Abstract
Isolated cells from rat lacrimal glands were studied with the tight-seal whole-cell recording technique. Cells were dialysed with K-free solutions containing a high concentration of Ca2+ buffer in order to record Ca-dependent Cl- currents at a Ca2+ level fixed between 0.1 and 10 microM. HEDTA was preferable to EGTA as a Ca2+ buffer, even under conditions of equivalent equilibrium buffering power. After replacement of all internal K+ with Na+, the cells displayed a small conductance component which could be abolished by removal of external K+ or by external application of 2 mM-tetraethylammonium. It is suggested that this conductance is due to Ca-dependent K+ channels. The main part of the cell current was Cl selective. The Cl- conductance was negligible at 0.5 microM-Ca2+, and fully activated at 2 microM-Ca2+. The dose-response curve relating Cl- currents to the internal Ca2+ concentration, [Ca]i, was steeper than predicted by a simple binding isotherm reaction. Relaxations observed in response to voltage jumps could, in most cases, be fitted with single exponentials. At [Ca]i 0.5 microM, the curve relating the relaxation time constant, tau, to the membrane potential, displayed a maximum near +20 mV and 250 ms. At hyperpolarized potentials, tau varied by an e-fold factor in 130 mV. At [Ca]i 1 microM, tau decreased from 100 ms at -120 mV to 60 ms at +60 mV. Relaxation analysis gave an estimate of the variation of the channel open state