Abstract
Generalized microscopic reversibility implies that the apparent rate of any catalytic process in a complex mechanism is paralleled by substrate desorption in such a way that this ratio is held constant within the reaction mechanism [Whitehead (1976) Biochem. J. 159, 449--456]. The physical and evolutionary significances of this concept, for both polymeric and monomeric enzymes, are discussed. For polymeric enzymes, generalized microscopic reversibility of necessity occurs if, within the same reaction sequence, the substrate stabilizes one type of conformation of the active site only. Generalized microscopic reversibility suppresses the kinetic co-operativity of the slow transition model [Ainslie, Shill & Neet (1972) J. Biol. Chem. 247, 7088--7096]. This situation is obtained if the free-energy difference between the corresponding transition states of the two enzyme forms is held constant along the reaction co-ordinate. This situation implies that the 'extra costs' of energy (required to pass each energy barrier) that are not covered by the corresponding binding energies of the transition states vary in a similar way along the two reaction co-ordinates. The regulatory behaviour of monomeric enzymes is discussed in the light of the concept of 'catalytic perfection' proposed by Albery & Knowles [(1976) Biochemistry 15, 5631--5640]. These authors claim that an enzyme will be catalytically 'perfect' when its catalytic efficiency is maximum. If this situation occurs for a monomeric enzyme obeying either the slow transition or the mnemonical model, it can be shown that the kinetic co-operativity disappears. In other words, kinetic co-operativity of a monomeric enzyme is 'paid for' at the expense of catalytic efficiency, and the monomeric enzyme cannot be simultaneously co-operative and catalytically very efficient. This