Abstract
1. Liver microsomes form lipid peroxide when incubated with ascorbate or NADPH, but not with NADH. Increasing the concentration of ascorbate beyond the optimum (0·5mm) decreases the rate of lipid peroxide formation, but this effect does not occur with NADPH. Other reducing agents such as p-phenylenediamine or ferricyanide were not able to replace ascorbate and induce lipid peroxide formation. 2. The rate of ascorbate-induced peroxidation is optimum at pH6·0 whereas the rate of the NADPH system is optimum at pH7·0. Both systems require phosphate for maximum activity. 3. Lipid peroxide formation occurs at the maximum specific rate in very dilute microsome suspensions (0·15mg. of protein/ml.). 4. Treatment of microsomes with deoxycholate and other detergents causes membrane disintegration and inhibits lipid peroxide formation. 5. Lipid peroxide formation is accompanied by a rapid uptake of oxygen and there is a large excess of oxygen utilized for each molecule of malonaldehyde measured in the peroxide method. 6. Boiled microsomes form lipid peroxide in the presence of ascorbate, but not if NADPH is added. 7. Lipid peroxide formation induced by NADPH is strongly inhibited by p-chloromercuribenzoate, weakly inhibited by N-ethylmaleimide and unaffected by iodoacetamide. Ascorbate-induced peroxidation in untreated microsomes is unaffected by p-chloromercuribenzoate, but inhibited if boiled microsomes are used. These experiments may be interpreted on the basis that a ferredoxin-type protein forms part of the system in which NADPH induces lipid peroxide formation. 