Abstract
The kinetics of light-induced electron transfer in reaction centers (RCs) from the purple photosynthetic bacterium Rhodobacter sphaeroides were studied in the presence of the detergent lauryldimethylamine-N-oxide (LDAO). After the light-induced electron transfer from the primary donor (P) to the acceptor quinone complex, the dark re-reduction of P+ reflects recombination from the reduced acceptor quinones, QA- or QB-. The secondary quinone, QB, which is loosely bound to the RC, determines the rate of this process. Electron transfer to QB slows down the return of the electron to P+, giving rise to a slow phase of the recovery kinetics with time tau P approximately 1 s, whereas charge recombination in RCs lacking QB generates a fast phase with time tau AP approximately 0.1 s. The amount of quinone bound to RC micelles can be reduced by increasing the detergent concentration. The characteristic time of the slow component of P+ dark relaxation, observed at low quinone content per RC micelle (at high detergent concentration), is about 1.2-1.5 s, in sharp contrast to expectations from previous models, according to which the time of the slow component should approach the time of the fast component (about 0.1 s) when the quinone concentration approaches zero. To account for this large discrepancy, a new quantitative approach has been developed to analyze the kinetics of electron transfer in isolated RCs with the following key features: 1) The exchange of quinone between different micelles (RC and detergent micelles) occurs more slowly than electron transfer from QB- to P+; 2) The exchange