Abstract
The rate of muscle force redevelopment after release-restretch protocols has previously been interpreted using a simple two-state cross-bridge cycling model with rate constants for transitions between non-force-bearing and force-bearing states, f, and between force-bearing and non-force-bearing states, g. Changes in the rate constant of force redevelopment, as with varying levels of Ca2+ activation, have traditionally been attributed to Ca(2+)-dependent f. The current work adds to this original model a state of unactivated, noncycling cross-bridges. The resulting differential equation for activated, force-bearing cross-bridges, Ncf, was Ncf = -[g+f(K/(K + 1))] Ncf+f(K/(K + 1))NT, where K is an equilibrium constant defining the distribution between cycling and noncycling cross-bridges and NT is the total number of cross-bridges. Cooperativity by which force-bearing cross-bridges participate in their own activation was introduced by making K depend on Ncf. Model results demonstrated that such cooperativity, which tends to enhance force generation at low levels of Ca2+ activation, has a counter-intuitive effect of slowing force redevelopment. These dynamic effects of cooperativity are most pronounced at low Ca2+ activation. As Ca2+ activation increases, the cooperative effects become less important to the dynamics of force redevelopment and, at the highest levels of Ca2+ activation, the dynamics of force redevelopment reflect factors other than cooperative mechanisms. These results expand on earlier interpretations of Ca2+ dependence of force redevelopment; rather than Ca(2+)-dependent f, Ca(2+)-dependent force redevelopment arises from changing expressions of cooperativity between force-bearing cross-bridges and activation.
Full text
PDF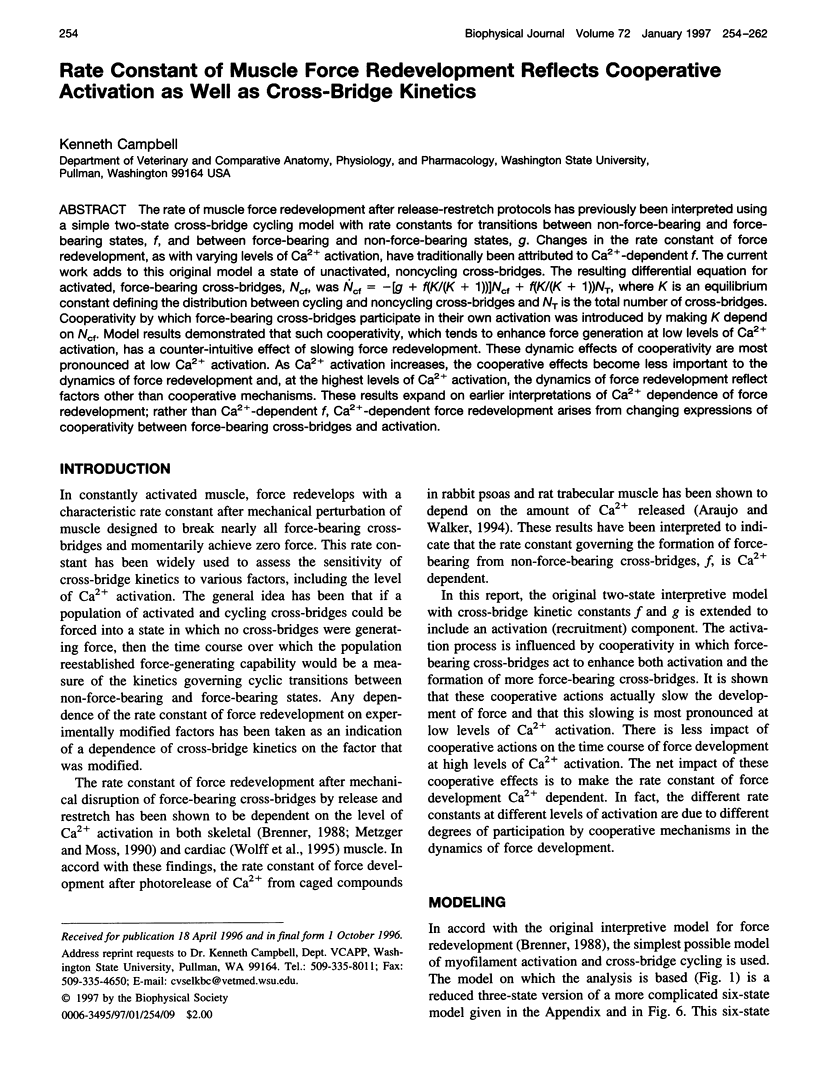
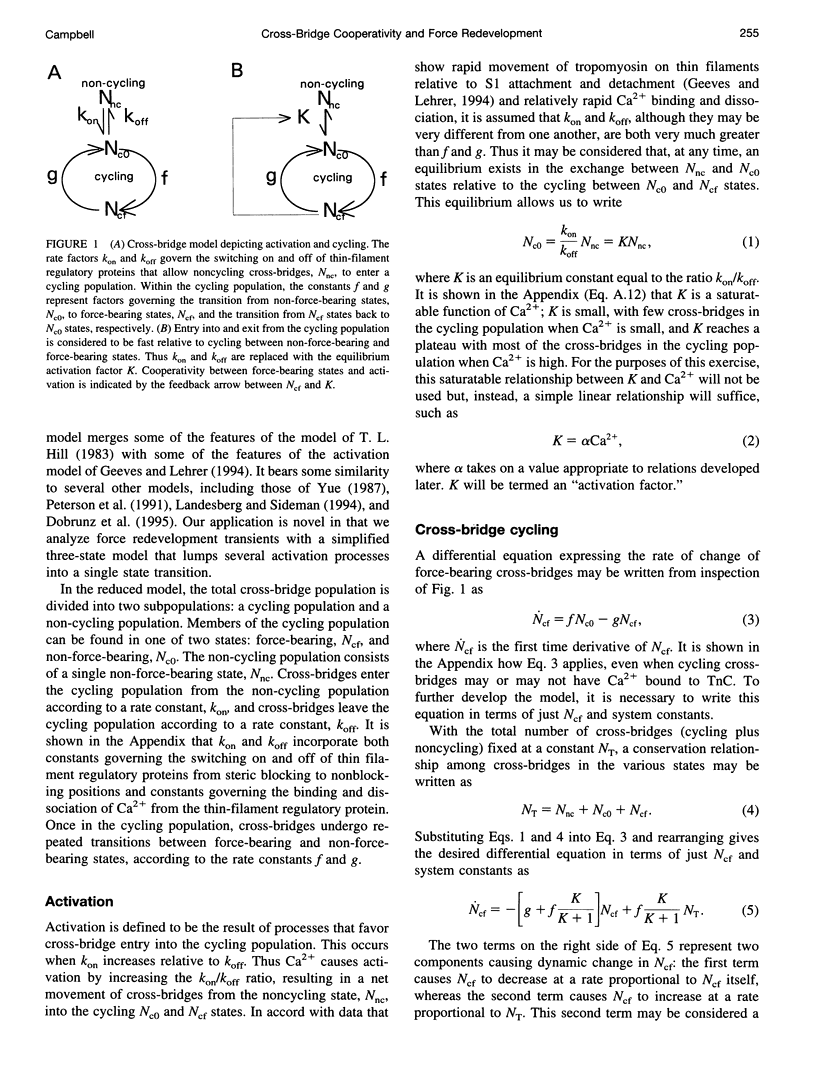
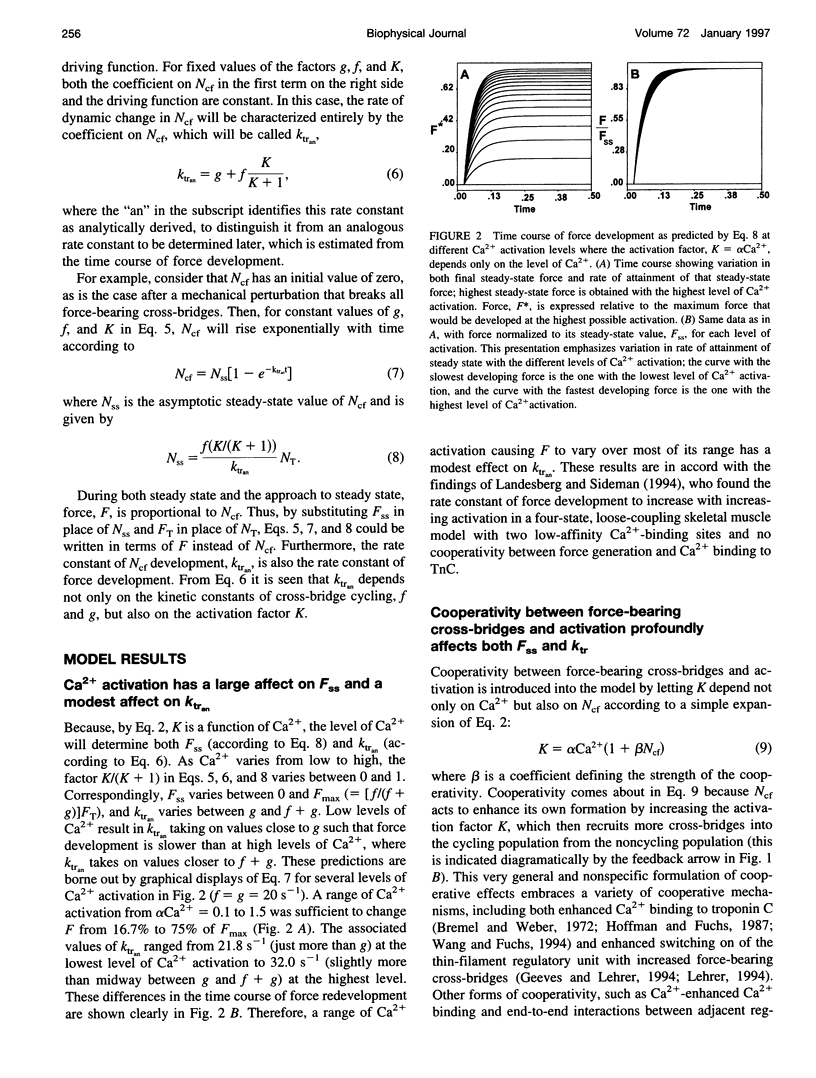
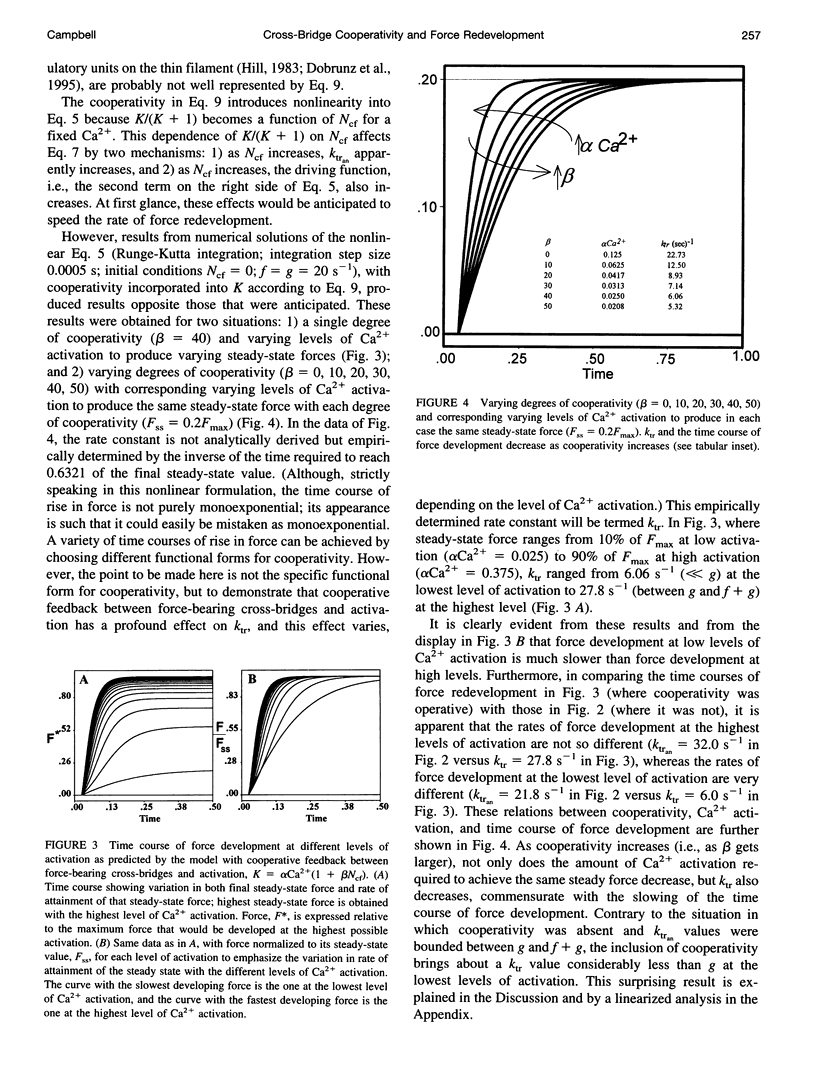
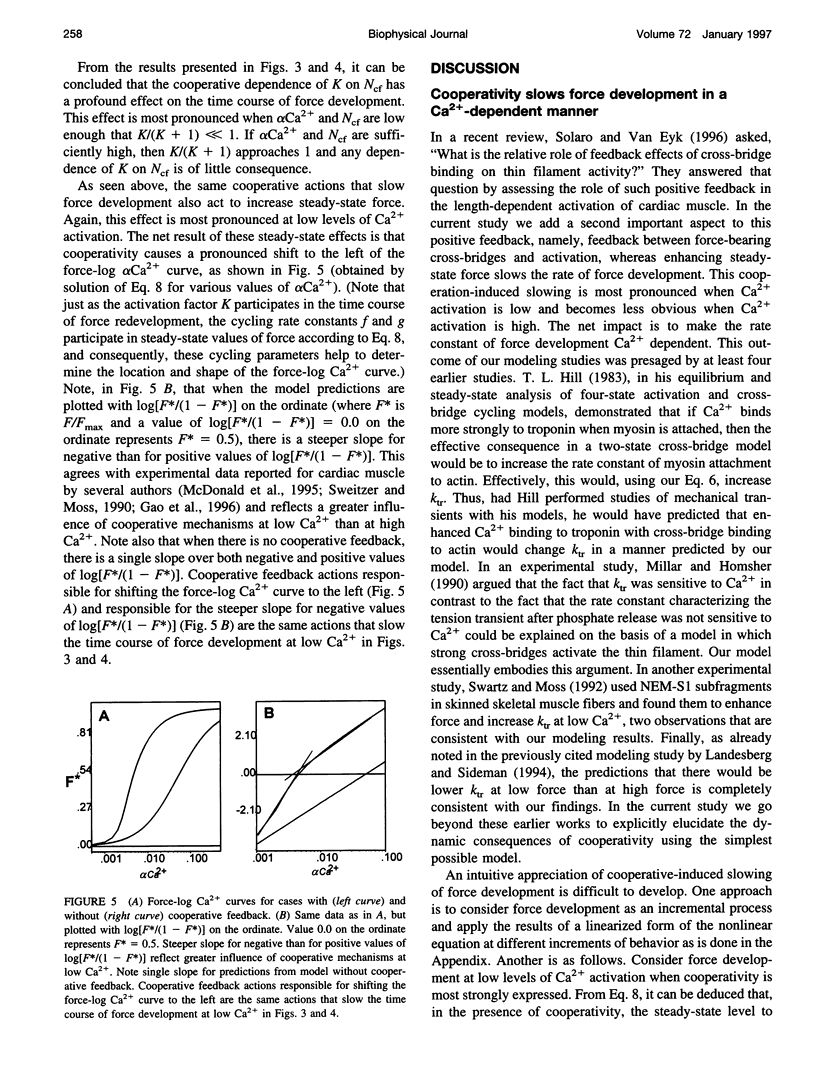
