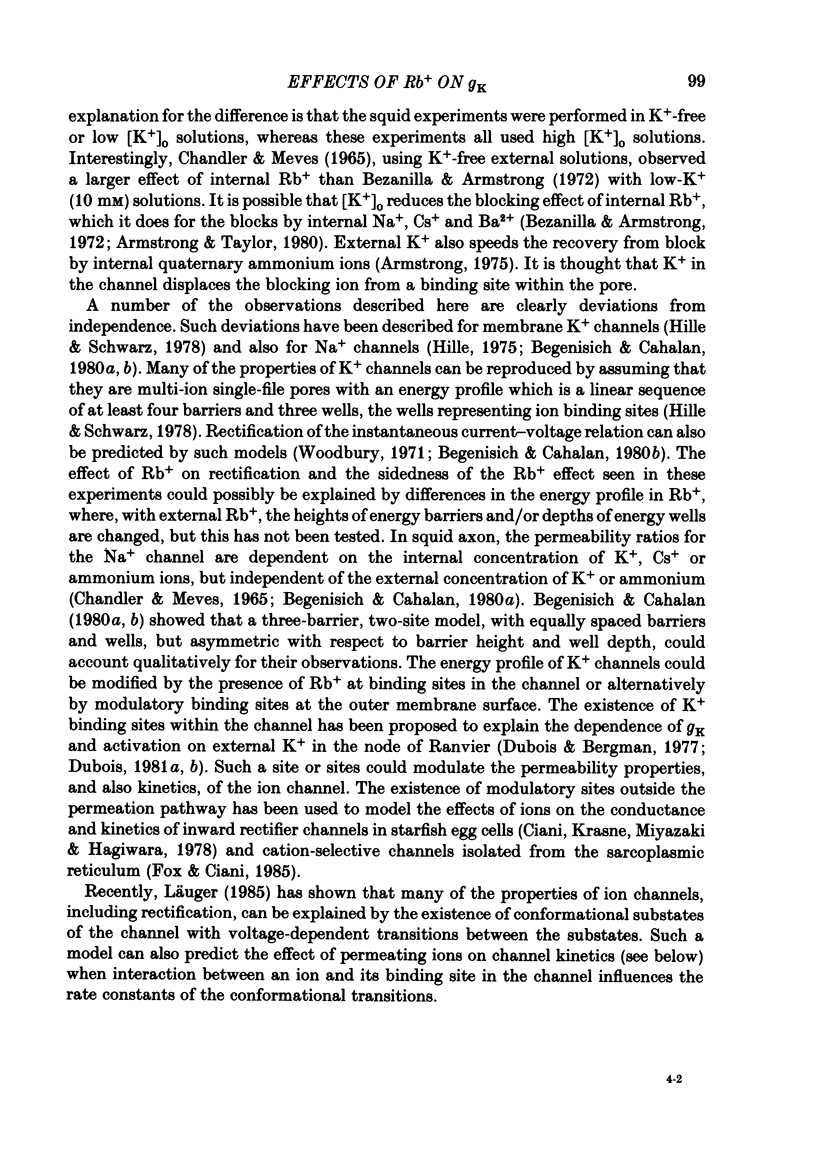
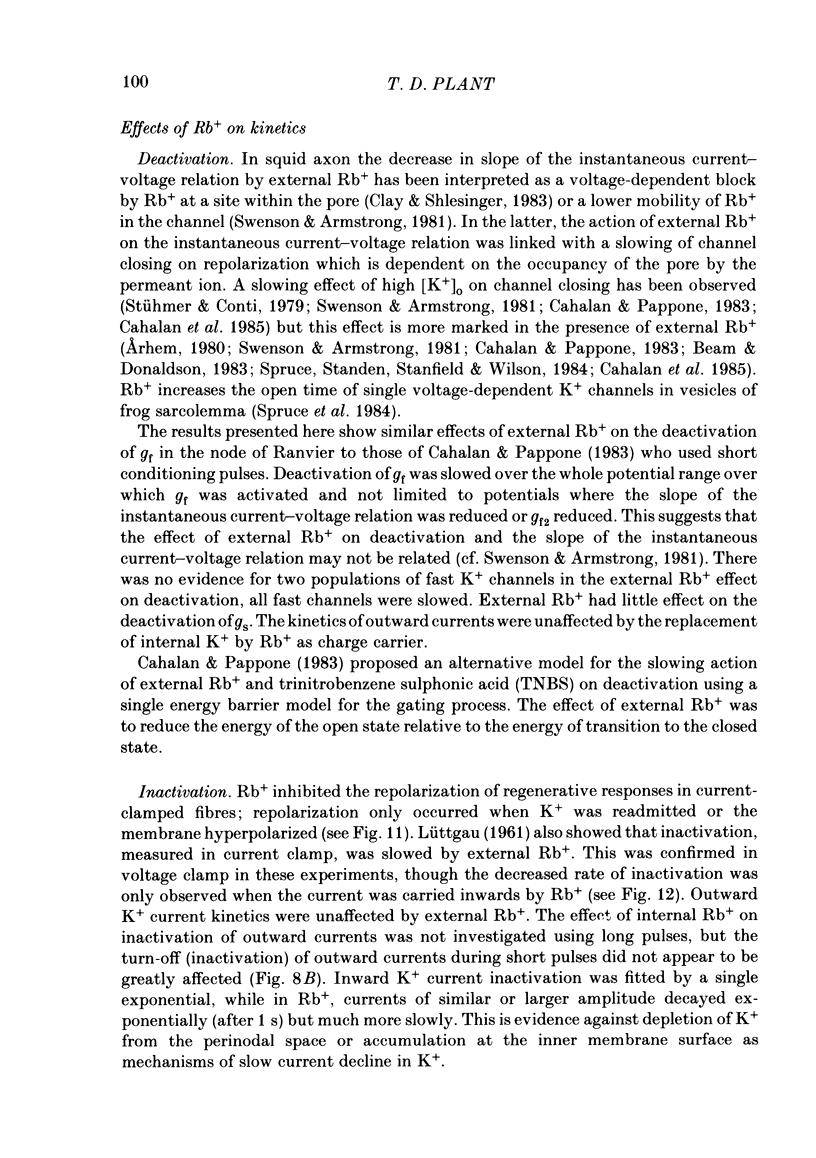
