Abstract
1. Tension and intracellular calcium concentration ([Ca2+]i) were measured in isolated ferret papillary muscles exposed to hypertonic solutions. [Ca2+]i was measured with aequorin which was microinjected into surface cells of the preparation. Correction was made for the effects of ionic strength on aequorin sensitivity to Ca2+. 2. Application of 100 mM-mannitol increased both developed tension and the intracellular Ca2+ signals on contraction (the Ca2+ transients). 300 mM-mannitol increased the Ca2+ transients further but led to a decrease in developed tension. 3. Mannitol caused a concentration-dependent slowing in the time course of a stimulated contraction but had no effect on that of the Ca2+ transient. 4. As the mannitol concentration was increased, the muscles exhibited increased viscosity which was demonstrated by measuring the tension response to a sudden stretch during diastole. This is probably a consequence of cell shrinkage and may cause the slower time course of the contraction. 5. In the presence of 300 mM-mannitol, oscillations of diastolic [Ca2+]i were detectable in both stimulated and quiescent preparations. However, in stimulated preparations the oscillations in mannitol were smaller than when a Ca2+ transient of similar amplitude was achieved by other means. 6. Immediately after the application or removal of mannitol large spontaneous Ca2+ signals were often observed. These signals were even larger in Na+-free solutions, suggesting that they cannot be attributed to Na+-Ca2+ exchange. 