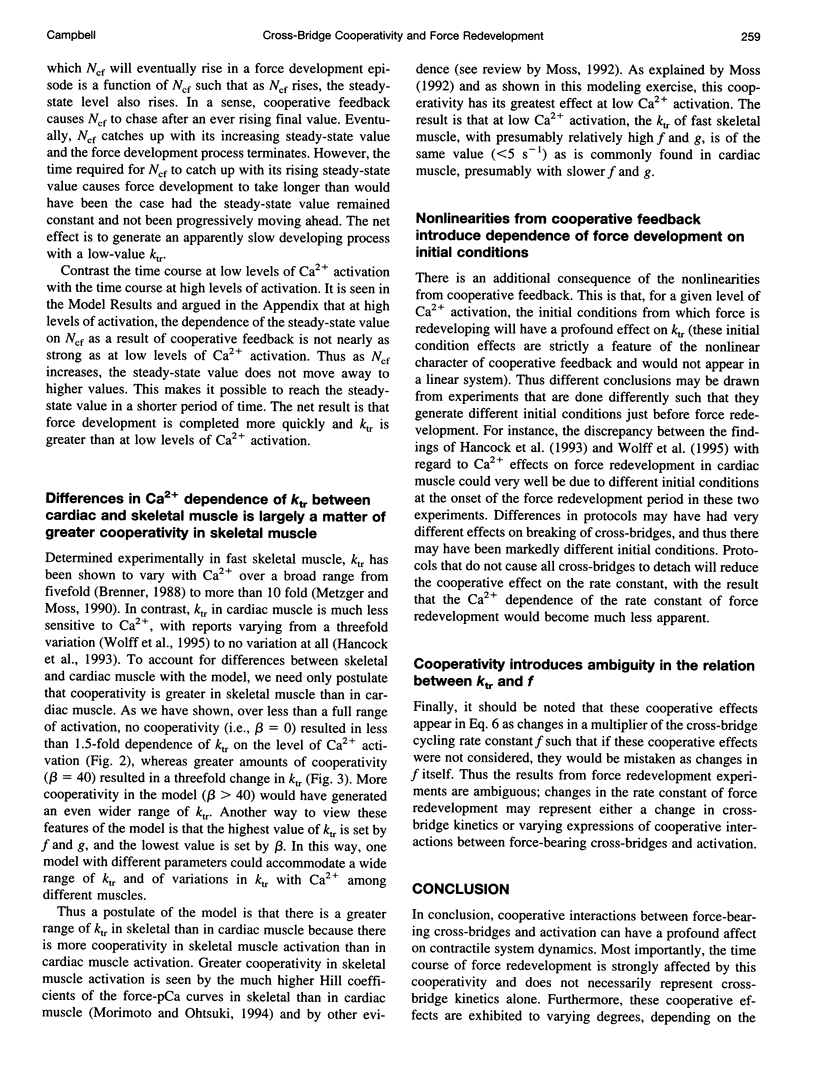
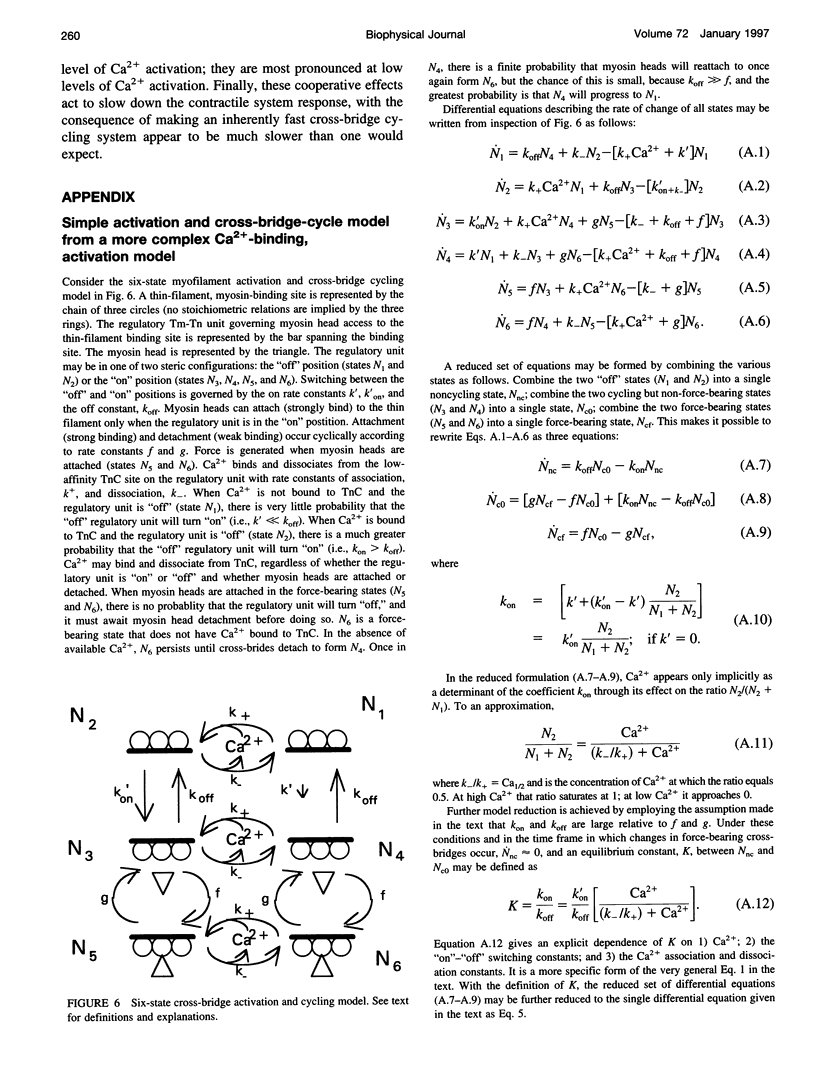
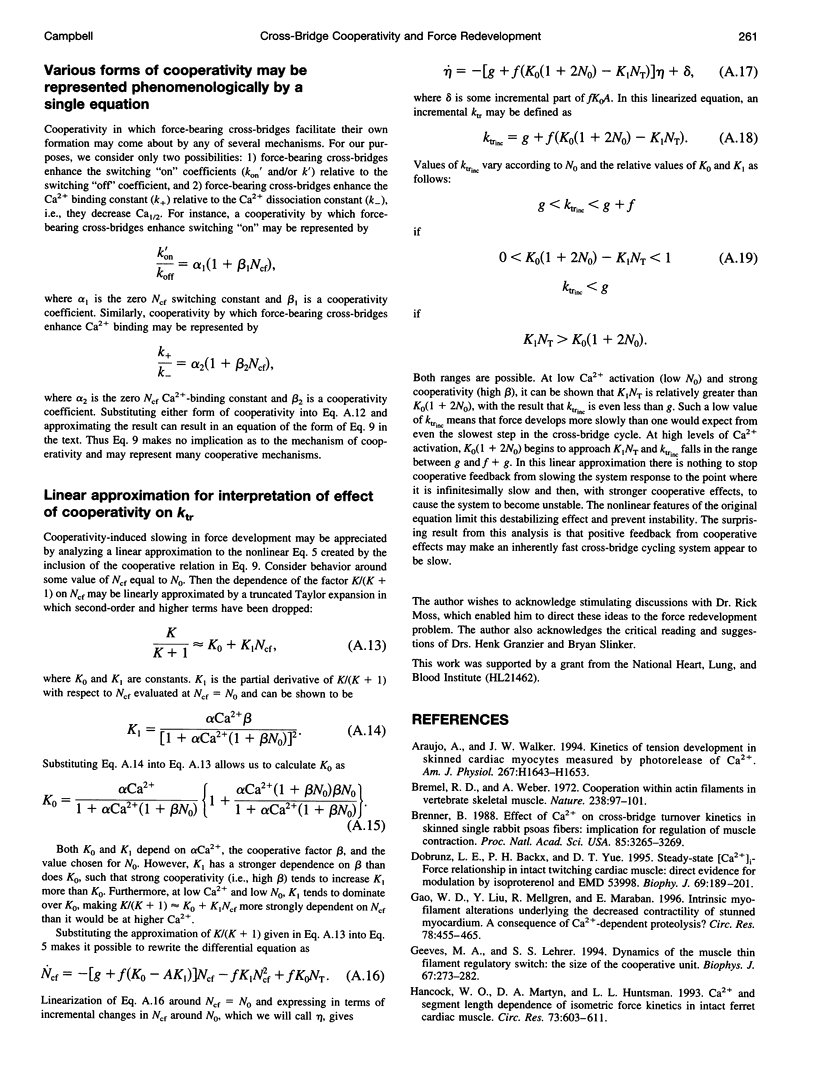
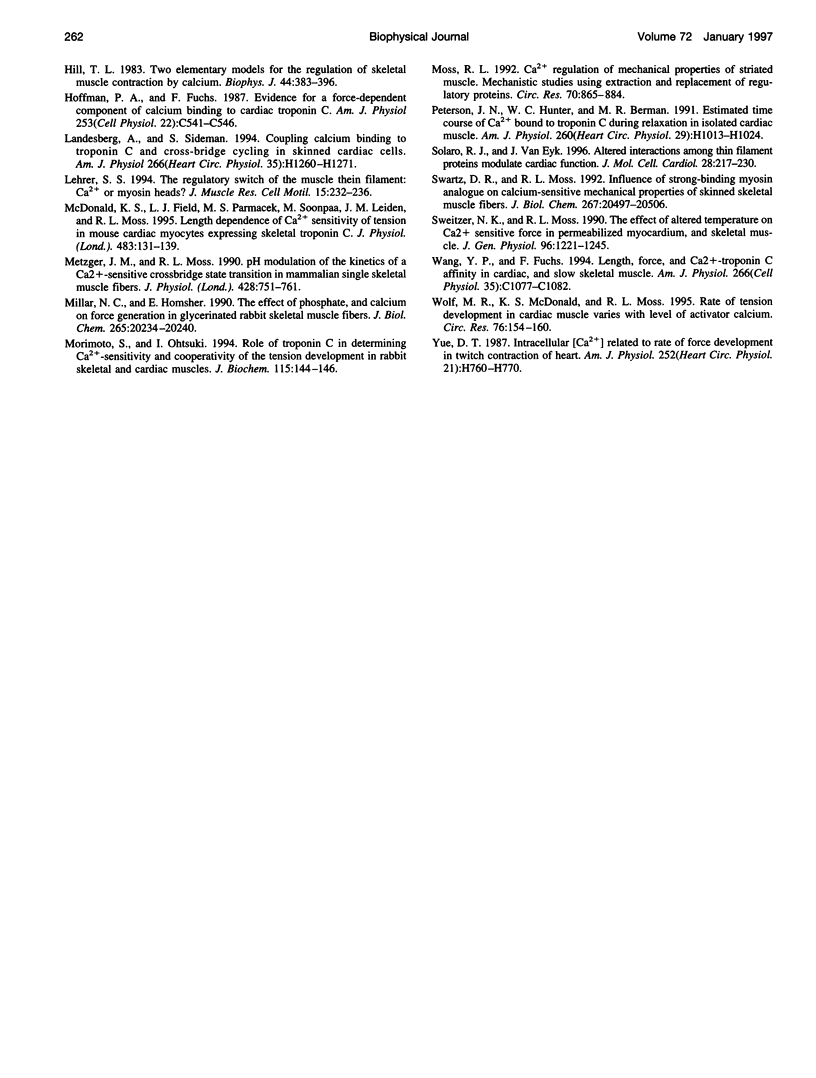
Selected References
These references are in PubMed. This may not be the complete list of references from this article.
- Araujo A., Walker J. W. Kinetics of tension development in skinned cardiac myocytes measured by photorelease of Ca2+. Am J Physiol. 1994 Nov;267(5 Pt 2):H1643–H1653. doi: 10.1152/ajpheart.1994.267.5.H1643. [DOI] [PubMed] [Google Scholar]
- Bremel R. D., Weber A. Cooperation within actin filament in vertebrate skeletal muscle. Nat New Biol. 1972 Jul 26;238(82):97–101. doi: 10.1038/newbio238097a0. [DOI] [PubMed] [Google Scholar]
- Brenner B. Effect of Ca2+ on cross-bridge turnover kinetics in skinned single rabbit psoas fibers: implications for regulation of muscle contraction. Proc Natl Acad Sci U S A. 1988 May;85(9):3265–3269. doi: 10.1073/pnas.85.9.3265. [DOI] [PMC free article] [PubMed] [Google Scholar]
