Abstract
1. 86Rb and 42K have been used to assess potassium exchange in frog skeletal muscle which had been metabolically exhausted by electrical stimulation (1 Hz) after treatment with 2 mM-cyanide and 1 mM-iodoacetate. These conditions led to the development of rigor. 2. Poisoning by itself induced a small but variable increase in tracer efflux. Complete mechanical exhaustion subsequent to electrical stimulation was, however, accompanied by a 5-6 fold increase in the rate coefficient for both 86Rb and 42K efflux. In the case of rubidium this was maintained for at least 20 min and often for up to 1 h. 3. The increase in tracer efflux induced by metabolic exhaustion was inhibited by barium (0.03-5 mM) in a reversible and concentration-dependent manner. Inhibition was also observed with glibenclamide (3-100 microM), tolbutamide (0.3-2 mM), TEA (5-100 mM) and the local anaesthetics lignocaine (1-3 mM) and tetracaine (1 mM). Quinine produced a dual response consisting of an inhibitory component which was most clearly seen at low concentrations (0.3 mM) and an enhancement of tracer efflux that became increasingly dominant at higher concentrations (1-10 mM). 4. Both apamin (30 and 100 nM) and Israeli scorpion (Leiurus quinquestriatus) venom (16 micrograms ml-1) produced little or no block of the tracer efflux activated by metabolic exhaustion. Similarly 4-aminopyridine (3 mM) and decamethonium (0.3 mM) were without obvious effect. 5. It is concluded that metabolic exhaustion of frog skeletal muscle leads to an increased permeability to both 42K and 86Rb. Our results with channel blockers suggest that this K+ permeability can be attributed neither to the delayed rectifier nor to an apamin- or charybdotoxin-sensitive calcium-activated K+ permeability (PK(Ca) but may be predominantly due to activation of ATP-sensitive channels similar to those found in the beta-cells of pancreatic islets.
Full text
PDF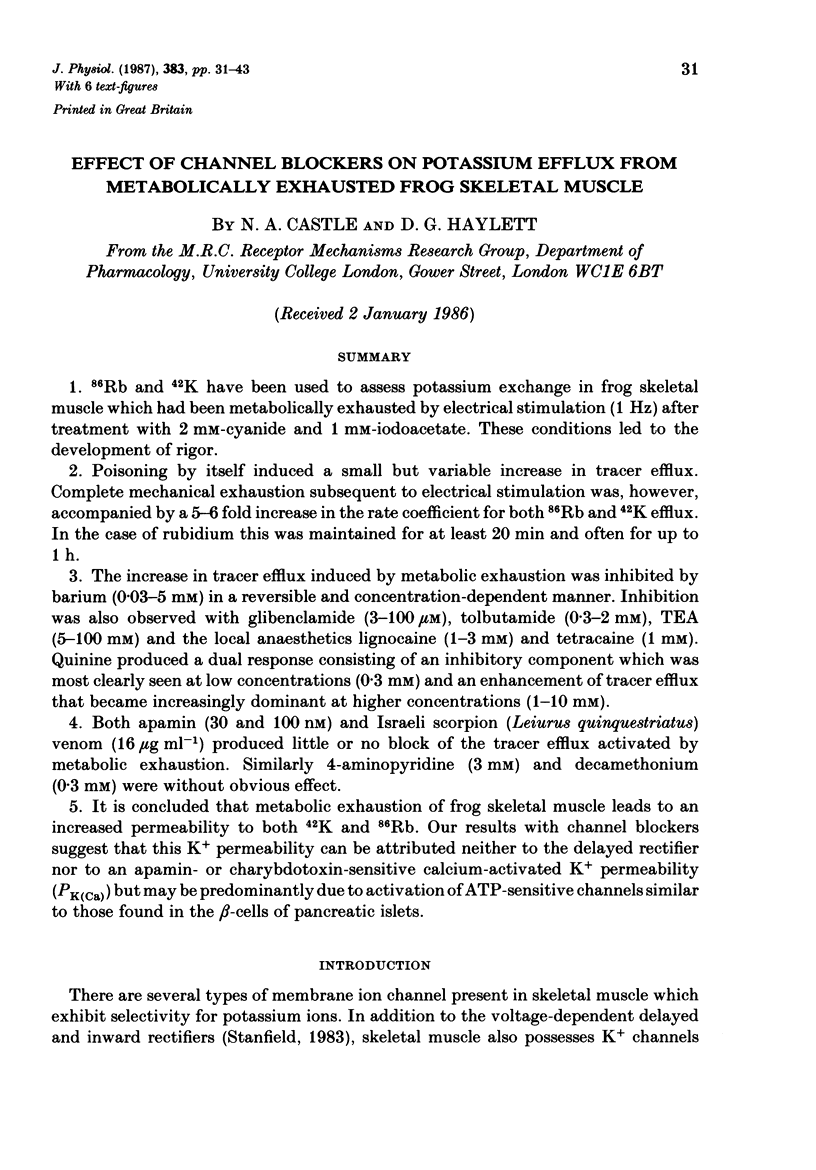
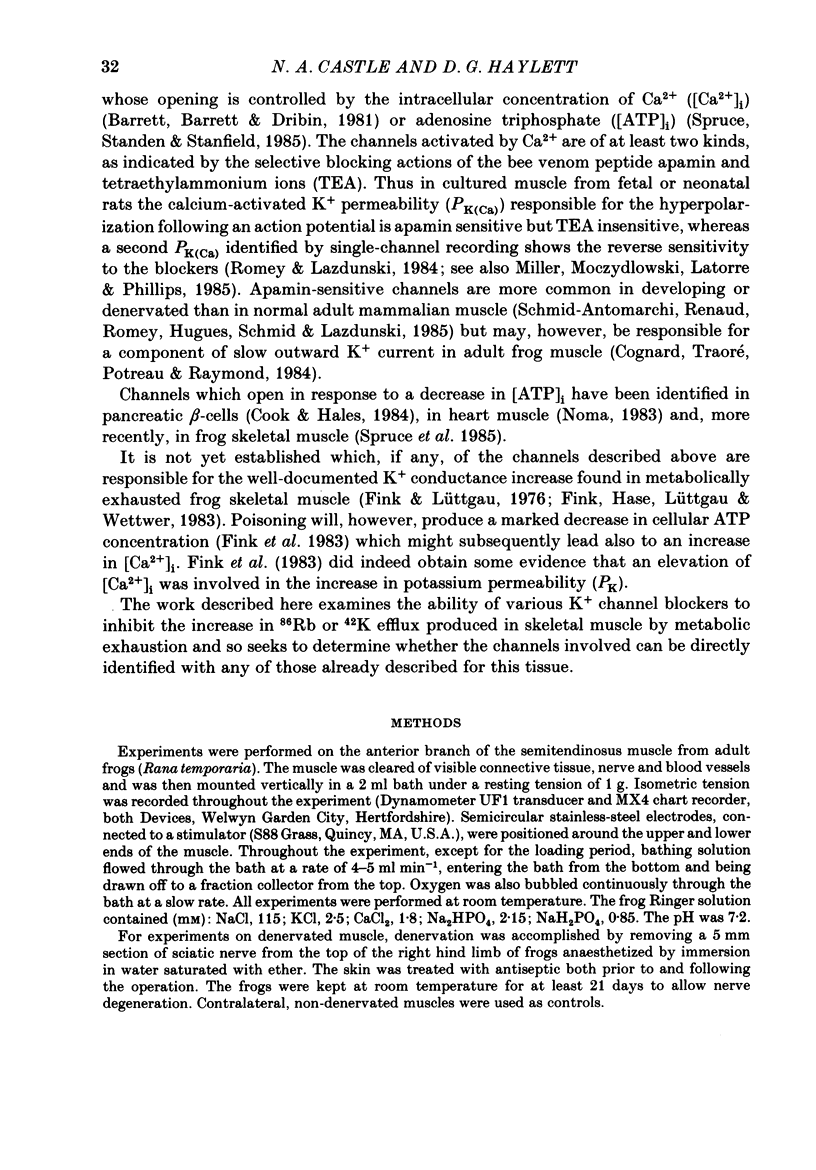
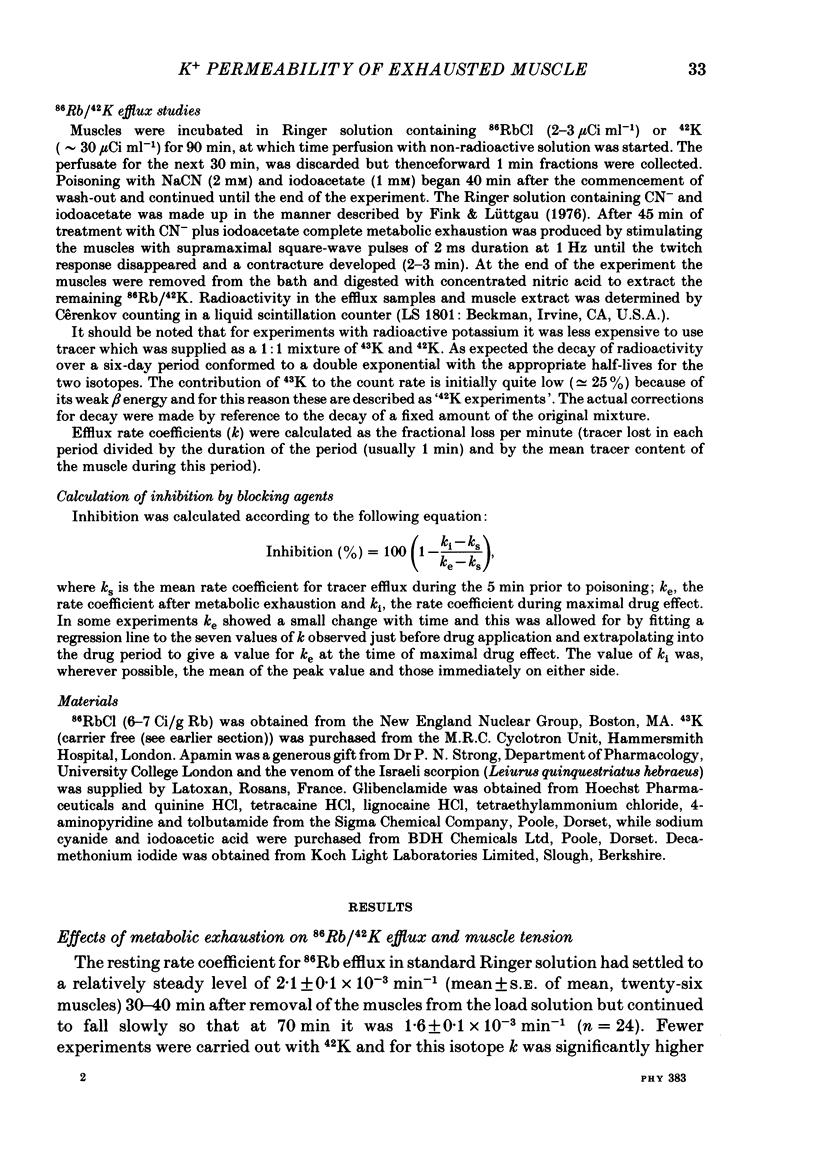
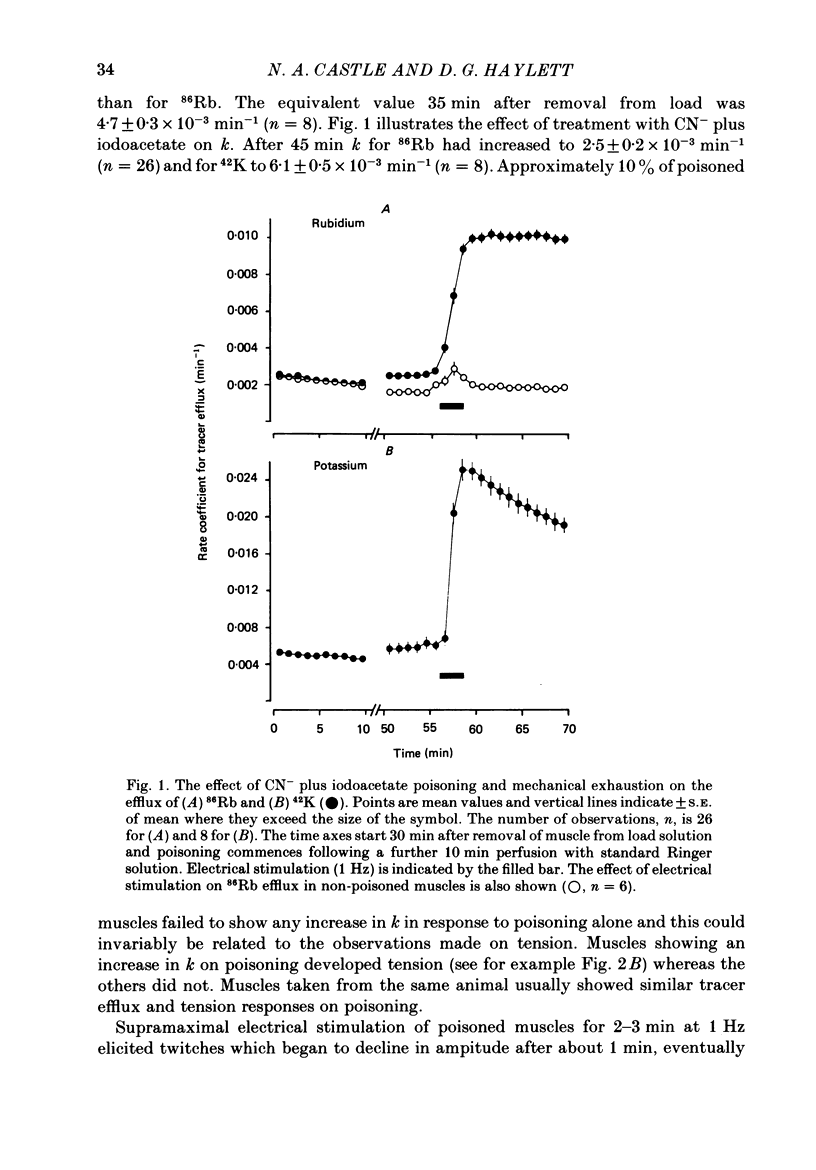
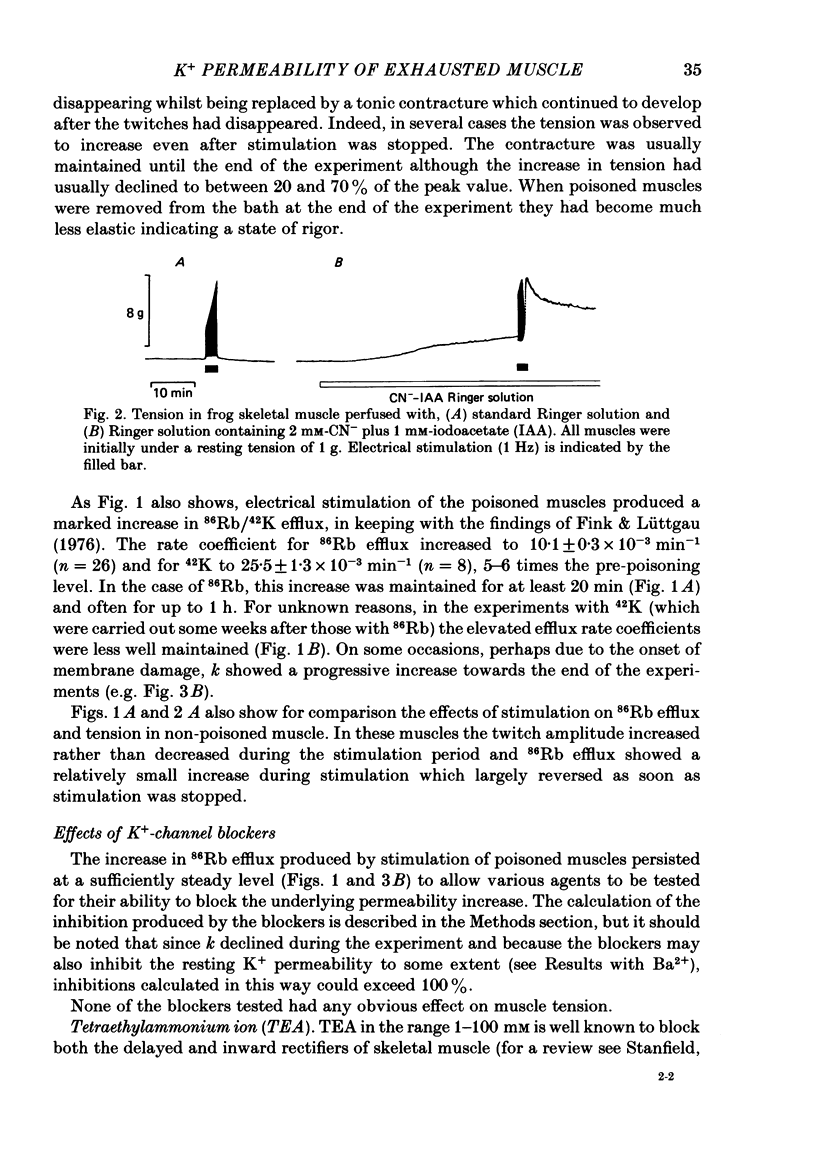
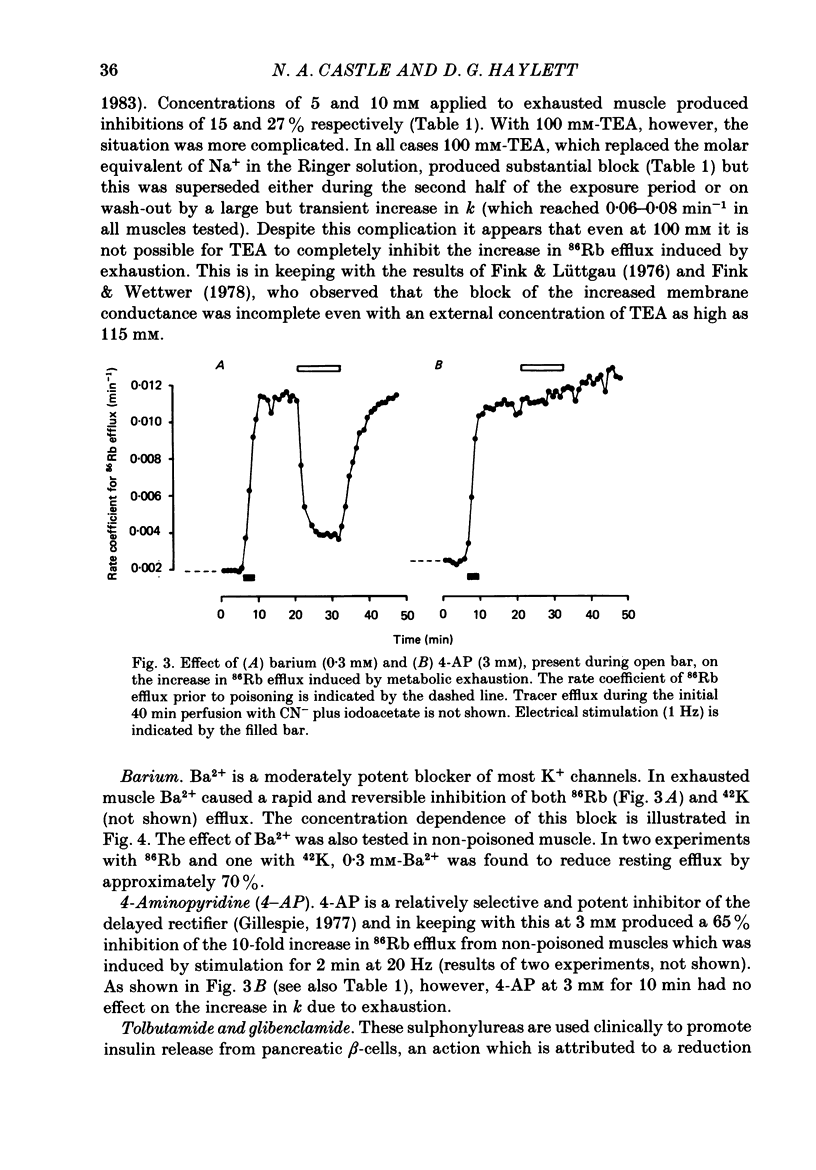
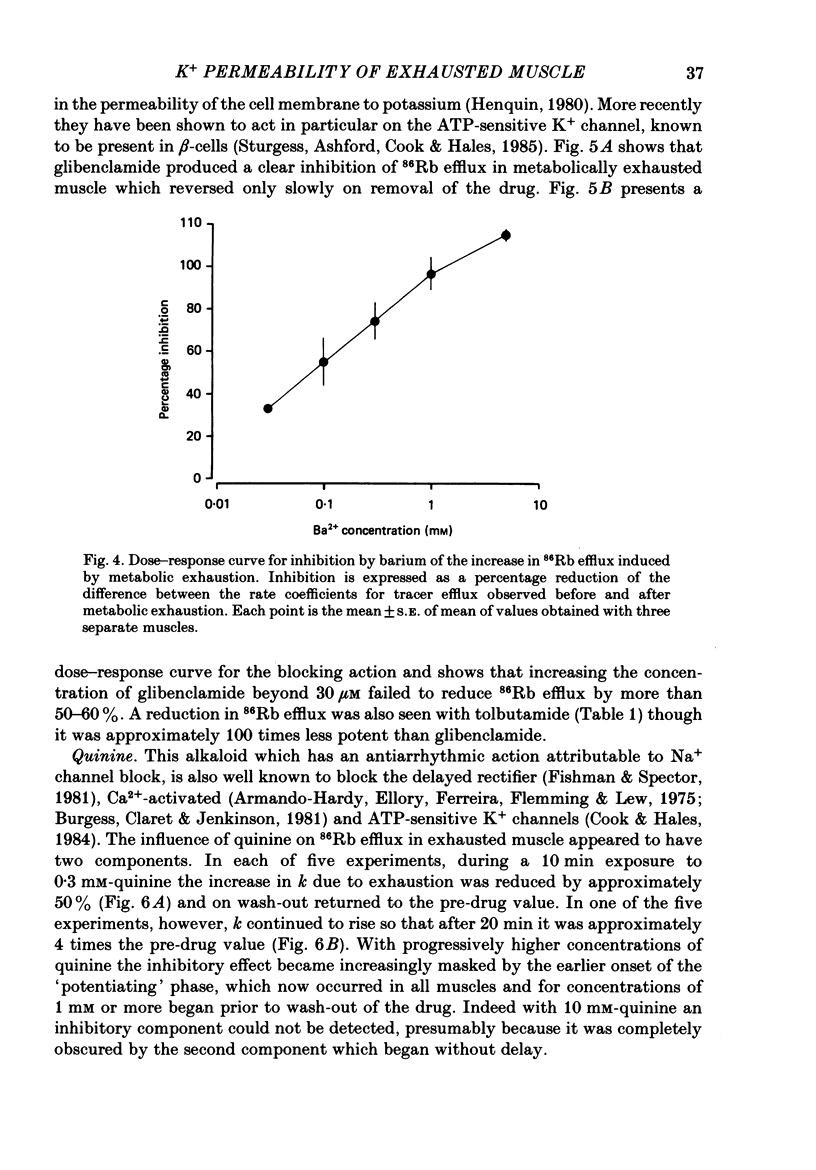
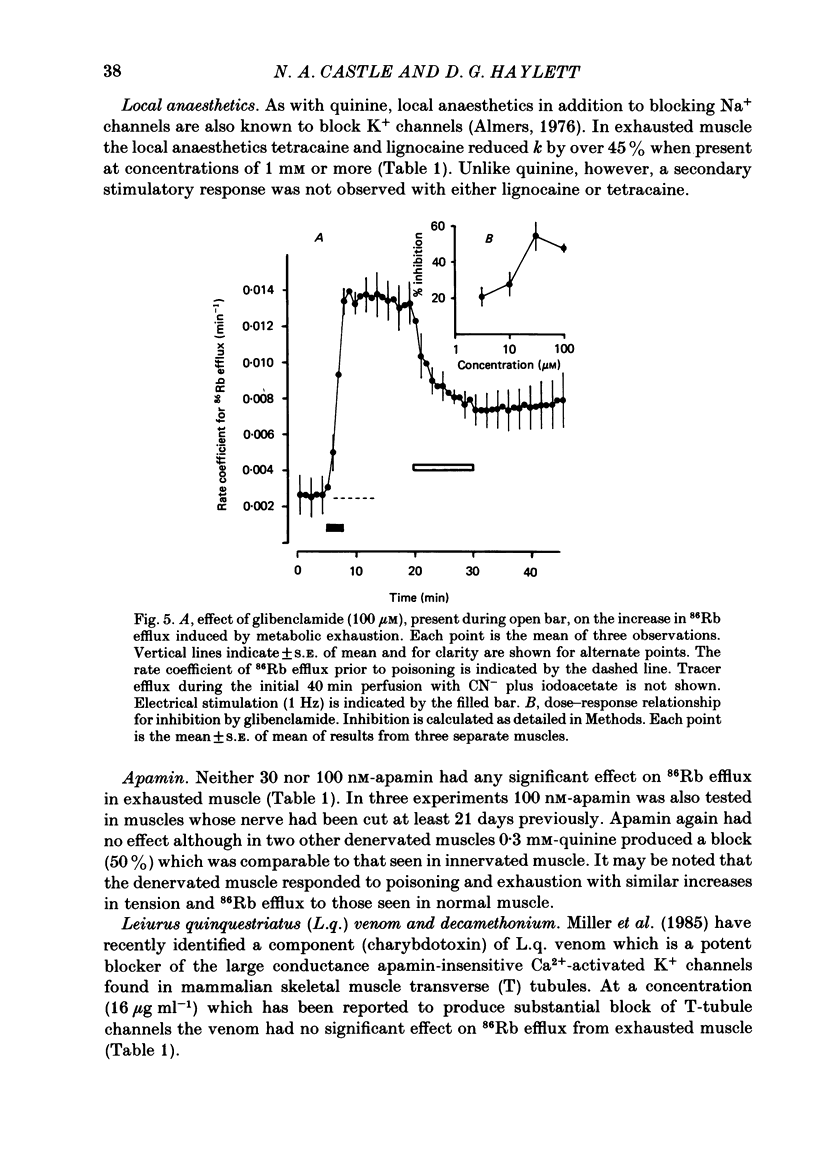
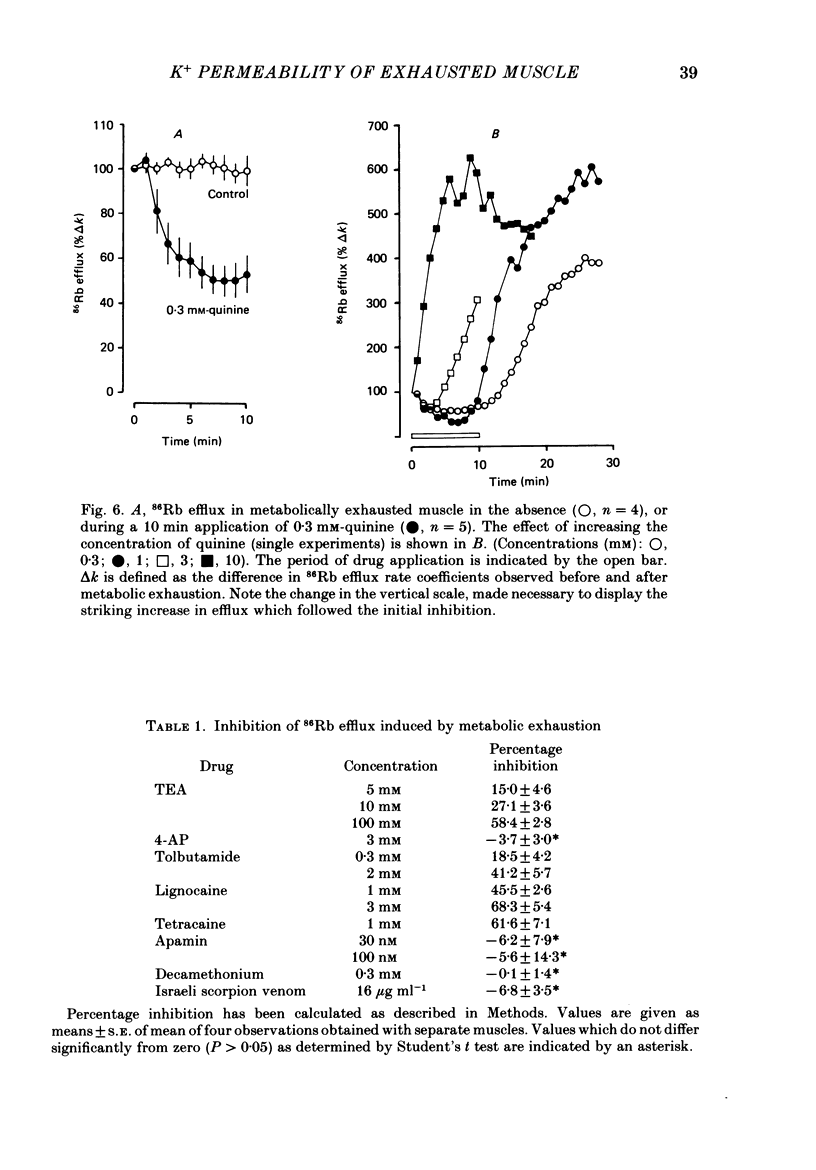
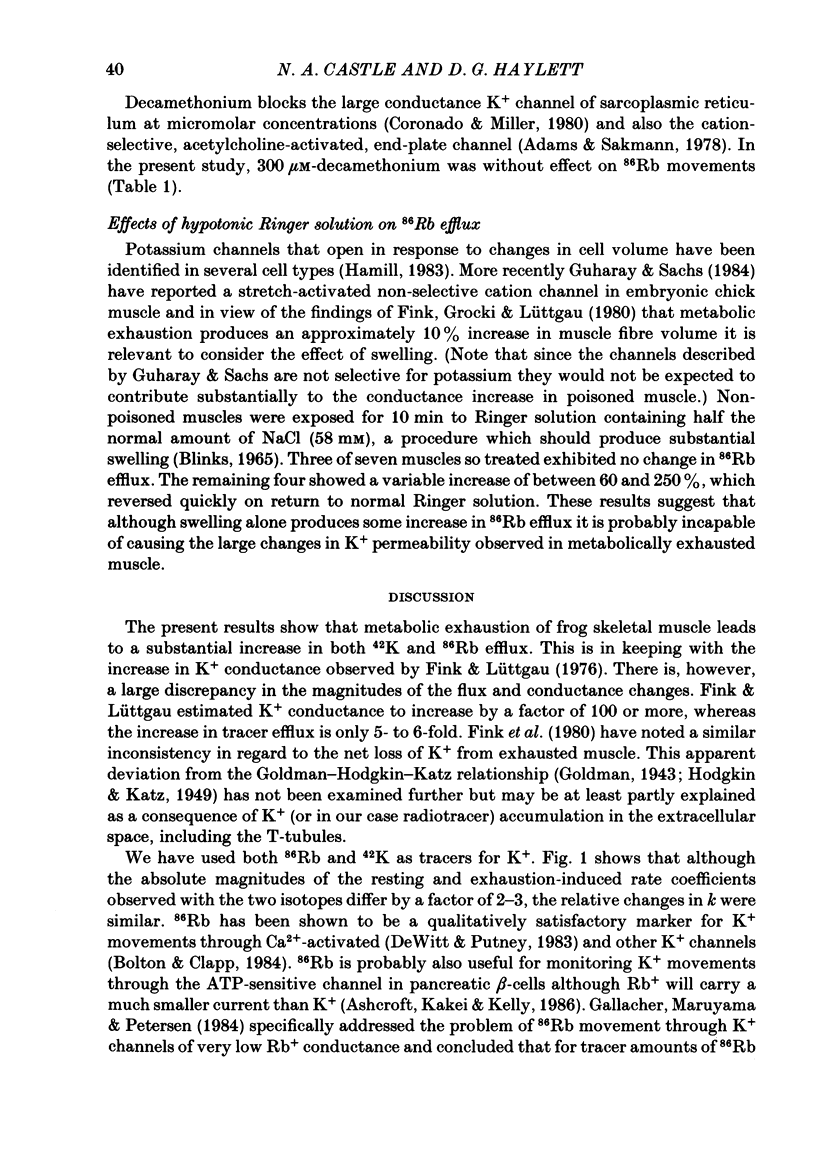
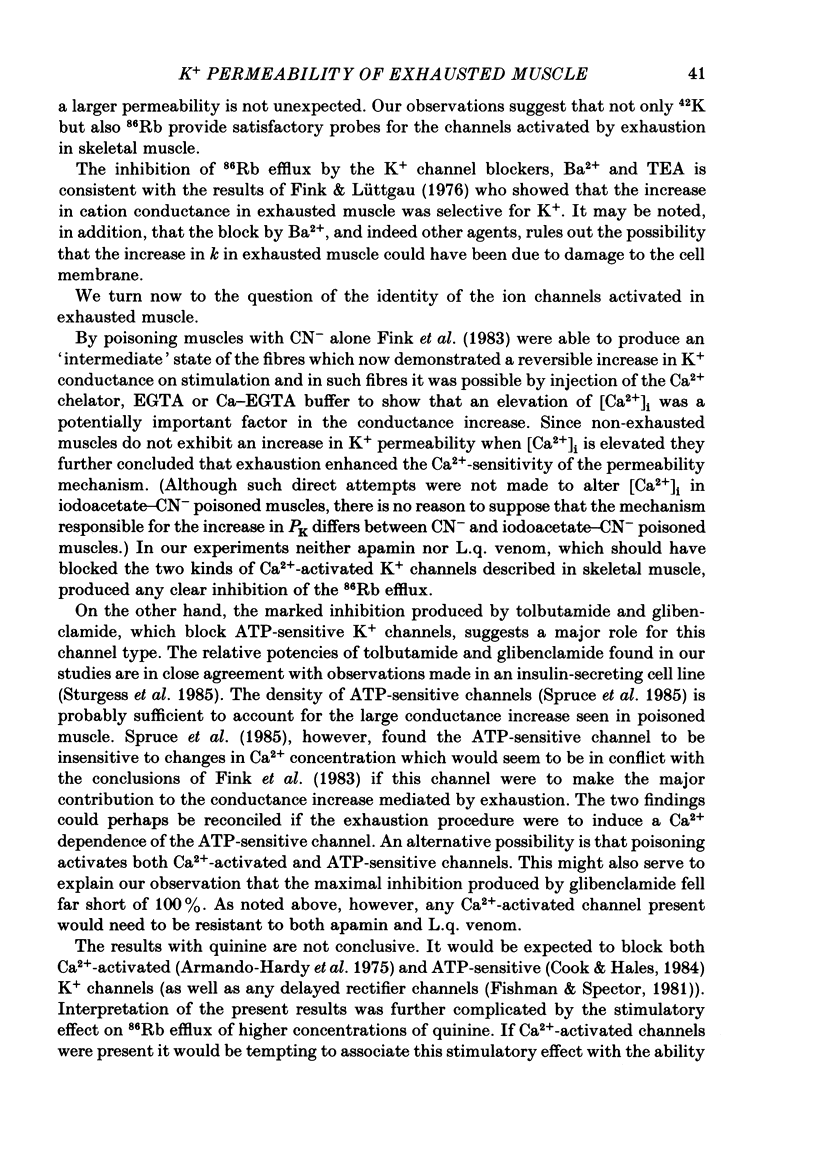
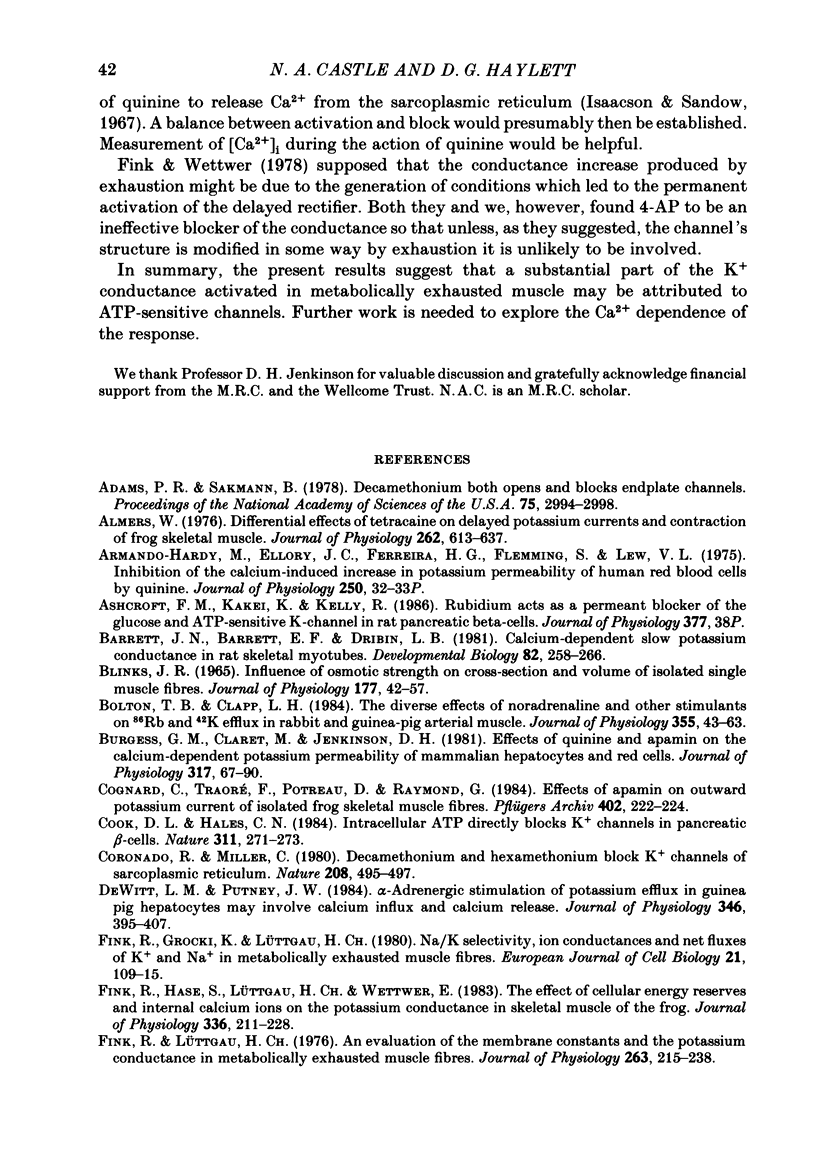