7. The increase in developed tension in 100 mM-mannitol can be accounted for by the increased Ca2+ transients in combination with the inhibitory effects of ionic strength on myofibrillar tension production (Kentish, 1984). The decrease in developed tension at 300 mM-mannitol is dominated by the inhibitory effect of increased ionic strength on maximum Ca2+-activated tension.
Full text
PDF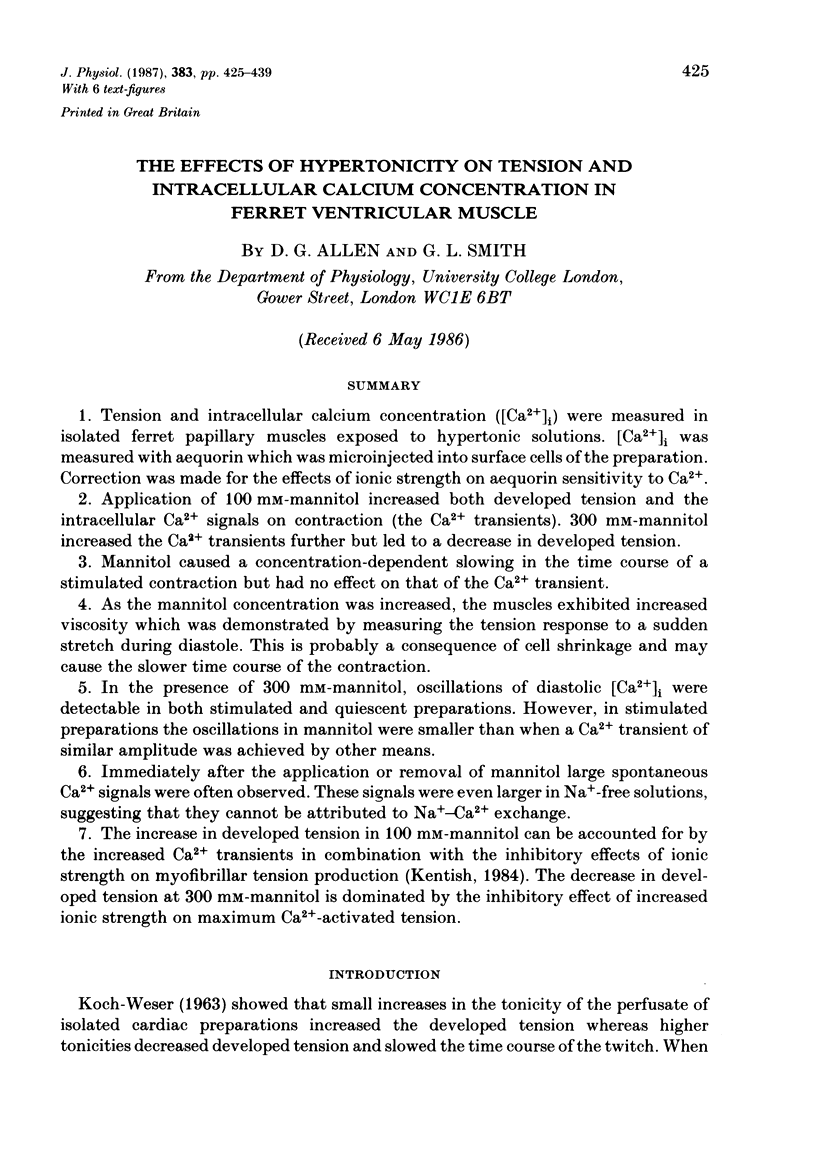
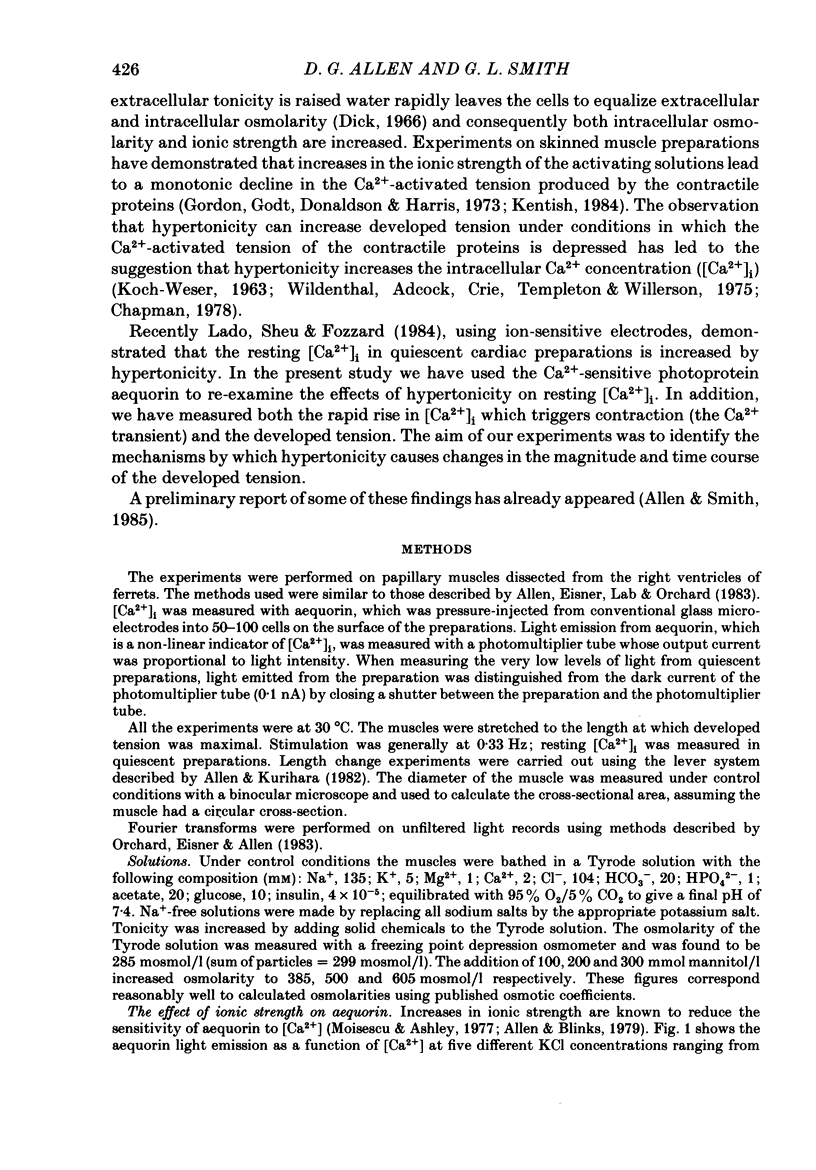
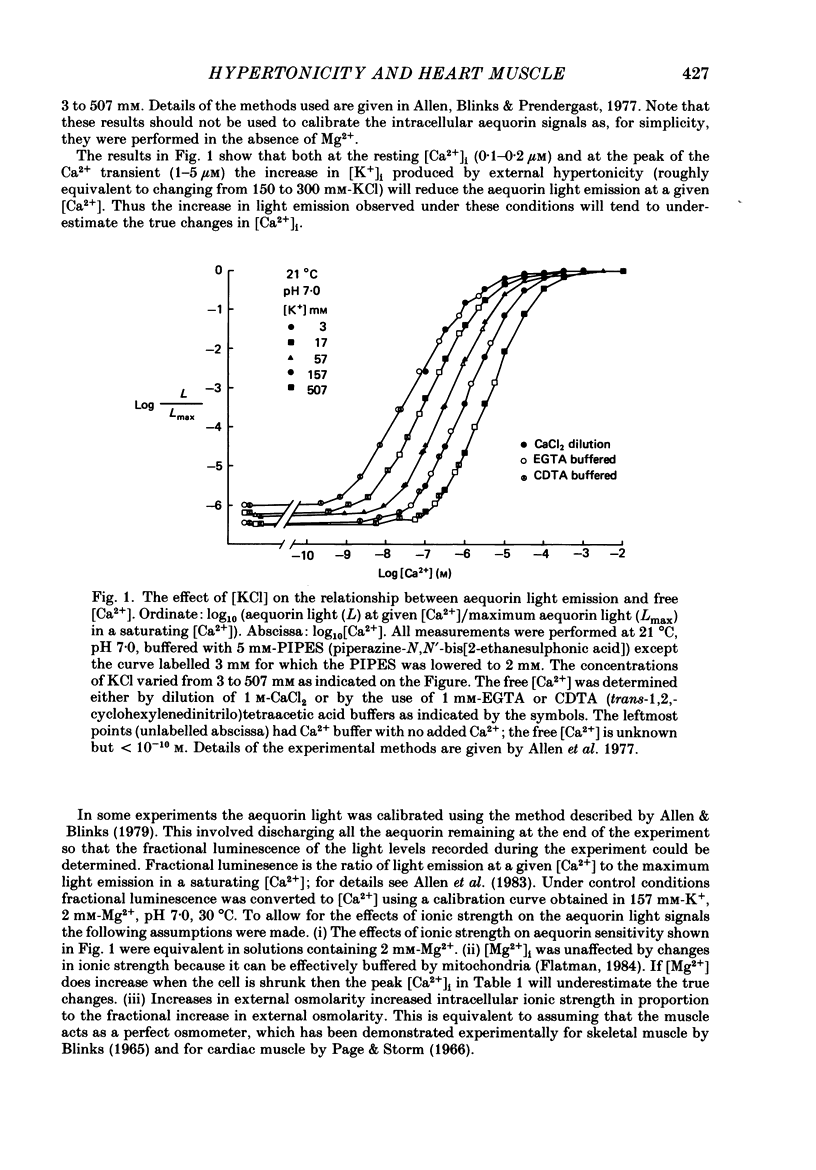
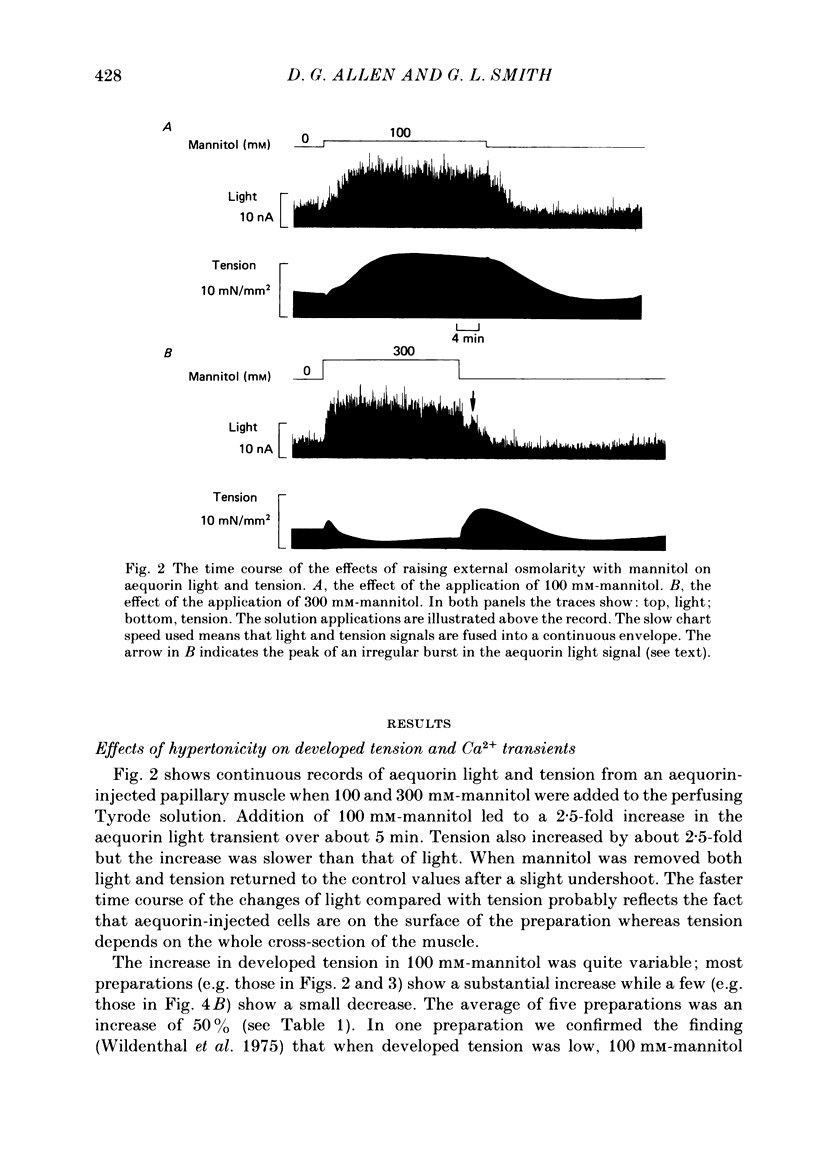
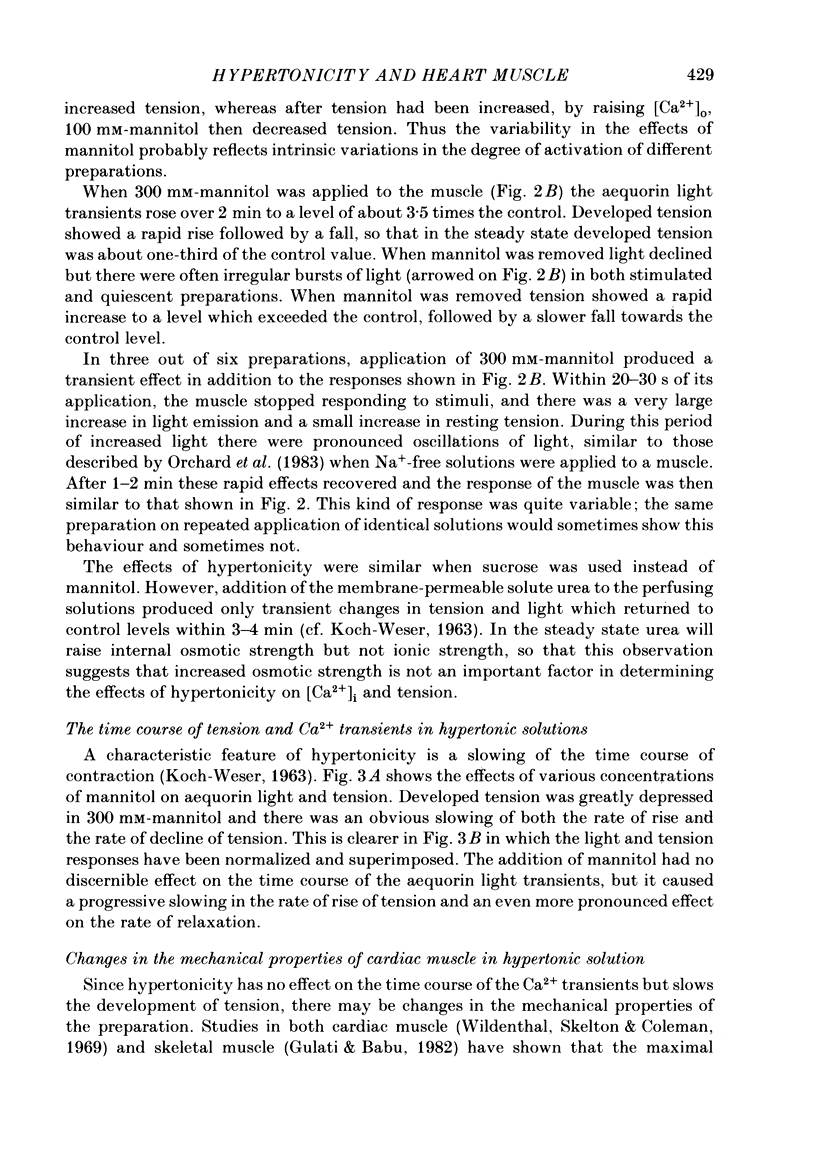
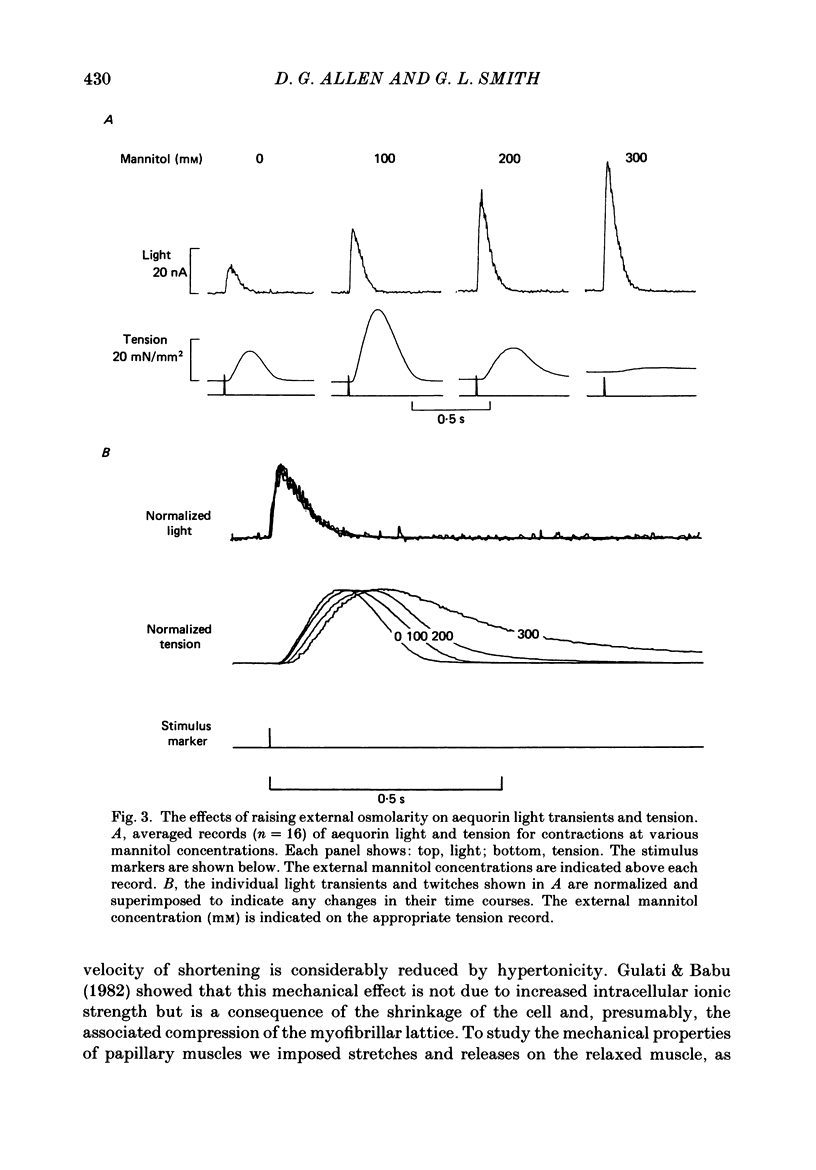
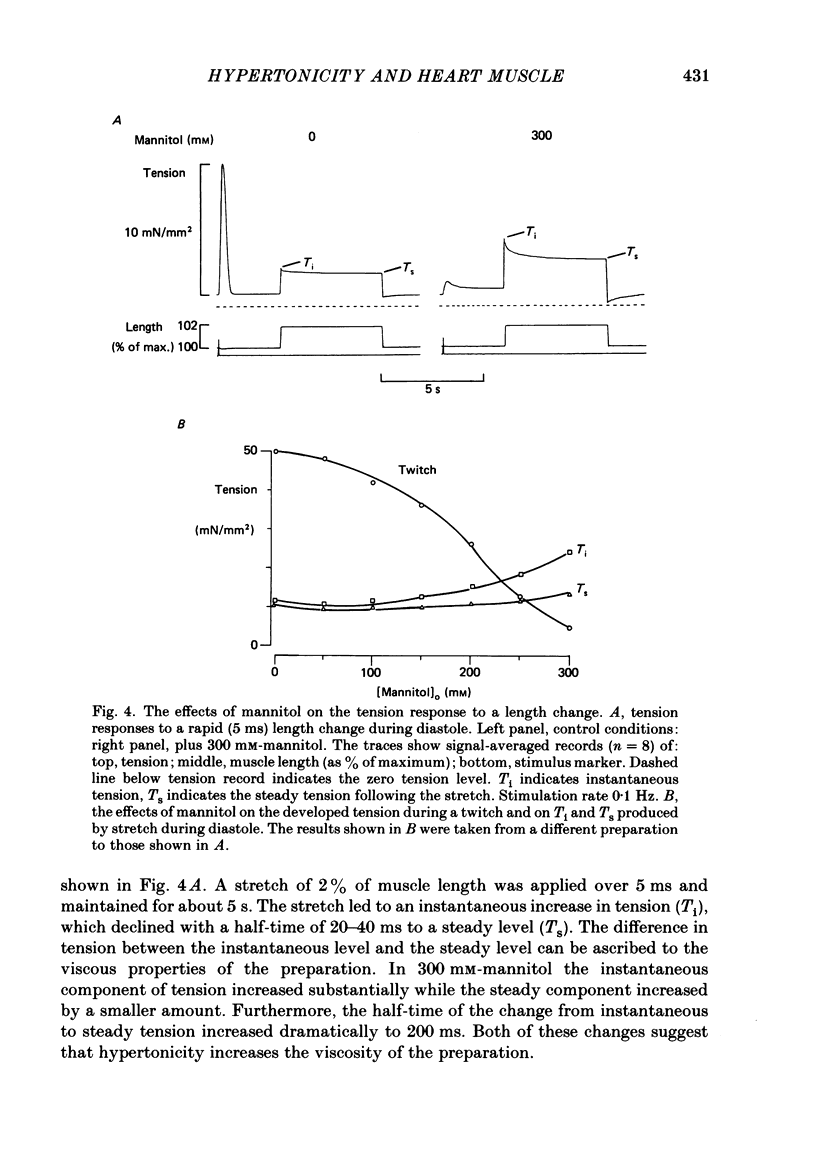
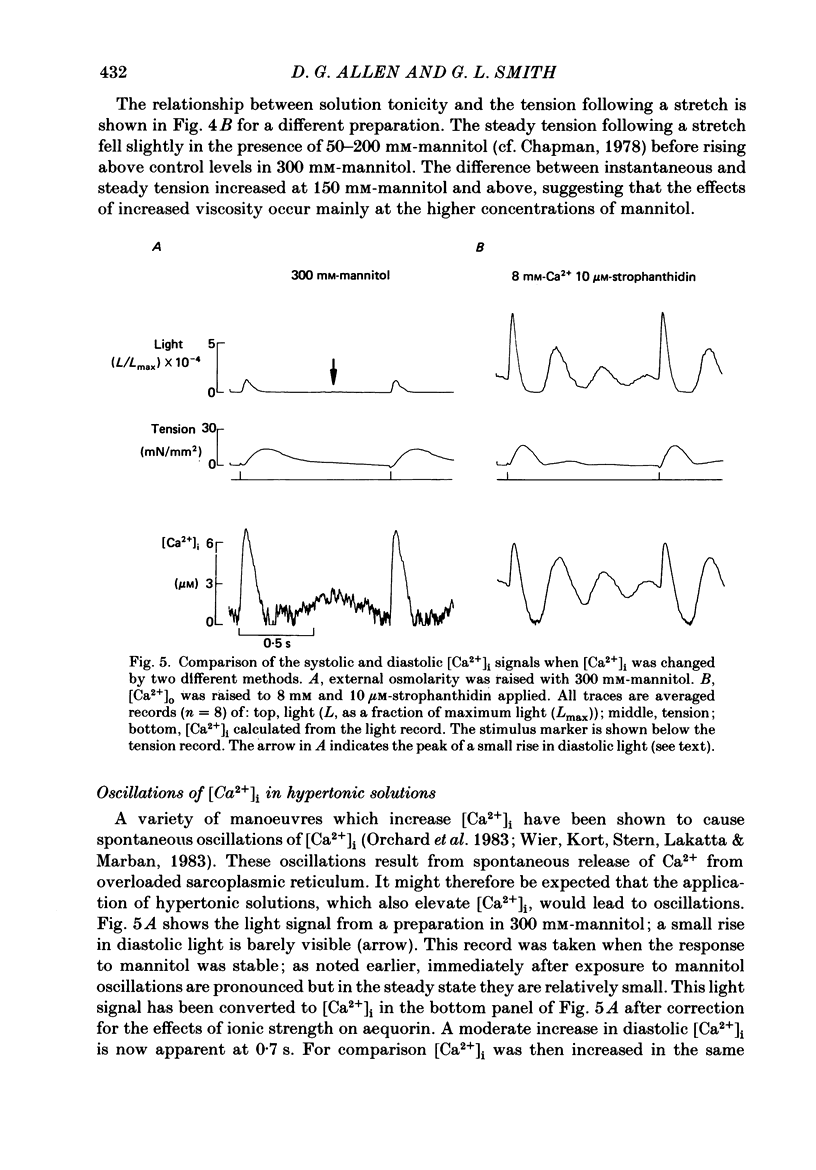
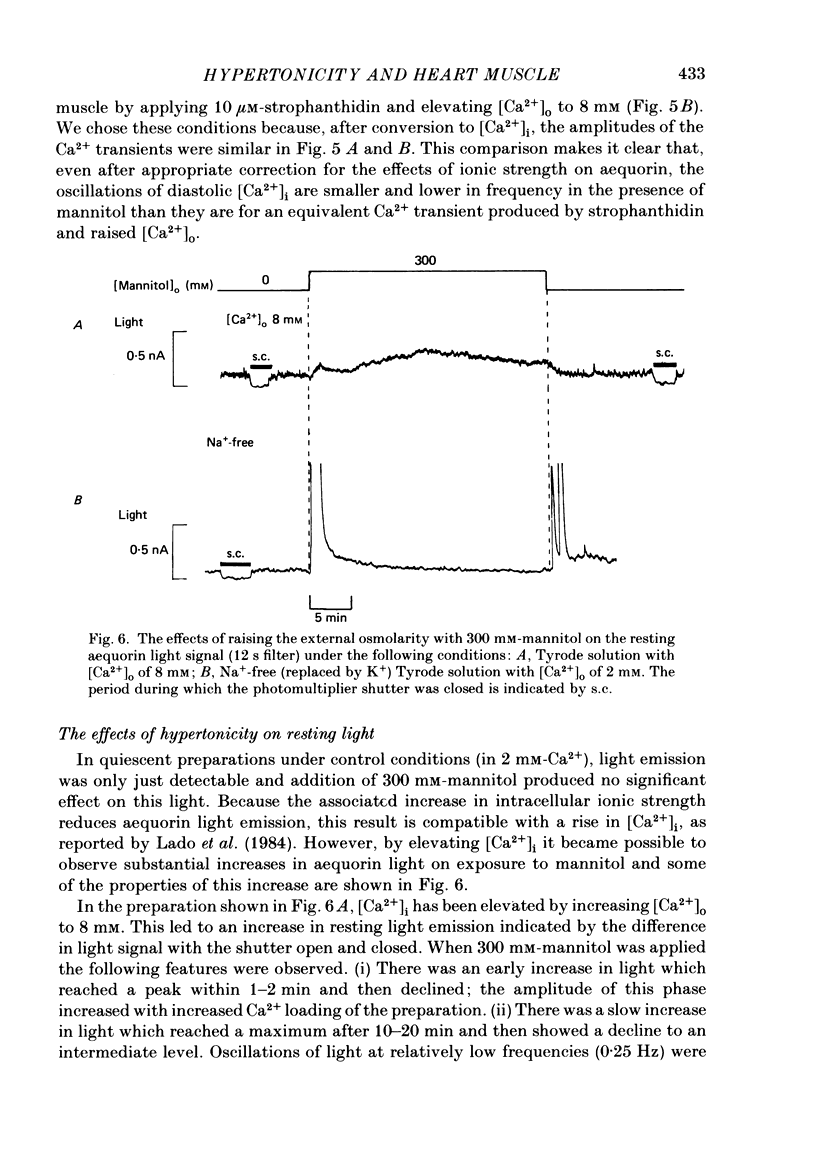
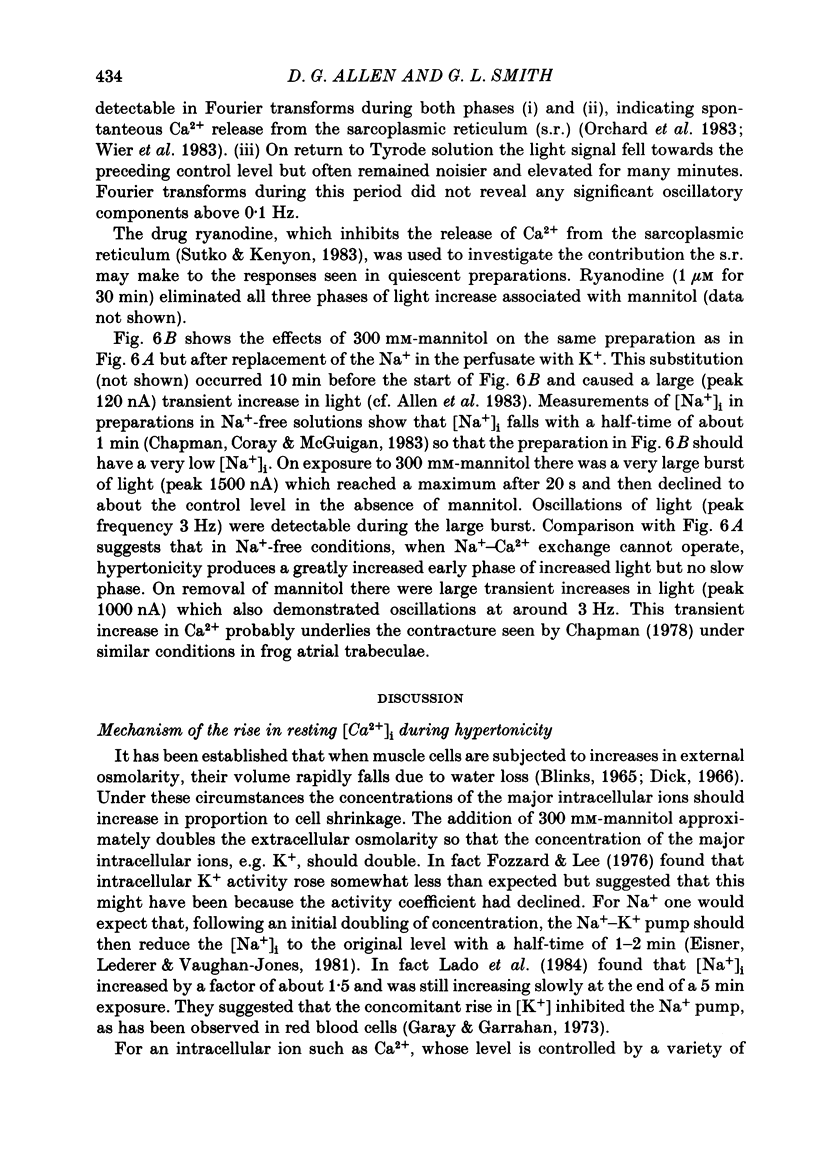
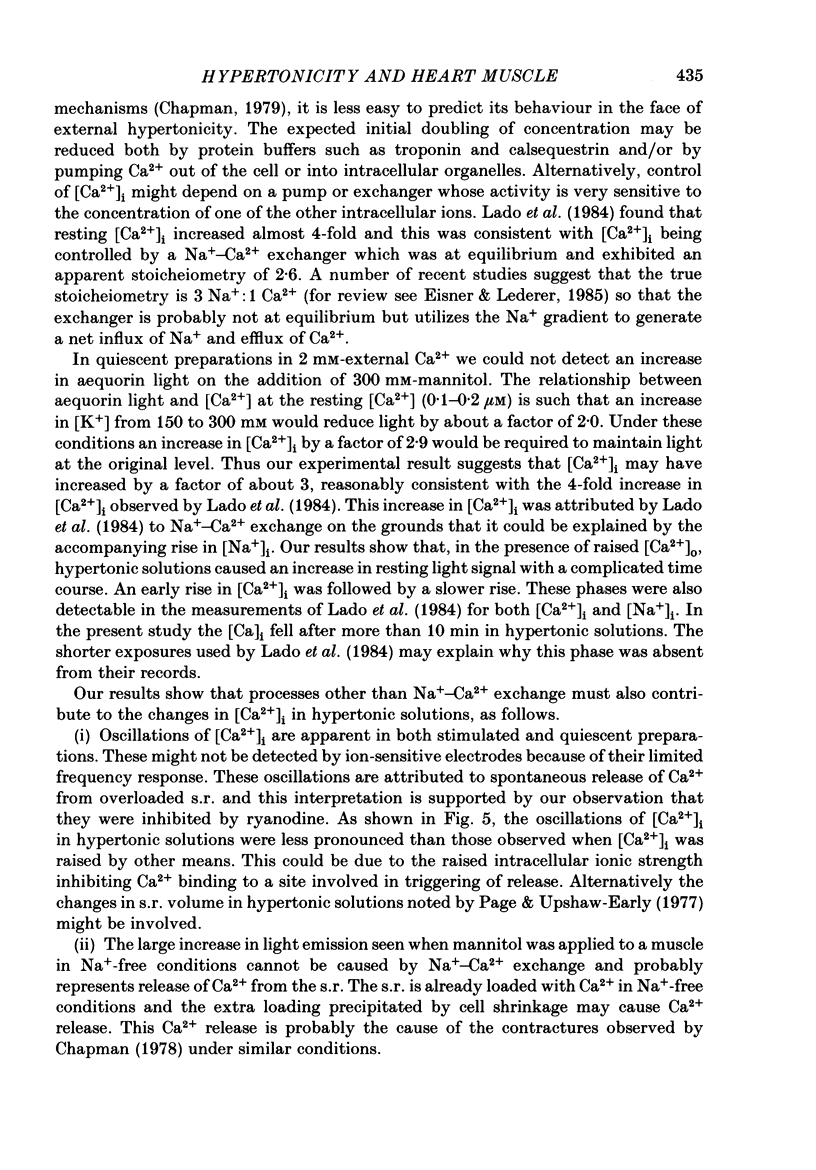
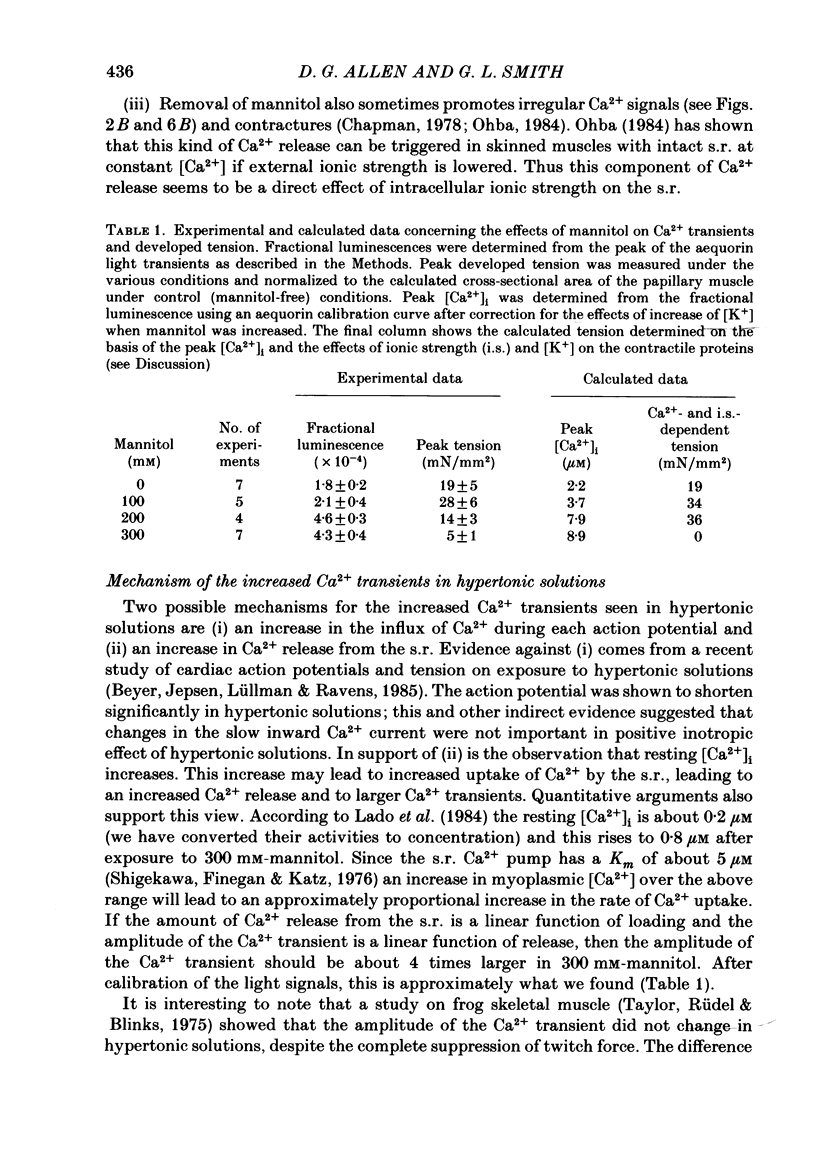
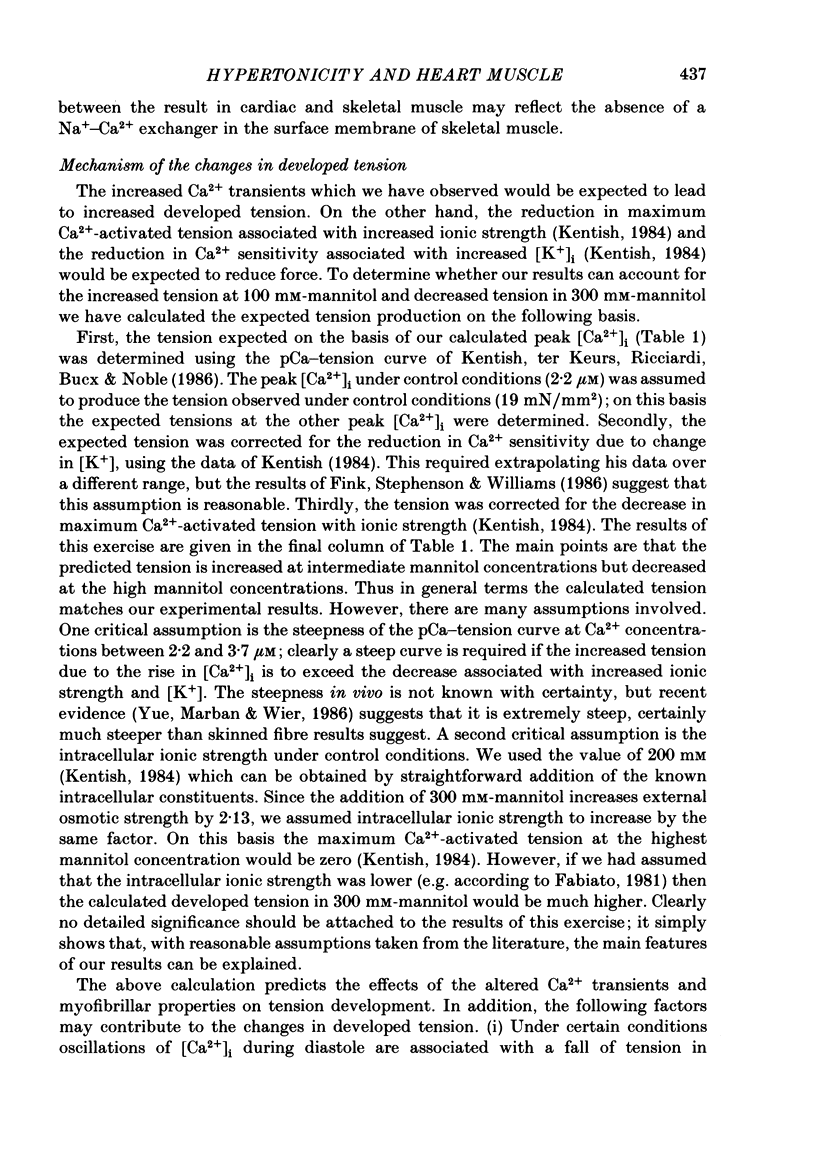
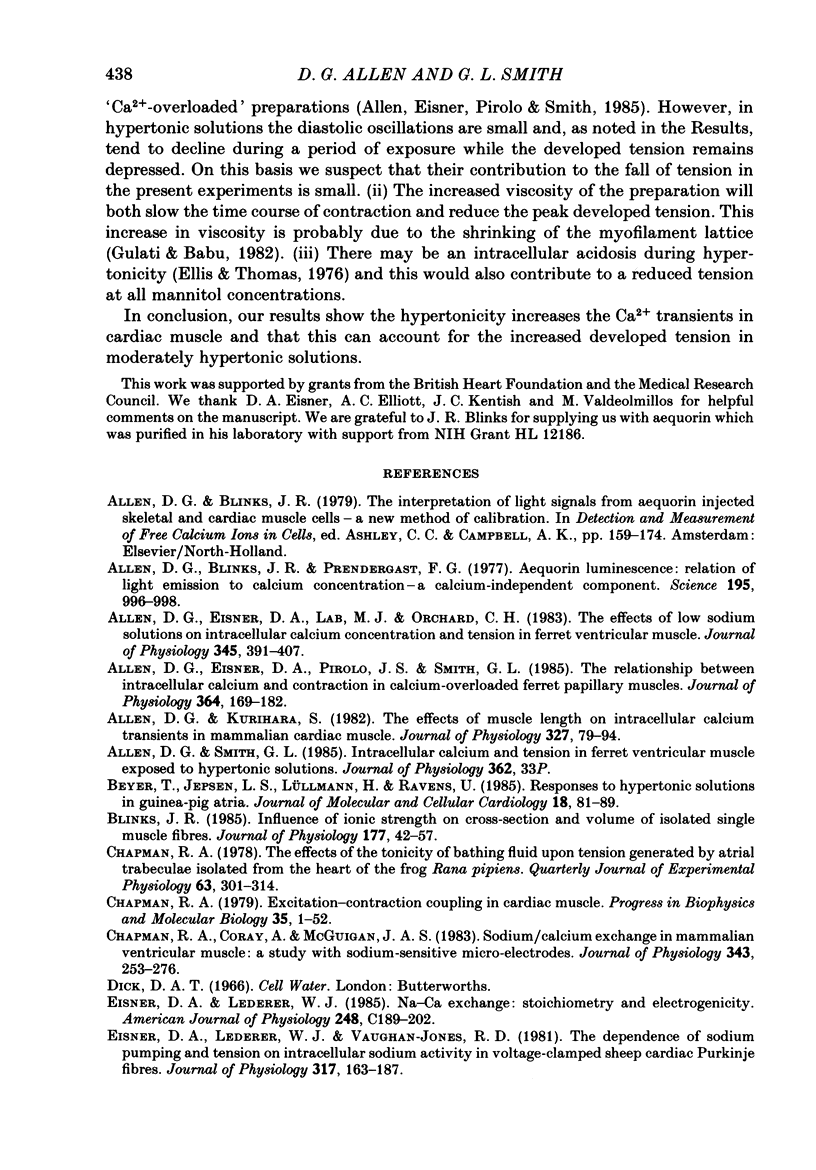
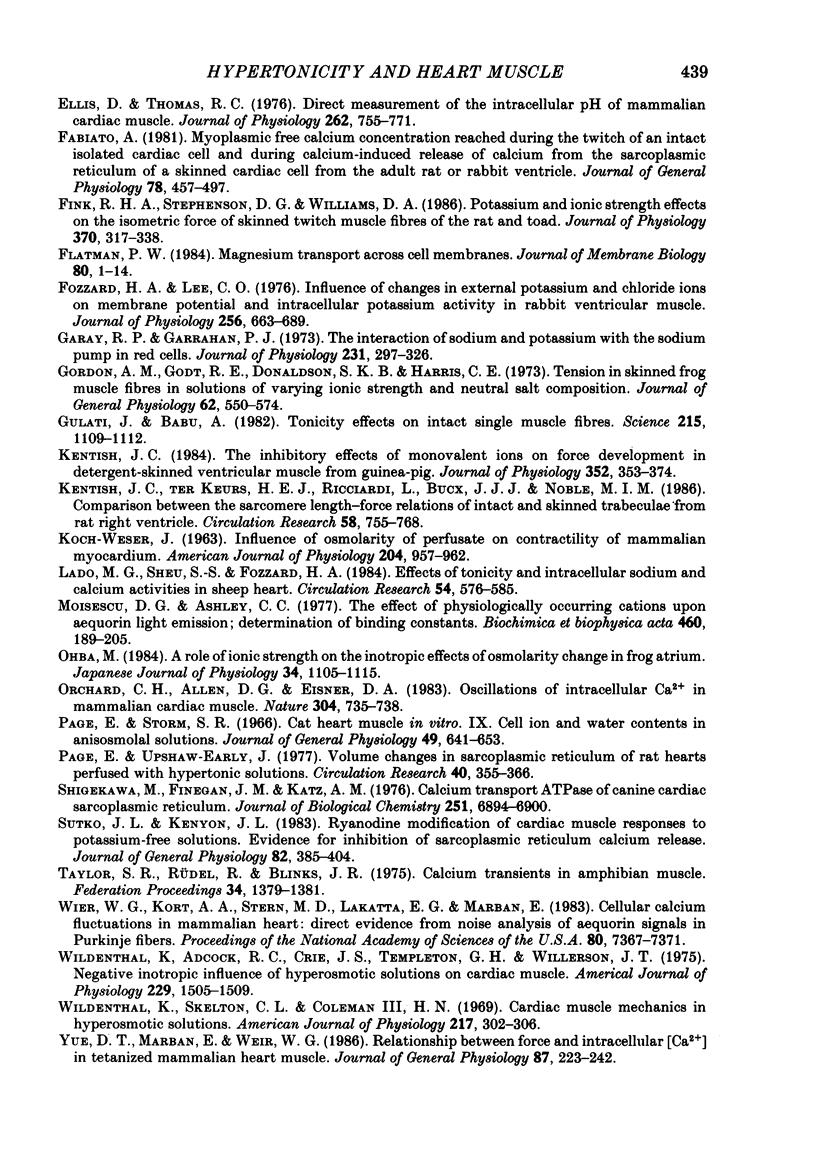
Selected References
These references are in PubMed. This may not be the complete list of references from this article.
- Allen D. G., Blinks J. R., Prendergast F. G. Aequorin luminescence: relation of light emission to calcium concentration--a calcium-independent component. Science. 1977 Mar 11;195(4282):996–998. doi: 10.1126/science.841325. [DOI] [PubMed] [Google Scholar]
