Abstract
Forces between type I collagen triple helices are studied in solvents of varying hydrogen-bonding ability. The swelling of collagen fibers in reconstituted films is controlled by the concentration of soluble polymers that are excluded from the fibers and that compete osmotically with collagen for available solvent. The interaxial spacing between the triple helices as a function of the polymer concentration is measured by x-ray diffraction. Exponential-like changes in the spacing with increasing osmotic stress, qualitatively similar to the forces previously found in aqueous solution, are also seen in formamide and ethylene glycol. These are solvents that, like water, are capable of forming three-dimensional hydrogen-bond networks. In solvents that either cannot form a network or have a greatly impaired ability to form a hydrogen-bonded network, strikingly different behavior is observed. A hard-wall repulsion is seen with collagen solvated by ethanol, 2-propanol, and N,N-dimethylformamide. The spacing between helices hardly changes with increasing polymer concentration until the stress exceeds some threshold where removal of the solvent becomes energetically favorable. No solvation of collagen is observed in dimethoxyethane. In solvents with an intermediate ability to form hydrogen-bonded networks, methanol, 2-methoxyethanol, or N-methylformamide, the change in spacing with polymer concentration is intermediate between exponential-like and hard-wall. These results provide direct evidence that the exponential repulsion observed between collagen helices at 0-8-A surface separations in water is due to the energetic cost associated with perturbing the hydrogen-bonded network of solvent molecules between the collagen surfaces.
Full text
PDF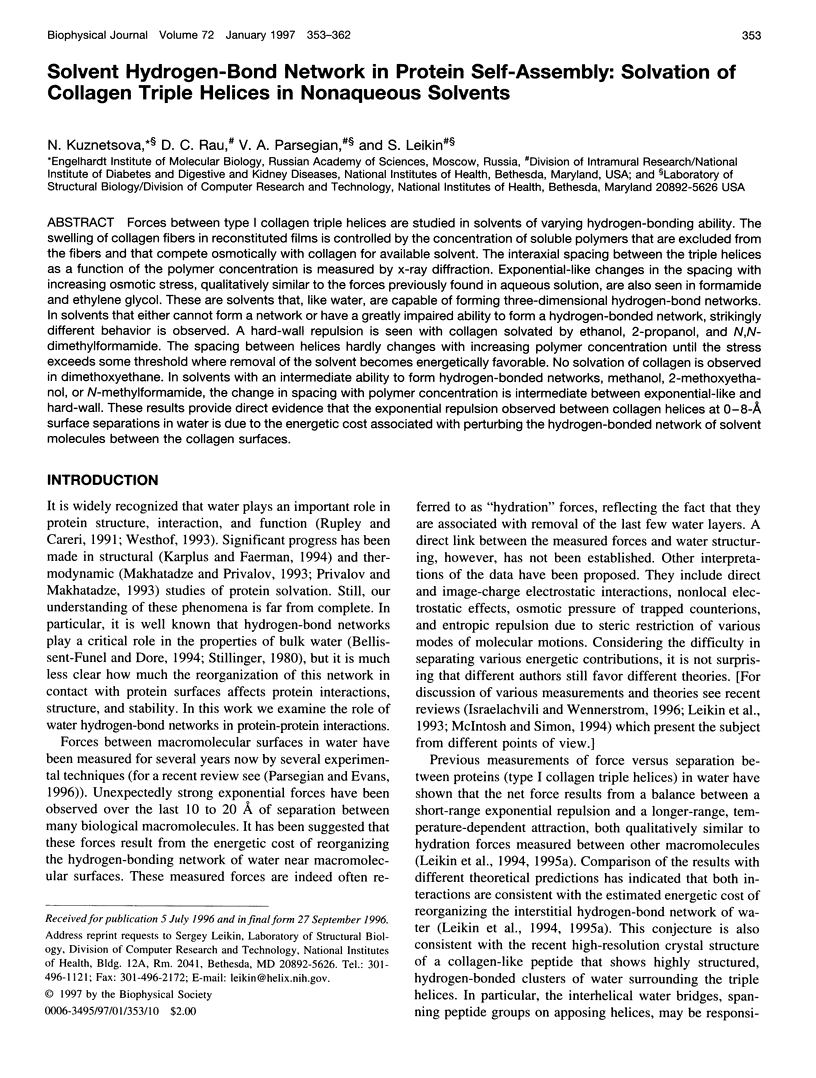
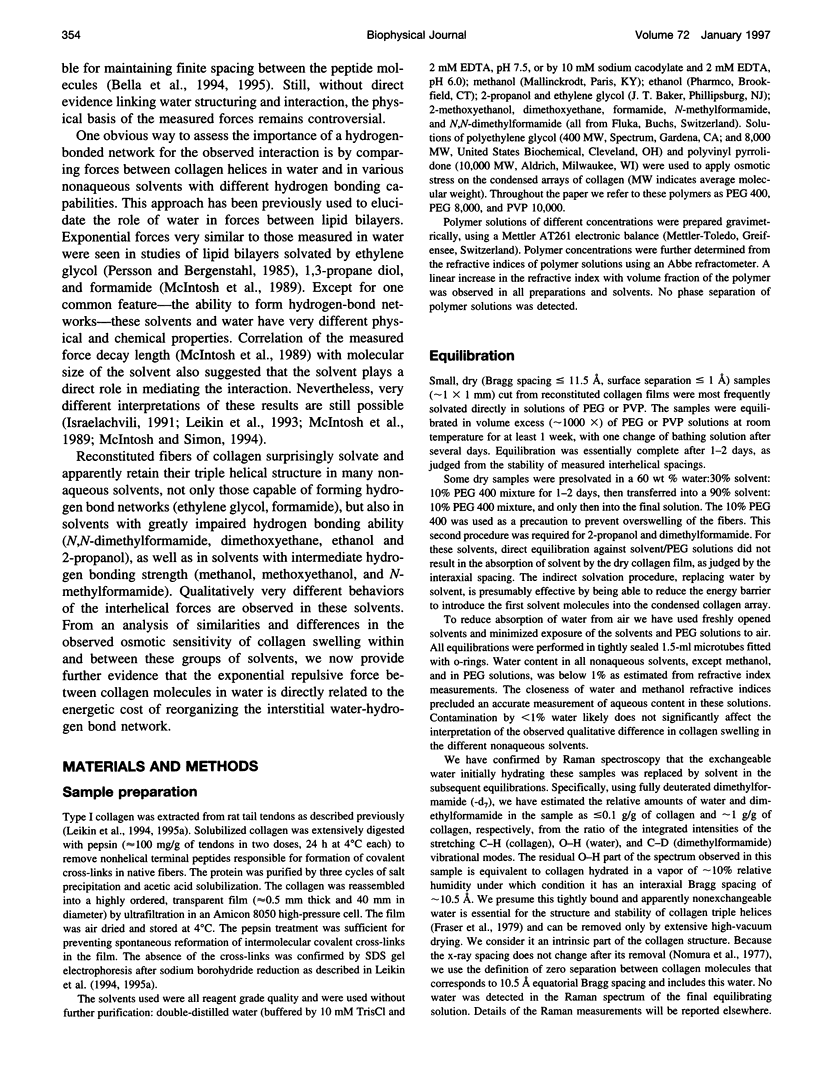
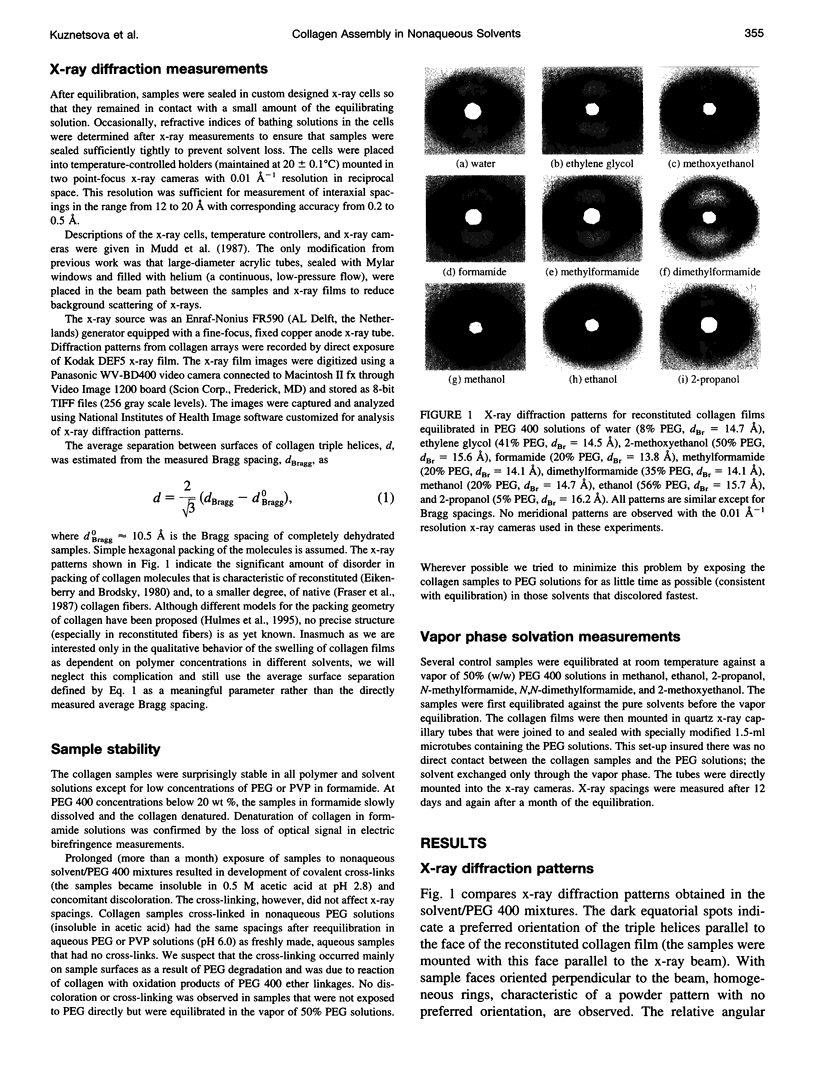
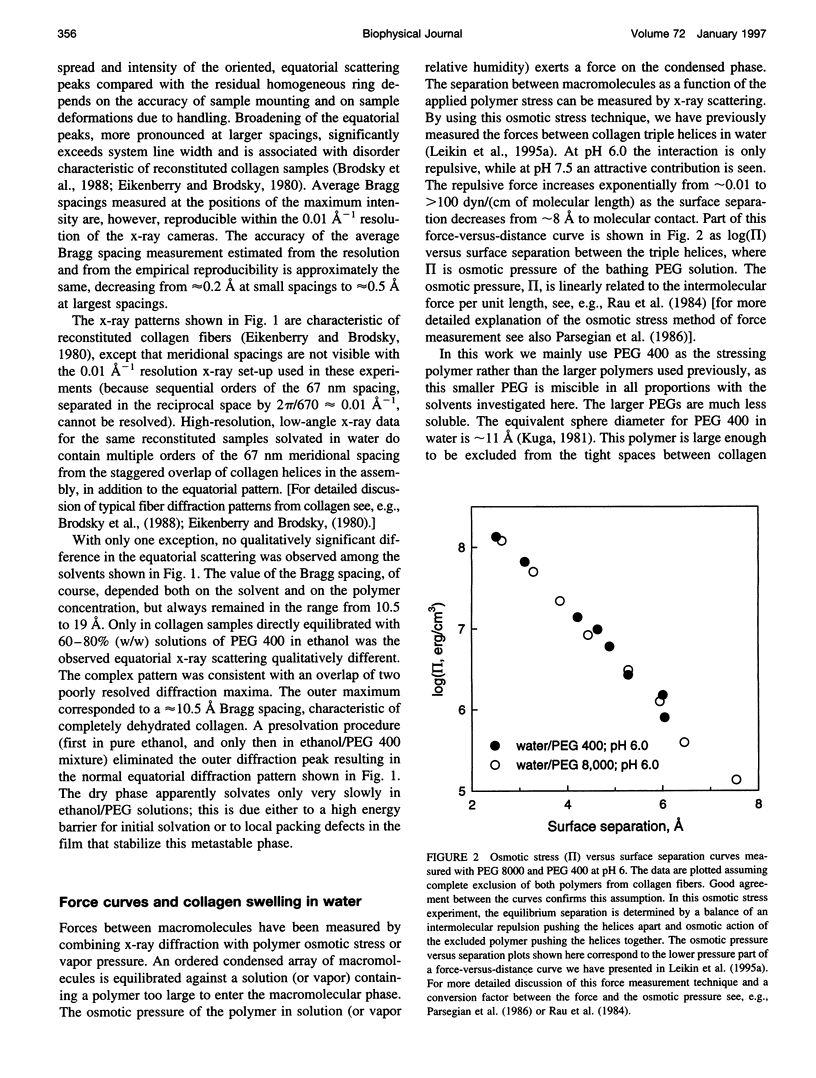
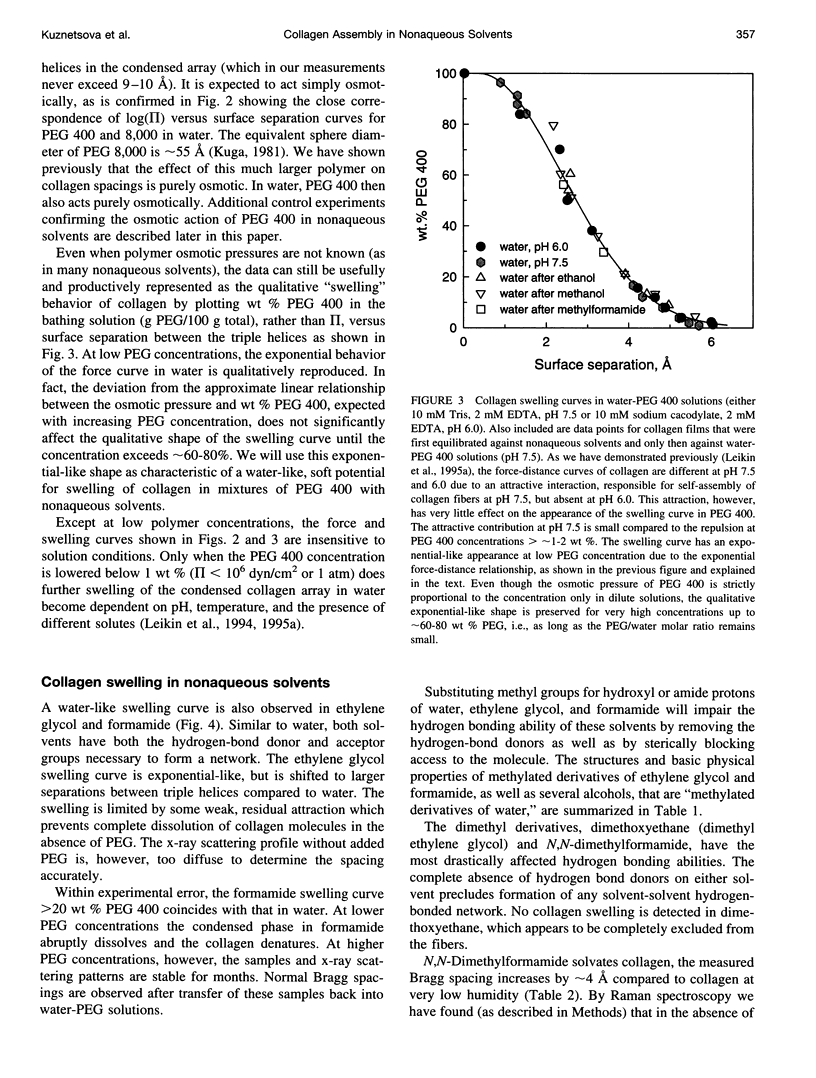