of quinone between the detergent "phase" and the QB binding site within the same RC micelle is much faster than electron transfer between QA- and P+; 3) The time of the slow component of P+ dark relaxation is determined by (n) > or = 1, the average number of quinones in RC micelles, calculated only for those RC micelles that have at least one quinone per RC (in excess of QA). An analytical function is derived that relates the time of the slow component of P+ relaxation, tau P, and the relative amplitude of the slow phase. This provides a useful means of determining the true equilibrium constant of electron transfer between QA and QB (LAB), and the association equilibrium constant of quinone binding at the QB site (KQ+). We found that LAB = 22 +/- 3 and KQ = 0.6 +/- 0.2 at pH 7.5. The analysis shows that saturation of the QB binding site in detergent-solubilized RCs is difficult to achieve with hydrophobic quinones. This has important implications for the interpretation of apparent dependencies of QB function on environmental parameters (e.g. pH) and on mutational alterations. The model accounts for the effects of detergent and quinone concentration on electron transfer in the acceptor quinone complex, and the conclusions are of general significance for the study of quinone-binding membrane proteins in detergent solutions.
Full text
PDF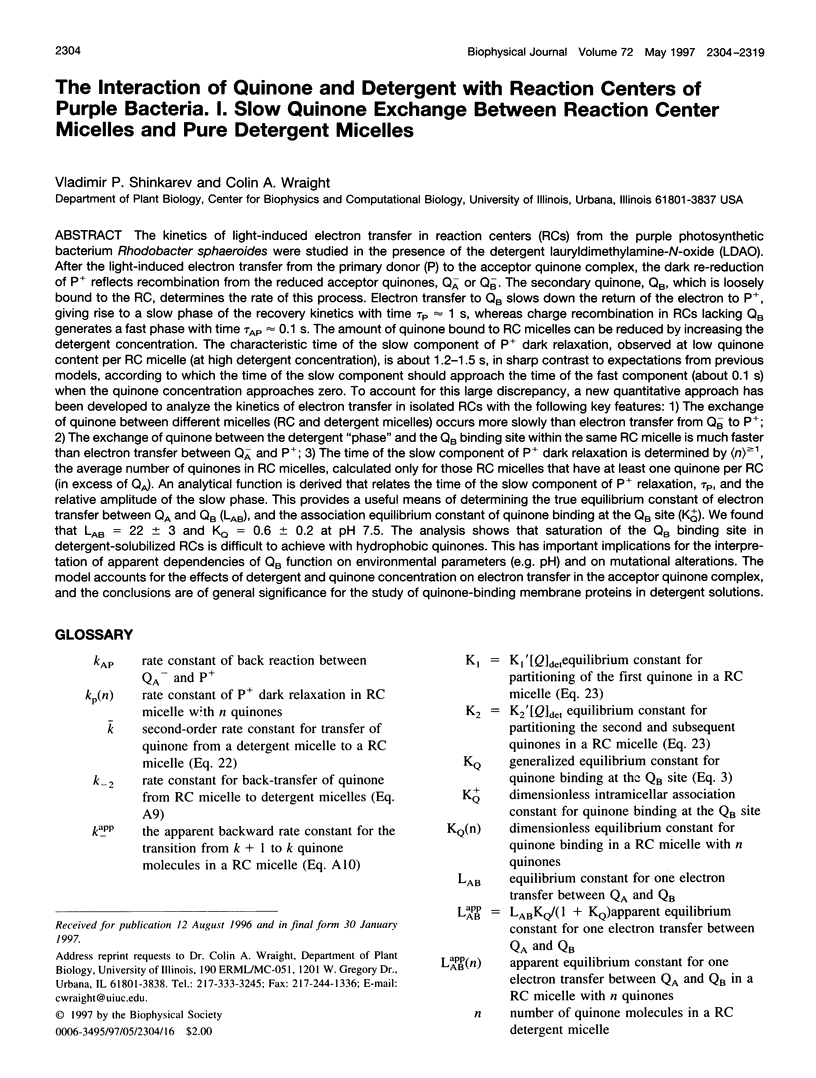
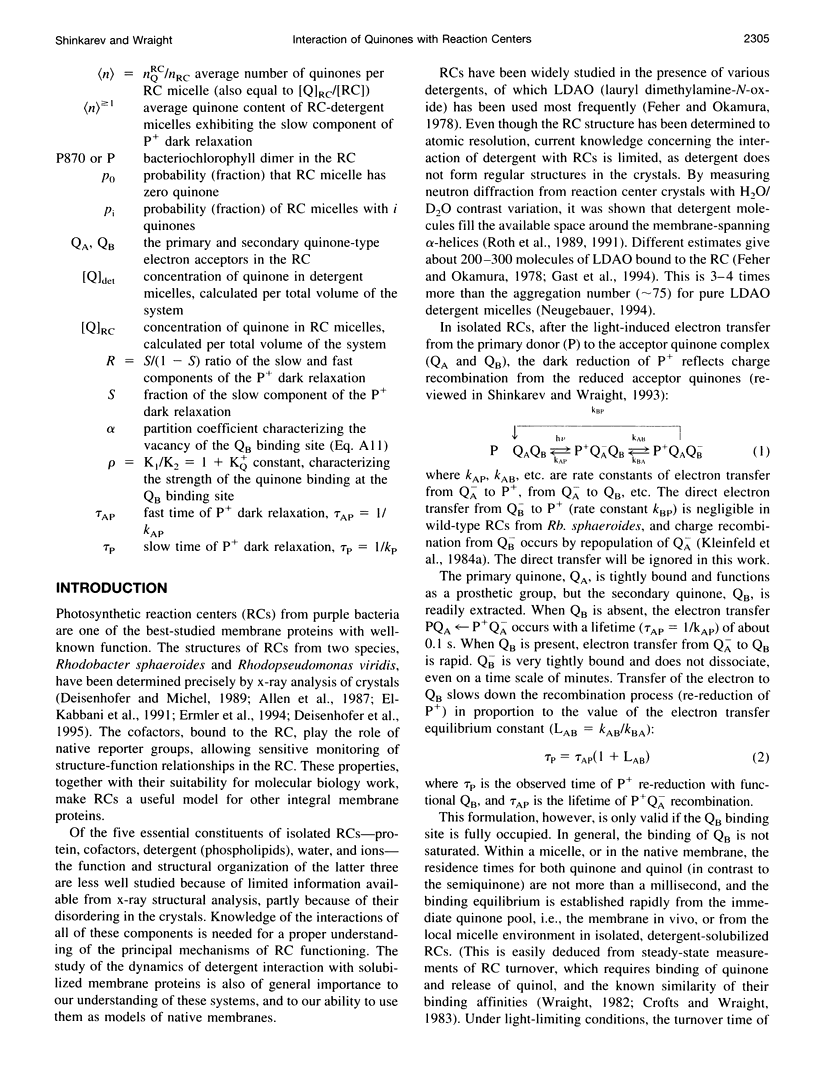
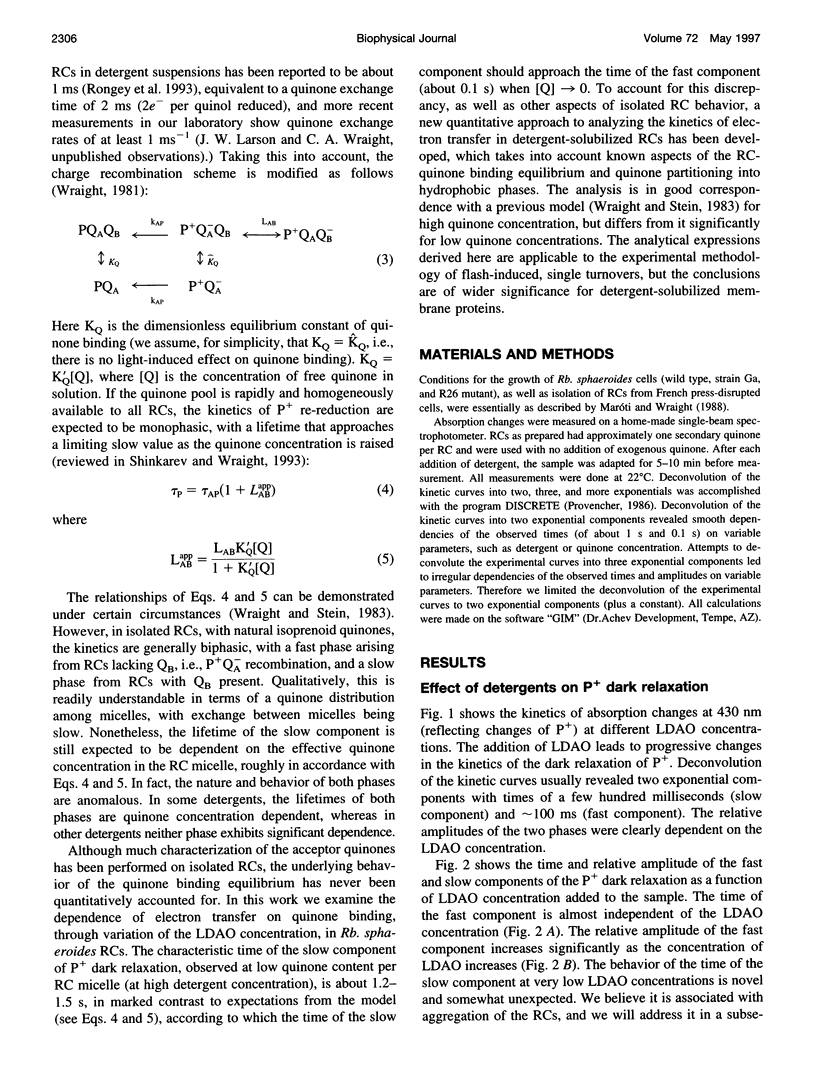
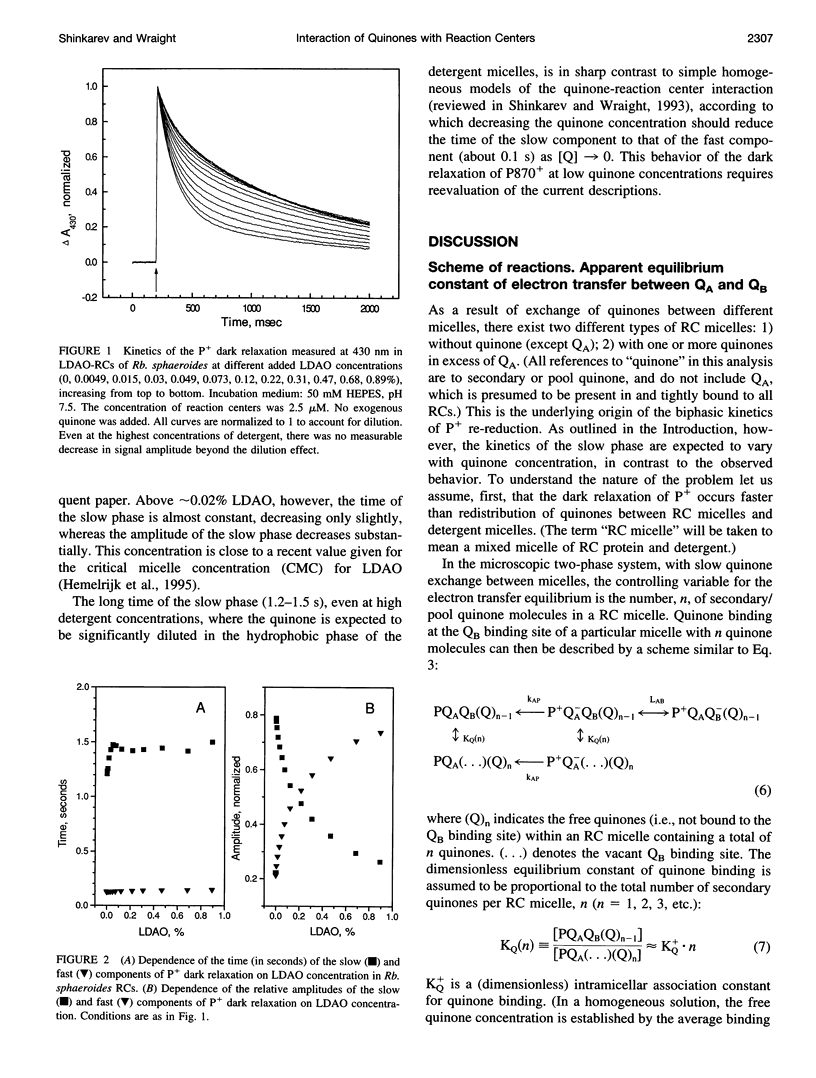
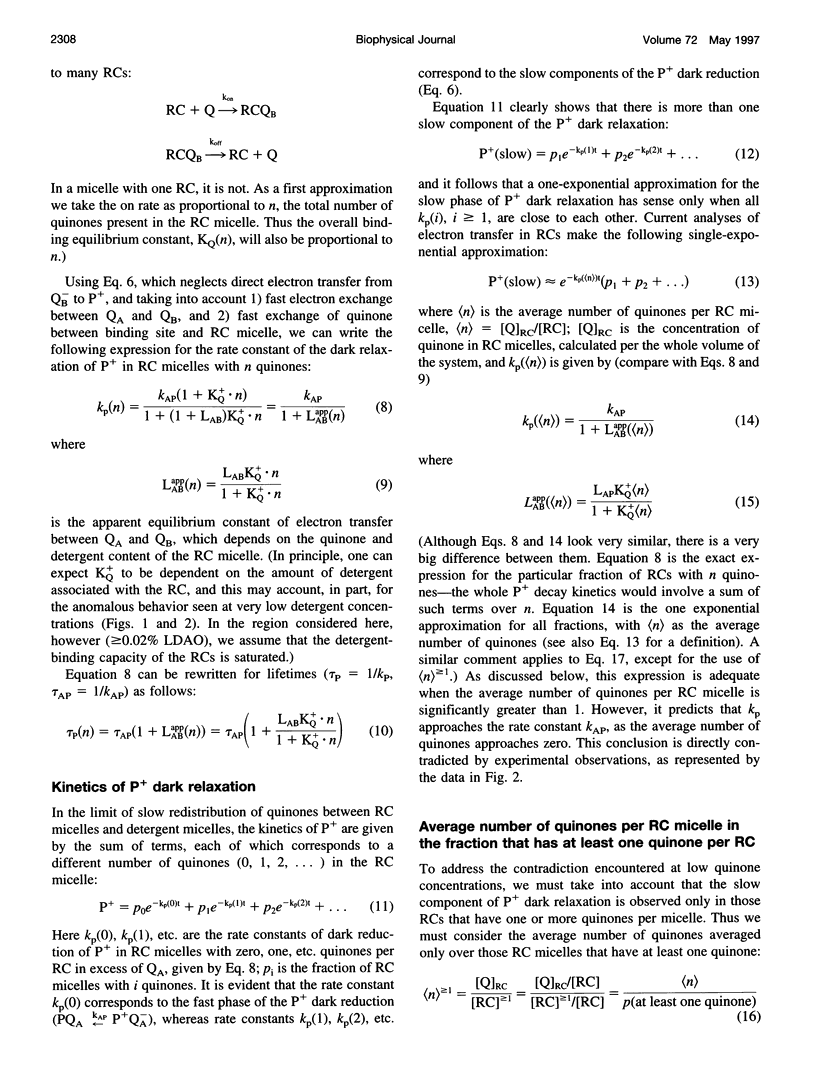
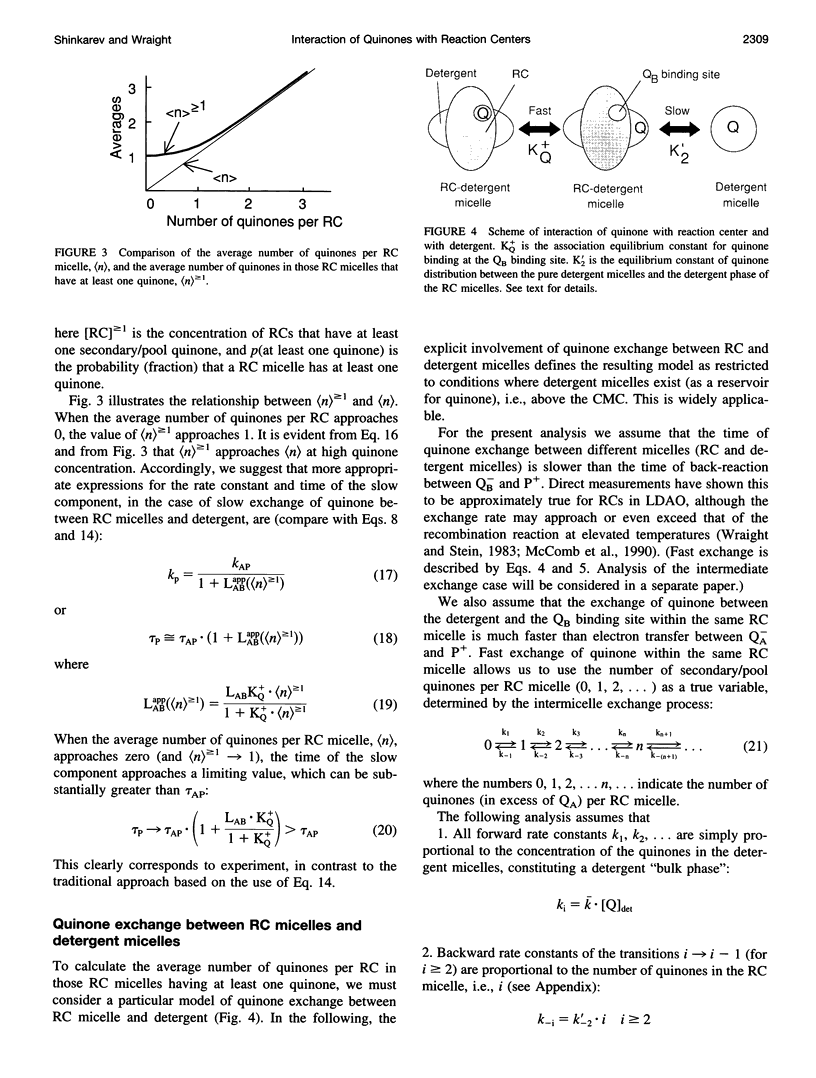
