Abstract
Intracellular Cl activity (aiCl), and intracellular pH (pHi) were measured in isolated sheep cardiac Purkinje fibres using a liquid ion exchanger Cl-selective micro-electrode and a glass recessed-tip, pH-selective micro-electrode. Removal of external Cl (glucuronate substituted) produced a fall in aiCl from about 20 to about 4 mmol/l: the residual level is probably caused by intracellular interference on the Cl-sensitive electrode. Re-exposure of the fibre to increased levels of external Cl produced, in the steady state, increased levels of aiCl. The dependence of steady-state aiCl upon external Cl activity, aoCl, was roughly hyperbolic with 50% recovery occurring at an aoCl of about 9.5 mmol/l. At all levels of external Cl tested, Cl was accumulated to a level much higher than that predicted for passive electrochemical equilibrium. Exposure of a Cl-depleted fibre to various levels of external Cl produced an exponential rise with time in aiCl. The initial rate-of-rise in aiCl was estimated to be a saturating function of aoCl, with a half-maximal effect occurring at an aoCl of about 33 mmol/l. The rate-of-rise was about 10-fold greater than that predicted from constant-field theory using published values for PCl, the Cl permeability coefficient. Steady-state aiCl was essentially insensitive to changes in external HCO3 concentration, [HCO3]o, if these changes were made at a constant external pH, pHo, i.e. when a reduction in [HCO3]o was accompanied by a simultaneous reduction in the partial pressure of CO2, PCO2. In contrast, if PCO2 was maintained constant, then a change in [HCO3]o (thus producing a change in pHo) resulted in an inverse change in aiCl. This