probability, Po, with potential. At [Ca]i 0.5 microM, Po varied by an e-fold factor in 50-70 mV at hyperpolarized potentials, and saturated above +60 mV. At [Ca]i 1 microM, Po varied e-fold in 100 to 110 mV at hyperpolarized potentials, and saturated near +20 mV. External Cl- was substituted with various anions. From reversal potential measurements, the following permeability sequence was obtained: I- greater than NO3- greater than Br- greater than Cl- greater than F- greater than isethionate, methanesulphonate greater than glutamate. The corresponding normalized permeability coefficients were 2.7, 2.4, 1.6, 1, 0.2, 0.1, 0.1, 0.05. Replacement of external Cl- with Br-, isethionate, methanesulphonate or glutamate did not alter current kinetics as obtained during or after a depolarizing voltage jump.(ABSTRACT TRUNCATED AT 400 WORDS)
Full text
PDF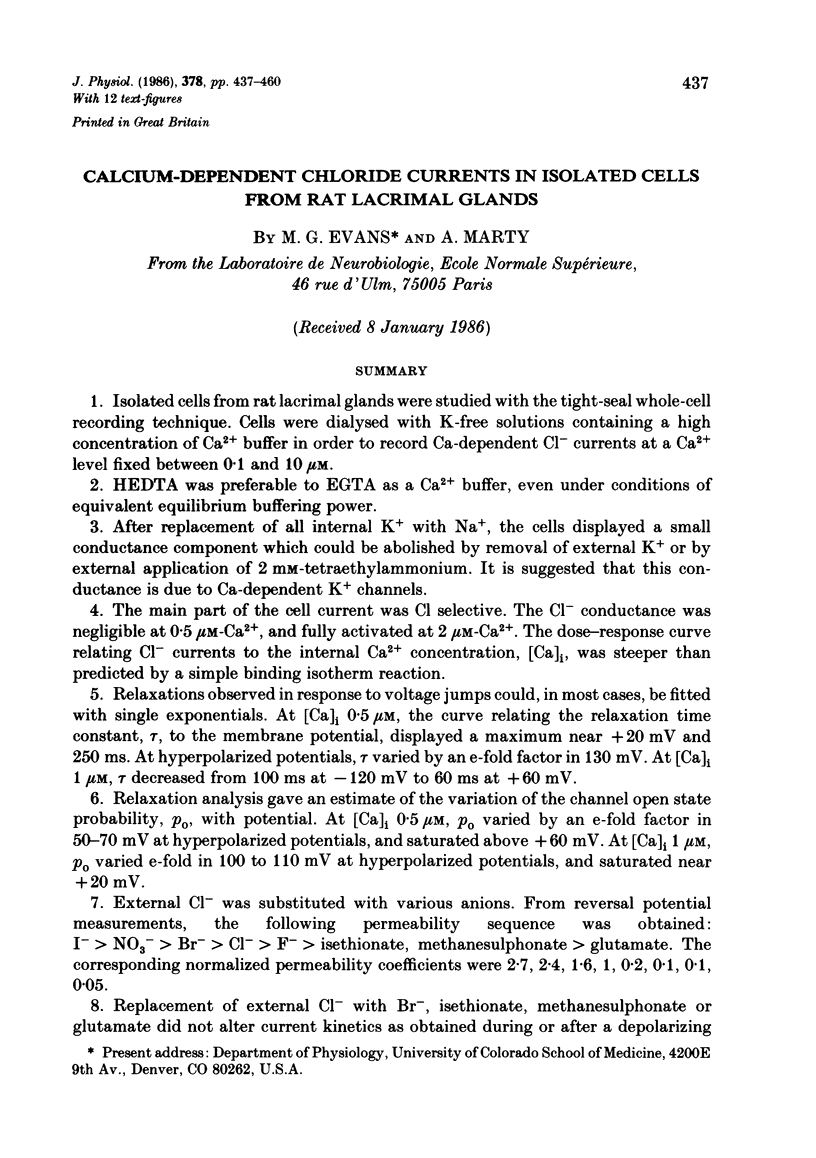
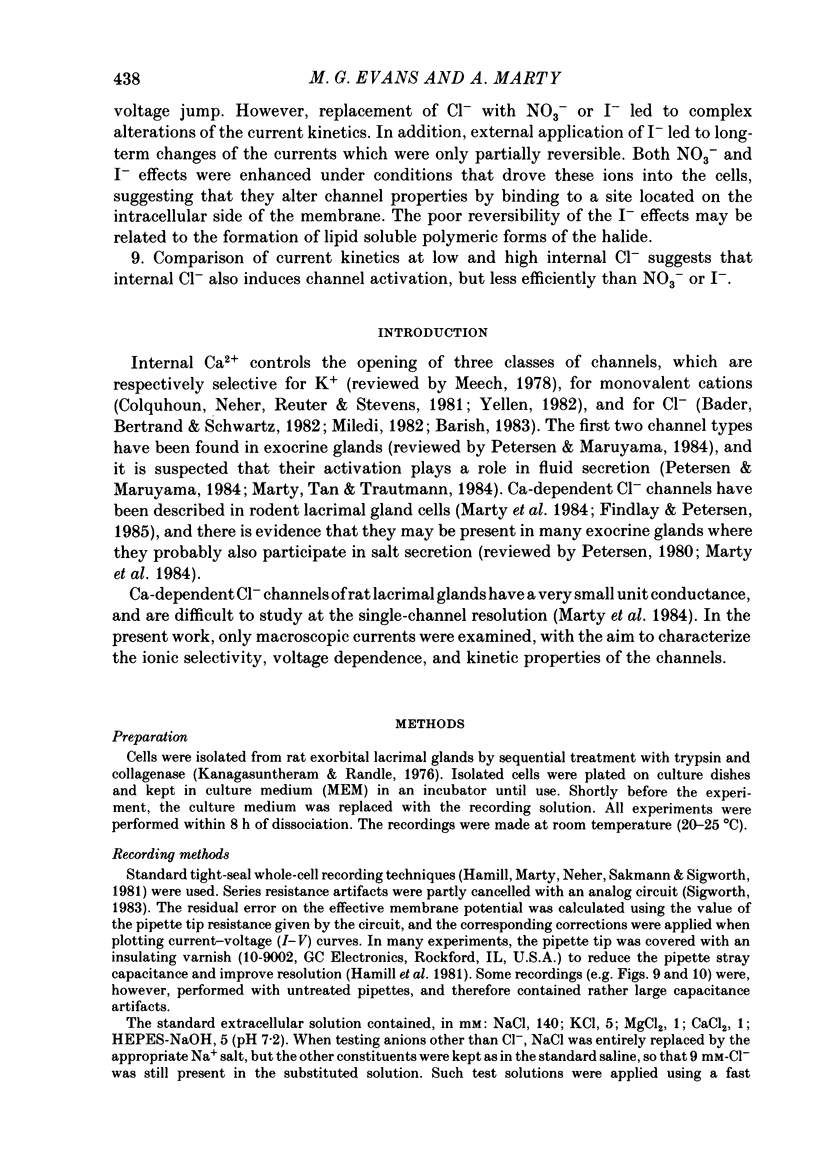
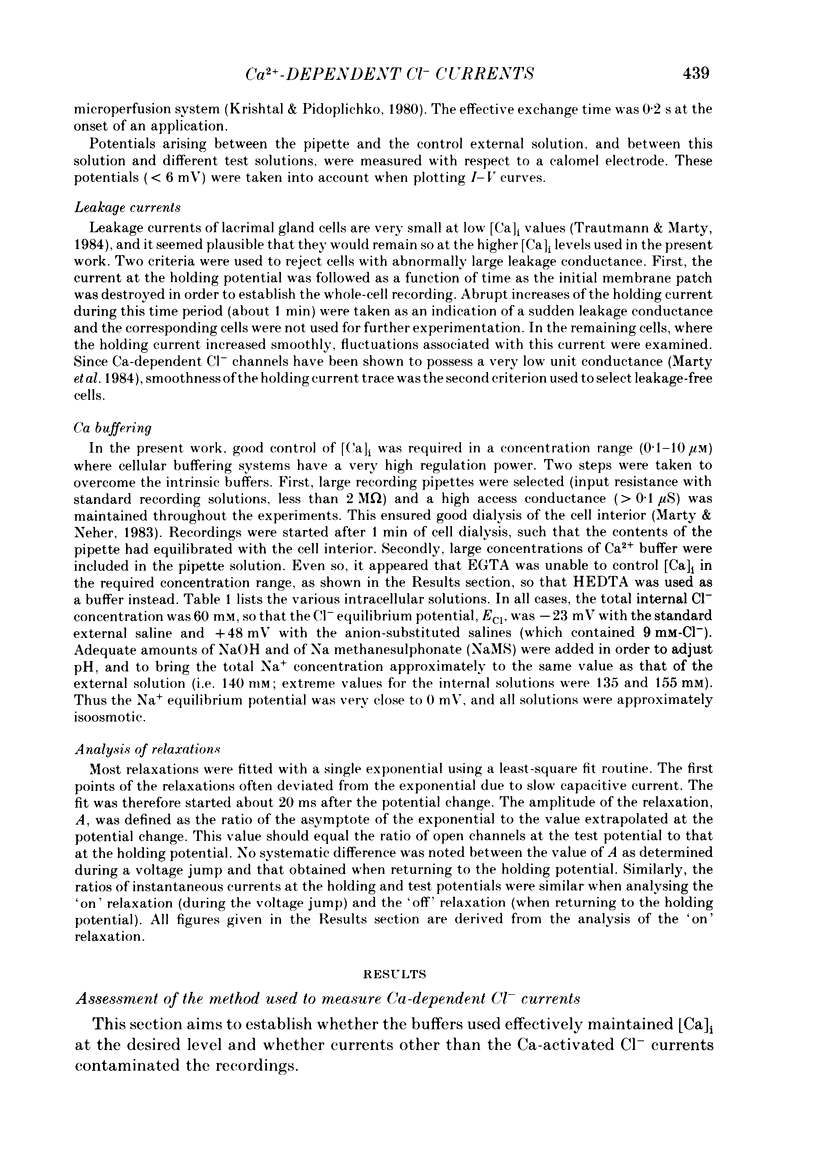
