Abstract
The difference between the ionic bases for electroresponsiveness in the three types of neurone in the ventromedial hypothalamic nucleus (v.m.h.) were studied in in vitro brain slice preparations of the guinea-pig. The current-voltage relationships in all three types of neurone showed anomalous rectification. Application of tetrodotoxin (TTX) abolished the fast action potentials in all three types of cell. In type A cells, injections of outward current pulses did not evoke spikes when the cells were perfused with TTX alone, but the addition of tetraethylammonium chloride elicited broad spikes. In type B and C cells, broad spikes could be evoked with TTX alone. These results suggest the presence of a minimal high-threshold Ca2+ current in type A neurones, and a more prominent one in type B and C neurones. In type B neurones, Ca2+ conductance blockage with Mn2+ or Co2+, or replacement of Ca2+ by Mg2+, abolished the low-threshold response (l.t.r.). Substitution with Ba2+ did not increase the duration of the l.t.r. significantly, suggesting that under normal conditions the falling phase of the response was caused by inactivation of Ca2+ conductance. In type B and C neurones, the amplitude and duration of the after-hyperpolarization (a.h.p.) following direct activation by long outward current pulses were markedly reduced in Ca2+-free solution. These findings indicate that a large component of this response was generated by the Ca2+-dependent K+ conductance increase. In type A cells the a.h.p. amplitude was originally small and was not affected by the above treatment, suggesting that the participation of this conductance was minimal in this type. In type C neurones, the membrane potential following an inward current pulse showed a delayed return to the base line. This delay was