Abstract
31P nuclear magnetic resonance was used to measure the relative concentrations of phosphorus-containing metabolites in Langendorff-perfused ferret hearts. Intracellular concentrations of inorganic phosphate ([Pi]i), phosphocreatine [( PCr]i), ATP ([ATP]i) and [H+] (pHi) were determined. Exposure of the heart to strophanthidin (10-40 microM) produced an increase in developed pressure over 5-10 min. In the presence of strophanthidin, [ATP]i was unchanged, [PCr]i showed a small fall, [Pi]i showed a small rise and there was a small acidosis. Exposure of the heart to a solution in which all the Na had been replaced by K (0 Na(K) solution) produced an increase of resting pressure which then decayed. During this contracture [PCr]i fell transiently and [Pi]i rose transiently with approximately the same time course as the contracture. However, [ATP]i remained constant throughout. The exposure to the 0 Na(K) solution also produced an intracellular acidosis. The changes in [PCr]i and [Pi]i and the intracellular acidosis were all increased during perfusion with 0 Na(K) if the heart had previously been exposed to strophanthidin. The efflux of lactate from the heart was increased during the exposure to the 0 Na(K) solution. The magnitude of this increase was enhanced by prior exposure to strophanthidin. The increase of intracellular lactate (calculated from this efflux) was sufficient to account for the observed intracellular acidification. An increase