- Allen D. G., Eisner D. A., Lab M. J., Orchard C. H. The effects of low sodium solutions on intracellular calcium concentration and tension in ferret ventricular muscle. J Physiol. 1983 Dec;345:391–407. doi: 10.1113/jphysiol.1983.sp014984. [DOI] [PMC free article] [PubMed] [Google Scholar]
- Allen D. G., Eisner D. A., Pirolo J. S., Smith G. L. The relationship between intracellular calcium and contraction in calcium-overloaded ferret papillary muscles. J Physiol. 1985 Jul;364:169–182. doi: 10.1113/jphysiol.1985.sp015737. [DOI] [PMC free article] [PubMed] [Google Scholar]
- Allen D. G., Kurihara S. The effects of muscle length on intracellular calcium transients in mammalian cardiac muscle. J Physiol. 1982 Jun;327:79–94. doi: 10.1113/jphysiol.1982.sp014221. [DOI] [PMC free article] [PubMed] [Google Scholar]
- BLINKS J. R. INFLUENCE OF OSMOTIC STRENGTH ON CROSS-SECTION AND VOLUME OF ISOLATED SINGLE MUSCLE FIBRES. J Physiol. 1965 Mar;177:42–57. doi: 10.1113/jphysiol.1965.sp007574. [DOI] [PMC free article] [PubMed] [Google Scholar]
- Beyer T., Jepsen L. S., Lüllmann H., Ravens U. Responses to hypertonic solutions in guinea-pig atria: changes in action potentials, force of contraction and calcium content. J Mol Cell Cardiol. 1986 Jan;18(1):81–89. doi: 10.1016/s0022-2828(86)80985-3. [DOI] [PubMed] [Google Scholar]