change in aiCl was also accompanied by a change in pHi: when aiCl increased, pHi decreased and vice versa. The anion-exchange inhibitor, DIDS (4,4-diisothiocyanato-stilbene disulphonic acid) abolished the effect on aiCl of changes in [HCO3]o and pHo (at constant PCO2). Furthermore DIDS reduced the influence of pHo upon pHi. Both the fall of aiCl in Cl-free solution and the subsequent reuptake of Cl following re-exposure to Cl-containing solution were slowed by a reduction in [HCO3]o (constant pHo, reduced PCO2). Both reuptake and wash-out of Cl were saturating functions of [HCO3]o with half-maximal effect occurring at an [HCO3]o of 1-1.3 mmol/l. The reuptake of Cl was little affected by removal of external Na (bis,2-hydroxy ethyl, dimethyl ammonium substituted). The reuptake of Cl was unaffected by amiloride (1 mmol/l) but slowed by piretanide (1 mmol/l).(ABSTRACT TRUNCATED AT 400 WORDS)
Full text
PDF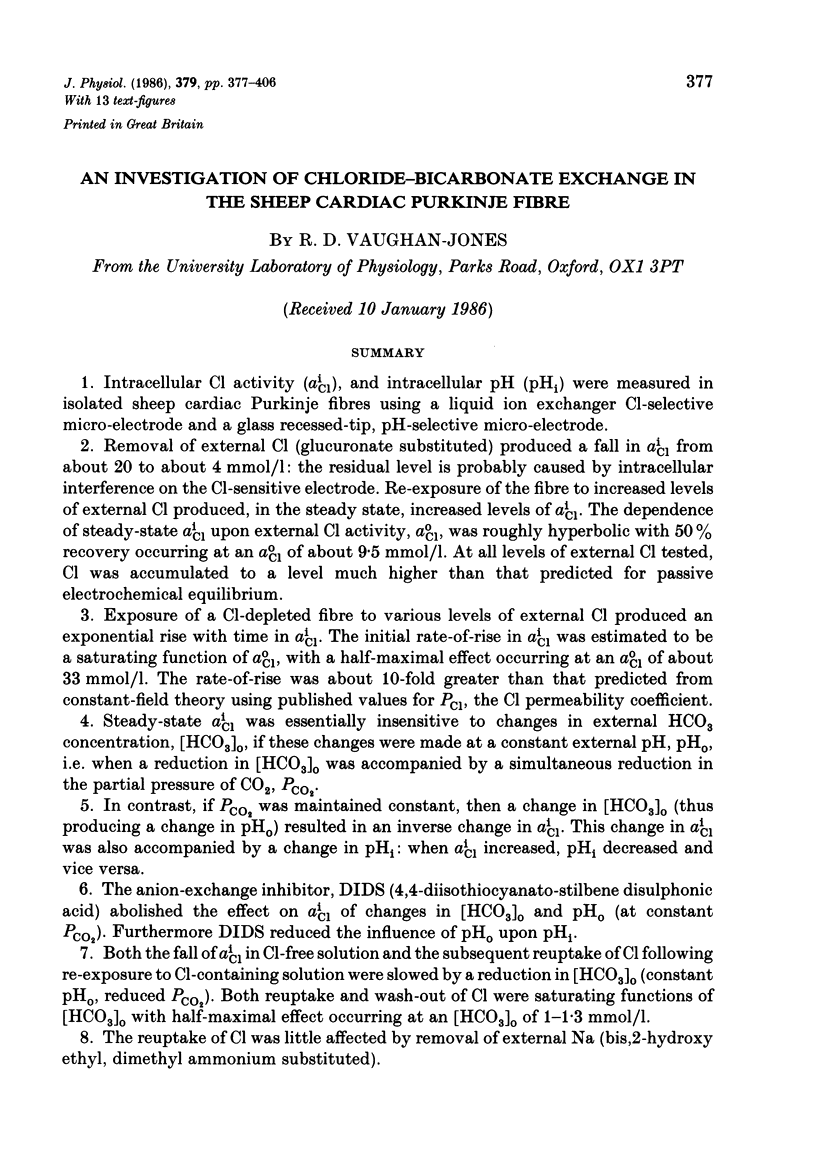
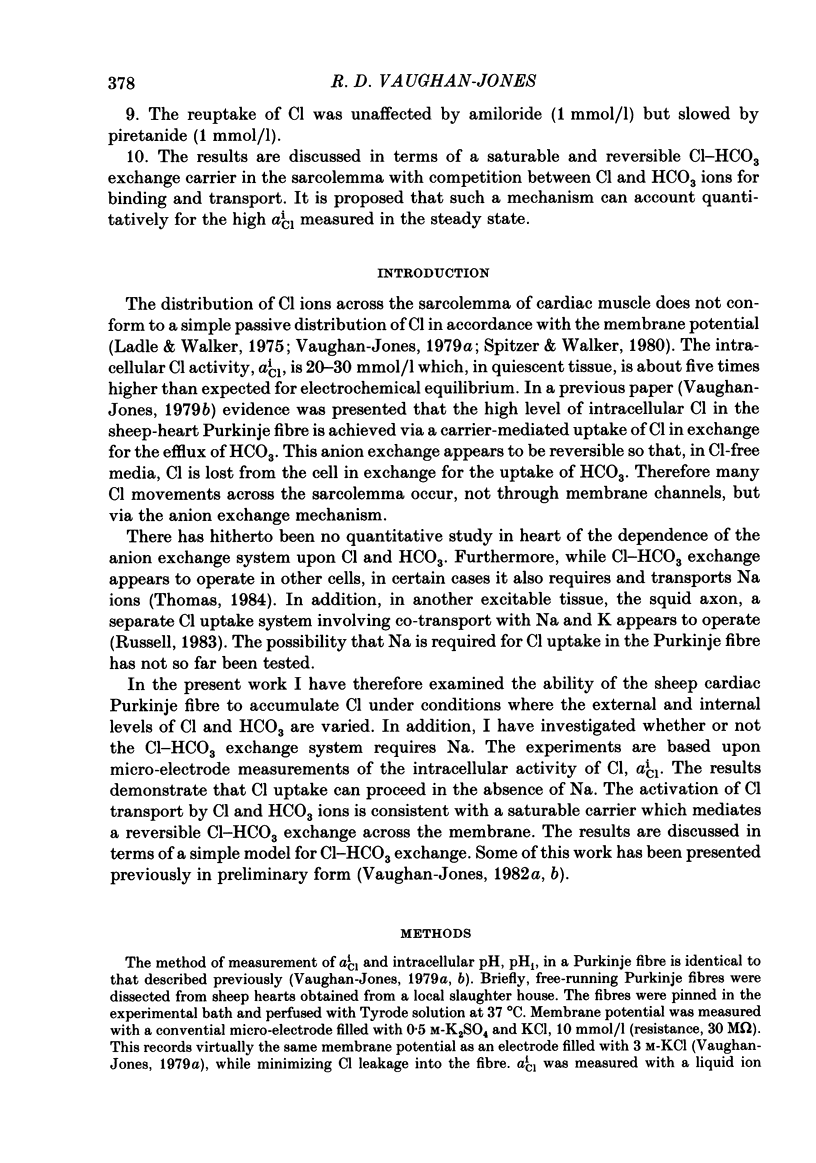
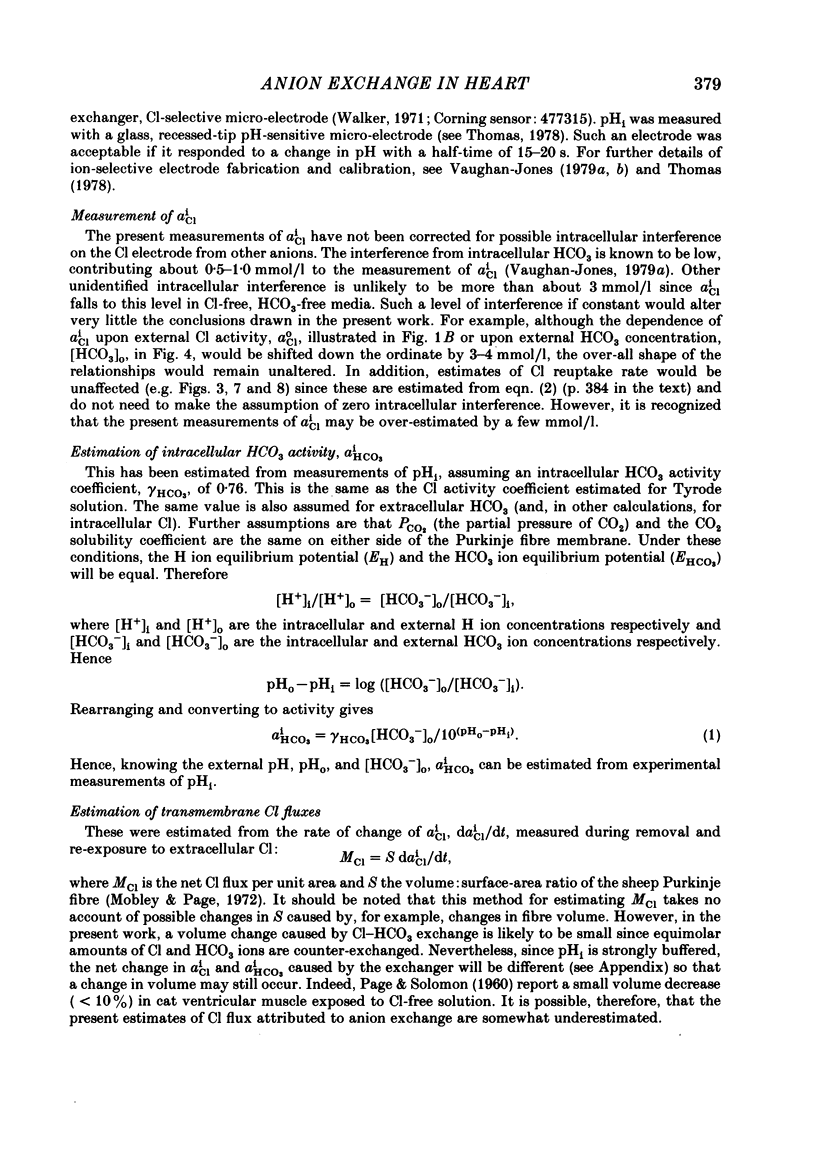
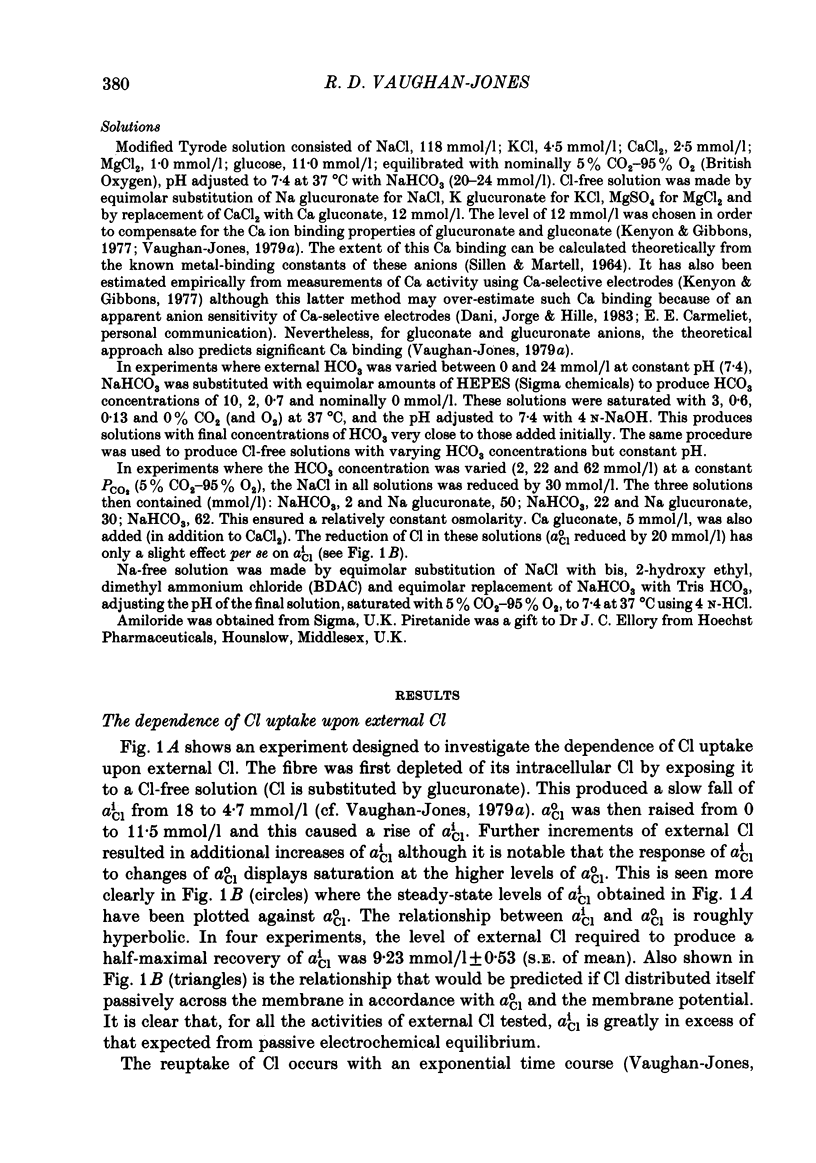
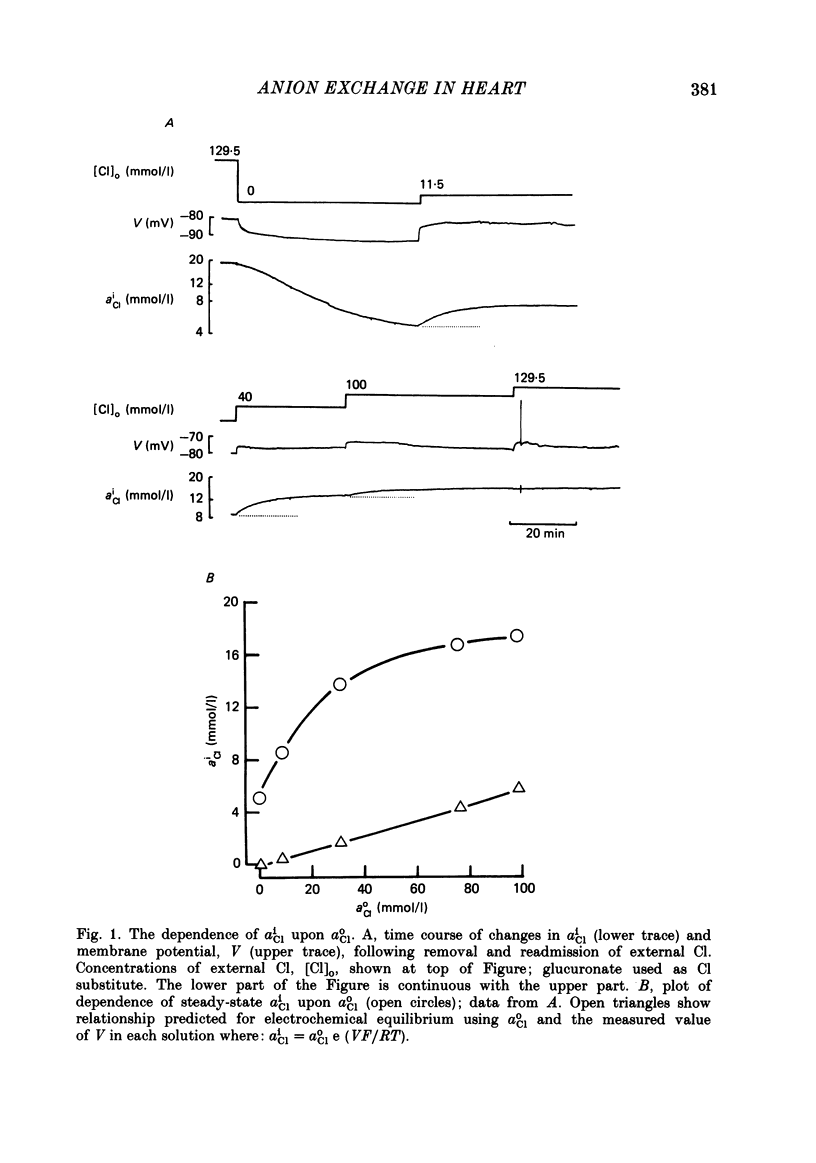