of lactate efflux could also be measured when an isolated papillary muscle was exposed to the 0 Na(K) solution. The intracellular acidification produced by Na removal was substantially decreased after prevention of glycolysis either by substrate depletion or by the application of iodoacetate. Elevation of the extracellular calcium concentration ([Ca2+]o) produced a large increase of developed pressure which was accompanied by a small transient increase of [Pi]i, a decrease of [PCr]i and a small intracellular acidosis. There was also an increase of lactate efflux. After exposure to strophanthidin the same increase of [Ca2+]o decreased developed pressure. The associated rise in [Pi]i and fall in [PCr]i were increased but there was no significant acidosis under these conditions. In addition to other explanations (Allen, Eisner, Pirolo & Smith, 1985a), it is likely that the fall of force is partly accounted for by the rise of [Pi]i.
Full text
PDF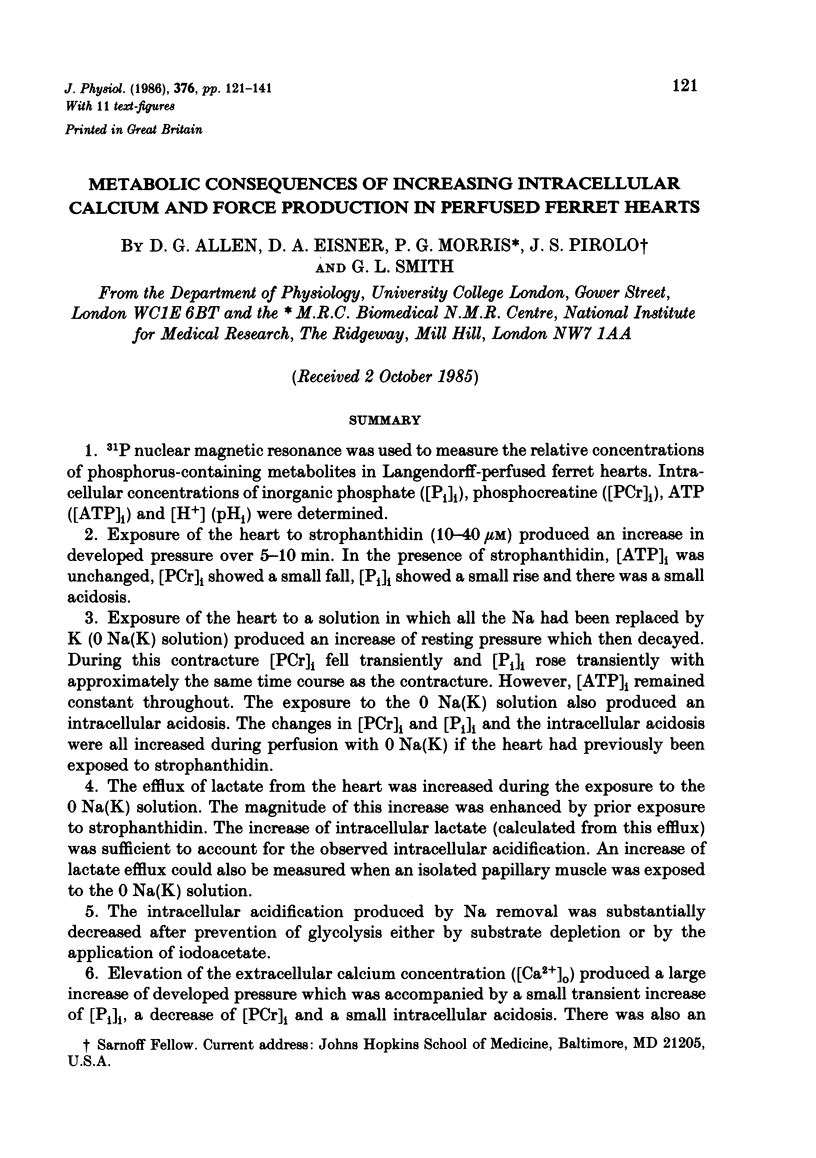
