Abstract
The synaptic cleft may be represented as a very thin disk of extracellular fluid. It is possible that at high stimulation frequencies the interval between pulses would be insufficient for diffusion of Ca2+ from the periphery of the cleft to replace extracellular Ca2+ depleted at the center of the cleft as a result of activation of postsynaptic, Ca2(+)-permeable channels. Computer modeling was employed to assess the impact of activation of glutamate receptor channels (GRCs) in the postsynaptic membrane on the level of extracellular Ca2+ within the synaptic cleft. The model includes calcium influx from the synaptic cleft into the postsynaptic compartment through GRC and calcium efflux through calcium pumps and Na/Ca exchangers. Concentrations of extracellular Ca2+ inside the cleft are estimated by using a compartmental model incorporating flux across the postsynaptic membrane and radial diffusion from the edges of the cleft. The simulations suggest that substantial extracellular Ca2+ depletion can occur in the clefts during activation of GRCs, particularly at high stimulation frequencies used to induce long-term potentiation (LTP). Only minimal transitory changes in extracellular Ca2+ are observed at low frequencies. These frequency-dependent alterations in extracellular Ca2+ dynamics are a direct reflection of the activity of GRCs and could be involved in the modulation of presynaptic function via a retrograde messenger mechanism, if there are extracellular Ca2+ sensors on the presynaptic membranes. The recently cloned extracellular Ca2(+)-sensing receptors that are known to be present