Abstract
Physiological mole fractions of long isoprenic chain ubiquinone (UQ[10]) and plastoquinone (PQ9) were incorporated inside a supported bilayer by vesicle fusion. The template of the bilayer was an especially designed microporous electrode that allows the direct electrochemistry of water insoluble molecules in a water environment. The artificial structure, made by self-assembly procedures, consisted of a bilayer laterally in contact with a built-in gold electrode at which direct electron transfers between the redox heads of the quinones molecules and the electrode can proceed. The mass balances of quinone and lipid in the structure were determined by radiolabeling and spectrophotometry. A dimyristoyl phosphatdylcholine stable surface concentration of 250 +/- 50 pmol x cm(-2), unaffected by the presence of the quinone, was measured in the fluid monolayer. The mole fraction of quinone was between 1 and 3 mol%, remaining unchanged when going from the vesicles to the supported layers. The lipid molecules and the quinone pool were both laterally mobile, and cyclic voltammetry was used to investigate the redox properties of UQ10 and PQ9 over a wide pH range. Below pH 12, the two electrons-two protons electrochemical process at the gold electrode appeared under kinetic control. Thus all thermodynamic deductions must be anchored in the observed reversibility of the quinone/hydroquinol anion transformation at pH > 13. Within the experimental uncertainty, the standard potentials and the pK(a)'s of the pertinent redox forms of UQ10 and PQ9 were found to be essentially identical. This differs slightly from the literature in which