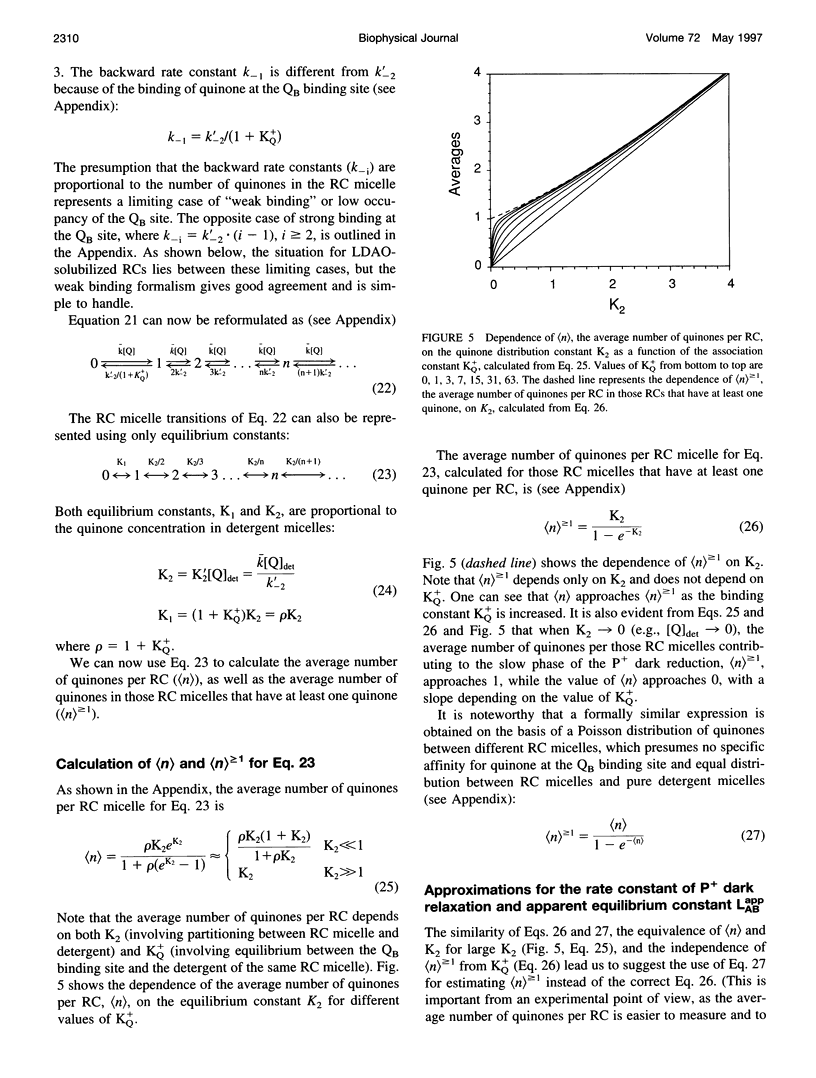
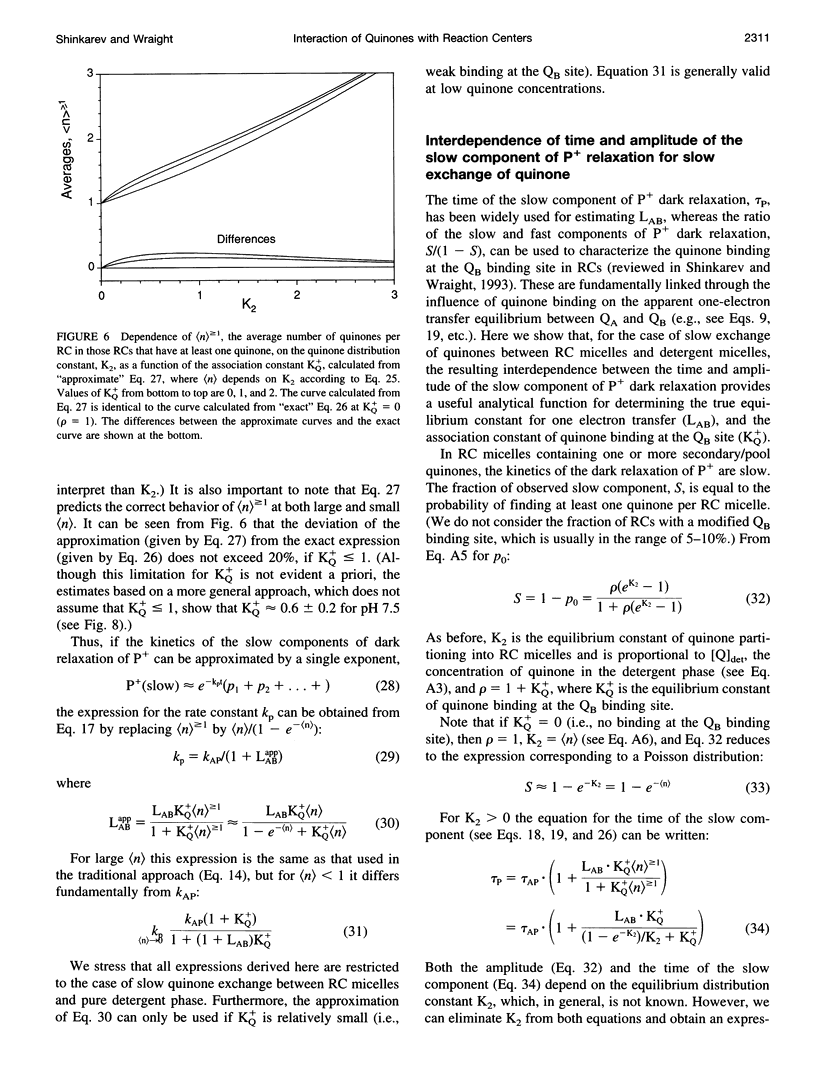
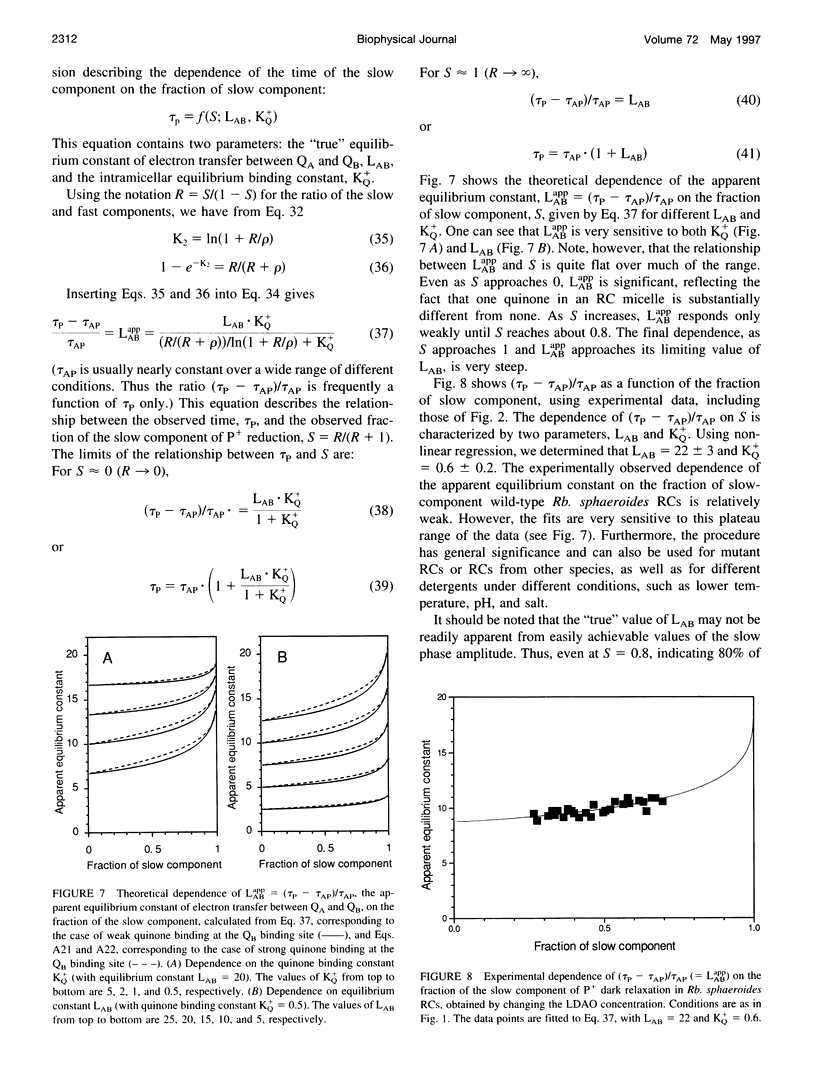
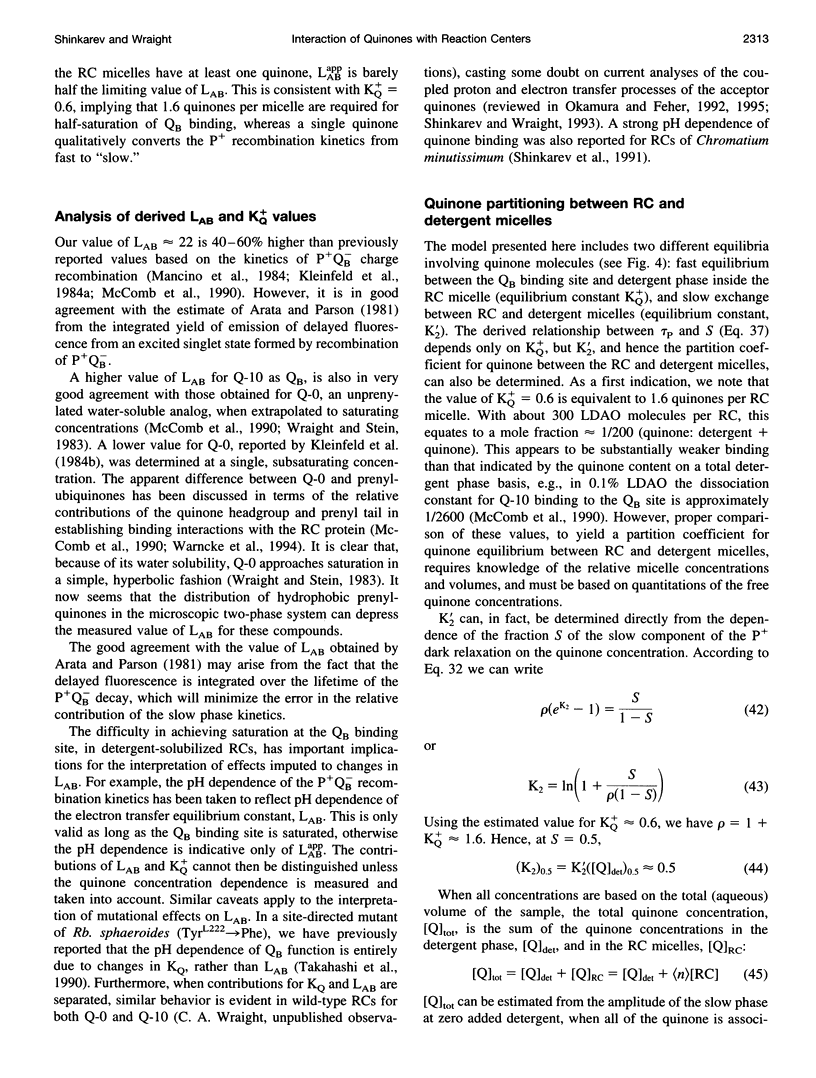
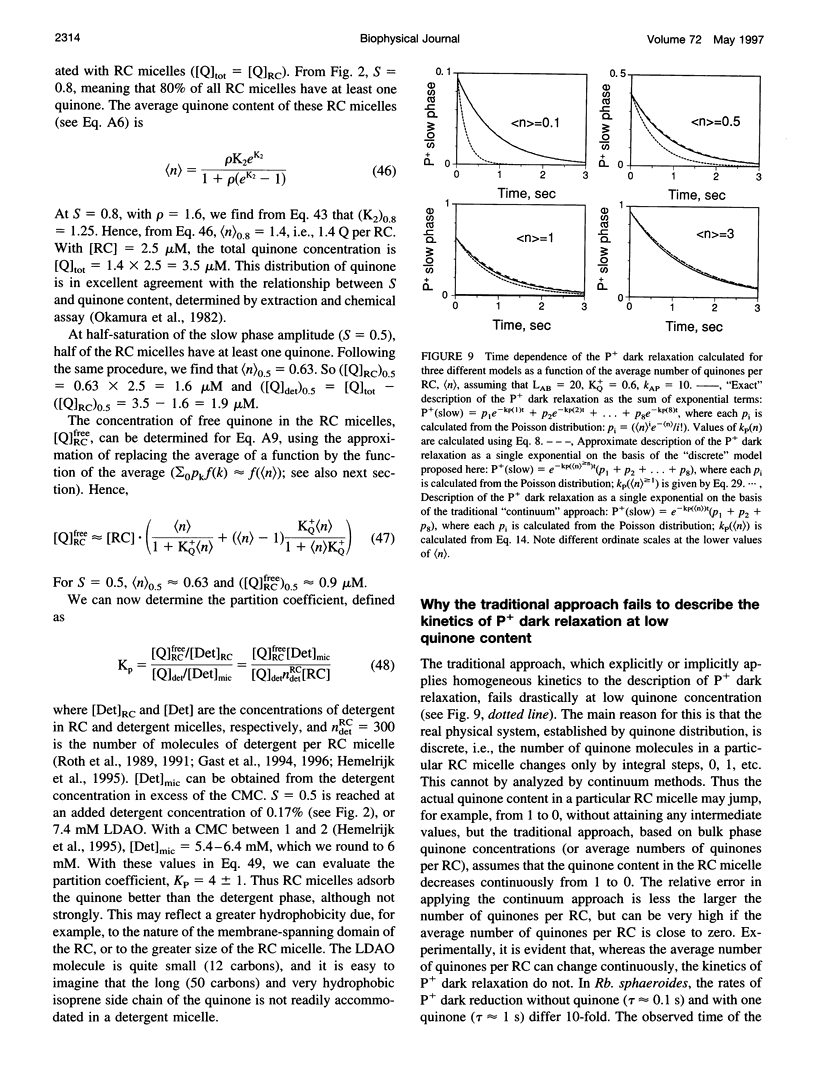
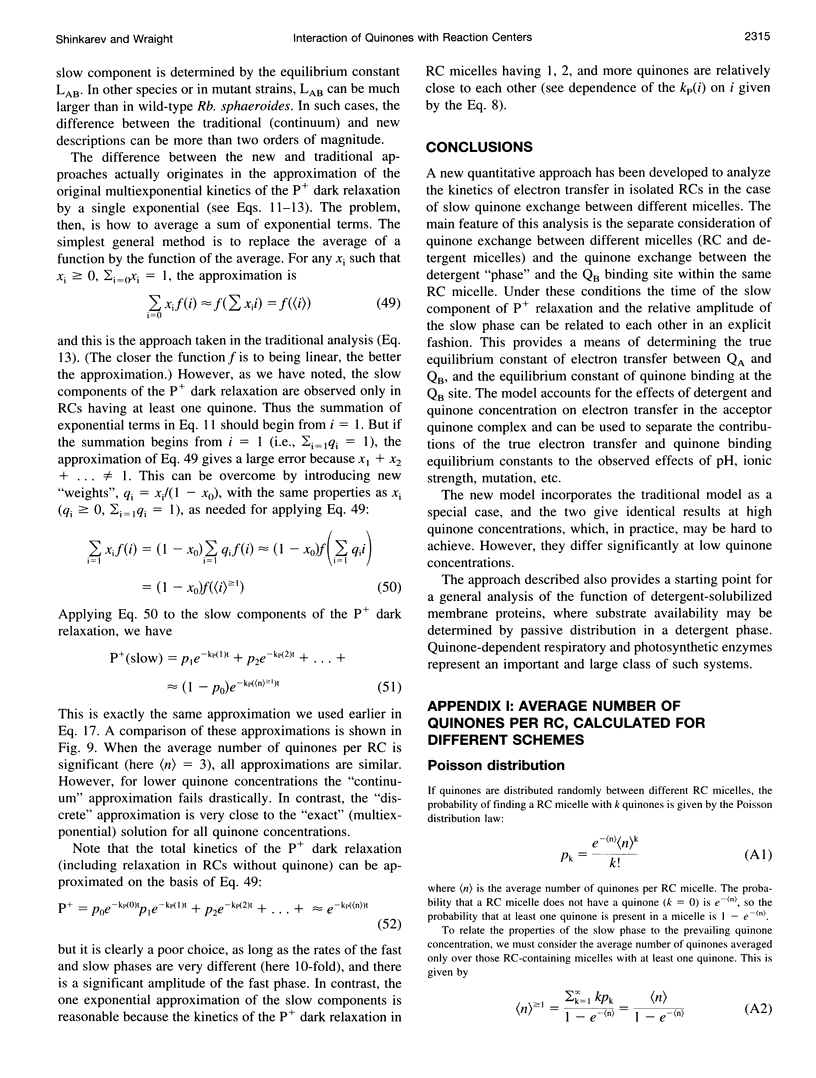
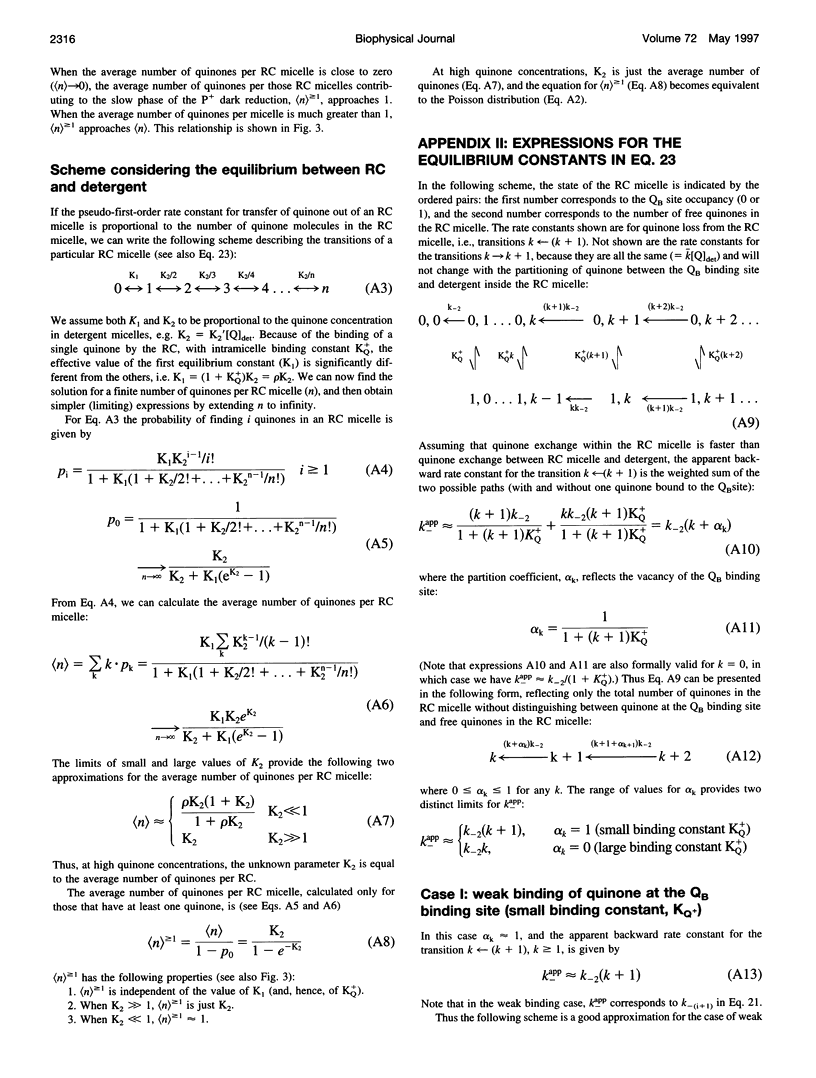
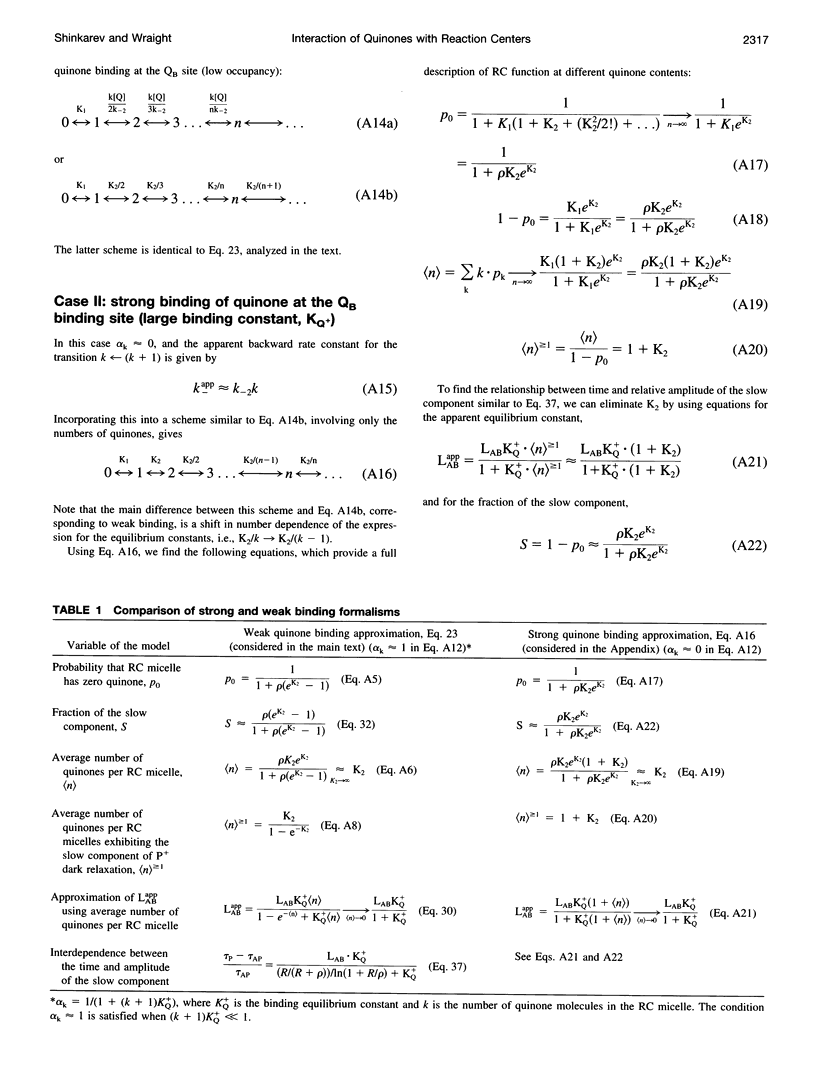
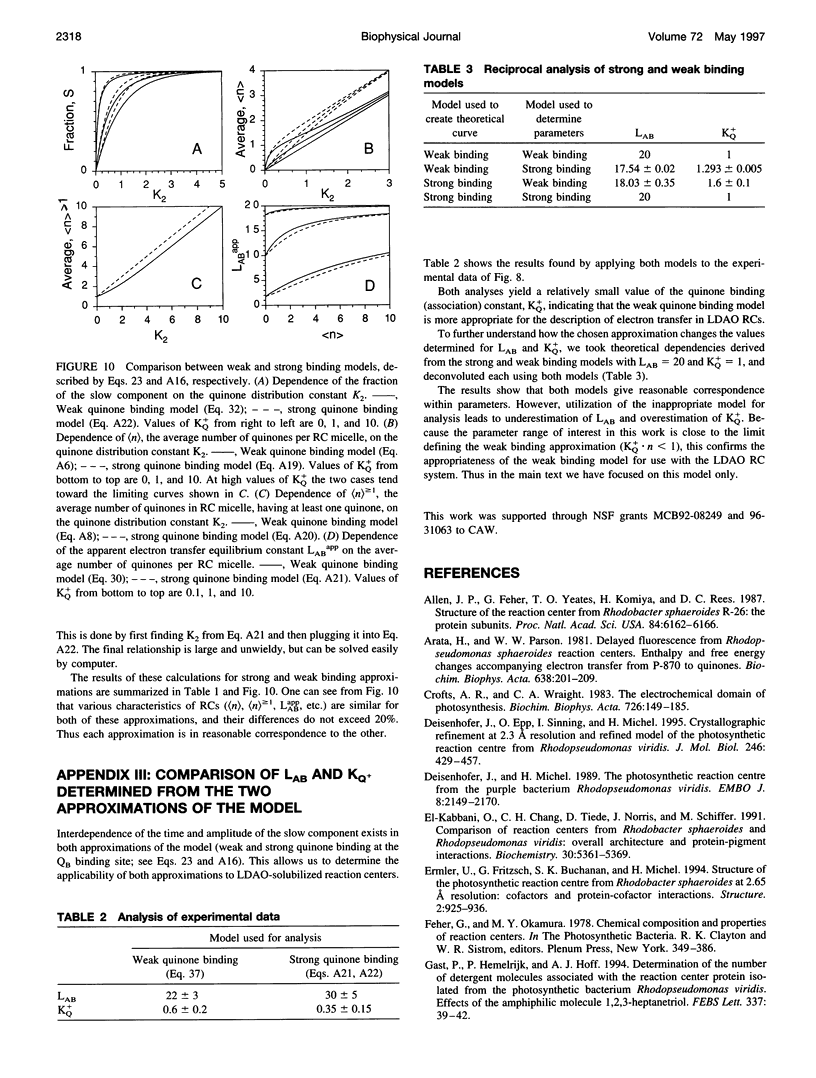
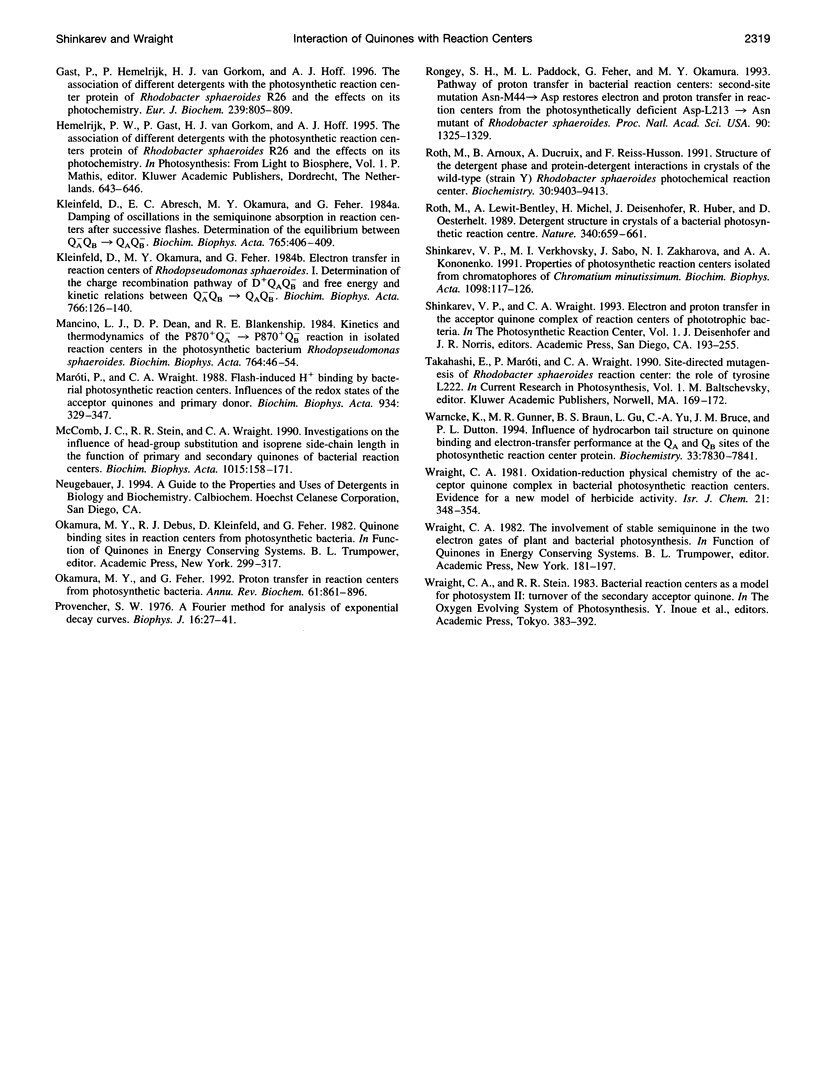
Images in this article
Selected References
These references are in PubMed. This may not be the complete list of references from this article.
