Abstract
The fluxes of Na and K across the human red cell membrane have been studied as functions of temperature and external cation composition. In media containing any of a variety of organic compounds as the principal cation (choline, N-methyl-D-glucamine (NMDG), arginine, L-lysine and trimethyl-phenylammonium), the ouabain plus bumetanide-insensitive influxes and effluxes of Na and K displayed marked paradoxical temperature dependence such that the flux minimum, which normally occurs at about 8-10 degrees C in a NaCl medium, was shifted up to about 20 degrees C. Inhibitor and anion replacement studies excluded contributions by the major carrier-mediated systems evident at 37 degrees C. At 0 degrees C in NMDG, about 1 mM-external Na and 10 mM-external K were required half-maximally to inhibit the K and Na influxes respectively. When the K(86Rb) efflux in NMDG media at 0 degrees C was measured in the presence of low concentrations of a series of external inorganic ions and guanidine, the order of potency for reduction of the efflux was Li greater than Mg = Ca greater than Ba greater than Sr greater than Na greater than Rb = Cs = guanidine greater than K. The influxes of the neutral amino acid L-leucine and the cationic species L-lysine both showed simple monotonic temperature dependence in Na and NMDG media. These effects show that the permeability of the human red cell membrane to inorganic univalent cations at low temperatures is markedly dependent on the external ionic conditions. Low permeability is favoured by the presence of cations with a high charge density.
Full text
PDF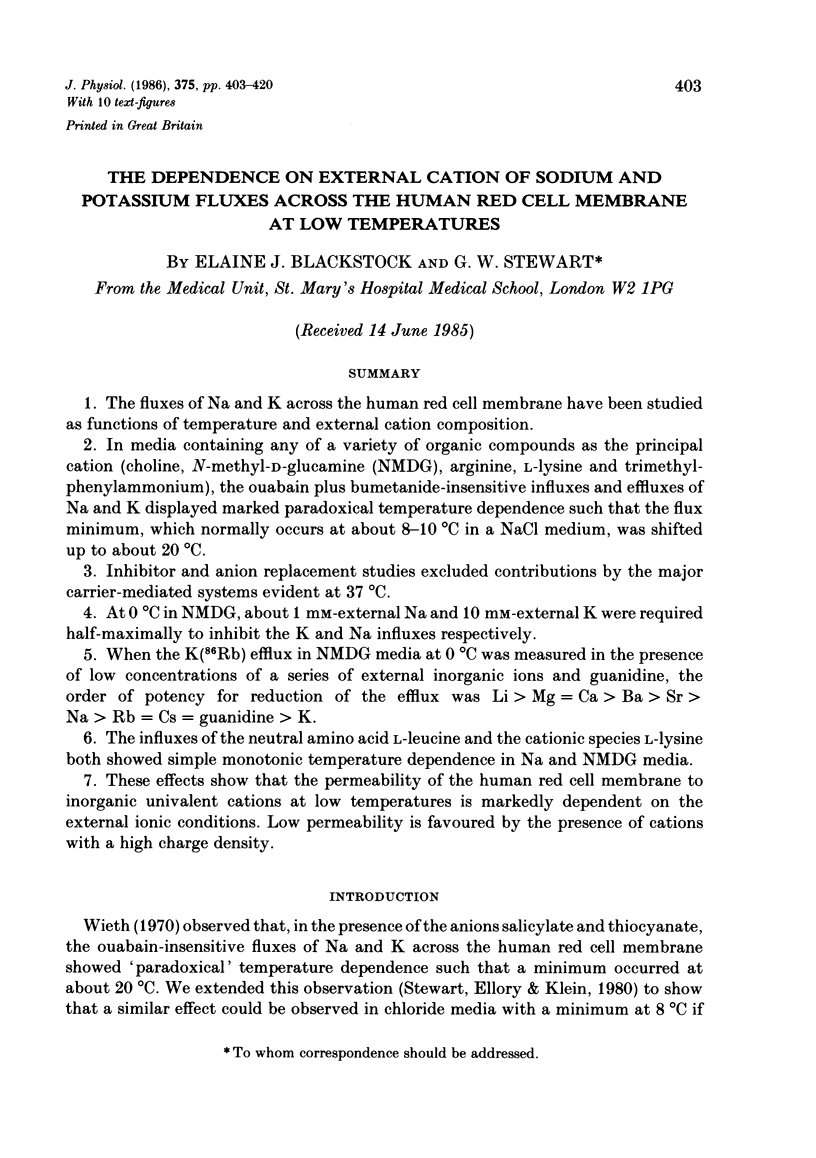
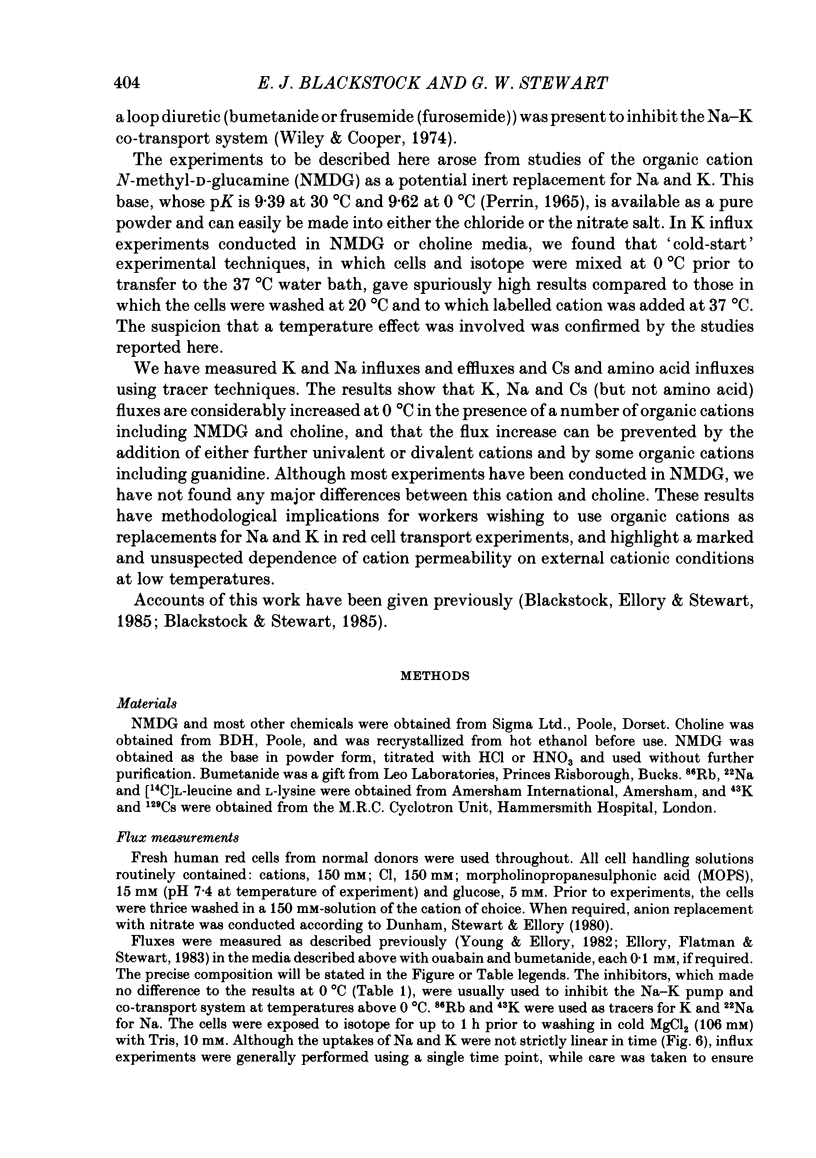
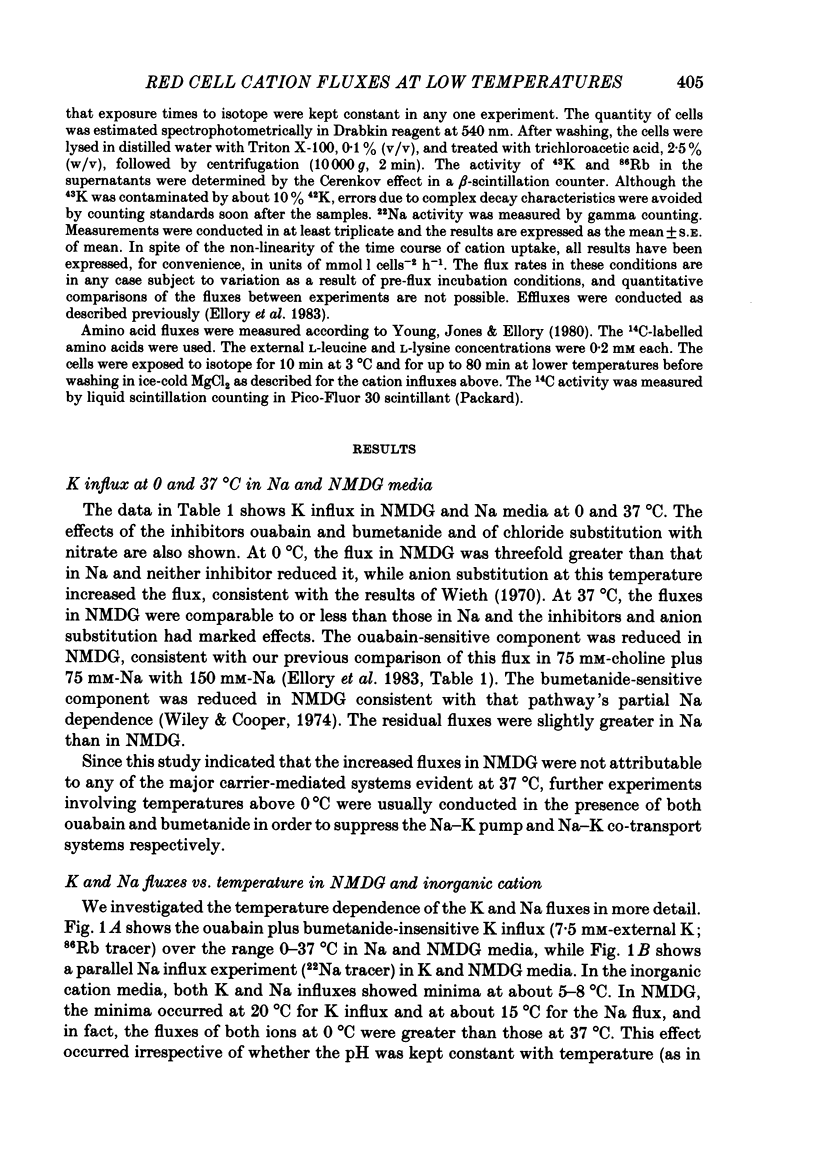
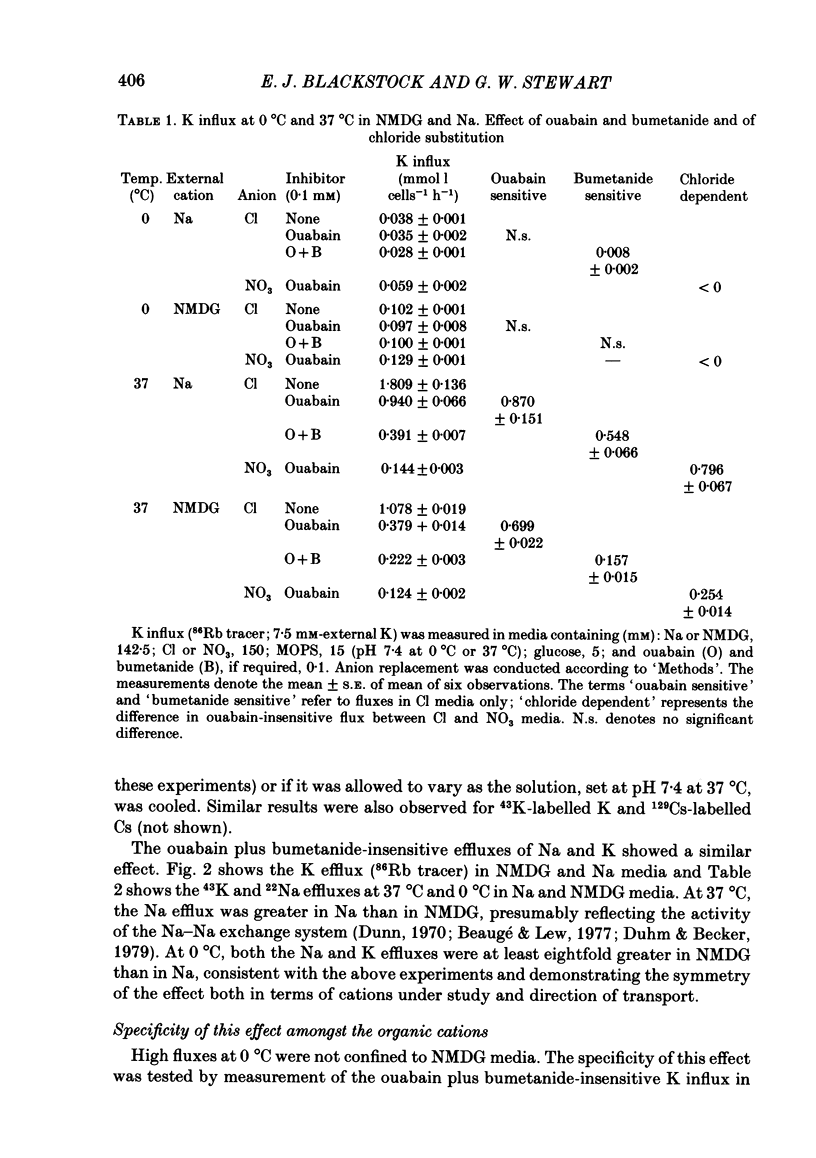