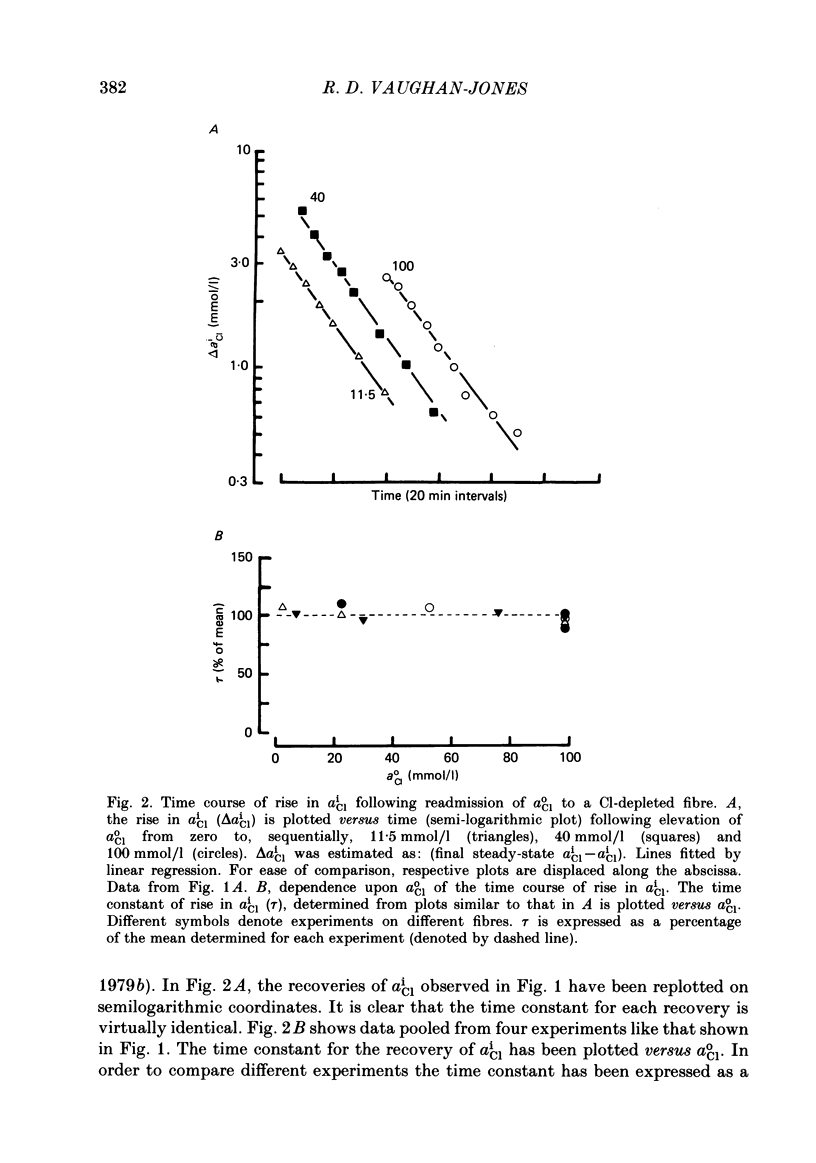
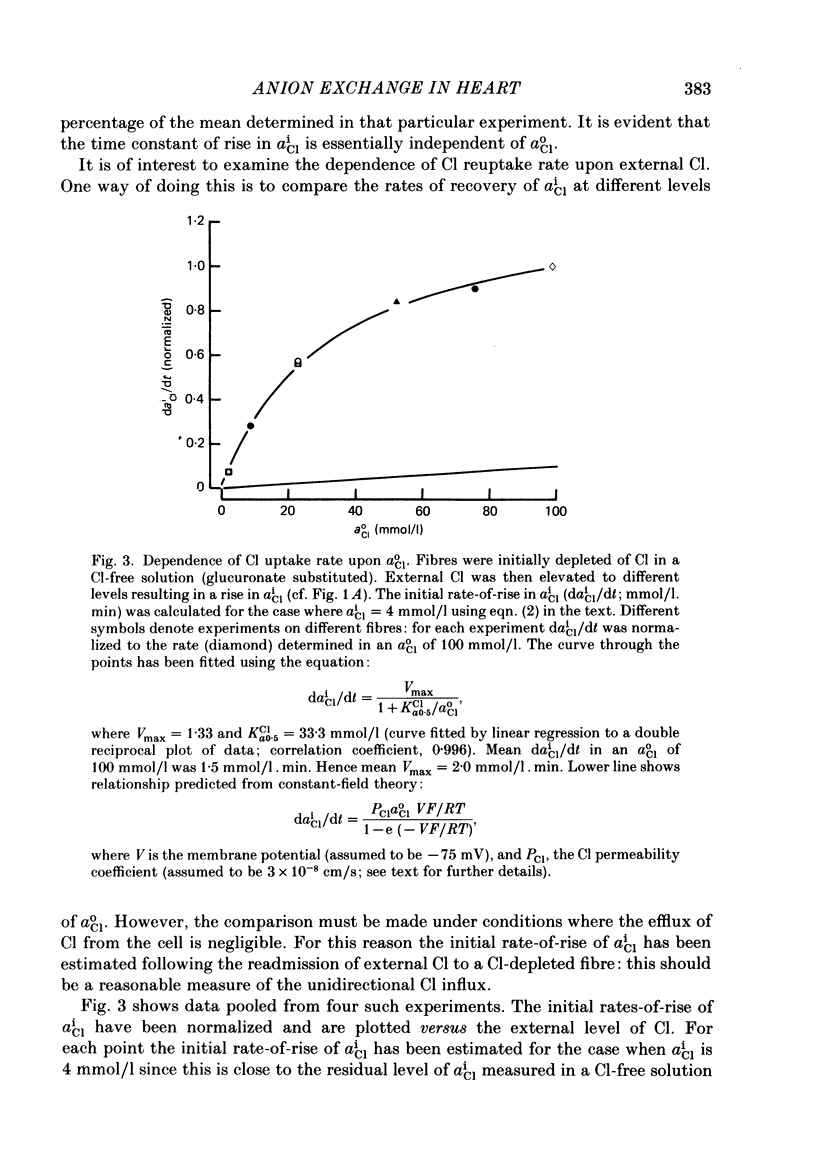
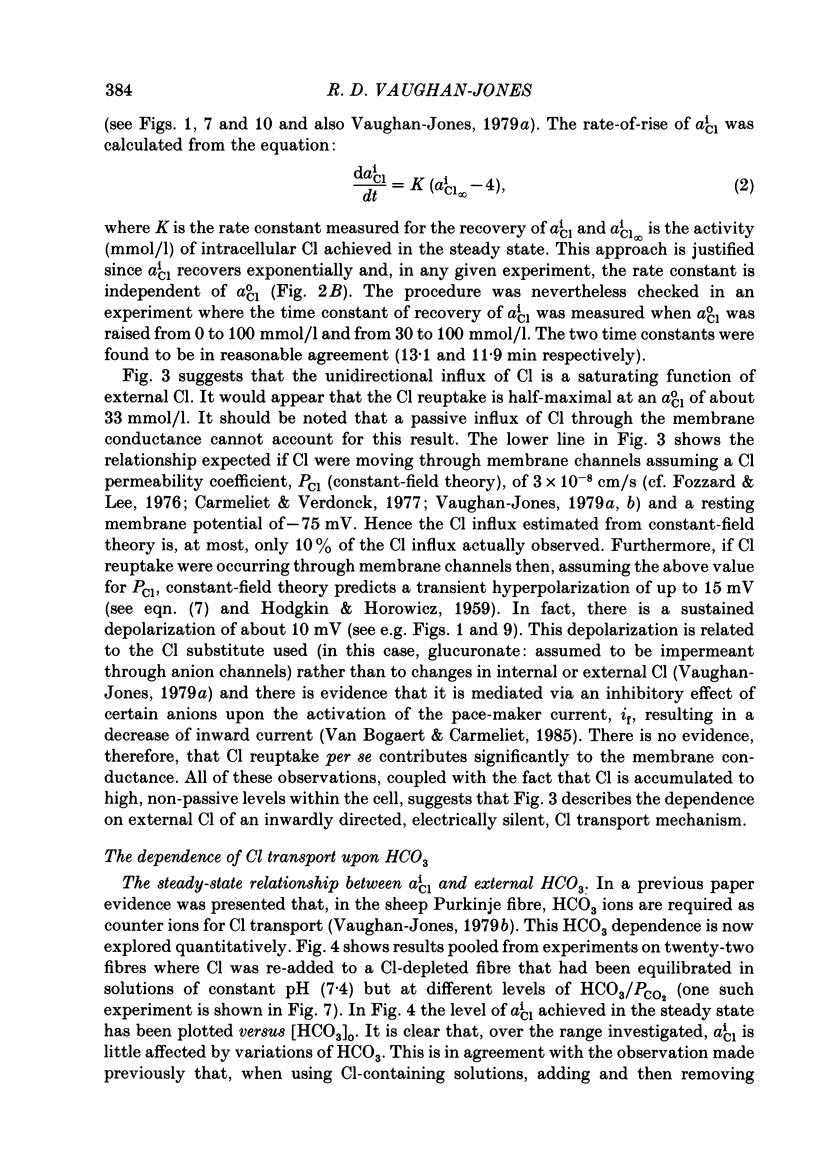
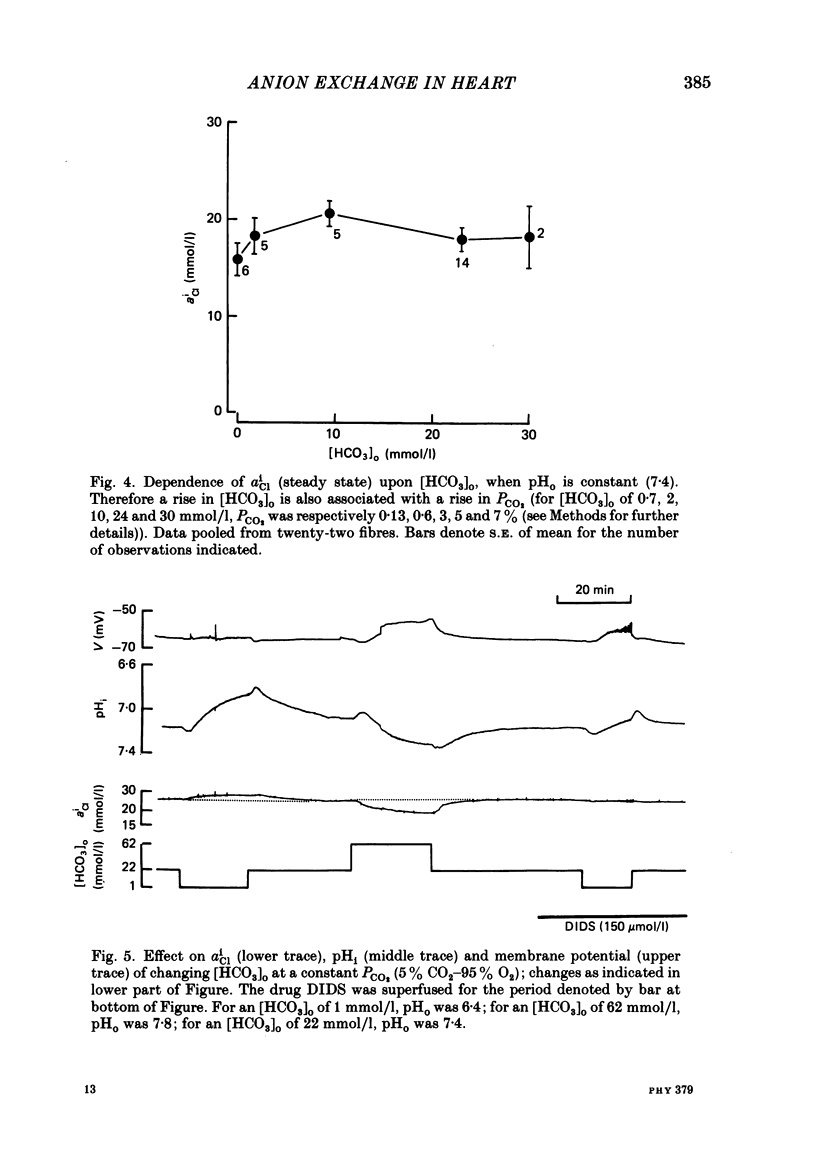
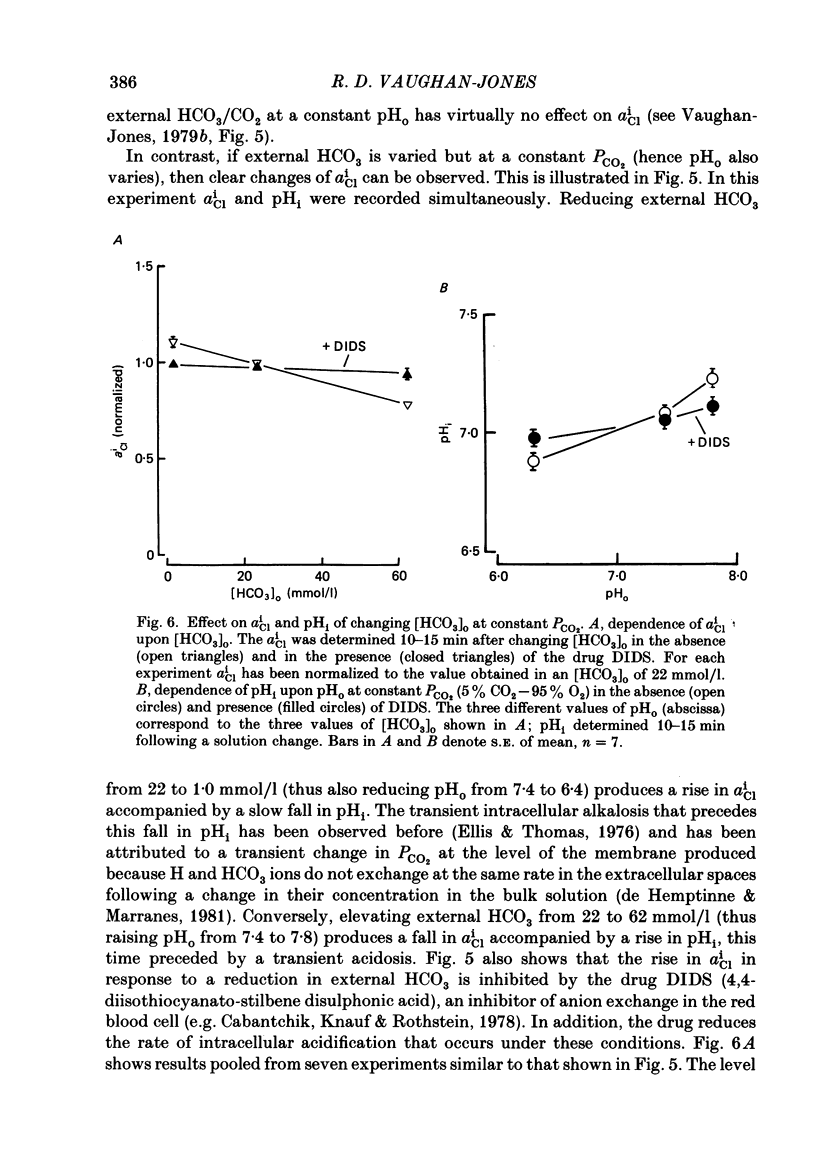
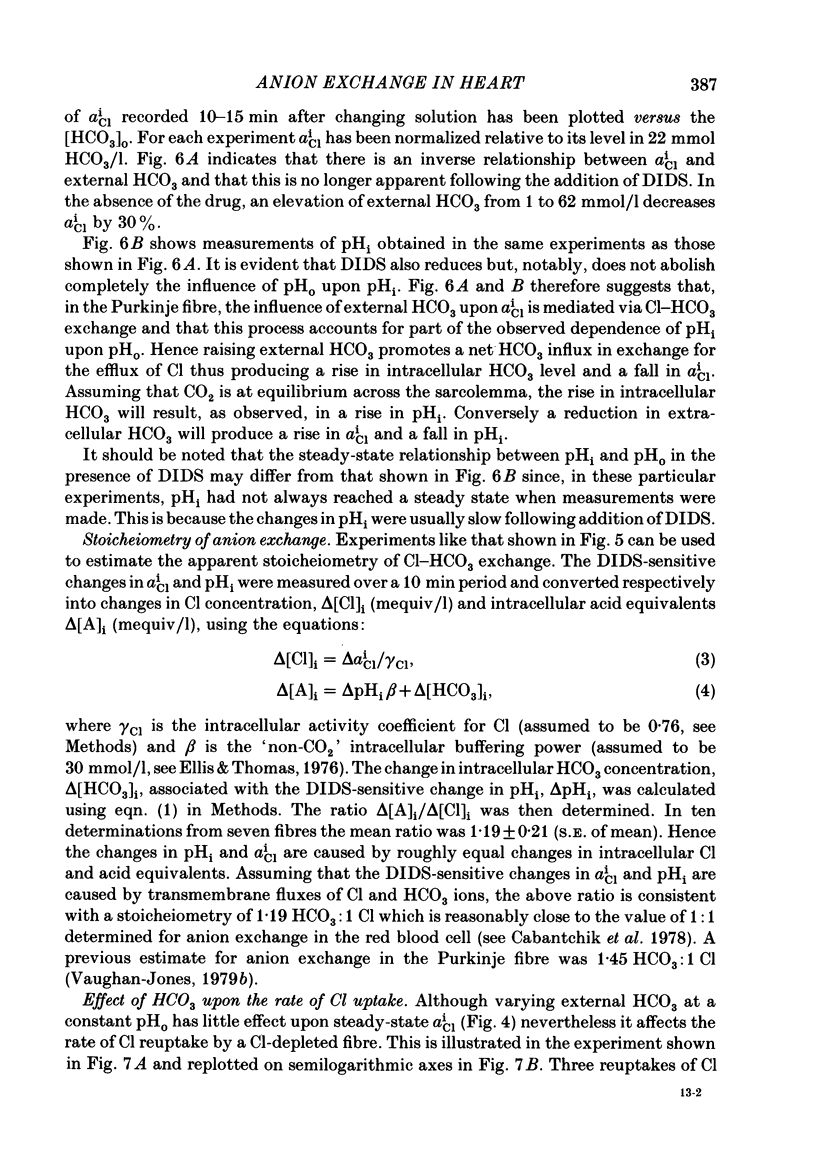
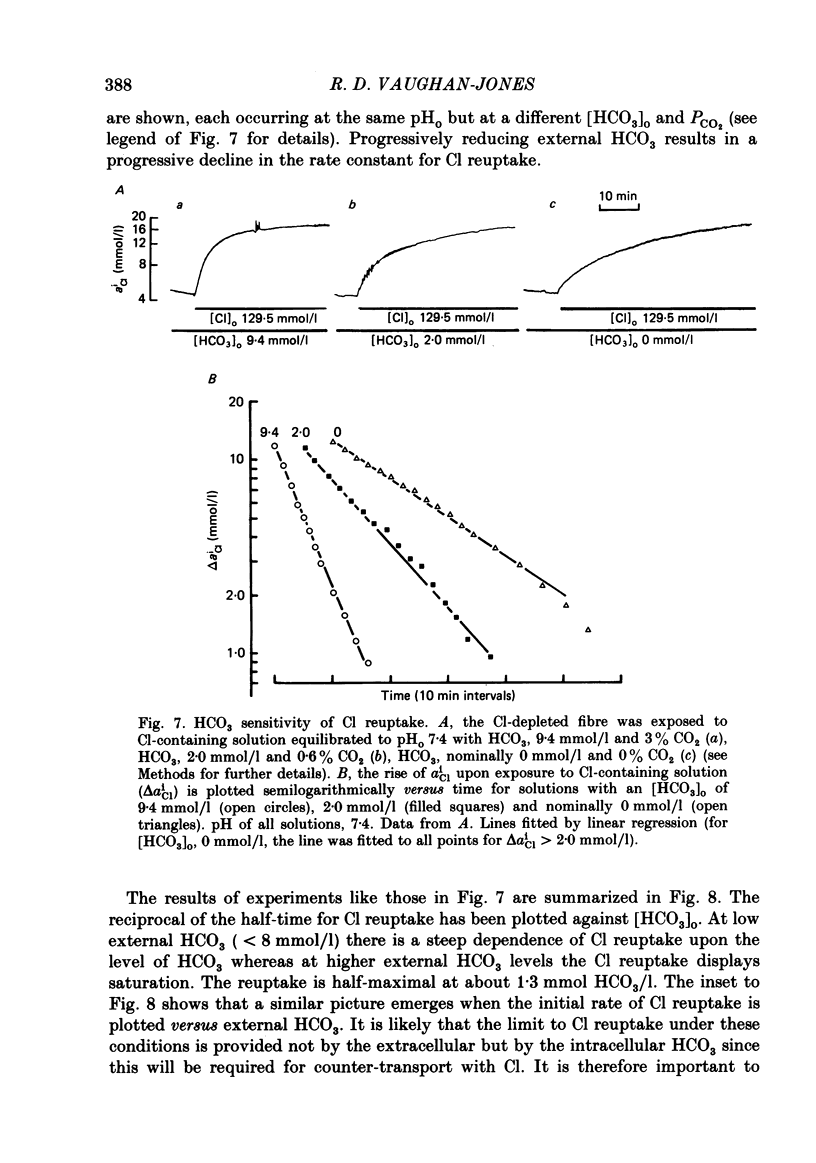
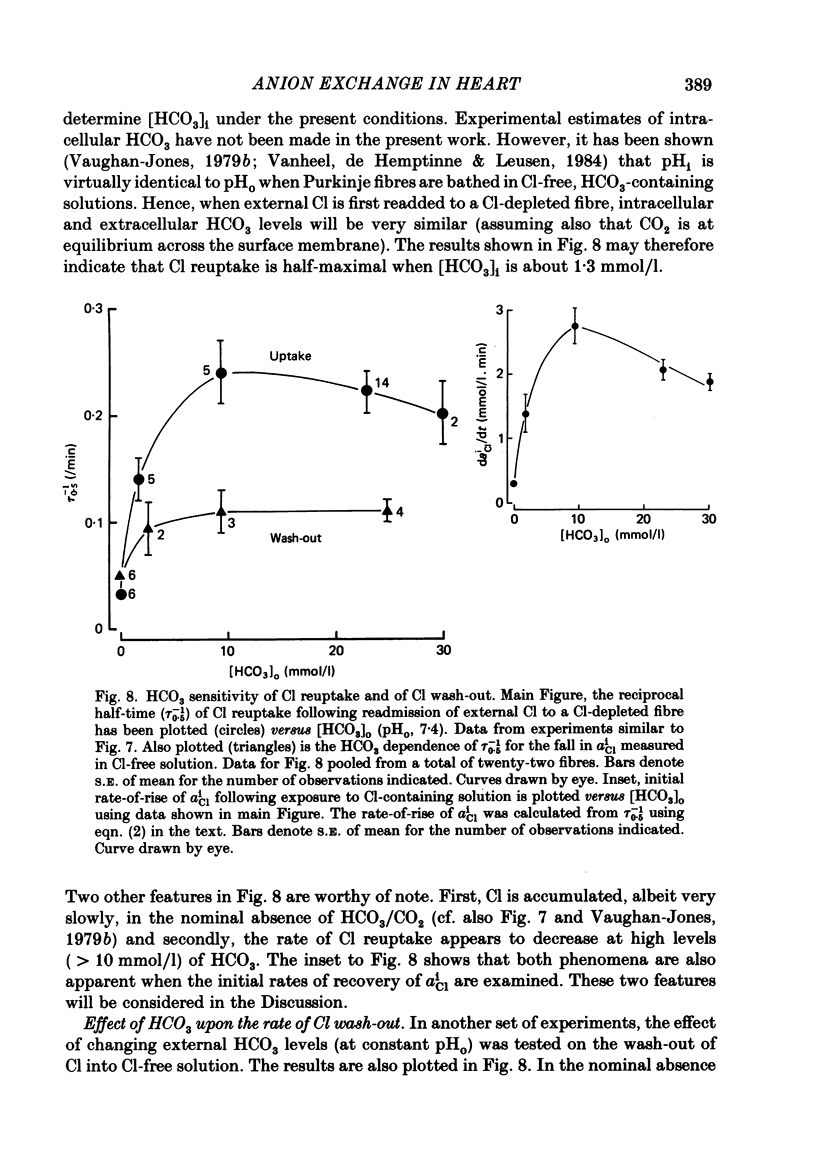
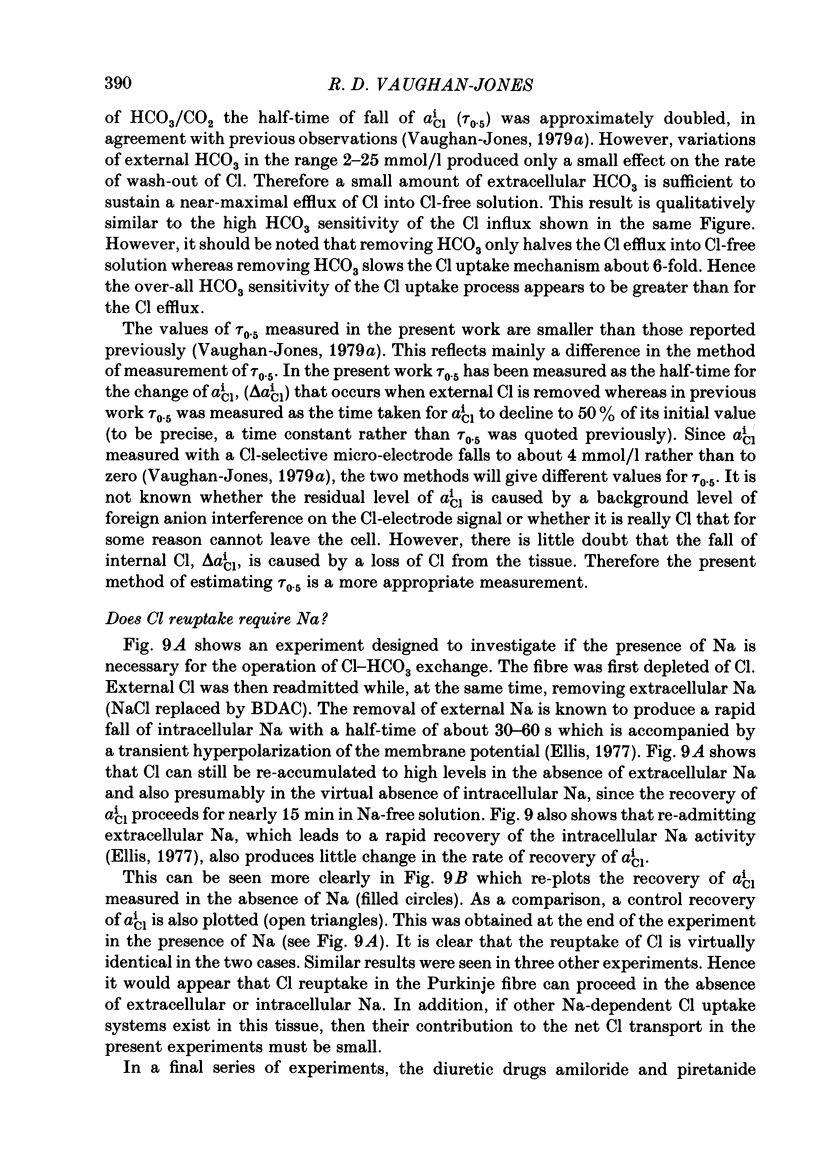
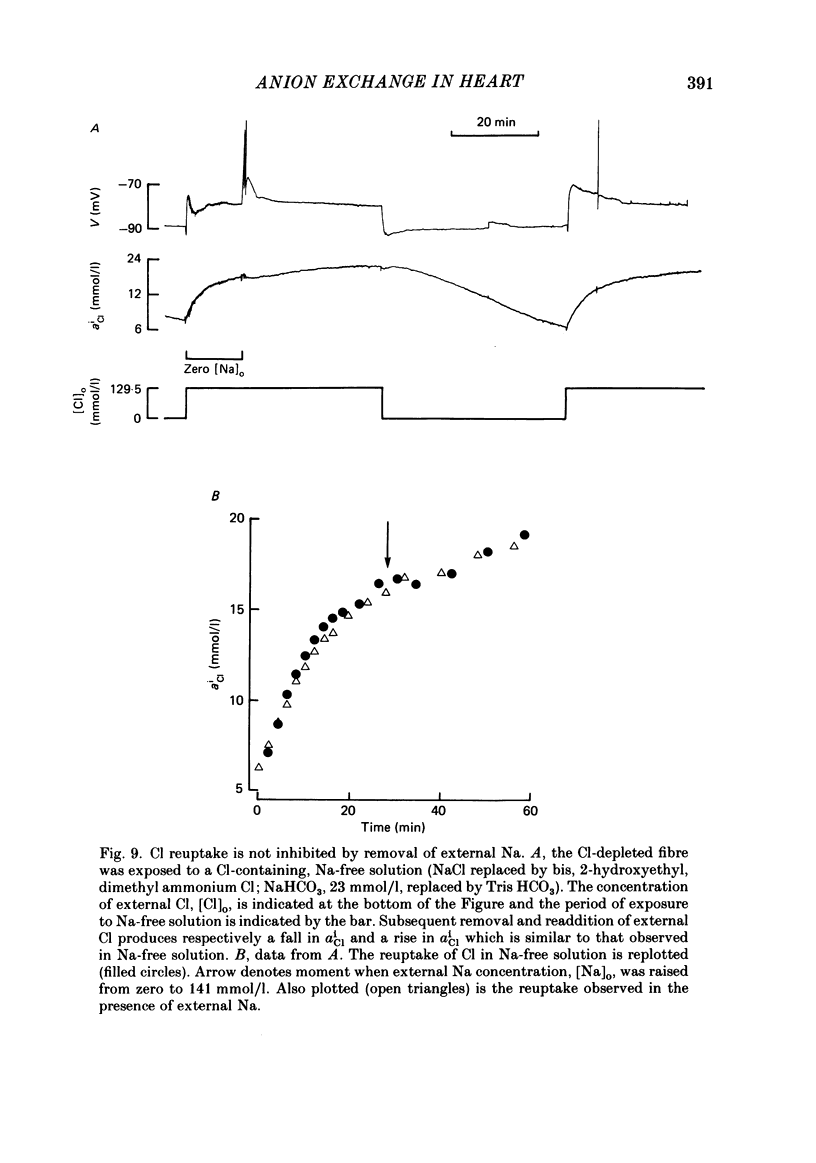
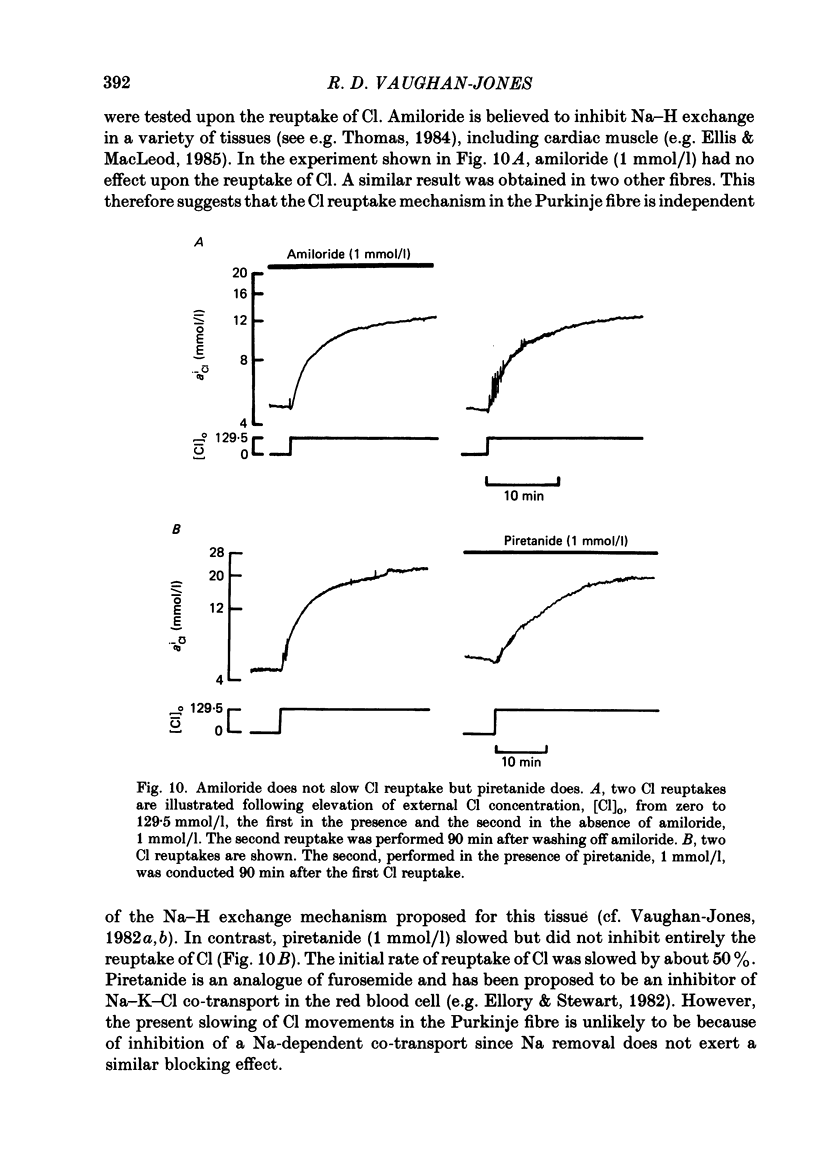
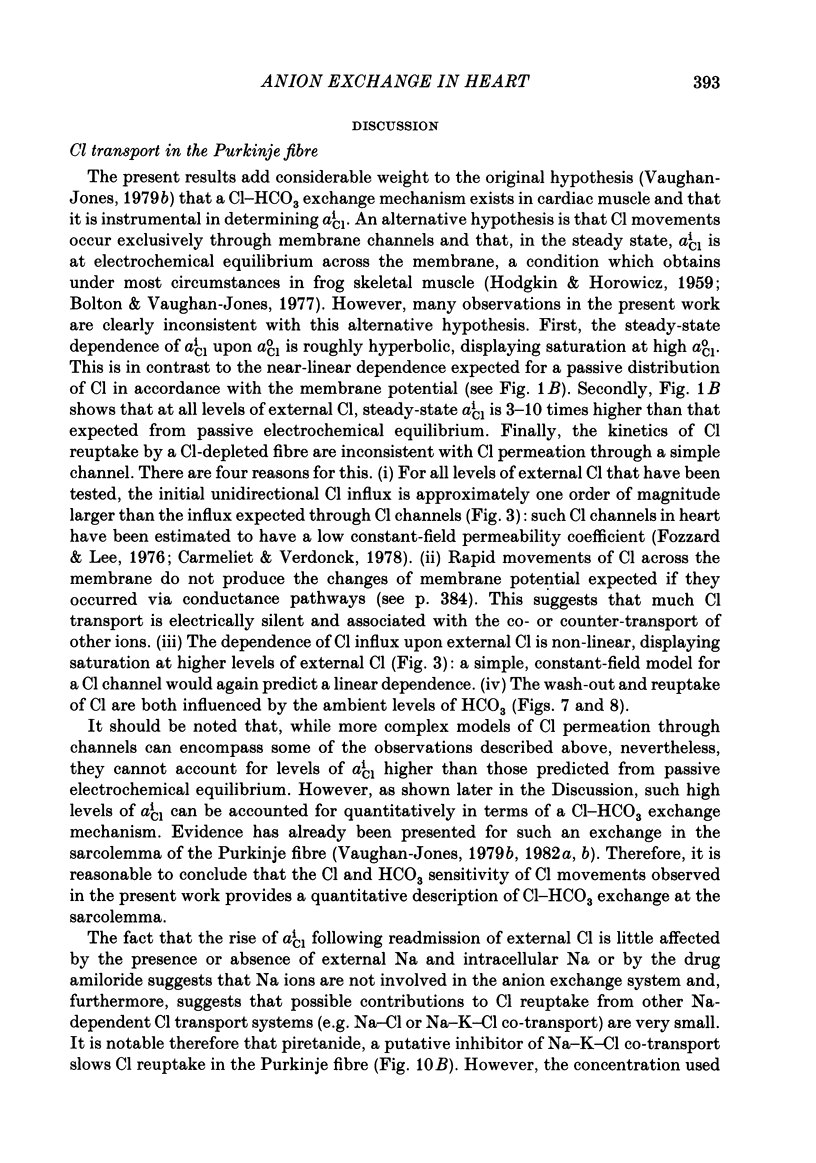
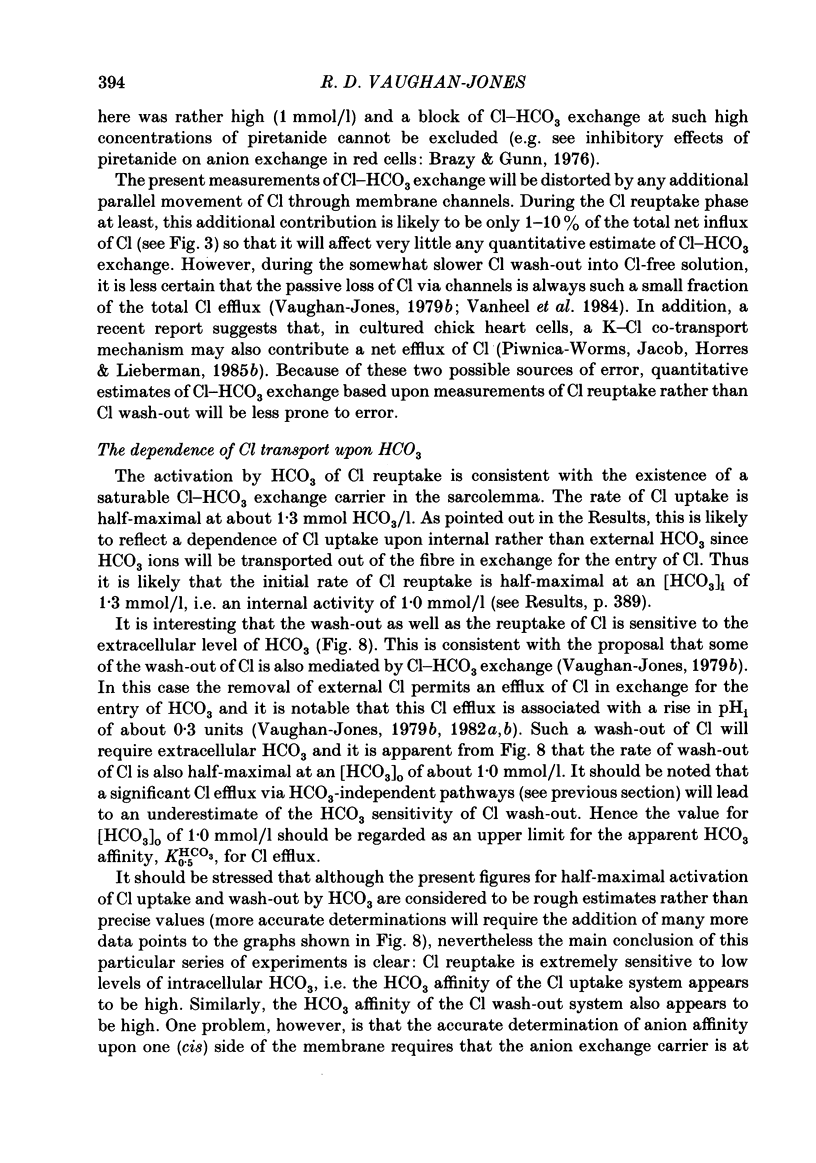
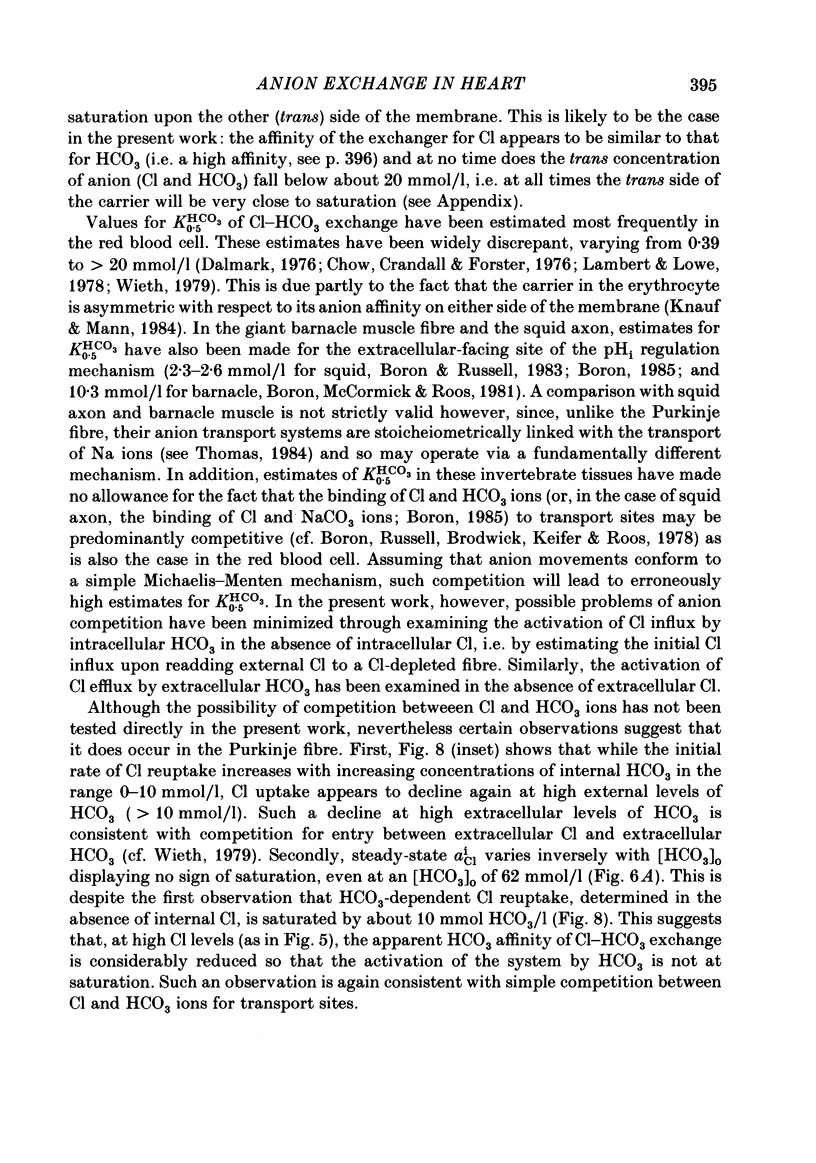