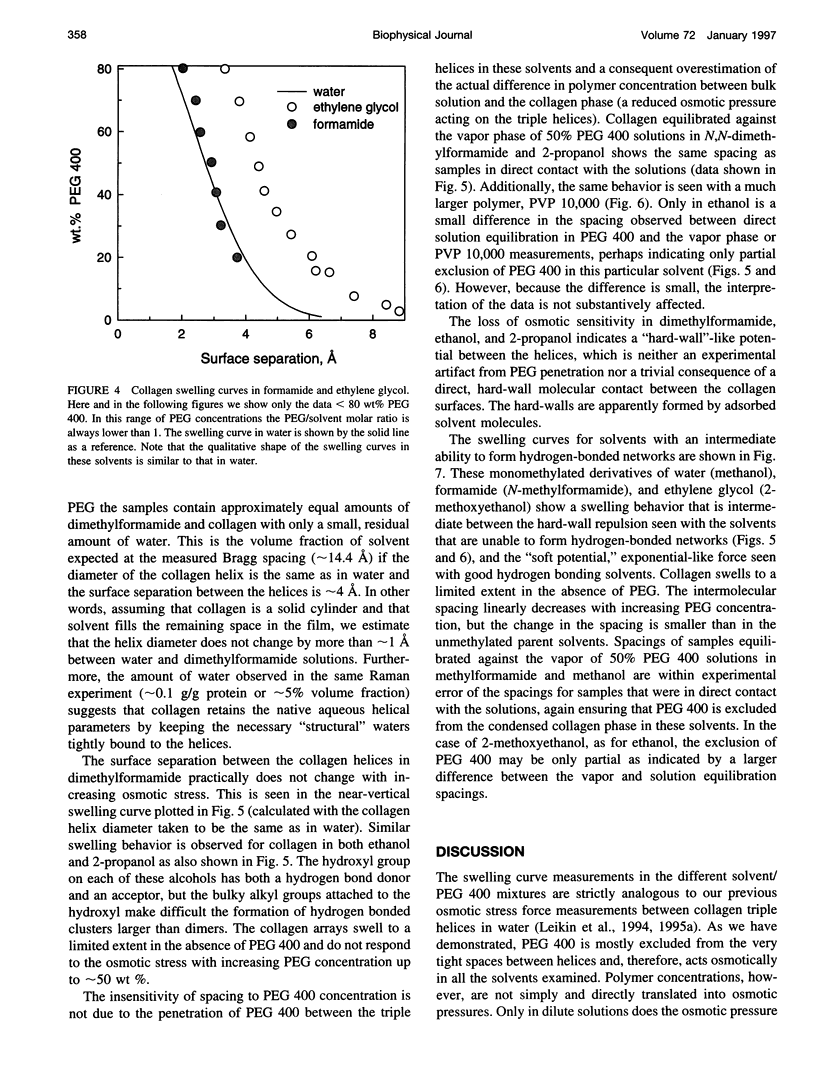
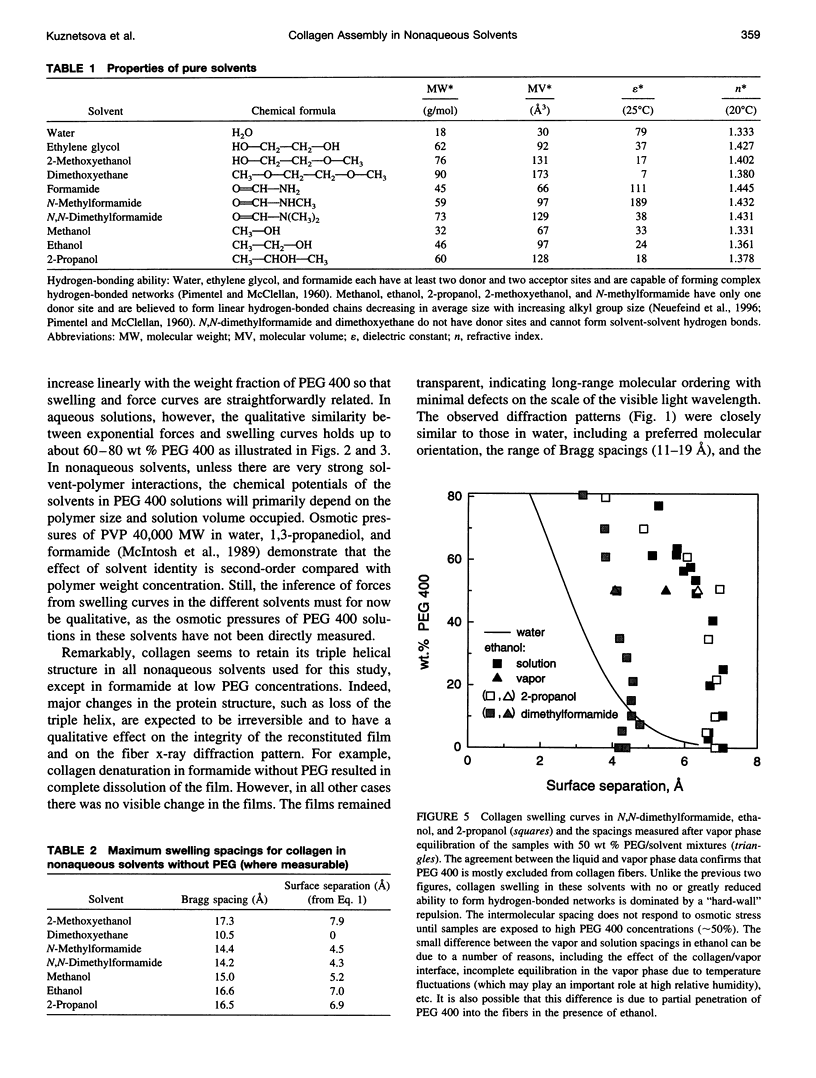
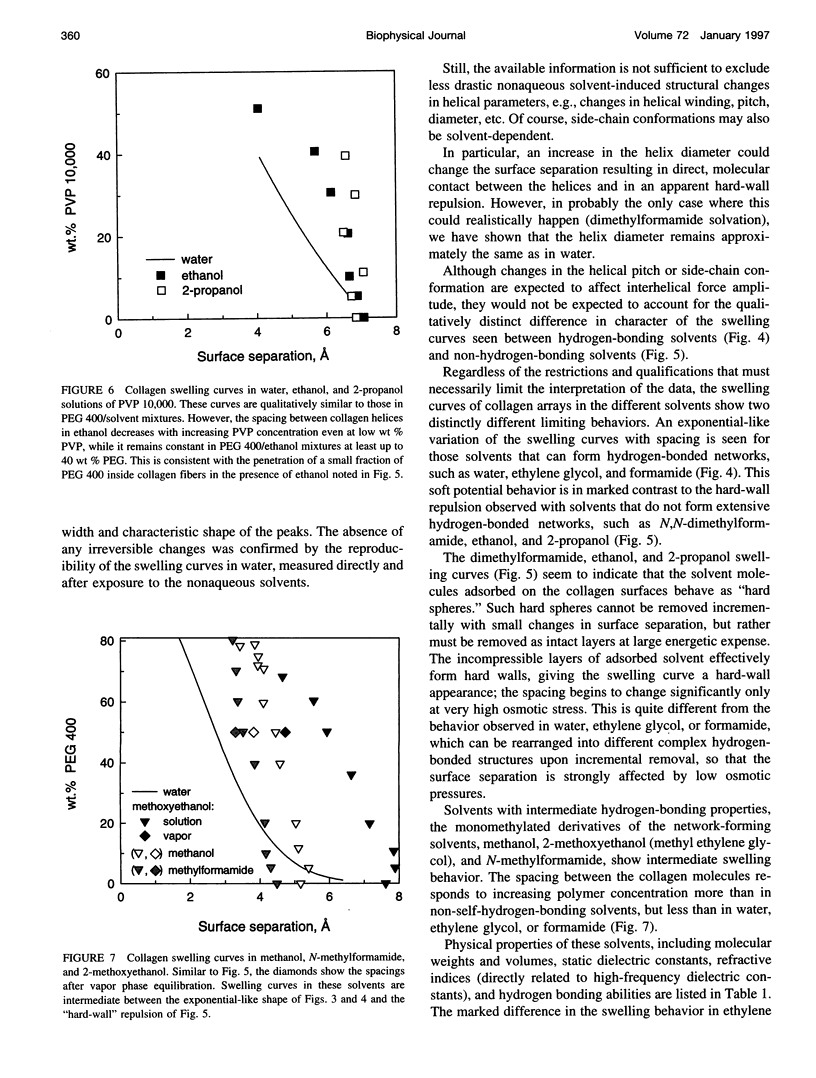
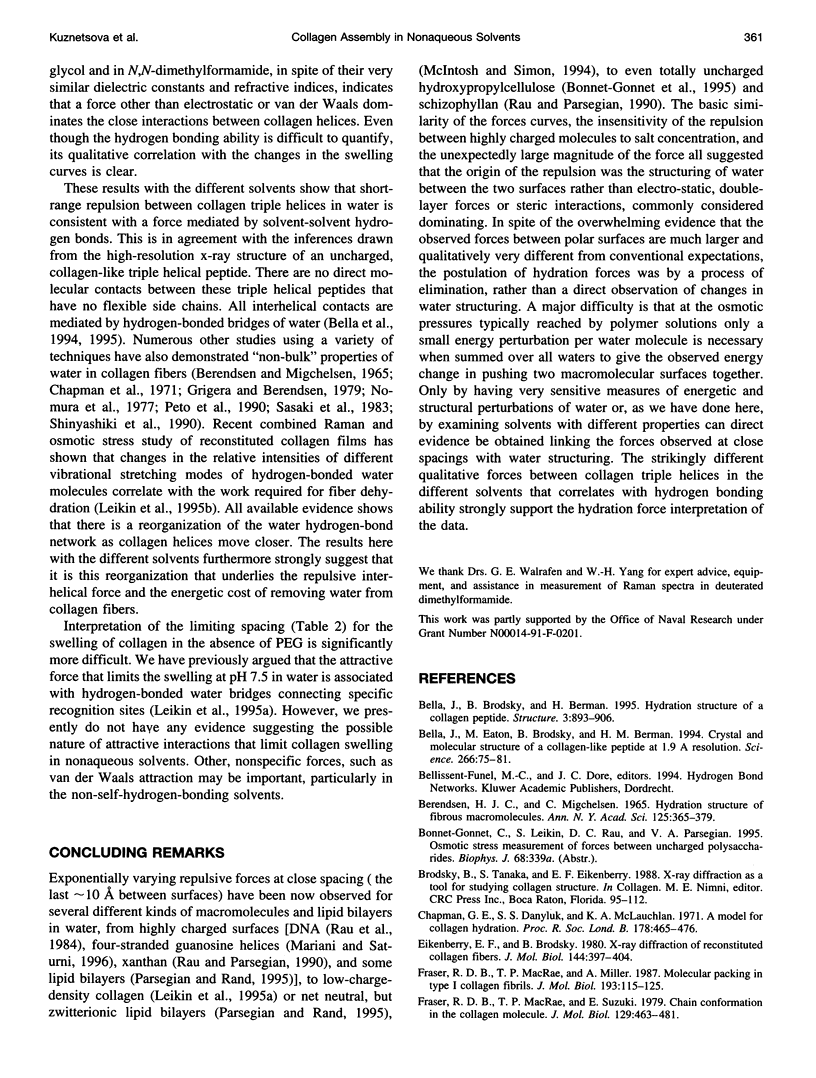
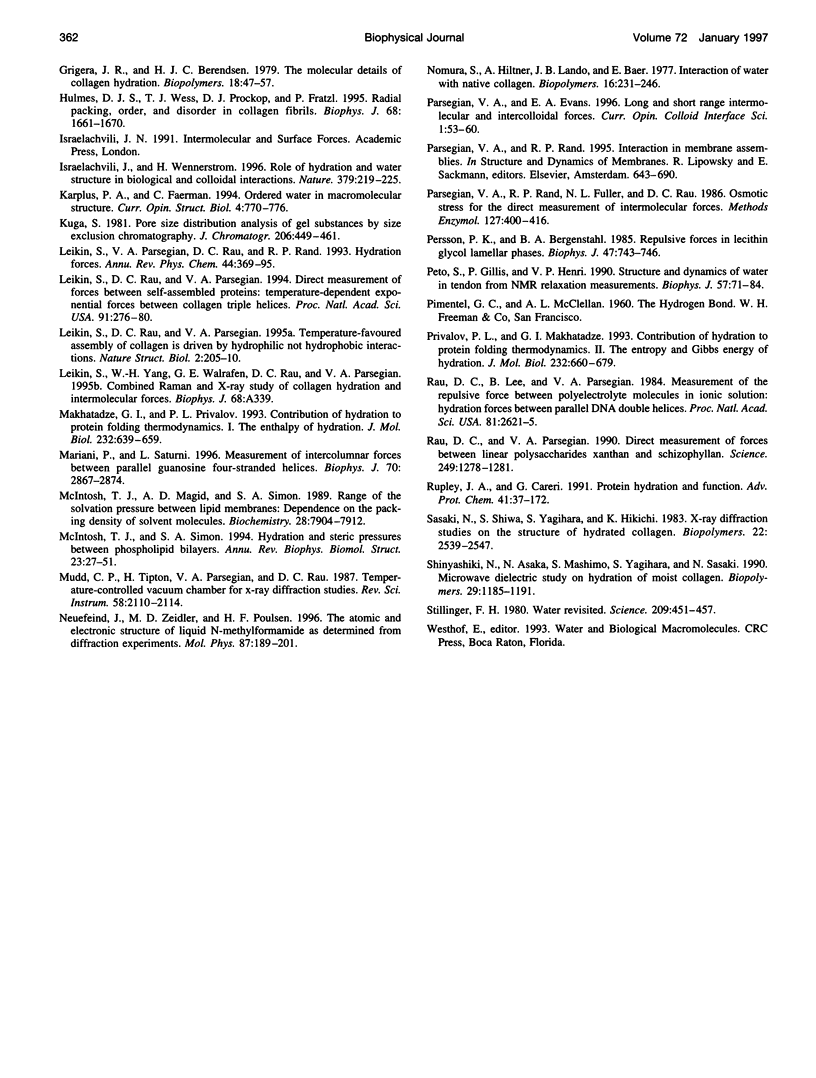
Images in this article
Selected References
These references are in PubMed. This may not be the complete list of references from this article.
- Bella J., Brodsky B., Berman H. M. Hydration structure of a collagen peptide. Structure. 1995 Sep 15;3(9):893–906. doi: 10.1016/S0969-2126(01)00224-6. [DOI] [PubMed] [Google Scholar]
- Bella J., Eaton M., Brodsky B., Berman H. M. Crystal and molecular structure of a collagen-like peptide at 1.9 A resolution. Science. 1994 Oct 7;266(5182):75–81. doi: 10.1126/science.7695699. [DOI] [PubMed] [Google Scholar]
- Chapman G. E., Danyluk S. S., McLauchlan K. A. a model for collagen hydration. Proc R Soc Lond B Biol Sci. 1971 Sep 28;178(1053):465–476. doi: 10.1098/rspb.1971.0076. [DOI] [PubMed] [Google Scholar]