produced by transient K+ conductance, since it was reduced by 4-aminopyridine. The frequency-current (f-I) relation of the first interval in type A and C cells was scarcely affected in Ca2+-free solution, while the slope of the initial firing f-I curves in type B neurones which had the l.t.r. became flatter. Furthermore, the f-I curves of the third interval in type C cells became steeper in Ca2+-free solution. The data indicate that the distinct membrane characteristics related to the heterogeneity among cells in the v.m.h. can be attributed to their specific ionic mechanisms, with the type A neurones showing a minimal high-threshold Ca2+ current, the type B having l.t.r. and the type C having a transient K+ (IA) current.
Full text
PDF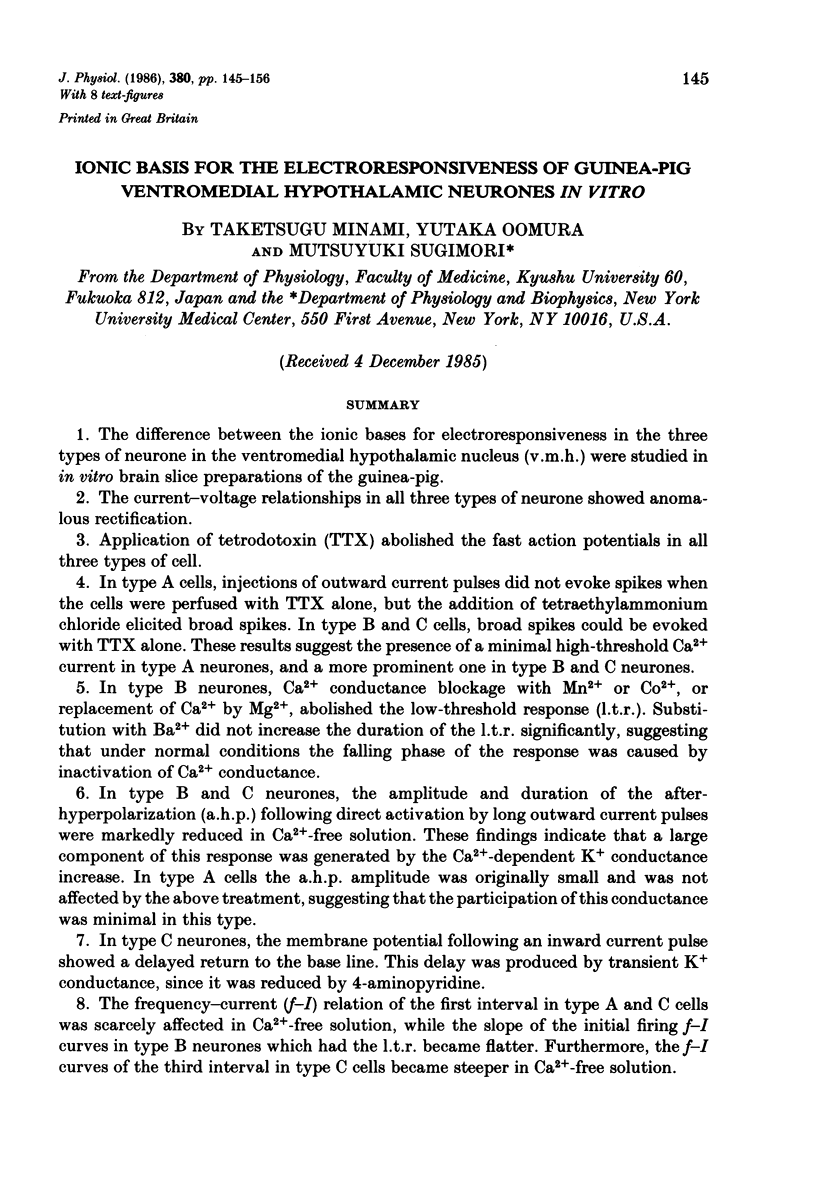
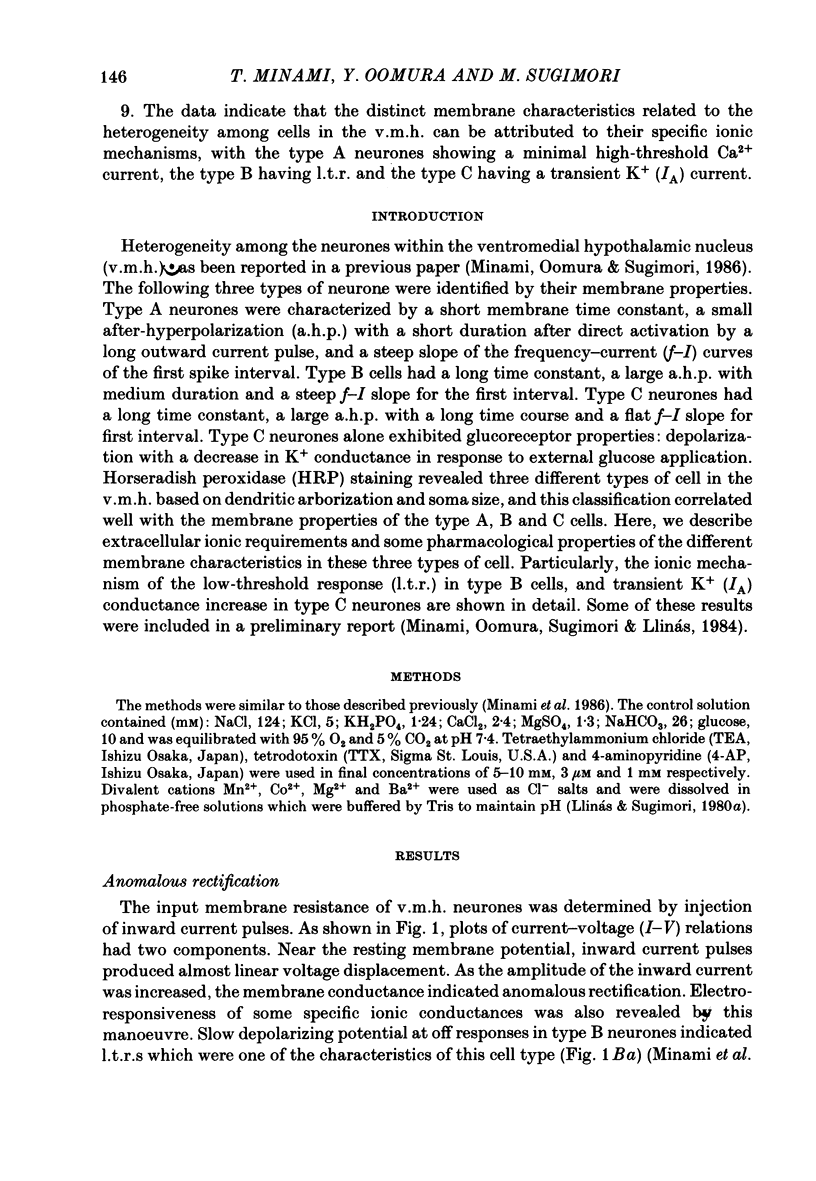
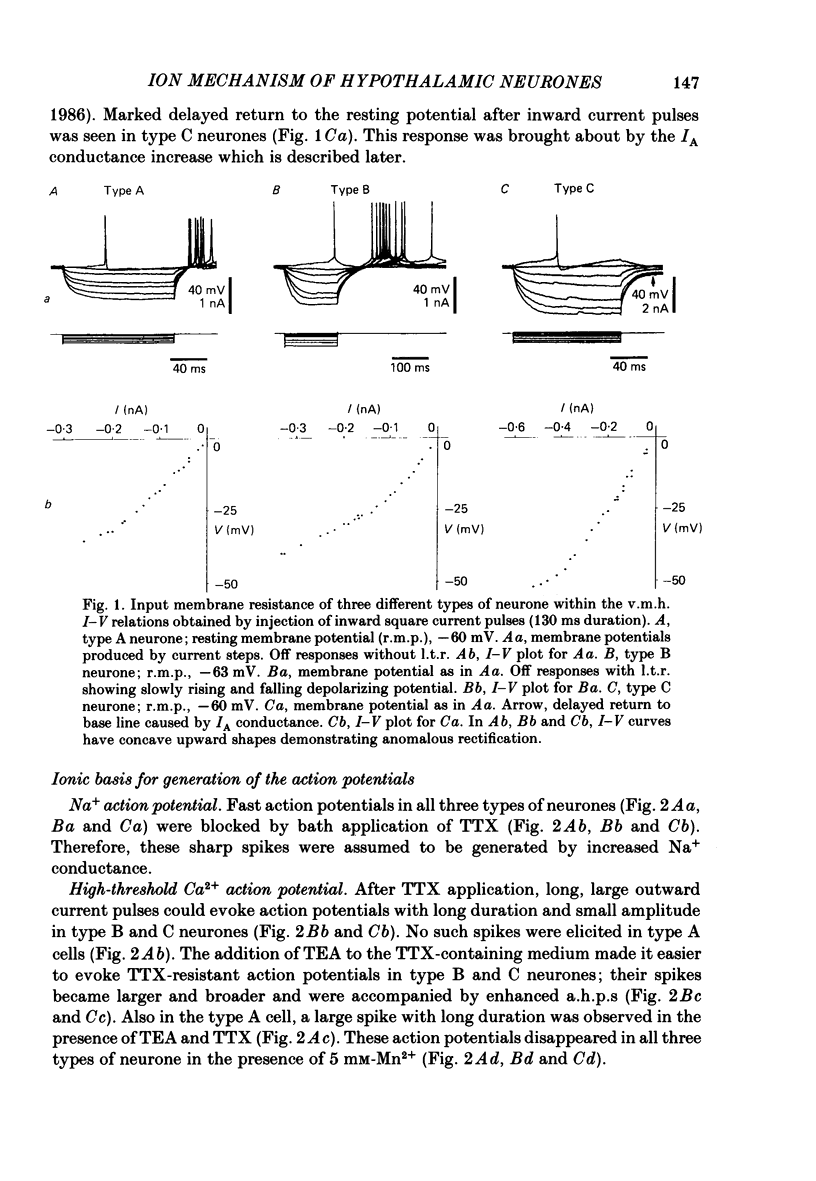
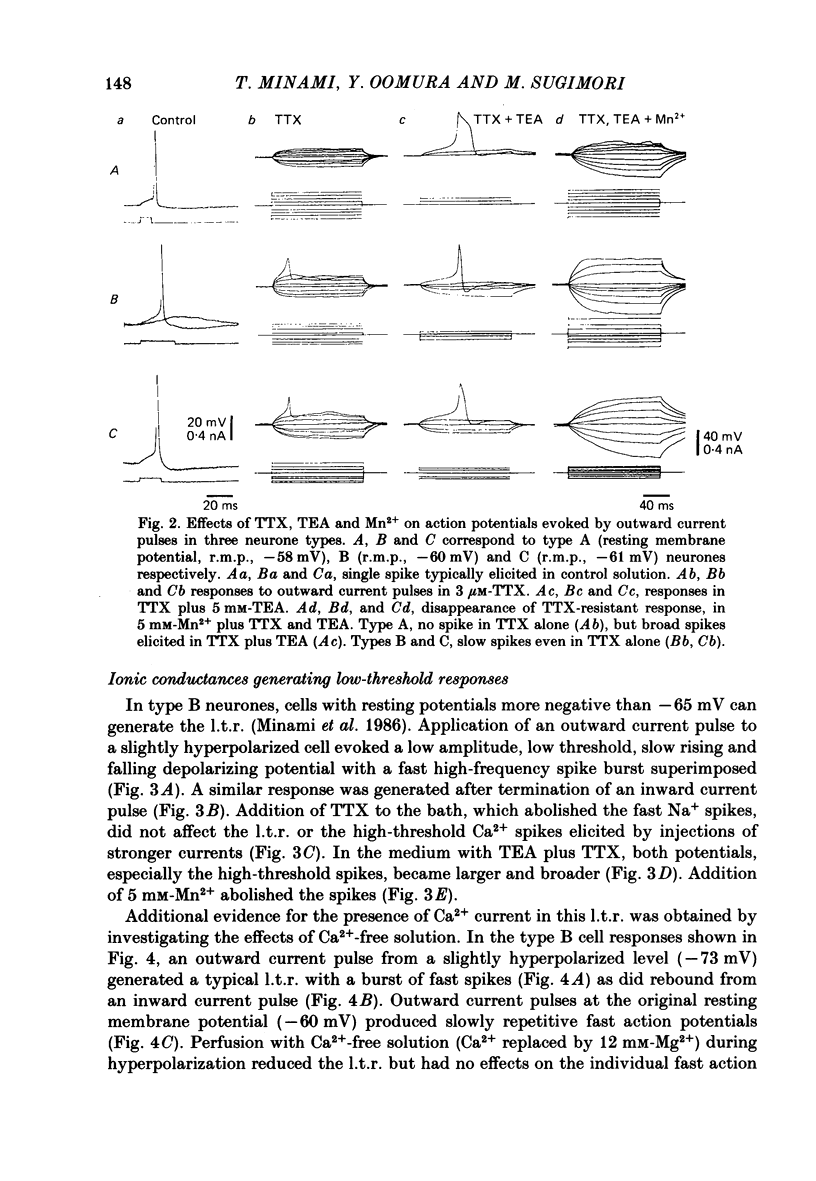