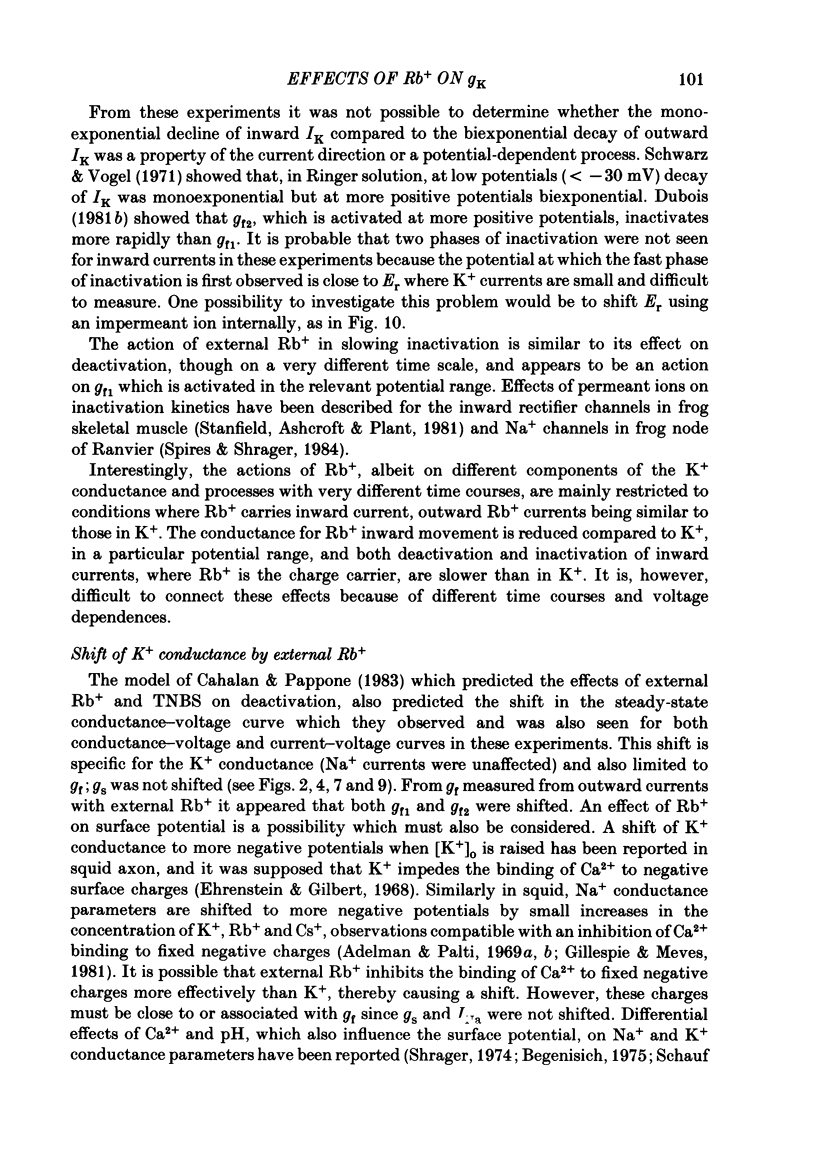
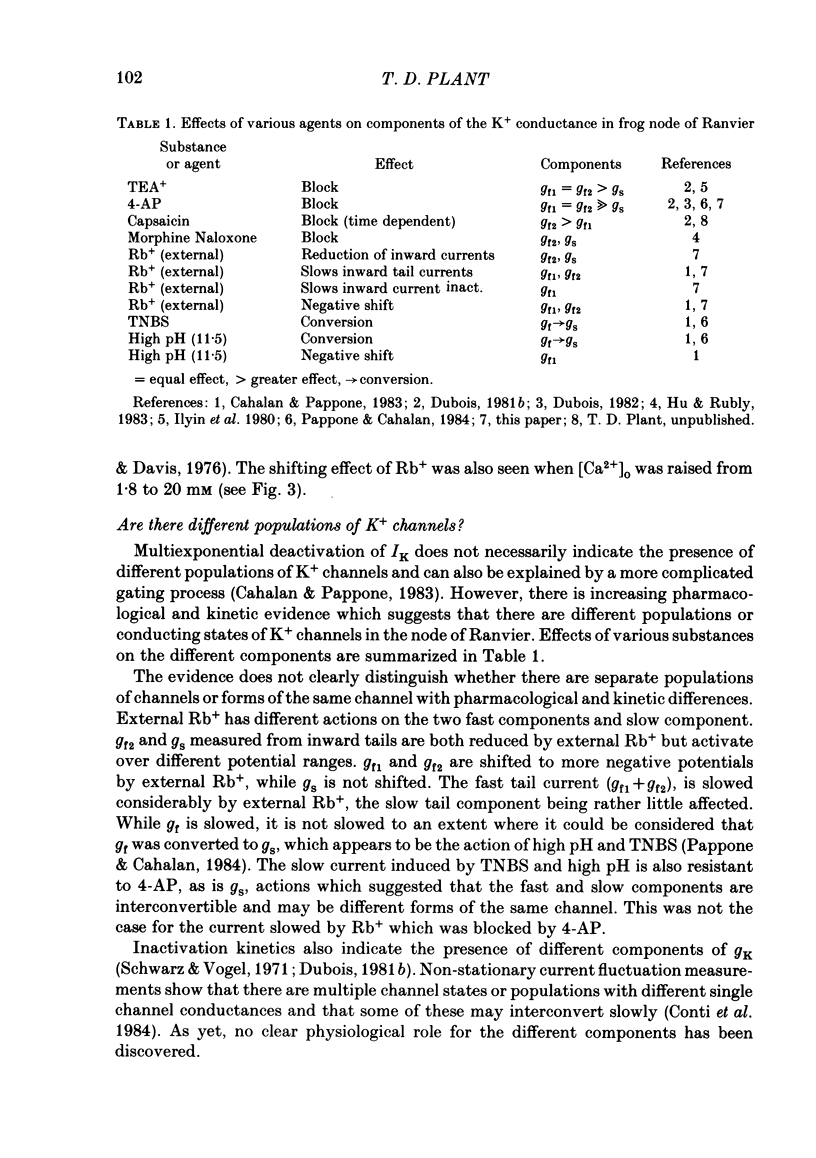