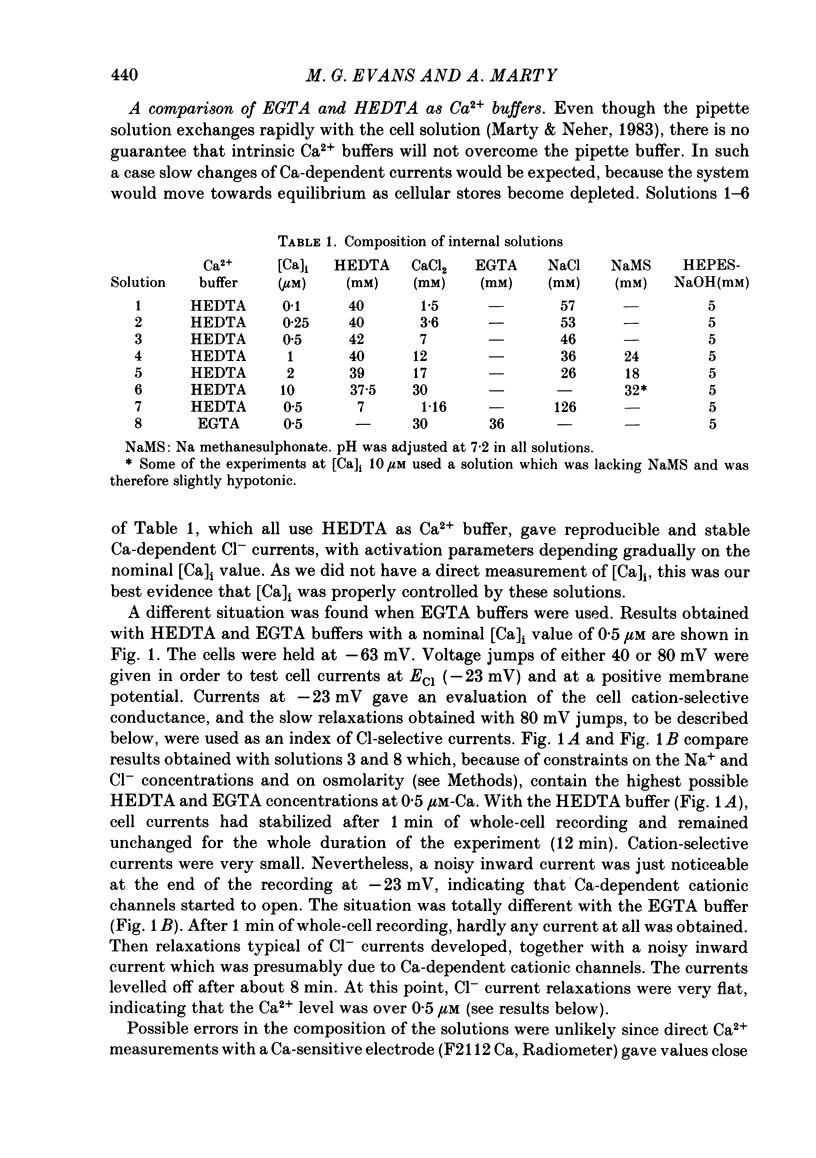
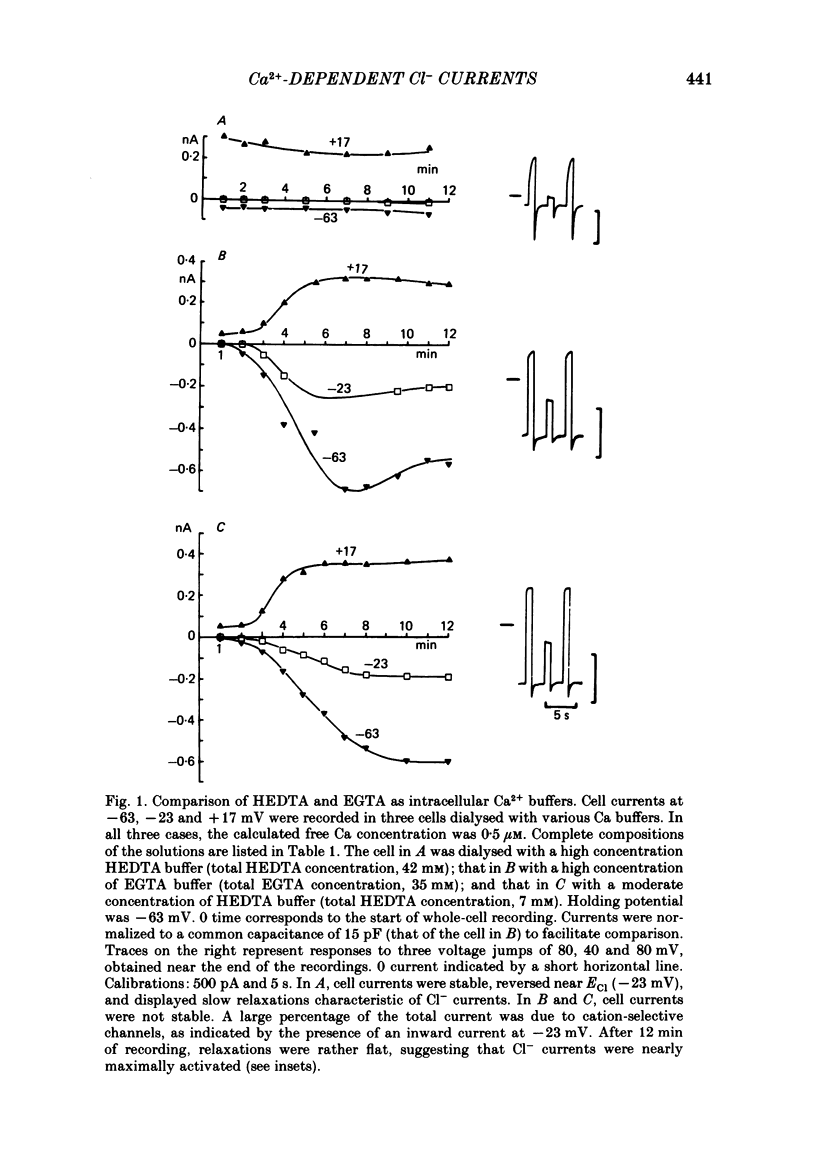
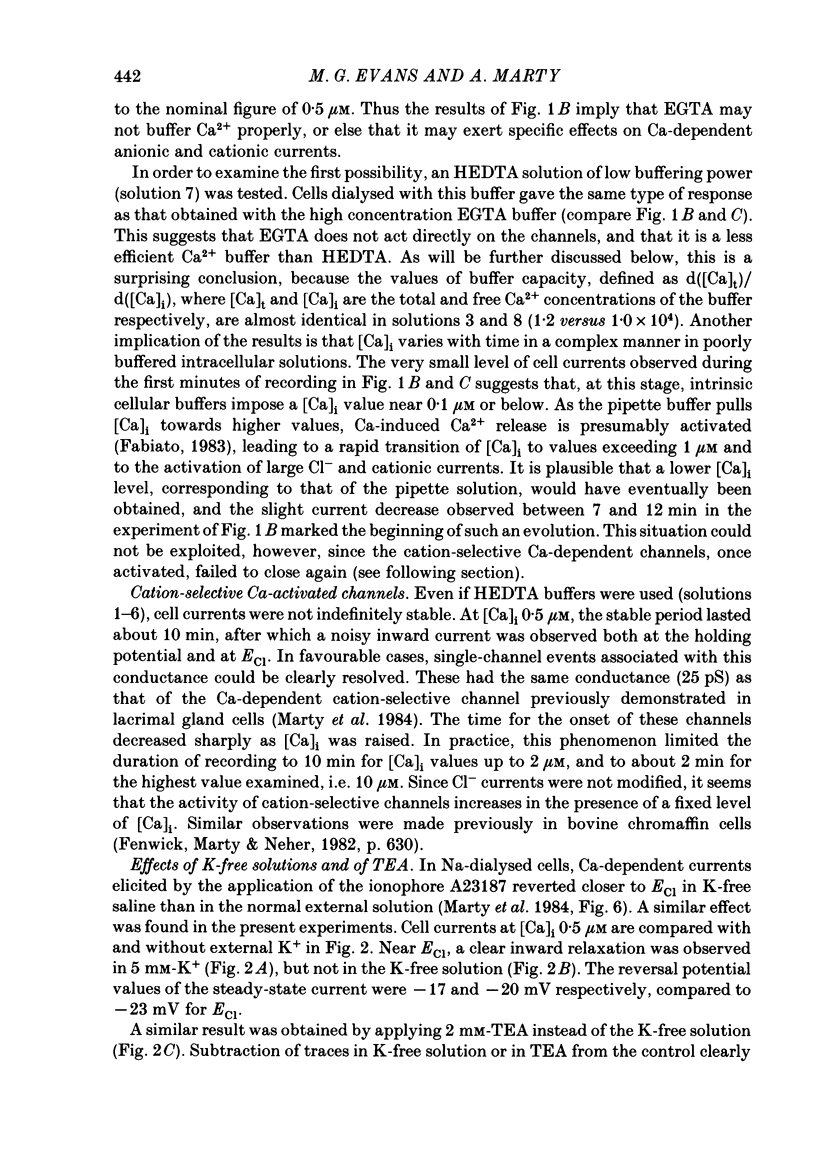
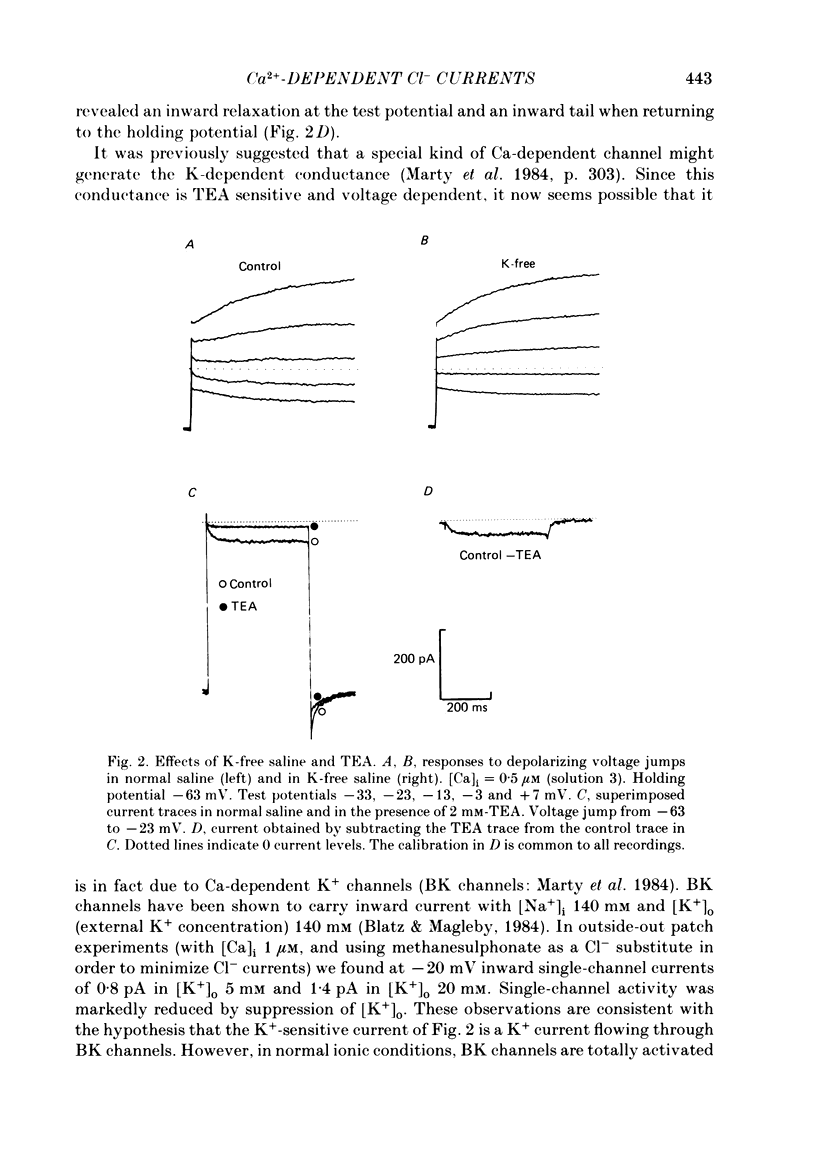
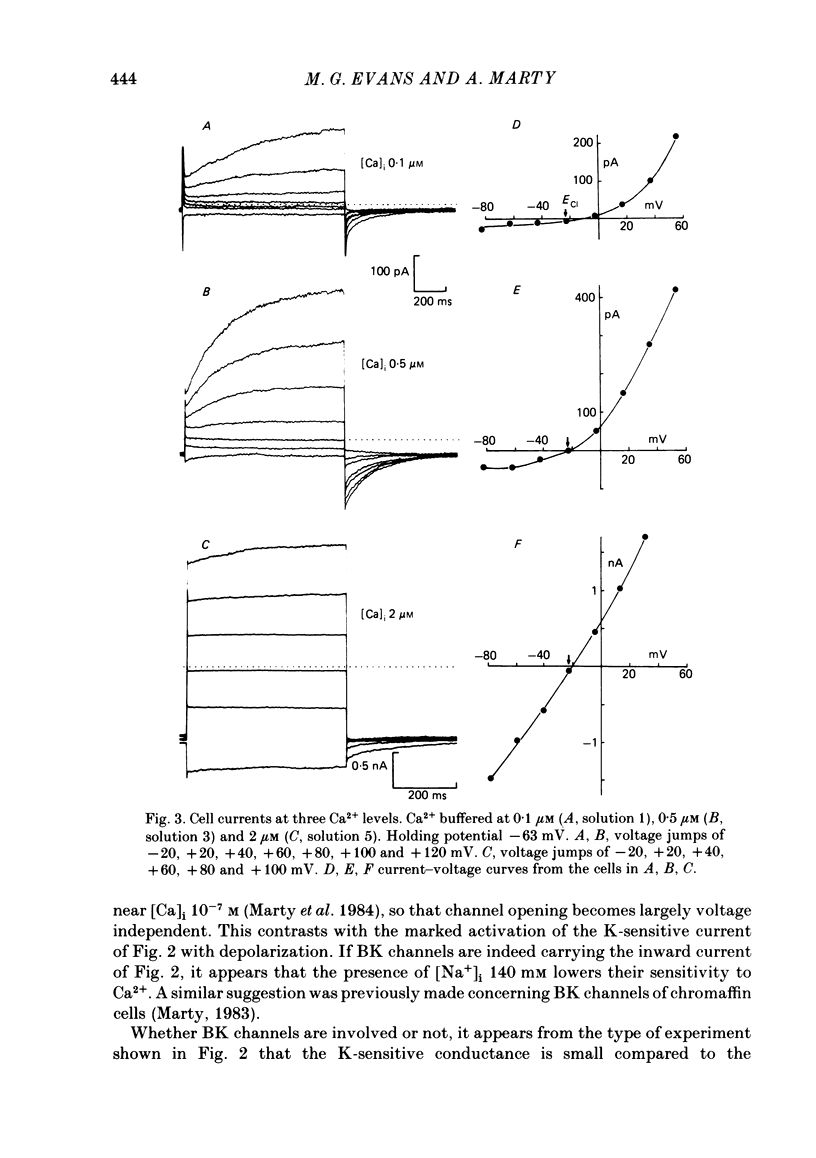
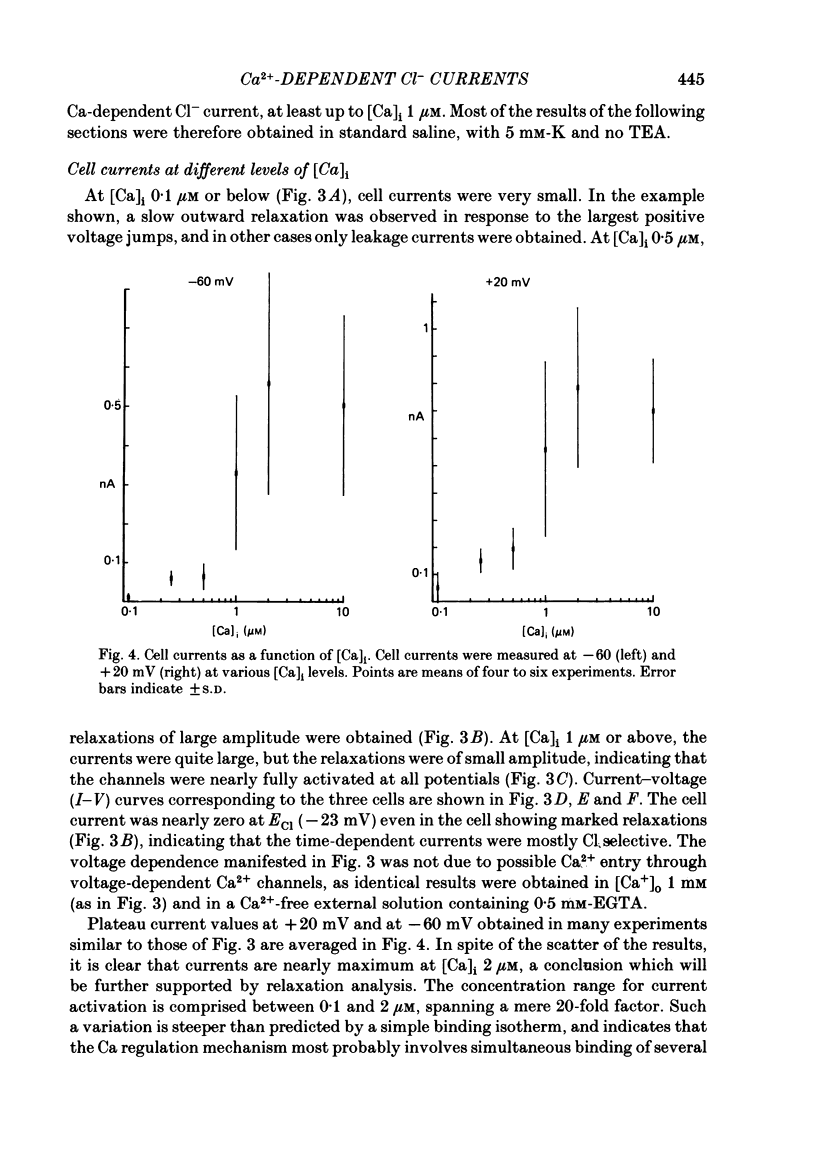
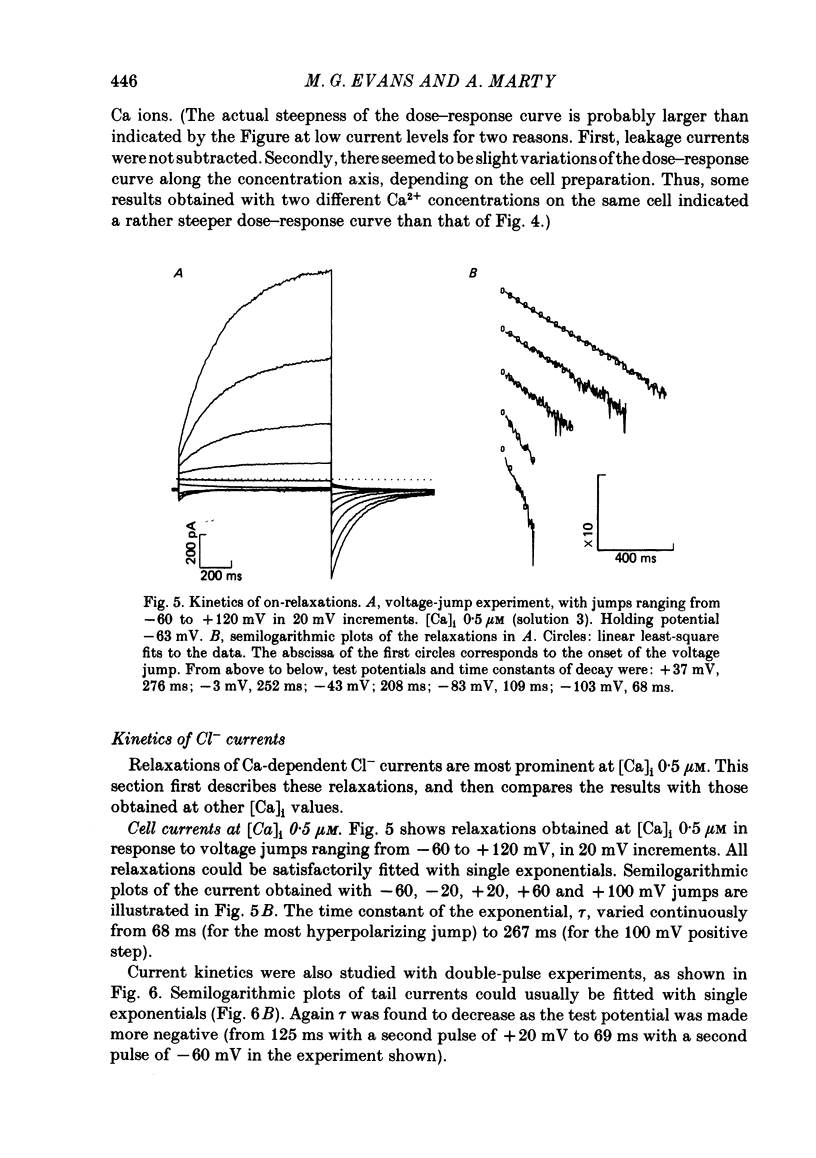
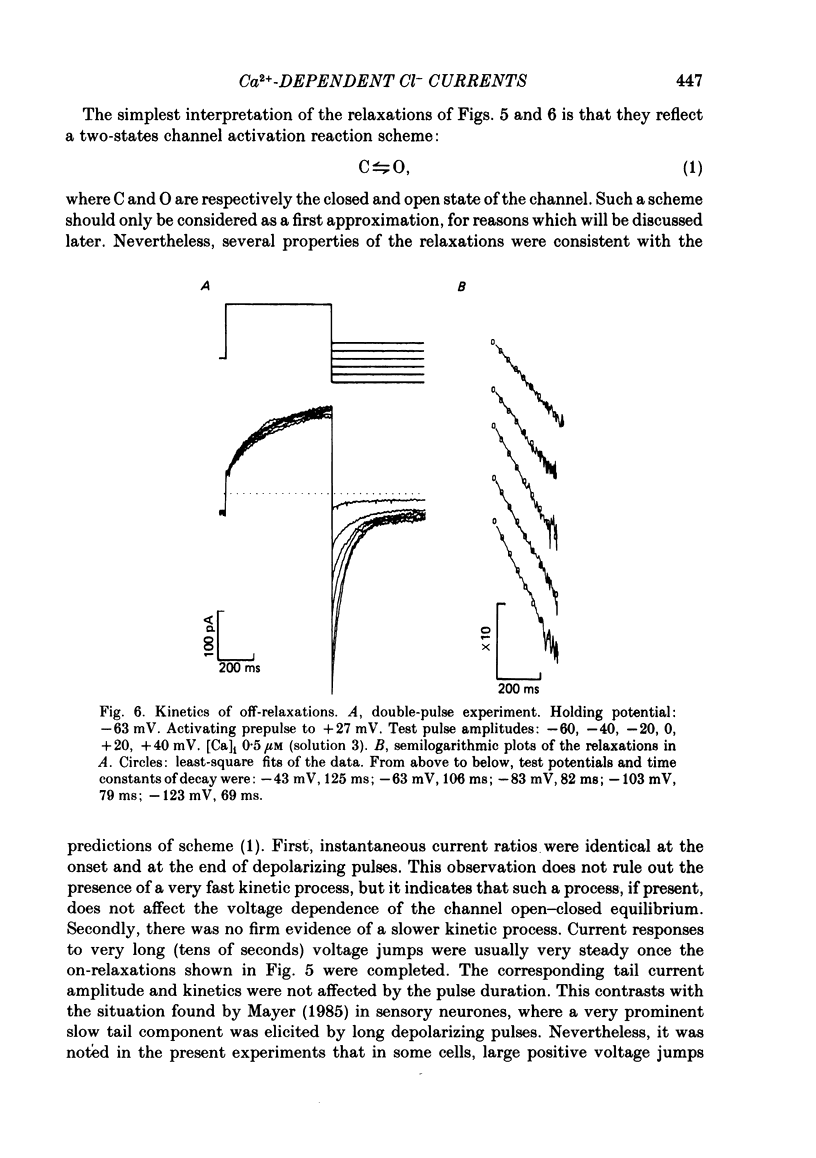
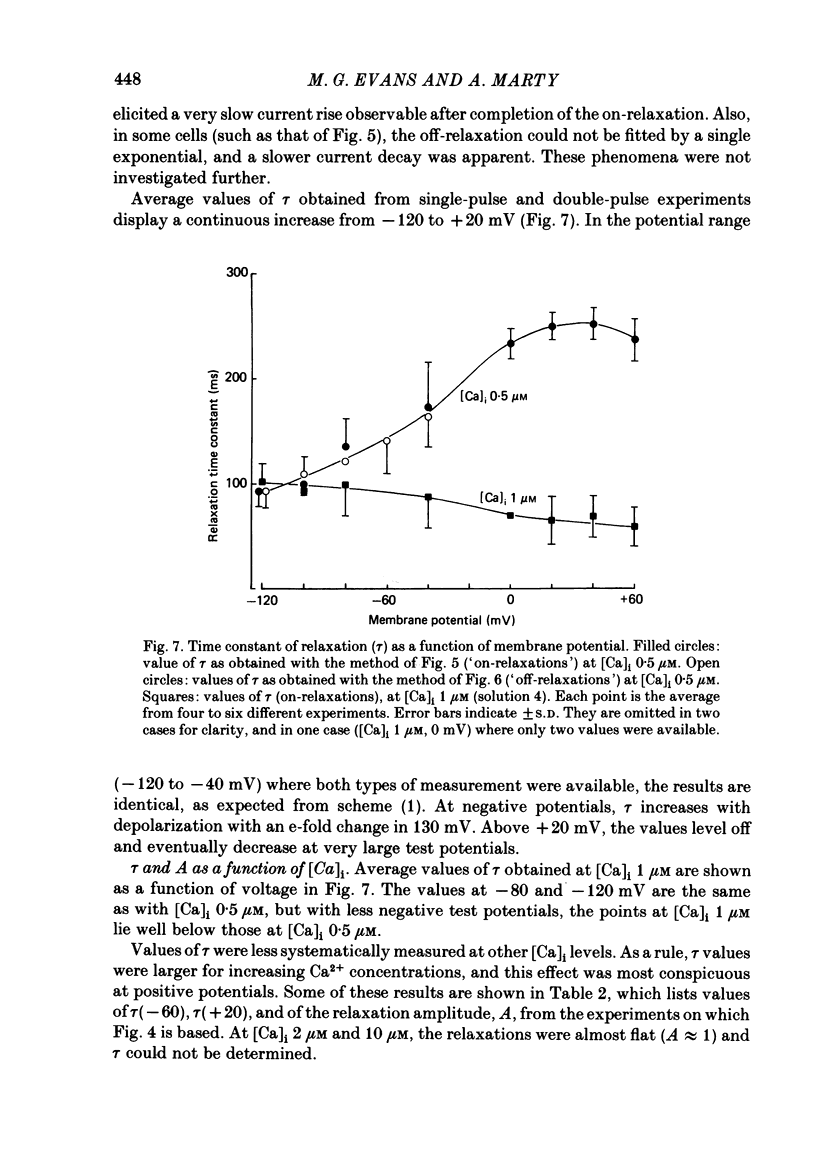
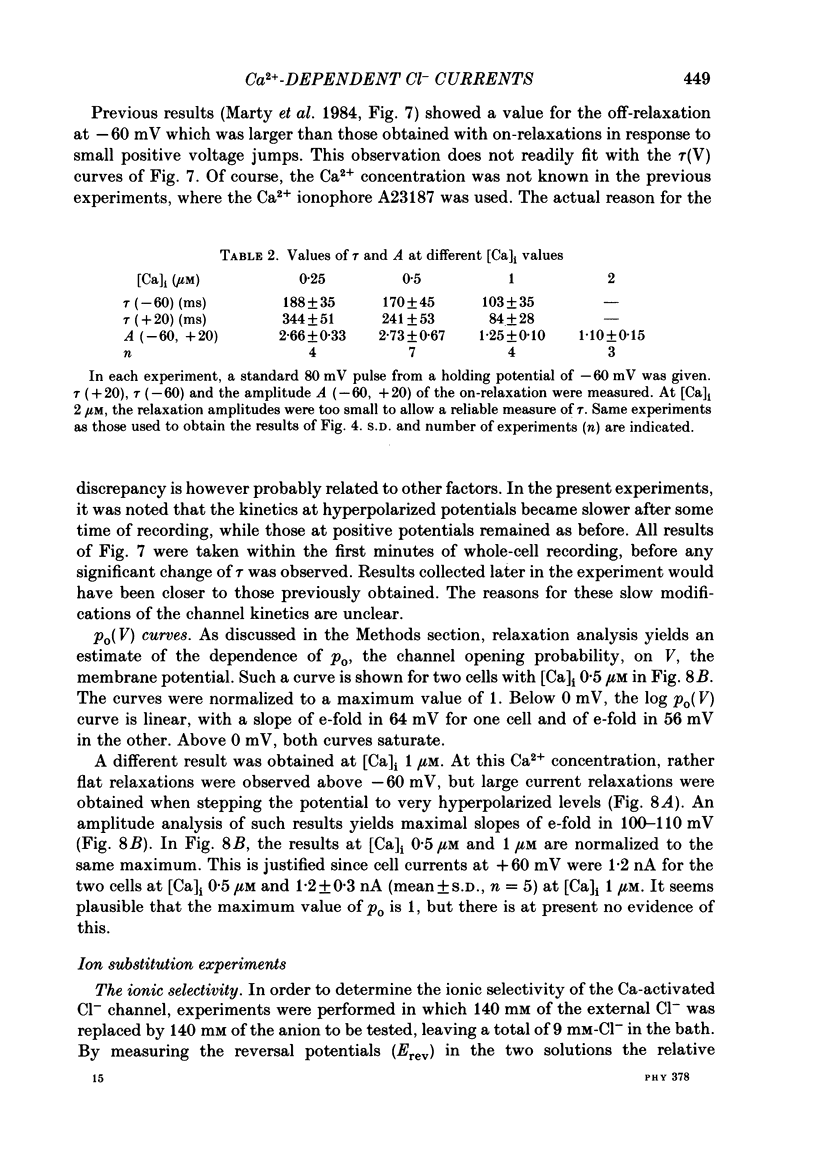
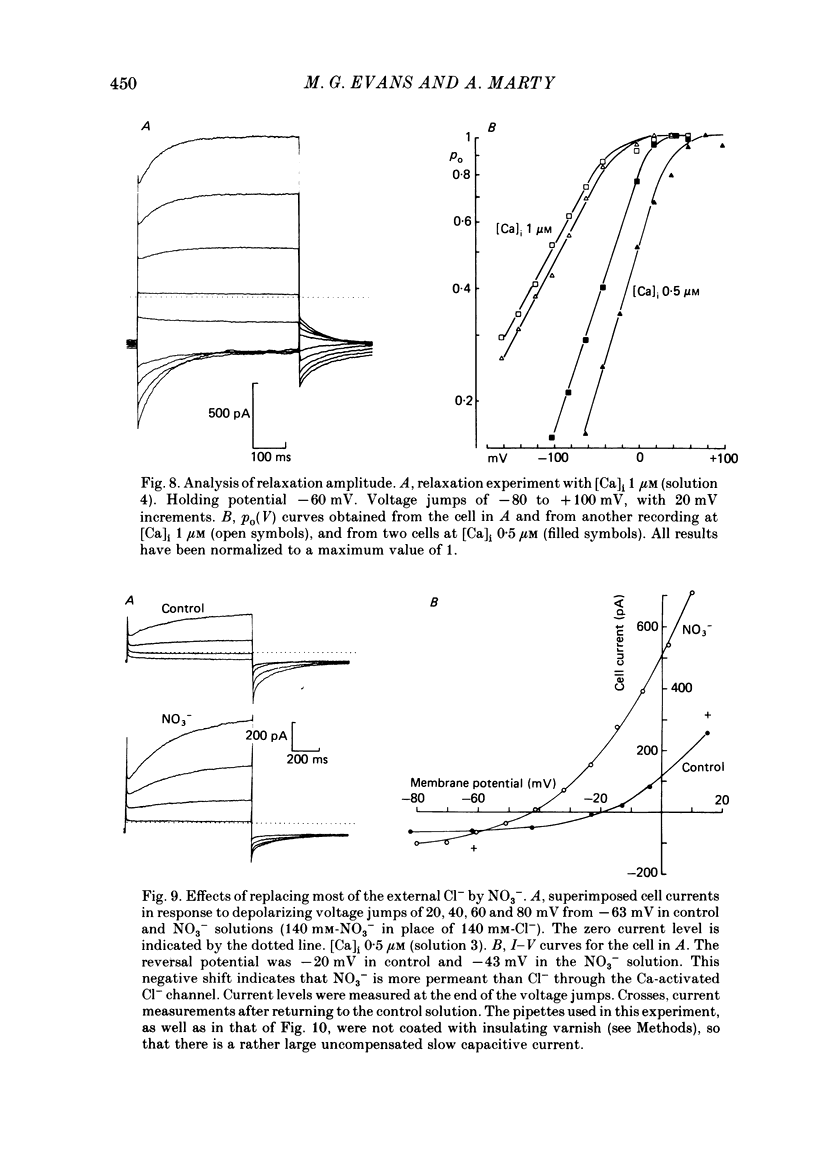
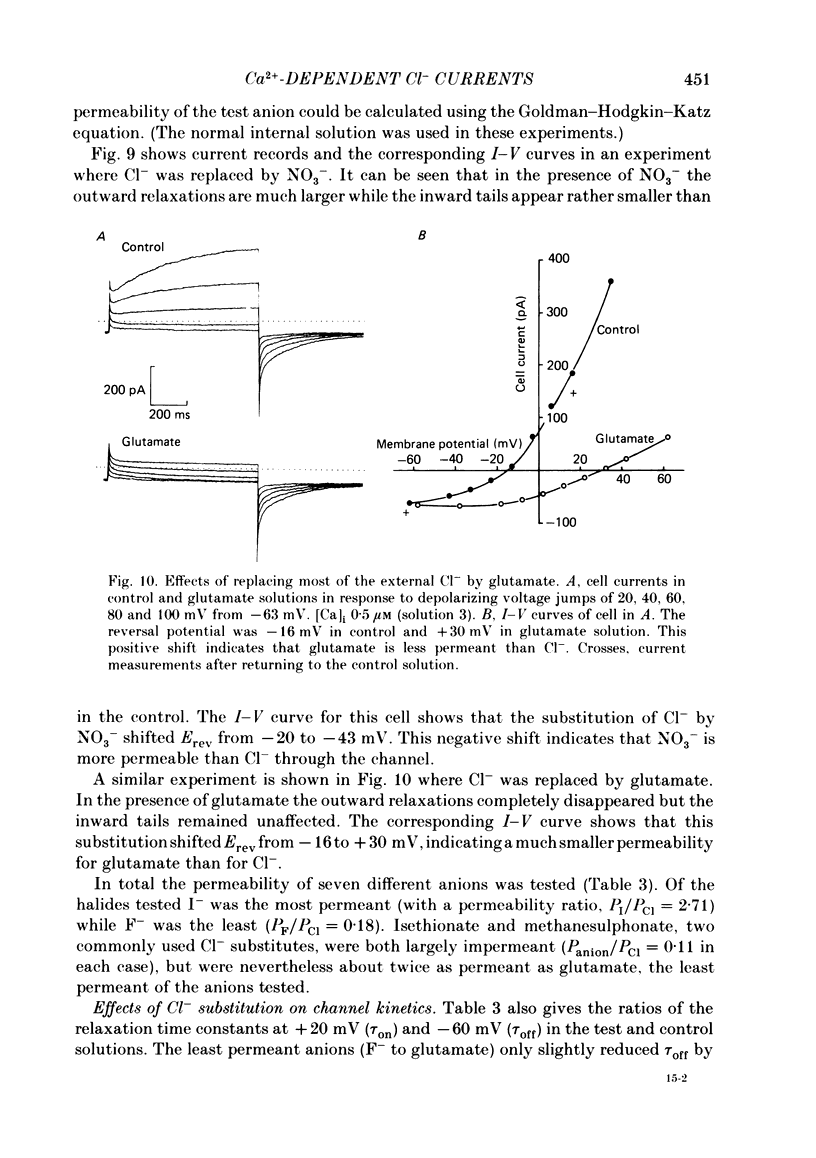