is precisely what has been found experimentally in a number of cases.
Full text
PDF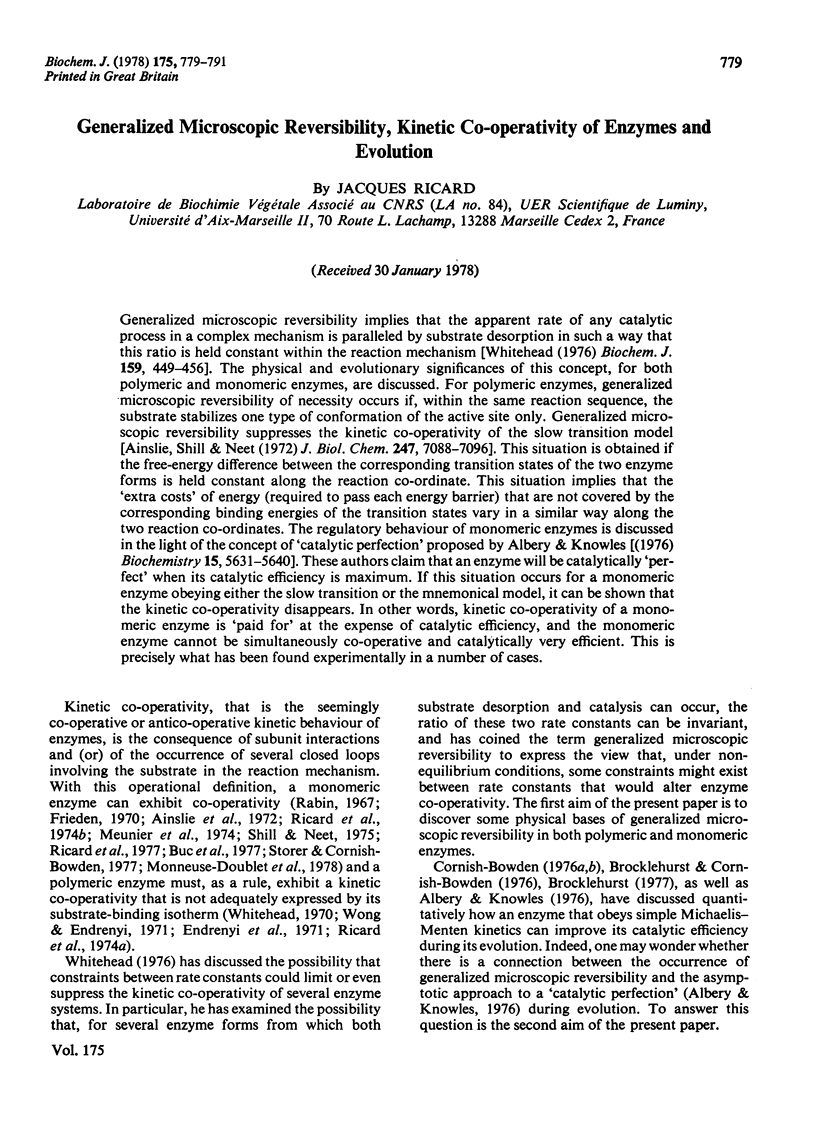
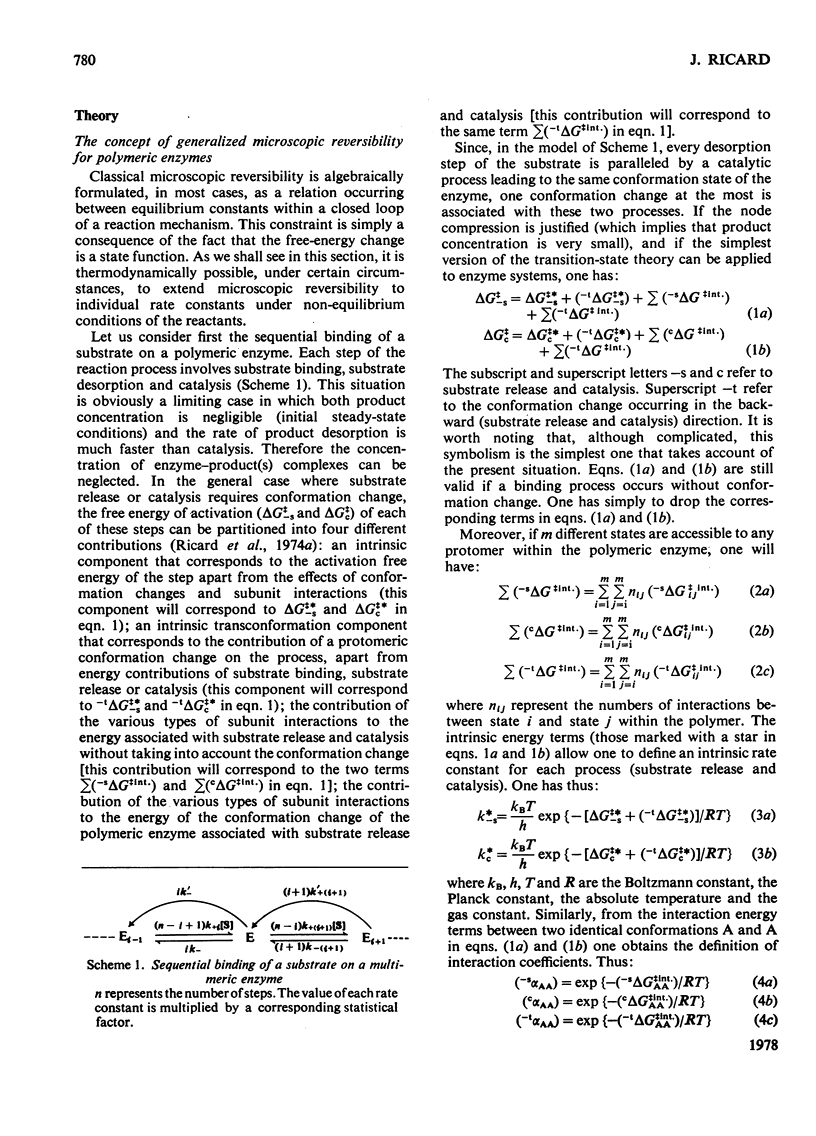
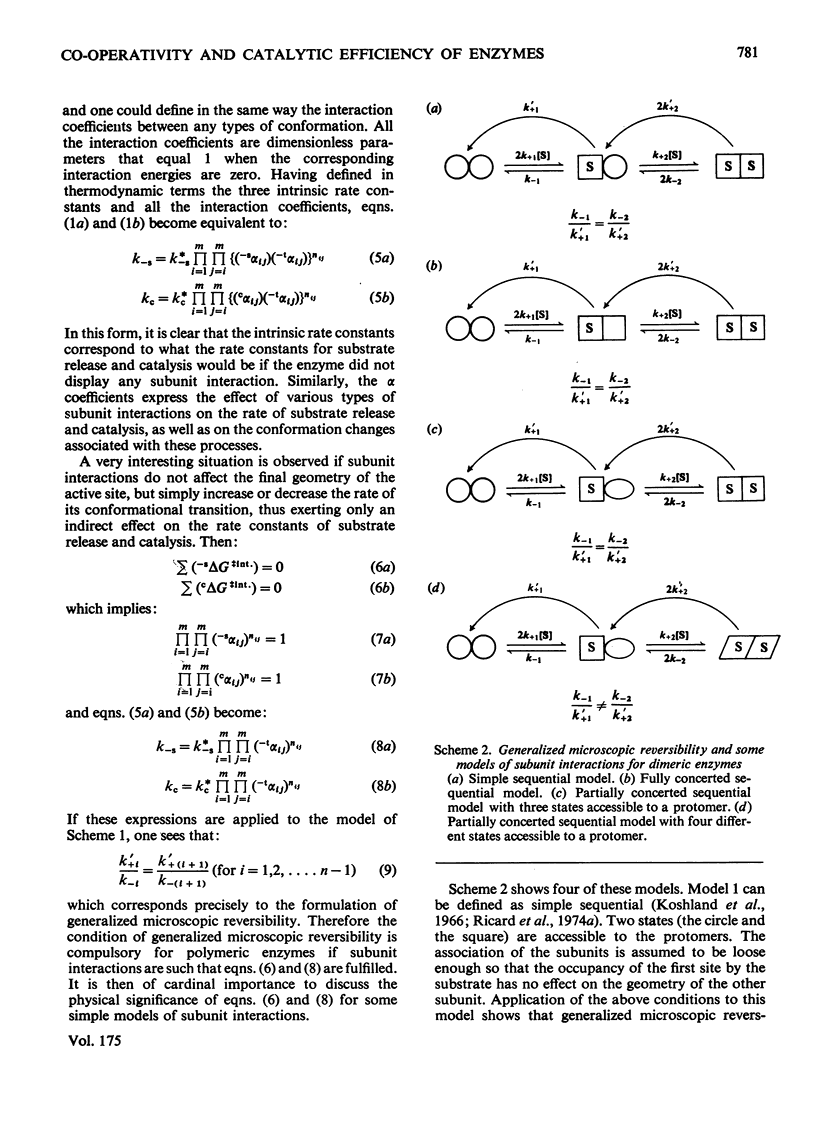
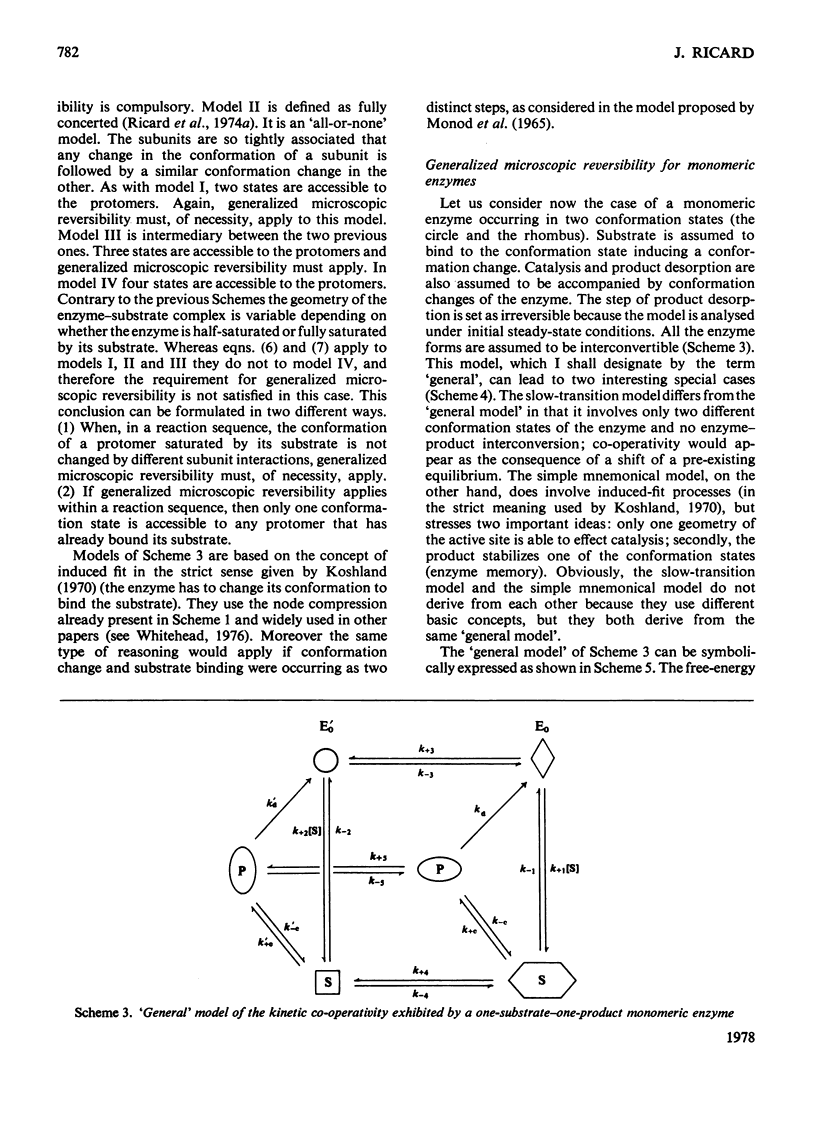
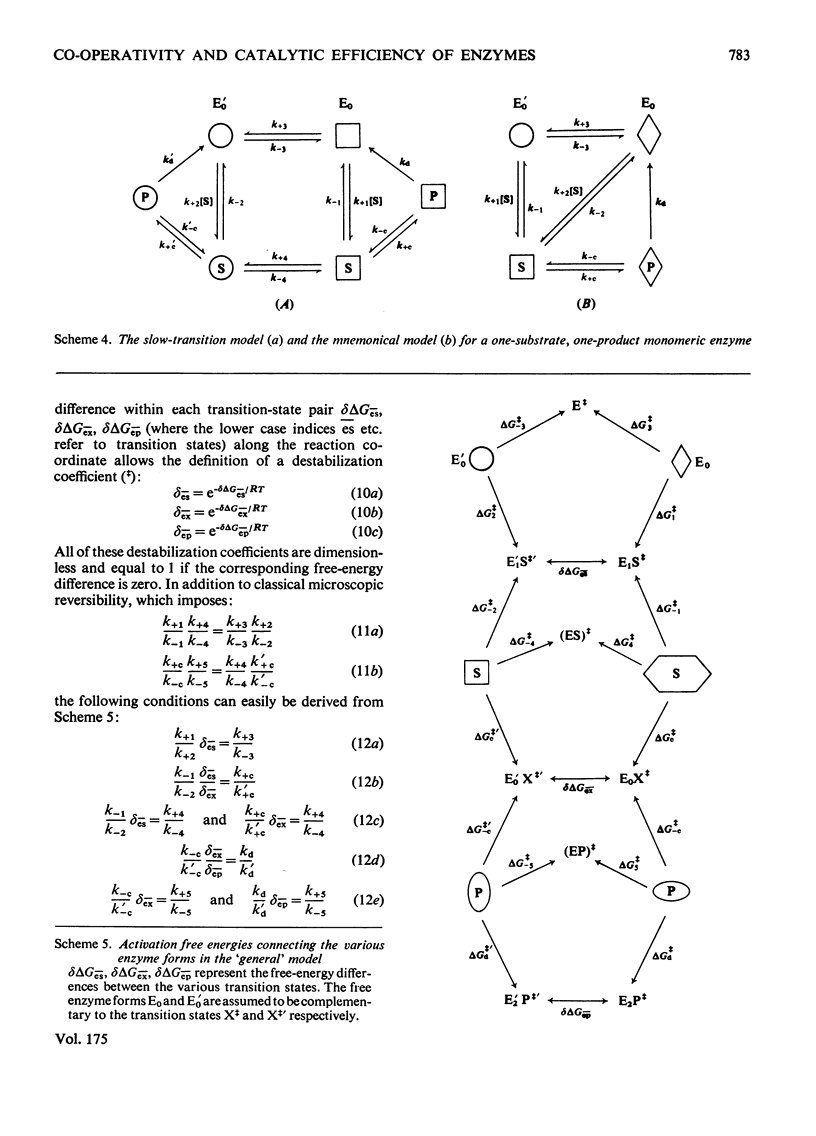
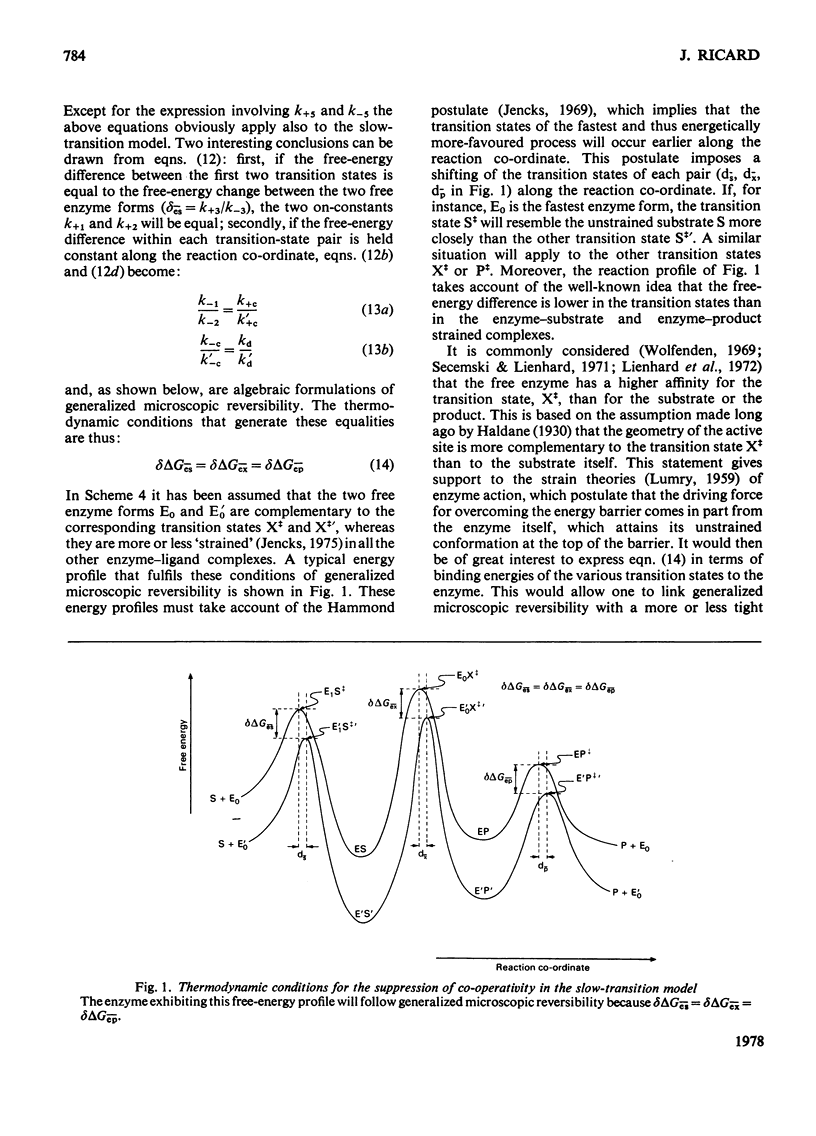
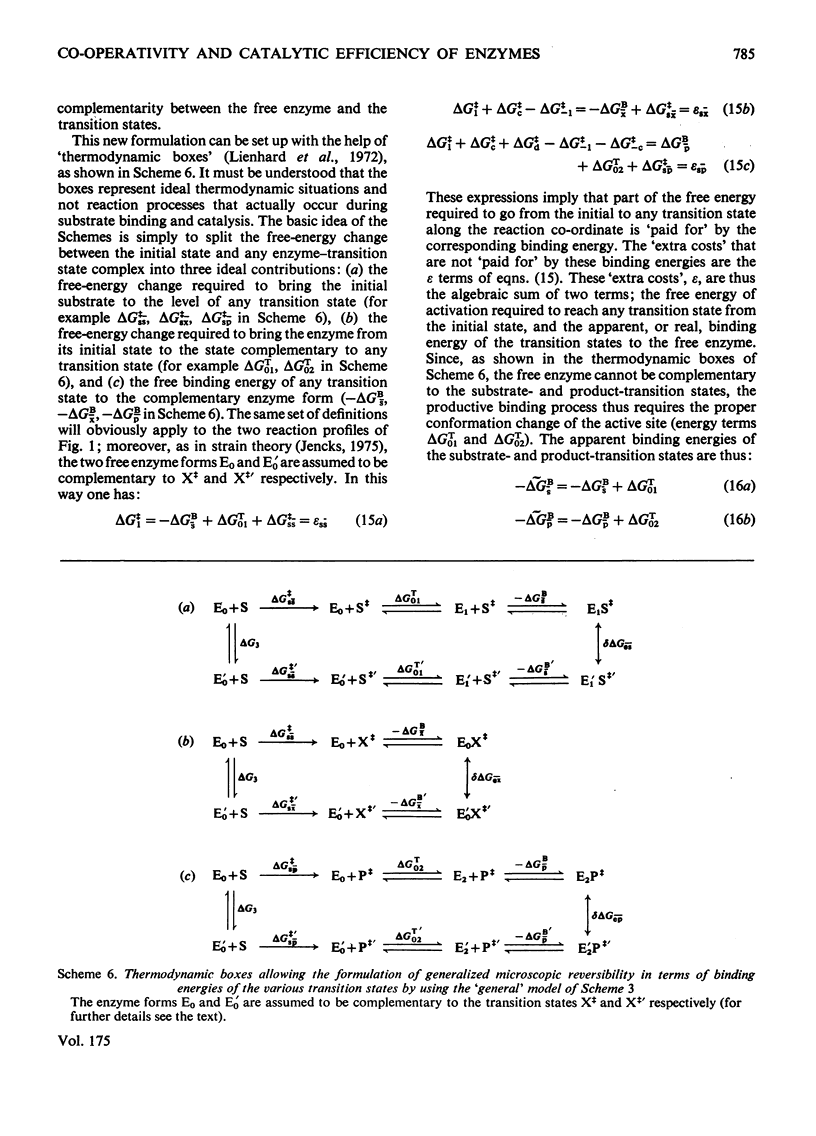