- Chapman R. A., Coray A., McGuigan J. A. Sodium/calcium exchange in mammalian ventricular muscle: a study with sodium-sensitive micro-electrodes. J Physiol. 1983 Oct;343:253–276. doi: 10.1113/jphysiol.1983.sp014891. [DOI] [PMC free article] [PubMed] [Google Scholar]
- Chapman R. A. Excitation-contraction coupling in cardiac muscle. Prog Biophys Mol Biol. 1979;35(1):1–52. doi: 10.1016/0079-6107(80)90002-4. [DOI] [PubMed] [Google Scholar]
- Chapman R. A. The effects of changes of the tonicity of the bathing fluid upon the tension generated by atrial trabeculae isolated from the heart of the frog, Rana pipiens. Q J Exp Physiol Cogn Med Sci. 1978 Oct;63(4):301–314. doi: 10.1113/expphysiol.1978.sp002444. [DOI] [PubMed] [Google Scholar]
- Eisner D. A., Lederer W. J. Na-Ca exchange: stoichiometry and electrogenicity. Am J Physiol. 1985 Mar;248(3 Pt 1):C189–C202. doi: 10.1152/ajpcell.1985.248.3.C189. [DOI] [PubMed] [Google Scholar]
- Eisner D. A., Lederer W. J., Vaughan-Jones R. D. The dependence of sodium pumping and tension on intracellular sodium activity in voltage-clamped sheep Purkinje fibres. J Physiol. 1981 Aug;317:163–187. doi: 10.1113/jphysiol.1981.sp013819. [DOI] [PMC free article] [PubMed] [Google Scholar]
- Ellis D., Thomas R. C. Direct measurement of the intracellular pH of mammalian cardiac muscle. J Physiol. 1976 Nov;262(3):755–771. doi: 10.1113/jphysiol.1976.sp011619. [DOI] [PMC free article] [PubMed] [Google Scholar]