in nerve terminals in hippocampus and other areas of the brain could potentially play such a role.
Full text
PDF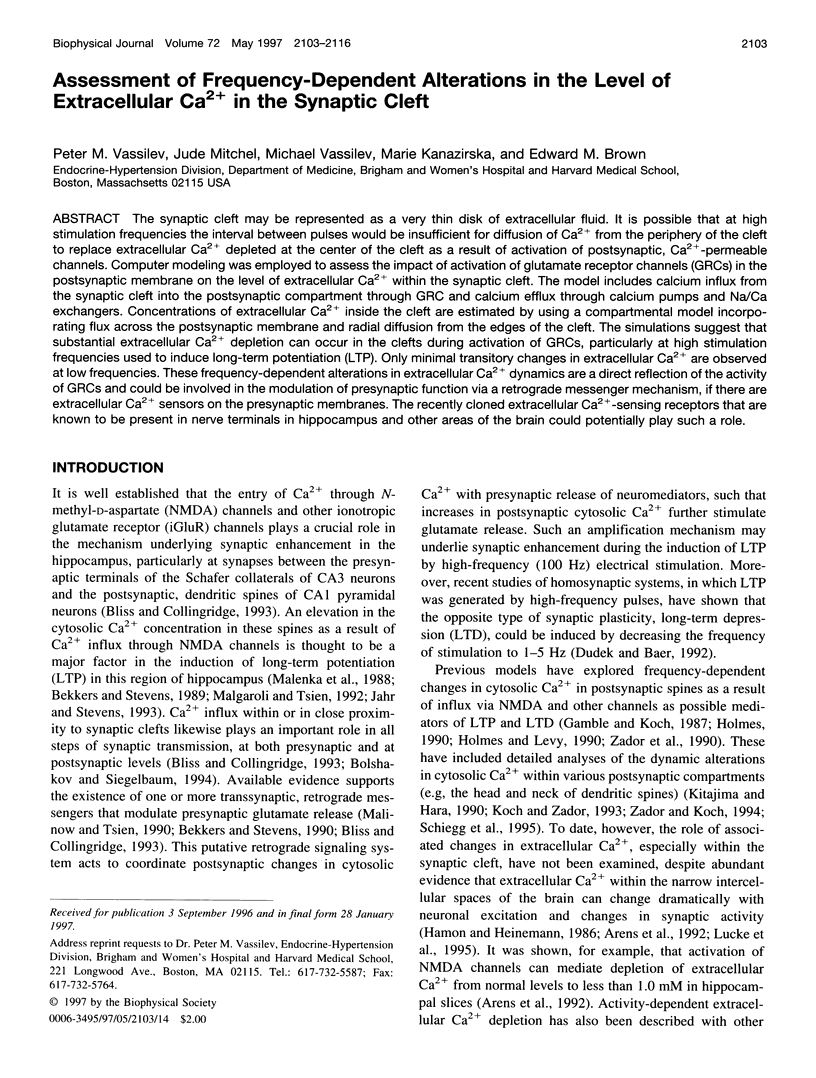
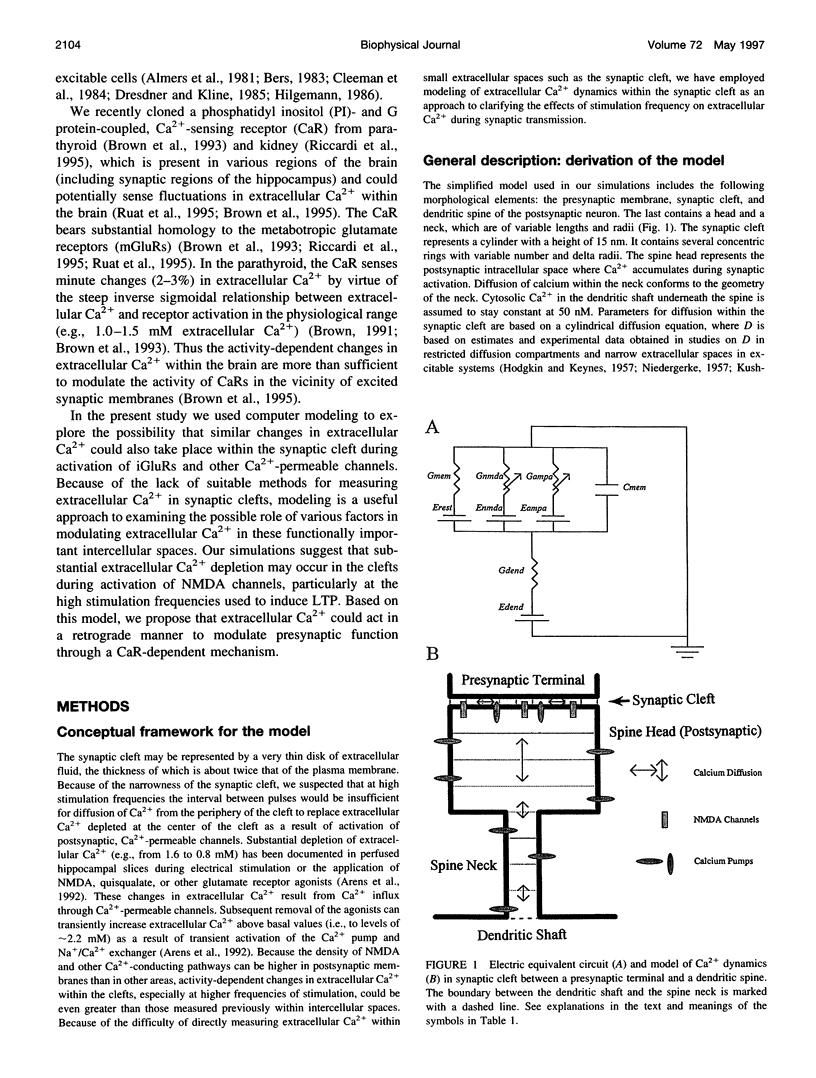
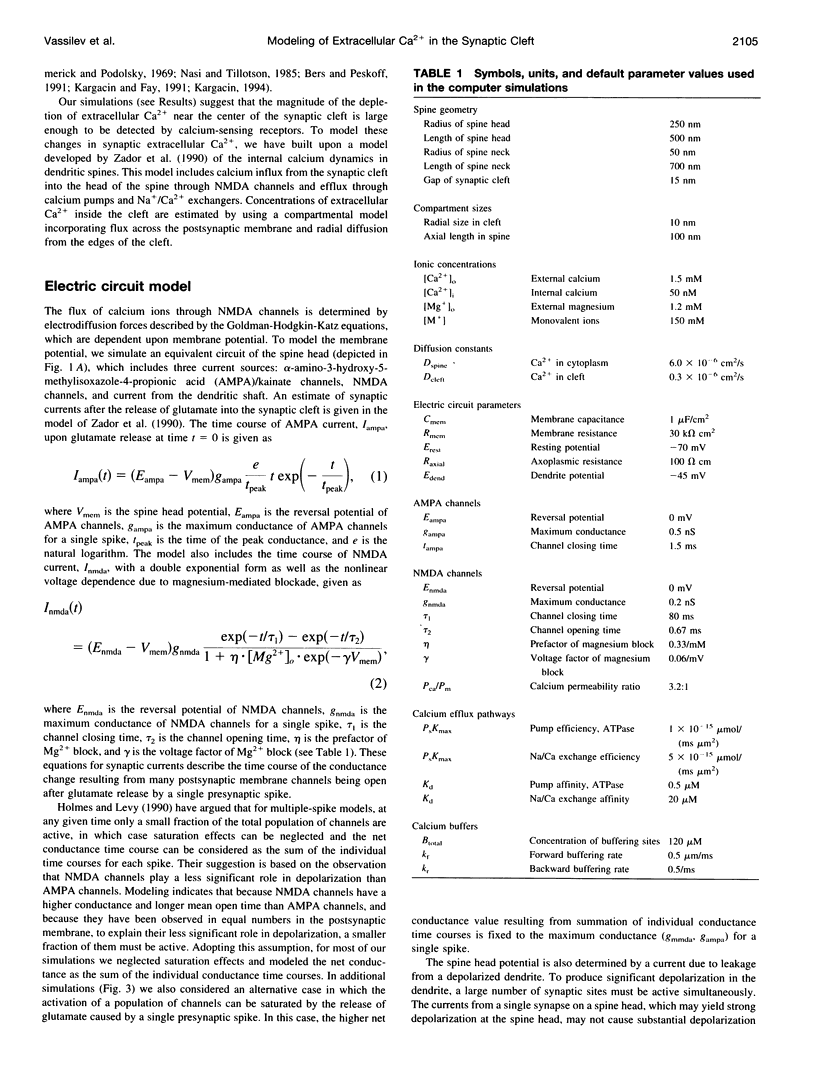
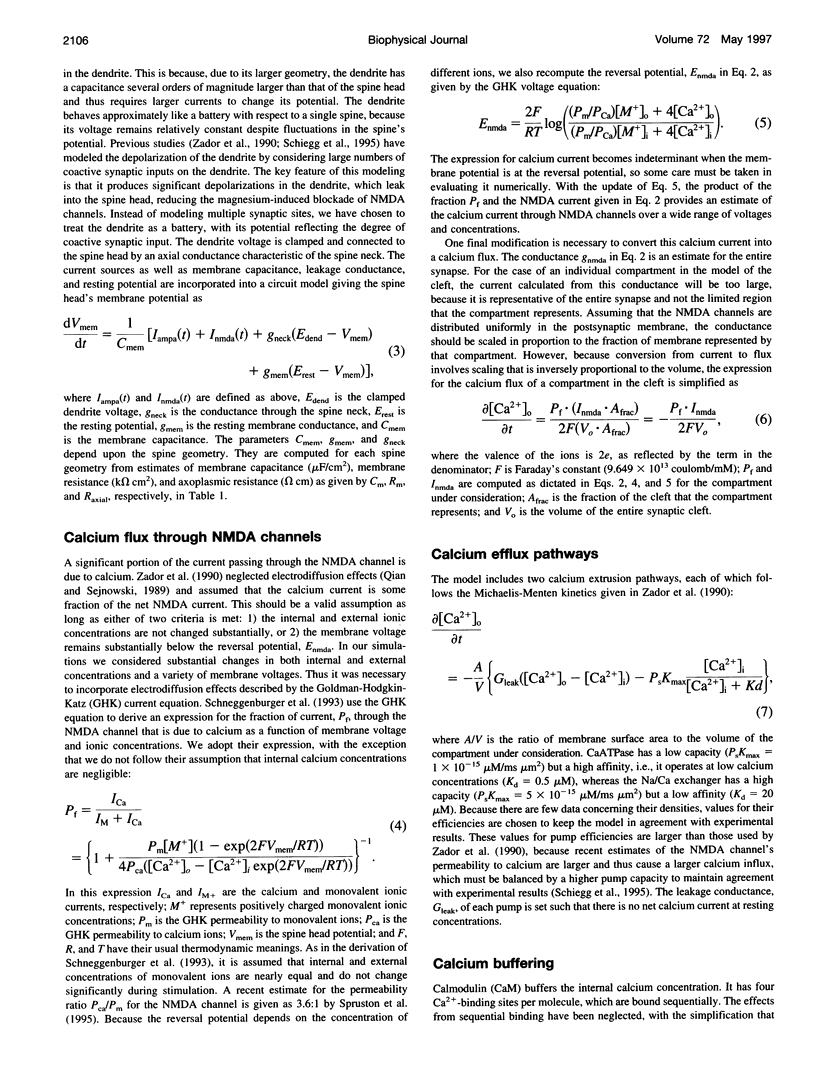
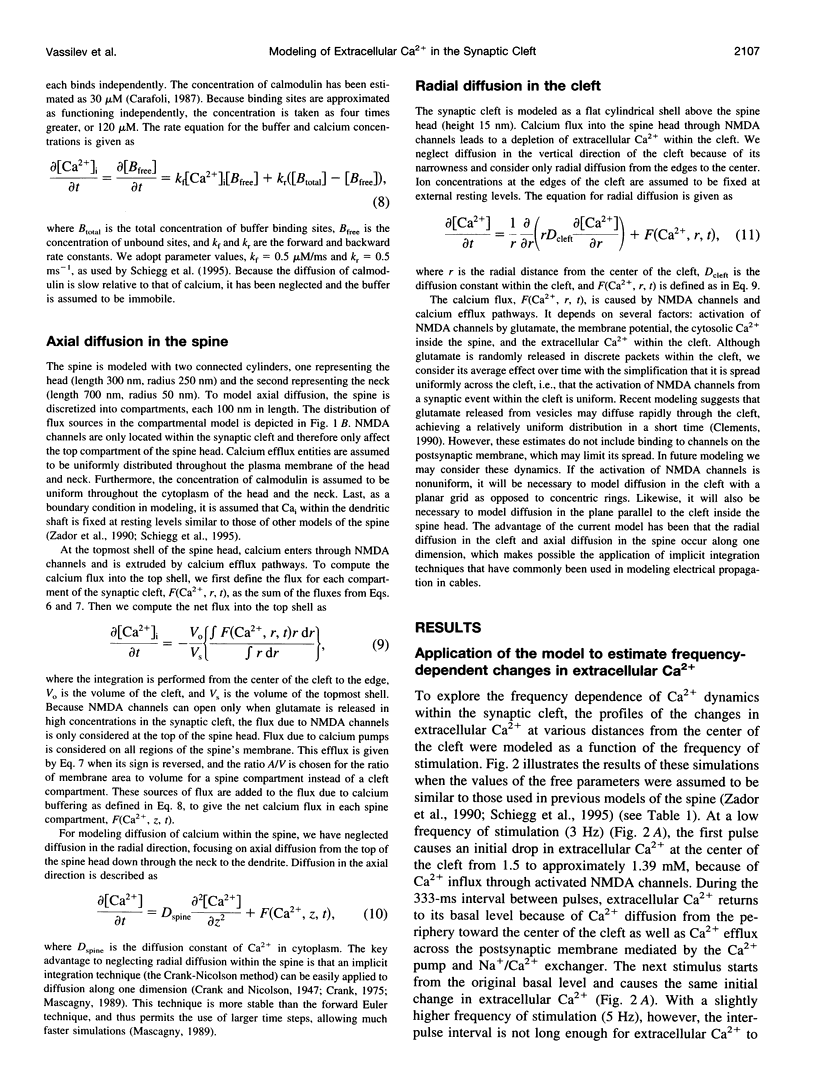
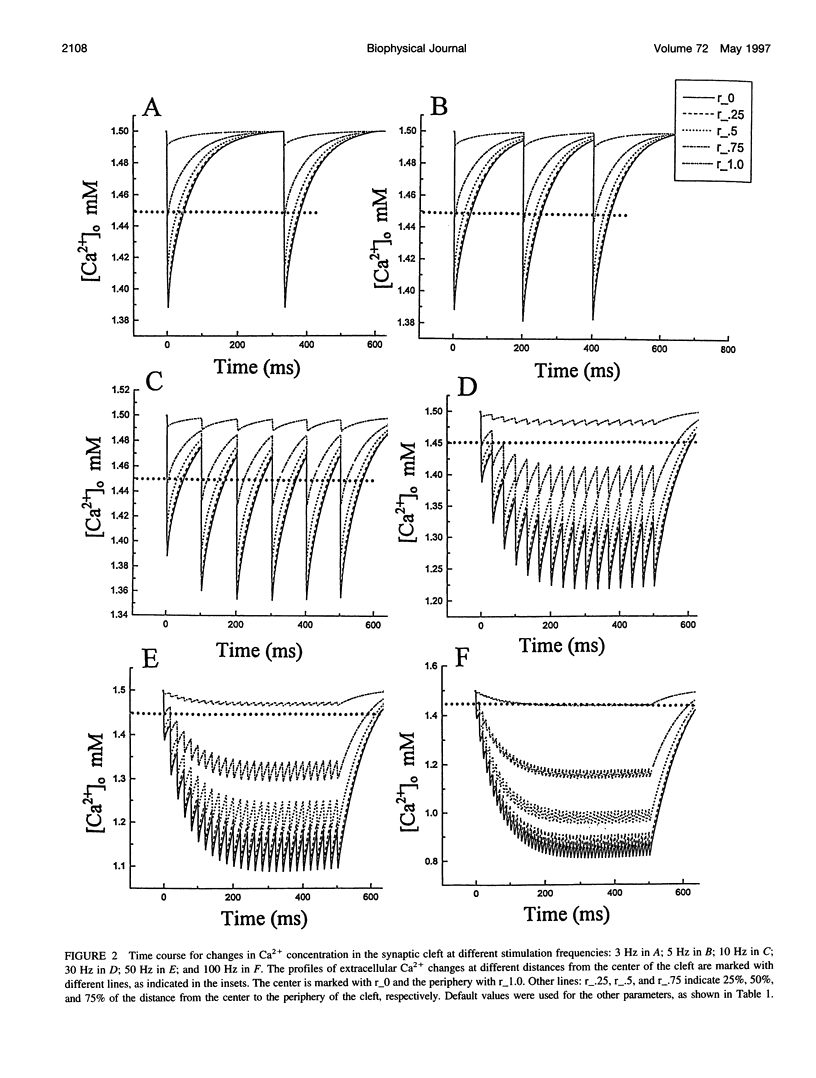
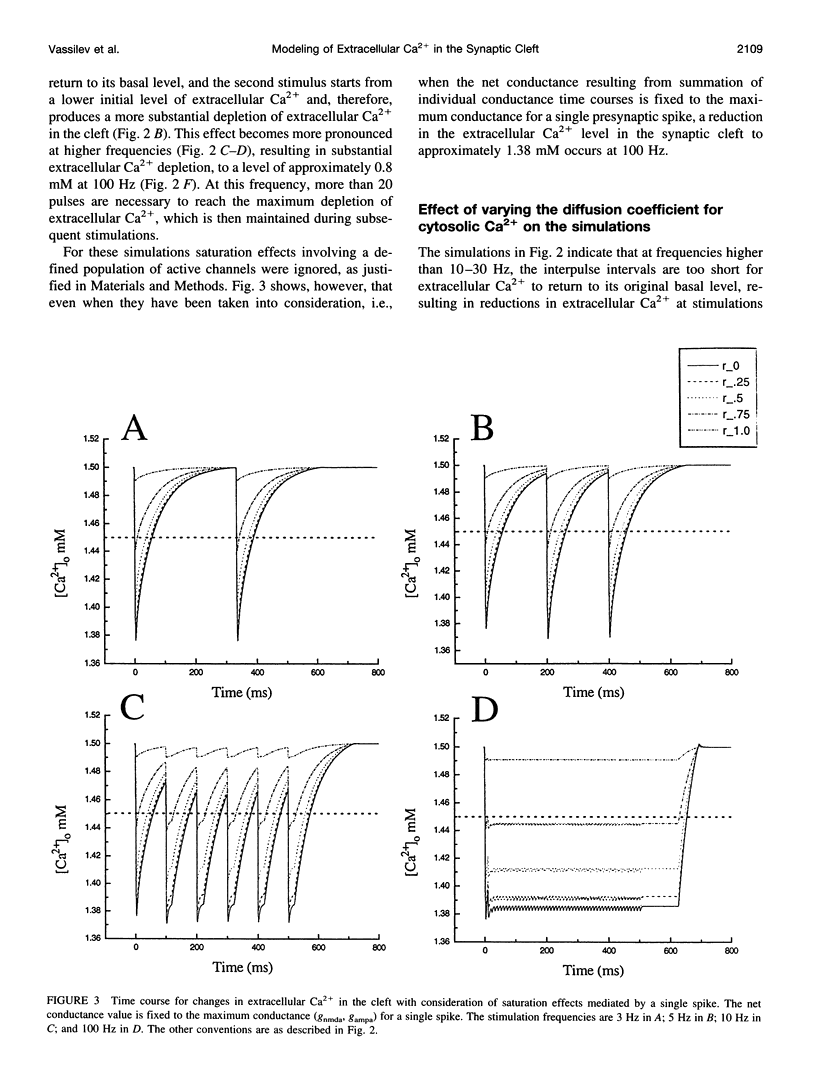