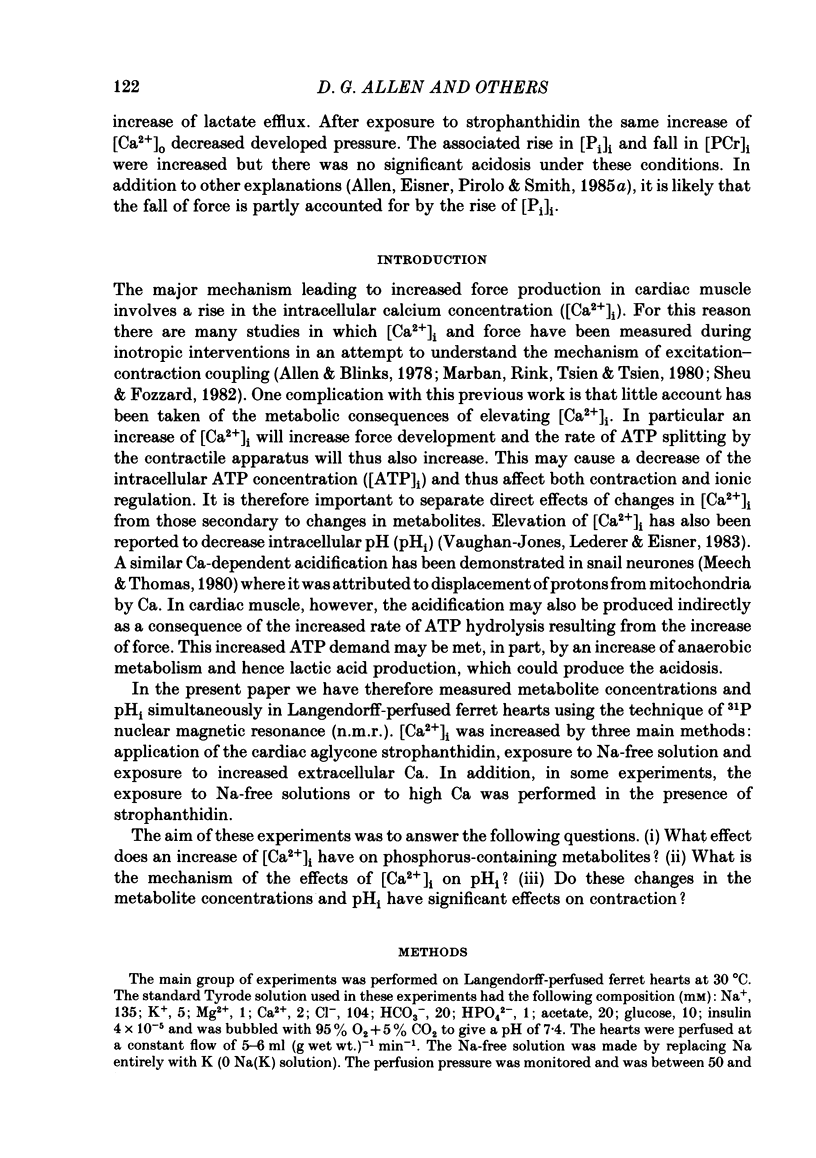
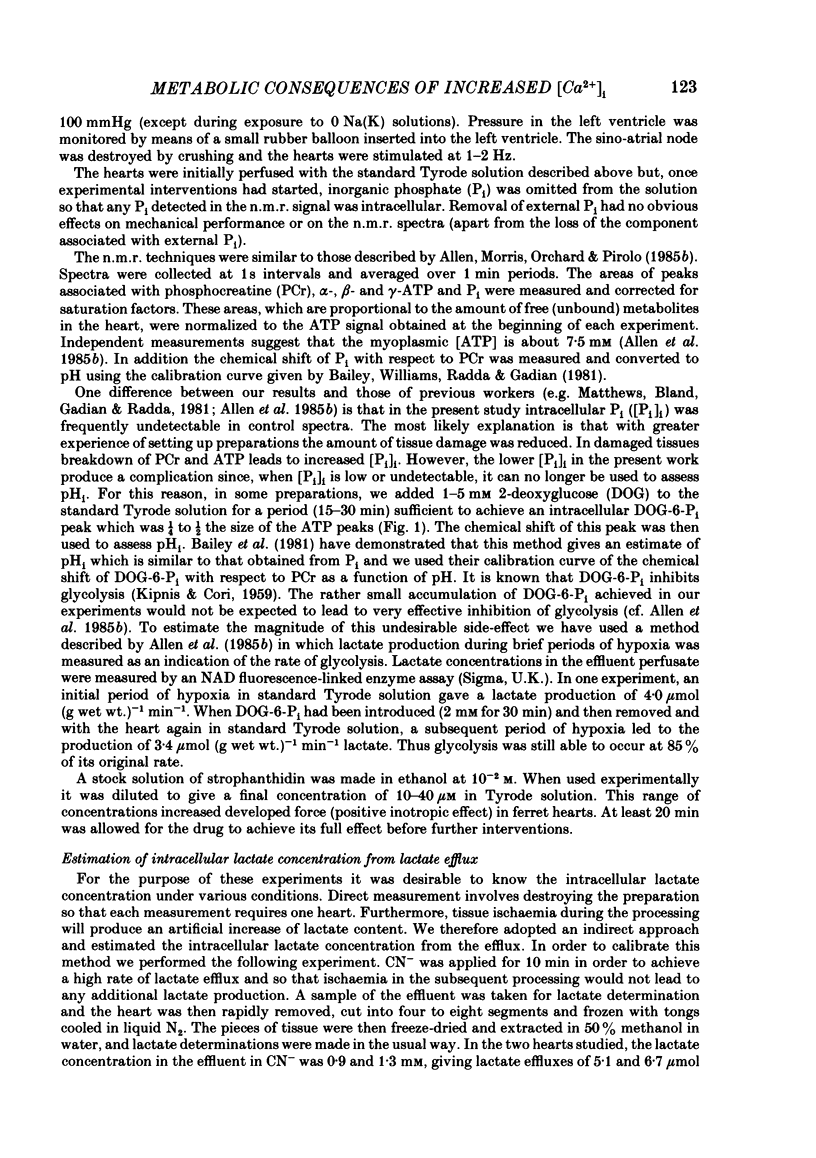
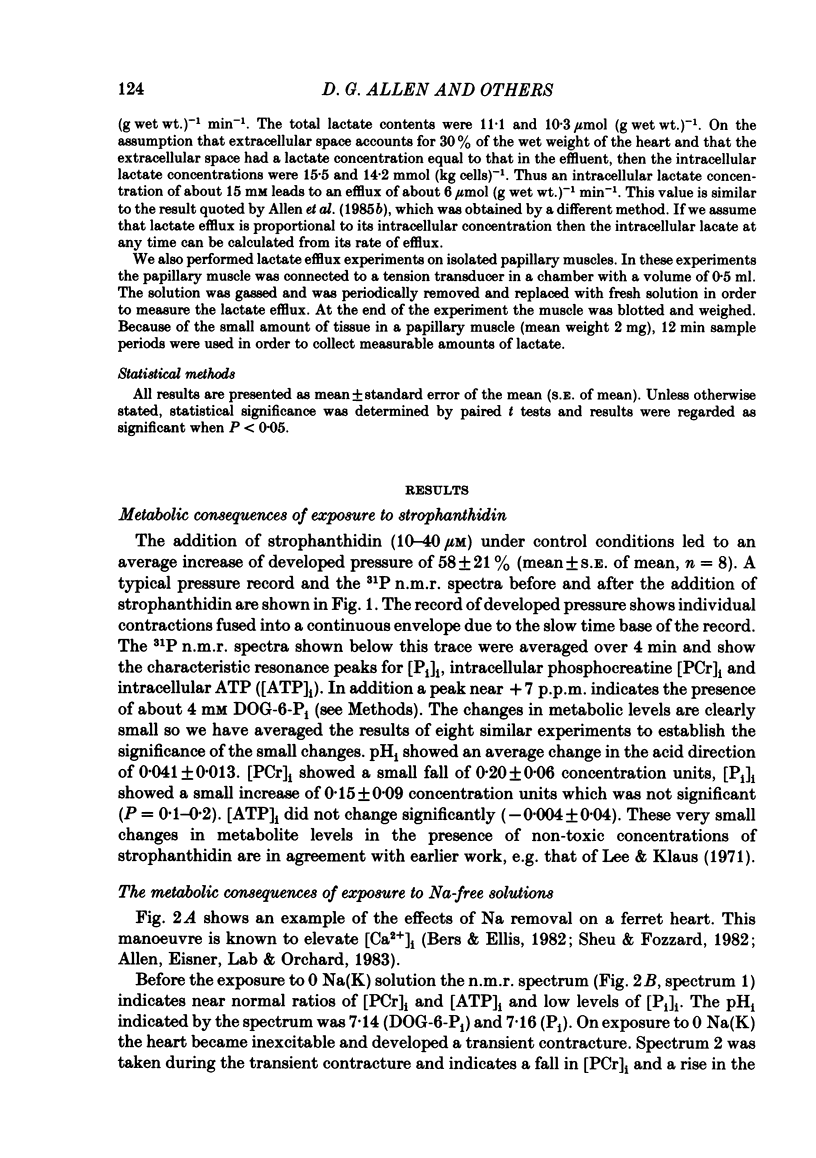
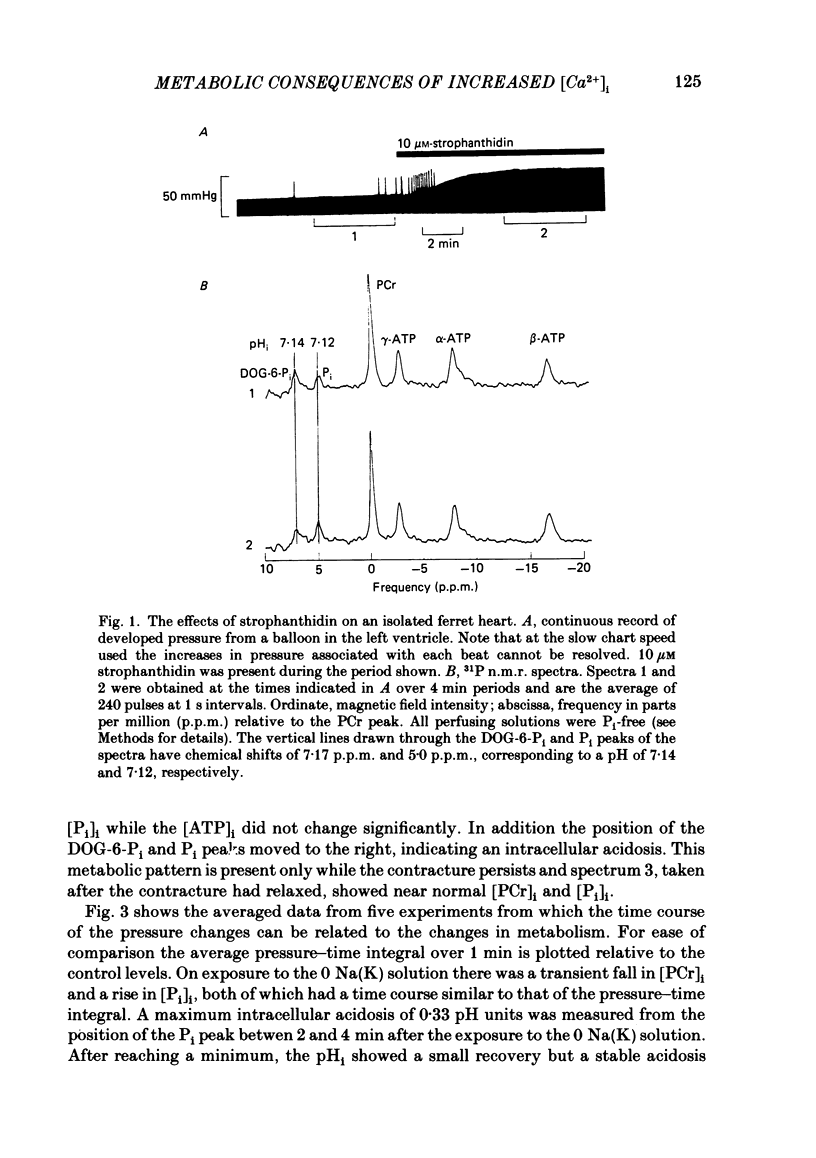
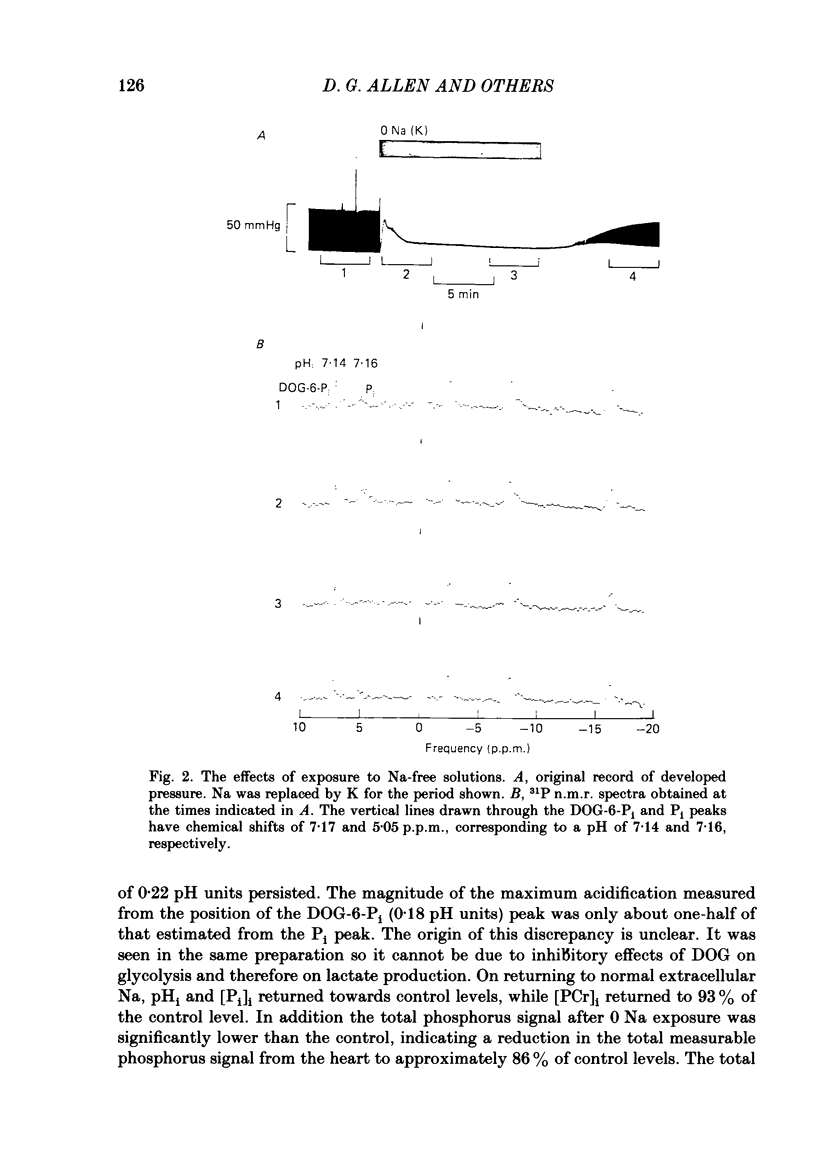
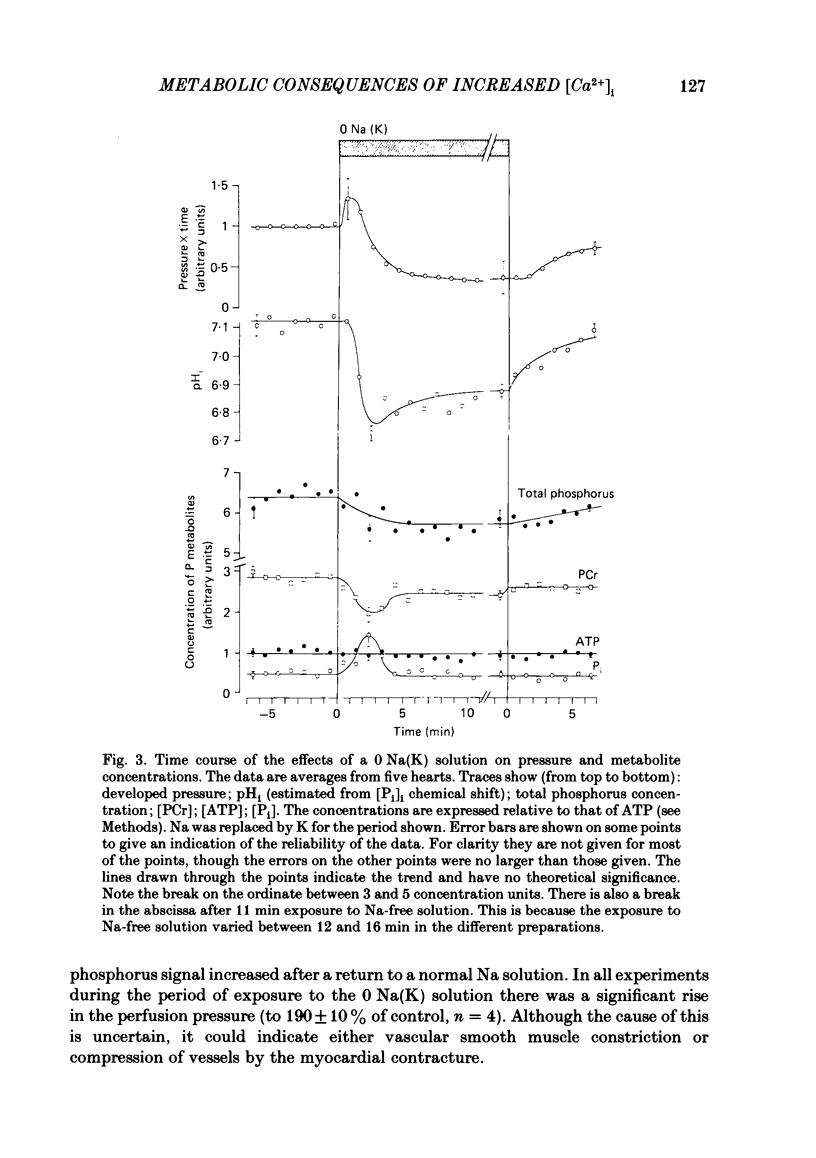
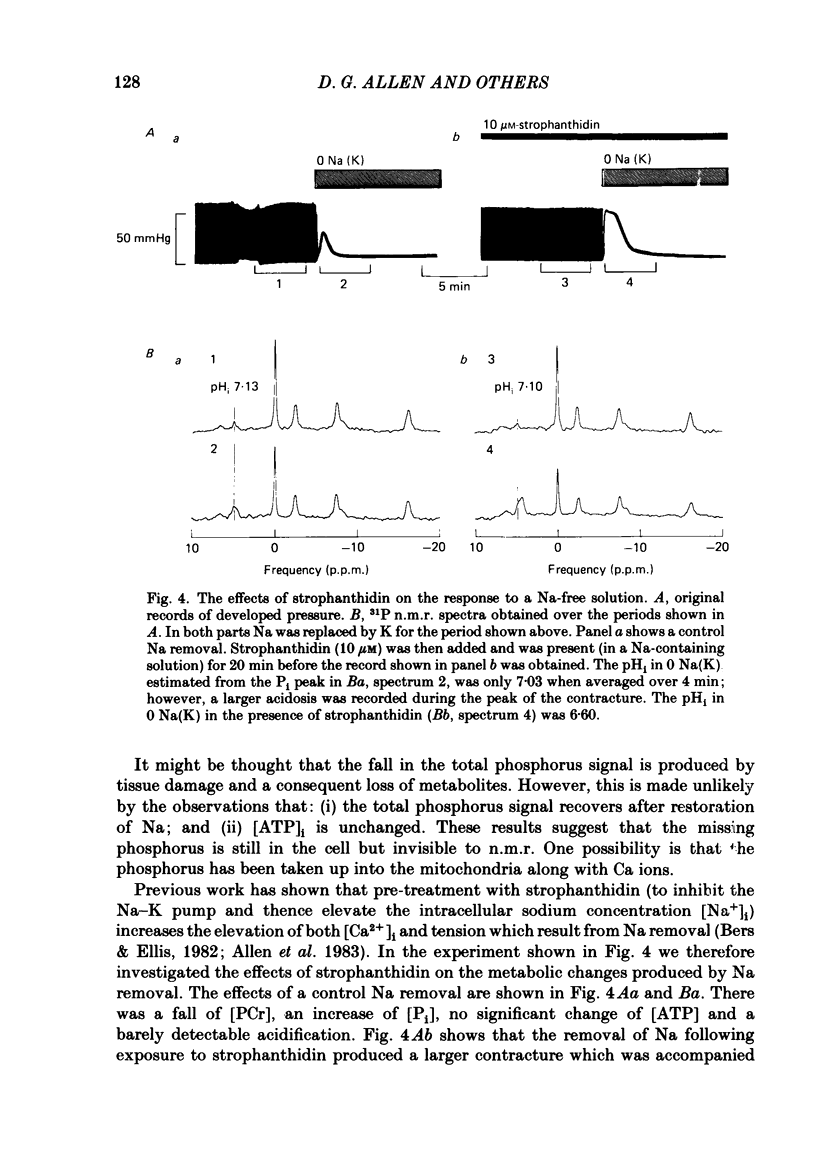
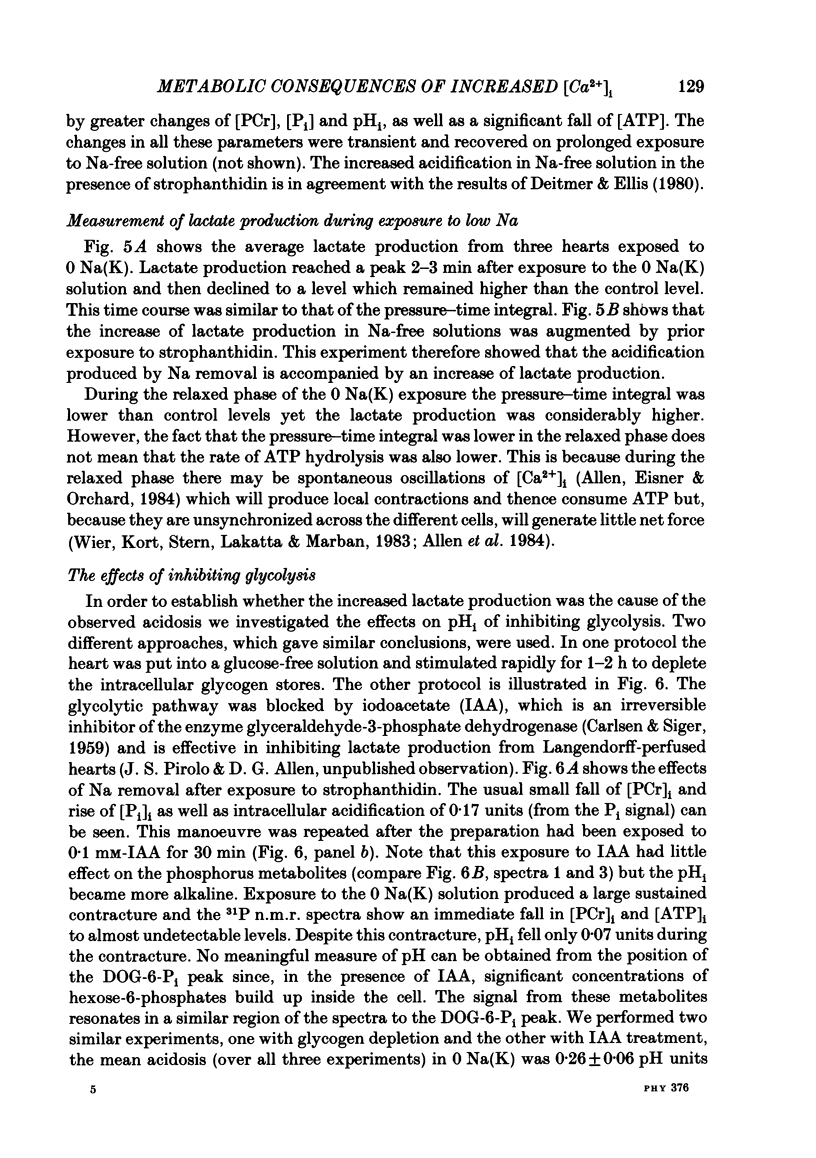
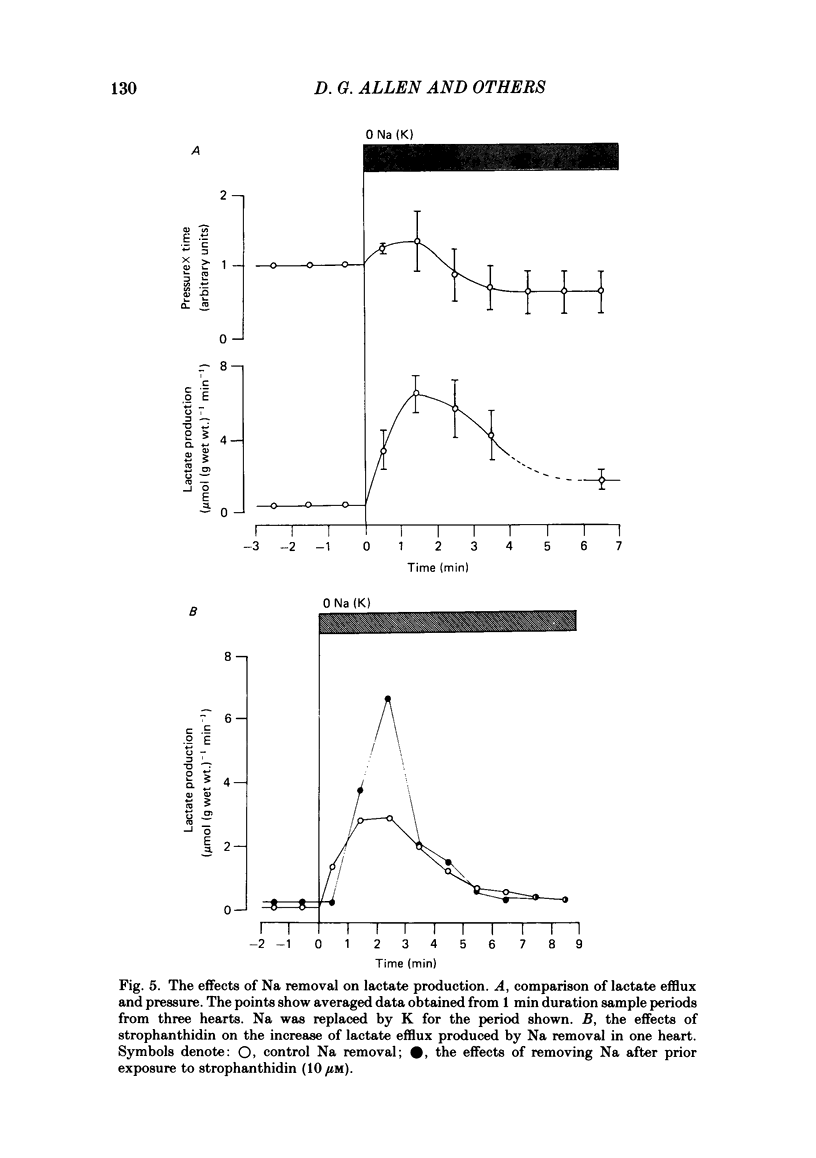
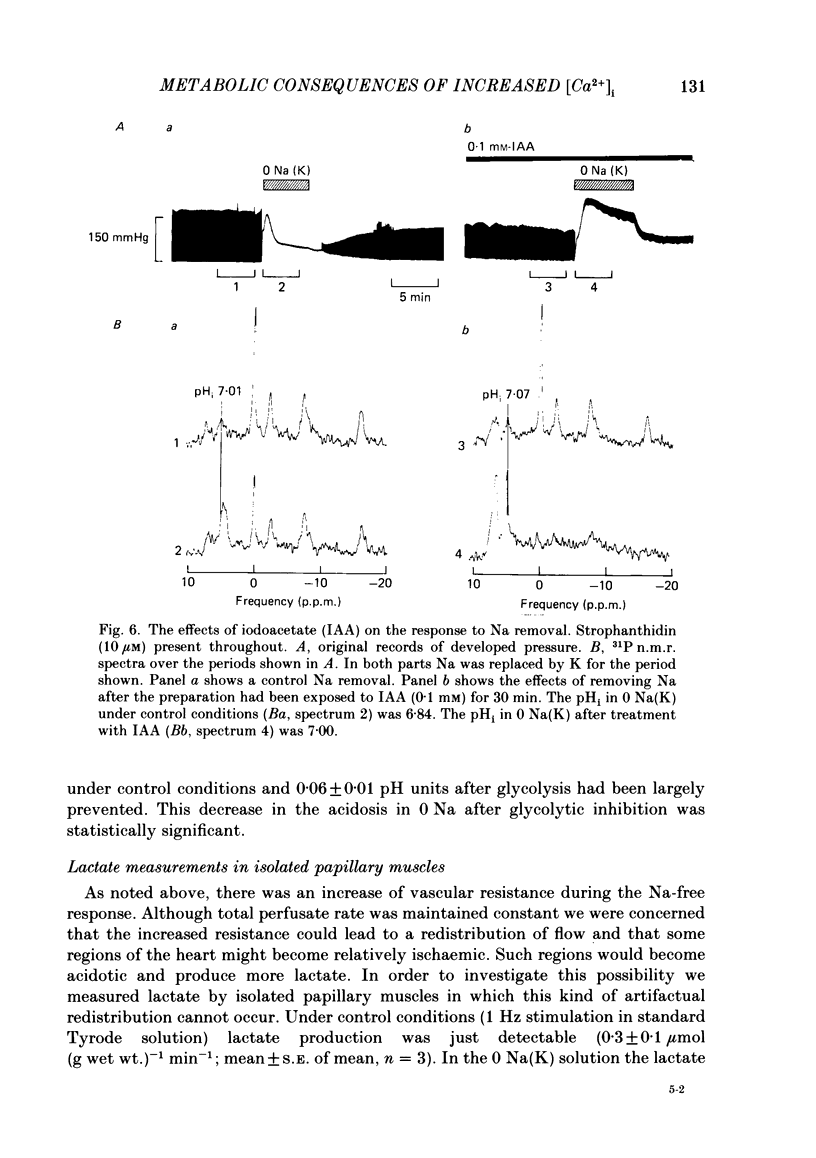
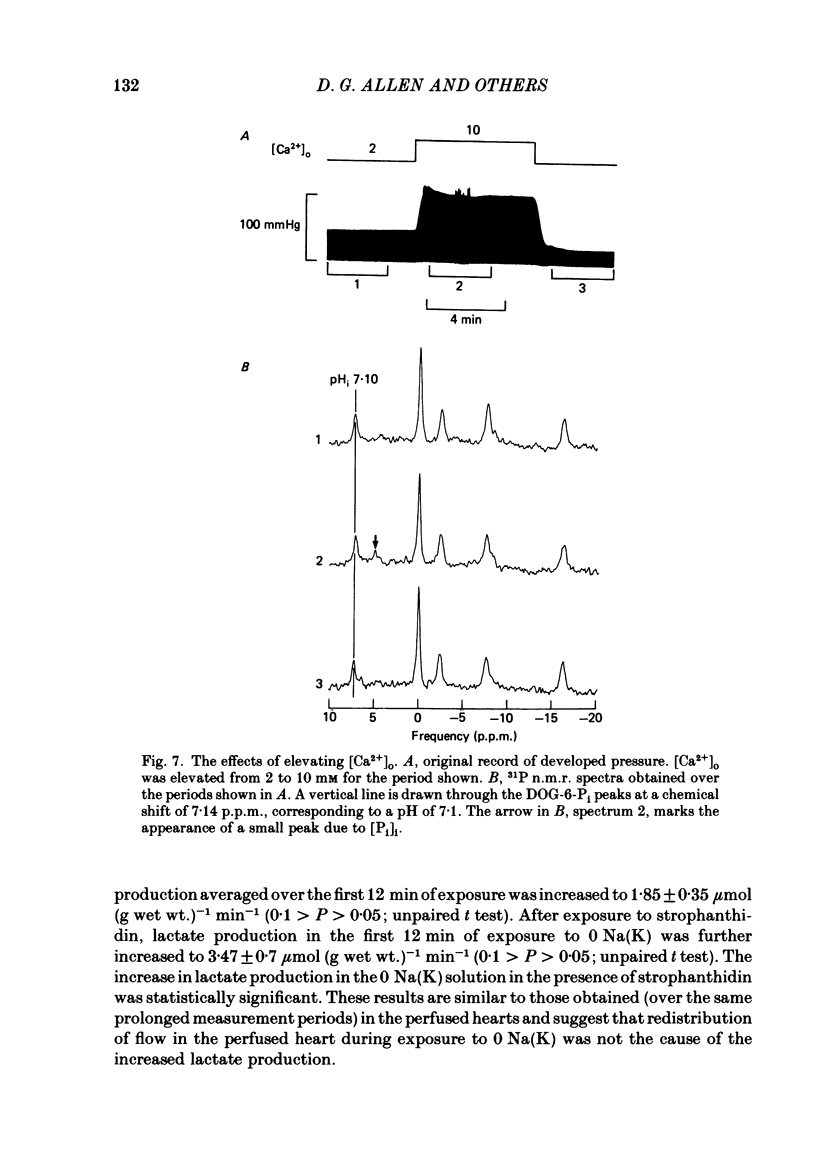
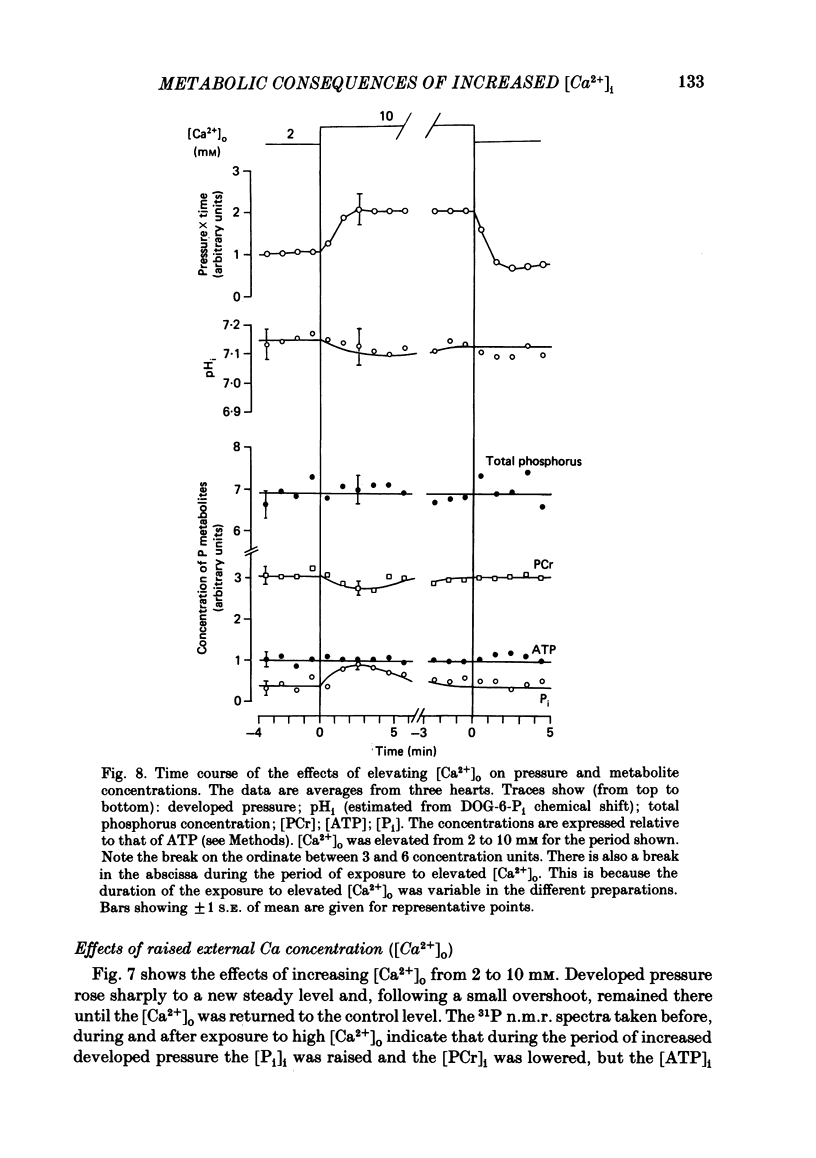