8. Most heavy-metal ions, with the exception of inorganic iron (Fe2+ or Fe3+), which activates, inhibit both ascorbate-induced and NADPH-induced peroxidation. Mg2+ increases the rate of peroxidation whereas Ca2+ inhibits it. 9. Lipid peroxide formation is inhibited strongly by GSH and weakly by cysteine. Ascorbate-induced peroxidation is much more sensitive than NADPH-induced peroxidation. 10. Peroxidation is strongly inhibited by addition of low concentrations (0·01–0·1mm) of cytochrome c or of haemoglobin. 11. It is considered that lipid peroxide formation occurs as a result of the operation of the microsomal electron-transport chain switching from hydroxylation to oxidize unsaturated lipids of the endoplasmic reticulum.
Full text
PDF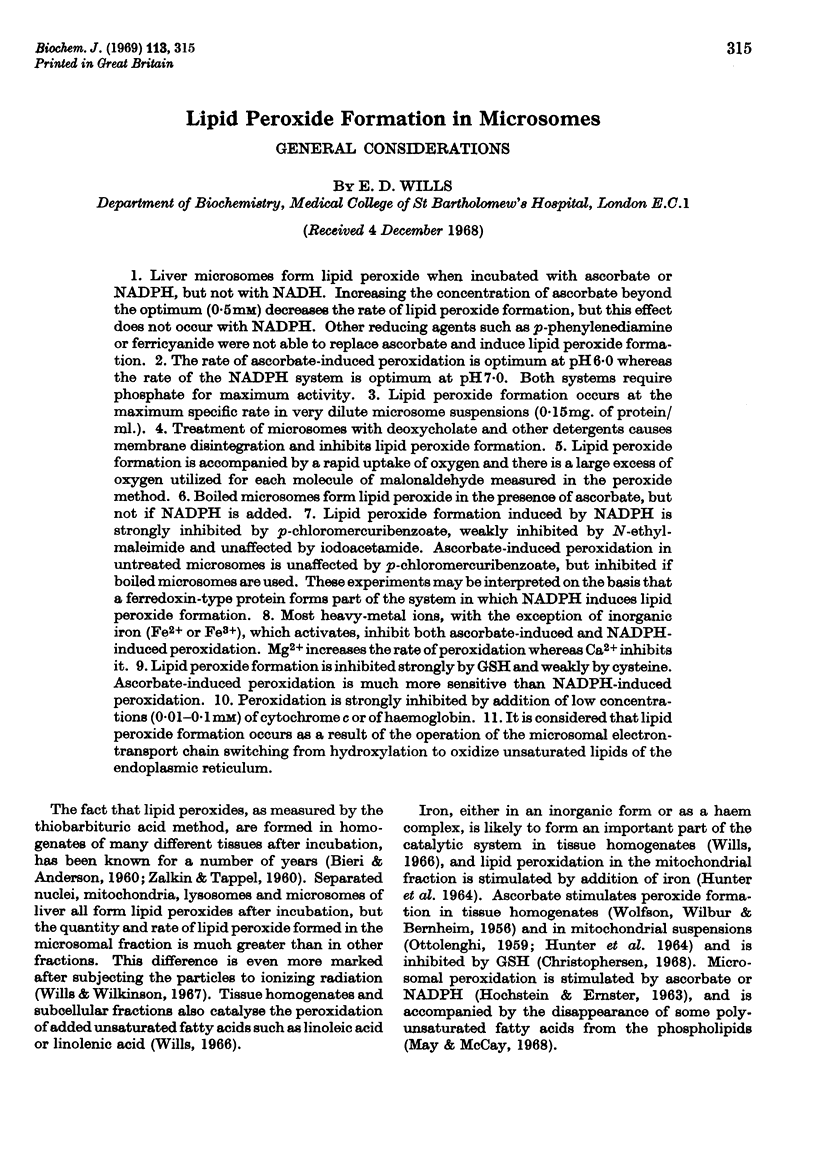
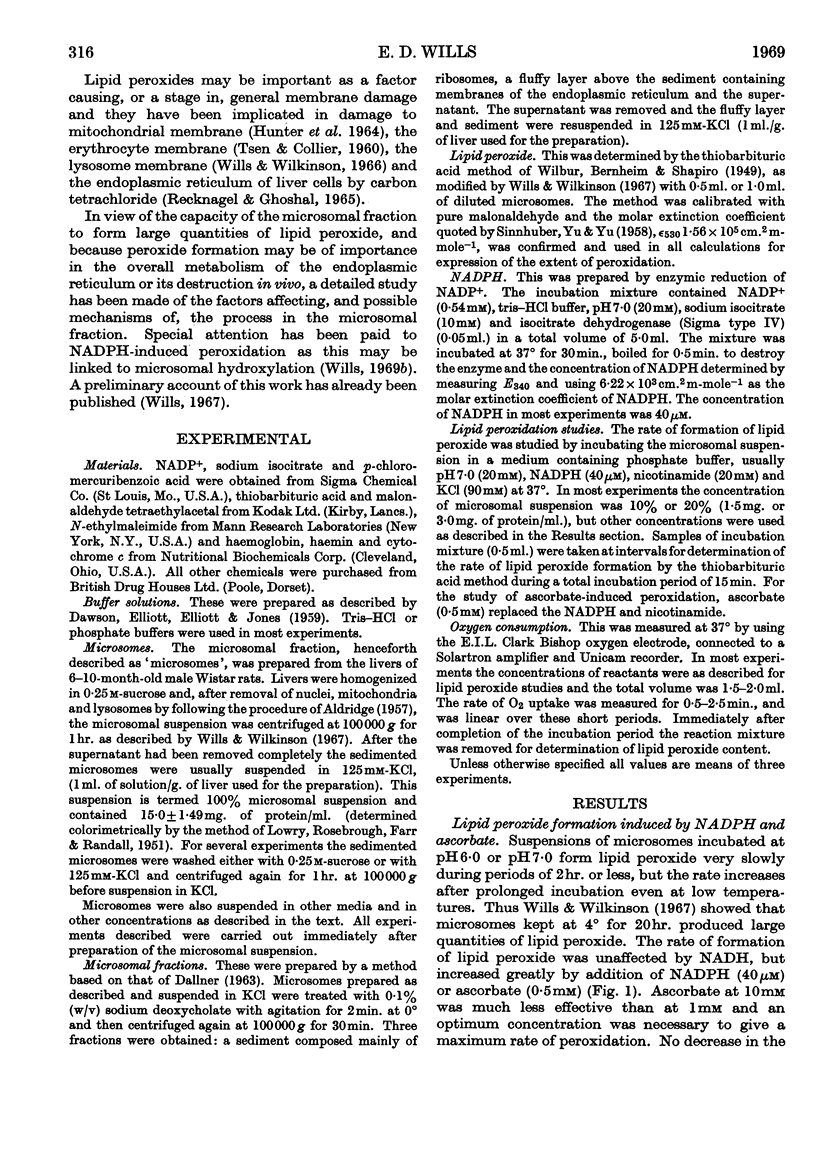
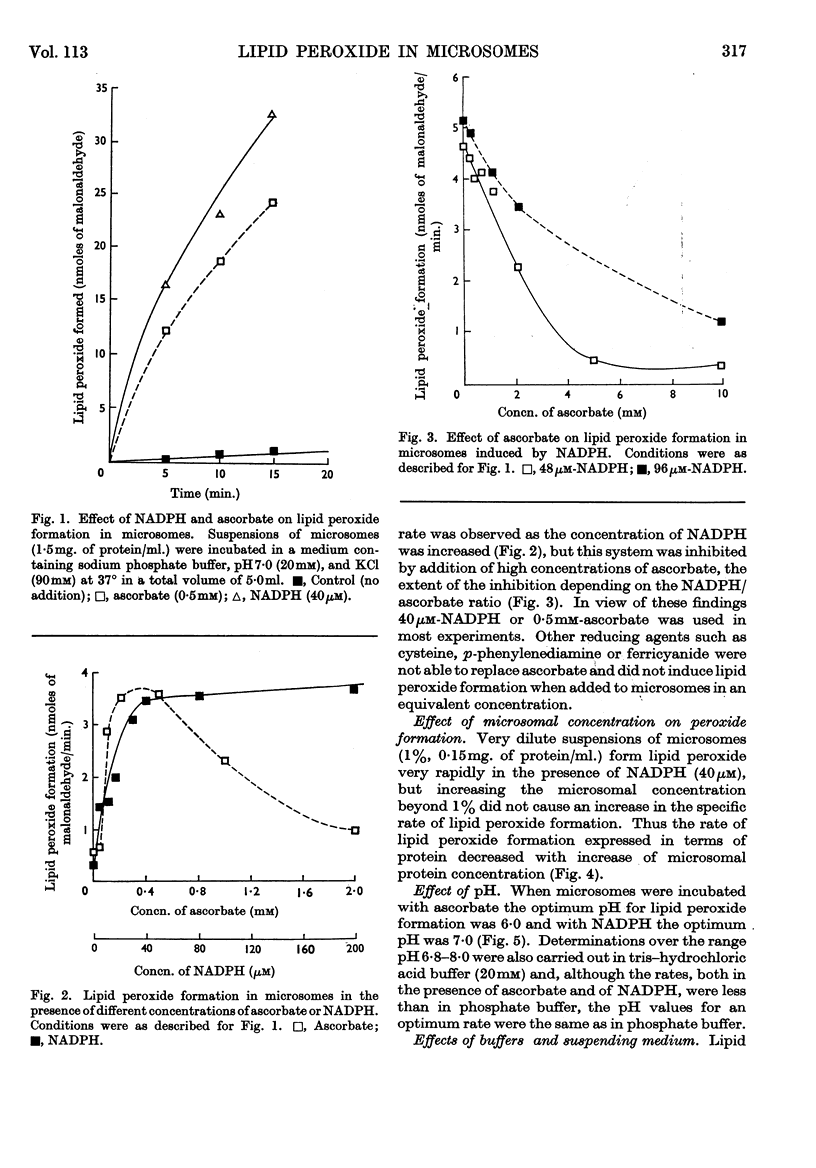