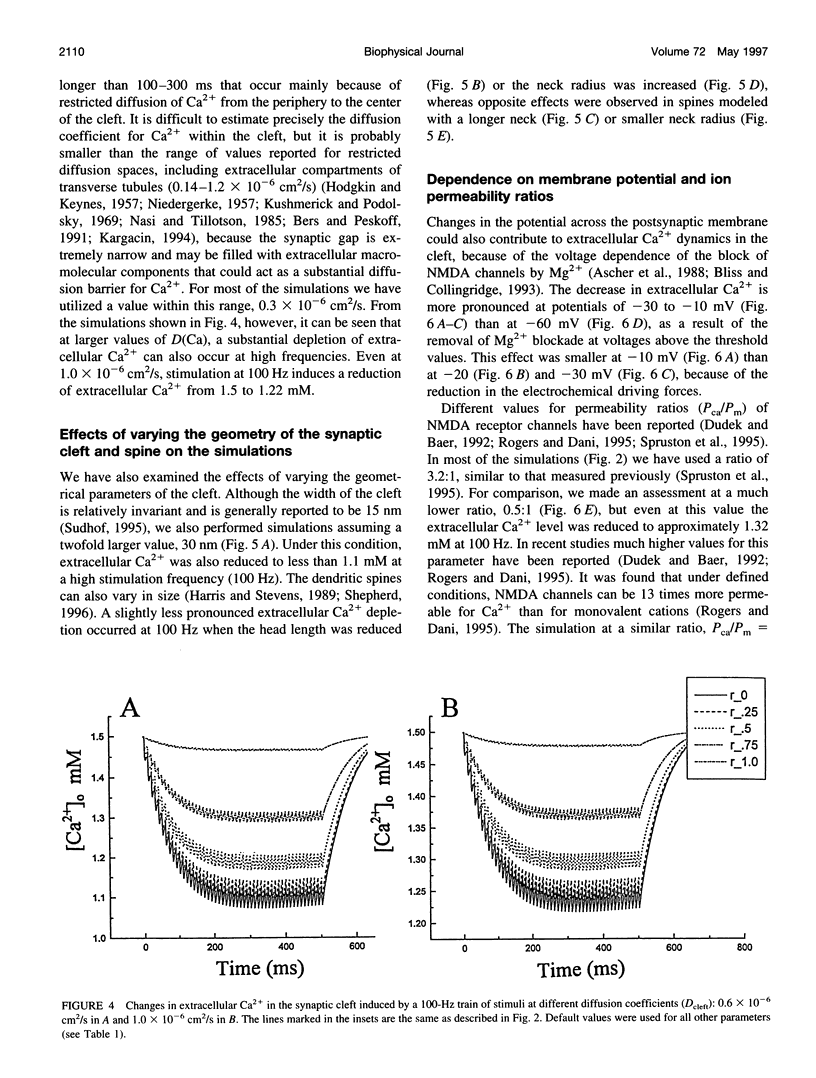
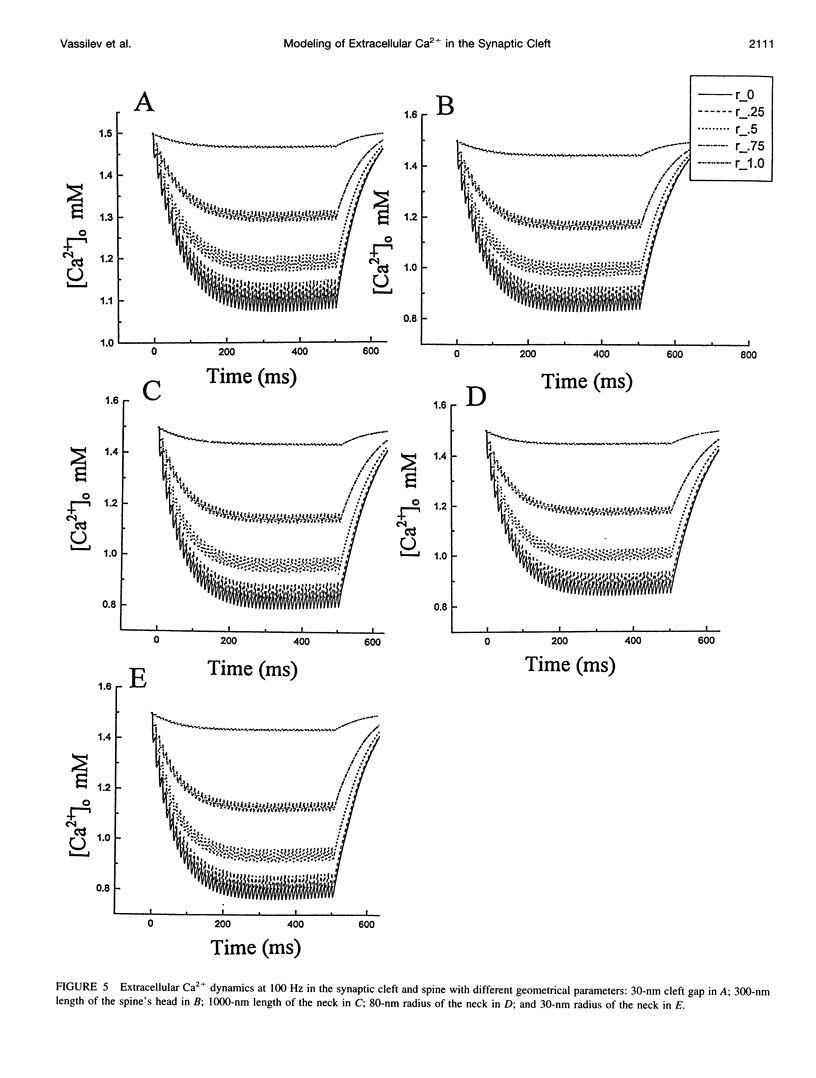
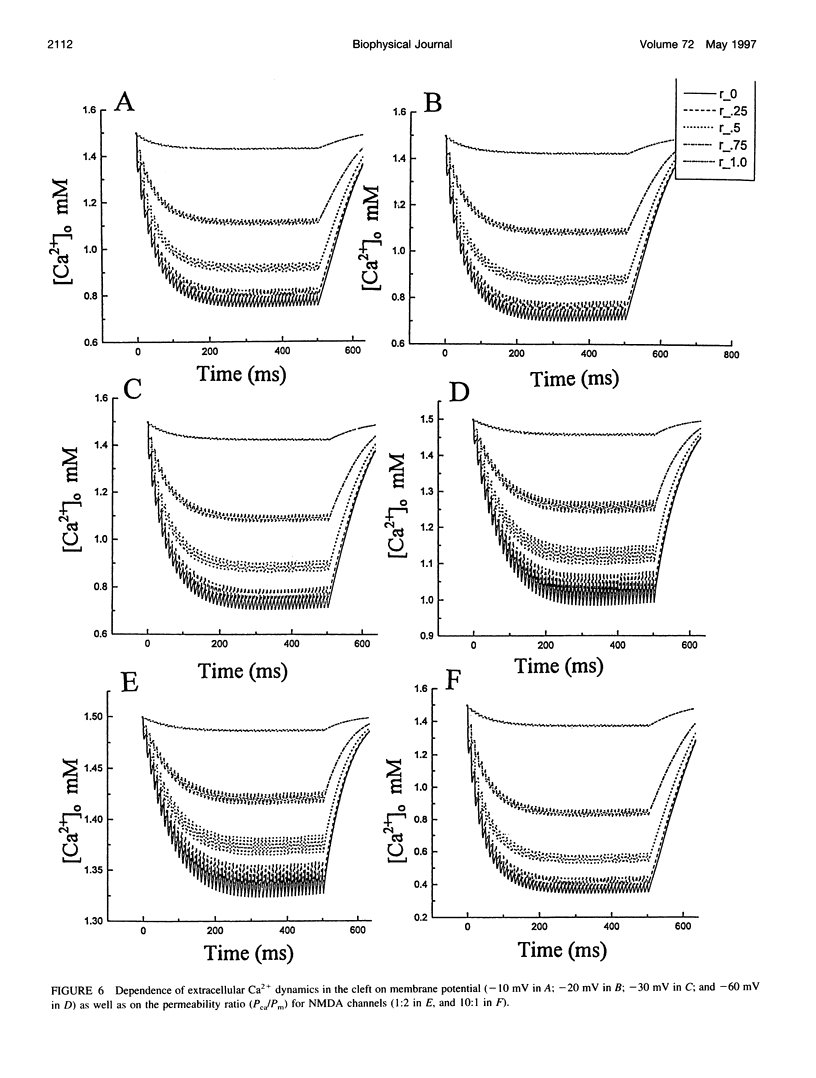
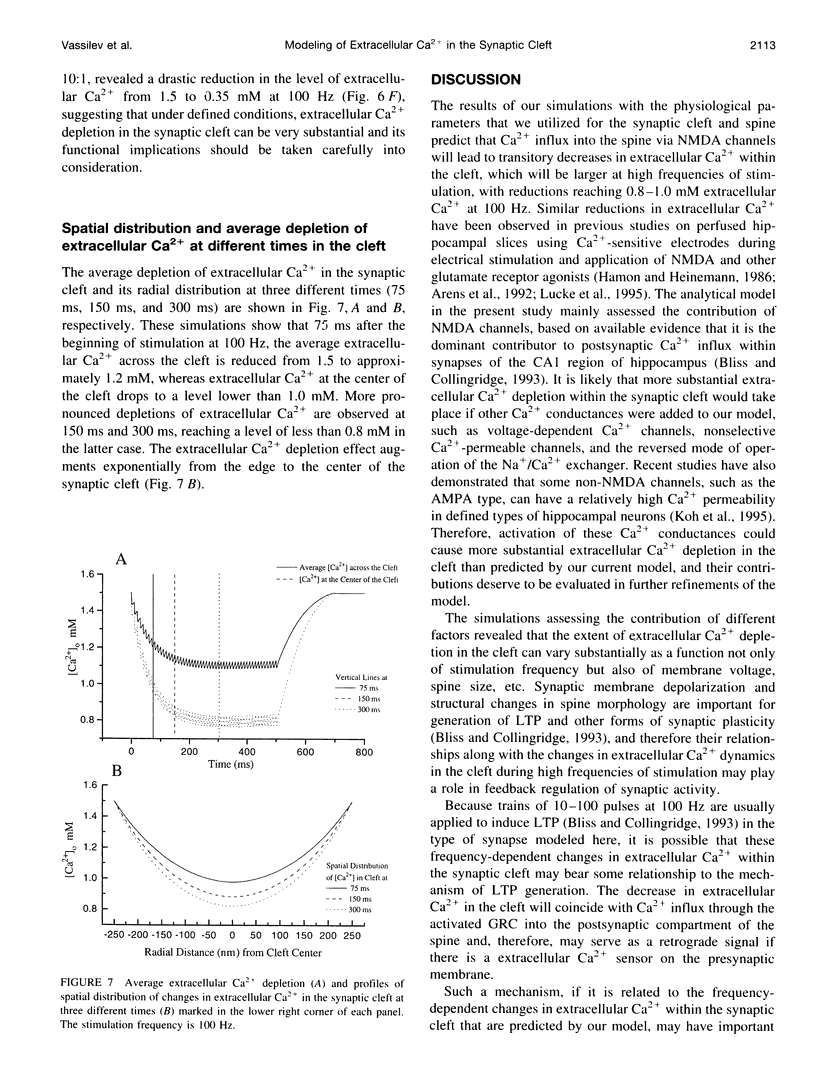
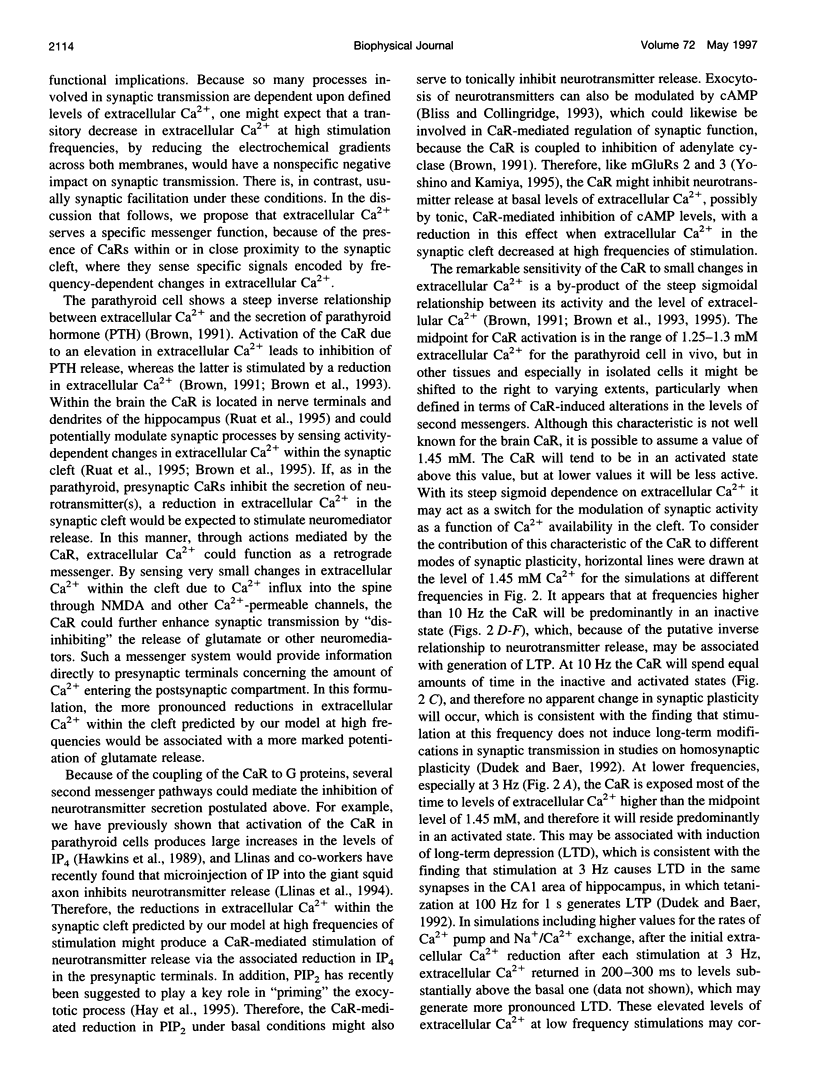
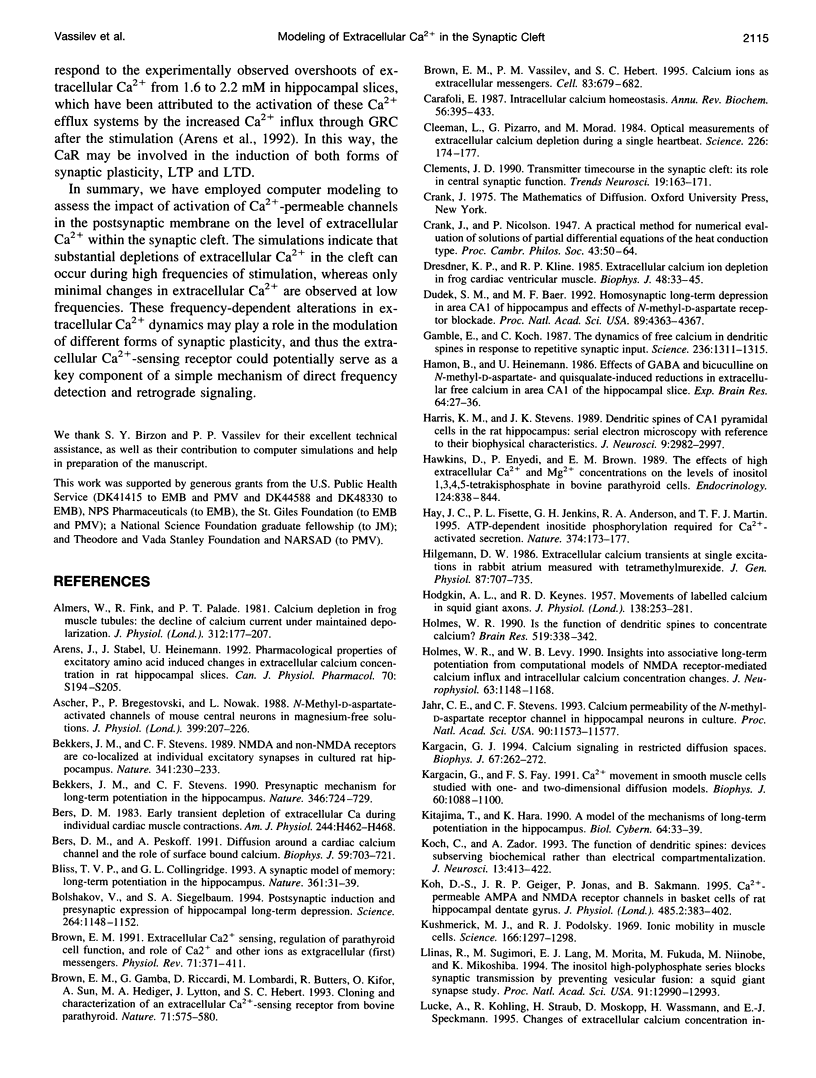
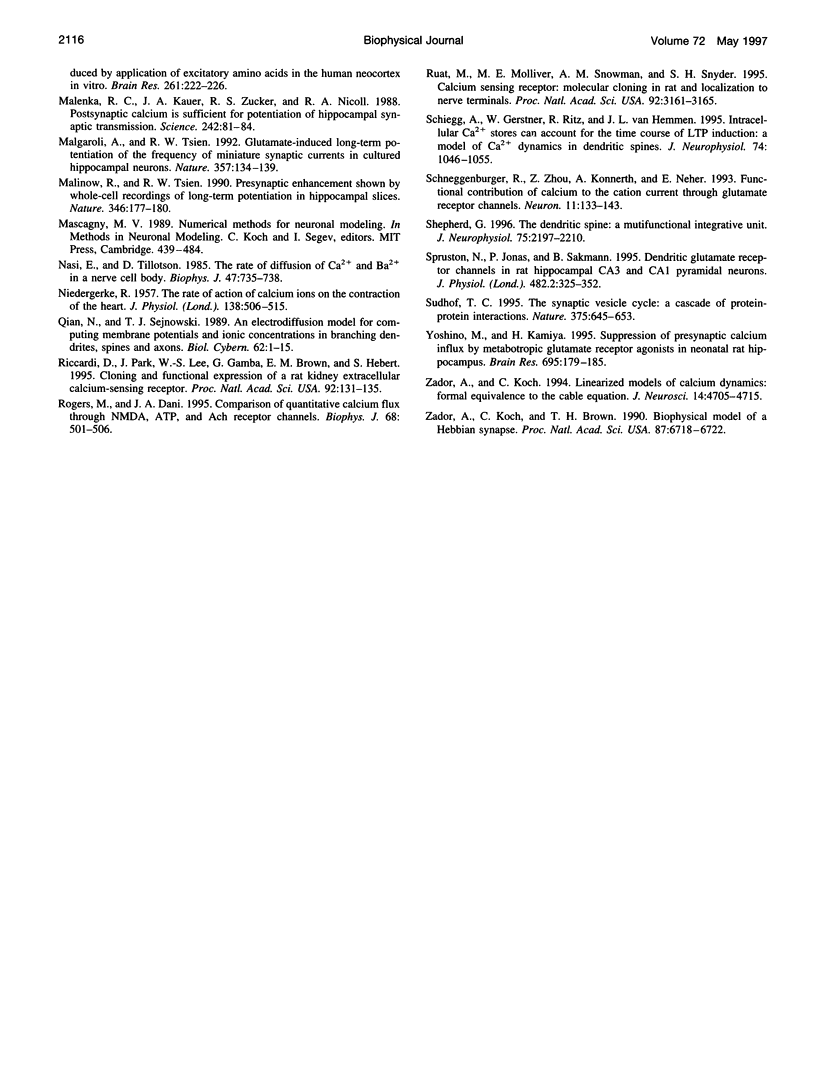
Selected References
These references are in PubMed. This may not be the complete list of references from this article.