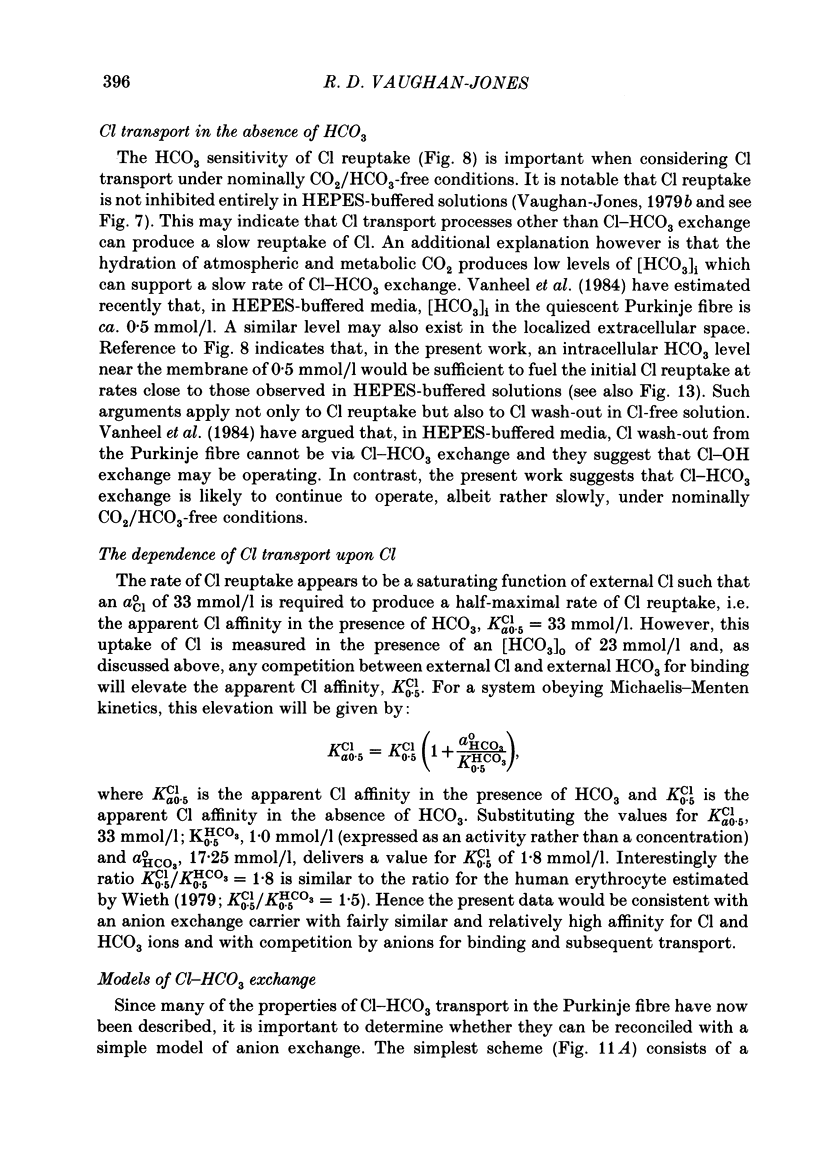
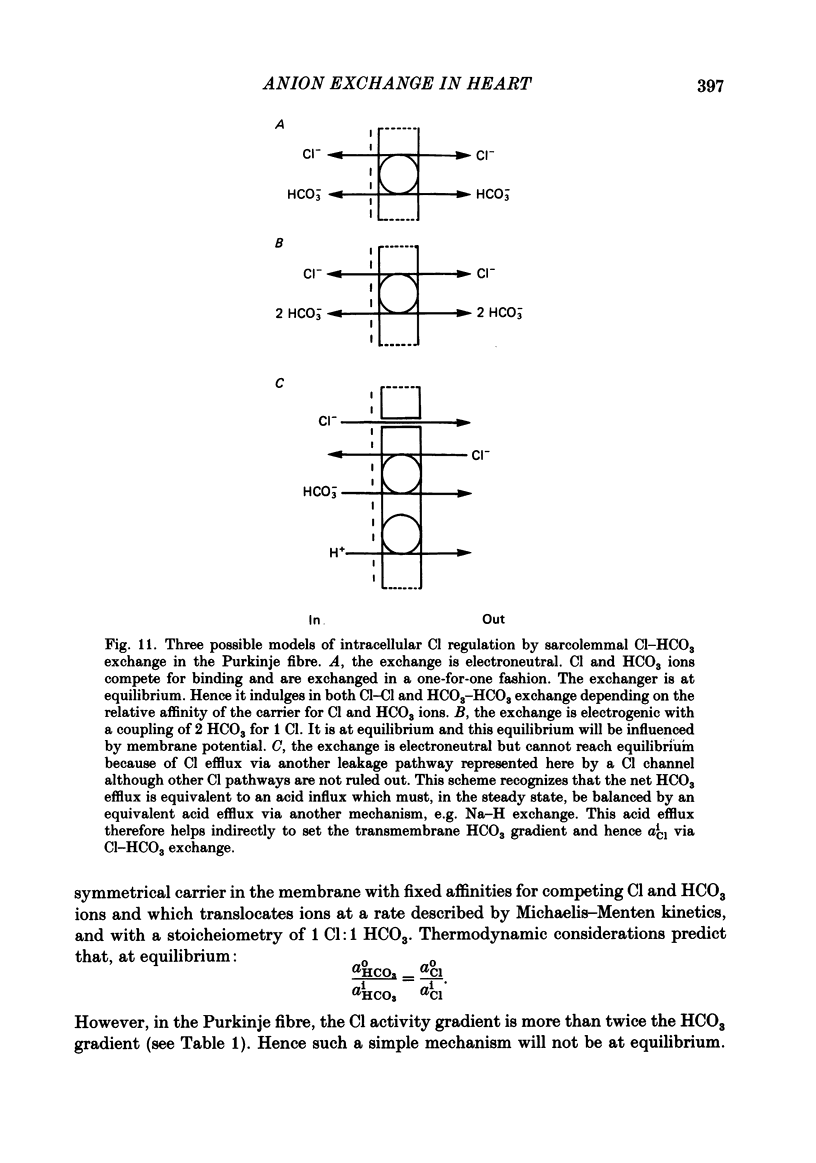
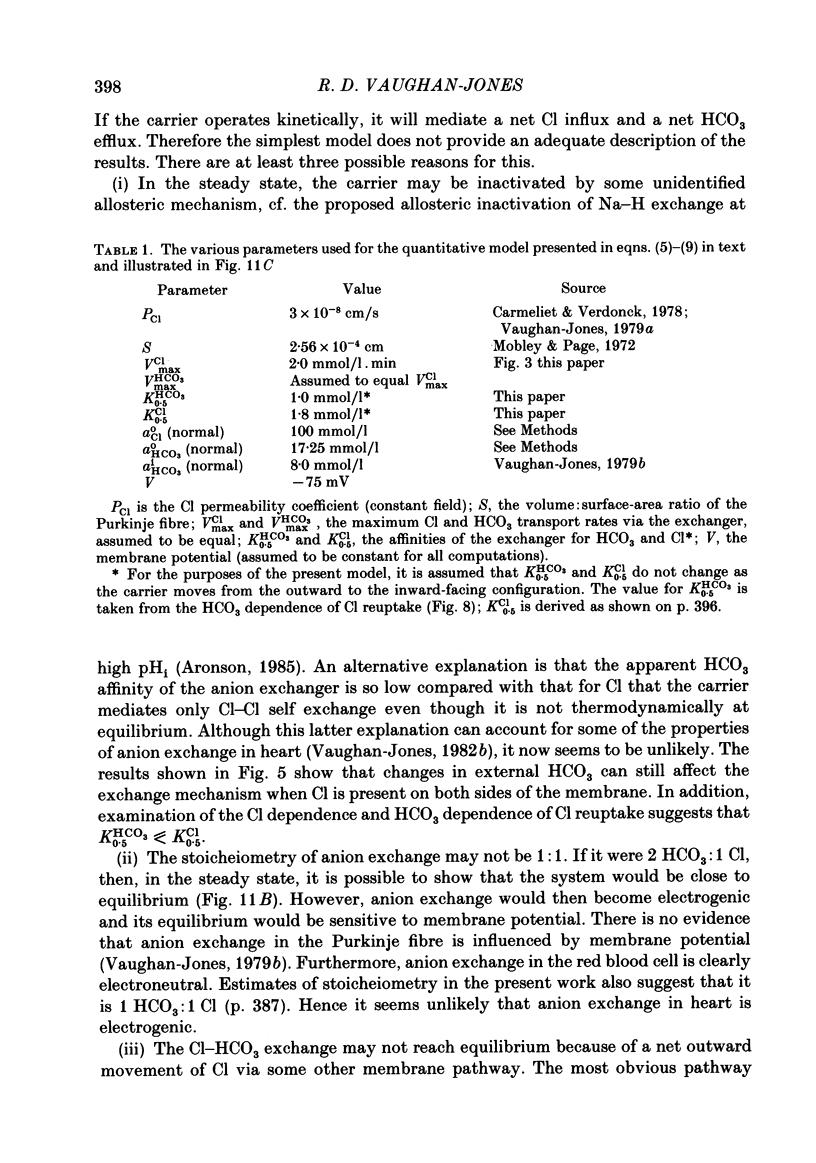
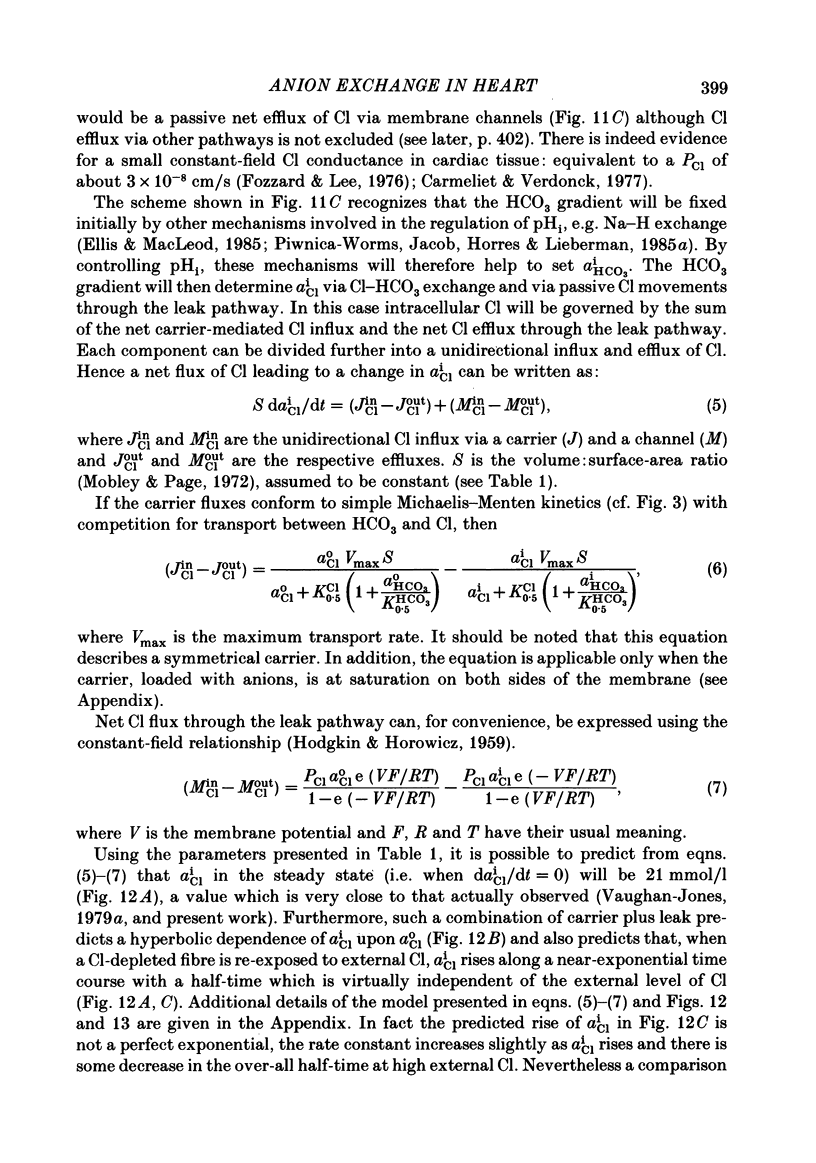
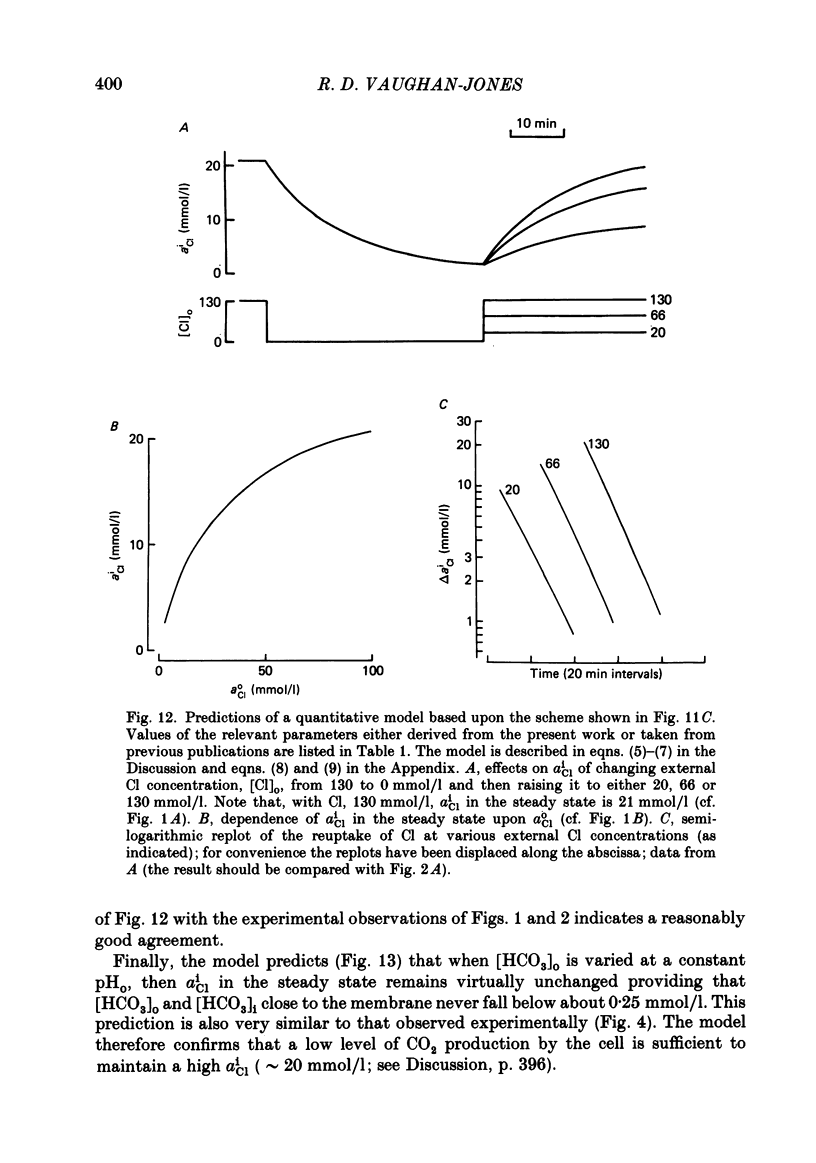
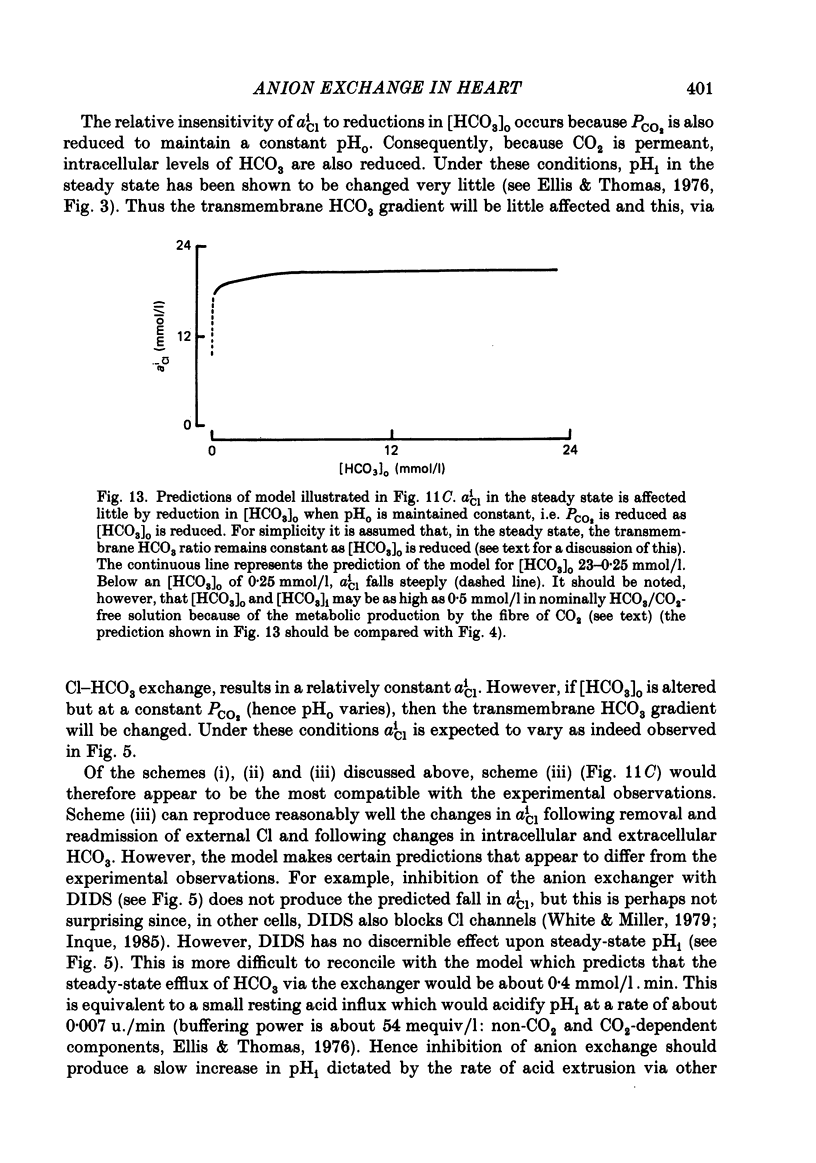
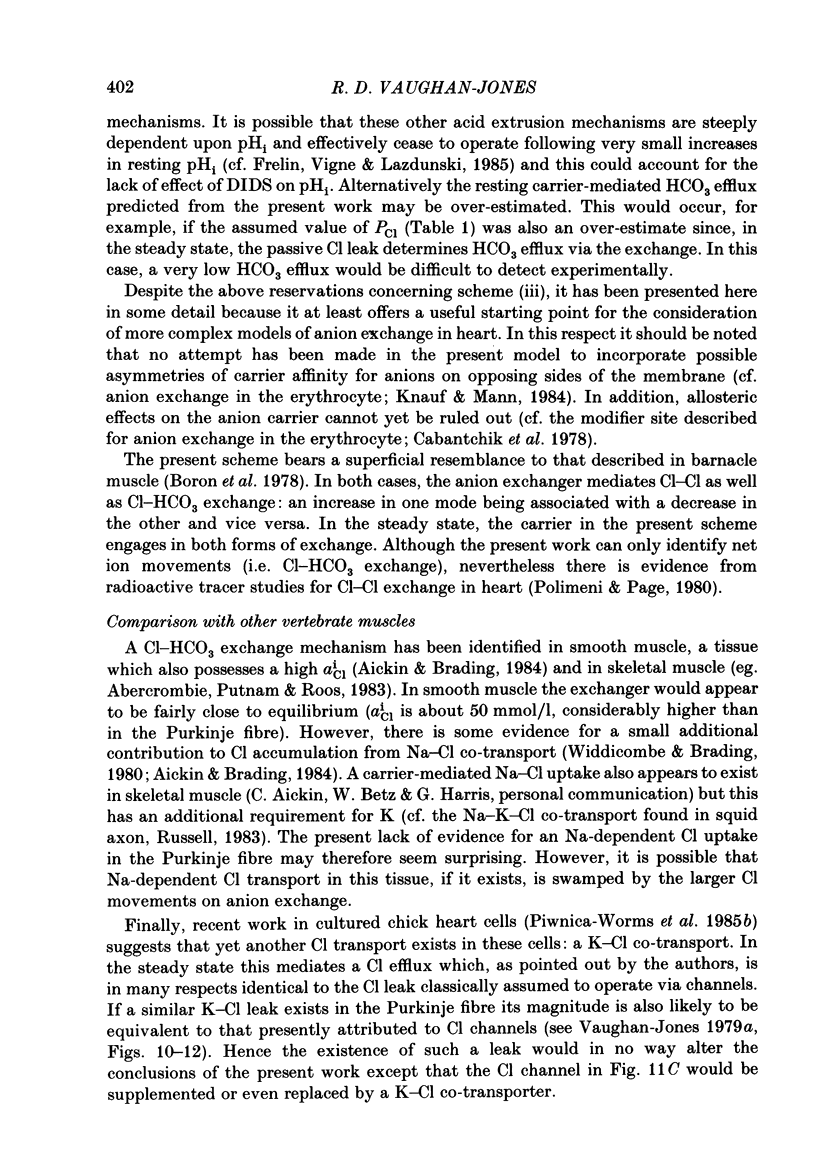
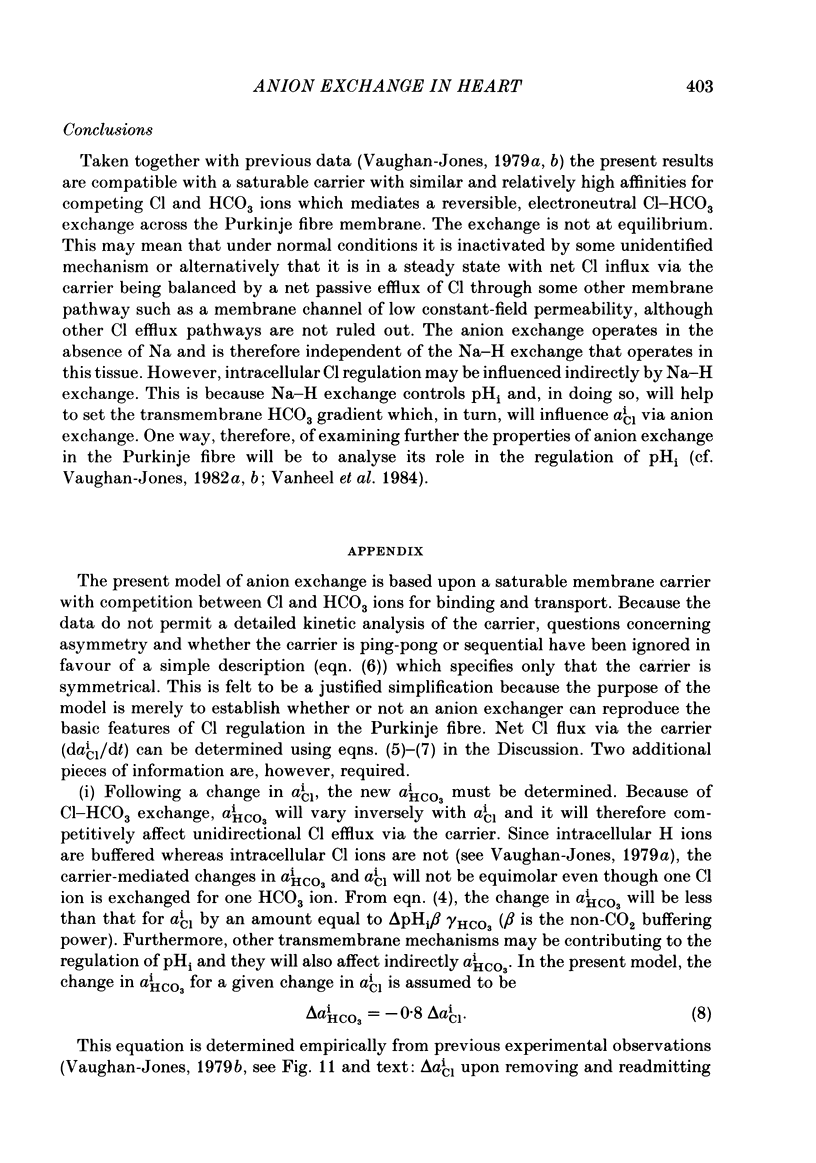
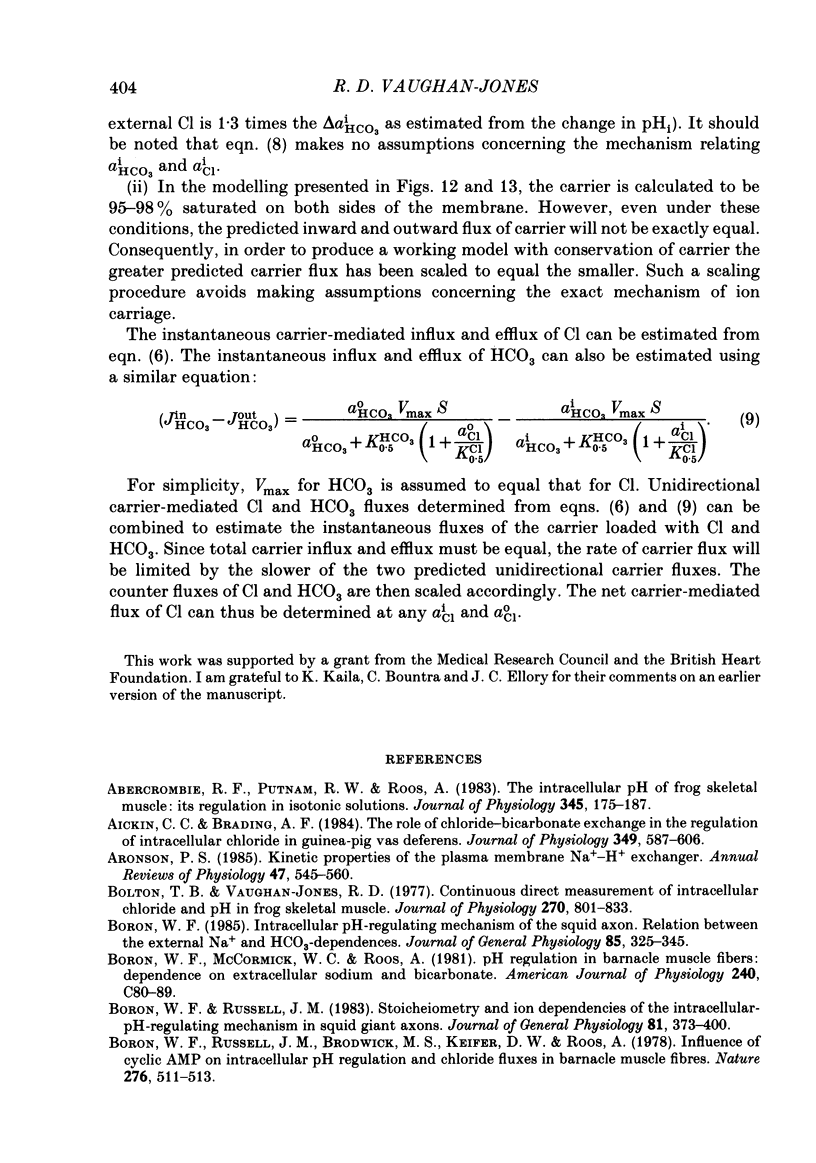
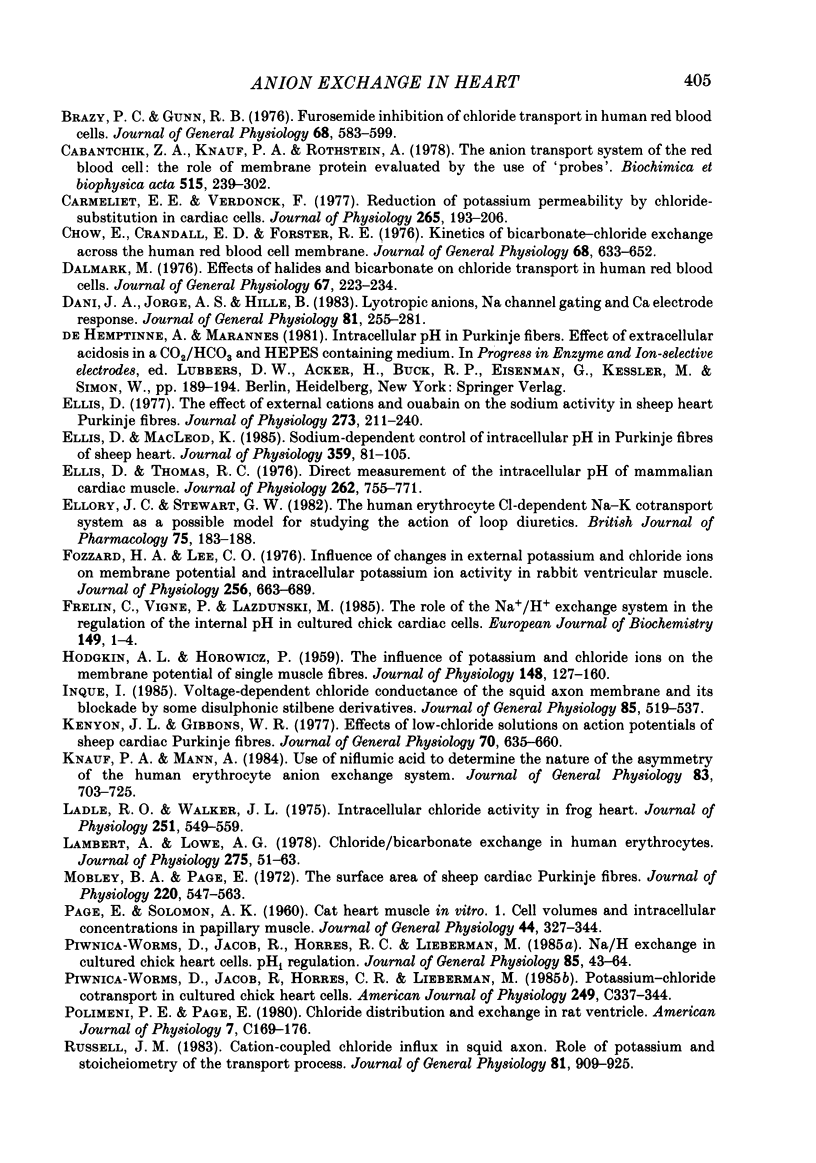
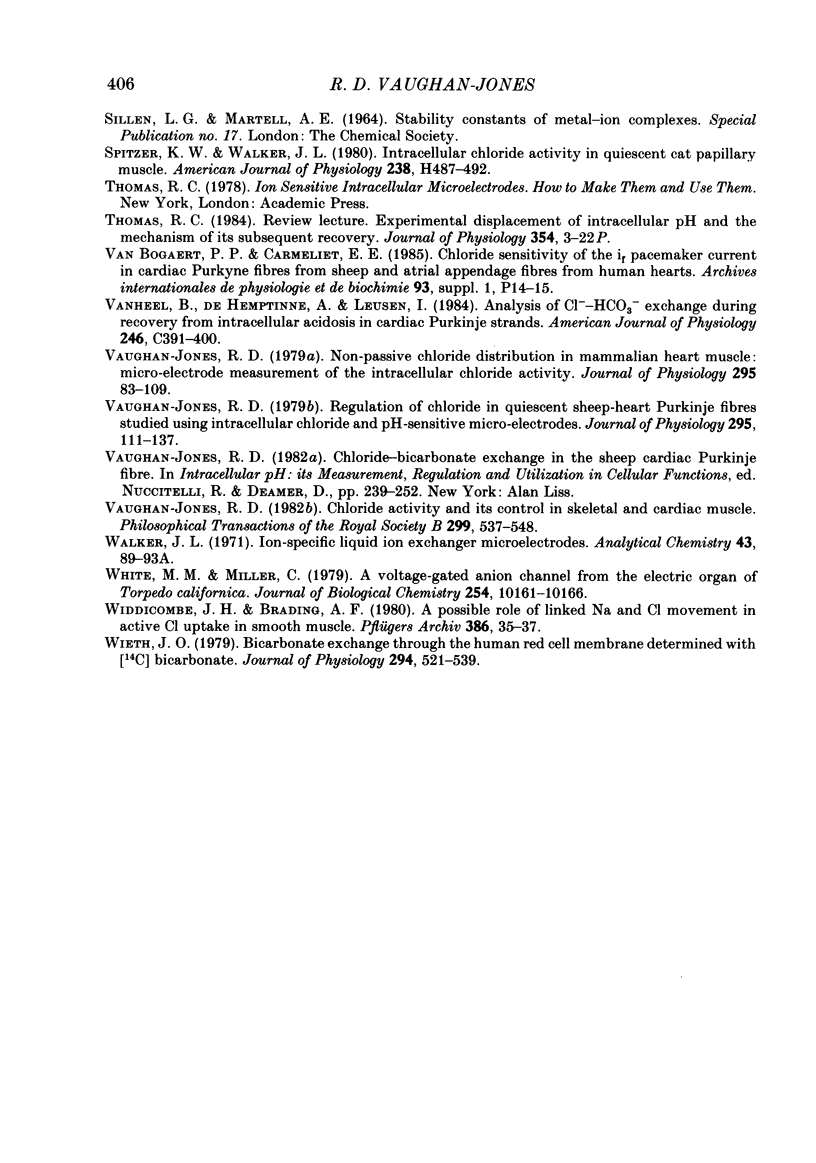
Selected References
These references are in PubMed. This may not be the complete list of references from this article.
