Abstract
The homogeneous nucleation rate for sickle hemoglobin polymerization has been measured for concentrations from 3.9 to 4.9 mM and temperatures from 13 degrees C to 35 degrees C by observing the stochastic fluctuations of the time to complete 10% of the reaction after photolysis of the carboxy derivative. To allow efficient data collection, a mesh was used to divide the photolysis beam into an array of smaller beams, which allowed parallel observation of about 100 different regions. Nucleation rates measured here are consistent with more restricted previously published data and, when combined with directly measured monomer addition rates, are consistent with previous analysis of progress curves. By describing these rates with equilibrium nucleation theory, the concentration of nuclei and hence their stability can be ascertained. Consequently, the chemical potential by which a monomer is attached to the polymer is determined. This attachment energy ranges from -6.6 to -8.0 kcal/mol between 15 degrees C and 35 degrees C. The enthalpic part of that chemical potential is found to be equal to the enthalpy determined by solubility measurements, as expected from thermodynamic considerations. The entropic portion of the contact chemical potential contributes from -21.4 to -8.7 kcal/mol. The vibrational chemical potential of monomers in the polymer ranges from -25.7 to -27.4 kcal/mol over the same temperatures.
Full text
PDF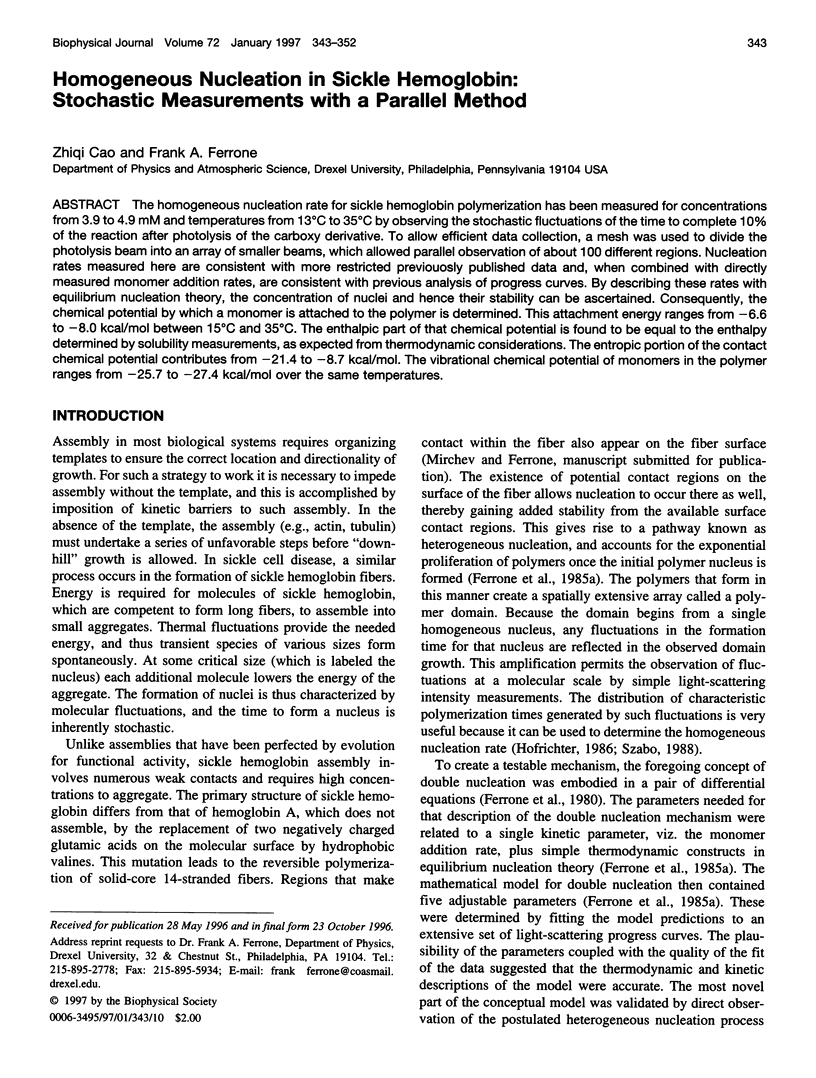
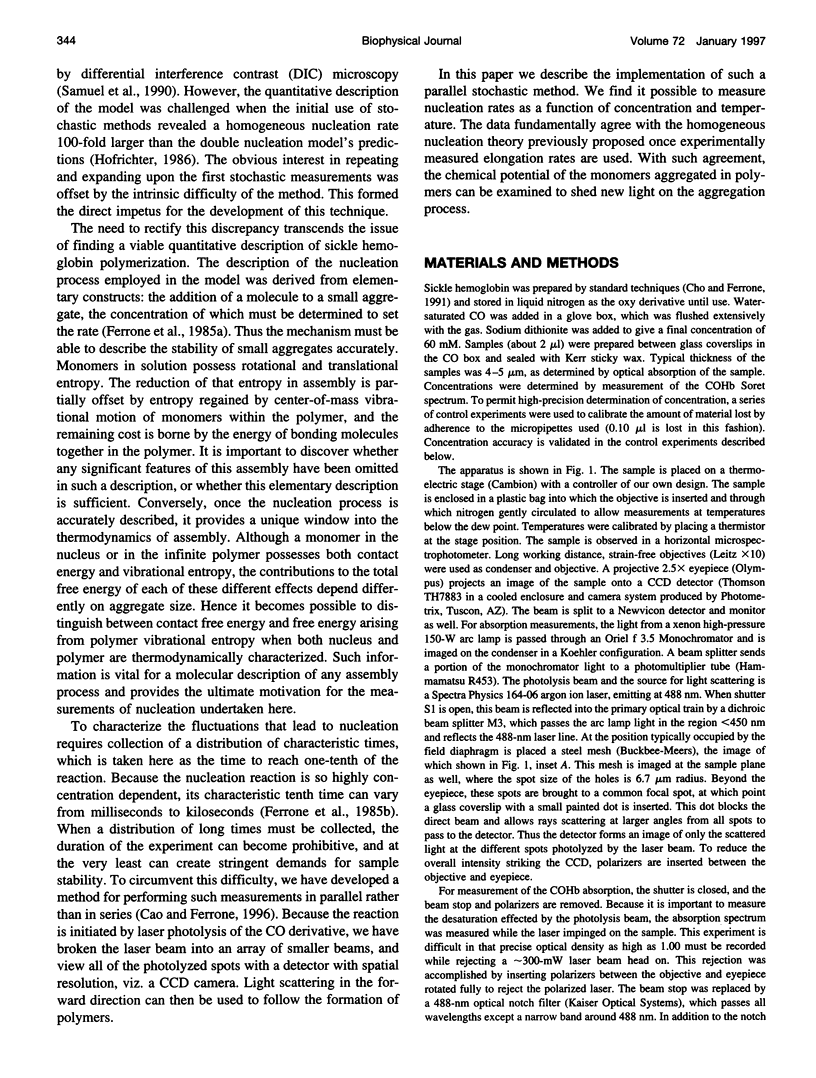
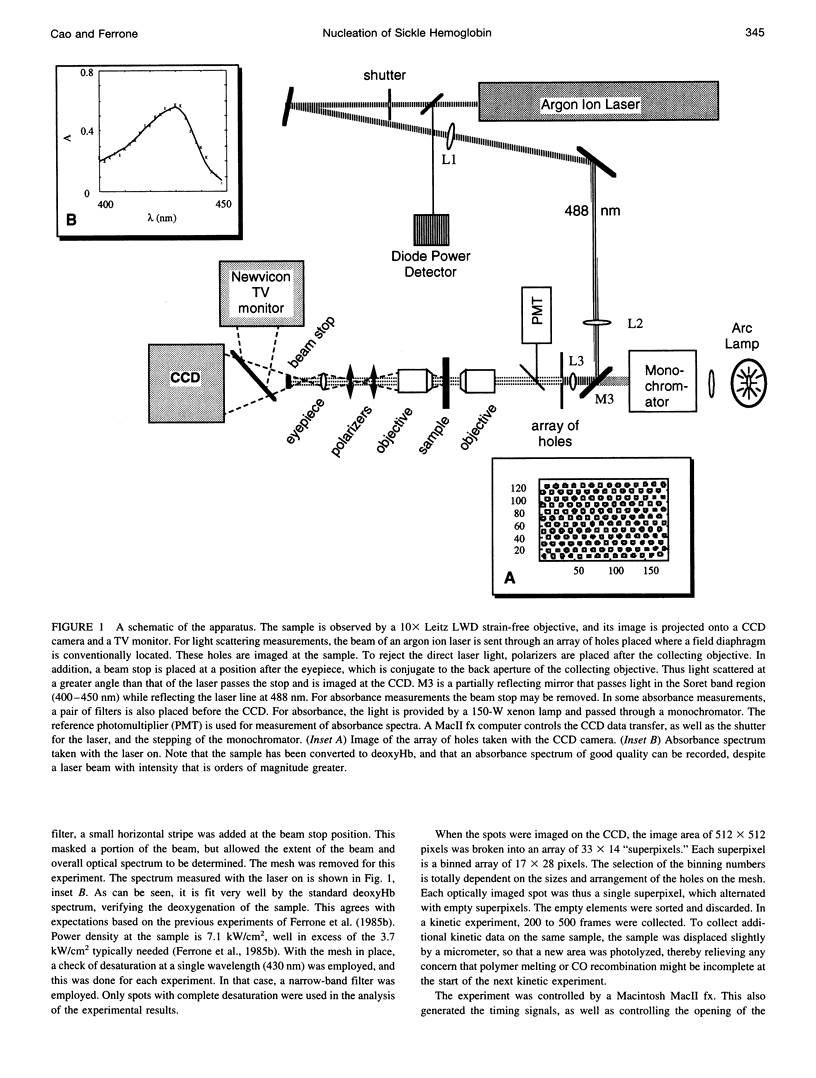
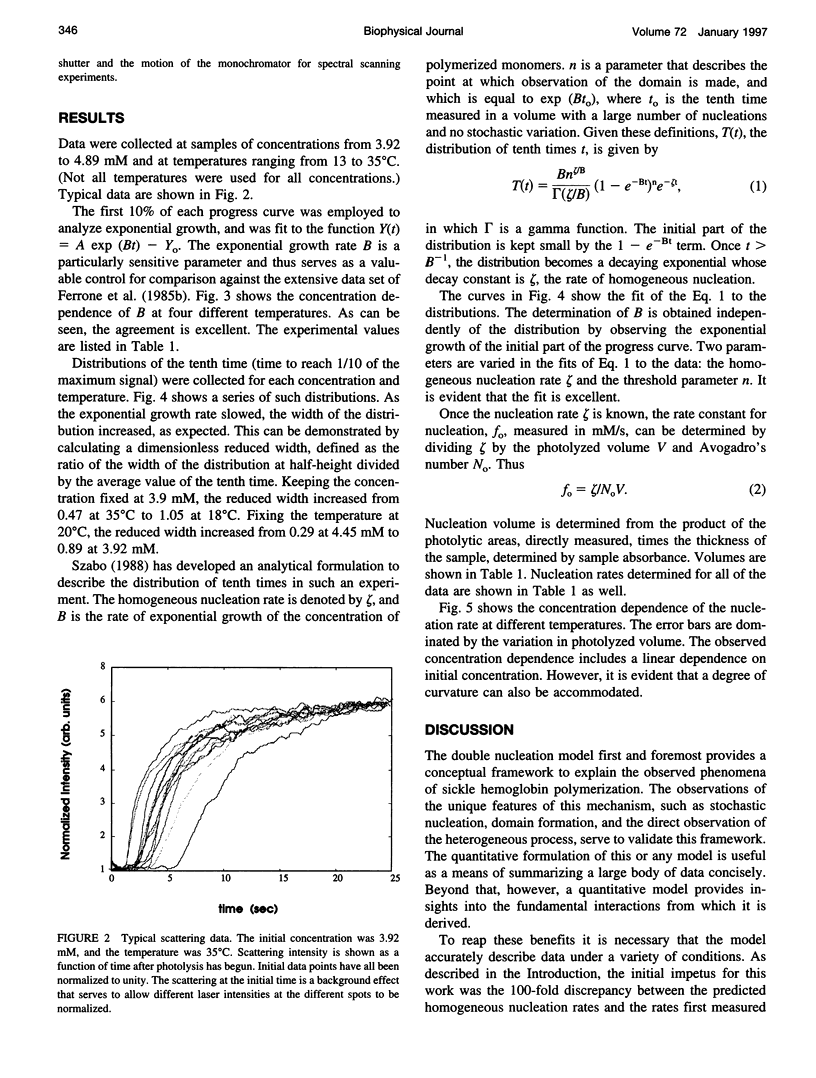
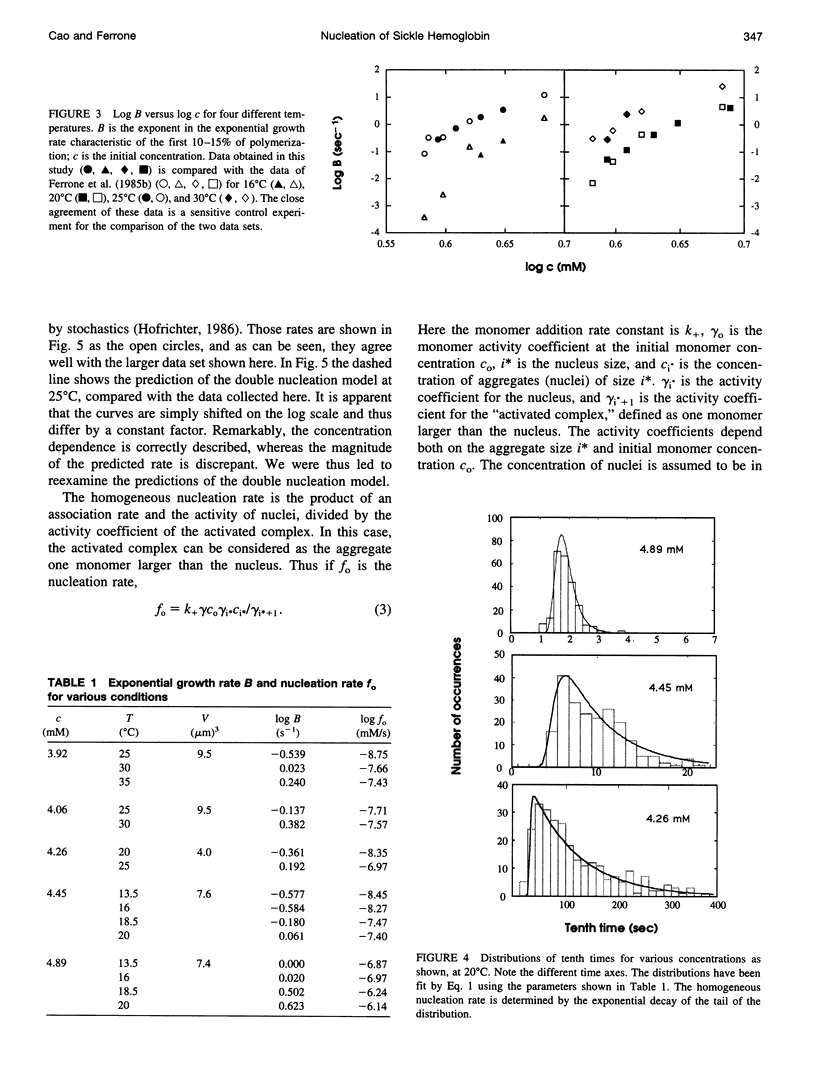
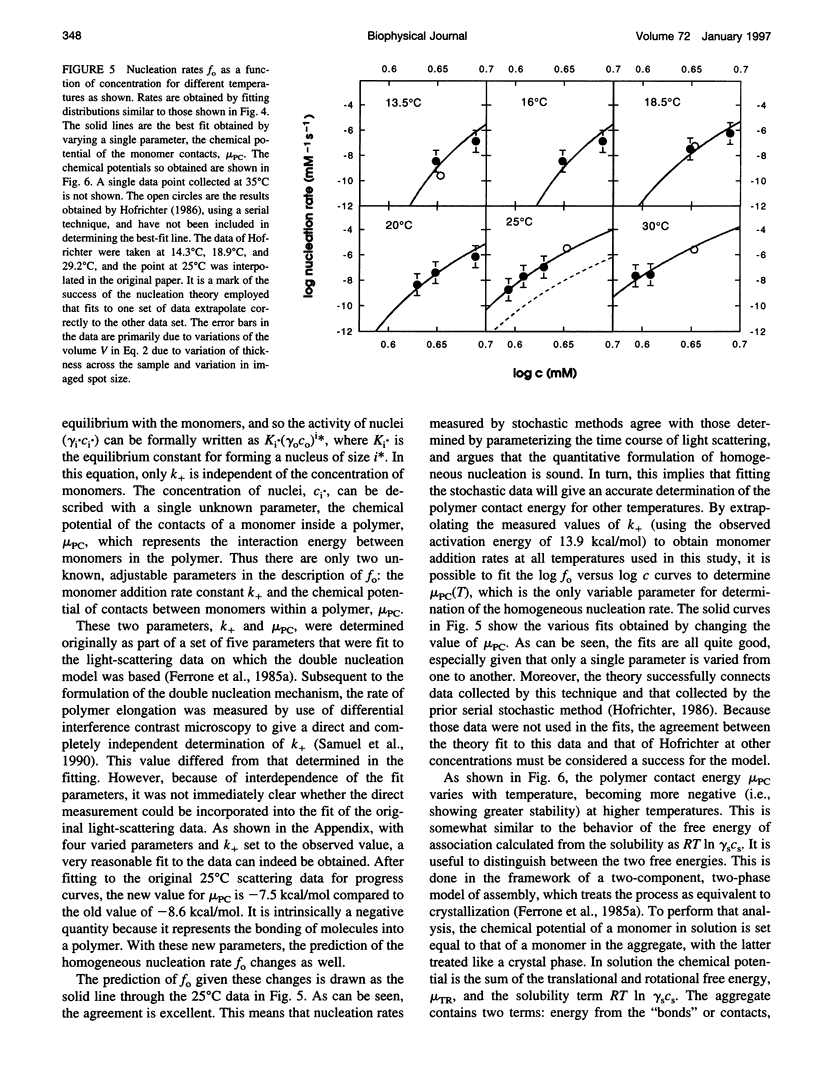