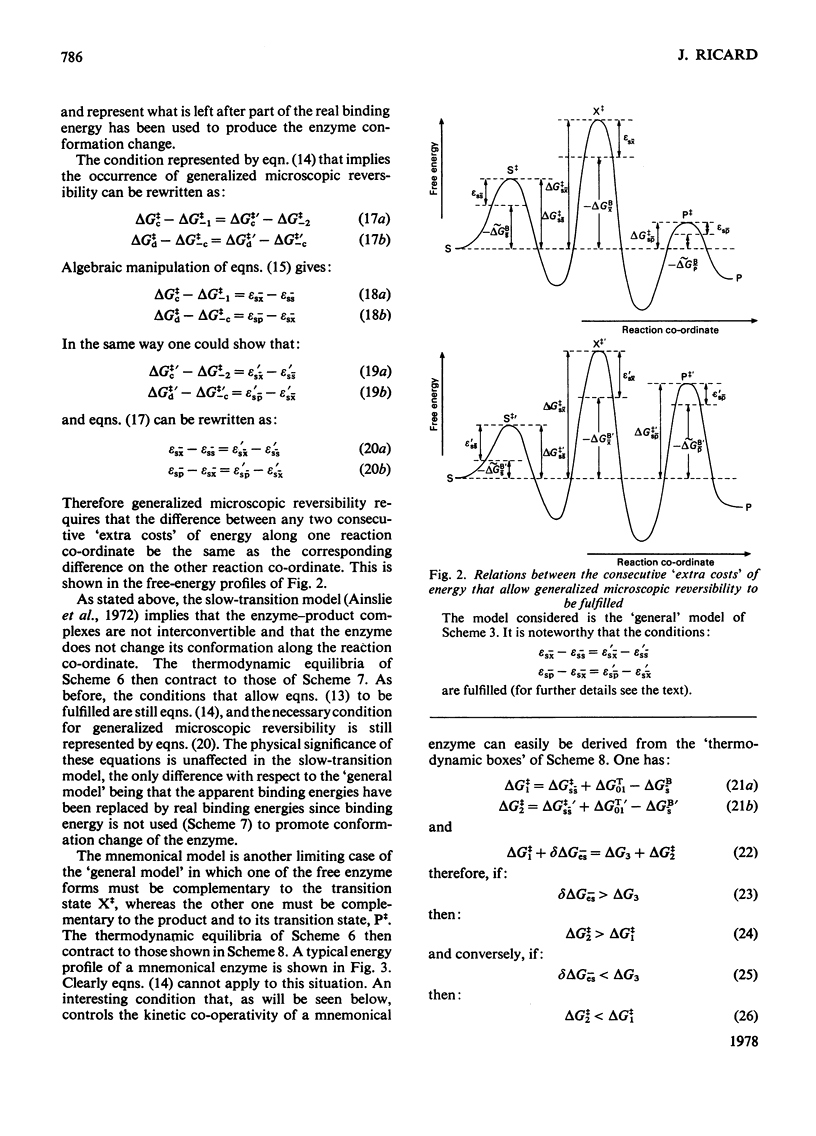
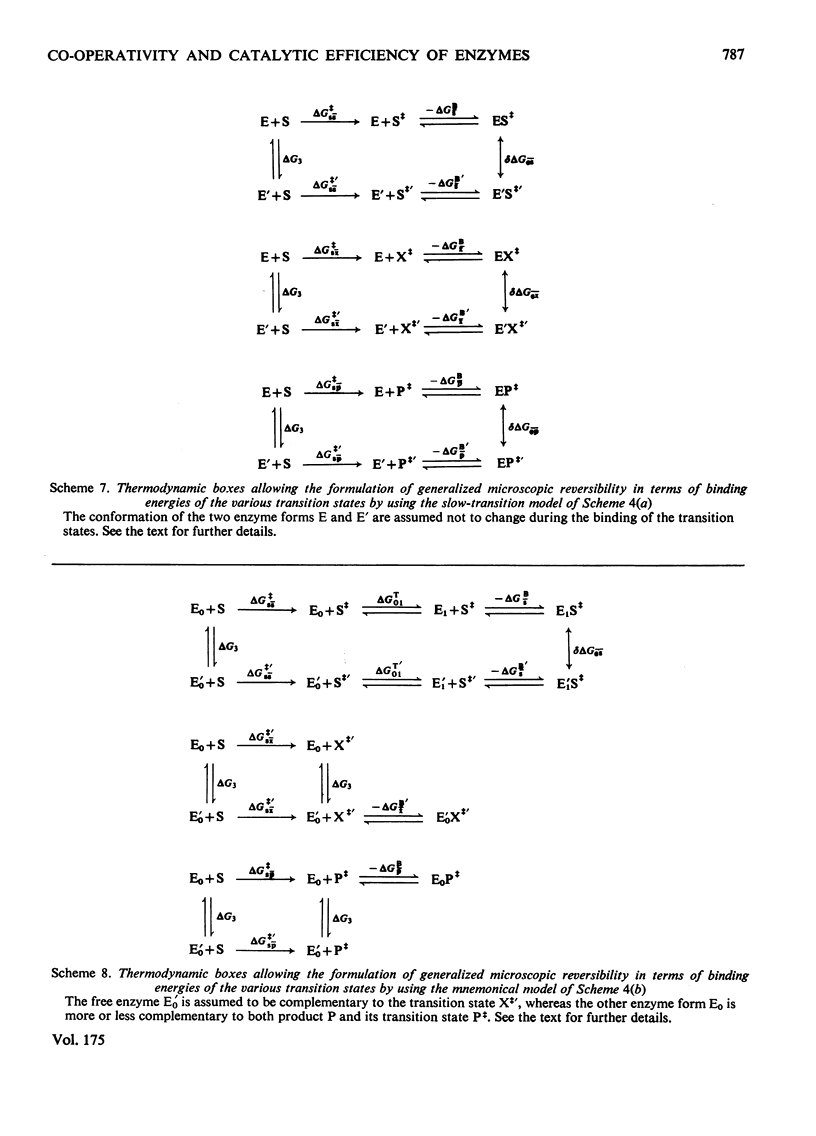
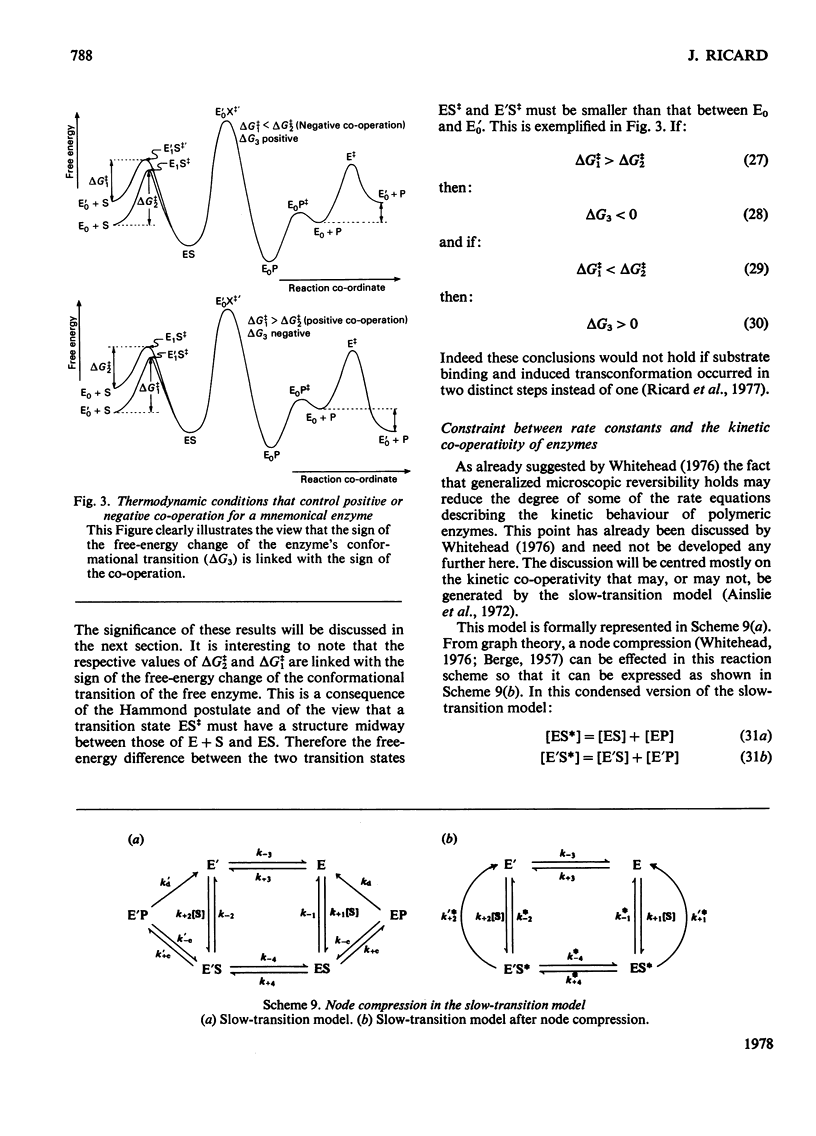
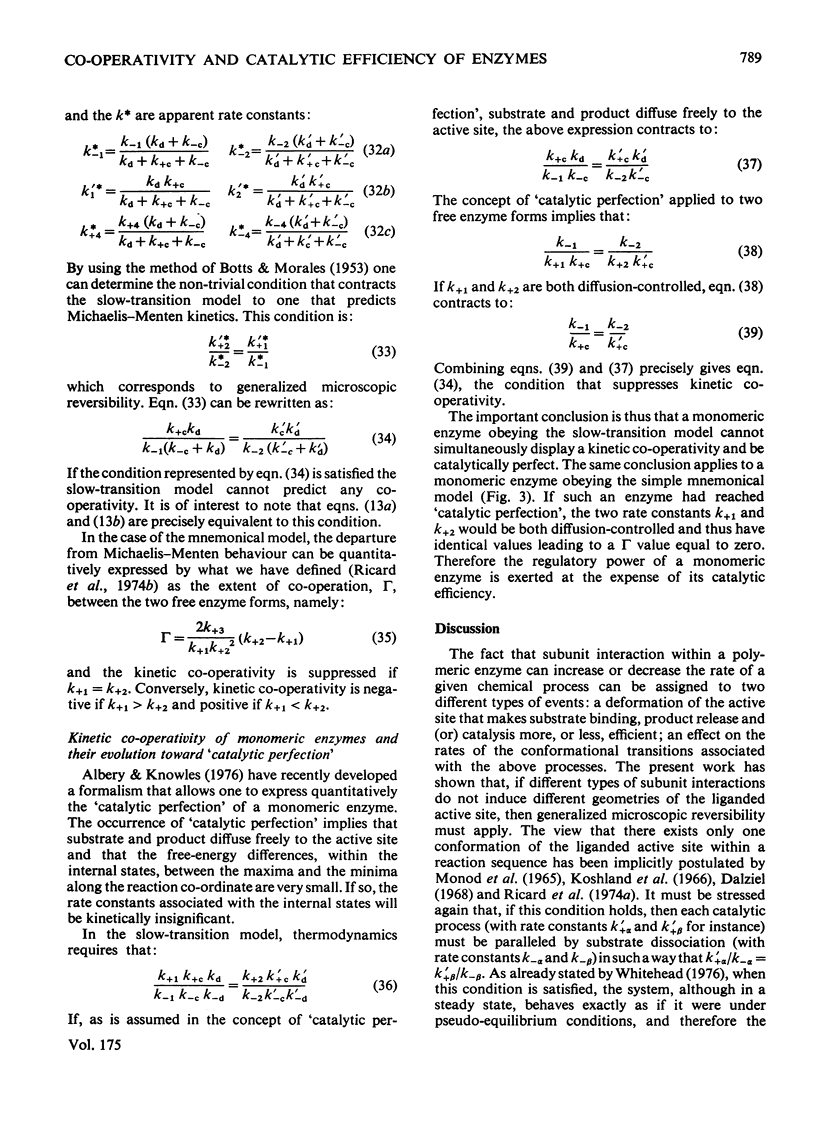
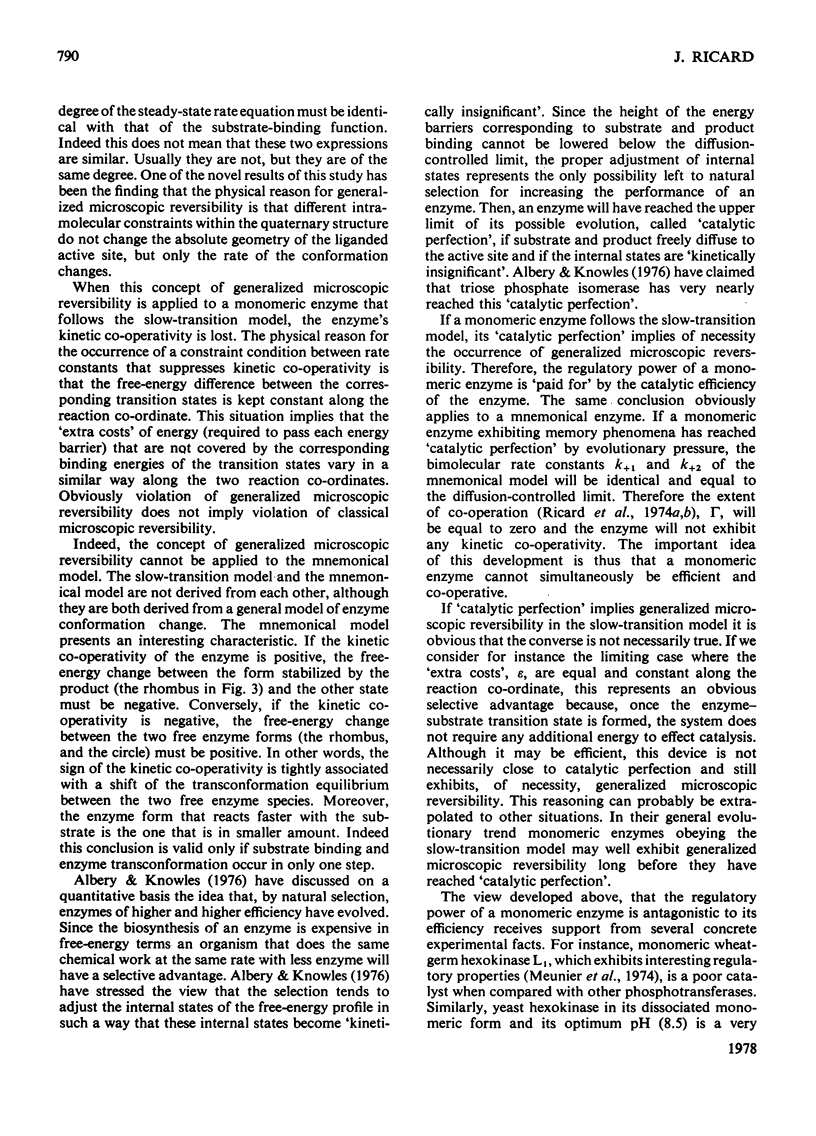
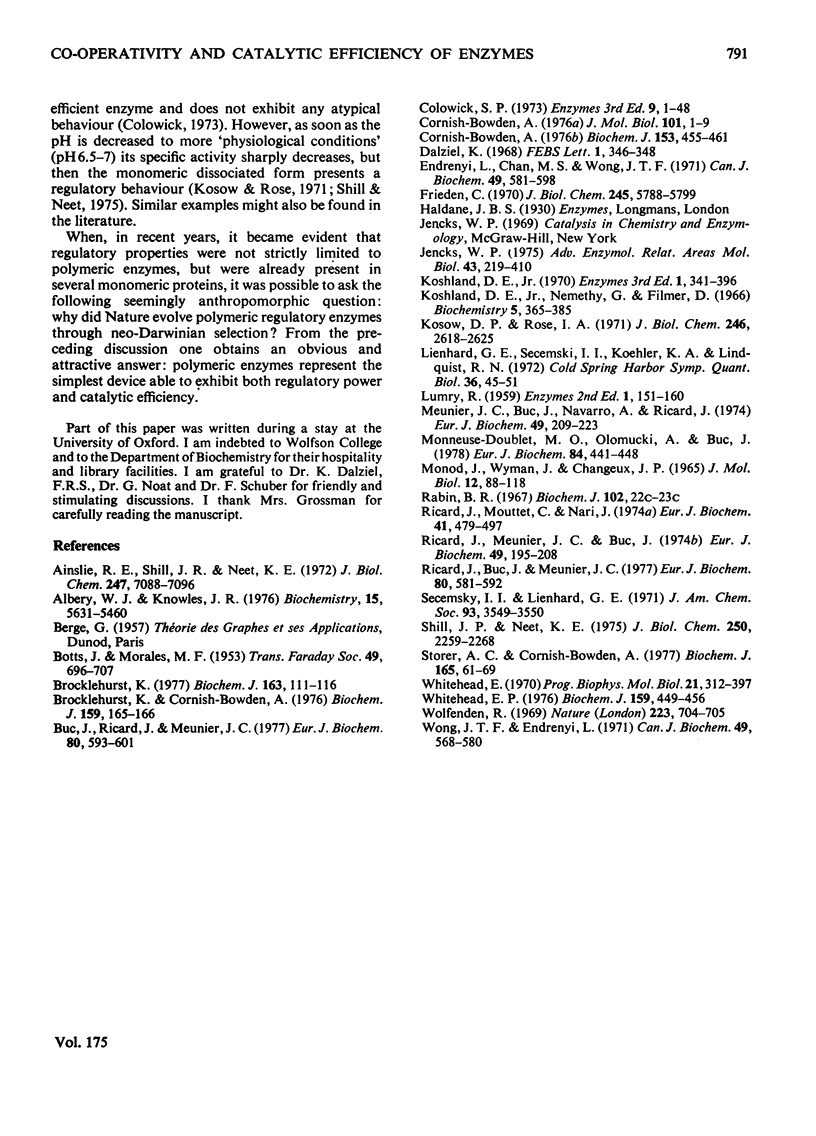
Selected References
These references are in PubMed. This may not be the complete list of references from this article.