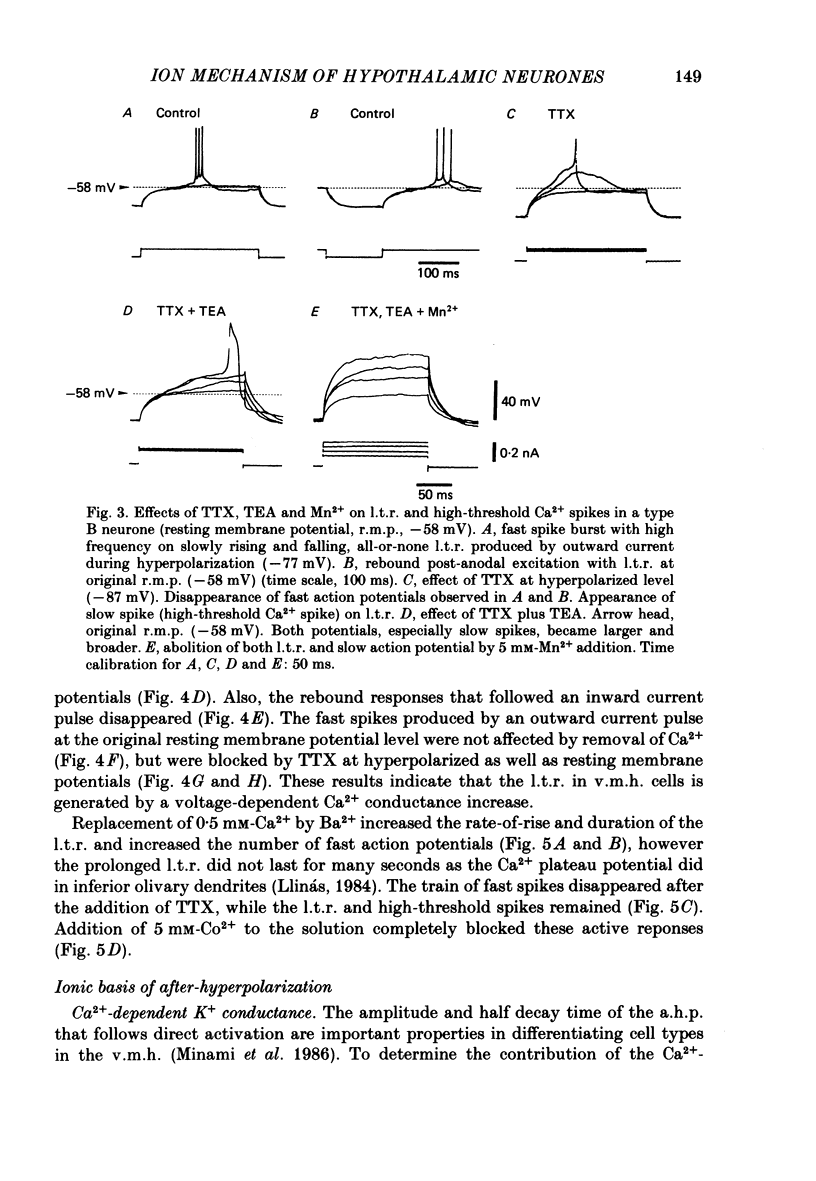
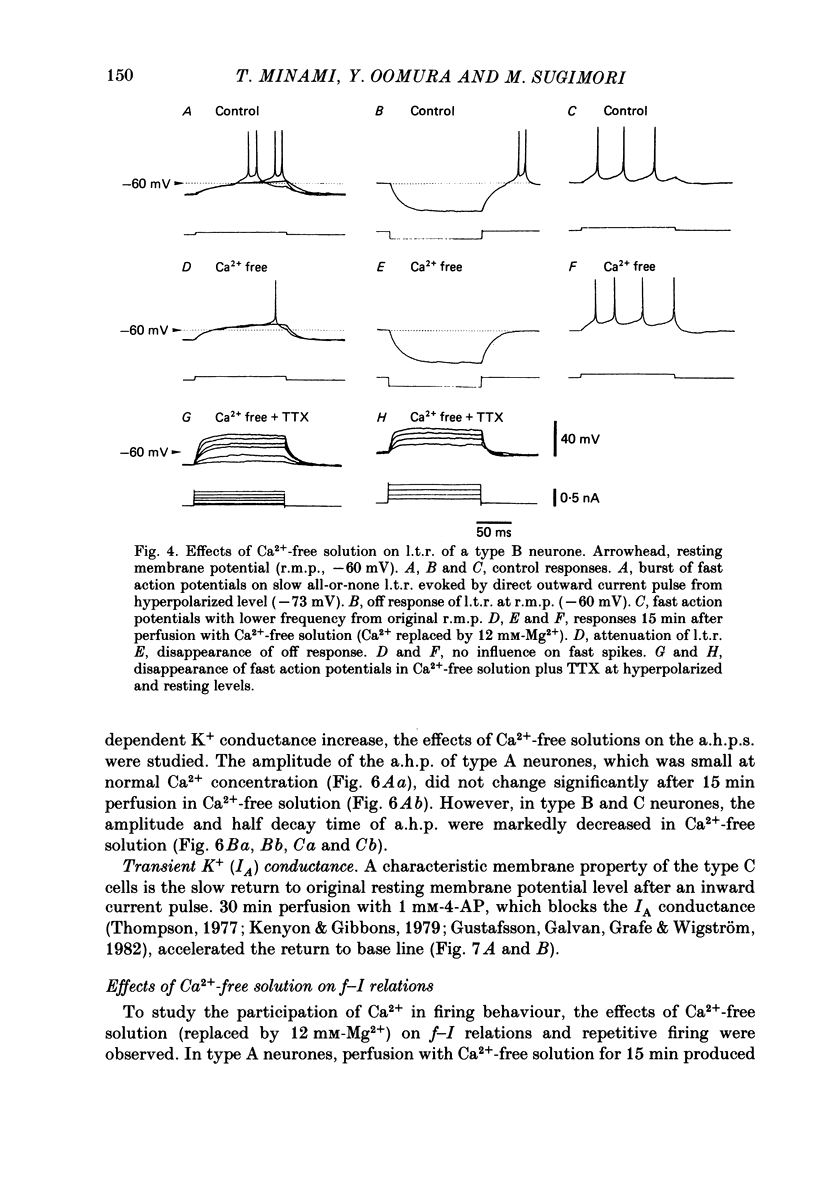
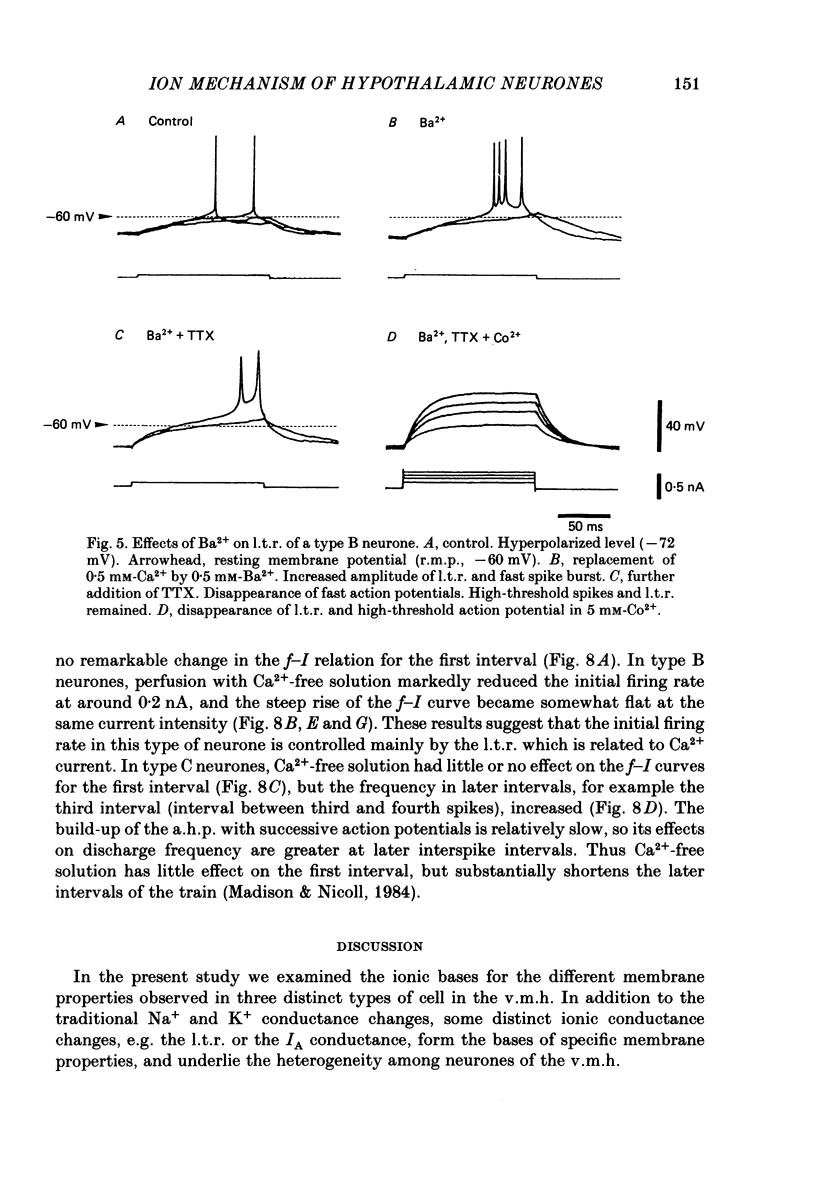
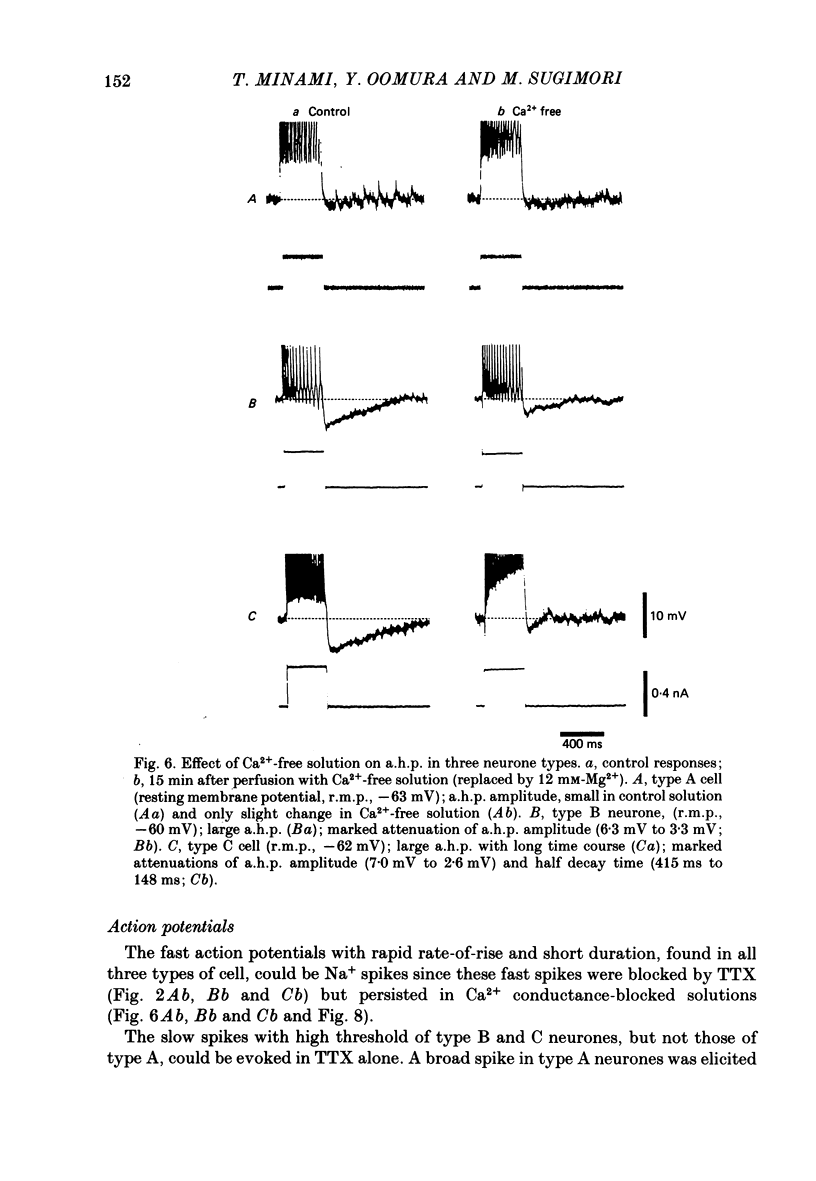
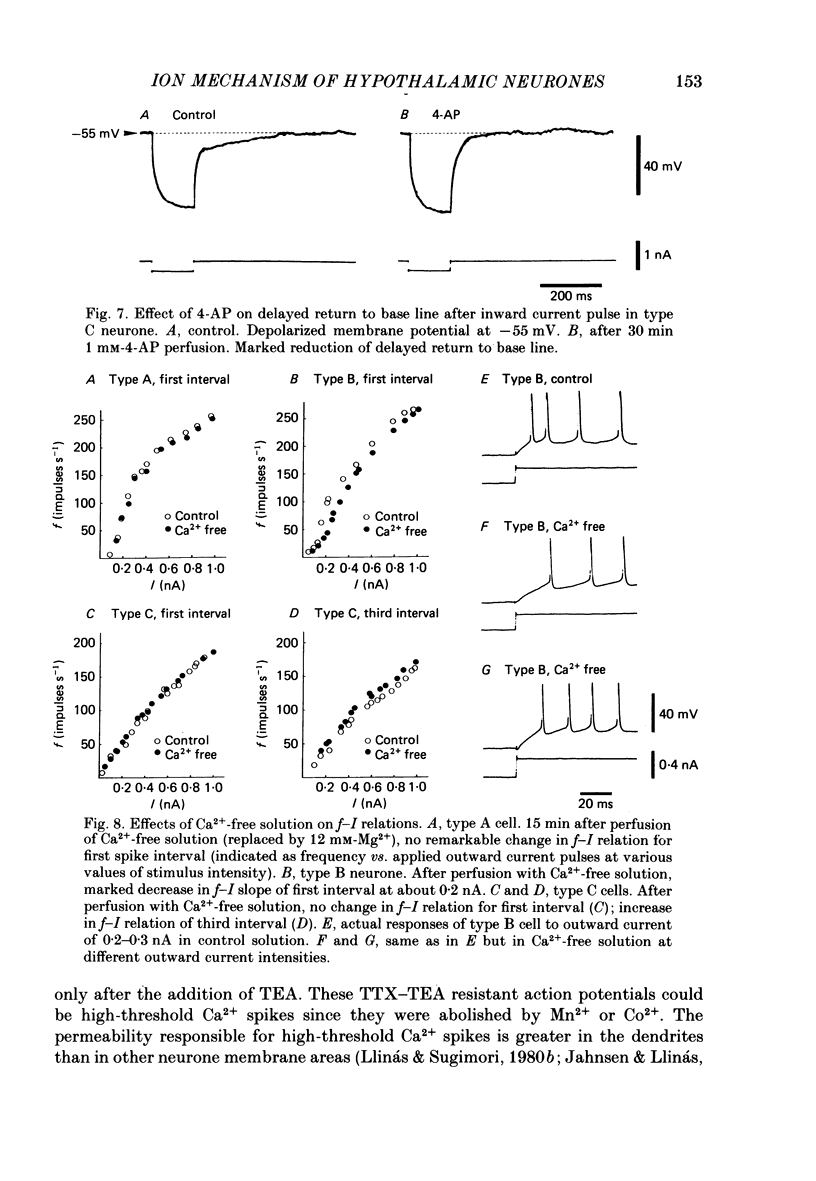
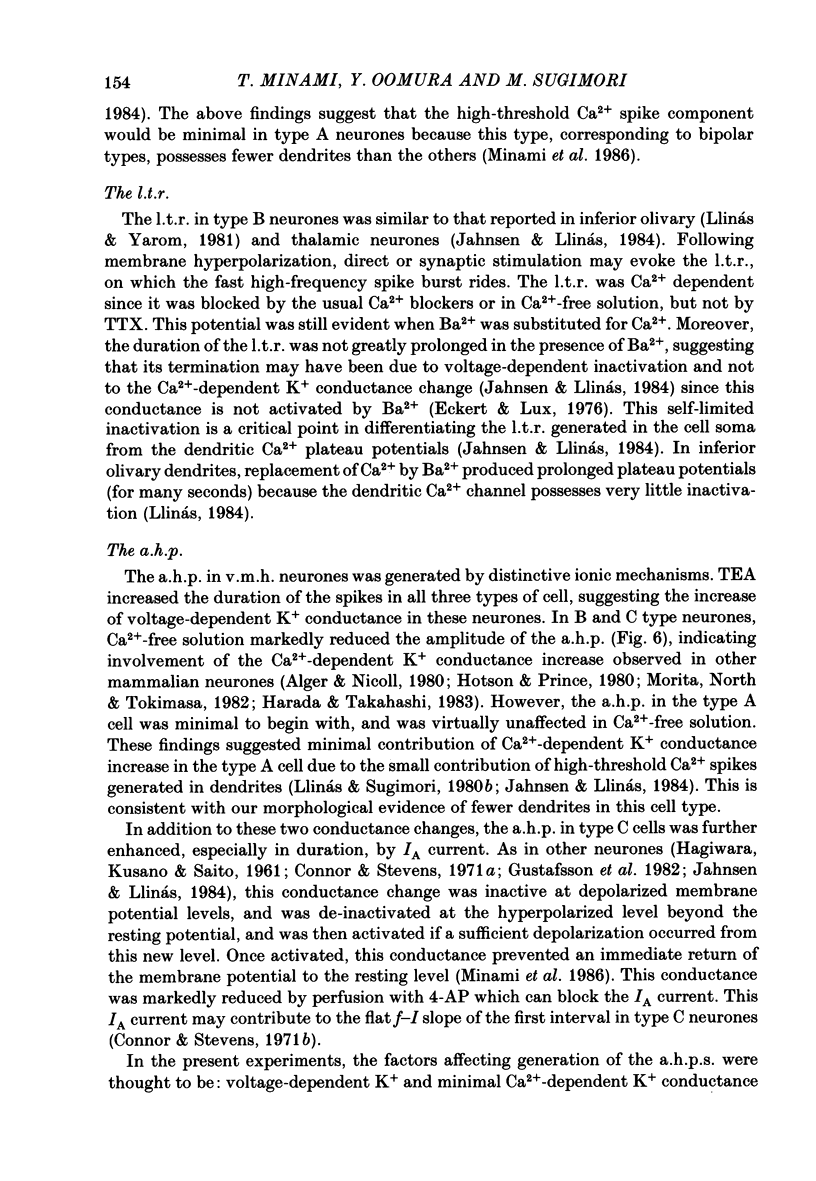
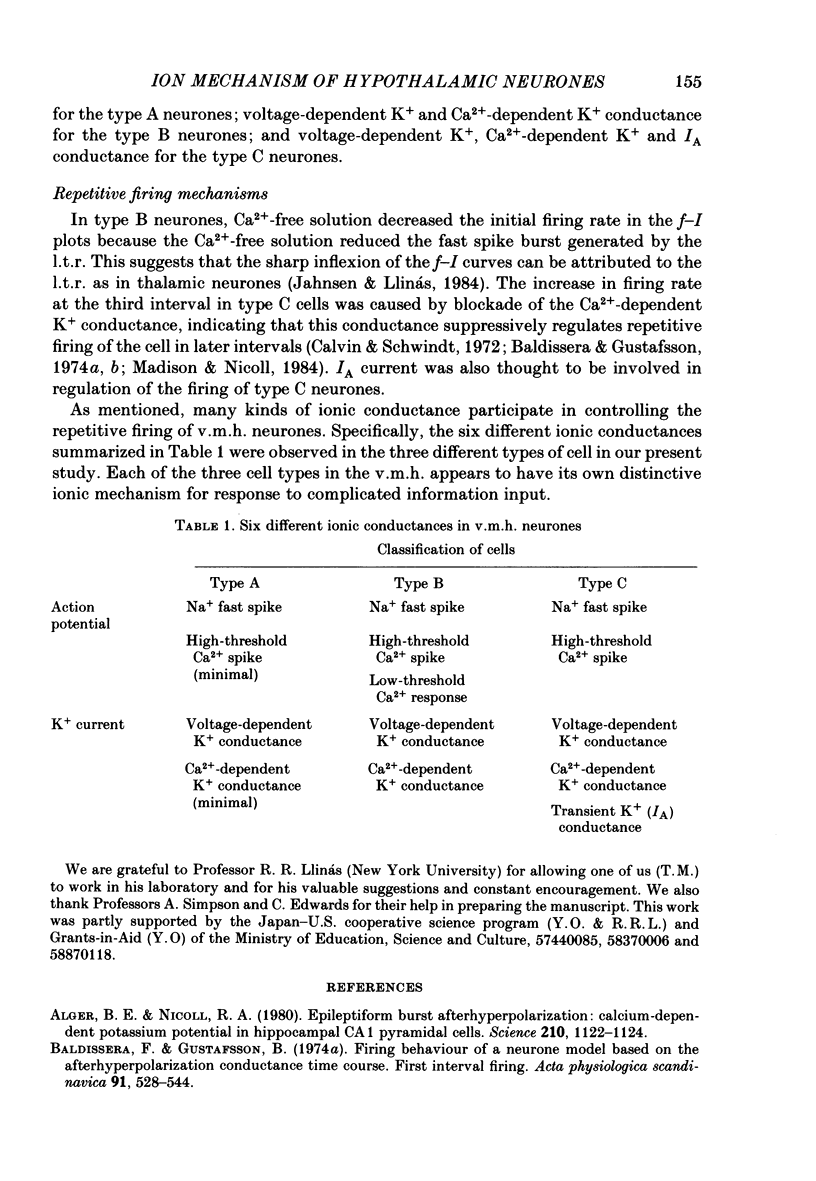
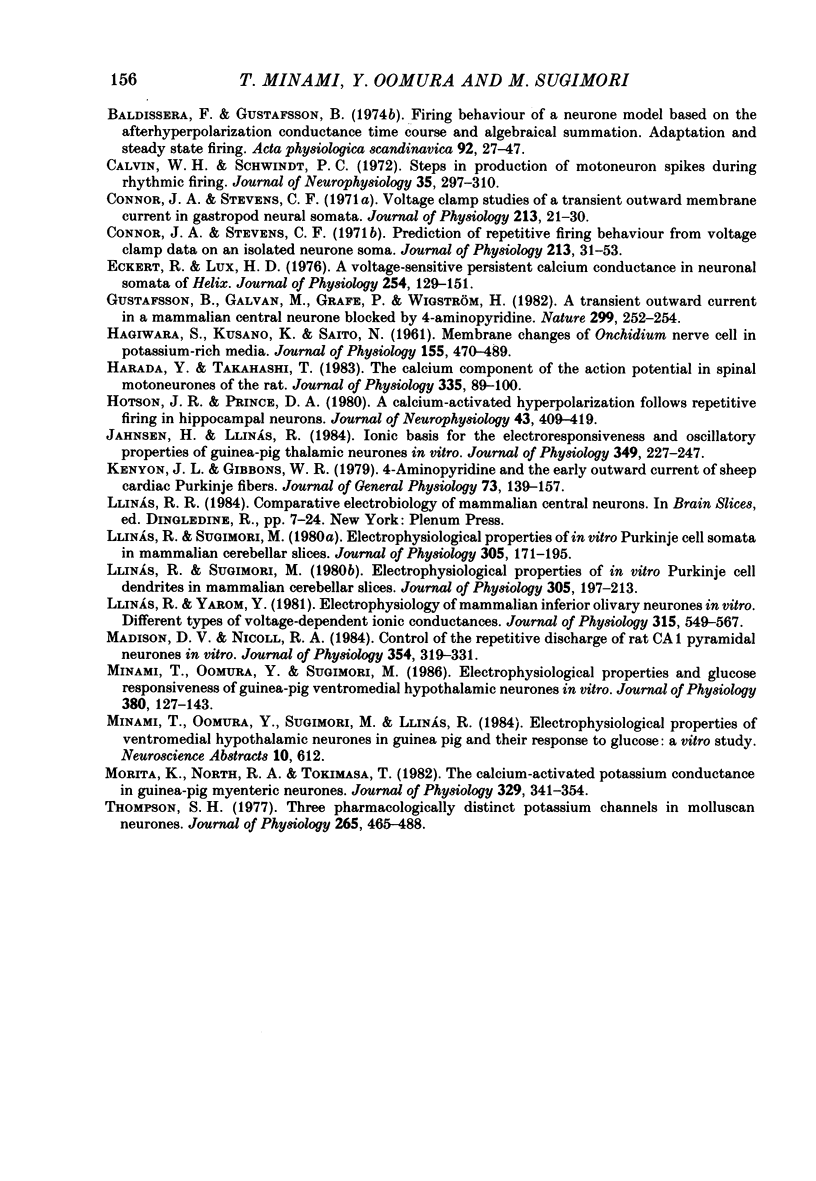
Selected References
These references are in PubMed. This may not be the complete list of references from this article.