- Allen J. P., Feher G., Yeates T. O., Komiya H., Rees D. C. Structure of the reaction center from Rhodobacter sphaeroides R-26: the protein subunits. Proc Natl Acad Sci U S A. 1987 Sep;84(17):6162–6166. doi: 10.1073/pnas.84.17.6162. [DOI] [PMC free article] [PubMed] [Google Scholar]
- Deisenhofer J., Epp O., Sinning I., Michel H. Crystallographic refinement at 2.3 A resolution and refined model of the photosynthetic reaction centre from Rhodopseudomonas viridis. J Mol Biol. 1995 Feb 24;246(3):429–457. doi: 10.1006/jmbi.1994.0097. [DOI] [PubMed] [Google Scholar]
- Deisenhofer J., Michel H. Nobel lecture. The photosynthetic reaction centre from the purple bacterium Rhodopseudomonas viridis. EMBO J. 1989 Aug;8(8):2149–2170. doi: 10.1002/j.1460-2075.1989.tb08338.x. [DOI] [PMC free article] [PubMed] [Google Scholar]
- Ermler U., Fritzsch G., Buchanan S. K., Michel H. Structure of the photosynthetic reaction centre from Rhodobacter sphaeroides at 2.65 A resolution: cofactors and protein-cofactor interactions. Structure. 1994 Oct 15;2(10):925–936. doi: 10.1016/s0969-2126(94)00094-8. [DOI] [PubMed] [Google Scholar]
- Gast P., Hemelrijk P. W., Van Gorkom H. J., Hoff A. J. The association of different detergents with the photosynthetic reaction center protein of Rhodobacter sphaeroides R26 and the effects on its photochemistry. Eur J Biochem. 1996 Aug 1;239(3):805–809. doi: 10.1111/j.1432-1033.1996.0805u.x. [DOI] [PubMed] [Google Scholar]
- Gast P., Hemelrijk P., Hoff A. J. Determination of the number of detergent molecules associated with the reaction center protein isolated from the photosynthetic bacterium Rhodopseudomonas viridis. Effects of the amphiphilic molecule 1,2,3-heptanetriol. FEBS Lett. 1994 Jan 3;337(1):39–42. doi: 10.1016/0014-5793(94)80625-x. [DOI] [PubMed] [Google Scholar]
- Kleinfeld D., Okamura M. Y., Feher G. Electron transfer in reaction centers of Rhodopseudomonas sphaeroides. I. Determination of the charge recombination pathway of D+QAQ(-)B and free energy and kinetic relations between Q(-)AQB and QAQ(-)B. Biochim Biophys Acta. 1984 Jul 27;766(1):126–140. doi: 10.1016/0005-2728(84)90224-x. [DOI] [PubMed] [Google Scholar]
- McComb J. C., Stein R. R., Wraight C. A. Investigations on the influence of headgroup substitution and isoprene side-chain length in the function of primary and secondary quinones of bacterial reaction centers. Biochim Biophys Acta. 1990 Jan 4;1015(1):156–171. doi: 10.1016/0005-2728(90)90227-u. [DOI] [PubMed] [Google Scholar]
- Okamura M. Y., Feher G. Proton transfer in reaction centers from photosynthetic bacteria. Annu Rev Biochem. 1992;61:861–896. doi: 10.1146/annurev.bi.61.070192.004241. [DOI] [PubMed] [Google Scholar]
- Provencher S. W. A Fourier method for the analysis of exponential decay curves. Biophys J. 1976 Jan;16(1):27–41. doi: 10.1016/S0006-3495(76)85660-3. [DOI] [PMC free article] [PubMed] [Google Scholar]
- Rongey S. H., Paddock M. L., Feher G., Okamura M. Y. Pathway of proton transfer in bacterial reaction centers: second-site mutation Asn-M44-->Asp restores electron and proton transfer in reaction centers from the photosynthetically deficient Asp-L213-->Asn mutant of Rhodobacter sphaeroides. Proc Natl Acad Sci U S A. 1993 Feb 15;90(4):1325–1329. doi: 10.1073/pnas.90.4.1325. [DOI] [PMC free article] [PubMed] [Google Scholar]
- Roth M., Arnoux B., Ducruix A., Reiss-Husson F. Structure of the detergent phase and protein-detergent interactions in crystals of the wild-type (strain Y) Rhodobacter sphaeroides photochemical reaction center. Biochemistry. 1991 Oct 1;30(39):9403–9413. doi: 10.1021/bi00103a003. [DOI] [PubMed] [Google Scholar]
- Warncke K., Gunner M. R., Braun B. S., Gu L., Yu C. A., Bruce J. M., Dutton P. L. Influence of hydrocarbon tail structure on quinone binding and electron-transfer performance at the QA and QB sites of the photosynthetic reaction center protein. Biochemistry. 1994 Jun 28;33(25):7830–7841. doi: 10.1021/bi00191a010. [DOI] [PubMed] [Google Scholar]
- el-Kabbani O., Chang C. H., Tiede D., Norris J., Schiffer M. Comparison of reaction centers from Rhodobacter sphaeroides and Rhodopseudomonas viridis: overall architecture and protein-pigment interactions. Biochemistry. 1991 Jun 4;30(22):5361–5369. doi: 10.1021/bi00236a006. [DOI] [PubMed] [Google Scholar]