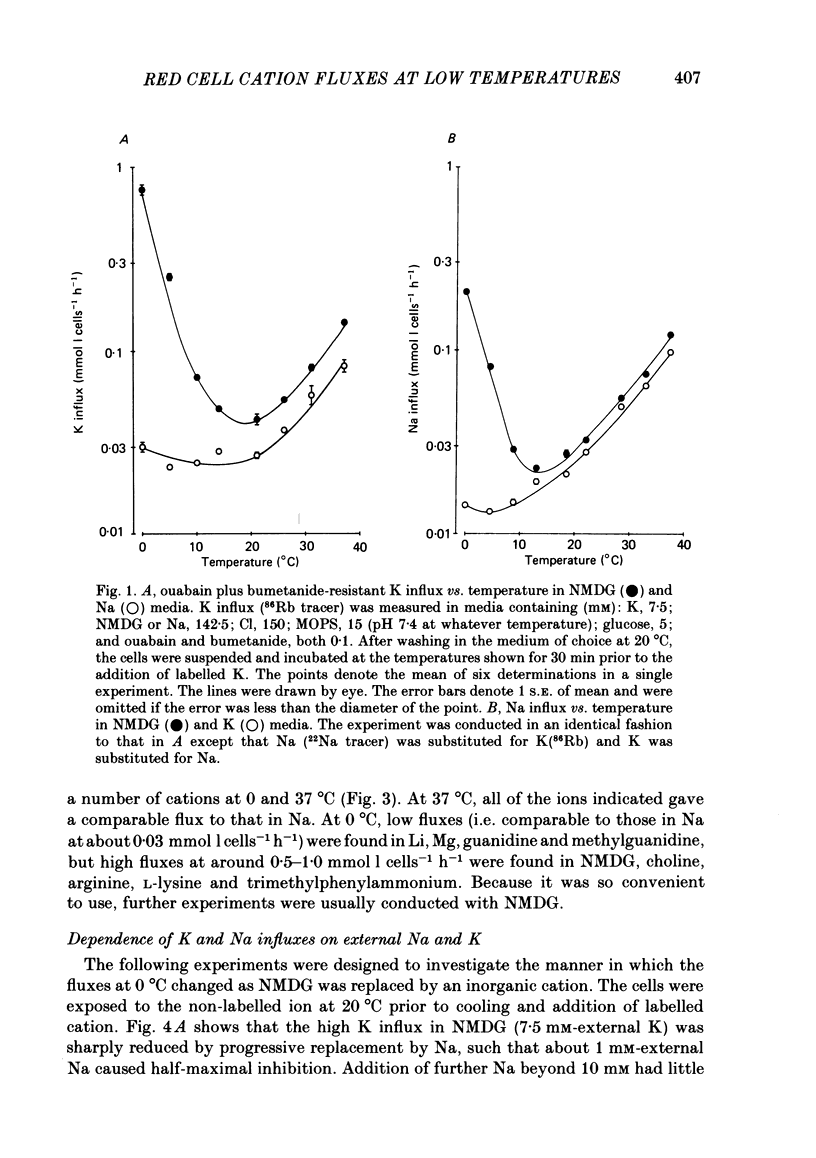
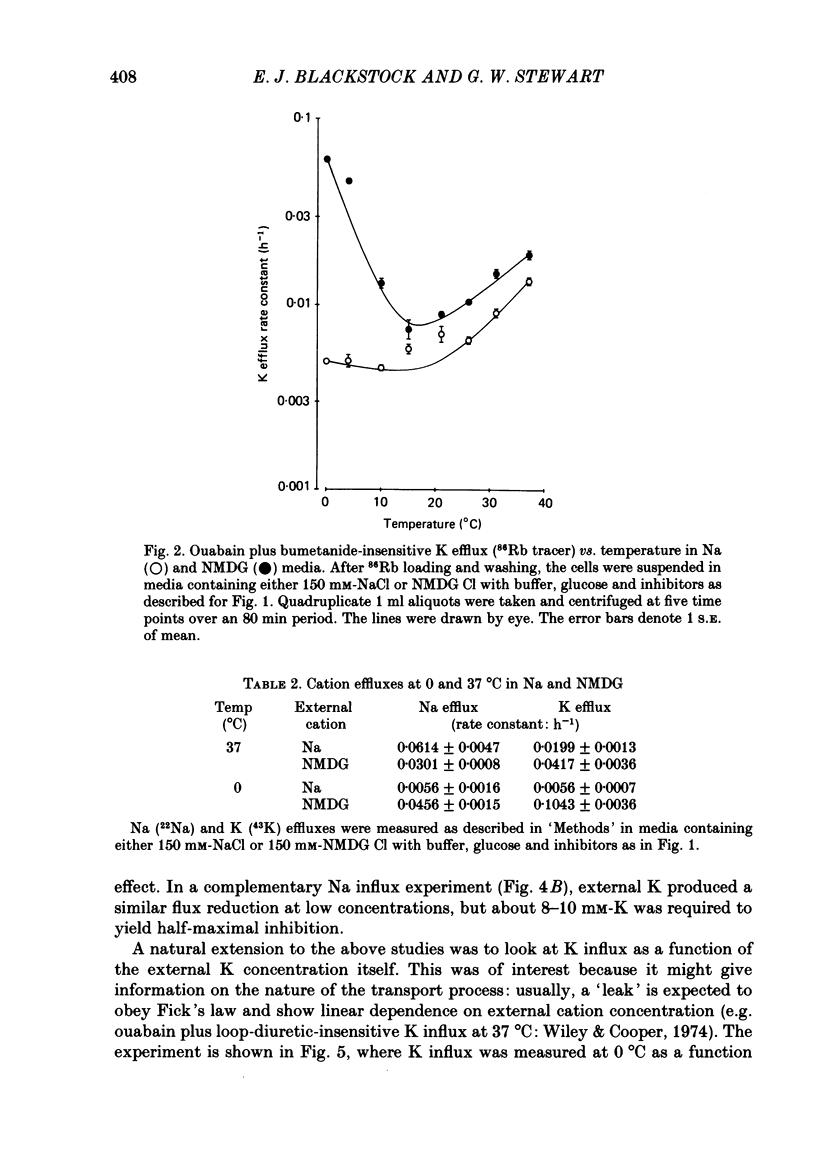
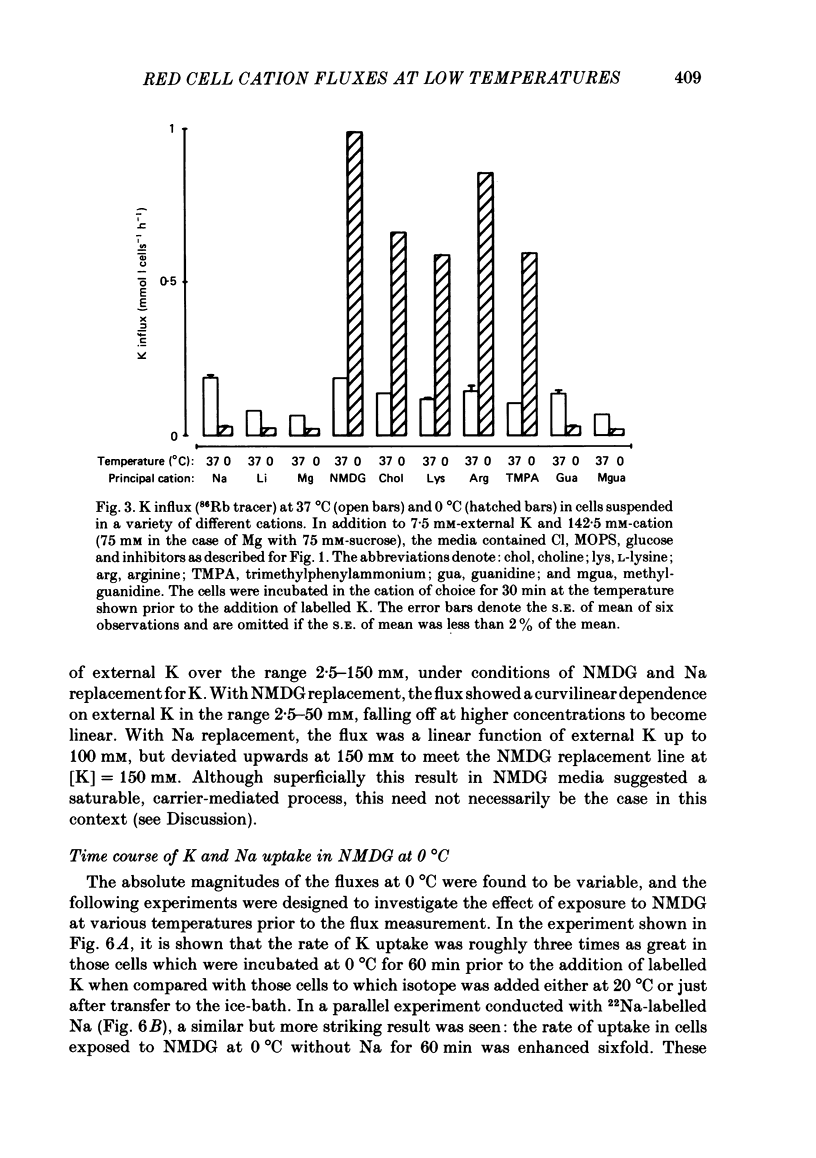
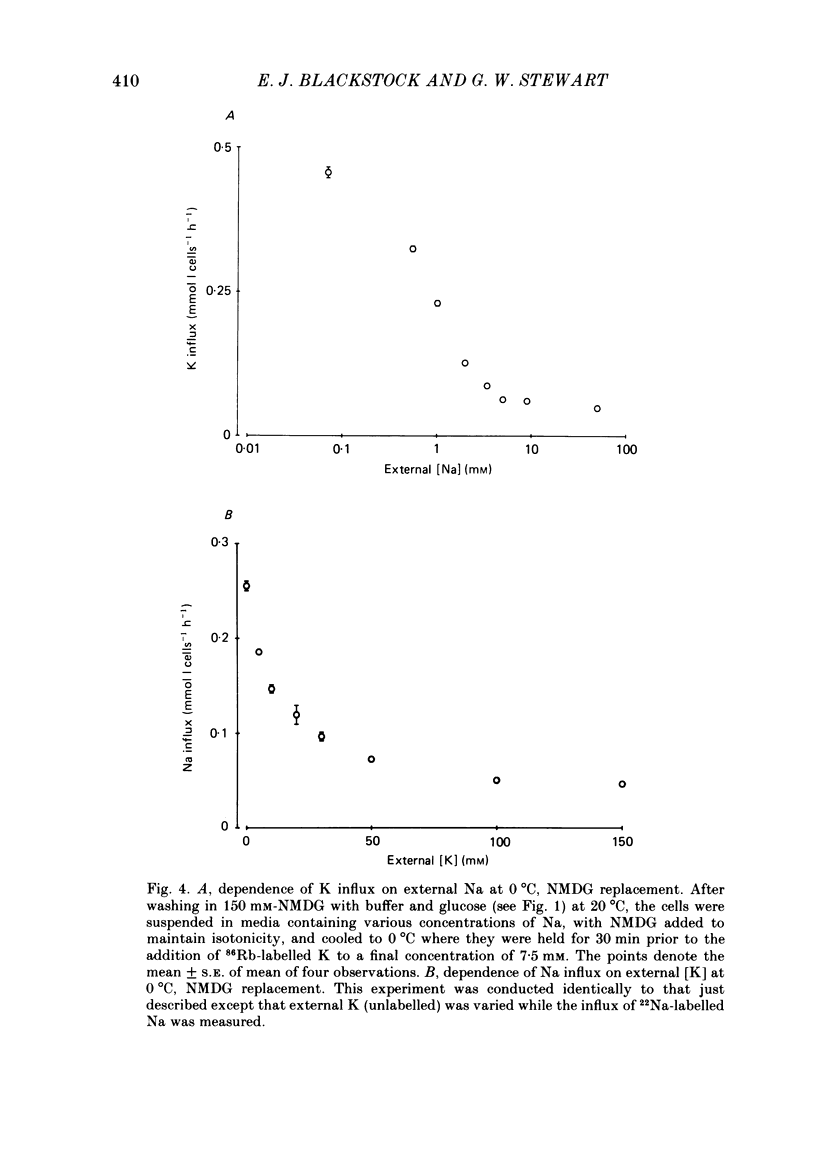
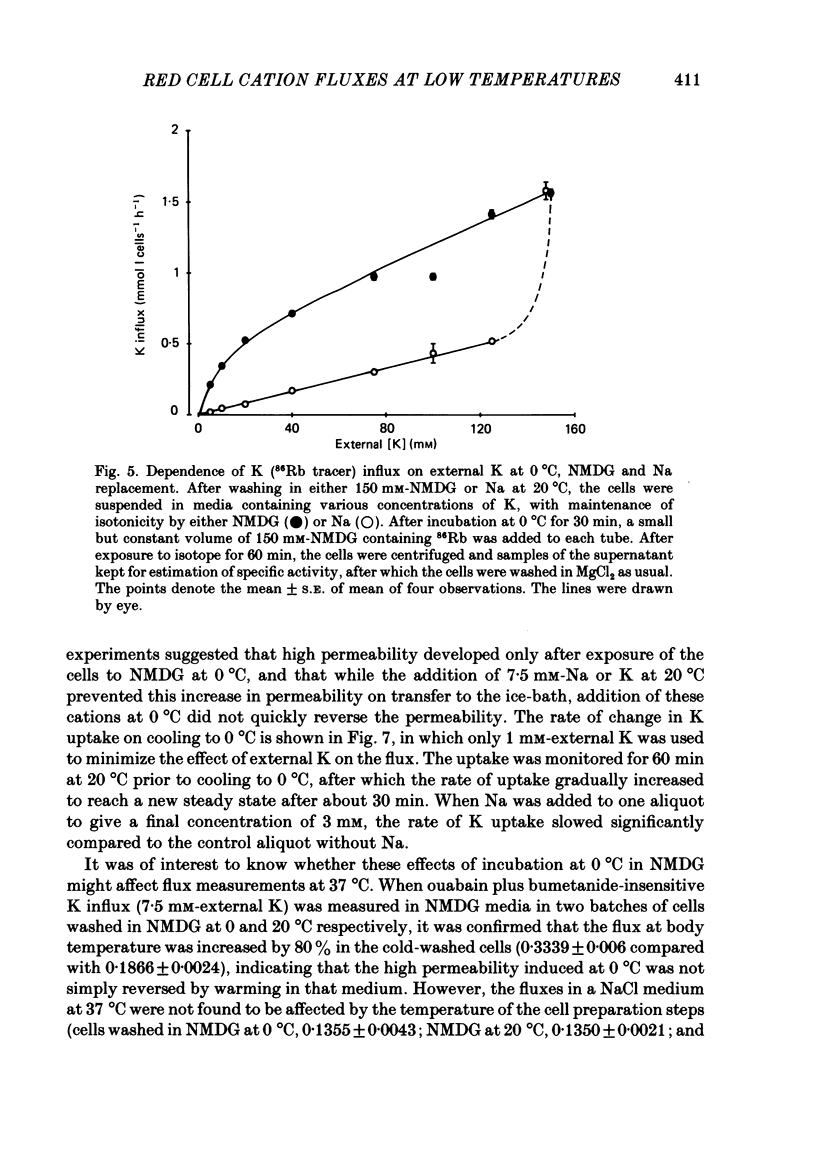
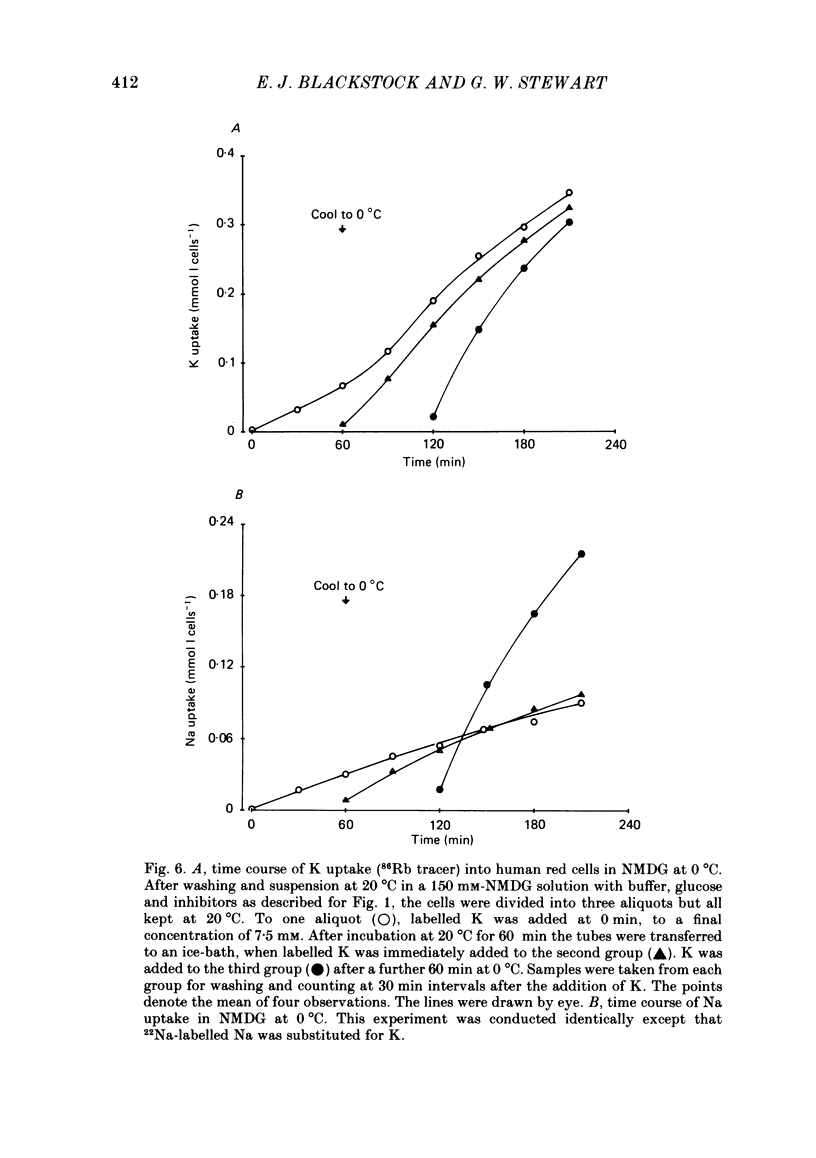
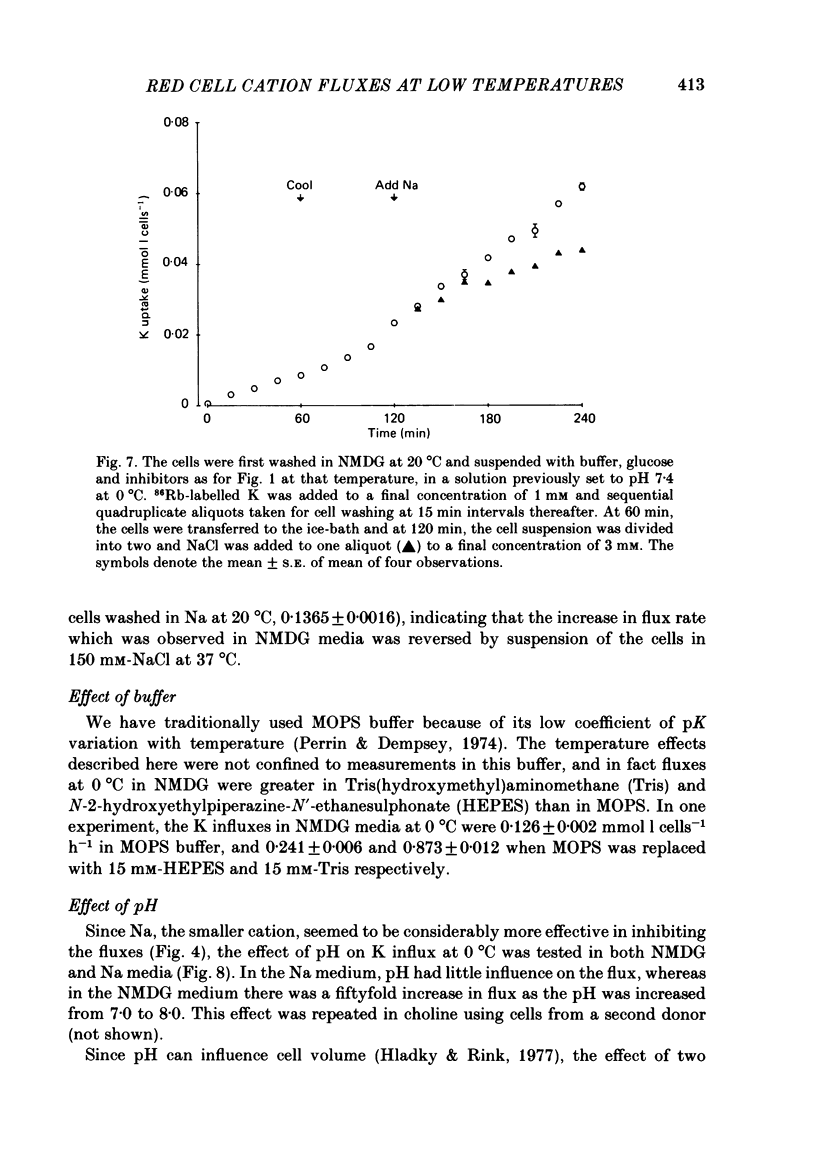
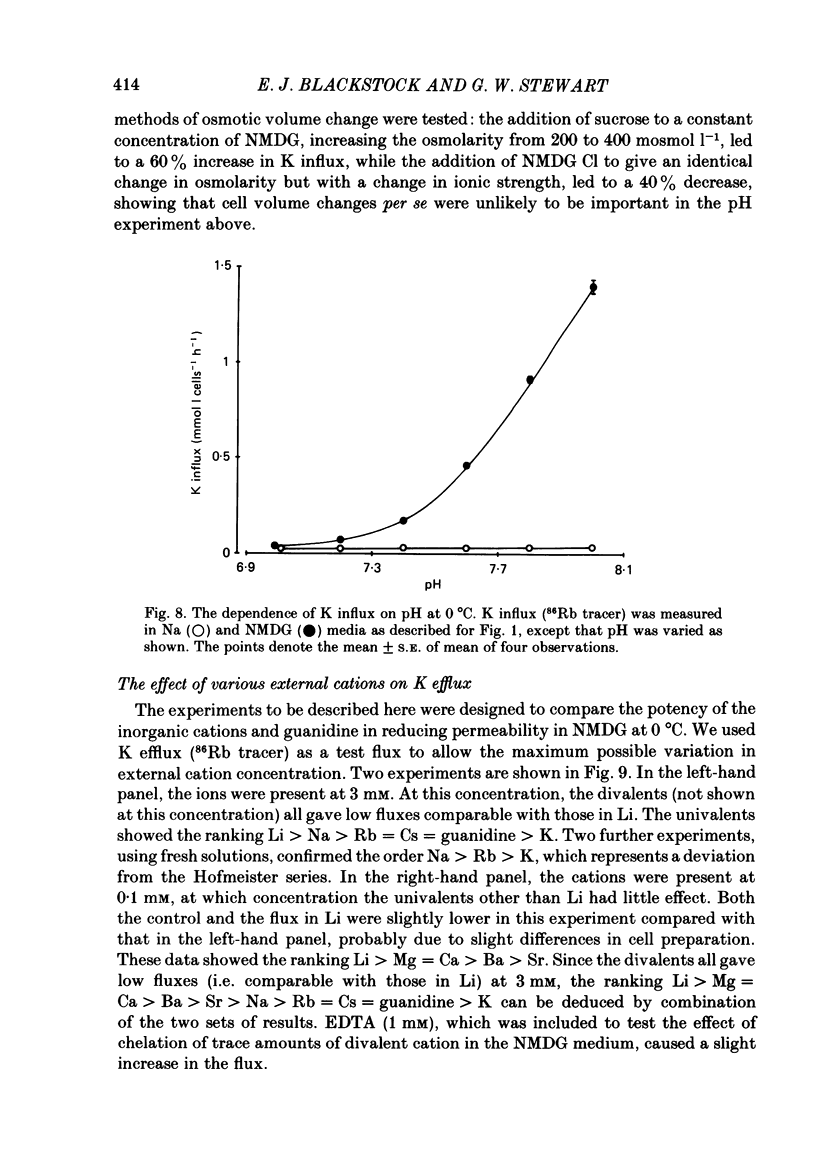