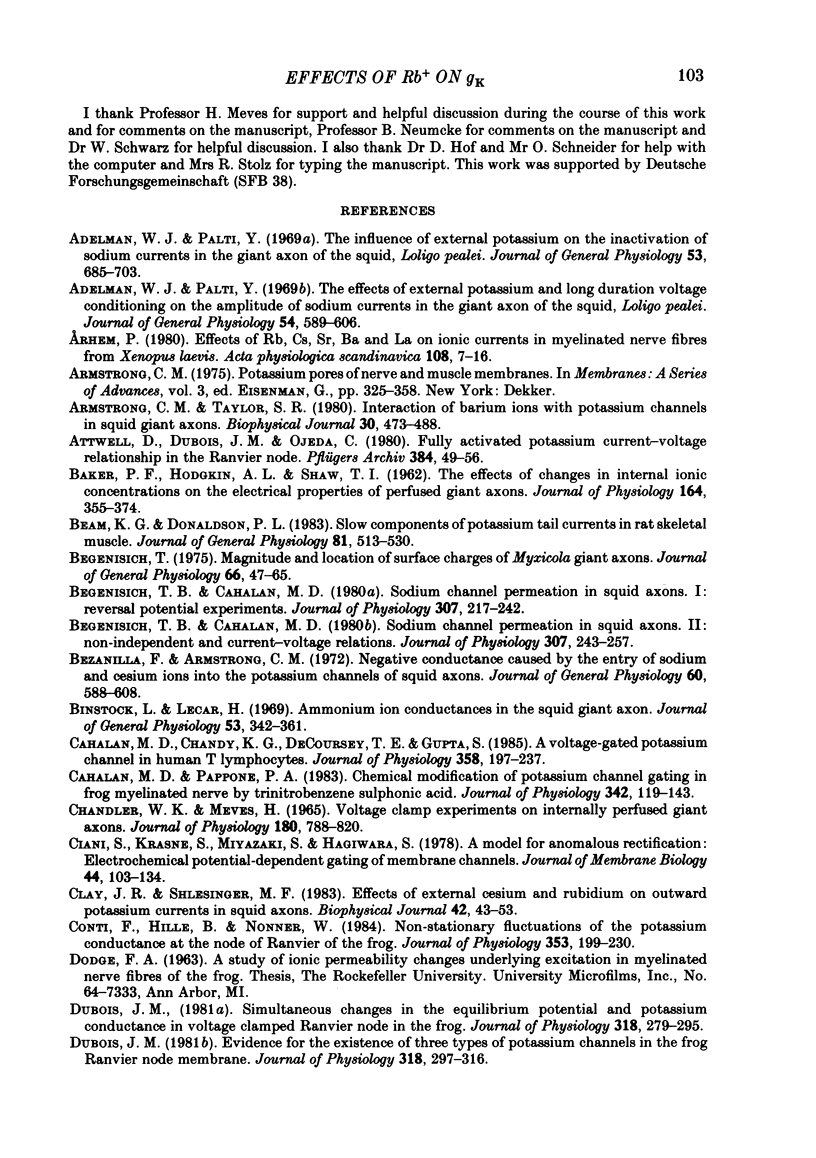
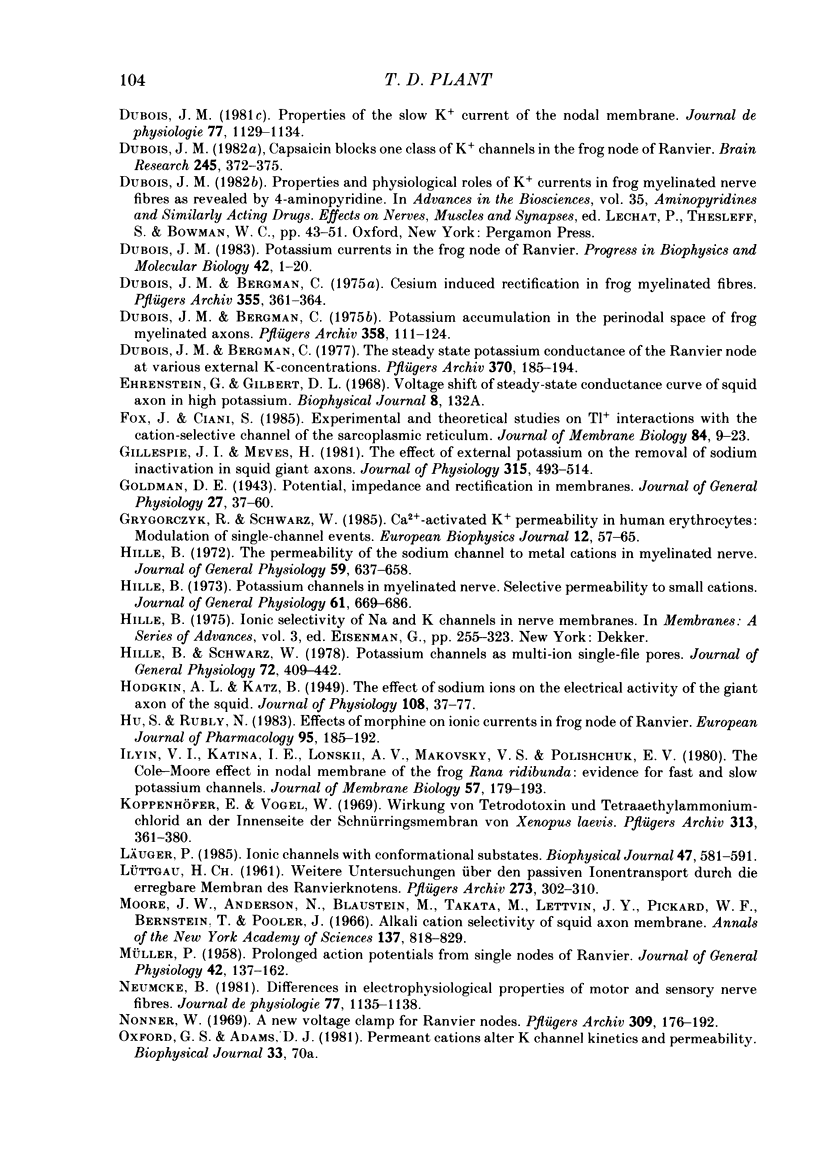