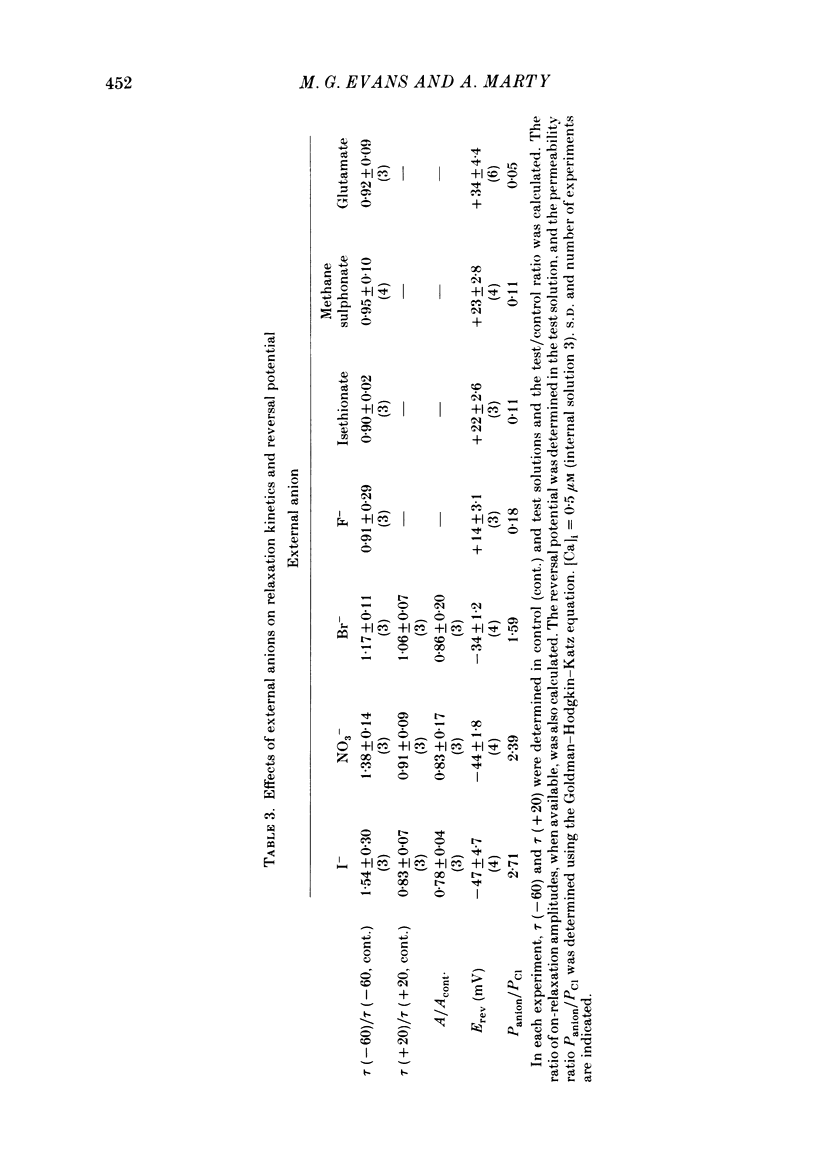
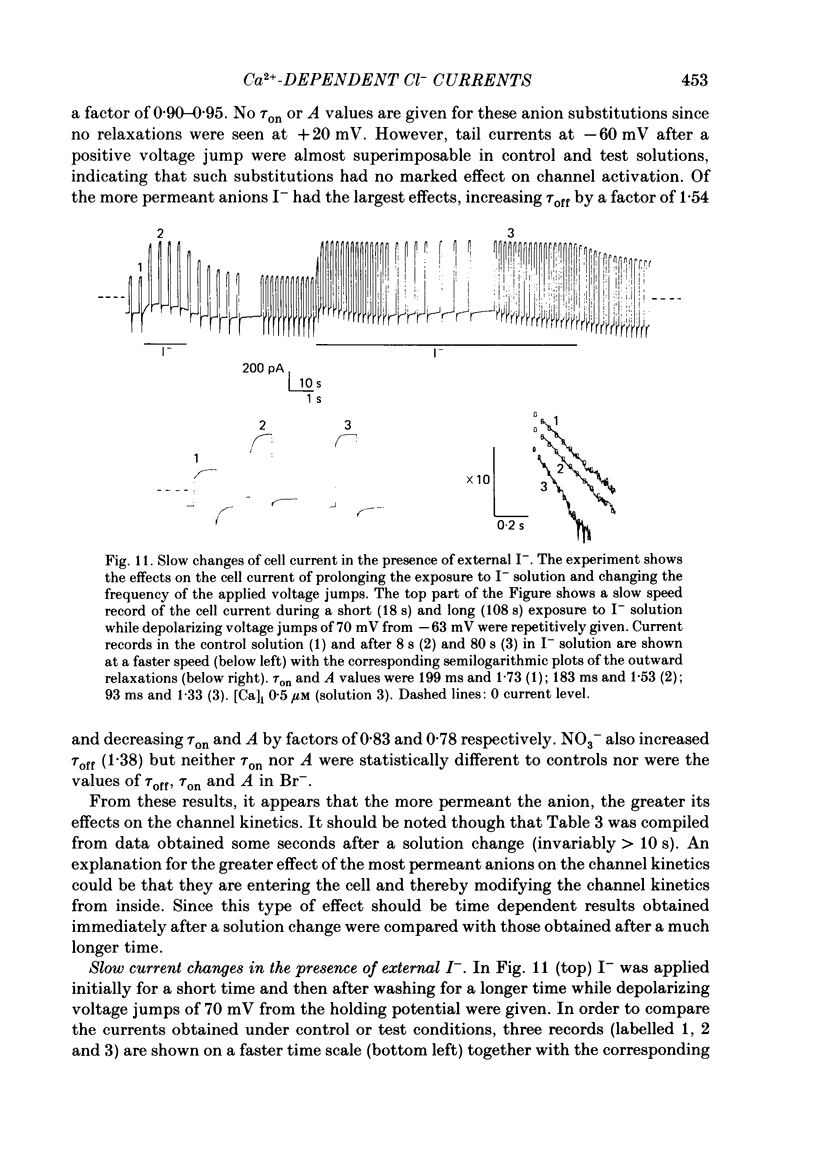
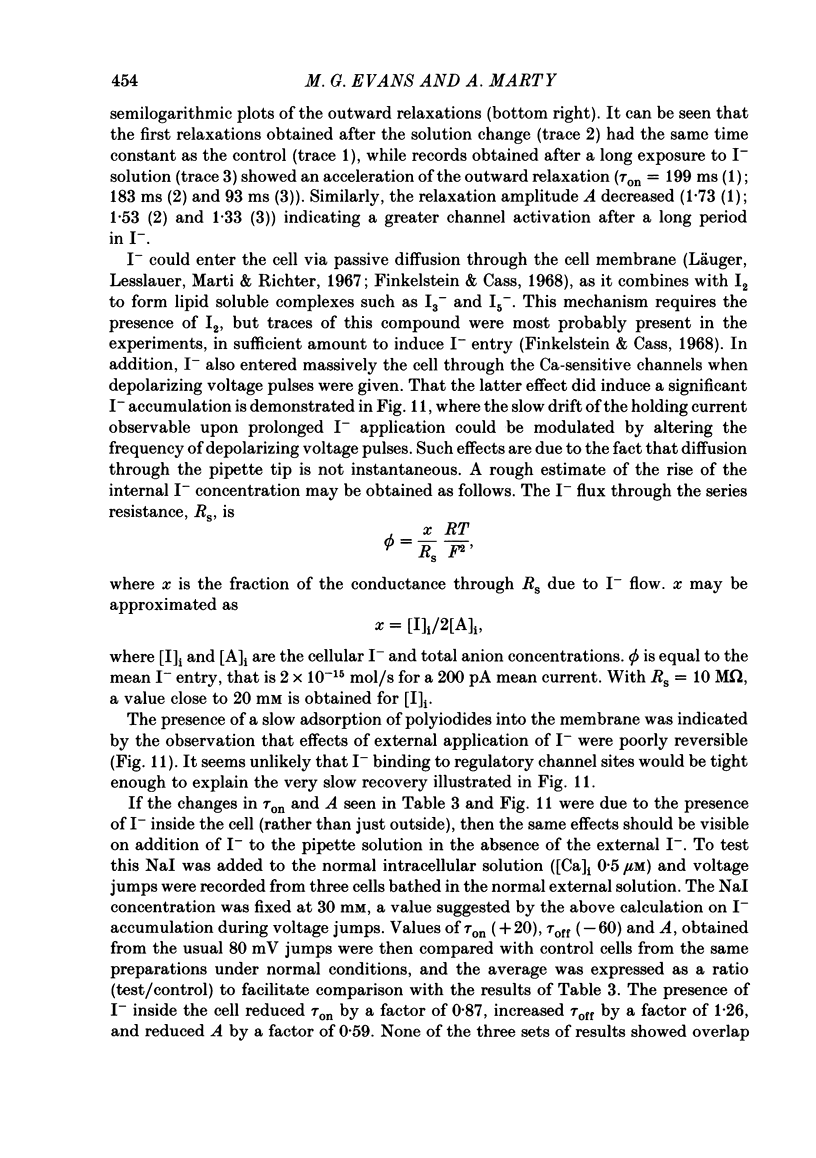
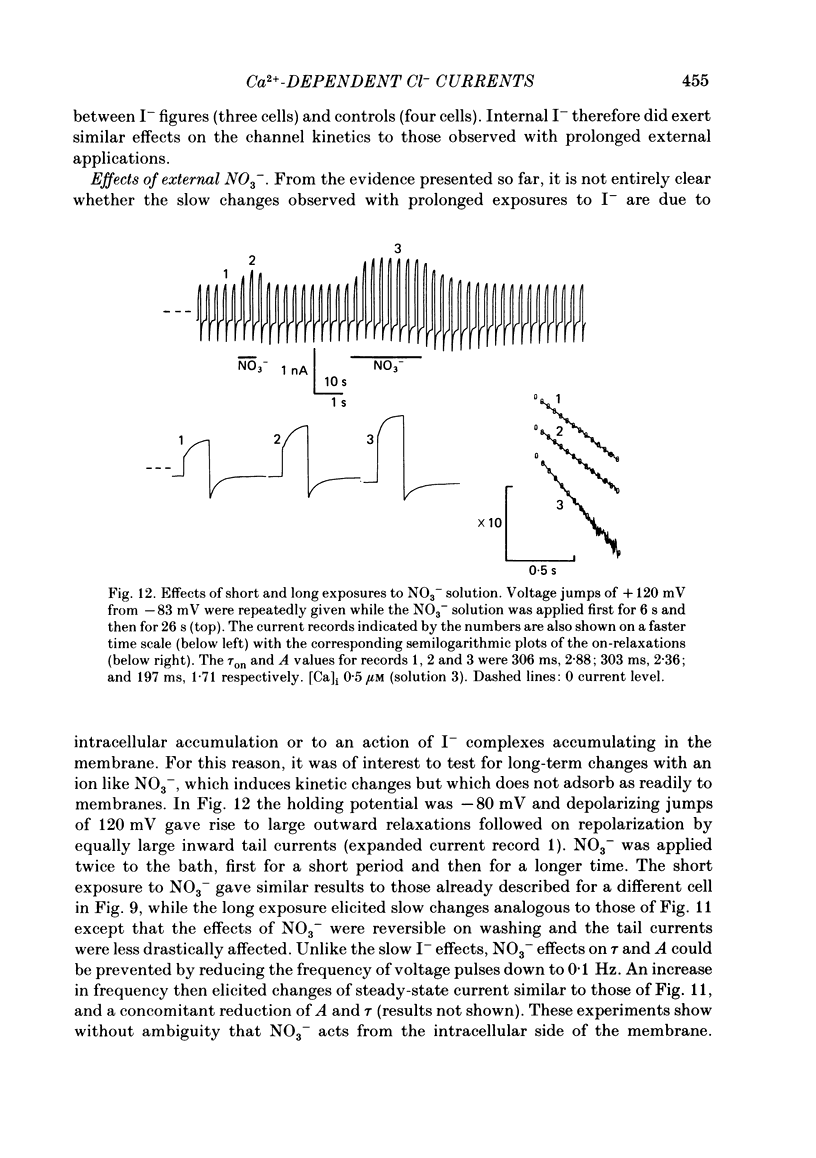
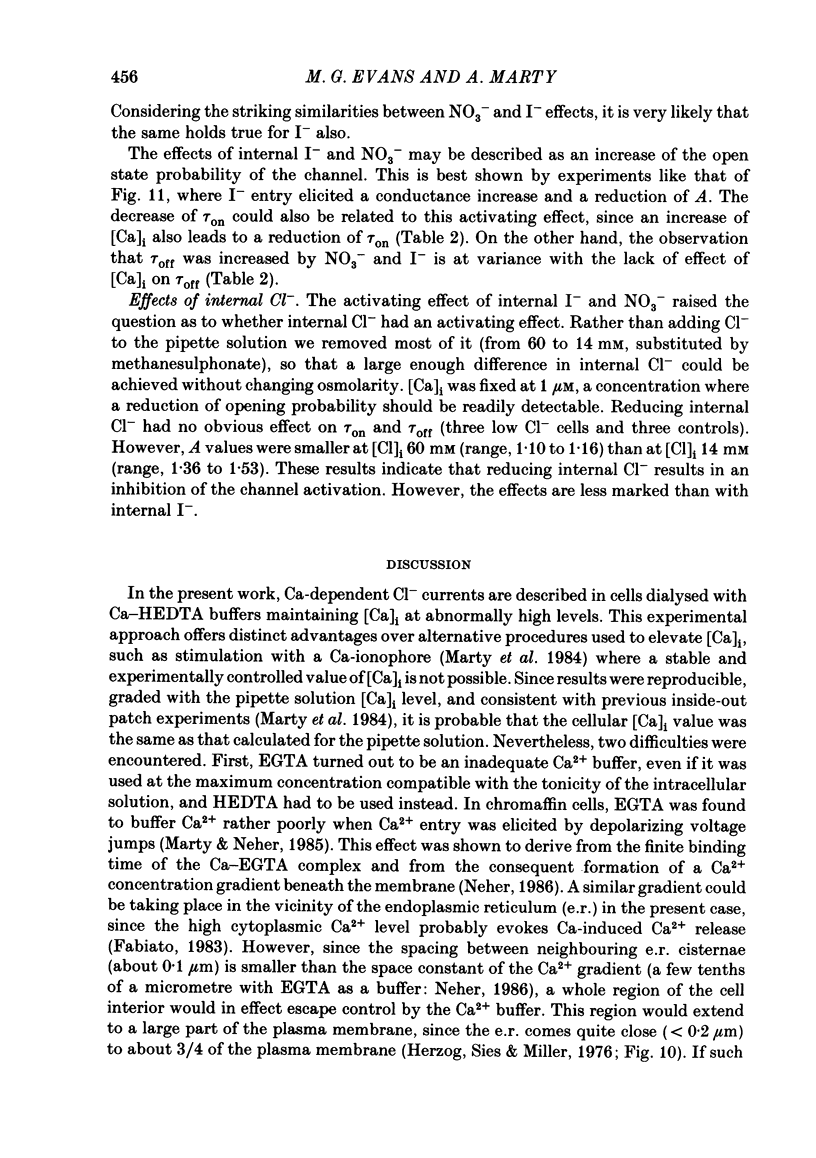
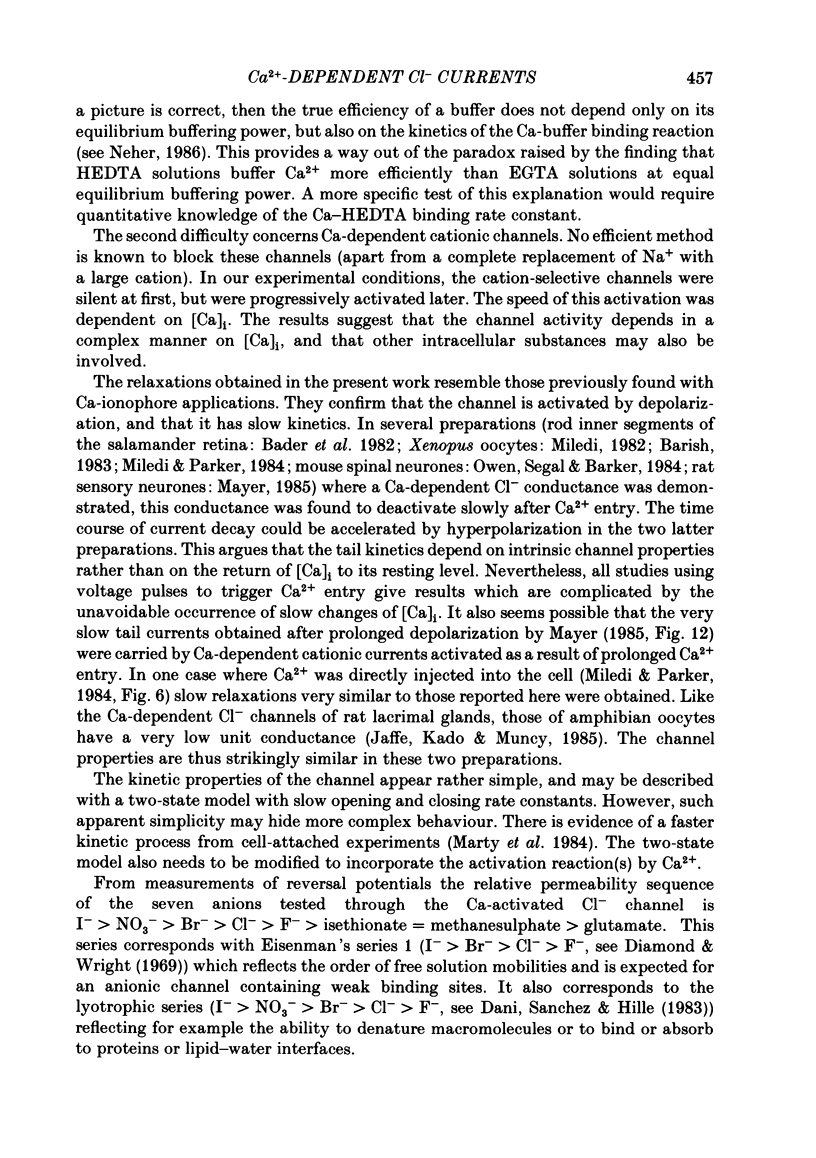
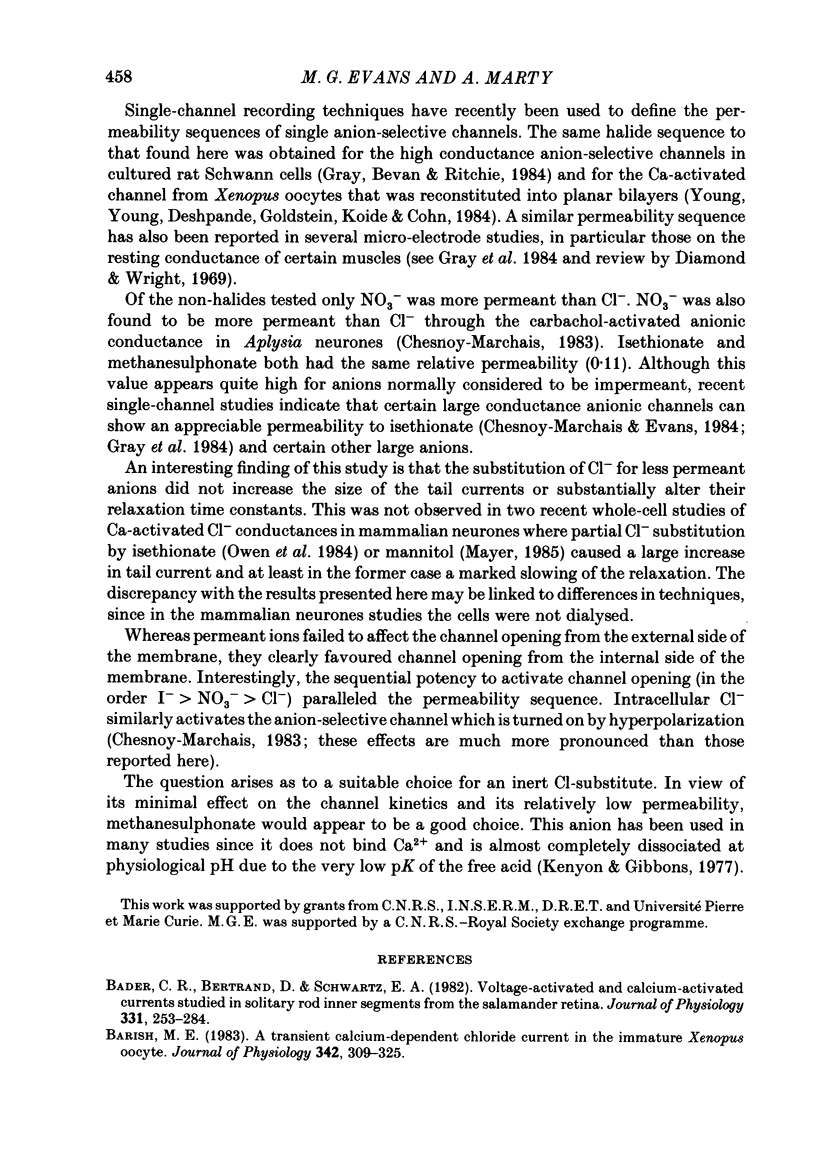
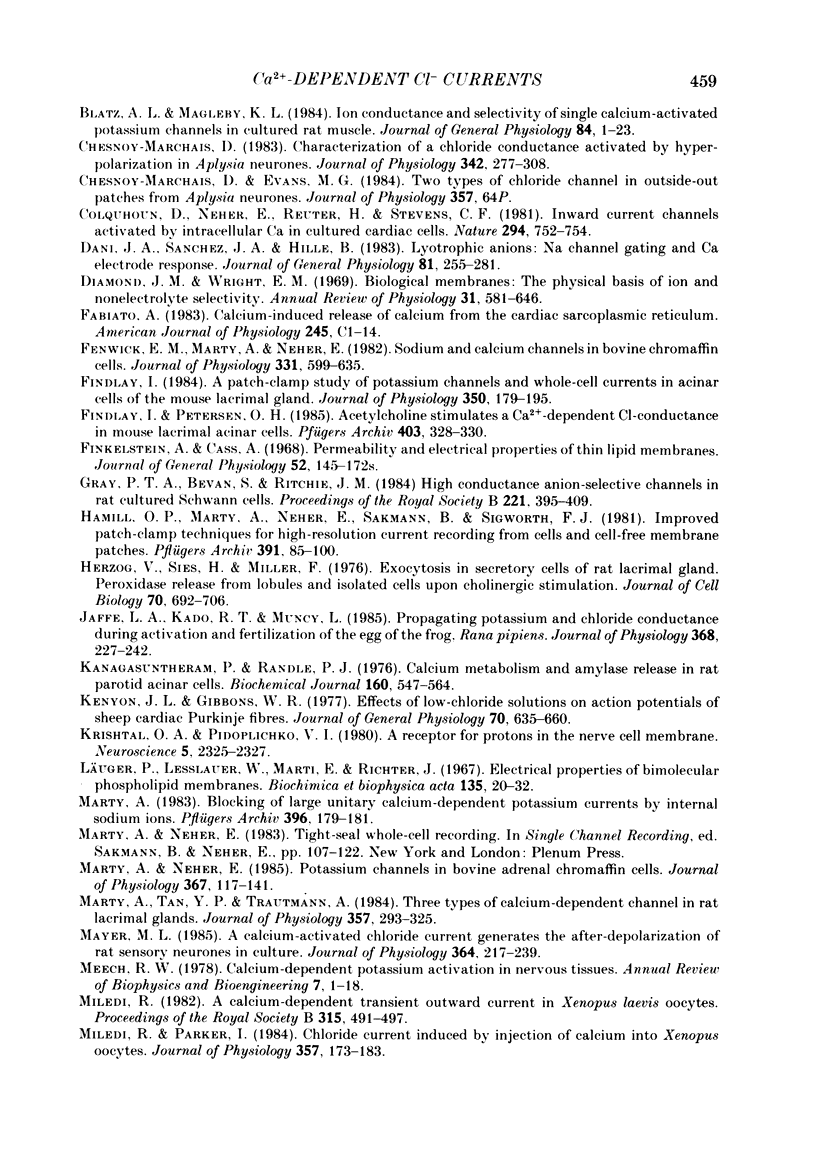
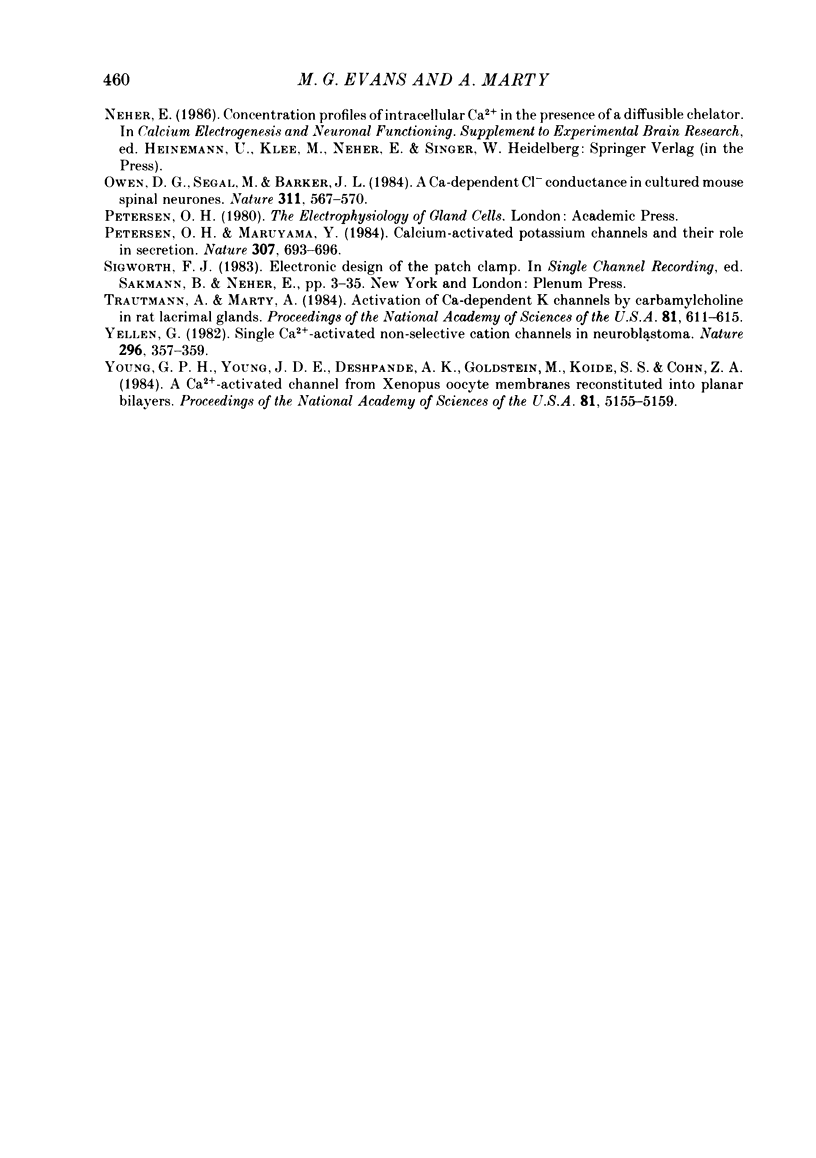
Selected References
These references are in PubMed. This may not be the complete list of references from this article.