- Alger B. E., Nicoll R. A. Epileptiform burst afterhyperolarization: calcium-dependent potassium potential in hippocampal CA1 pyramidal cells. Science. 1980 Dec 5;210(4474):1122–1124. doi: 10.1126/science.7444438. [DOI] [PubMed] [Google Scholar]
- Baldissera F., Gustafsson B. Firing behaviour of a neurone model based on the afterhyperpolarization conductance time course and algebraical summation. Adaptation and steady state firing. Acta Physiol Scand. 1974 Sep;92(1):27–47. doi: 10.1111/j.1748-1716.1974.tb05720.x. [DOI] [PubMed] [Google Scholar]
- Baldissera F., Gustafsson B. Firing behaviour of a neurone model based on the afterhyperpolarization conductance time course. First interval firing. Acta Physiol Scand. 1974 Aug;91(4):528–544. doi: 10.1111/j.1748-1716.1974.tb05708.x. [DOI] [PubMed] [Google Scholar]
- Calvin W. H., Schwindt P. C. Steps in production of motoneuron spikes during rhythmic firing. J Neurophysiol. 1972 May;35(3):297–310. doi: 10.1152/jn.1972.35.3.297. [DOI] [PubMed] [Google Scholar]
- Connor J. A., Stevens C. F. Prediction of repetitive firing behaviour from voltage clamp data on an isolated neurone soma. J Physiol. 1971 Feb;213(1):31–53. doi: 10.1113/jphysiol.1971.sp009366. [DOI] [PMC free article] [PubMed] [Google Scholar]
- Connor J. A., Stevens C. F. Voltage clamp studies of a transient outward membrane current in gastropod neural somata. J Physiol. 1971 Feb;213(1):21–30. doi: 10.1113/jphysiol.1971.sp009365. [DOI] [PMC free article] [PubMed] [Google Scholar]
- Eckert R., Lux H. D. A voltage-sensitive persistent calcium conductance in neuronal somata of Helix. J Physiol. 1976 Jan;254(1):129–151. doi: 10.1113/jphysiol.1976.sp011225. [DOI] [PMC free article] [PubMed] [Google Scholar]
- Gustafsson B., Galvan M., Grafe P., Wigström H. A transient outward current in a mammalian central neurone blocked by 4-aminopyridine. Nature. 1982 Sep 16;299(5880):252–254. doi: 10.1038/299252a0. [DOI] [PubMed] [Google Scholar]
- HAGIWARA S., KUSANO K., SAITO N. Membrane changes of Onchidium nerve cell in potassium-rich media. J Physiol. 1961 Mar;155:470–489. doi: 10.1113/jphysiol.1961.sp006640. [DOI] [PMC free article] [PubMed] [Google Scholar]
- Harada Y., Takahashi T. The calcium component of the action potential in spinal motoneurones of the rat. J Physiol. 1983 Feb;335:89–100. doi: 10.1113/jphysiol.1983.sp014521. [DOI] [PMC free article] [PubMed] [Google Scholar]
- Hotson J. R., Prince D. A. A calcium-activated hyperpolarization follows repetitive firing in hippocampal neurons. J Neurophysiol. 1980 Feb;43(2):409–419. doi: 10.1152/jn.1980.43.2.409. [DOI] [PubMed] [Google Scholar]
- Jahnsen H., Llinás R. Ionic basis for the electro-responsiveness and oscillatory properties of guinea-pig thalamic neurones in vitro. J Physiol. 1984 Apr;349:227–247. doi: 10.1113/jphysiol.1984.sp015154. [DOI] [PMC free article] [PubMed] [Google Scholar]
- Kenyon J. L., Gibbons W. R. 4-Aminopyridine and the early outward current of sheep cardiac Purkinje fibers. J Gen Physiol. 1979 Feb;73(2):139–157. doi: 10.1085/jgp.73.2.139. [DOI] [PMC free article] [PubMed] [Google Scholar]
- Llinás R., Sugimori M. Electrophysiological properties of in vitro Purkinje cell dendrites in mammalian cerebellar slices. J Physiol. 1980 Aug;305:197–213. doi: 10.1113/jphysiol.1980.sp013358. [DOI] [PMC free article] [PubMed] [Google Scholar]
- Llinás R., Sugimori M. Electrophysiological properties of in vitro Purkinje cell somata in mammalian cerebellar slices. J Physiol. 1980 Aug;305:171–195. doi: 10.1113/jphysiol.1980.sp013357. [DOI] [PMC free article] [PubMed] [Google Scholar]
- Llinás R., Yarom Y. Electrophysiology of mammalian inferior olivary neurones in vitro. Different types of voltage-dependent ionic conductances. J Physiol. 1981 Jun;315:549–567. doi: 10.1113/jphysiol.1981.sp013763. [DOI] [PMC free article] [PubMed] [Google Scholar]
- Madison D. V., Nicoll R. A. Control of the repetitive discharge of rat CA 1 pyramidal neurones in vitro. J Physiol. 1984 Sep;354:319–331. doi: 10.1113/jphysiol.1984.sp015378. [DOI] [PMC free article] [PubMed] [Google Scholar]
- Minami T., Oomura Y., Sugimori M. Electrophysiological properties and glucose responsiveness of guinea-pig ventromedial hypothalamic neurones in vitro. J Physiol. 1986 Nov;380:127–143. doi: 10.1113/jphysiol.1986.sp016276. [DOI] [PMC free article] [PubMed] [Google Scholar]
- Morita K., North R. A., Tokimasa T. The calcium-activated potassium conductance in guinea-pig myenteric neurones. J Physiol. 1982 Aug;329:341–354. doi: 10.1113/jphysiol.1982.sp014306. [DOI] [PMC free article] [PubMed] [Google Scholar]
- Thompson S. H. Three pharmacologically distinct potassium channels in molluscan neurones. J Physiol. 1977 Feb;265(2):465–488. doi: 10.1113/jphysiol.1977.sp011725. [DOI] [PMC free article] [PubMed] [Google Scholar]