- Dobrunz L. E., Backx P. H., Yue D. T. Steady-state [Ca2+]i-force relationship in intact twitching cardiac muscle: direct evidence for modulation by isoproterenol and EMD 53998. Biophys J. 1995 Jul;69(1):189–201. doi: 10.1016/S0006-3495(95)79889-7. [DOI] [PMC free article] [PubMed] [Google Scholar]
- Gao W. D., Liu Y., Mellgren R., Marban E. Intrinsic myofilament alterations underlying the decreased contractility of stunned myocardium. A consequence of Ca2+-dependent proteolysis? Circ Res. 1996 Mar;78(3):455–465. doi: 10.1161/01.res.78.3.455. [DOI] [PubMed] [Google Scholar]
- Geeves M. A., Lehrer S. S. Dynamics of the muscle thin filament regulatory switch: the size of the cooperative unit. Biophys J. 1994 Jul;67(1):273–282. doi: 10.1016/S0006-3495(94)80478-3. [DOI] [PMC free article] [PubMed] [Google Scholar]
- Hancock W. O., Martyn D. A., Huntsman L. L. Ca2+ and segment length dependence of isometric force kinetics in intact ferret cardiac muscle. Circ Res. 1993 Oct;73(4):603–611. doi: 10.1161/01.res.73.4.603. [DOI] [PubMed] [Google Scholar]