- Almers W., Fink R., Palade P. T. Calcium depletion in frog muscle tubules: the decline of calcium current under maintained depolarization. J Physiol. 1981 Mar;312:177–207. doi: 10.1113/jphysiol.1981.sp013623. [DOI] [PMC free article] [PubMed] [Google Scholar]
- Arens J., Stabel J., Heinemann U. Pharmacological properties of excitatory amino acid induced changes in extracellular calcium concentration in rat hippocampal slices. Can J Physiol Pharmacol. 1992;70 (Suppl):S194–S205. doi: 10.1139/y92-263. [DOI] [PubMed] [Google Scholar]
- Ascher P., Bregestovski P., Nowak L. N-methyl-D-aspartate-activated channels of mouse central neurones in magnesium-free solutions. J Physiol. 1988 May;399:207–226. doi: 10.1113/jphysiol.1988.sp017076. [DOI] [PMC free article] [PubMed] [Google Scholar]
- Bekkers J. M., Stevens C. F. NMDA and non-NMDA receptors are co-localized at individual excitatory synapses in cultured rat hippocampus. Nature. 1989 Sep 21;341(6239):230–233. doi: 10.1038/341230a0. [DOI] [PubMed] [Google Scholar]
- Bekkers J. M., Stevens C. F. Presynaptic mechanism for long-term potentiation in the hippocampus. Nature. 1990 Aug 23;346(6286):724–729. doi: 10.1038/346724a0. [DOI] [PubMed] [Google Scholar]
- Bers D. M. Early transient depletion of extracellular Ca during individual cardiac muscle contractions. Am J Physiol. 1983 Mar;244(3):H462–H468. doi: 10.1152/ajpheart.1983.244.3.H462. [DOI] [PubMed] [Google Scholar]
- Bers D. M., Peskoff A. Diffusion around a cardiac calcium channel and the role of surface bound calcium. Biophys J. 1991 Mar;59(3):703–721. doi: 10.1016/S0006-3495(91)82284-6. [DOI] [PMC free article] [PubMed] [Google Scholar]
- Bliss T. V., Collingridge G. L. A synaptic model of memory: long-term potentiation in the hippocampus. Nature. 1993 Jan 7;361(6407):31–39. doi: 10.1038/361031a0. [DOI] [PubMed] [Google Scholar]
- Bolshakov V. Y., Siegelbaum S. A. Postsynaptic induction and presynaptic expression of hippocampal long-term depression. Science. 1994 May 20;264(5162):1148–1152. doi: 10.1126/science.7909958. [DOI] [PubMed] [Google Scholar]
- Brown E. M. Extracellular Ca2+ sensing, regulation of parathyroid cell function, and role of Ca2+ and other ions as extracellular (first) messengers. Physiol Rev. 1991 Apr;71(2):371–411. doi: 10.1152/physrev.1991.71.2.371. [DOI] [PubMed] [Google Scholar]
- Brown E. M., Gamba G., Riccardi D., Lombardi M., Butters R., Kifor O., Sun A., Hediger M. A., Lytton J., Hebert S. C. Cloning and characterization of an extracellular Ca(2+)-sensing receptor from bovine parathyroid. Nature. 1993 Dec 9;366(6455):575–580. doi: 10.1038/366575a0. [DOI] [PubMed] [Google Scholar]
- Brown E. M., Vassilev P. M., Hebert S. C. Calcium ions as extracellular messengers. Cell. 1995 Dec 1;83(5):679–682. doi: 10.1016/0092-8674(95)90180-9. [DOI] [PubMed] [Google Scholar]
- Carafoli E. Intracellular calcium homeostasis. Annu Rev Biochem. 1987;56:395–433. doi: 10.1146/annurev.bi.56.070187.002143. [DOI] [PubMed] [Google Scholar]
- Cleemann L., Pizarro G., Morad M. Optical measurements of extracellular calcium depletion during a single heartbeat. Science. 1984 Oct 12;226(4671):174–177. doi: 10.1126/science.6091269. [DOI] [PubMed] [Google Scholar]
- Clements J. D. Transmitter timecourse in the synaptic cleft: its role in central synaptic function. Trends Neurosci. 1996 May;19(5):163–171. doi: 10.1016/s0166-2236(96)10024-2. [DOI] [PubMed] [Google Scholar]
- Dresdner K. P., Kline R. P. Extracellular calcium ion depletion in frog cardiac ventricular muscle. Biophys J. 1985 Jul;48(1):33–45. doi: 10.1016/S0006-3495(85)83758-9. [DOI] [PMC free article] [PubMed] [Google Scholar]
- Dudek S. M., Bear M. F. Homosynaptic long-term depression in area CA1 of hippocampus and effects of N-methyl-D-aspartate receptor blockade. Proc Natl Acad Sci U S A. 1992 May 15;89(10):4363–4367. doi: 10.1073/pnas.89.10.4363. [DOI] [PMC free article] [PubMed] [Google Scholar]
- Gamble E., Koch C. The dynamics of free calcium in dendritic spines in response to repetitive synaptic input. Science. 1987 Jun 5;236(4806):1311–1315. doi: 10.1126/science.3495885. [DOI] [PubMed] [Google Scholar]
- HODGKIN A. L., KEYNES R. D. Movements of labelled calcium in squid giant axons. J Physiol. 1957 Sep 30;138(2):253–281. doi: 10.1113/jphysiol.1957.sp005850. [DOI] [PMC free article] [PubMed] [Google Scholar]
- Hamon B., Heinemann U. Effects of GABA and bicuculline on N-methyl-D-aspartate- and quisqualate-induced reductions in extracellular free calcium in area CA1 of the hippocampal slice. Exp Brain Res. 1986;64(1):27–36. doi: 10.1007/BF00238198. [DOI] [PubMed] [Google Scholar]
- Harris K. M., Stevens J. K. Dendritic spines of CA 1 pyramidal cells in the rat hippocampus: serial electron microscopy with reference to their biophysical characteristics. J Neurosci. 1989 Aug;9(8):2982–2997. doi: 10.1523/JNEUROSCI.09-08-02982.1989. [DOI] [PMC free article] [PubMed] [Google Scholar]
- Hawkins D., Enyedi P., Brown E. The effects of high extracellular Ca2+ and Mg2+ concentrations on the levels of inositol 1,3,4,5-tetrakisphosphate in bovine parathyroid cells. Endocrinology. 1989 Feb;124(2):838–844. doi: 10.1210/endo-124-2-838. [DOI] [PubMed] [Google Scholar]
- Hay J. C., Fisette P. L., Jenkins G. H., Fukami K., Takenawa T., Anderson R. A., Martin T. F. ATP-dependent inositide phosphorylation required for Ca(2+)-activated secretion. Nature. 1995 Mar 9;374(6518):173–177. doi: 10.1038/374173a0. [DOI] [PubMed] [Google Scholar]
- Hilgemann D. W. Extracellular calcium transients at single excitations in rabbit atrium measured with tetramethylmurexide. J Gen Physiol. 1986 May;87(5):707–735. doi: 10.1085/jgp.87.5.707. [DOI] [PMC free article] [PubMed] [Google Scholar]
- Holmes W. R. Is the function of dendritic spines to concentrate calcium? Brain Res. 1990 Jun 11;519(1-2):338–342. doi: 10.1016/0006-8993(90)90098-v. [DOI] [PubMed] [Google Scholar]
- Holmes W. R., Levy W. B. Insights into associative long-term potentiation from computational models of NMDA receptor-mediated calcium influx and intracellular calcium concentration changes. J Neurophysiol. 1990 May;63(5):1148–1168. doi: 10.1152/jn.1990.63.5.1148. [DOI] [PubMed] [Google Scholar]