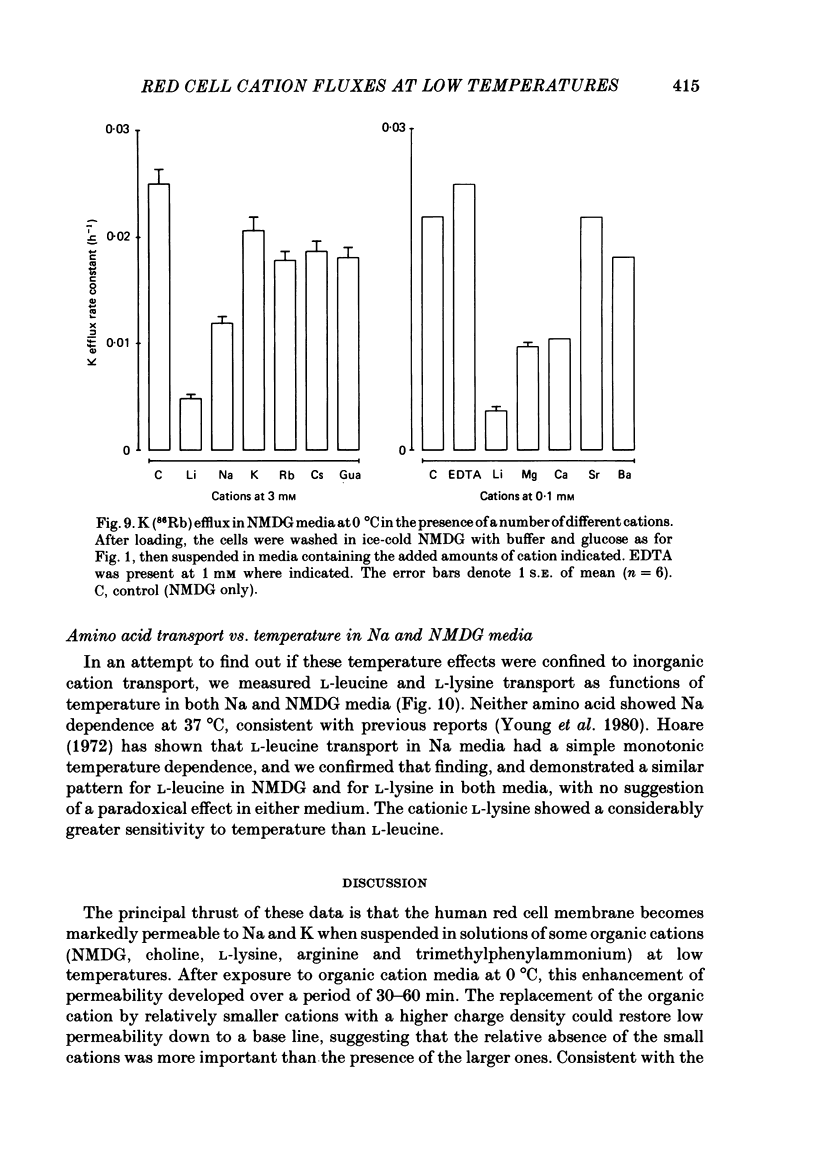
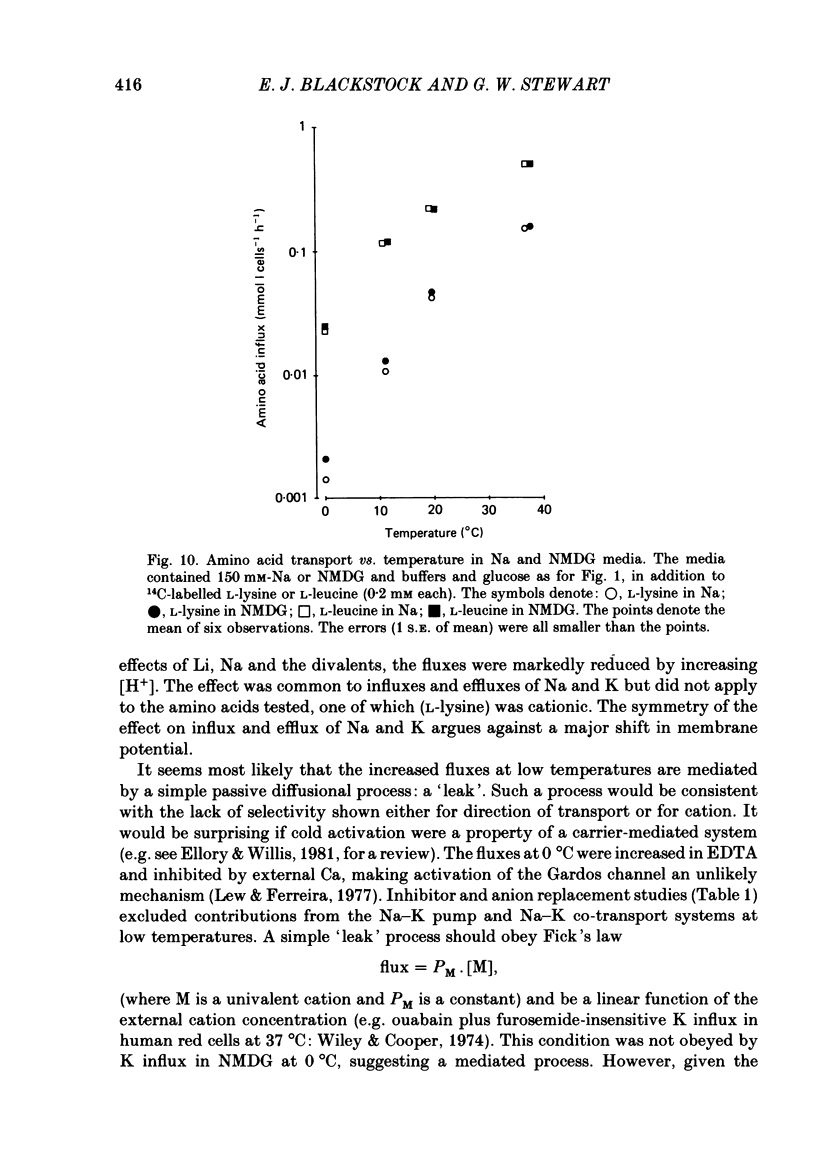
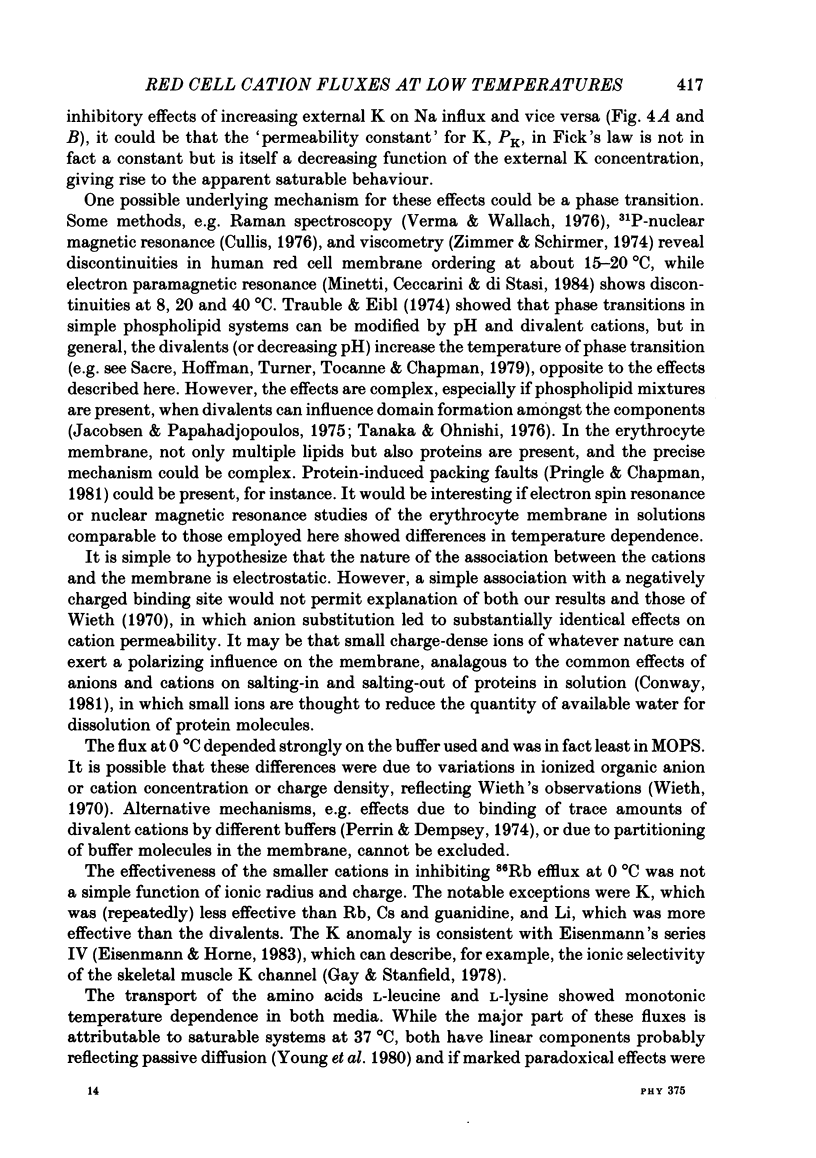
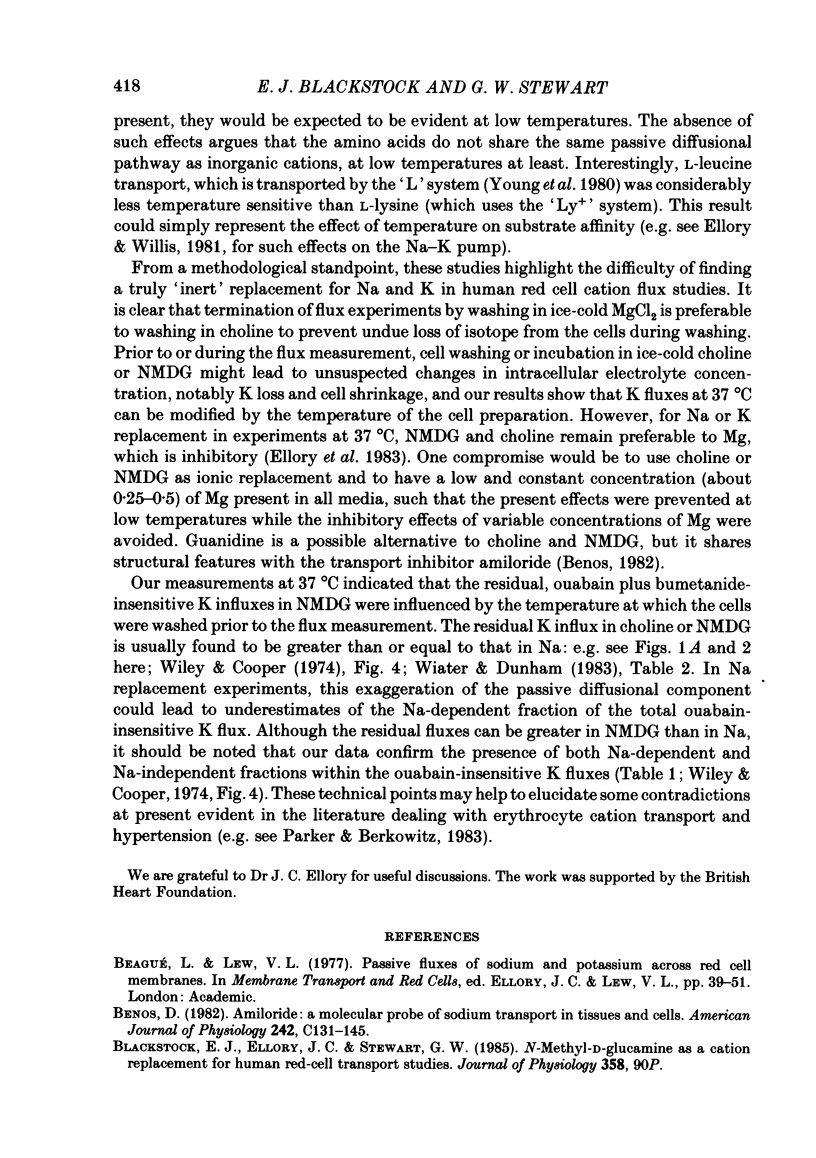
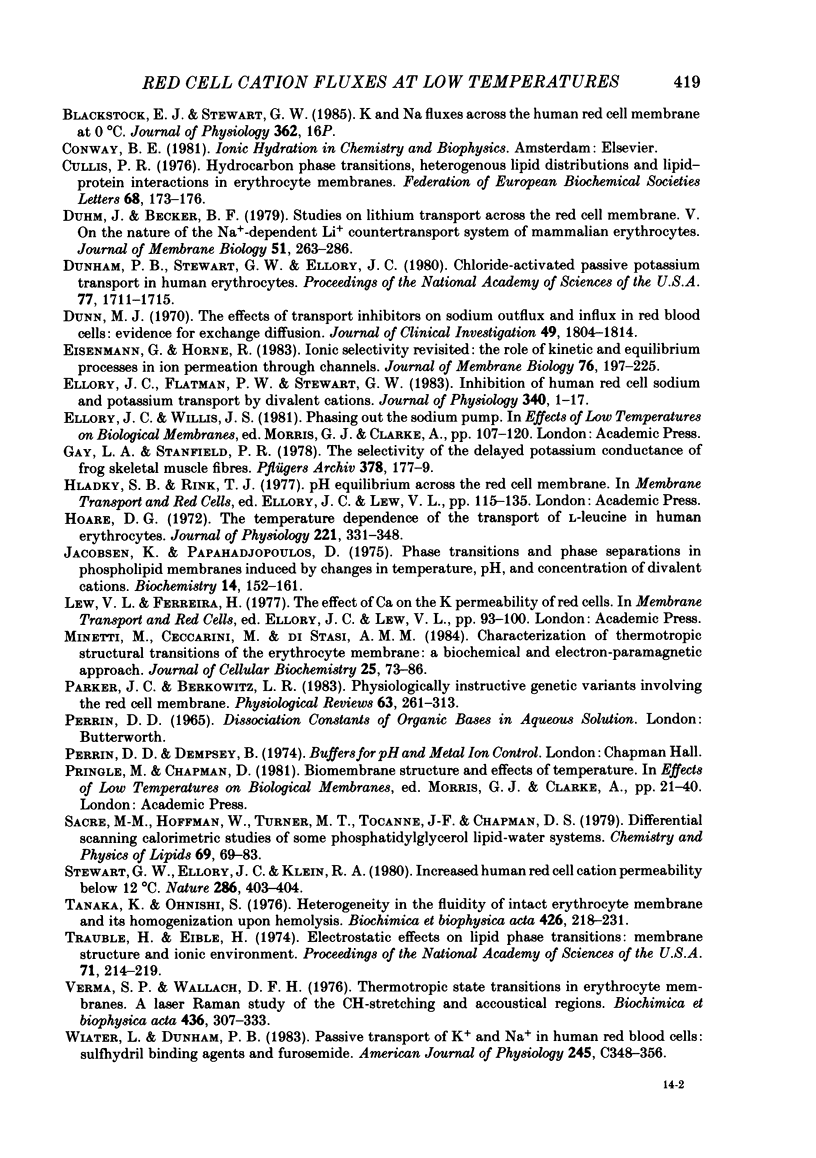
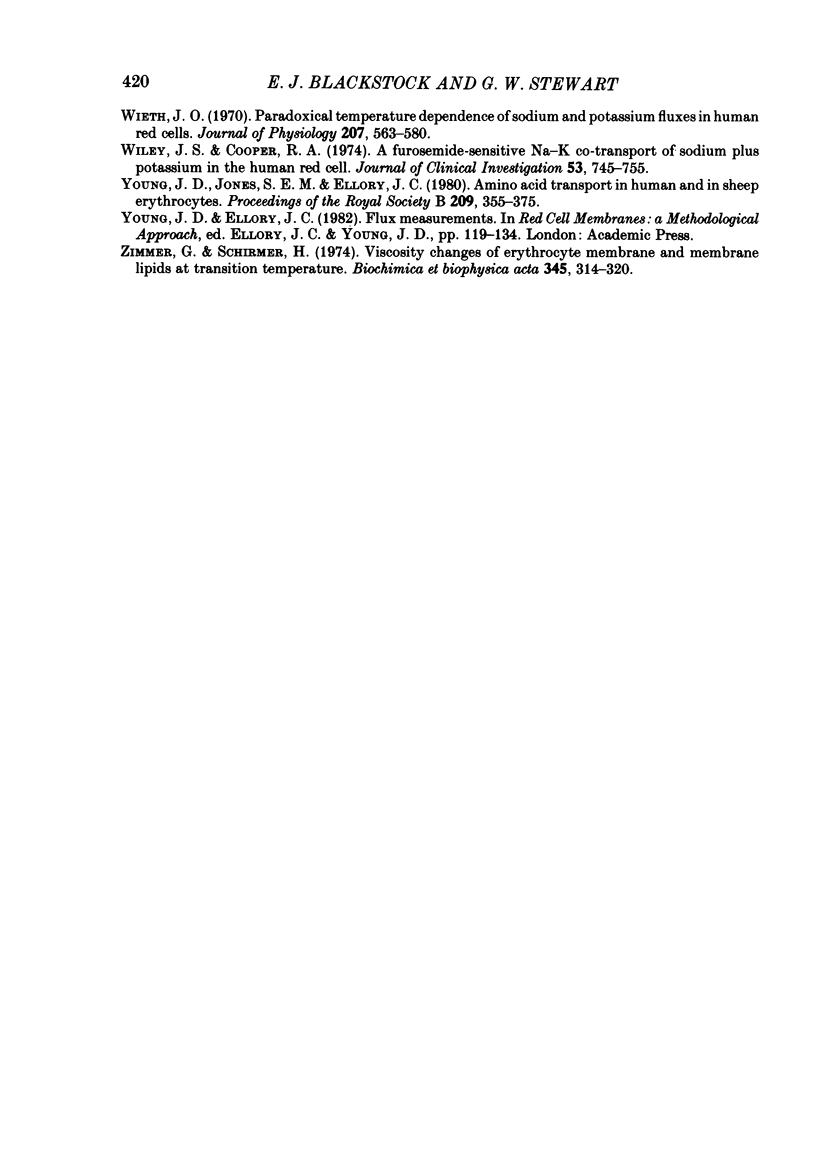
Selected References
These references are in PubMed. This may not be the complete list of references from this article.