- Fabiato A. Myoplasmic free calcium concentration reached during the twitch of an intact isolated cardiac cell and during calcium-induced release of calcium from the sarcoplasmic reticulum of a skinned cardiac cell from the adult rat or rabbit ventricle. J Gen Physiol. 1981 Nov;78(5):457–497. doi: 10.1085/jgp.78.5.457. [DOI] [PMC free article] [PubMed] [Google Scholar]
- Fink R. H., Stephenson D. G., Williams D. A. Potassium and ionic strength effects on the isometric force of skinned twitch muscle fibres of the rat and toad. J Physiol. 1986 Jan;370:317–337. doi: 10.1113/jphysiol.1986.sp015937. [DOI] [PMC free article] [PubMed] [Google Scholar]
- Flatman P. W. Magnesium transport across cell membranes. J Membr Biol. 1984;80(1):1–14. doi: 10.1007/BF01868686. [DOI] [PubMed] [Google Scholar]
- Fozzard H. A., Lee C. O. Influence of changes in external potassium and chloride ions on membrane potential and intracellular potassium ion activity in rabbit ventricular muscle. J Physiol. 1976 Apr;256(3):663–689. doi: 10.1113/jphysiol.1976.sp011345. [DOI] [PMC free article] [PubMed] [Google Scholar]
- Garay R. P., Garrahan P. J. The interaction of sodium and potassium with the sodium pump in red cells. J Physiol. 1973 Jun;231(2):297–325. doi: 10.1113/jphysiol.1973.sp010234. [DOI] [PMC free article] [PubMed] [Google Scholar]