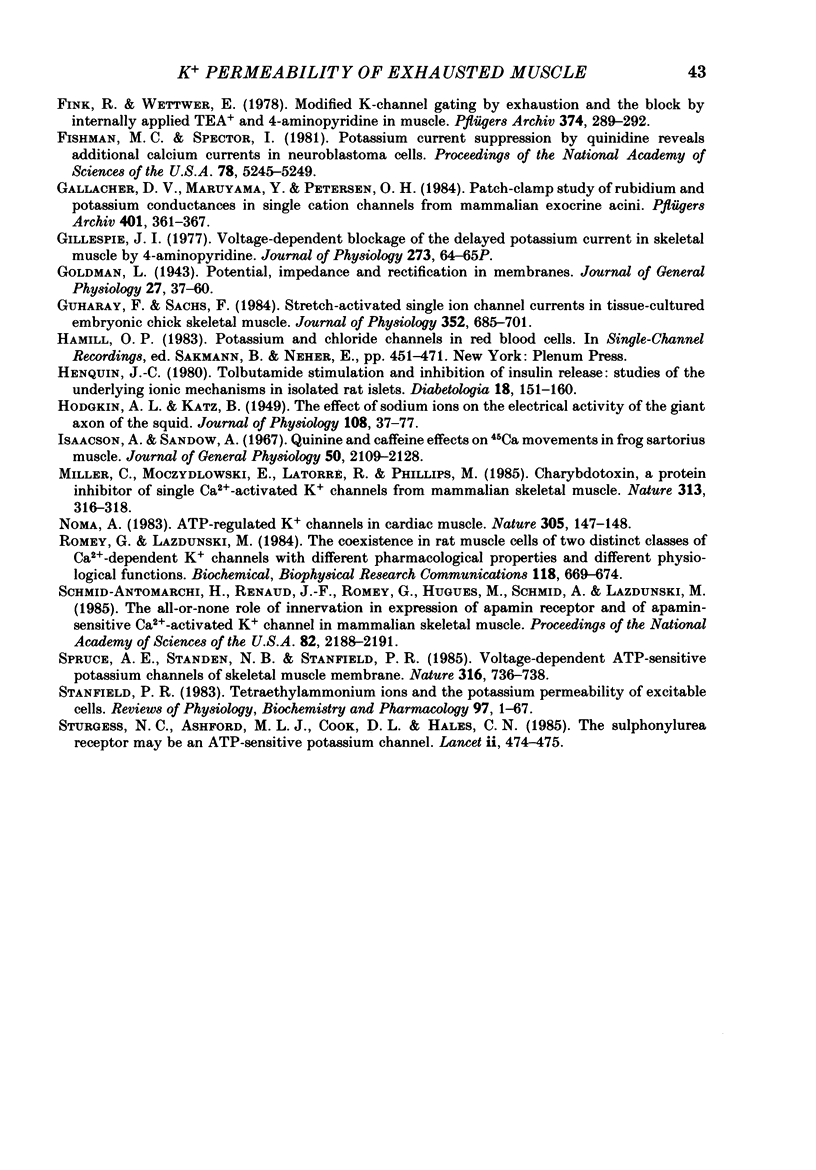
Selected References
These references are in PubMed. This may not be the complete list of references from this article.
- Adams P. R., Sakmann B. Decamethonium both opens and blocks endplate channels. Proc Natl Acad Sci U S A. 1978 Jun;75(6):2994–2998. doi: 10.1073/pnas.75.6.2994. [DOI] [PMC free article] [PubMed] [Google Scholar]
- Almers W. Differential effects of tetracaine on delayed potassium channels and displacement currents in frog skeletal muscle. J Physiol. 1976 Nov;262(3):613–637. doi: 10.1113/jphysiol.1976.sp011612. [DOI] [PMC free article] [PubMed] [Google Scholar]
- Armando-Hardy M., Ellory J. C., Ferreira H. G., Fleminger S., Lew V. L. Inhibition of the calcium-induced increase in the potassium permeability of human red blood cells by quinine. J Physiol. 1975 Aug;250(1):32P–33P. [PubMed] [Google Scholar]
- BLINKS J. R. INFLUENCE OF OSMOTIC STRENGTH ON CROSS-SECTION AND VOLUME OF ISOLATED SINGLE MUSCLE FIBRES. J Physiol. 1965 Mar;177:42–57. doi: 10.1113/jphysiol.1965.sp007574. [DOI] [PMC free article] [PubMed] [Google Scholar]
- Barrett J. N., Barrett E. F., Dribin L. B. Calcium-dependent slow potassium conductance in rat skeletal myotubes. Dev Biol. 1981 Mar;82(2):258–266. doi: 10.1016/0012-1606(81)90450-4. [DOI] [PubMed] [Google Scholar]