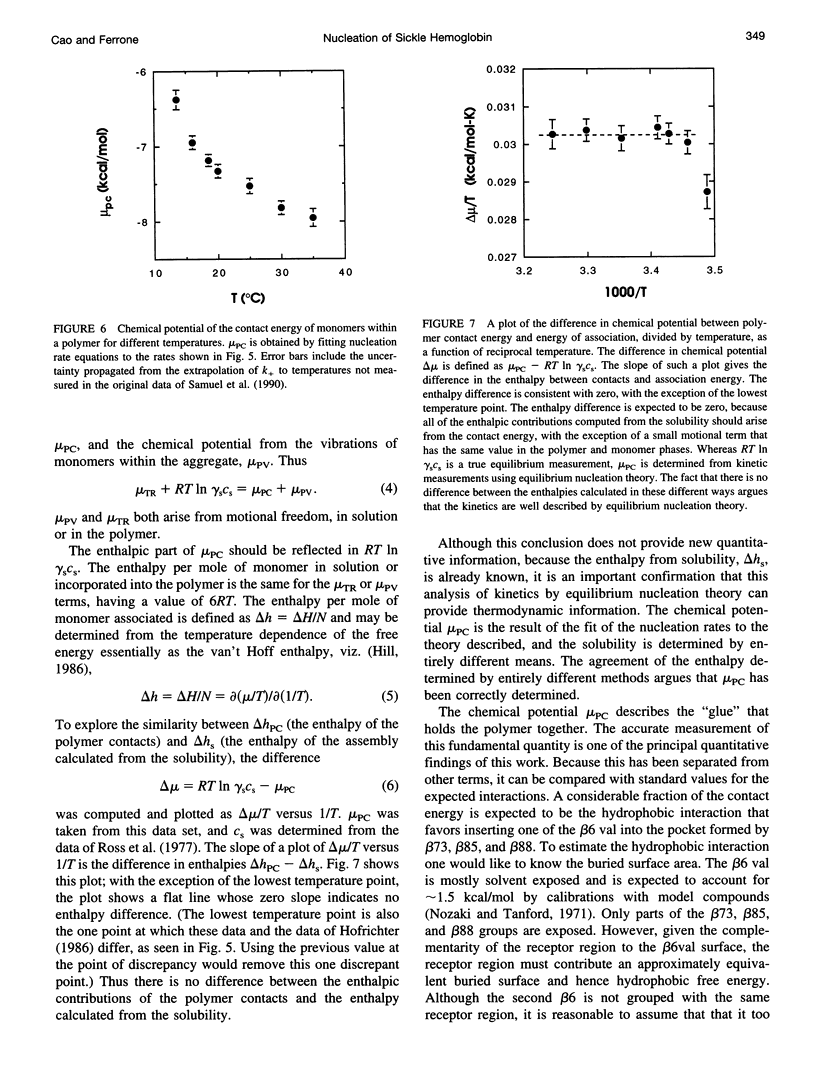
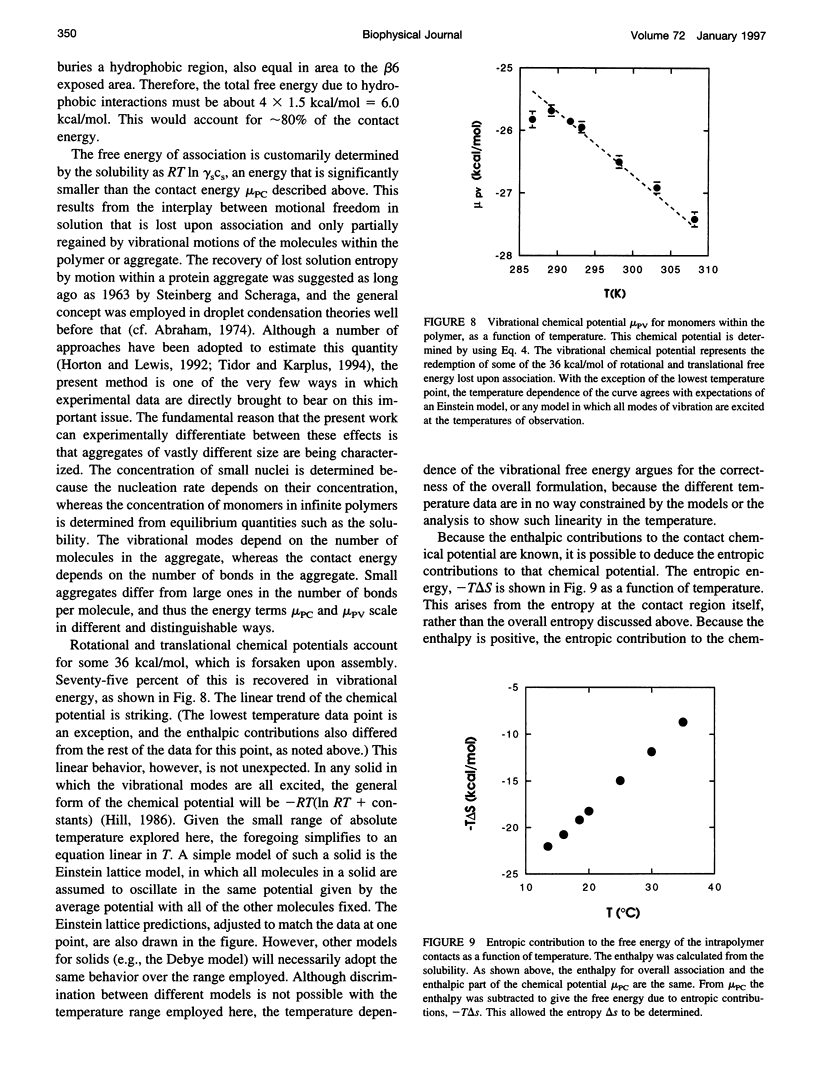
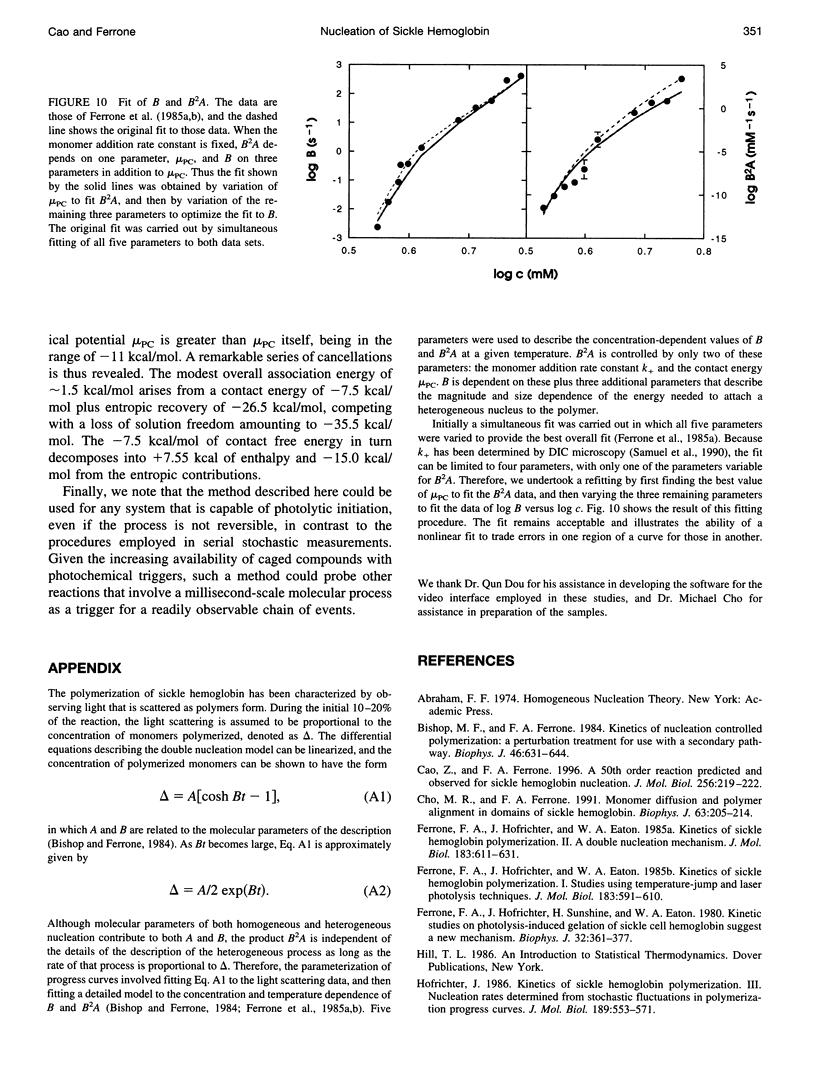
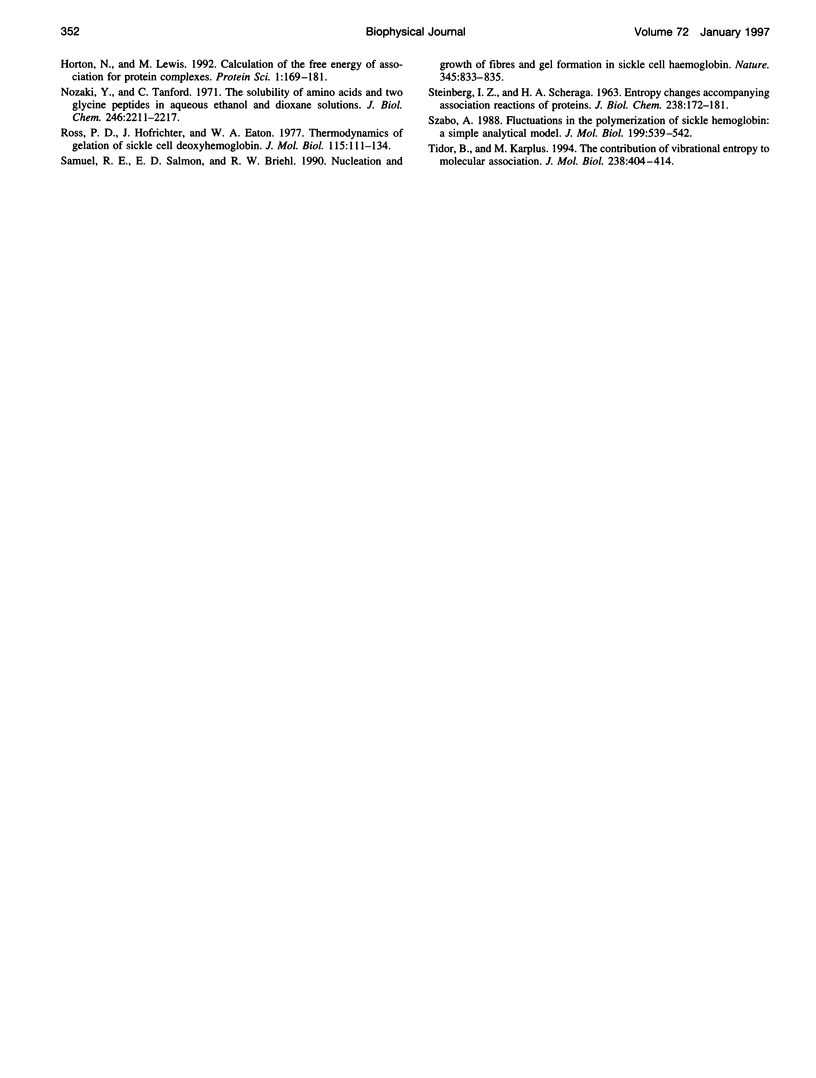
Selected References
These references are in PubMed. This may not be the complete list of references from this article.