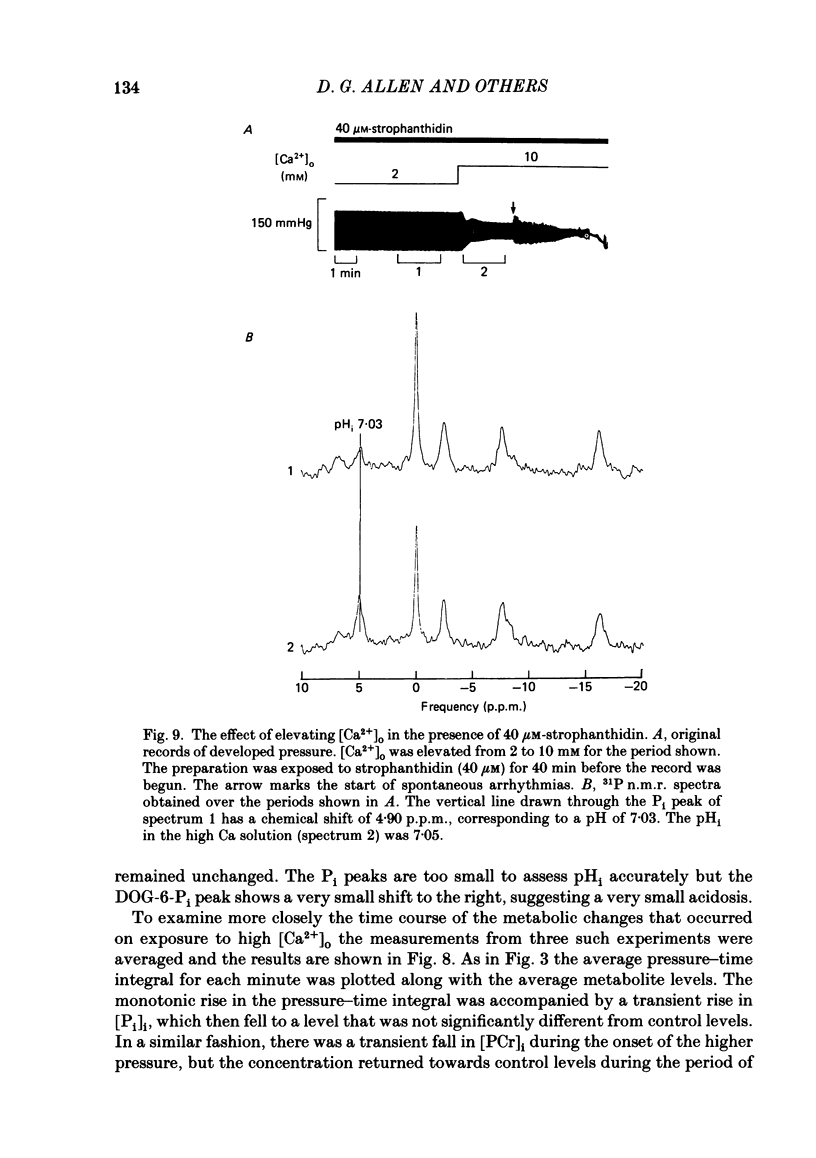
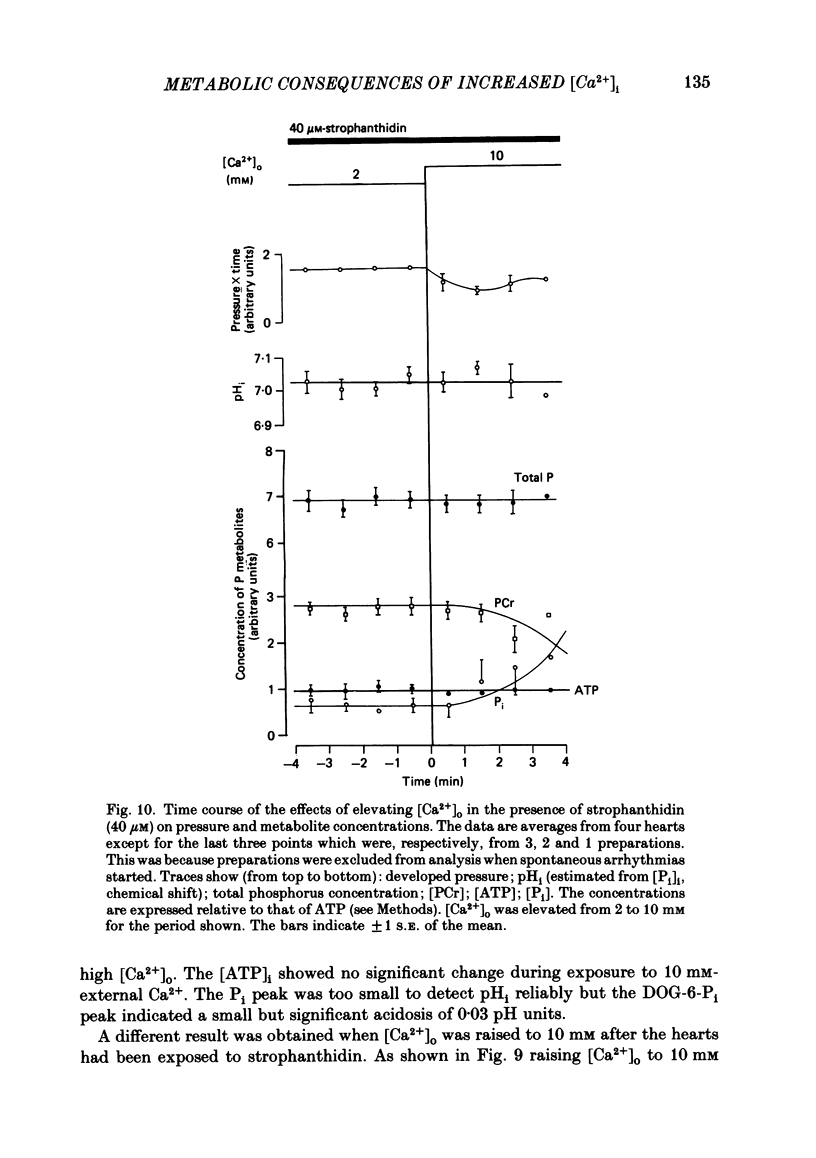
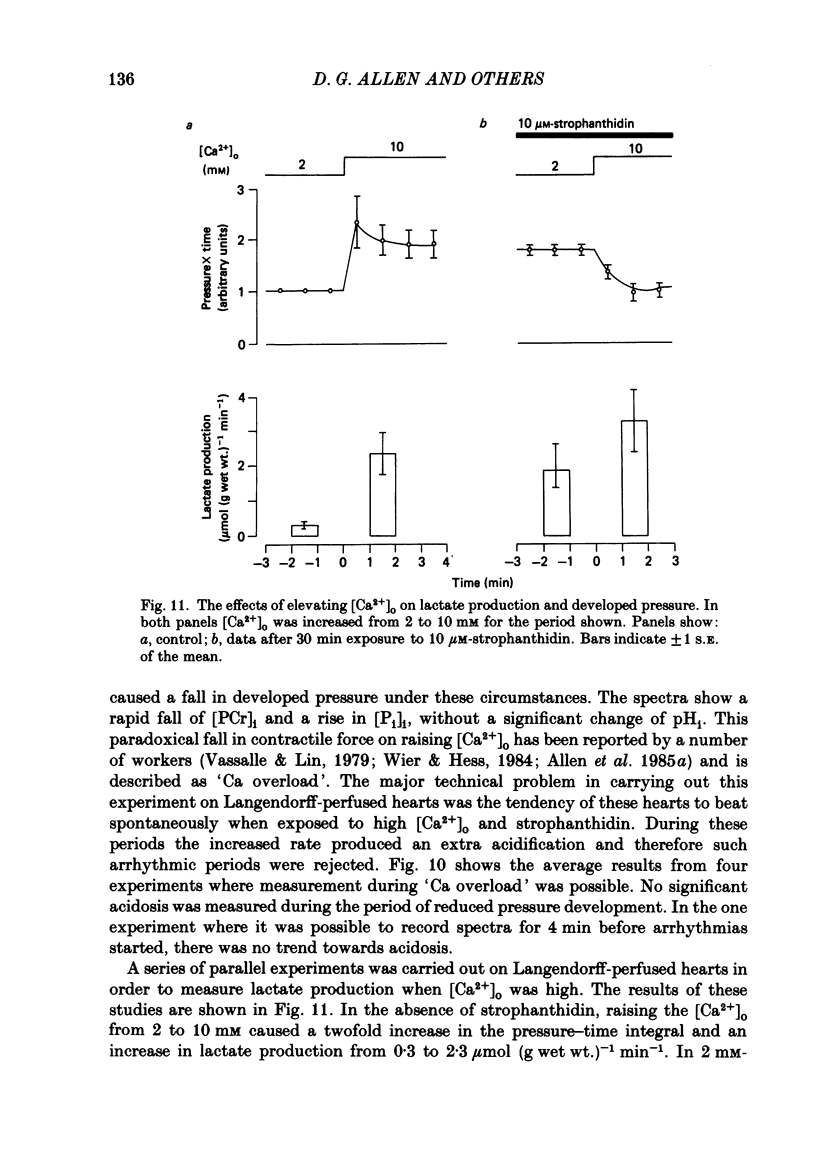
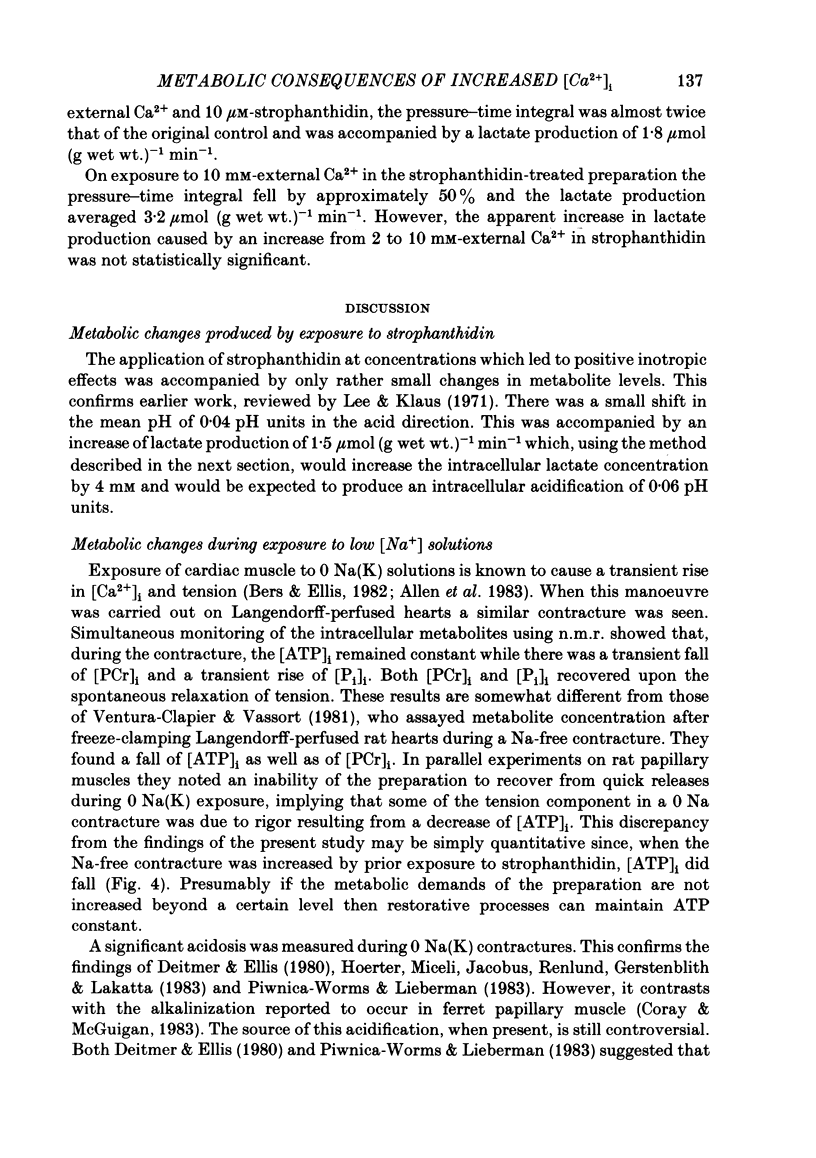
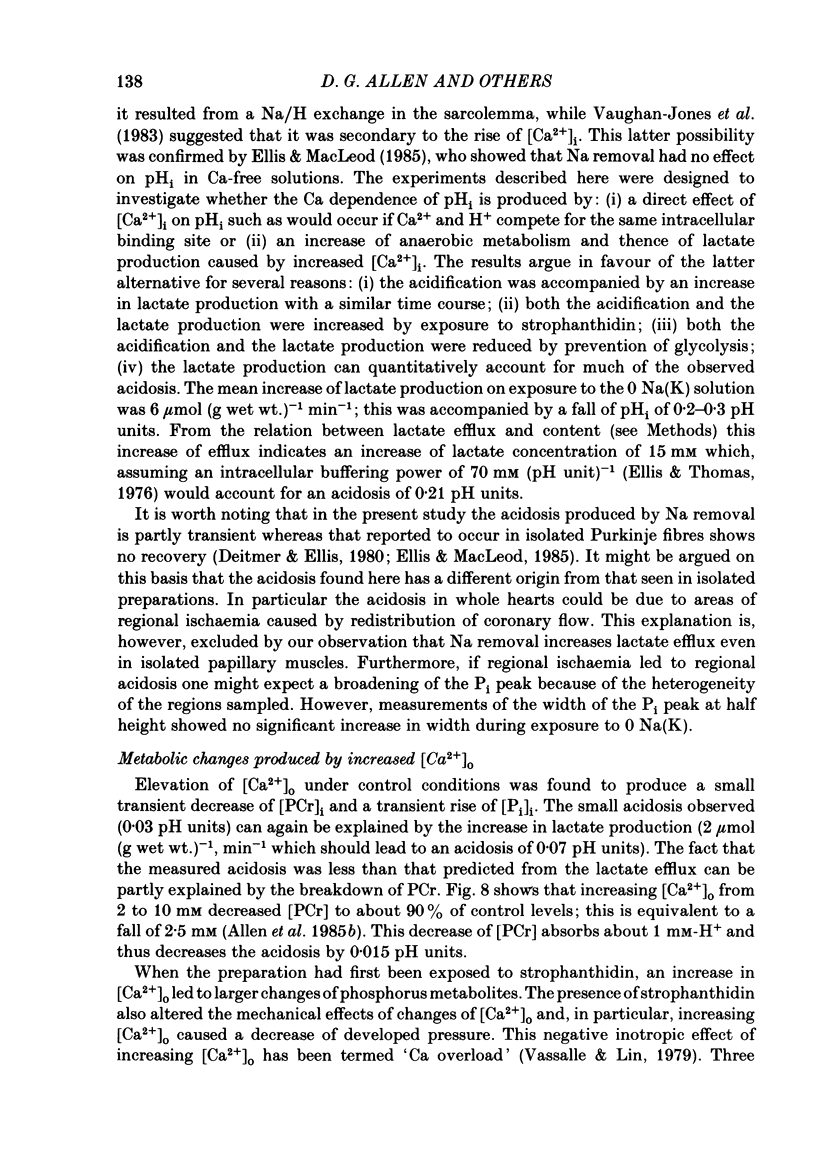
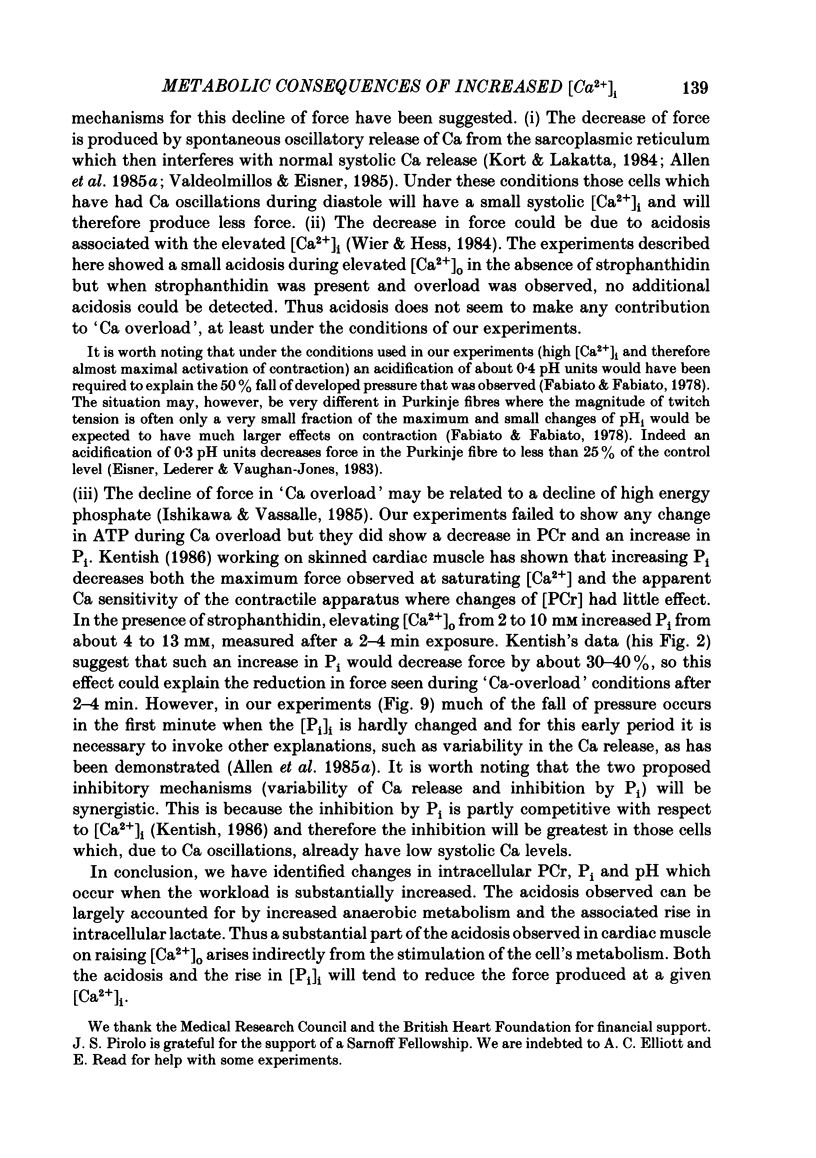
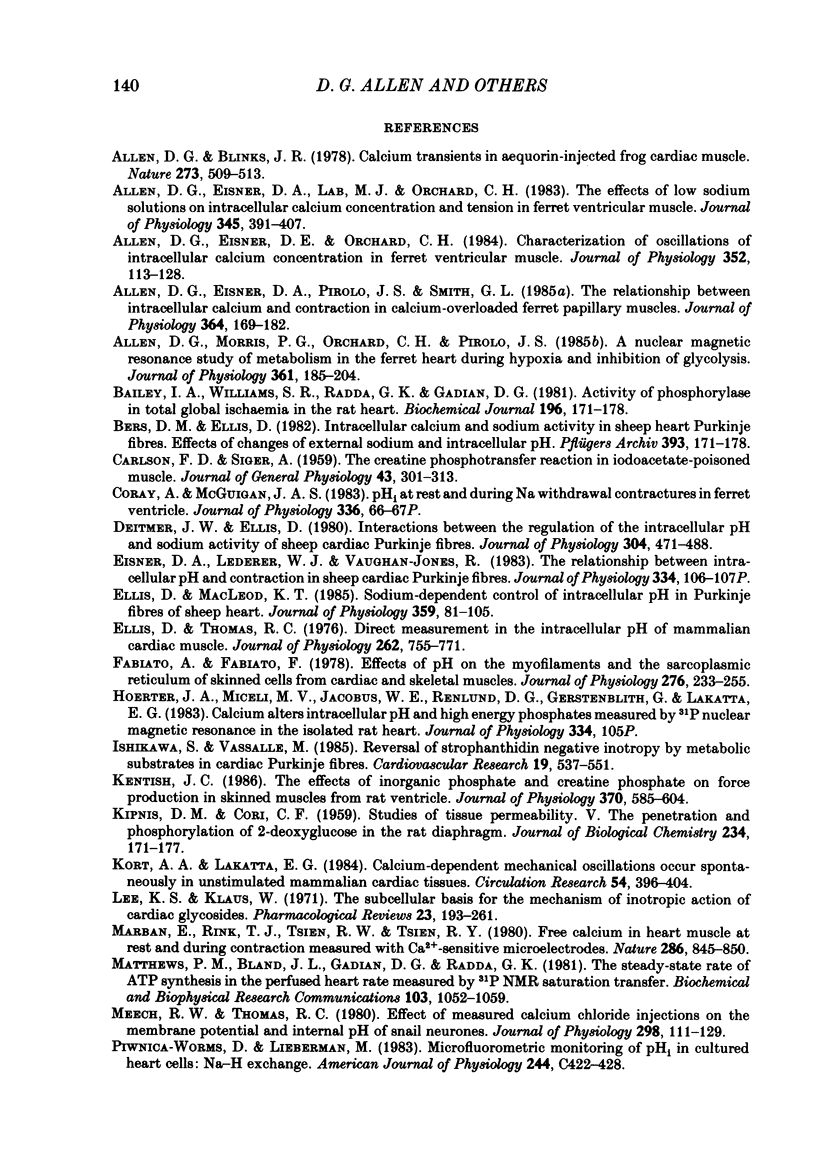
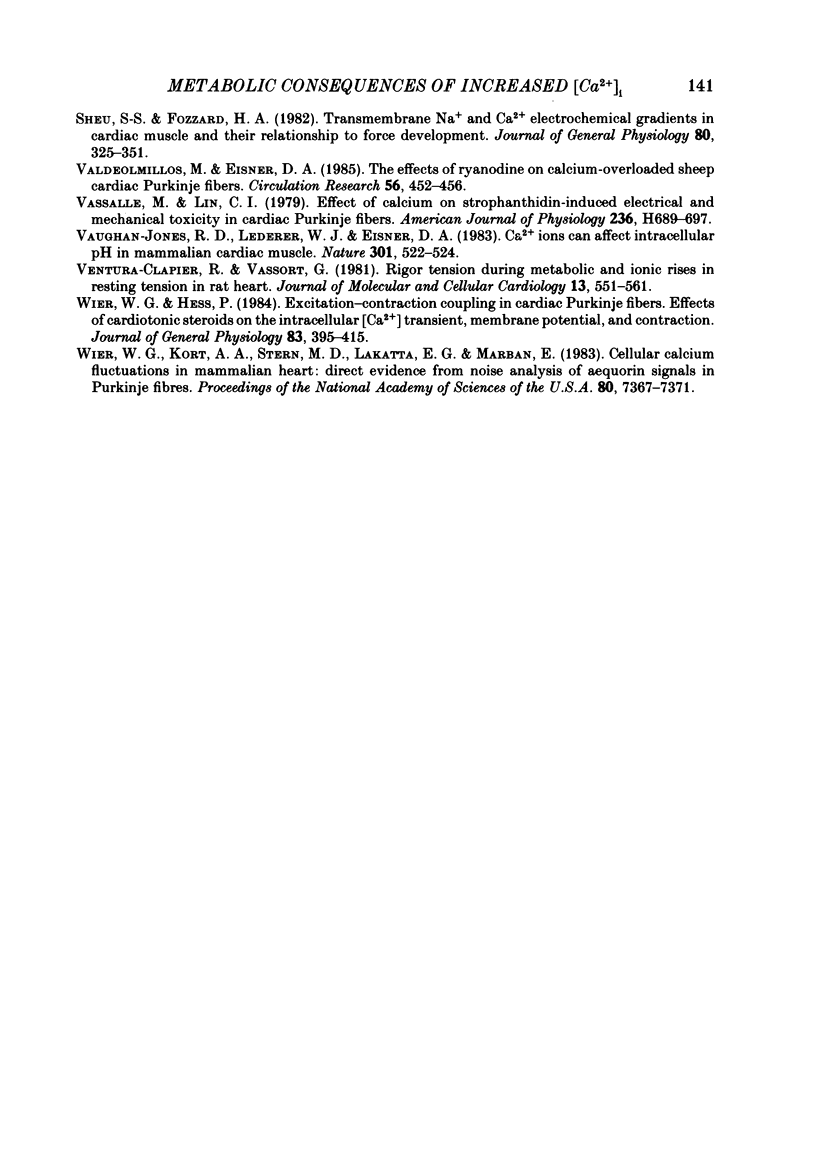
Images in this article
Selected References
These references are in PubMed. This may not be the complete list of references from this article.