- Bolton T. B., Clapp L. H. The diverse effects of noradrenaline and other stimulants on 86Rb and 42K efflux in rabbit and guinea-pig arterial muscle. J Physiol. 1984 Oct;355:43–63. doi: 10.1113/jphysiol.1984.sp015405. [DOI] [PMC free article] [PubMed] [Google Scholar]
- Burgess G. M., Claret M., Jenkinson D. H. Effects of quinine and apamin on the calcium-dependent potassium permeability of mammalian hepatocytes and red cells. J Physiol. 1981 Aug;317:67–90. doi: 10.1113/jphysiol.1981.sp013814. [DOI] [PMC free article] [PubMed] [Google Scholar]
- Cognard C., Traoré F., Potreau D., Raymond G. Effects of apamin on the outward potassium current of isolated frog skeletal muscle fibres. Pflugers Arch. 1984 Oct;402(2):222–224. doi: 10.1007/BF00583339. [DOI] [PubMed] [Google Scholar]
- Cook D. L., Hales C. N. Intracellular ATP directly blocks K+ channels in pancreatic B-cells. Nature. 1984 Sep 20;311(5983):271–273. doi: 10.1038/311271a0. [DOI] [PubMed] [Google Scholar]
- Coronado R., Miller C. Decamethonium and hexamethonium block K+ channels of sarcoplasmic reticulum. Nature. 1980 Dec 4;288(5790):495–497. doi: 10.1038/288495a0. [DOI] [PubMed] [Google Scholar]