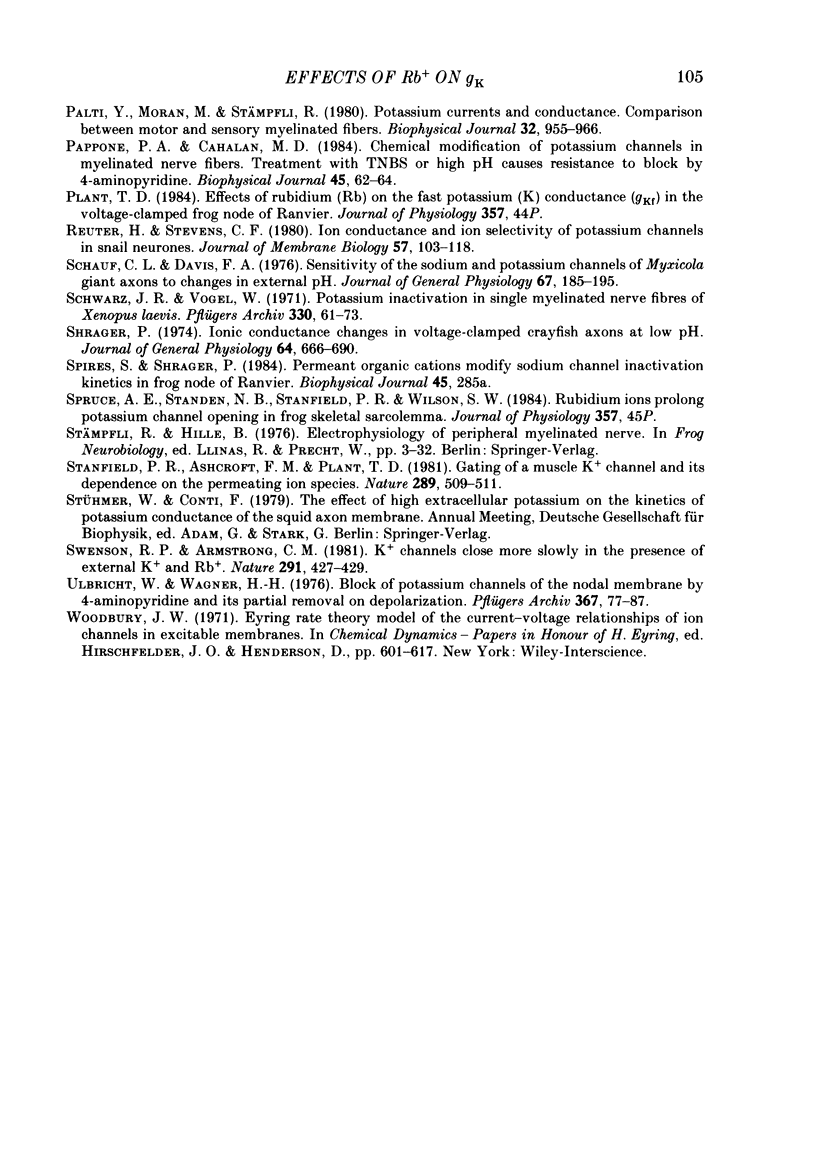
Selected References
These references are in PubMed. This may not be the complete list of references from this article.