- Gordon A. M., Godt R. E., Donaldson S. K., Harris C. E. Tension in skinned frog muscle fibers in solutions of varying ionic strength and neutral salt composition. J Gen Physiol. 1973 Nov;62(5):550–574. doi: 10.1085/jgp.62.5.550. [DOI] [PMC free article] [PubMed] [Google Scholar]
- Gulati J., Babu A. Tonicity effects on intact single muscle fibers: relation between force and cell volume. Science. 1982 Feb 26;215(4536):1109–1112. doi: 10.1126/science.6977845. [DOI] [PubMed] [Google Scholar]
- KOCH-WESER J. Influence of osmolarity of perfusate on contractility of mammalian myocardium. Am J Physiol. 1963 Jun;204:957–962. doi: 10.1152/ajplegacy.1963.204.6.957. [DOI] [PubMed] [Google Scholar]
- Kentish J. C. The inhibitory effects of monovalent ions on force development in detergent-skinned ventricular muscle from guinea-pig. J Physiol. 1984 Jul;352:353–374. doi: 10.1113/jphysiol.1984.sp015296. [DOI] [PMC free article] [PubMed] [Google Scholar]
- Kentish J. C., ter Keurs H. E., Ricciardi L., Bucx J. J., Noble M. I. Comparison between the sarcomere length-force relations of intact and skinned trabeculae from rat right ventricle. Influence of calcium concentrations on these relations. Circ Res. 1986 Jun;58(6):755–768. doi: 10.1161/01.res.58.6.755. [DOI] [PubMed] [Google Scholar]
- Lado M. G., Sheu S. S., Fozzard H. A. Effects of tonicity on tension and intracellular sodium and calcium activities in sheep heart. Circ Res. 1984 May;54(5):576–585. doi: 10.1161/01.res.54.5.576. [DOI] [PubMed] [Google Scholar]