- DeWitt L. M., Putney J. W., Jr Alpha-adrenergic stimulation of potassium efflux in guinea-pig hepatocytes may involve calcium influx and calcium release. J Physiol. 1984 Jan;346:395–407. doi: 10.1113/jphysiol.1984.sp015030. [DOI] [PMC free article] [PubMed] [Google Scholar]
- Fink R., Grocki K., Lüttgau H. C. Na/K selectivity, ion conductances and net fluxes of K+ and Na'n metabolically exhausted muscle fibres. Eur J Cell Biol. 1980 Apr;21(1):109–115. [PubMed] [Google Scholar]
- Fink R., Hase S., Lüttgau H. C., Wettwer E. The effect of cellular energy reserves and internal calcium ions on the potassium conductance in skeletal muscle of the frog. J Physiol. 1983 Mar;336:211–228. doi: 10.1113/jphysiol.1983.sp014577. [DOI] [PMC free article] [PubMed] [Google Scholar]
- Fink R., Lüttgau H. C. An evaluation of the membrane constants and the potassium conductance in metabolically exhausted muscle fibres. J Physiol. 1976 Dec;263(2):215–238. doi: 10.1113/jphysiol.1976.sp011629. [DOI] [PMC free article] [PubMed] [Google Scholar]
- Fink R., Wettwer E. Modified K-channel gating by exhaustion and the block by internally applied TEA+ and 4-aminopyridine in muscle. Pflugers Arch. 1978 May 31;374(3):289–292. doi: 10.1007/BF00585607. [DOI] [PubMed] [Google Scholar]