- Eikenberry E. F., Brodsky B. X-ray diffraction of reconstituted collagen fibers. J Mol Biol. 1980 Dec 15;144(3):397–404. doi: 10.1016/0022-2836(80)90098-4. [DOI] [PubMed] [Google Scholar]
- Fraser R. D., MacRae T. P., Miller A. Molecular packing in type I collagen fibrils. J Mol Biol. 1987 Jan 5;193(1):115–125. doi: 10.1016/0022-2836(87)90631-0. [DOI] [PubMed] [Google Scholar]
- Fraser R. D., MacRae T. P., Suzuki E. Chain conformation in the collagen molecule. J Mol Biol. 1979 Apr 15;129(3):463–481. doi: 10.1016/0022-2836(79)90507-2. [DOI] [PubMed] [Google Scholar]
- Hulmes D. J., Wess T. J., Prockop D. J., Fratzl P. Radial packing, order, and disorder in collagen fibrils. Biophys J. 1995 May;68(5):1661–1670. doi: 10.1016/S0006-3495(95)80391-7. [DOI] [PMC free article] [PubMed] [Google Scholar]
- Israelachvili J., Wennerström H. Role of hydration and water structure in biological and colloidal interactions. Nature. 1996 Jan 18;379(6562):219–225. doi: 10.1038/379219a0. [DOI] [PubMed] [Google Scholar]