the constants were deduced from the studies of model quinones in mixed solvents or of isoprenic quinones without a lipidic environment.
Full text
PDF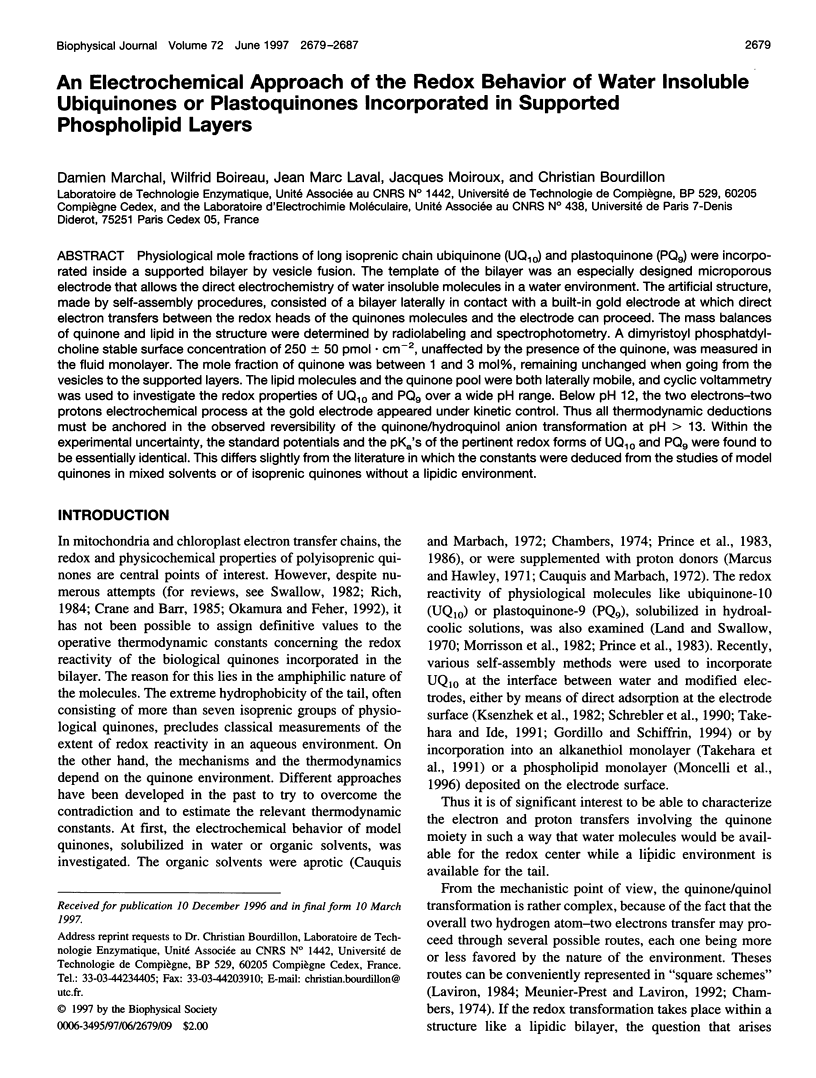
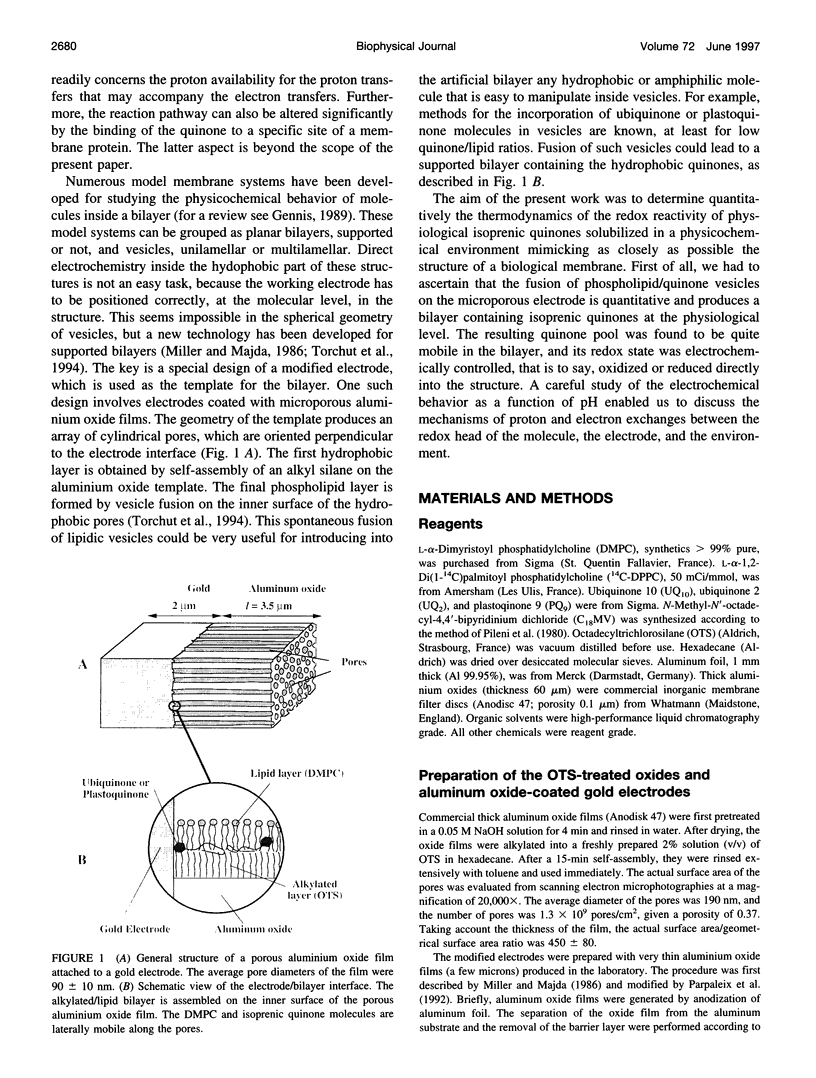
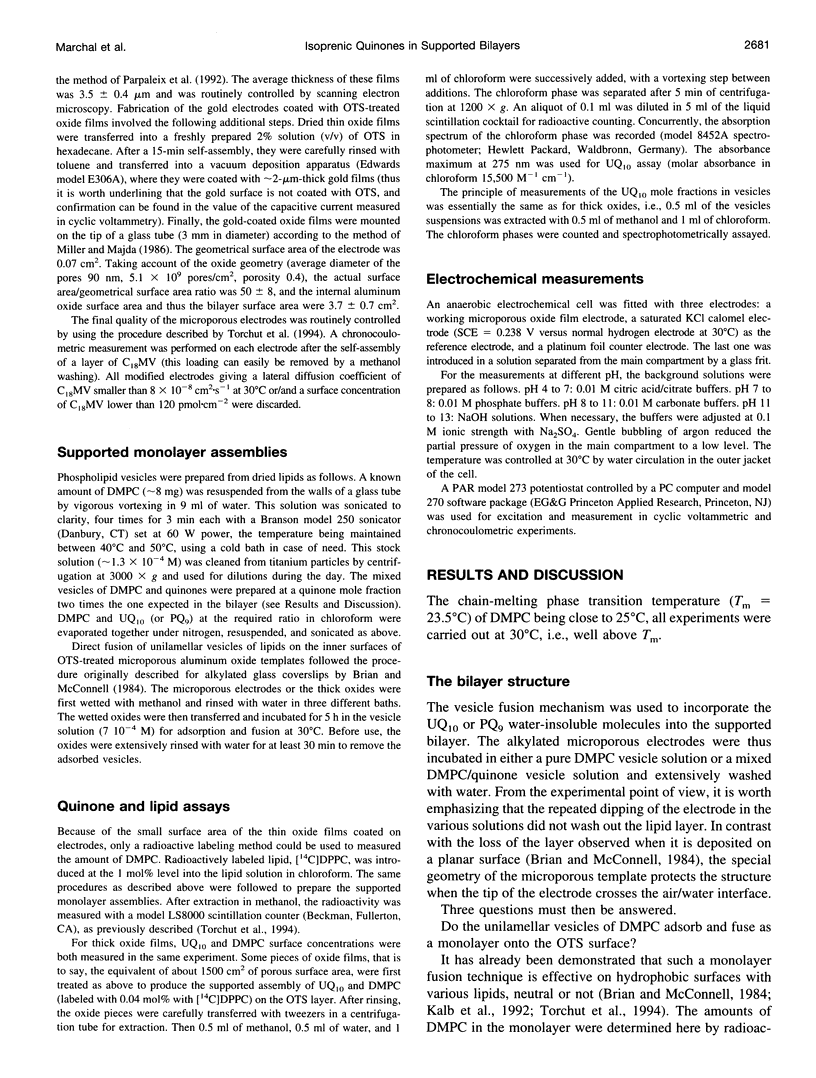
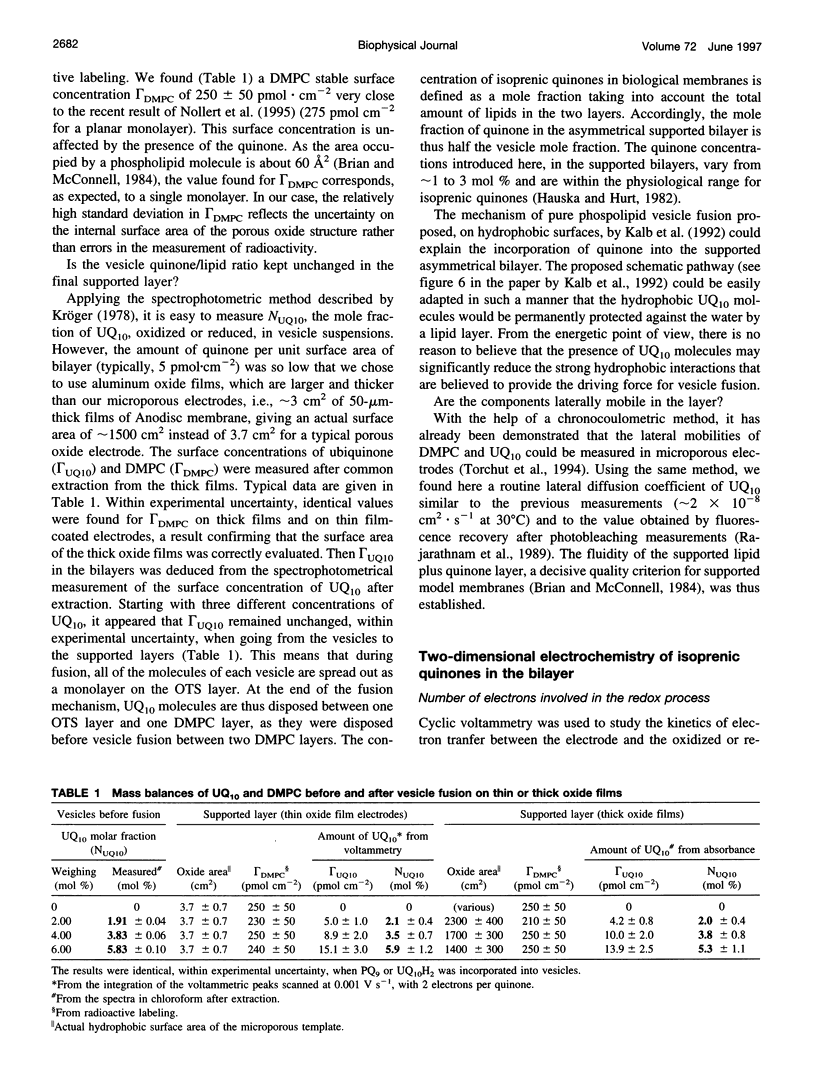