- Hill T. L. Two elementary models for the regulation of skeletal muscle contraction by calcium. Biophys J. 1983 Dec;44(3):383–396. doi: 10.1016/S0006-3495(83)84312-4. [DOI] [PMC free article] [PubMed] [Google Scholar]
- Hofmann P. A., Fuchs F. Evidence for a force-dependent component of calcium binding to cardiac troponin C. Am J Physiol. 1987 Oct;253(4 Pt 1):C541–C546. doi: 10.1152/ajpcell.1987.253.4.C541. [DOI] [PubMed] [Google Scholar]
- Landesberg A., Sideman S. Coupling calcium binding to troponin C and cross-bridge cycling in skinned cardiac cells. Am J Physiol. 1994 Mar;266(3 Pt 2):H1260–H1271. doi: 10.1152/ajpheart.1994.266.3.H1260. [DOI] [PubMed] [Google Scholar]
- Lehrer S. S. The regulatory switch of the muscle thin filament: Ca2+ or myosin heads? J Muscle Res Cell Motil. 1994 Jun;15(3):232–236. doi: 10.1007/BF00123476. [DOI] [PubMed] [Google Scholar]
- McDonald K. S., Field L. J., Parmacek M. S., Soonpaa M., Leiden J. M., Moss R. L. Length dependence of Ca2+ sensitivity of tension in mouse cardiac myocytes expressing skeletal troponin C. J Physiol. 1995 Feb 15;483(Pt 1):131–139. doi: 10.1113/jphysiol.1995.sp020573. [DOI] [PMC free article] [PubMed] [Google Scholar]
- Metzger J. M., Moss R. L. pH modulation of the kinetics of a Ca2(+)-sensitive cross-bridge state transition in mammalian single skeletal muscle fibres. J Physiol. 1990 Sep;428:751–764. doi: 10.1113/jphysiol.1990.sp018239. [DOI] [PMC free article] [PubMed] [Google Scholar]
- Millar N. C., Homsher E. The effect of phosphate and calcium on force generation in glycerinated rabbit skeletal muscle fibers. A steady-state and transient kinetic study. J Biol Chem. 1990 Nov 25;265(33):20234–20240. [PubMed] [Google Scholar]
- Morimoto S., Ohtsuki I. Role of troponin C in determining the Ca(2+)-sensitivity and cooperativity of the tension development in rabbit skeletal and cardiac muscles. J Biochem. 1994 Jan;115(1):144–146. doi: 10.1093/oxfordjournals.jbchem.a124289. [DOI] [PubMed] [Google Scholar]