- Moisescu D. G., Ashley C. C. The effect of physiologically occurring cations upon aequorin light emission. Determination of the binding constants. Biochim Biophys Acta. 1977 May 11;460(2):189–205. doi: 10.1016/0005-2728(77)90206-7. [DOI] [PubMed] [Google Scholar]
- Ohba M. A role of ionic strength on the inotropic effects of osmolarity change in frog atrium. Jpn J Physiol. 1984;34(6):1105–1115. doi: 10.2170/jjphysiol.34.1105. [DOI] [PubMed] [Google Scholar]
- Orchard C. H., Eisner D. A., Allen D. G. Oscillations of intracellular Ca2+ in mammalian cardiac muscle. Nature. 1983 Aug 25;304(5928):735–738. doi: 10.1038/304735a0. [DOI] [PubMed] [Google Scholar]
- Page E., Storm S. R. Cat heart muscle in vitro. IX. Cell ion and water contents in anisosmolal solutions. J Gen Physiol. 1966 Mar;49(4):641–653. doi: 10.1085/jgp.49.4.641. [DOI] [PMC free article] [PubMed] [Google Scholar]
- Page E., Upshaw-Earley J. Volume changes in sarcoplasmic reticulum of rat hearts perfused with hypertonic solutions. Circ Res. 1977 Apr;40(4):355–366. doi: 10.1161/01.res.40.4.355. [DOI] [PubMed] [Google Scholar]
- Shigekawa M., Finegan J. A., Katz A. M. Calcium transport ATPase of canine cardiac sarcoplasmic reticulum. A comparison with that of rabbit fast skeletal muscle sarcoplasmic reticulum. J Biol Chem. 1976 Nov 25;251(22):6894–6900. [PubMed] [Google Scholar]