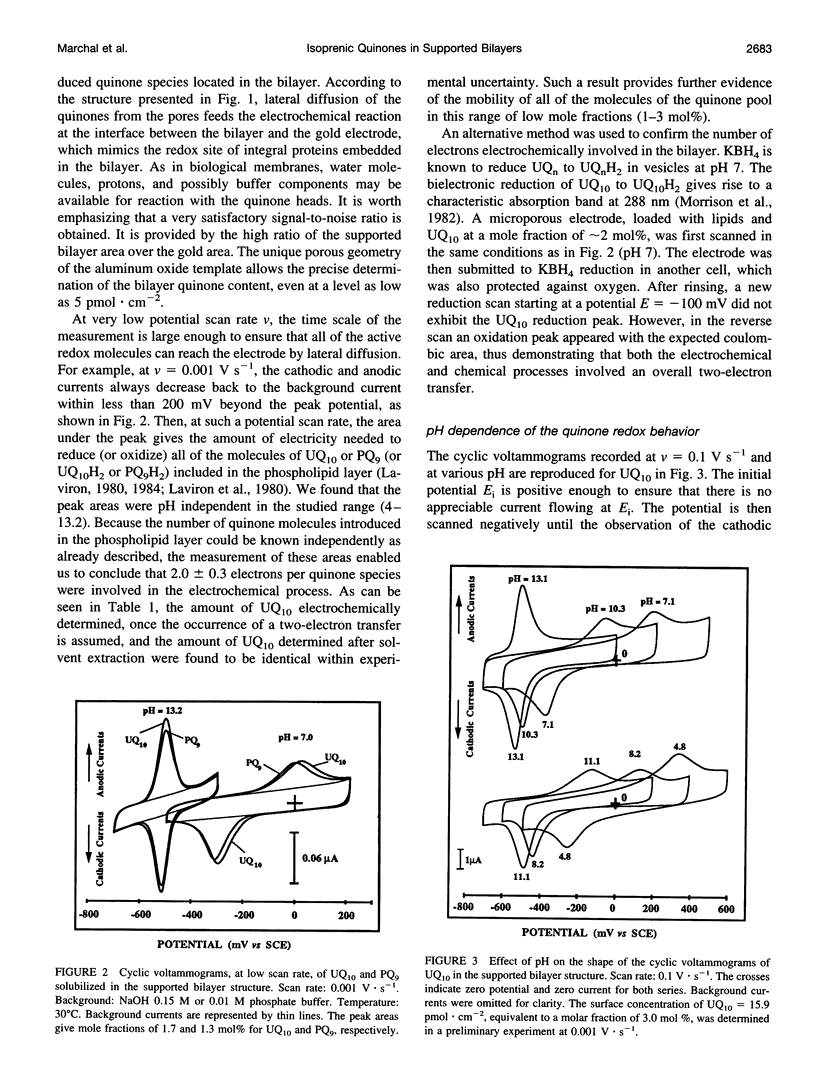
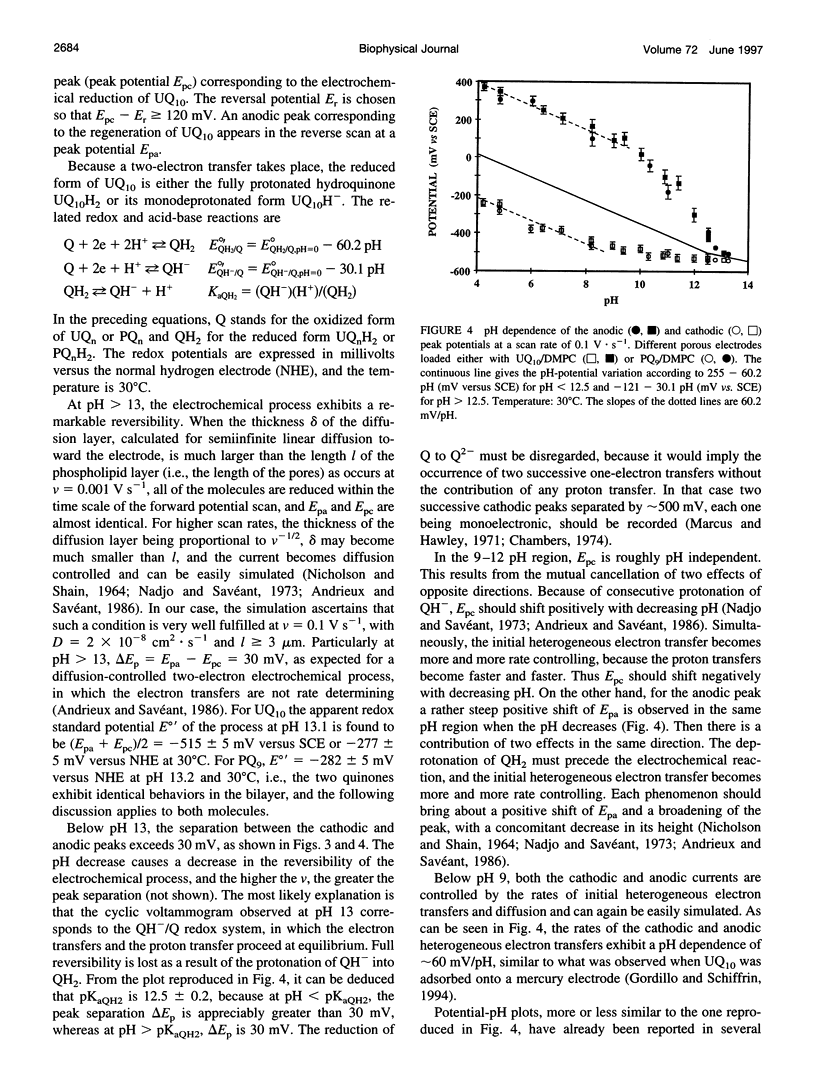
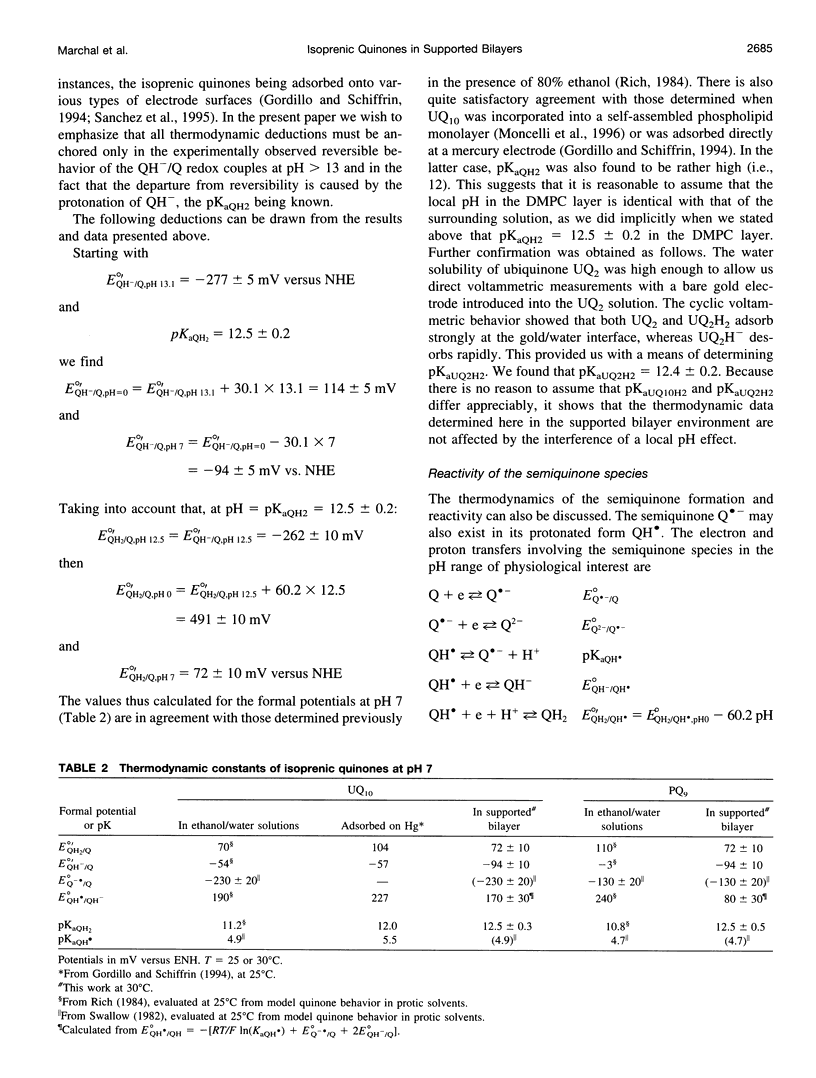
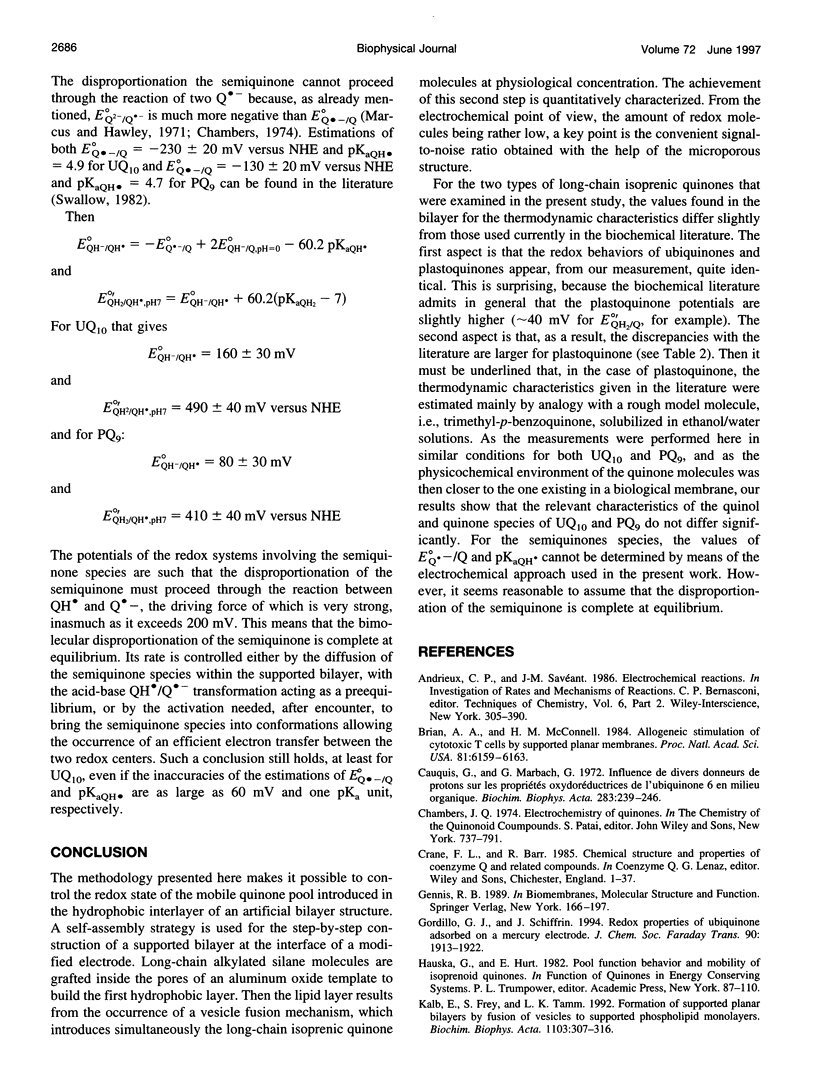
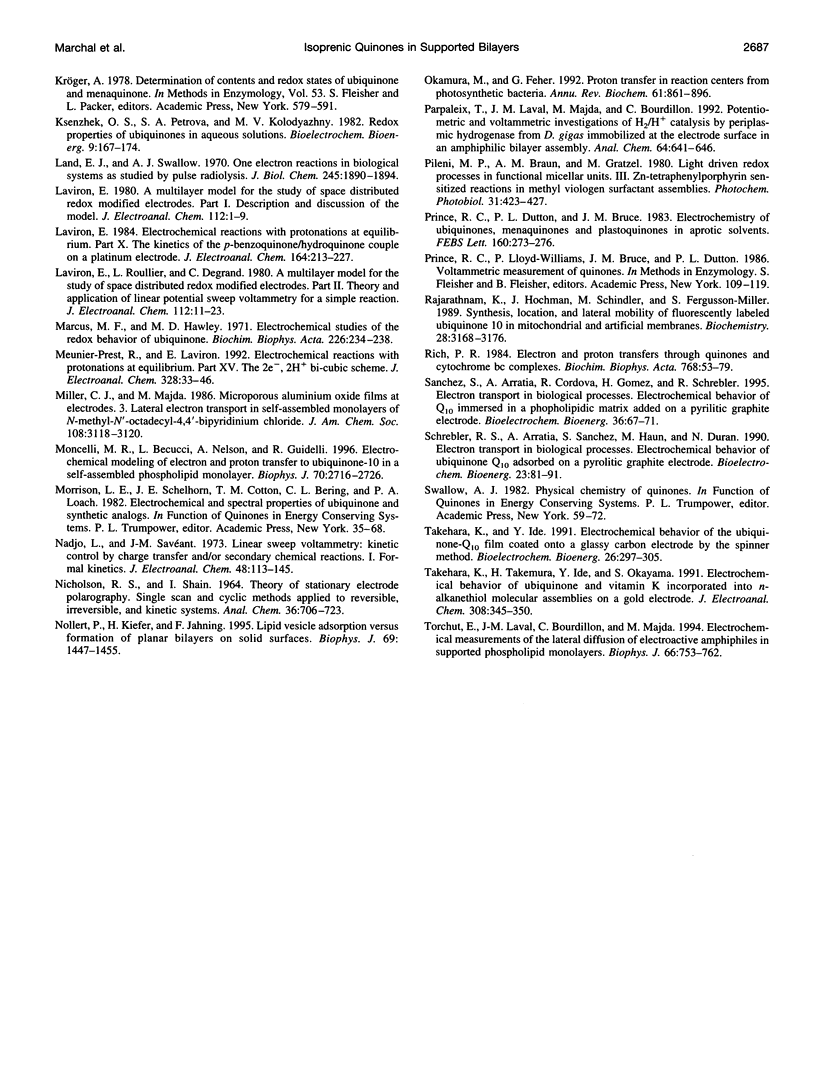
Images in this article
Selected References
These references are in PubMed. This may not be the complete list of references from this article.