- Adelman W. J., Jr, Palti Y. The effects of external potassium and long duration voltage conditioning on the amplitude of sodium currents in the giant axon of the squid, Loligo pealei. J Gen Physiol. 1969 Nov;54(5):589–606. doi: 10.1085/jgp.54.5.589. [DOI] [PMC free article] [PubMed] [Google Scholar]
- Adelman W. J., Jr, Palti Y. The influence of external potassium on the inactivation of sodium currents in the giant axon of the squid, Loligo pealei. J Gen Physiol. 1969 Jun;53(6):685–703. doi: 10.1085/jgp.53.6.685. [DOI] [PMC free article] [PubMed] [Google Scholar]
- Armstrong C. M. Potassium pores of nerve and muscle membranes. Membranes. 1975;3:325–358. [PubMed] [Google Scholar]
- Armstrong C. M., Taylor S. R. Interaction of barium ions with potassium channels in squid giant axons. Biophys J. 1980 Jun;30(3):473–488. doi: 10.1016/S0006-3495(80)85108-3. [DOI] [PMC free article] [PubMed] [Google Scholar]
- Attwell D., Dubois J. M., Ojeda C. Fully activated potassium current-voltage relationship in the Ranvier node: discrepancy between the results of two methods of analysis. Pflugers Arch. 1980 Mar;384(1):49–56. doi: 10.1007/BF00589513. [DOI] [PubMed] [Google Scholar]
- BAKER P. F., HODGKIN A. L., SHAW T. I. The effects of changes in internal ionic concentrations on the electrical properties of perfused giant axons. J Physiol. 1962 Nov;164:355–374. doi: 10.1113/jphysiol.1962.sp007026. [DOI] [PMC free article] [PubMed] [Google Scholar]
- Beam K. G., Donaldson P. L. Slow components of potassium tail currents in rat skeletal muscle. J Gen Physiol. 1983 Apr;81(4):513–530. doi: 10.1085/jgp.81.4.513. [DOI] [PMC free article] [PubMed] [Google Scholar]
- Begenisich T. B., Cahalan M. D. Sodium channel permeation in squid axons. I: Reversal potential experiments. J Physiol. 1980 Oct;307:217–242. doi: 10.1113/jphysiol.1980.sp013432. [DOI] [PMC free article] [PubMed] [Google Scholar]
- Begenisich T. B., Cahalan M. D. Sodium channel permeation in squid axons. II: Non-independence and current-voltage relations. J Physiol. 1980 Oct;307:243–257. doi: 10.1113/jphysiol.1980.sp013433. [DOI] [PMC free article] [PubMed] [Google Scholar]
- Begenisich T. Magnitude and location of surface charges on Myxicola giant axons. J Gen Physiol. 1975 Jul;66(1):47–65. doi: 10.1085/jgp.66.1.47. [DOI] [PMC free article] [PubMed] [Google Scholar]
- Bezanilla F., Armstrong C. M. Negative conductance caused by entry of sodium and cesium ions into the potassium channels of squid axons. J Gen Physiol. 1972 Nov;60(5):588–608. doi: 10.1085/jgp.60.5.588. [DOI] [PMC free article] [PubMed] [Google Scholar]
- Binstock L., Lecar H. Ammonium ion currents in the squid giant axon. J Gen Physiol. 1969 Mar;53(3):342–361. doi: 10.1085/jgp.53.3.342. [DOI] [PMC free article] [PubMed] [Google Scholar]
- Cahalan M. D., Chandy K. G., DeCoursey T. E., Gupta S. A voltage-gated potassium channel in human T lymphocytes. J Physiol. 1985 Jan;358:197–237. doi: 10.1113/jphysiol.1985.sp015548. [DOI] [PMC free article] [PubMed] [Google Scholar]
- Cahalan M. D., Pappone P. A. Chemical modification of potassium channel gating in frog myelinated nerve by trinitrobenzene sulphonic acid. J Physiol. 1983 Sep;342:119–143. doi: 10.1113/jphysiol.1983.sp014843. [DOI] [PMC free article] [PubMed] [Google Scholar]
- Chandler W. K., Meves H. Voltage clamp experiments on internally perfused giant axons. J Physiol. 1965 Oct;180(4):788–820. doi: 10.1113/jphysiol.1965.sp007732. [DOI] [PMC free article] [PubMed] [Google Scholar]
- Ciani S., Krasne S., Miyazaki S., Hagiwara S. A model for anomalous rectification: electrochemical-potential-dependent gating of membrane channels. J Membr Biol. 1978 Dec 15;44(2):103–134. doi: 10.1007/BF01976035. [DOI] [PubMed] [Google Scholar]