- Fishman M. C., Spector I. Potassium current suppression by quinidine reveals additional calcium currents in neuroblastoma cells. Proc Natl Acad Sci U S A. 1981 Aug;78(8):5245–5249. doi: 10.1073/pnas.78.8.5245. [DOI] [PMC free article] [PubMed] [Google Scholar]
- Gallacher D. V., Maruyama Y., Petersen O. H. Patch-clamp study of rubidium and potassium conductances in single cation channels from mammalian exocrine acini. Pflugers Arch. 1984 Aug;401(4):361–367. doi: 10.1007/BF00584336. [DOI] [PubMed] [Google Scholar]
- Gillespie J. I. Voltage-dependent blockage of the delayed potassium current in skeletal muscle by 4-aminopyridine [proceedings]. J Physiol. 1977 Dec;273(2):64P–65P. [PubMed] [Google Scholar]
- Goldman D. E. POTENTIAL, IMPEDANCE, AND RECTIFICATION IN MEMBRANES. J Gen Physiol. 1943 Sep 20;27(1):37–60. doi: 10.1085/jgp.27.1.37. [DOI] [PMC free article] [PubMed] [Google Scholar]
- Guharay F., Sachs F. Stretch-activated single ion channel currents in tissue-cultured embryonic chick skeletal muscle. J Physiol. 1984 Jul;352:685–701. doi: 10.1113/jphysiol.1984.sp015317. [DOI] [PMC free article] [PubMed] [Google Scholar]