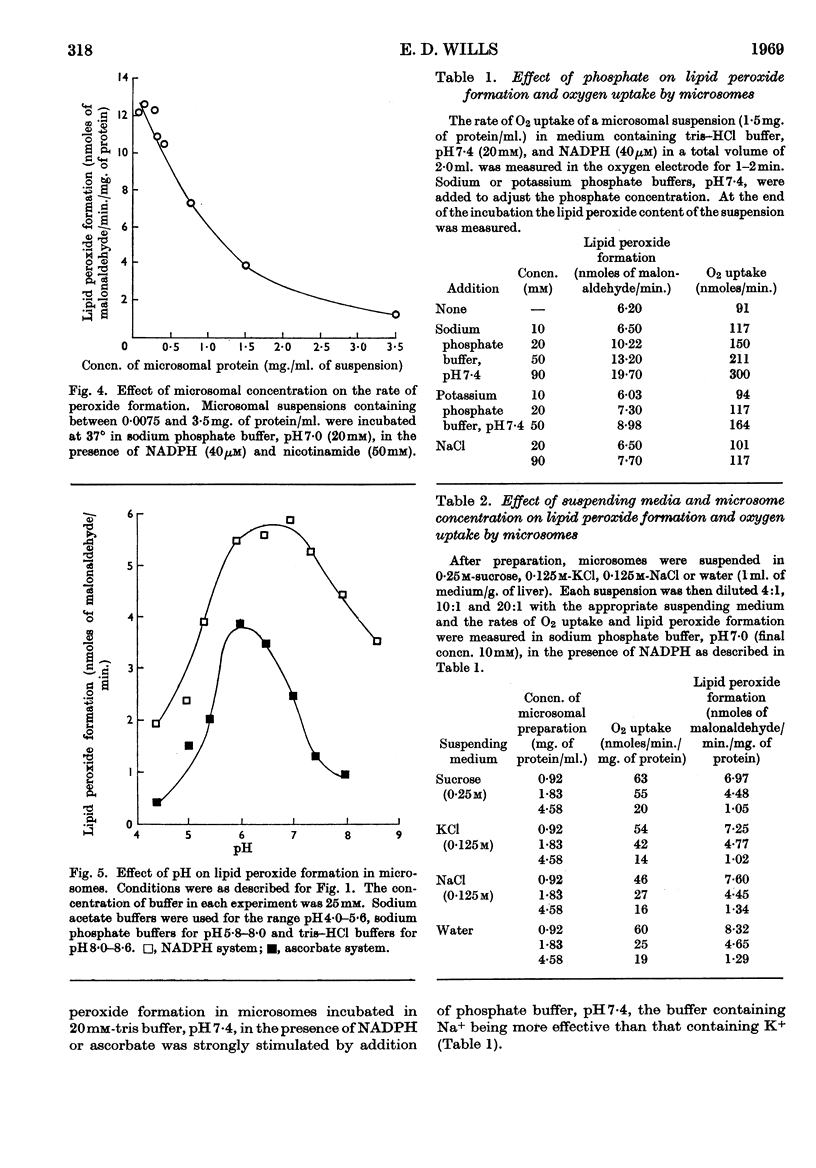
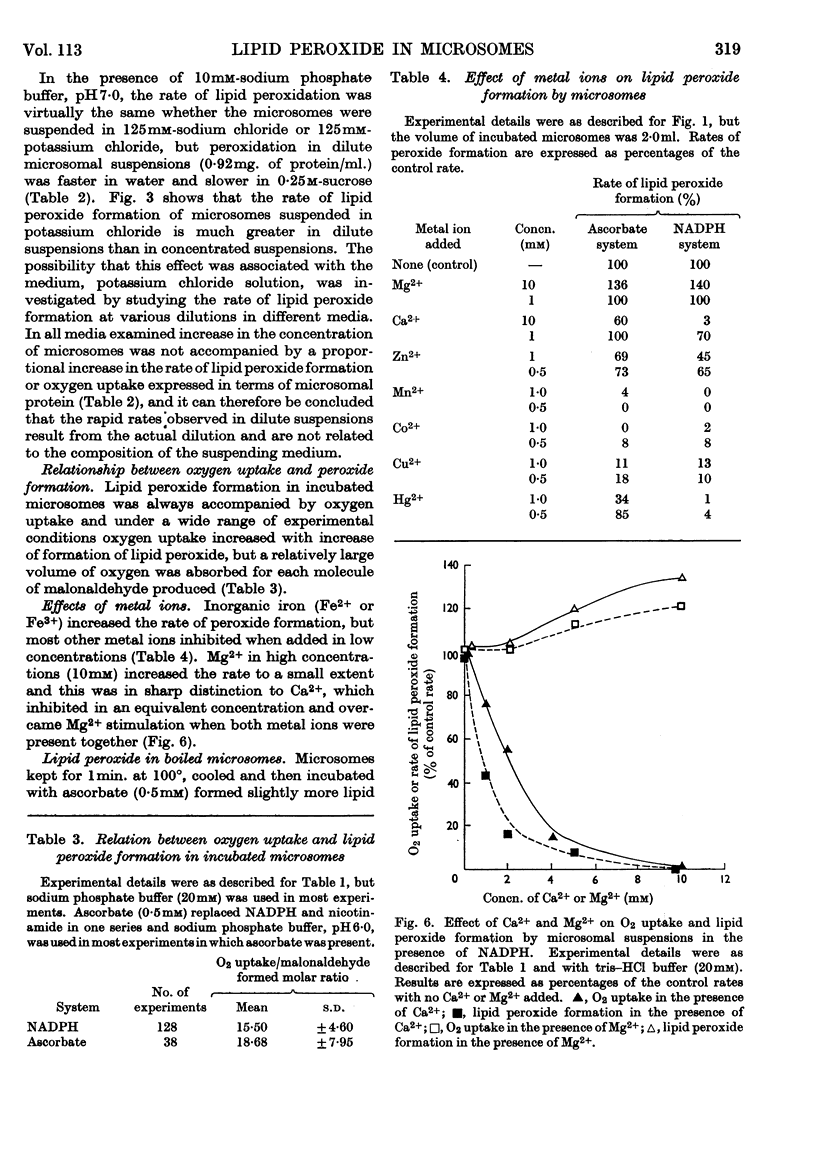
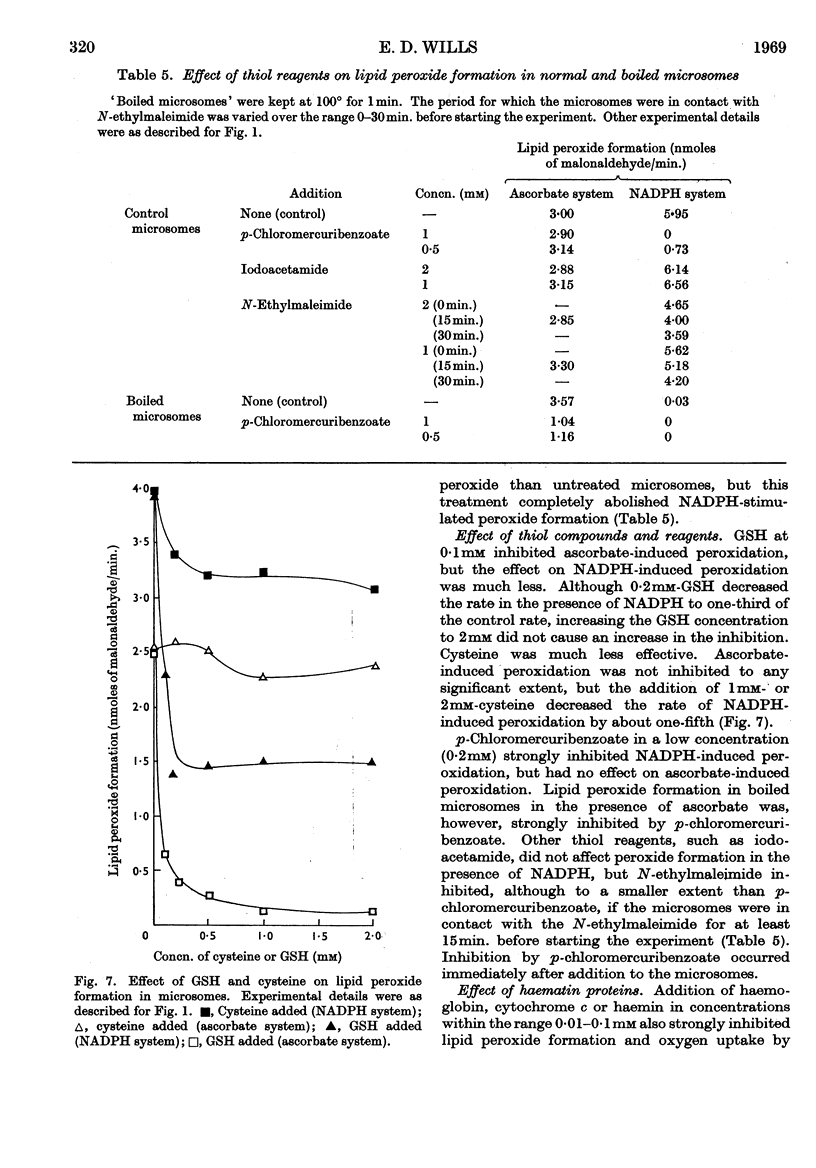
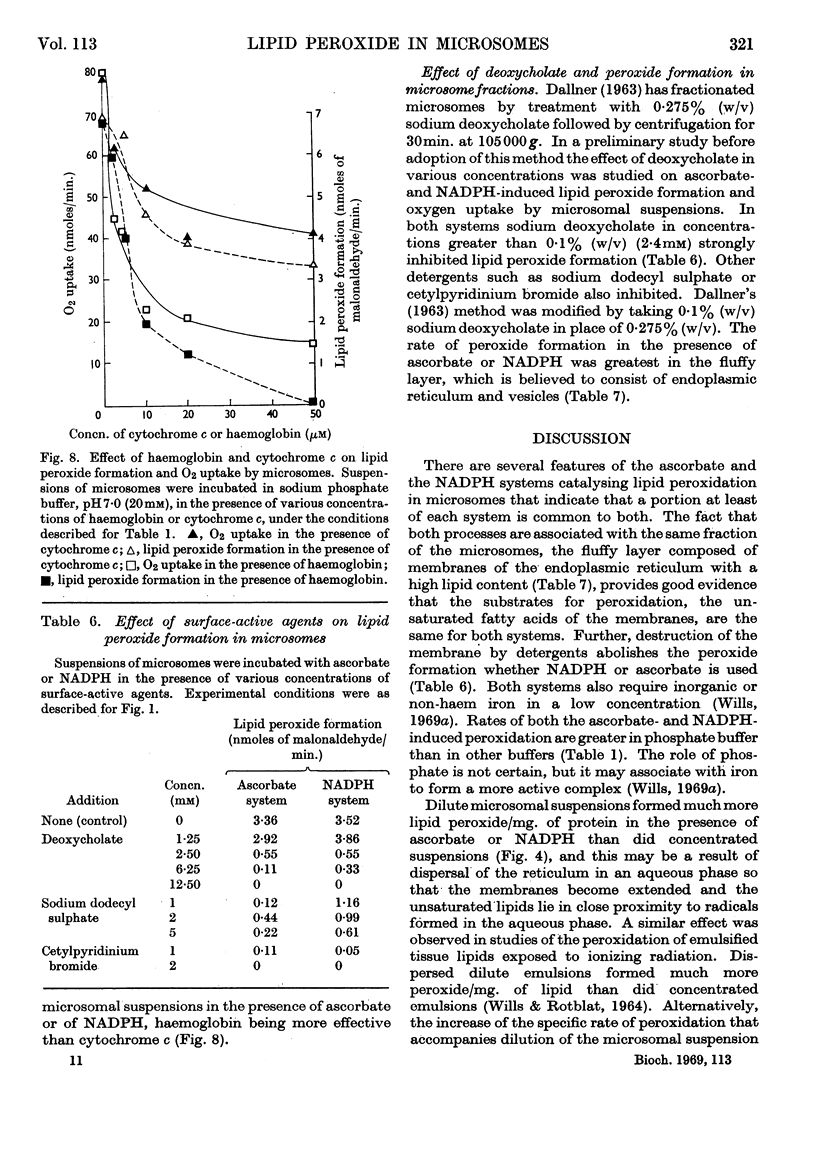
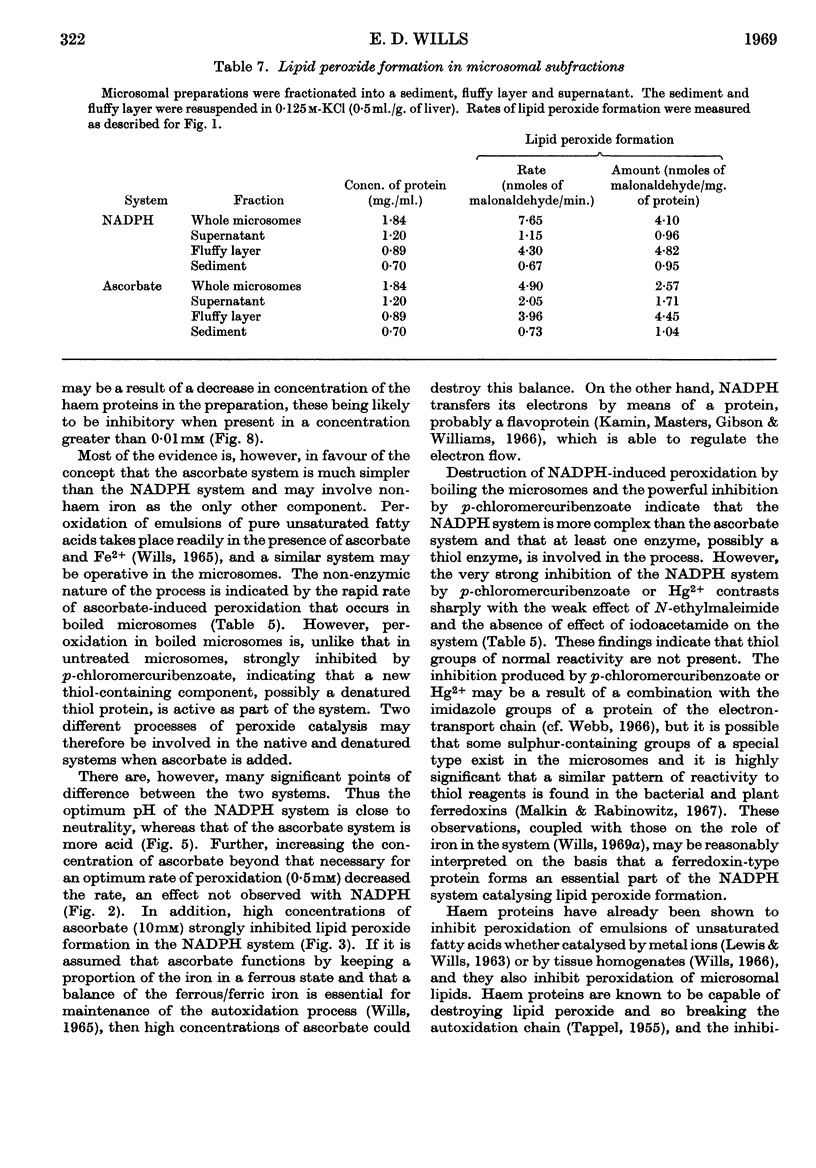
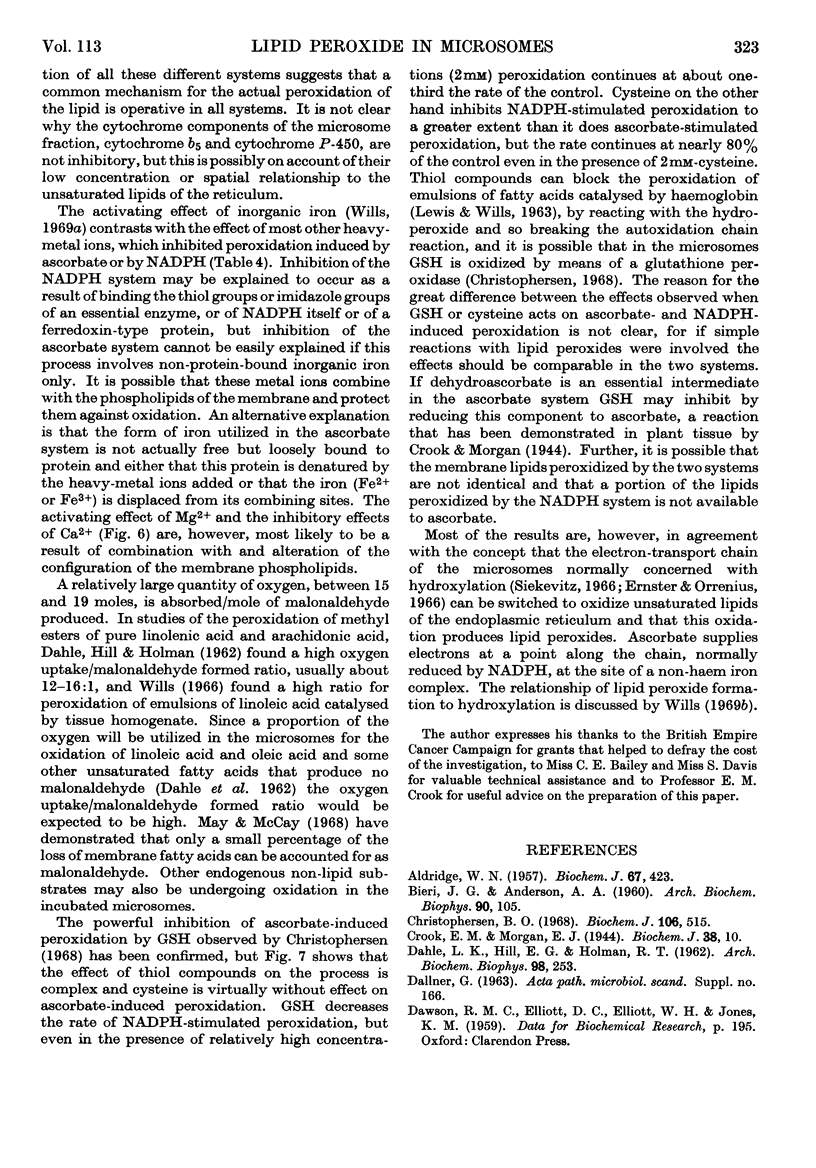
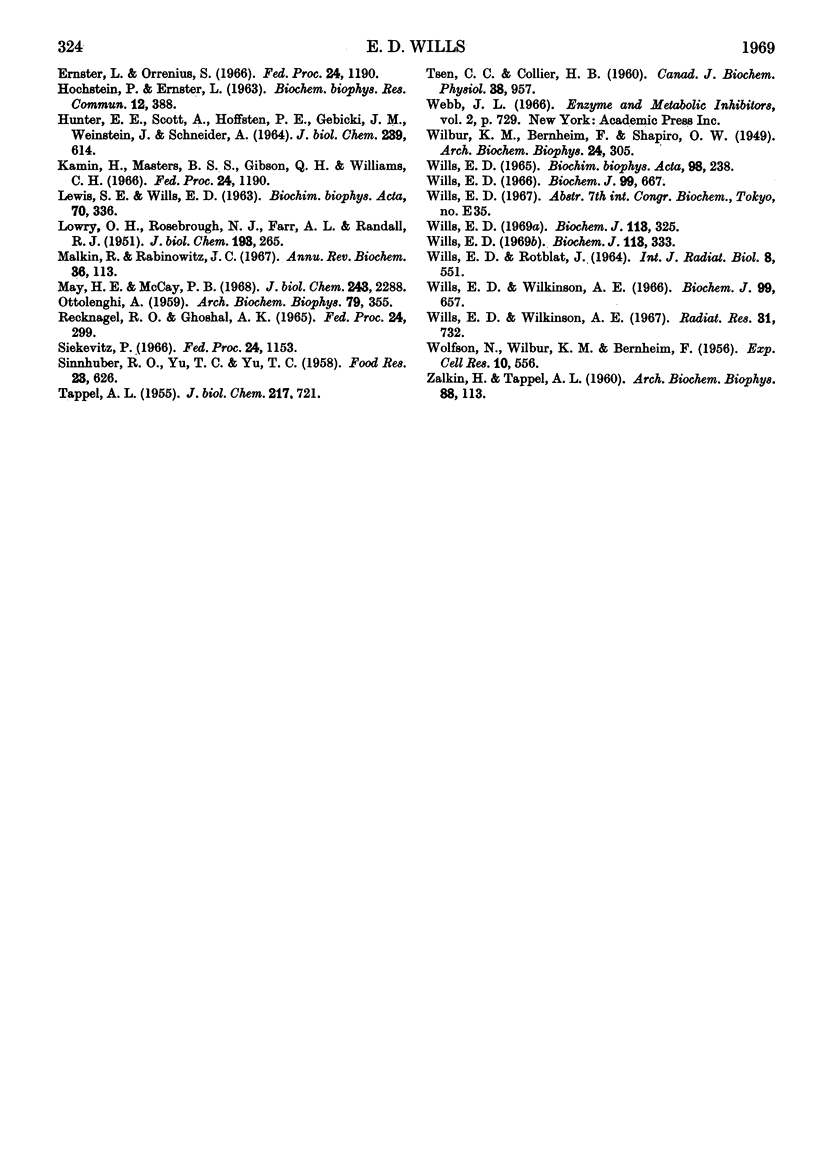
Selected References
These references are in PubMed. This may not be the complete list of references from this article.