- Abercrombie R. F., Putnam R. W., Roos A. The intracellular pH of frog skeletal muscle: its regulation in isotonic solutions. J Physiol. 1983 Dec;345:175–187. doi: 10.1113/jphysiol.1983.sp014973. [DOI] [PMC free article] [PubMed] [Google Scholar]
- Aickin C. C., Brading A. F. The role of chloride-bicarbonate exchange in the regulation of intracellular chloride in guinea-pig vas deferens. J Physiol. 1984 Apr;349:587–606. doi: 10.1113/jphysiol.1984.sp015175. [DOI] [PMC free article] [PubMed] [Google Scholar]
- Aronson P. S. Kinetic properties of the plasma membrane Na+-H+ exchanger. Annu Rev Physiol. 1985;47:545–560. doi: 10.1146/annurev.ph.47.030185.002553. [DOI] [PubMed] [Google Scholar]
- Bolton T. B., Vaughan-Jones R. D. Continuous direct measurement of intracellular chloride and pH in frog skeletal muscle. J Physiol. 1977 Sep;270(3):801–833. doi: 10.1113/jphysiol.1977.sp011983. [DOI] [PMC free article] [PubMed] [Google Scholar]
- Boron W. F. Intracellular pH-regulating mechanism of the squid axon. Relation between the external Na+ and HCO-3 dependences. J Gen Physiol. 1985 Mar;85(3):325–345. doi: 10.1085/jgp.85.3.325. [DOI] [PMC free article] [PubMed] [Google Scholar]
- Boron W. F., Russell J. M., Brodwick M. S., Keifer D. W., Roos A. Influence of cyclic AMP on intracellular pH regulation and chloride fluxes in barnacle muscle fibers. Nature. 1978 Nov 30;276(5687):511–513. doi: 10.1038/276511a0. [DOI] [PubMed] [Google Scholar]
- Boron W. F., Russell J. M. Stoichiometry and ion dependencies of the intracellular-pH-regulating mechanism in squid giant axons. J Gen Physiol. 1983 Mar;81(3):373–399. doi: 10.1085/jgp.81.3.373. [DOI] [PMC free article] [PubMed] [Google Scholar]
- Brazy P. C., Gunn R. B. Furosemide inhibition of chloride transport in human red blood cells. J Gen Physiol. 1976 Dec;68(6):583–599. doi: 10.1085/jgp.68.6.583. [DOI] [PMC free article] [PubMed] [Google Scholar]
- Cabantchik Z. I., Knauf P. A., Rothstein A. The anion transport system of the red blood cell. The role of membrane protein evaluated by the use of 'probes'. Biochim Biophys Acta. 1978 Sep 29;515(3):239–302. doi: 10.1016/0304-4157(78)90016-3. [DOI] [PubMed] [Google Scholar]
- Carmeliet E., Verdonck F. Reduction of potassium permeability by chloride substitution in cardiac cells. J Physiol. 1977 Feb;265(1):193–206. doi: 10.1113/jphysiol.1977.sp011712. [DOI] [PMC free article] [PubMed] [Google Scholar]
- Chow E. I., Crandall E. D., Forster R. E. Kinetics of bicarbonate-chloride exchange across the human red blood cell membrane. J Gen Physiol. 1976 Dec;68(6):633–652. doi: 10.1085/jgp.68.6.633. [DOI] [PMC free article] [PubMed] [Google Scholar]
- Dalmark M. Effects of halides and bicarbonate on chloride transport in human red blood cells. J Gen Physiol. 1976 Feb;67(2):223–234. doi: 10.1085/jgp.67.2.223. [DOI] [PMC free article] [PubMed] [Google Scholar]
- Dani J. A., Sanchez J. A., Hille B. Lyotropic anions. Na channel gating and Ca electrode response. J Gen Physiol. 1983 Feb;81(2):255–281. doi: 10.1085/jgp.81.2.255. [DOI] [PMC free article] [PubMed] [Google Scholar]
- Ellis D., MacLeod K. T. Sodium-dependent control of intracellular pH in Purkinje fibres of sheep heart. J Physiol. 1985 Feb;359:81–105. doi: 10.1113/jphysiol.1985.sp015576. [DOI] [PMC free article] [PubMed] [Google Scholar]
- Ellis D. The effects of external cations and ouabain on the intracellular sodium activity of sheep heart Purkinje fibres. J Physiol. 1977 Dec;273(1):211–240. doi: 10.1113/jphysiol.1977.sp012090. [DOI] [PMC free article] [PubMed] [Google Scholar]
- Ellis D., Thomas R. C. Direct measurement of the intracellular pH of mammalian cardiac muscle. J Physiol. 1976 Nov;262(3):755–771. doi: 10.1113/jphysiol.1976.sp011619. [DOI] [PMC free article] [PubMed] [Google Scholar]
- Ellory J. C., Stewart G. W. The human erythrocyte Cl-dependent Na-K cotransport system as a possible model for studying the action of loop diuretics. Br J Pharmacol. 1982 Jan;75(1):183–188. doi: 10.1111/j.1476-5381.1982.tb08771.x. [DOI] [PMC free article] [PubMed] [Google Scholar]
- Fozzard H. A., Lee C. O. Influence of changes in external potassium and chloride ions on membrane potential and intracellular potassium ion activity in rabbit ventricular muscle. J Physiol. 1976 Apr;256(3):663–689. doi: 10.1113/jphysiol.1976.sp011345. [DOI] [PMC free article] [PubMed] [Google Scholar]
- Frelin C., Vigne P., Lazdunski M. The role of the Na+/H+ exchange system in the regulation of the internal pH in cultured cardiac cells. Eur J Biochem. 1985 May 15;149(1):1–4. doi: 10.1111/j.1432-1033.1985.tb08884.x. [DOI] [PubMed] [Google Scholar]
- HODGKIN A. L., HOROWICZ P. The influence of potassium and chloride ions on the membrane potential of single muscle fibres. J Physiol. 1959 Oct;148:127–160. doi: 10.1113/jphysiol.1959.sp006278. [DOI] [PMC free article] [PubMed] [Google Scholar]
- Inoue I. Voltage-dependent chloride conductance of the squid axon membrane and its blockade by some disulfonic stilbene derivatives. J Gen Physiol. 1985 Apr;85(4):519–537. doi: 10.1085/jgp.85.4.519. [DOI] [PMC free article] [PubMed] [Google Scholar]
- Kenyon J. L., Gibbons W. R. Effects of low-chloride solutions on action potentials of sheep cardiac Purkinje fibers. J Gen Physiol. 1977 Nov;70(5):635–660. doi: 10.1085/jgp.70.5.635. [DOI] [PMC free article] [PubMed] [Google Scholar]
- Knauf P. A., Mann N. A. Use of niflumic acid to determine the nature of the asymmetry of the human erythrocyte anion exchange system. J Gen Physiol. 1984 May;83(5):703–725. doi: 10.1085/jgp.83.5.703. [DOI] [PMC free article] [PubMed] [Google Scholar]
- Ladle R. O., Walker J. L. Intracellular chloride activity in frog heart. J Physiol. 1975 Oct;251(2):549–559. doi: 10.1113/jphysiol.1975.sp011107. [DOI] [PMC free article] [PubMed] [Google Scholar]
- Lambert A., Lowe A. G. Chloride/bicarbonate exchange in human erythrocytes. J Physiol. 1978 Feb;275:51–63. doi: 10.1113/jphysiol.1978.sp012177. [DOI] [PMC free article] [PubMed] [Google Scholar]
- Mobley B. A., Page E. The surface area of sheep cardiac Purkinje fibres. J Physiol. 1972 Feb;220(3):547–563. doi: 10.1113/jphysiol.1972.sp009722. [DOI] [PMC free article] [PubMed] [Google Scholar]
- PAGE E., SOLOMON A. K. Cat heart muscle in vitro. I. Cell volumes and intracellular concentrations in papillary muscle. J Gen Physiol. 1960 Nov;44:327–344. doi: 10.1085/jgp.44.2.327. [DOI] [PMC free article] [PubMed] [Google Scholar]
- Piwnica-Worms D., Jacob R., Horres C. R., Lieberman M. Na/H exchange in cultured chick heart cells. pHi regulation. J Gen Physiol. 1985 Jan;85(1):43–64. doi: 10.1085/jgp.85.1.43. [DOI] [PMC free article] [PubMed] [Google Scholar]
- Piwnica-Worms D., Jacob R., Horres C. R., Lieberman M. Potassium-chloride cotransport in cultured chick heart cells. Am J Physiol. 1985 Sep;249(3 Pt 1):C337–C344. doi: 10.1152/ajpcell.1985.249.3.C337. [DOI] [PubMed] [Google Scholar]
- Polimeni P. I., Page E. Chloride distribution and exchange in rat ventricle. Am J Physiol. 1980 May;238(5):C169–C176. doi: 10.1152/ajpcell.1980.238.5.C169. [DOI] [PubMed] [Google Scholar]
- Russell J. M. Cation-coupled chloride influx in squid axon. Role of potassium and stoichiometry of the transport process. J Gen Physiol. 1983 Jun;81(6):909–925. doi: 10.1085/jgp.81.6.909. [DOI] [PMC free article] [PubMed] [Google Scholar]
- Spitzer K. W., Walker J. L. Intracellular chloride activity in quiescent cat papillary muscle. Am J Physiol. 1980 Apr;238(4):H487–H493. doi: 10.1152/ajpheart.1980.238.4.H487. [DOI] [PubMed] [Google Scholar]
- Thomas R. C. Experimental displacement of intracellular pH and the mechanism of its subsequent recovery. J Physiol. 1984 Sep;354:3P–22P. doi: 10.1113/jphysiol.1984.sp015397. [DOI] [PMC free article] [PubMed] [Google Scholar]
- Vanheel B., de Hemptinne A., Leusen I. Analysis of Cl- -HCO3(-) exchange during recovery from intracellular acidosis in cardiac Purkinje strands. Am J Physiol. 1984 May;246(5 Pt 1):C391–C400. doi: 10.1152/ajpcell.1984.246.5.C391. [DOI] [PubMed] [Google Scholar]
- Vaughan-Jones R. D. Chloride activity and its control in skeletal and cardiac muscle. Philos Trans R Soc Lond B Biol Sci. 1982 Dec 1;299(1097):537–548. doi: 10.1098/rstb.1982.0150. [DOI] [PubMed] [Google Scholar]
- Vaughan-Jones R. D. Non-passive chloride distribution in mammalian heart muscle: micro-electrode measurement of the intracellular chloride activity. J Physiol. 1979 Oct;295:83–109. doi: 10.1113/jphysiol.1979.sp012956. [DOI] [PMC free article] [PubMed] [Google Scholar]
- Vaughan-Jones R. D. Regulation of chloride in quiescent sheep-heart Purkinje fibres studied using intracellular chloride and pH-sensitive micro-electrodes. J Physiol. 1979 Oct;295:111–137. doi: 10.1113/jphysiol.1979.sp012957. [DOI] [PMC free article] [PubMed] [Google Scholar]
- White M. M., Miller C. A voltage-gated anion channel from the electric organ of Torpedo californica. J Biol Chem. 1979 Oct 25;254(20):10161–10166. [PubMed] [Google Scholar]
- Widdicombe J. H., Brading A. F. A possible role of linked Na and Cl movement in active Cl uptake in smooth muscle. Pflugers Arch. 1980 Jul;386(1):35–37. doi: 10.1007/BF00584184. [DOI] [PubMed] [Google Scholar]
- Wieth J. O. Bicarbonate exchange through the human red cell membrane determined with [14C] bicarbonate. J Physiol. 1979 Sep;294:521–539. doi: 10.1113/jphysiol.1979.sp012944. [DOI] [PMC free article] [PubMed] [Google Scholar]