- Jahr C. E., Stevens C. F. Calcium permeability of the N-methyl-D-aspartate receptor channel in hippocampal neurons in culture. Proc Natl Acad Sci U S A. 1993 Dec 15;90(24):11573–11577. doi: 10.1073/pnas.90.24.11573. [DOI] [PMC free article] [PubMed] [Google Scholar]
- Kargacin G. J. Calcium signaling in restricted diffusion spaces. Biophys J. 1994 Jul;67(1):262–272. doi: 10.1016/S0006-3495(94)80477-1. [DOI] [PMC free article] [PubMed] [Google Scholar]
- Kargacin G., Fay F. S. Ca2+ movement in smooth muscle cells studied with one- and two-dimensional diffusion models. Biophys J. 1991 Nov;60(5):1088–1100. doi: 10.1016/S0006-3495(91)82145-2. [DOI] [PMC free article] [PubMed] [Google Scholar]
- Kitajima T., Hara K. A model of the mechanisms of long-term potentiation in the hippocampus. Biol Cybern. 1990;64(1):33–39. doi: 10.1007/BF00203628. [DOI] [PubMed] [Google Scholar]
- Koch C., Zador A. The function of dendritic spines: devices subserving biochemical rather than electrical compartmentalization. J Neurosci. 1993 Feb;13(2):413–422. doi: 10.1523/JNEUROSCI.13-02-00413.1993. [DOI] [PMC free article] [PubMed] [Google Scholar]
- Koh D. S., Geiger J. R., Jonas P., Sakmann B. Ca(2+)-permeable AMPA and NMDA receptor channels in basket cells of rat hippocampal dentate gyrus. J Physiol. 1995 Jun 1;485(Pt 2):383–402. doi: 10.1113/jphysiol.1995.sp020737. [DOI] [PMC free article] [PubMed] [Google Scholar]
- Kushmerick M. J., Podolsky R. J. Ionic mobility in muscle cells. Science. 1969 Dec 5;166(3910):1297–1298. doi: 10.1126/science.166.3910.1297. [DOI] [PubMed] [Google Scholar]
- Llinás R., Sugimori M., Lang E. J., Morita M., Fukuda M., Niinobe M., Mikoshiba K. The inositol high-polyphosphate series blocks synaptic transmission by preventing vesicular fusion: a squid giant synapse study. Proc Natl Acad Sci U S A. 1994 Dec 20;91(26):12990–12993. doi: 10.1073/pnas.91.26.12990. [DOI] [PMC free article] [PubMed] [Google Scholar]
- Lücke A., Köhling R., Straub H., Moskopp D., Wassmann H., Speckmann E. J. Changes of extracellular calcium concentration induced by application of excitatory amino acids in the human neocortex in vitro. Brain Res. 1995 Feb 13;671(2):222–226. doi: 10.1016/0006-8993(94)01330-k. [DOI] [PubMed] [Google Scholar]
- Malenka R. C., Kauer J. A., Zucker R. S., Nicoll R. A. Postsynaptic calcium is sufficient for potentiation of hippocampal synaptic transmission. Science. 1988 Oct 7;242(4875):81–84. doi: 10.1126/science.2845577. [DOI] [PubMed] [Google Scholar]
- Malgaroli A., Tsien R. W. Glutamate-induced long-term potentiation of the frequency of miniature synaptic currents in cultured hippocampal neurons. Nature. 1992 May 14;357(6374):134–139. doi: 10.1038/357134a0. [DOI] [PubMed] [Google Scholar]
- Malinow R., Tsien R. W. Presynaptic enhancement shown by whole-cell recordings of long-term potentiation in hippocampal slices. Nature. 1990 Jul 12;346(6280):177–180. doi: 10.1038/346177a0. [DOI] [PubMed] [Google Scholar]
- NIEDERGERKE R. The rate of action of calcium ions on the contraction of the heart. J Physiol. 1957 Oct 30;138(3):506–515. doi: 10.1113/jphysiol.1957.sp005867. [DOI] [PMC free article] [PubMed] [Google Scholar]
- Nasi E., Tillotson D. The rate of diffusion of Ca2+ and Ba2+ in a nerve cell body. Biophys J. 1985 May;47(5):735–738. doi: 10.1016/S0006-3495(85)83972-2. [DOI] [PMC free article] [PubMed] [Google Scholar]
- Riccardi D., Park J., Lee W. S., Gamba G., Brown E. M., Hebert S. C. Cloning and functional expression of a rat kidney extracellular calcium/polyvalent cation-sensing receptor. Proc Natl Acad Sci U S A. 1995 Jan 3;92(1):131–135. doi: 10.1073/pnas.92.1.131. [DOI] [PMC free article] [PubMed] [Google Scholar]
- Rogers M., Dani J. A. Comparison of quantitative calcium flux through NMDA, ATP, and ACh receptor channels. Biophys J. 1995 Feb;68(2):501–506. doi: 10.1016/S0006-3495(95)80211-0. [DOI] [PMC free article] [PubMed] [Google Scholar]
- Ruat M., Molliver M. E., Snowman A. M., Snyder S. H. Calcium sensing receptor: molecular cloning in rat and localization to nerve terminals. Proc Natl Acad Sci U S A. 1995 Apr 11;92(8):3161–3165. doi: 10.1073/pnas.92.8.3161. [DOI] [PMC free article] [PubMed] [Google Scholar]
- Schiegg A., Gerstner W., Ritz R., van Hemmen J. L. Intracellular Ca2+ stores can account for the time course of LTP induction: a model of Ca2+ dynamics in dendritic spines. J Neurophysiol. 1995 Sep;74(3):1046–1055. doi: 10.1152/jn.1995.74.3.1046. [DOI] [PubMed] [Google Scholar]
- Schneggenburger R., Zhou Z., Konnerth A., Neher E. Fractional contribution of calcium to the cation current through glutamate receptor channels. Neuron. 1993 Jul;11(1):133–143. doi: 10.1016/0896-6273(93)90277-x. [DOI] [PubMed] [Google Scholar]
- Shepherd G. M. The dendritic spine: a multifunctional integrative unit. J Neurophysiol. 1996 Jun;75(6):2197–2210. doi: 10.1152/jn.1996.75.6.2197. [DOI] [PubMed] [Google Scholar]
- Spruston N., Jonas P., Sakmann B. Dendritic glutamate receptor channels in rat hippocampal CA3 and CA1 pyramidal neurons. J Physiol. 1995 Jan 15;482(Pt 2):325–352. doi: 10.1113/jphysiol.1995.sp020521. [DOI] [PMC free article] [PubMed] [Google Scholar]
- Südhof T. C. The synaptic vesicle cycle: a cascade of protein-protein interactions. Nature. 1995 Jun 22;375(6533):645–653. doi: 10.1038/375645a0. [DOI] [PubMed] [Google Scholar]
- Yoshino M., Kamiya H. Suppression of presynaptic calcium influx by metabotropic glutamate receptor agonists in neonatal rat hippocampus. Brain Res. 1995 Oct 16;695(2):179–185. doi: 10.1016/0006-8993(95)00743-a. [DOI] [PubMed] [Google Scholar]
- Zador A., Koch C., Brown T. H. Biophysical model of a Hebbian synapse. Proc Natl Acad Sci U S A. 1990 Sep;87(17):6718–6722. doi: 10.1073/pnas.87.17.6718. [DOI] [PMC free article] [PubMed] [Google Scholar]
- Zador A., Koch C. Linearized models of calcium dynamics: formal equivalence to the cable equation. J Neurosci. 1994 Aug;14(8):4705–4715. doi: 10.1523/JNEUROSCI.14-08-04705.1994. [DOI] [PMC free article] [PubMed] [Google Scholar]