- ALDRIDGE W. N. Liver and brain mitochondria. Biochem J. 1957 Nov;67(3):423–431. doi: 10.1042/bj0670423. [DOI] [PMC free article] [PubMed] [Google Scholar]
- Christophersen B. O. The inhibitory effect of reduced glutathione on the lipid peroxidation of the microsomal fraction and mitochondria. Biochem J. 1968 Jan;106(2):515–522. doi: 10.1042/bj1060515. [DOI] [PMC free article] [PubMed] [Google Scholar]
- Crook E. M., Morgan E. J. The reduction of dehydroascorbic acid in plant extracts. Biochem J. 1944;38(1):10–15. doi: 10.1042/bj0380010. [DOI] [PMC free article] [PubMed] [Google Scholar]
- DAHLE L. K., HILL E. G., HOLMAN R. T. The thiobarbituric acid reaction and the autoxidations of polyunsaturated fatty acid methyl esters. Arch Biochem Biophys. 1962 Aug;98:253–261. doi: 10.1016/0003-9861(62)90181-9. [DOI] [PubMed] [Google Scholar]
- Ernster L., Orrenius S. Substrate-induced synthesis of the hydroxylating enzyme system of liver microsomes. Fed Proc. 1965 Sep-Oct;24(5):1190–1199. [PubMed] [Google Scholar]
- HOCHSTEIN P., ERNSTER L. ADP-ACTIVATED LIPID PEROXIDATION COUPLED TO THE TPNH OXIDASE SYSTEM OF MICROSOMES. Biochem Biophys Res Commun. 1963 Aug 14;12:388–394. doi: 10.1016/0006-291x(63)90111-6. [DOI] [PubMed] [Google Scholar]
- HUNTER F. E., Jr, SCOTT A., HOFFSTEN P. E., GEBICKI J. M., WEINSTEIN J., SCHNEIDER A. STUDIES ON THE MECHANISM OF SWELLING, LYSIS, AND DISTINTEGRATION OF ISOLATED LIVER MITOCHONDRIA EXPOSED TO MIXTURES OF OXIDIZED AND REDUCED GLUTATHIONE. J Biol Chem. 1964 Feb;239:614–621. [PubMed] [Google Scholar]
- LEWIS S. E., WILLS E. D. Inhibition of the autoxidation of unsaturated fatty acids by haematin proteins. Biochim Biophys Acta. 1963 Jun 18;70:336–338. doi: 10.1016/0006-3002(63)90757-1. [DOI] [PubMed] [Google Scholar]
- LOWRY O. H., ROSEBROUGH N. J., FARR A. L., RANDALL R. J. Protein measurement with the Folin phenol reagent. J Biol Chem. 1951 Nov;193(1):265–275. [PubMed] [Google Scholar]
- May H. E., McCay P. B. Reduced triphosphopyridine nucleotide oxidase-catalyzed alterations of membrane phospholipids. I. Nature of the lipid alterations. J Biol Chem. 1968 May 10;243(9):2288–2295. [PubMed] [Google Scholar]
- Siekevitz P. Electron transport systems in microsomes. Origin and functional nature of microsomes. Fed Proc. 1965 Sep-Oct;24(5):1153–1155. [PubMed] [Google Scholar]
- TAPPEL A. L. Unsaturated lipide oxidation catalyzed by hematin compounds. J Biol Chem. 1955 Dec;217(2):721–733. [PubMed] [Google Scholar]
- WILBUR K. M., BERNHEIM F., SHAPIRO O. W. The thiobarbituric acid reagent as a test for the oxidation of unsaturated fatty acids by various agents. Arch Biochem. 1949 Dec;24(2):305–313. [PubMed] [Google Scholar]
- WILLS E. D. MECHANISMS OF LIPID PEROXIDE FORMATION IN TISSUES. ROLE OF METALS AND HAEMATIN PROTEINS IN THE CATALYSIS OF THE OXIDATION UNSATURATED FATTY ACIDS. Biochim Biophys Acta. 1965 Apr 5;98:238–251. doi: 10.1016/0005-2760(65)90118-9. [DOI] [PubMed] [Google Scholar]
- WILLS E. D., ROTBLAT J. THE FORMATION OF PEROXIDES IN TISSUE LIPIDS AND UNSATURATED FATTY ACIDS BY IRRADIATION. Int J Radiat Biol Relat Stud Phys Chem Med. 1964;8:551–567. doi: 10.1080/09553006414550701. [DOI] [PubMed] [Google Scholar]
- WOLFSON N., WILBUR K. M., BERNHEIM F. Lipid peroxide formation in regenerating rat liver. Exp Cell Res. 1956 Apr;10(2):556–558. doi: 10.1016/0014-4827(56)90031-3. [DOI] [PubMed] [Google Scholar]
- Wills E. D. Lipid peroxide formation in microsomes. Relationship of hydroxylation to lipid peroxide formation. Biochem J. 1969 Jun;113(2):333–341. doi: 10.1042/bj1130333. [DOI] [PMC free article] [PubMed] [Google Scholar]
- Wills E. D. Lipid peroxide formation in microsomes. The role of non-haem iron. Biochem J. 1969 Jun;113(2):325–332. doi: 10.1042/bj1130325. [DOI] [PMC free article] [PubMed] [Google Scholar]
- Wills E. D. Mechanisms of lipid peroxide formation in animal tissues. Biochem J. 1966 Jun;99(3):667–676. doi: 10.1042/bj0990667. [DOI] [PMC free article] [PubMed] [Google Scholar]
- Wills E. D., Wilkinson A. E. Release of enzymes from lysosomes by irradiation and the relation of lipid peroxide formation to enzyme release. Biochem J. 1966 Jun;99(3):657–666. doi: 10.1042/bj0990657. [DOI] [PMC free article] [PubMed] [Google Scholar]
- ZALKIN H., TAPPEL A. L. Studies of the mechanism of vitamin E action. IV. Lipide peroxidation in the vitamin E-deficient rabbit. Arch Biochem Biophys. 1960 May;88:113–117. doi: 10.1016/0003-9861(60)90205-8. [DOI] [PubMed] [Google Scholar]