- Bishop M. F., Ferrone F. A. Kinetics of nucleation-controlled polymerization. A perturbation treatment for use with a secondary pathway. Biophys J. 1984 Nov;46(5):631–644. doi: 10.1016/S0006-3495(84)84062-X. [DOI] [PMC free article] [PubMed] [Google Scholar]
- Cao Z., Ferrone F. A. A 50th order reaction predicted and observed for sickle hemoglobin nucleation. J Mol Biol. 1996 Feb 23;256(2):219–222. doi: 10.1006/jmbi.1996.0079. [DOI] [PubMed] [Google Scholar]
- Cho M. R., Ferrone F. A. Monomer diffusion and polymer alignment in domains of sickle hemoglobin. Biophys J. 1992 Jul;63(1):205–214. doi: 10.1016/S0006-3495(92)81595-3. [DOI] [PMC free article] [PubMed] [Google Scholar]
- Ferrone F. A., Hofrichter J., Eaton W. A. Kinetics of sickle hemoglobin polymerization. I. Studies using temperature-jump and laser photolysis techniques. J Mol Biol. 1985 Jun 25;183(4):591–610. doi: 10.1016/0022-2836(85)90174-3. [DOI] [PubMed] [Google Scholar]
- Ferrone F. A., Hofrichter J., Eaton W. A. Kinetics of sickle hemoglobin polymerization. II. A double nucleation mechanism. J Mol Biol. 1985 Jun 25;183(4):611–631. doi: 10.1016/0022-2836(85)90175-5. [DOI] [PubMed] [Google Scholar]
- Ferrone F. A., Hofrichter J., Sunshine H. R., Eaton W. A. Kinetic studies on photolysis-induced gelation of sickle cell hemoglobin suggest a new mechanism. Biophys J. 1980 Oct;32(1):361–380. doi: 10.1016/S0006-3495(80)84962-9. [DOI] [PMC free article] [PubMed] [Google Scholar]
- Hofrichter J. Kinetics of sickle hemoglobin polymerization. III. Nucleation rates determined from stochastic fluctuations in polymerization progress curves. J Mol Biol. 1986 Jun 5;189(3):553–571. doi: 10.1016/0022-2836(86)90324-4. [DOI] [PubMed] [Google Scholar]
- Horton N., Lewis M. Calculation of the free energy of association for protein complexes. Protein Sci. 1992 Jan;1(1):169–181. doi: 10.1002/pro.5560010117. [DOI] [PMC free article] [PubMed] [Google Scholar]
- Nozaki Y., Tanford C. The solubility of amino acids and two glycine peptides in aqueous ethanol and dioxane solutions. Establishment of a hydrophobicity scale. J Biol Chem. 1971 Apr 10;246(7):2211–2217. [PubMed] [Google Scholar]
- Ross P. D., Hofrichter J., Eaton W. A. Thermodynamics of gelation of sickle cell deoxyhemoglobin. J Mol Biol. 1977 Sep 15;115(2):111–134. doi: 10.1016/0022-2836(77)90093-6. [DOI] [PubMed] [Google Scholar]
- STEINBERG I. Z., SCHERAGA H. A. Entropy changes accompanying association reactions of proteins. J Biol Chem. 1963 Jan;238:172–181. [PubMed] [Google Scholar]
- Samuel R. E., Salmon E. D., Briehl R. W. Nucleation and growth of fibres and gel formation in sickle cell haemoglobin. Nature. 1990 Jun 28;345(6278):833–835. doi: 10.1038/345833a0. [DOI] [PubMed] [Google Scholar]
- Szabo A. Fluctuations in the polymerization of sickle hemoglobin. A simple analytic model. J Mol Biol. 1988 Feb 5;199(3):539–542. doi: 10.1016/0022-2836(88)90624-9. [DOI] [PubMed] [Google Scholar]
- Tidor B., Karplus M. The contribution of vibrational entropy to molecular association. The dimerization of insulin. J Mol Biol. 1994 May 6;238(3):405–414. doi: 10.1006/jmbi.1994.1300. [DOI] [PubMed] [Google Scholar]