- Leikin S., Parsegian V. A., Rau D. C., Rand R. P. Hydration forces. Annu Rev Phys Chem. 1993;44:369–395. doi: 10.1146/annurev.pc.44.100193.002101. [DOI] [PubMed] [Google Scholar]
- Leikin S., Rau D. C., Parsegian V. A. Direct measurement of forces between self-assembled proteins: temperature-dependent exponential forces between collagen triple helices. Proc Natl Acad Sci U S A. 1994 Jan 4;91(1):276–280. doi: 10.1073/pnas.91.1.276. [DOI] [PMC free article] [PubMed] [Google Scholar]
- Leikin S., Rau D. C., Parsegian V. A. Temperature-favoured assembly of collagen is driven by hydrophilic not hydrophobic interactions. Nat Struct Biol. 1995 Mar;2(3):205–210. doi: 10.1038/nsb0395-205. [DOI] [PubMed] [Google Scholar]
- Makhatadze G. I., Privalov P. L. Contribution of hydration to protein folding thermodynamics. I. The enthalpy of hydration. J Mol Biol. 1993 Jul 20;232(2):639–659. doi: 10.1006/jmbi.1993.1416. [DOI] [PubMed] [Google Scholar]
- Mariani P., Saturni L. Measurement of intercolumnar forces between parallel guanosine four-stranded helices. Biophys J. 1996 Jun;70(6):2867–2874. doi: 10.1016/S0006-3495(96)79856-9. [DOI] [PMC free article] [PubMed] [Google Scholar]
- McIntosh T. J., Magid A. D., Simon S. A. Range of the solvation pressure between lipid membranes: dependence on the packing density of solvent molecules. Biochemistry. 1989 Sep 19;28(19):7904–7912. doi: 10.1021/bi00445a053. [DOI] [PubMed] [Google Scholar]
- McIntosh T. J., Simon S. A. Hydration and steric pressures between phospholipid bilayers. Annu Rev Biophys Biomol Struct. 1994;23:27–51. doi: 10.1146/annurev.bb.23.060194.000331. [DOI] [PubMed] [Google Scholar]
- Nomura S., Hiltner A., Lando J. B., Baer E. Interaction of water with native collagen. Biopolymers. 1977 Feb;16(2):231–246. doi: 10.1002/bip.1977.360160202. [DOI] [PubMed] [Google Scholar]
- Parsegian V. A., Rand R. P., Fuller N. L., Rau D. C. Osmotic stress for the direct measurement of intermolecular forces. Methods Enzymol. 1986;127:400–416. doi: 10.1016/0076-6879(86)27032-9. [DOI] [PubMed] [Google Scholar]