- Benos D. J. Amiloride: a molecular probe of sodium transport in tissues and cells. Am J Physiol. 1982 Mar;242(3):C131–C145. doi: 10.1152/ajpcell.1982.242.3.C131. [DOI] [PubMed] [Google Scholar]
- Cullis P. R. Hydrocarbon phase transitions, heterogeneous lipid distributions and lipid-protein interactions in erythrocyte membranes. FEBS Lett. 1976 Oct 1;68(2):173–176. doi: 10.1016/0014-5793(76)80430-9. [DOI] [PubMed] [Google Scholar]
- Duhm J., Becker B. F. Studies on lithium transport across the red cell membrane. V. On the nature of the Na+-dependent Li+ countertransport system of mammalian erythrocytes. J Membr Biol. 1979 Dec 31;51(3-4):263–286. doi: 10.1007/BF01869087. [DOI] [PubMed] [Google Scholar]
- Dunham P. B., Stewart G. W., Ellory J. C. Chloride-activated passive potassium transport in human erythrocytes. Proc Natl Acad Sci U S A. 1980 Mar;77(3):1711–1715. doi: 10.1073/pnas.77.3.1711. [DOI] [PMC free article] [PubMed] [Google Scholar]
- Dunn M. J. The effects of transport inhibitors on sodium outflux and influx in red blood cells: evidence for exchange diffusion. J Clin Invest. 1970 Oct;49(10):1804–1814. doi: 10.1172/JCI106398. [DOI] [PMC free article] [PubMed] [Google Scholar]
- Eisenman G., Horn R. Ionic selectivity revisited: the role of kinetic and equilibrium processes in ion permeation through channels. J Membr Biol. 1983;76(3):197–225. doi: 10.1007/BF01870364. [DOI] [PubMed] [Google Scholar]
- Ellory J. C., Flatman P. W., Stewart G. W. Inhibition of human red cell sodium and potassium transport by divalent cations. J Physiol. 1983 Jul;340:1–17. doi: 10.1113/jphysiol.1983.sp014746. [DOI] [PMC free article] [PubMed] [Google Scholar]
- Gay L. A., Stanfield P. R. The selectivity of the delayed potassium conductance of frog skeletal muscle fibers. Pflugers Arch. 1978 Dec 28;378(2):177–179. doi: 10.1007/BF00584453. [DOI] [PubMed] [Google Scholar]
- Hoare D. G. The temperature dependence of the transport of L-leucine in human erythrocytes. J Physiol. 1972 Mar;221(2):331–348. doi: 10.1113/jphysiol.1972.sp009754. [DOI] [PMC free article] [PubMed] [Google Scholar]
- Jacobson K., Papahadjopoulos D. Phase transitions and phase separations in phospholipid membranes induced by changes in temperature, pH, and concentration of bivalent cations. Biochemistry. 1975 Jan 14;14(1):152–161. doi: 10.1021/bi00672a026. [DOI] [PubMed] [Google Scholar]
- Minetti M., Ceccarini M., Di Stasi A. M. Characterization of thermotropic structural transitions of the erythrocyte membrane: a biochemical and electron-paramagnetic resonance approach. J Cell Biochem. 1984;25(2):73–86. doi: 10.1002/jcb.240250203. [DOI] [PubMed] [Google Scholar]
- Parker J. C., Berkowitz L. R. Physiologically instructive genetic variants involving the human red cell membrane. Physiol Rev. 1983 Jan;63(1):261–313. doi: 10.1152/physrev.1983.63.1.261. [DOI] [PubMed] [Google Scholar]
- Stewart G. W., Ellory J. C., Klein R. A. Increased human red cell cation passive permeability below 12 degrees C. Nature. 1980 Jul 24;286(5771):403–404. doi: 10.1038/286403a0. [DOI] [PubMed] [Google Scholar]
- Tanaka K. I., Ohnishi S. Heterogeneity in the fluidity of intact erythrocyte membrane and its homogenization upon hemolysis. Biochim Biophys Acta. 1976 Mar 5;426(2):218–231. doi: 10.1016/0005-2736(76)90333-3. [DOI] [PubMed] [Google Scholar]
- Träuble H., Eibl H. Electrostatic effects on lipid phase transitions: membrane structure and ionic environment. Proc Natl Acad Sci U S A. 1974 Jan;71(1):214–219. doi: 10.1073/pnas.71.1.214. [DOI] [PMC free article] [PubMed] [Google Scholar]
- Verma S. P., Wallach D. F. Multiple thermotropic state transitions in erythrocyte membranes. A laser-Raman study of the CH-stretching and acoustical regions. Biochim Biophys Acta. 1976 Jun 17;436(2):307–318. doi: 10.1016/0005-2736(76)90196-6. [DOI] [PubMed] [Google Scholar]
- Wiater L. A., Dunham P. B. Passive transport of K+ and Na+ in human red blood cells: sulfhydryl binding agents and furosemide. Am J Physiol. 1983 Nov;245(5 Pt 1):C348–C356. doi: 10.1152/ajpcell.1983.245.5.C348. [DOI] [PubMed] [Google Scholar]
- Wieth J. O. Paradoxical temperature dependence of sodium and potassium fluxes in human red cells. J Physiol. 1970 May;207(3):563–580. doi: 10.1113/jphysiol.1970.sp009081. [DOI] [PMC free article] [PubMed] [Google Scholar]
- Wiley J. S., Cooper R. A. A furosemide-sensitive cotransport of sodium plus potassium in the human red cell. J Clin Invest. 1974 Mar;53(3):745–755. doi: 10.1172/JCI107613. [DOI] [PMC free article] [PubMed] [Google Scholar]
- Young J. D., Jones S. E., Ellory J. C. Amino acid transport in human and in sheep erythrocytes. Proc R Soc Lond B Biol Sci. 1980 Sep 26;209(1176):355–375. doi: 10.1098/rspb.1980.0100. [DOI] [PubMed] [Google Scholar]