- Sutko J. L., Kenyon J. L. Ryanodine modification of cardiac muscle responses to potassium-free solutions. Evidence for inhibition of sarcoplasmic reticulum calcium release. J Gen Physiol. 1983 Sep;82(3):385–404. doi: 10.1085/jgp.82.3.385. [DOI] [PMC free article] [PubMed] [Google Scholar]
- Taylor S. R., Rüdel R., Blinks J. R. Calcium transients in amphibian muscle. Fed Proc. 1975 Apr;34(5):1379–1381. [PubMed] [Google Scholar]
- Wier W. G., Kort A. A., Stern M. D., Lakatta E. G., Marban E. Cellular calcium fluctuations in mammalian heart: direct evidence from noise analysis of aequorin signals in Purkinje fibers. Proc Natl Acad Sci U S A. 1983 Dec;80(23):7367–7371. doi: 10.1073/pnas.80.23.7367. [DOI] [PMC free article] [PubMed] [Google Scholar]
- Wildenthal K., Adcock R. C., Crie J. S., Templeton G. H., Willerson J. T. Negative inotropic influence of hyperosmotic solutions on cardiac muscle. Am J Physiol. 1975 Dec;229(6):1505–1509. doi: 10.1152/ajplegacy.1975.229.6.1505. [DOI] [PubMed] [Google Scholar]
- Wildenthal K., Skelton C. L., Coleman H. N., 3rd Cardiac muscle mechanics in hyperosmotic solutions. Am J Physiol. 1969 Jul;217(1):302–306. doi: 10.1152/ajplegacy.1969.217.1.302. [DOI] [PubMed] [Google Scholar]
- Yue D. T., Marban E., Wier W. G. Relationship between force and intracellular [Ca2+] in tetanized mammalian heart muscle. J Gen Physiol. 1986 Feb;87(2):223–242. doi: 10.1085/jgp.87.2.223. [DOI] [PMC free article] [PubMed] [Google Scholar]