- Moss R. L. Ca2+ regulation of mechanical properties of striated muscle. Mechanistic studies using extraction and replacement of regulatory proteins. Circ Res. 1992 May;70(5):865–884. doi: 10.1161/01.res.70.5.865. [DOI] [PubMed] [Google Scholar]
- Peterson J. N., Hunter W. C., Berman M. R. Estimated time course of Ca2+ bound to troponin C during relaxation in isolated cardiac muscle. Am J Physiol. 1991 Mar;260(3 Pt 2):H1013–H1024. doi: 10.1152/ajpheart.1991.260.3.H1013. [DOI] [PubMed] [Google Scholar]
- Solaro R. J., Van Eyk J. Altered interactions among thin filament proteins modulate cardiac function. J Mol Cell Cardiol. 1996 Feb;28(2):217–230. doi: 10.1006/jmcc.1996.0021. [DOI] [PubMed] [Google Scholar]
- Swartz D. R., Moss R. L. Influence of a strong-binding myosin analogue on calcium-sensitive mechanical properties of skinned skeletal muscle fibers. J Biol Chem. 1992 Oct 5;267(28):20497–20506. [PubMed] [Google Scholar]
- Sweitzer N. K., Moss R. L. The effect of altered temperature on Ca2(+)-sensitive force in permeabilized myocardium and skeletal muscle. Evidence for force dependence of thin filament activation. J Gen Physiol. 1990 Dec;96(6):1221–1245. doi: 10.1085/jgp.96.6.1221. [DOI] [PMC free article] [PubMed] [Google Scholar]
- Wang Y. P., Fuchs F. Length, force, and Ca(2+)-troponin C affinity in cardiac and slow skeletal muscle. Am J Physiol. 1994 Apr;266(4 Pt 1):C1077–C1082. doi: 10.1152/ajpcell.1994.266.4.C1077. [DOI] [PubMed] [Google Scholar]
- Wolff M. R., McDonald K. S., Moss R. L. Rate of tension development in cardiac muscle varies with level of activator calcium. Circ Res. 1995 Jan;76(1):154–160. doi: 10.1161/01.res.76.1.154. [DOI] [PubMed] [Google Scholar]
- Yue D. T. Intracellular [Ca2+] related to rate of force development in twitch contraction of heart. Am J Physiol. 1987 Apr;252(4 Pt 2):H760–H770. doi: 10.1152/ajpheart.1987.252.4.H760. [DOI] [PubMed] [Google Scholar]