- Ainslie G. R., Jr, Shill J. P., Neet K. E. Transients and cooperativity. A slow transition model for relating transients and cooperative kinetics of enzymes. J Biol Chem. 1972 Nov 10;247(21):7088–7096. [PubMed] [Google Scholar]
- Brocklehurst K., Cornish-Bowden A. The pre-eminence of k(cat) in the manifestation of optimal enzymic activity delineated by using the Briggs-Haldane two-step irreversible kinetic model. Biochem J. 1976 Oct 1;159(1):165–166. doi: 10.1042/bj1590165. [DOI] [PMC free article] [PubMed] [Google Scholar]
- Brocklehurst K. Evolution of enzyme catalytic power. Characteristics of optimal catalysis evaluated for the simplest plausible kinetic model. Biochem J. 1977 Apr 1;163(1):111–116. doi: 10.1042/bj1630111. [DOI] [PMC free article] [PubMed] [Google Scholar]
- Buc J., Ricard J., Meunier J. C. Enzyme memory. 2. Kinetics and thermodynamics of the slow conformation changes of wheat-germ hexokinase LI. Eur J Biochem. 1977 Nov 1;80(2):593–601. doi: 10.1111/j.1432-1033.1977.tb11916.x. [DOI] [PubMed] [Google Scholar]
- Cornish-Bowden A. The effect of natural selection on enzymic catalysis. J Mol Biol. 1976 Feb 15;101(1):1–9. doi: 10.1016/0022-2836(76)90062-0. [DOI] [PubMed] [Google Scholar]
- Endrenyi L., Chan M. S., Wong J. T. Interpretation of nonhyperbolic behavior in enzymic systems. II. Quantitative characteristics of rate and binding functions. Can J Biochem. 1971 May;49(5):581–598. doi: 10.1139/o71-085. [DOI] [PubMed] [Google Scholar]
- Frieden C. Kinetic aspects of regulation of metabolic processes. The hysteretic enzyme concept. J Biol Chem. 1970 Nov 10;245(21):5788–5799. [PubMed] [Google Scholar]
- Jencks W. P. Binding energy, specificity, and enzymic catalysis: the circe effect. Adv Enzymol Relat Areas Mol Biol. 1975;43:219–410. doi: 10.1002/9780470122884.ch4. [DOI] [PubMed] [Google Scholar]
- Jones T. I., Vaage O., Zimmer T. L., Frøholm L. O., Laland S. G. The presence of protein bound intermediates in the biosynthesis of gramicidin S. FEBS Lett. 1968 Oct;1(5):346–348. doi: 10.1016/0014-5793(68)80152-8. [DOI] [PubMed] [Google Scholar]
- Koshland D. E., Jr, Némethy G., Filmer D. Comparison of experimental binding data and theoretical models in proteins containing subunits. Biochemistry. 1966 Jan;5(1):365–385. doi: 10.1021/bi00865a047. [DOI] [PubMed] [Google Scholar]
- Kosow D. P., Rose I. A. Activators of yeast hexokinase. J Biol Chem. 1971 Apr 25;246(8):2618–2625. [PubMed] [Google Scholar]
- Lienhard G. E., Secemski I. I., Koehler K. A., Lindquist R. N. Enzymatic catalysis and the transition state theory of reaction rates: transition state analogs. Cold Spring Harb Symp Quant Biol. 1972;36:45–51. doi: 10.1101/sqb.1972.036.01.009. [DOI] [PubMed] [Google Scholar]
- MONOD J., WYMAN J., CHANGEUX J. P. ON THE NATURE OF ALLOSTERIC TRANSITIONS: A PLAUSIBLE MODEL. J Mol Biol. 1965 May;12:88–118. doi: 10.1016/s0022-2836(65)80285-6. [DOI] [PubMed] [Google Scholar]
- Meunier J. C., Buc J., Navarro A., Ricard J. Regulatory behavior of monomeric enzymes. 2. A wheat-germ hexokinase as a mnemonical enzyme. Eur J Biochem. 1974 Nov 1;49(1):209–223. doi: 10.1111/j.1432-1033.1974.tb03826.x. [DOI] [PubMed] [Google Scholar]
- Monneuse-Doublet M. O., Olomucki A., Buc J. Investigation on the kinetic mechanism of octopine dehydrogenase. A regulatory behavior. Eur J Biochem. 1978 Mar 15;84(2):441–448. doi: 10.1111/j.1432-1033.1978.tb12185.x. [DOI] [PubMed] [Google Scholar]
- Ricard J., Buc J., Meunier J. C. Enzyme memory. 1. A transient kinetic study of wheat-germ hexokinase LI. Eur J Biochem. 1977 Nov 1;80(2):581–592. doi: 10.1111/j.1432-1033.1977.tb11915.x. [DOI] [PubMed] [Google Scholar]
- Ricard J., Meunier J. C., Buc J. Regulatory behavior of monomeric enzymes. 1. The mnemonical enzyme concept. Eur J Biochem. 1974 Nov 1;49(1):195–208. doi: 10.1111/j.1432-1033.1974.tb03825.x. [DOI] [PubMed] [Google Scholar]
- Ricard J., Mouttet C., Nari J. Subunit interactions in enzyme catalysis. Kinetic models for one-substrate polymeric enzymes. Eur J Biochem. 1974 Feb 1;41(3):479–497. doi: 10.1111/j.1432-1033.1974.tb03290.x. [DOI] [PubMed] [Google Scholar]
- Secemski I. I., Lienhard G. E. The role of strain in catalysis by lysozyme. J Am Chem Soc. 1971 Jul 14;93(14):3549–3550. doi: 10.1021/ja00743a054. [DOI] [PubMed] [Google Scholar]
- Storer A. C., Cornish-Bowden A. Kinetic evidence for a 'mnemonical' mechanism for rat liver glucokinase. Biochem J. 1977 Jul 1;165(1):61–69. doi: 10.1042/bj1650061. [DOI] [PMC free article] [PubMed] [Google Scholar]
- Whitehead E. P. Simplifications of the derivations and forms of steady-state equations for non-equilibrium random substrate-modifier and allosteric enzyme mechanisms. Biochem J. 1976 Dec 1;159(3):449–456. doi: 10.1042/bj1590449a. [DOI] [PMC free article] [PubMed] [Google Scholar]
- Whitehead E. The regulation of enzyme activity and allosteric transition. Prog Biophys Mol Biol. 1970;21:321–397. doi: 10.1016/0079-6107(70)90028-3. [DOI] [PubMed] [Google Scholar]
- Wolfenden R. Transition state analogues for enzyme catalysis. Nature. 1969 Aug 16;223(5207):704–705. doi: 10.1038/223704a0. [DOI] [PubMed] [Google Scholar]
- Wong J. T., Endrenyi L. Interpretation of nonhyperbolic behavior in enzymic systems. I. Differentiation of model mechanisms. Can J Biochem. 1971 May;49(5):568–580. doi: 10.1139/o71-084. [DOI] [PubMed] [Google Scholar]