- HODGKIN A. L., KATZ B. The effect of sodium ions on the electrical activity of giant axon of the squid. J Physiol. 1949 Mar 1;108(1):37–77. doi: 10.1113/jphysiol.1949.sp004310. [DOI] [PMC free article] [PubMed] [Google Scholar]
- Henquin J. C. Tolbutamide stimulation and inhibition of insulin release: studies of the underlying ionic mechanisms in isolated rat islets. Diabetologia. 1980;18(2):151–160. doi: 10.1007/BF00290493. [DOI] [PubMed] [Google Scholar]
- Isaacson A., Sandow A. Quinine and caffeine effects on 45Ca movements in frog sartorius muscle. J Gen Physiol. 1967 Sep;50(8):2109–2128. doi: 10.1085/jgp.50.8.2109. [DOI] [PMC free article] [PubMed] [Google Scholar]
- Miller C., Moczydlowski E., Latorre R., Phillips M. Charybdotoxin, a protein inhibitor of single Ca2+-activated K+ channels from mammalian skeletal muscle. Nature. 1985 Jan 24;313(6000):316–318. doi: 10.1038/313316a0. [DOI] [PubMed] [Google Scholar]
- Noma A. ATP-regulated K+ channels in cardiac muscle. Nature. 1983 Sep 8;305(5930):147–148. doi: 10.1038/305147a0. [DOI] [PubMed] [Google Scholar]