- Allen D. G., Blinks J. R. Calcium transients in aequorin-injected frog cardiac muscle. Nature. 1978 Jun 15;273(5663):509–513. doi: 10.1038/273509a0. [DOI] [PubMed] [Google Scholar]
- Allen D. G., Eisner D. A., Lab M. J., Orchard C. H. The effects of low sodium solutions on intracellular calcium concentration and tension in ferret ventricular muscle. J Physiol. 1983 Dec;345:391–407. doi: 10.1113/jphysiol.1983.sp014984. [DOI] [PMC free article] [PubMed] [Google Scholar]
- Allen D. G., Eisner D. A., Orchard C. H. Characterization of oscillations of intracellular calcium concentration in ferret ventricular muscle. J Physiol. 1984 Jul;352:113–128. doi: 10.1113/jphysiol.1984.sp015281. [DOI] [PMC free article] [PubMed] [Google Scholar]
- Allen D. G., Eisner D. A., Pirolo J. S., Smith G. L. The relationship between intracellular calcium and contraction in calcium-overloaded ferret papillary muscles. J Physiol. 1985 Jul;364:169–182. doi: 10.1113/jphysiol.1985.sp015737. [DOI] [PMC free article] [PubMed] [Google Scholar]
- Allen D. G., Morris P. G., Orchard C. H., Pirolo J. S. A nuclear magnetic resonance study of metabolism in the ferret heart during hypoxia and inhibition of glycolysis. J Physiol. 1985 Apr;361:185–204. doi: 10.1113/jphysiol.1985.sp015640. [DOI] [PMC free article] [PubMed] [Google Scholar]
- Bailey I. A., Williams S. R., Radda G. K., Gadian D. G. Activity of phosphorylase in total global ischaemia in the rat heart. A phosphorus-31 nuclear-magnetic-resonance study. Biochem J. 1981 Apr 15;196(1):171–178. doi: 10.1042/bj1960171. [DOI] [PMC free article] [PubMed] [Google Scholar]
- Bers D. M., Ellis D. Intracellular calcium and sodium activity in sheep heart Purkinje fibres. Effect of changes of external sodium and intracellular pH. Pflugers Arch. 1982 Apr;393(2):171–178. doi: 10.1007/BF00582941. [DOI] [PubMed] [Google Scholar]
- CARLSON F. D., SIGER A. The creatine phosphoryltransfer reaction in iodoacetate-poisoned muscle. J Gen Physiol. 1959 Nov;43:301–313. doi: 10.1085/jgp.43.2.301. [DOI] [PMC free article] [PubMed] [Google Scholar]
- Deitmer J. W., Ellis D. Interactions between the regulation of the intracellular pH and sodium activity of sheep cardiac Purkinje fibres. J Physiol. 1980 Jul;304:471–488. doi: 10.1113/jphysiol.1980.sp013337. [DOI] [PMC free article] [PubMed] [Google Scholar]
- Ellis D., MacLeod K. T. Sodium-dependent control of intracellular pH in Purkinje fibres of sheep heart. J Physiol. 1985 Feb;359:81–105. doi: 10.1113/jphysiol.1985.sp015576. [DOI] [PMC free article] [PubMed] [Google Scholar]
- Ellis D., Thomas R. C. Direct measurement of the intracellular pH of mammalian cardiac muscle. J Physiol. 1976 Nov;262(3):755–771. doi: 10.1113/jphysiol.1976.sp011619. [DOI] [PMC free article] [PubMed] [Google Scholar]
- Fabiato A., Fabiato F. Effects of pH on the myofilaments and the sarcoplasmic reticulum of skinned cells from cardiace and skeletal muscles. J Physiol. 1978 Mar;276:233–255. doi: 10.1113/jphysiol.1978.sp012231. [DOI] [PMC free article] [PubMed] [Google Scholar]
- Ishikawa S., Vassalle M. Reversal of strophanthidin negative inotropy by metabolic substrates in cardiac Purkinje fibres. Cardiovasc Res. 1985 Sep;19(9):537–551. doi: 10.1093/cvr/19.9.537. [DOI] [PubMed] [Google Scholar]
- KIPNIS D. M., CORI C. F. Studies of tissue permeability. V. The penetration and phosphorylation of 2-deoxyglucose in the rat diaphragm. J Biol Chem. 1959 Jan;234(1):171–177. [PubMed] [Google Scholar]
- Kentish J. C. The effects of inorganic phosphate and creatine phosphate on force production in skinned muscles from rat ventricle. J Physiol. 1986 Jan;370:585–604. doi: 10.1113/jphysiol.1986.sp015952. [DOI] [PMC free article] [PubMed] [Google Scholar]
- Kort A. A., Lakatta E. G. Calcium-dependent mechanical oscillations occur spontaneously in unstimulated mammalian cardiac tissues. Circ Res. 1984 Apr;54(4):396–404. doi: 10.1161/01.res.54.4.396. [DOI] [PubMed] [Google Scholar]
- Lee K. S., Klaus W. The subcellular basis for the mechanism of inotropic action of cardiac glycosides. Pharmacol Rev. 1971 Sep;23(3):193–261. [PubMed] [Google Scholar]
- Marban E., Rink T. J., Tsien R. W., Tsien R. Y. Free calcium in heart muscle at rest and during contraction measured with Ca2+ -sensitive microelectrodes. Nature. 1980 Aug 28;286(5776):845–850. doi: 10.1038/286845a0. [DOI] [PubMed] [Google Scholar]
- Matthews P. M., Bland J. L., Gadian D. G., Radda G. K. The steady-state rate of ATP synthesis in the perfused rat heart measured by 31P NMR saturation transfer. Biochem Biophys Res Commun. 1981 Dec 15;103(3):1052–1059. doi: 10.1016/0006-291x(81)90915-3. [DOI] [PubMed] [Google Scholar]
- Meech R. W., Thomas R. C. Effect of measured calcium chloride injections on the membrane potential and internal pH of snail neurones. J Physiol. 1980 Jan;298:111–129. doi: 10.1113/jphysiol.1980.sp013070. [DOI] [PMC free article] [PubMed] [Google Scholar]
- Piwnica-Worms D., Lieberman M. Microfluorometric monitoring of pHi in cultured heart cells: Na+-H+ exchange. Am J Physiol. 1983 May;244(5):C422–C428. doi: 10.1152/ajpcell.1983.244.5.C422. [DOI] [PubMed] [Google Scholar]
- Sheu S. S., Fozzard H. A. Transmembrane Na+ and Ca2+ electrochemical gradients in cardiac muscle and their relationship to force development. J Gen Physiol. 1982 Sep;80(3):325–351. doi: 10.1085/jgp.80.3.325. [DOI] [PMC free article] [PubMed] [Google Scholar]
- Valdeolmillos M., Eisner D. A. The effects of ryanodine on calcium-overloaded sheep cardiac Purkinje fibers. Circ Res. 1985 Mar;56(3):452–456. doi: 10.1161/01.res.56.3.452. [DOI] [PubMed] [Google Scholar]
- Vassalle M., Lin C. I. Effect of calcium on strophanthidin-induced electrical and mechanical toxicity in cardiac Purkinje fibers. Am J Physiol. 1979 May;236(5):H689–H697. doi: 10.1152/ajpheart.1979.236.5.H689. [DOI] [PubMed] [Google Scholar]
- Vaughan-Jones R. D., Lederer W. J., Eisner D. A. Ca2+ ions can affect intracellular pH in mammalian cardiac muscle. Nature. 1983 Feb 10;301(5900):522–524. doi: 10.1038/301522a0. [DOI] [PubMed] [Google Scholar]
- Ventura-Clapier R., Vassort G. Rigor tension during metabolic and ionic rises in resting tension in rat heart. J Mol Cell Cardiol. 1981 Jun;13(6):551–561. doi: 10.1016/0022-2828(81)90326-6. [DOI] [PubMed] [Google Scholar]
- Wier W. G., Hess P. Excitation-contraction coupling in cardiac Purkinje fibers. Effects of cardiotonic steroids on the intracellular [Ca2+] transient, membrane potential, and contraction. J Gen Physiol. 1984 Mar;83(3):395–415. doi: 10.1085/jgp.83.3.395. [DOI] [PMC free article] [PubMed] [Google Scholar]
- Wier W. G., Kort A. A., Stern M. D., Lakatta E. G., Marban E. Cellular calcium fluctuations in mammalian heart: direct evidence from noise analysis of aequorin signals in Purkinje fibers. Proc Natl Acad Sci U S A. 1983 Dec;80(23):7367–7371. doi: 10.1073/pnas.80.23.7367. [DOI] [PMC free article] [PubMed] [Google Scholar]