- Clay J. R., Shlesinger M. F. Effects of external cesium and rubidium on outward potassium currents in squid axons. Biophys J. 1983 Apr;42(1):43–53. doi: 10.1016/S0006-3495(83)84367-7. [DOI] [PMC free article] [PubMed] [Google Scholar]
- Conti F., Hille B., Nonner W. Non-stationary fluctuations of the potassium conductance at the node of ranvier of the frog. J Physiol. 1984 Aug;353:199–230. doi: 10.1113/jphysiol.1984.sp015332. [DOI] [PMC free article] [PubMed] [Google Scholar]
- Dubois J. M., Bergman C. Cesium induced rectifications in frog myelinated fibres. Pflugers Arch. 1975 Apr 2;355(4):361–364. doi: 10.1007/BF00579857. [DOI] [PubMed] [Google Scholar]
- Dubois J. M., Bergman C. Potassium accumulation in the perinodal space of frog myelinated axons. Pflugers Arch. 1975 Jul 21;358(2):111–124. doi: 10.1007/BF00583922. [DOI] [PubMed] [Google Scholar]
- Dubois J. M., Bergman C. The steady-state potassium conductance of the Ranvier node at various external K-concentrations. Pflugers Arch. 1977 Aug 29;370(2):185–194. doi: 10.1007/BF00581693. [DOI] [PubMed] [Google Scholar]
- Dubois J. M. Capsaicin blocks one class of K+ channels in the frog node of Ranvier. Brain Res. 1982 Aug 12;245(2):372–375. doi: 10.1016/0006-8993(82)90820-4. [DOI] [PubMed] [Google Scholar]
- Dubois J. M. Evidence for the existence of three types of potassium channels in the frog Ranvier node membrane. J Physiol. 1981 Sep;318:297–316. doi: 10.1113/jphysiol.1981.sp013865. [DOI] [PMC free article] [PubMed] [Google Scholar]
- Dubois J. M. Potassium currents in the frog node of Ranvier. Prog Biophys Mol Biol. 1983;42(1):1–20. doi: 10.1016/0079-6107(83)90002-0. [DOI] [PubMed] [Google Scholar]
- Dubois J. M. Properties of the slow K+ current of the nodal membrane. J Physiol (Paris) 1981 May;77(9):1129–1134. [PubMed] [Google Scholar]
- Dubois J. M. Simultaneous changes in the equilibrium potential and potassium conductance in voltage clamped Ranvier node in the frog. J Physiol. 1981 Sep;318:279–295. doi: 10.1113/jphysiol.1981.sp013864. [DOI] [PMC free article] [PubMed] [Google Scholar]
- Fox J., Ciani S. Experimental and theoretical studies on Tl+ interactions with the cation-selective channel of the sarcoplasmic reticulum. J Membr Biol. 1985;84(1):9–23. doi: 10.1007/BF01871644. [DOI] [PubMed] [Google Scholar]
- Gillespie J. I., Meves H. The effect of external potassium on the removal of sodium inactivation in squid giant axons. J Physiol. 1981 Jun;315:493–514. doi: 10.1113/jphysiol.1981.sp013760. [DOI] [PMC free article] [PubMed] [Google Scholar]
- Goldman D. E. POTENTIAL, IMPEDANCE, AND RECTIFICATION IN MEMBRANES. J Gen Physiol. 1943 Sep 20;27(1):37–60. doi: 10.1085/jgp.27.1.37. [DOI] [PMC free article] [PubMed] [Google Scholar]
- Grygorczyk R., Schwarz W. Ca2+-activated K+ permeability in human erythrocytes: modulation of single-channel events. Eur Biophys J. 1985;12(2):57–65. doi: 10.1007/BF00260428. [DOI] [PubMed] [Google Scholar]
- HODGKIN A. L., KATZ B. The effect of sodium ions on the electrical activity of giant axon of the squid. J Physiol. 1949 Mar 1;108(1):37–77. doi: 10.1113/jphysiol.1949.sp004310. [DOI] [PMC free article] [PubMed] [Google Scholar]
- Hille B. Ionic selectivity of Na and K channels of nerve membranes. Membranes. 1975;3:255–323. [PubMed] [Google Scholar]
- Hille B. Potassium channels in myelinated nerve. Selective permeability to small cations. J Gen Physiol. 1973 Jun;61(6):669–686. doi: 10.1085/jgp.61.6.669. [DOI] [PMC free article] [PubMed] [Google Scholar]
- Hille B., Schwarz W. Potassium channels as multi-ion single-file pores. J Gen Physiol. 1978 Oct;72(4):409–442. doi: 10.1085/jgp.72.4.409. [DOI] [PMC free article] [PubMed] [Google Scholar]