- Romey G., Lazdunski M. The coexistence in rat muscle cells of two distinct classes of Ca2+-dependent K+ channels with different pharmacological properties and different physiological functions. Biochem Biophys Res Commun. 1984 Jan 30;118(2):669–674. doi: 10.1016/0006-291x(84)91355-x. [DOI] [PubMed] [Google Scholar]
- Schmid-Antomarchi H., Renaud J. F., Romey G., Hugues M., Schmid A., Lazdunski M. The all-or-none role of innervation in expression of apamin receptor and of apamin-sensitive Ca2+-activated K+ channel in mammalian skeletal muscle. Proc Natl Acad Sci U S A. 1985 Apr;82(7):2188–2191. doi: 10.1073/pnas.82.7.2188. [DOI] [PMC free article] [PubMed] [Google Scholar]
- Spruce A. E., Standen N. B., Stanfield P. R. Voltage-dependent ATP-sensitive potassium channels of skeletal muscle membrane. Nature. 1985 Aug 22;316(6030):736–738. doi: 10.1038/316736a0. [DOI] [PubMed] [Google Scholar]
- Stanfield P. R. Tetraethylammonium ions and the potassium permeability of excitable cells. Rev Physiol Biochem Pharmacol. 1983;97:1–67. doi: 10.1007/BFb0035345. [DOI] [PubMed] [Google Scholar]
- Sturgess N. C., Ashford M. L., Cook D. L., Hales C. N. The sulphonylurea receptor may be an ATP-sensitive potassium channel. Lancet. 1985 Aug 31;2(8453):474–475. doi: 10.1016/s0140-6736(85)90403-9. [DOI] [PubMed] [Google Scholar]