- Persson P. K., Bergenståhl B. A. Repulsive forces in lecithin glycol lamellar phases. Biophys J. 1985 May;47(5):743–746. doi: 10.1016/S0006-3495(85)83974-6. [DOI] [PMC free article] [PubMed] [Google Scholar]
- Peto S., Gillis P., Henri V. P. Structure and dynamics of water in tendon from NMR relaxation measurements. Biophys J. 1990 Jan;57(1):71–84. doi: 10.1016/S0006-3495(90)82508-X. [DOI] [PMC free article] [PubMed] [Google Scholar]
- Privalov P. L., Makhatadze G. I. Contribution of hydration to protein folding thermodynamics. II. The entropy and Gibbs energy of hydration. J Mol Biol. 1993 Jul 20;232(2):660–679. doi: 10.1006/jmbi.1993.1417. [DOI] [PubMed] [Google Scholar]
- Rau D. C., Lee B., Parsegian V. A. Measurement of the repulsive force between polyelectrolyte molecules in ionic solution: hydration forces between parallel DNA double helices. Proc Natl Acad Sci U S A. 1984 May;81(9):2621–2625. doi: 10.1073/pnas.81.9.2621. [DOI] [PMC free article] [PubMed] [Google Scholar]
- Rau D. C., Parsegian V. A. Direct measurement of forces between linear polysaccharides xanthan and schizophyllan. Science. 1990 Sep 14;249(4974):1278–1281. doi: 10.1126/science.2144663. [DOI] [PubMed] [Google Scholar]
- Rupley J. A., Careri G. Protein hydration and function. Adv Protein Chem. 1991;41:37–172. doi: 10.1016/s0065-3233(08)60197-7. [DOI] [PubMed] [Google Scholar]
- Sasaki N., Shiwa S., Yagihara S., Hikichi K. X-ray diffraction studies on the structure of hydrated collagen. Biopolymers. 1983 Dec;22(12):2539–2547. doi: 10.1002/bip.360221208. [DOI] [PubMed] [Google Scholar]
- Shinyashiki N., Asaka N., Mashimo S., Yagihara S., Sasaki N. Microwave dielectric study on hydration of moist collagen. 1990 Jul-Aug 5Biopolymers. 29(8-9):1185–1191. doi: 10.1002/bip.360290809. [DOI] [PubMed] [Google Scholar]
- Stillinger F. H. Water revisited. Science. 1980 Jul 25;209(4455):451–457. doi: 10.1126/science.209.4455.451. [DOI] [PubMed] [Google Scholar]