- Bader C. R., Bertrand D., Schwartz E. A. Voltage-activated and calcium-activated currents studied in solitary rod inner segments from the salamander retina. J Physiol. 1982 Oct;331:253–284. doi: 10.1113/jphysiol.1982.sp014372. [DOI] [PMC free article] [PubMed] [Google Scholar]
- Barish M. E. A transient calcium-dependent chloride current in the immature Xenopus oocyte. J Physiol. 1983 Sep;342:309–325. doi: 10.1113/jphysiol.1983.sp014852. [DOI] [PMC free article] [PubMed] [Google Scholar]
- Blatz A. L., Magleby K. L. Ion conductance and selectivity of single calcium-activated potassium channels in cultured rat muscle. J Gen Physiol. 1984 Jul;84(1):1–23. doi: 10.1085/jgp.84.1.1. [DOI] [PMC free article] [PubMed] [Google Scholar]
- Chesnoy-Marchais D. Characterization of a chloride conductance activated by hyperpolarization in Aplysia neurones. J Physiol. 1983 Sep;342:277–308. doi: 10.1113/jphysiol.1983.sp014851. [DOI] [PMC free article] [PubMed] [Google Scholar]
- Colquhoun D., Neher E., Reuter H., Stevens C. F. Inward current channels activated by intracellular Ca in cultured cardiac cells. Nature. 1981 Dec 24;294(5843):752–754. doi: 10.1038/294752a0. [DOI] [PubMed] [Google Scholar]
- Dani J. A., Sanchez J. A., Hille B. Lyotropic anions. Na channel gating and Ca electrode response. J Gen Physiol. 1983 Feb;81(2):255–281. doi: 10.1085/jgp.81.2.255. [DOI] [PMC free article] [PubMed] [Google Scholar]
- Diamond J. M., Wright E. M. Biological membranes: the physical basis of ion and nonelectrolyte selectivity. Annu Rev Physiol. 1969;31:581–646. doi: 10.1146/annurev.ph.31.030169.003053. [DOI] [PubMed] [Google Scholar]
- Fenwick E. M., Marty A., Neher E. Sodium and calcium channels in bovine chromaffin cells. J Physiol. 1982 Oct;331:599–635. doi: 10.1113/jphysiol.1982.sp014394. [DOI] [PMC free article] [PubMed] [Google Scholar]
- Findlay I. A patch-clamp study of potassium channels and whole-cell currents in acinar cells of the mouse lacrimal gland. J Physiol. 1984 May;350:179–195. doi: 10.1113/jphysiol.1984.sp015195. [DOI] [PMC free article] [PubMed] [Google Scholar]
- Findlay I., Petersen O. H. Acetylcholine stimulates a Ca2+-dependent C1- conductance in mouse lacrimal acinar cells. Pflugers Arch. 1985 Mar;403(3):328–330. doi: 10.1007/BF00583609. [DOI] [PubMed] [Google Scholar]
- Finkelstein A., Cass A. Permeability and electrical properties of thin lipid membranes. J Gen Physiol. 1968 Jul 1;52(1):145–172. [PMC free article] [PubMed] [Google Scholar]
- Gray P. T., Bevan S., Ritchie J. M. High conductance anion-selective channels in rat cultured Schwann cells. Proc R Soc Lond B Biol Sci. 1984 Jun 22;221(1225):395–409. doi: 10.1098/rspb.1984.0041. [DOI] [PubMed] [Google Scholar]
- Hamill O. P., Marty A., Neher E., Sakmann B., Sigworth F. J. Improved patch-clamp techniques for high-resolution current recording from cells and cell-free membrane patches. Pflugers Arch. 1981 Aug;391(2):85–100. doi: 10.1007/BF00656997. [DOI] [PubMed] [Google Scholar]
- Herzog V., Sies H., Miller F. Exocytosis in secretory cells of rat lacrimal gland. Peroxidase release from lobules and isolated cells upon cholinergic stimulation. J Cell Biol. 1976 Sep;70(3):692–706. doi: 10.1083/jcb.70.3.692. [DOI] [PMC free article] [PubMed] [Google Scholar]
- Jaffe L. A., Kado R. T., Muncy L. Propagating potassium and chloride conductances during activation and fertilization of the egg of the frog, Rana pipiens. J Physiol. 1985 Nov;368:227–242. doi: 10.1113/jphysiol.1985.sp015855. [DOI] [PMC free article] [PubMed] [Google Scholar]
- Kanagasuntheram P., Randle P. J. Calcium metabolism and amylase release in rat parotid acinar cells. Biochem J. 1976 Dec 15;160(3):547–564. doi: 10.1042/bj1600547. [DOI] [PMC free article] [PubMed] [Google Scholar]
- Kenyon J. L., Gibbons W. R. Effects of low-chloride solutions on action potentials of sheep cardiac Purkinje fibers. J Gen Physiol. 1977 Nov;70(5):635–660. doi: 10.1085/jgp.70.5.635. [DOI] [PMC free article] [PubMed] [Google Scholar]
- Krishtal O. A., Pidoplichko V. I. A receptor for protons in the nerve cell membrane. Neuroscience. 1980;5(12):2325–2327. doi: 10.1016/0306-4522(80)90149-9. [DOI] [PubMed] [Google Scholar]
- Läuger P., Lesslauer W., Marti E., Richter J. Electrical properties of bimolecular phospholipid membranes. Biochim Biophys Acta. 1967 Feb 1;135(1):20–32. doi: 10.1016/0005-2736(67)90004-1. [DOI] [PubMed] [Google Scholar]
- Marty A. Blocking of large unitary calcium-dependent potassium currents by internal sodium ions. Pflugers Arch. 1983 Feb;396(2):179–181. doi: 10.1007/BF00615524. [DOI] [PubMed] [Google Scholar]
- Marty A., Neher E. Potassium channels in cultured bovine adrenal chromaffin cells. J Physiol. 1985 Oct;367:117–141. doi: 10.1113/jphysiol.1985.sp015817. [DOI] [PMC free article] [PubMed] [Google Scholar]
- Marty A., Tan Y. P., Trautmann A. Three types of calcium-dependent channel in rat lacrimal glands. J Physiol. 1984 Dec;357:293–325. doi: 10.1113/jphysiol.1984.sp015501. [DOI] [PMC free article] [PubMed] [Google Scholar]
- Mayer M. L. A calcium-activated chloride current generates the after-depolarization of rat sensory neurones in culture. J Physiol. 1985 Jul;364:217–239. doi: 10.1113/jphysiol.1985.sp015740. [DOI] [PMC free article] [PubMed] [Google Scholar]
- Meech R. W. Calcium-dependent potassium activation in nervous tissues. Annu Rev Biophys Bioeng. 1978;7:1–18. doi: 10.1146/annurev.bb.07.060178.000245. [DOI] [PubMed] [Google Scholar]
- Miledi R. A calcium-dependent transient outward current in Xenopus laevis oocytes. Proc R Soc Lond B Biol Sci. 1982 Jul 22;215(1201):491–497. doi: 10.1098/rspb.1982.0056. [DOI] [PubMed] [Google Scholar]
- Miledi R., Parker I. Chloride current induced by injection of calcium into Xenopus oocytes. J Physiol. 1984 Dec;357:173–183. doi: 10.1113/jphysiol.1984.sp015495. [DOI] [PMC free article] [PubMed] [Google Scholar]
- Owen D. G., Segal M., Barker J. L. A Ca-dependent Cl- conductance in cultured mouse spinal neurones. Nature. 1984 Oct 11;311(5986):567–570. doi: 10.1038/311567a0. [DOI] [PubMed] [Google Scholar]
- Petersen O. H., Maruyama Y. Calcium-activated potassium channels and their role in secretion. Nature. 1984 Feb 23;307(5953):693–696. doi: 10.1038/307693a0. [DOI] [PubMed] [Google Scholar]
- Trautmann A., Marty A. Activation of Ca-dependent K channels by carbamoylcholine in rat lacrimal glands. Proc Natl Acad Sci U S A. 1984 Jan;81(2):611–615. doi: 10.1073/pnas.81.2.611. [DOI] [PMC free article] [PubMed] [Google Scholar]
- Yellen G. Single Ca2+-activated nonselective cation channels in neuroblastoma. Nature. 1982 Mar 25;296(5855):357–359. doi: 10.1038/296357a0. [DOI] [PubMed] [Google Scholar]
- Young G. P., Young J. D., Deshpande A. K., Goldstein M., Koide S. S., Cohn Z. A. A Ca2+-activated channel from Xenopus laevis oocyte membranes reconstituted into planar bilayers. Proc Natl Acad Sci U S A. 1984 Aug;81(16):5155–5159. doi: 10.1073/pnas.81.16.5155. [DOI] [PMC free article] [PubMed] [Google Scholar]