- Hille B. The permeability of the sodium channel to metal cations in myelinated nerve. J Gen Physiol. 1972 Jun;59(6):637–658. doi: 10.1085/jgp.59.6.637. [DOI] [PMC free article] [PubMed] [Google Scholar]
- Ilyin V. I., Katina I. E., Lonskii A. V., Makovsky V. S., Polishchuk E. V. The Cole-Moore effect in nodal membrane of the frog Rana ridibunda: evidence for fast and slow potassium channels. J Membr Biol. 1980 Dec 30;57(3):179–193. doi: 10.1007/BF01869586. [DOI] [PubMed] [Google Scholar]
- Koppenhöfer E., Vogel W. Wirkung von Tetrodotoxin und Tetraäthylammoniumchlorid an der Innenseite der Schnürringsmembran von Xenopus laevis. Pflugers Arch. 1969;313(4):361–380. doi: 10.1007/BF00593959. [DOI] [PubMed] [Google Scholar]
- Läuger P. Ionic channels with conformational substates. Biophys J. 1985 May;47(5):581–590. doi: 10.1016/S0006-3495(85)83954-0. [DOI] [PMC free article] [PubMed] [Google Scholar]
- MUELLER P. Prolonged action potentials from single nodes of Ranvier. J Gen Physiol. 1958 Sep 20;42(1):137–162. doi: 10.1085/jgp.42.1.137. [DOI] [PMC free article] [PubMed] [Google Scholar]
- Moore J. W., Anderson N., Blaustein M., Takata M., Lettvin J. Y., Pickard W. F., Bernstein T., Pooler J. Alkali cation selectivity of squid axon membrane. Ann N Y Acad Sci. 1966 Jul 14;137(2):818–829. doi: 10.1111/j.1749-6632.1966.tb50202.x. [DOI] [PubMed] [Google Scholar]
- Neumcke B. Differences in electrophysiological properties of motor and sensory nerve fibres. J Physiol (Paris) 1981 May;77(9):1135–1138. [PubMed] [Google Scholar]
- Nonner W. A new voltage clamp method for Ranvier nodes. Pflugers Arch. 1969;309(2):176–192. doi: 10.1007/BF00586967. [DOI] [PubMed] [Google Scholar]
- Palti Y., Moran N., Stämpfli R. Potassium currents and conductance. Comparison between motor and sensory myelinated fibers. Biophys J. 1980 Dec;32(3):955–966. doi: 10.1016/S0006-3495(80)85029-6. [DOI] [PMC free article] [PubMed] [Google Scholar]
- Pappone P. A., Cahalan M. D. Chemical Modification of Potassium Channels in Myelinated Nerve Fibers: Treatment With TNBS or High pH Causes Resistance to Block by 4-Aminopyridine. Biophys J. 1984 Jan;45(1):62–64. doi: 10.1016/S0006-3495(84)84109-0. [DOI] [PMC free article] [PubMed] [Google Scholar]
- Reuter H., Stevens C. F. Ion conductance and ion selectivity of potassium channels in snail neurones. J Membr Biol. 1980 Dec 15;57(2):103–118. doi: 10.1007/BF01868997. [DOI] [PubMed] [Google Scholar]
- Schauf C. L., Davis F. A. Sensitivity of the sodium and potassium channels of Myxicola giant axons to changes in external pH. J Gen Physiol. 1976 Feb;67(2):185–195. doi: 10.1085/jgp.67.2.185. [DOI] [PMC free article] [PubMed] [Google Scholar]
- Schwarz J. R., Vogel W. Potassium inactivation in single myelinated nerve fibres of Xenopus laevis. Pflugers Arch. 1971;330(1):61–73. doi: 10.1007/BF00588735. [DOI] [PubMed] [Google Scholar]
- Shrager P. Ionic conductance changes in voltage clamped crayfish axons at low pH. J Gen Physiol. 1974 Dec;64(6):666–690. doi: 10.1085/jgp.64.6.666. [DOI] [PMC free article] [PubMed] [Google Scholar]
- Stanfield P. R., Ashcroft F. M., Plant T. D. Gating of a muscle K+ channel and its dependence on the permeating ion species. Nature. 1981 Feb 5;289(5797):509–511. doi: 10.1038/289509a0. [DOI] [PubMed] [Google Scholar]
- Swenson R. P., Jr, Armstrong C. M. K+ channels close more slowly in the presence of external K+ and Rb+. Nature. 1981 Jun 4;291(5814):427–429. doi: 10.1038/291427a0. [DOI] [PubMed] [Google Scholar]
- Ulbricht W., Wagner H. H. Block of potassium channels of the nodal membrane by 4-aminopyridine and its partial removal on depolarization. Pflugers Arch. 1976 Nov 30;367(1):77–87. doi: 10.1007/BF00583659. [DOI] [PubMed] [Google Scholar]