- Brian A. A., McConnell H. M. Allogeneic stimulation of cytotoxic T cells by supported planar membranes. Proc Natl Acad Sci U S A. 1984 Oct;81(19):6159–6163. doi: 10.1073/pnas.81.19.6159. [DOI] [PMC free article] [PubMed] [Google Scholar]
- Cauquis G., Marbach G. Influence de divers donneurs de protons sur les propriétés oxydoréductrices de l'ubiquinone 6 en milieu organique. Biochim Biophys Acta. 1972 Nov 17;283(2):239–246. doi: 10.1016/0005-2728(72)90240-x. [DOI] [PubMed] [Google Scholar]
- Kalb E., Frey S., Tamm L. K. Formation of supported planar bilayers by fusion of vesicles to supported phospholipid monolayers. Biochim Biophys Acta. 1992 Jan 31;1103(2):307–316. doi: 10.1016/0005-2736(92)90101-q. [DOI] [PubMed] [Google Scholar]
- Kröger A. Determination of contents and redox states of ubiquinone and menaquinone. Methods Enzymol. 1978;53:579–591. doi: 10.1016/s0076-6879(78)53059-0. [DOI] [PubMed] [Google Scholar]
- Land E. J., Swallow A. J. One-electron reactions in biochemical systems as studied by pulse radiolysis. 3. Ubiquinone. J Biol Chem. 1970 Apr 25;245(8):1890–1894. [PubMed] [Google Scholar]
- Marcus M. F., Hawley M. D. Electrochemical studies of the redox behavior of ubiquinone-I. Biochim Biophys Acta. 1971 Mar 2;226(2):234–238. doi: 10.1016/0005-2728(71)90090-9. [DOI] [PubMed] [Google Scholar]
- Moncelli M. R., Becucci L., Nelson A., Guidelli R. Electrochemical modeling of electron and proton transfer to ubiquinone-10 in a self-assembled phospholipid monolayer. Biophys J. 1996 Jun;70(6):2716–2726. doi: 10.1016/S0006-3495(96)79841-7. [DOI] [PMC free article] [PubMed] [Google Scholar]
- Nollert P., Kiefer H., Jähnig F. Lipid vesicle adsorption versus formation of planar bilayers on solid surfaces. Biophys J. 1995 Oct;69(4):1447–1455. doi: 10.1016/S0006-3495(95)80014-7. [DOI] [PMC free article] [PubMed] [Google Scholar]
- Okamura M. Y., Feher G. Proton transfer in reaction centers from photosynthetic bacteria. Annu Rev Biochem. 1992;61:861–896. doi: 10.1146/annurev.bi.61.070192.004241. [DOI] [PubMed] [Google Scholar]
- Parpaleix T., Laval J. M., Majda M., Bourdillon C. Potentiometric and voltammetric investigations of H2/H+ catalysis by periplasmic hydrogenase from Desulfovibrio gigas immobilized at the electrode surface in an amphiphilic bilayer assembly. Anal Chem. 1992 Mar 15;64(6):641–646. doi: 10.1021/ac00030a013. [DOI] [PubMed] [Google Scholar]
- Prince R. C., Lloyd-Williams P., Bruce J. M., Dutton P. L. Voltammetric measurements of quinones. Methods Enzymol. 1986;125:109–119. doi: 10.1016/s0076-6879(86)25010-7. [DOI] [PubMed] [Google Scholar]
- Rajarathnam K., Hochman J., Schindler M., Ferguson-Miller S. Synthesis, location, and lateral mobility of fluorescently labeled ubiquinone 10 in mitochondrial and artificial membranes. Biochemistry. 1989 Apr 18;28(8):3168–3176. doi: 10.1021/bi00434a009. [DOI] [PubMed] [Google Scholar]
- Rich P. R. Electron and proton transfers through quinones and cytochrome bc complexes. Biochim Biophys Acta. 1984 Apr 9;768(1):53–79. doi: 10.1016/0304-4173(84)90007-7. [DOI] [PubMed] [Google Scholar]
- Torchut E., Laval J. M., Bourdillon C., Majda M. Electrochemical measurements of the lateral diffusion of electroactive amphiphiles in supported phospholipid monolayers. Biophys J. 1994 Mar;66(3 Pt 1):753–762. doi: 10.1016/s0006-3495(94)80851-3. [DOI] [PMC free article] [PubMed] [Google Scholar]