Abstract
A general route for the synthesis of chloromethyl ketone derivatives of fatty acids is described. 5-Chloro-4-oxopentanoic acid, 7-chloro-6-oxoheptanoic acid, 9-chloro-8-oxononanoic acid and 11-chloro-10-oxoundecanoic acid were synthesized by this method and tested as covalent inhibitors of pig heart acetoacetyl-CoA thiolase. The K1 decreased by approx. 20-fold for each pair of methylenes added to the chain length, showing that the initial stage in inhibitor binding occurs at a non-polar region of the protein. This region is probably located at the enzyme active site, since inhibition was prevented by acetoacetyl-CoA or acetyl-CoA but not by CoA. The site of modification by chloromethyl ketone derivatives of fatty acids is restricted to a thiol group, since inactivation of the enzyme was prevented by reversible thiomethylation of the active-site thiol. In contrast, an amino-directed reagent, citraconic anhydride, still inactivated the enzyme, even when the active-site thiol was protected. Evidence that the enzyme thiol was particularly reactive came from studies on the pH-dependence of the alkylation reaction and thiol-competition experiments. Inhibition of the enzyme proceeded suprisingly well at acidic pH values and a 10(5) molar excess of external thiol over active-site thiol was required to prevent inhibition by 0.3 mM-9-chloro-8-oxononanoic acid. In addition to inhibiting isolated acetoacetyl-CoA thiolase, in hepatocytes the chloromethyl ketone derivatives of fatty acids also inhibited chloresterol synthesis, which uses this enzyme as an early step in the biosynthetic pathway. In isolated cells, the chloromethyl ketone derivatives of fatty acids were considerably less specific in their inhibitory action compared with 3-acetylenic derivatives of fatty acids, which act as suicide inhibitors of acetoacetyl-CoA thiolase. However, 9-chloro-8-oxononanoic acid was also an effective inhibitor of both hepatic cholesterol and fatty acid synthesis in mice in vivo, whereas the acetylenic fatty acid derivative, dec-3-ynoic acid, was completely ineffective. The effective inhibitory dose of 9-chloro-8-oxononanoic acid (2.5-5 mg/kg) was substantially lower than the estimated LD50 for the inhibitor (100 mg/kg).
Full text
PDF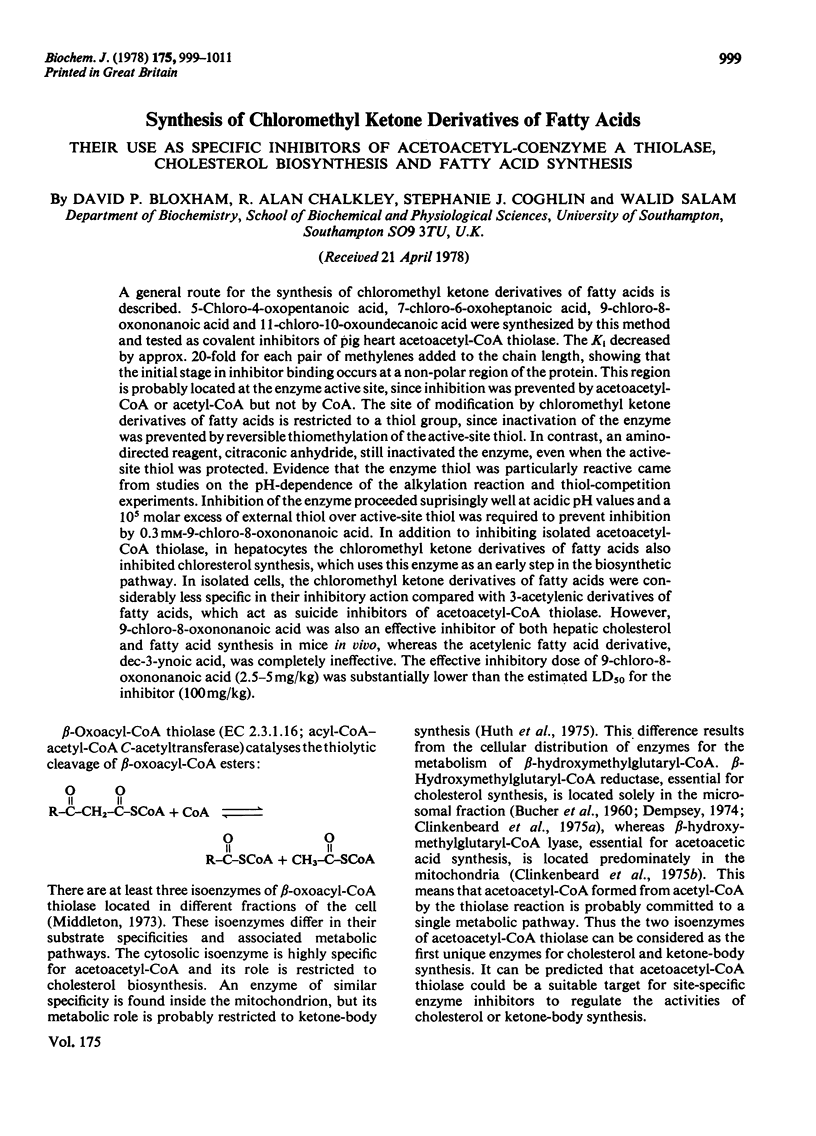
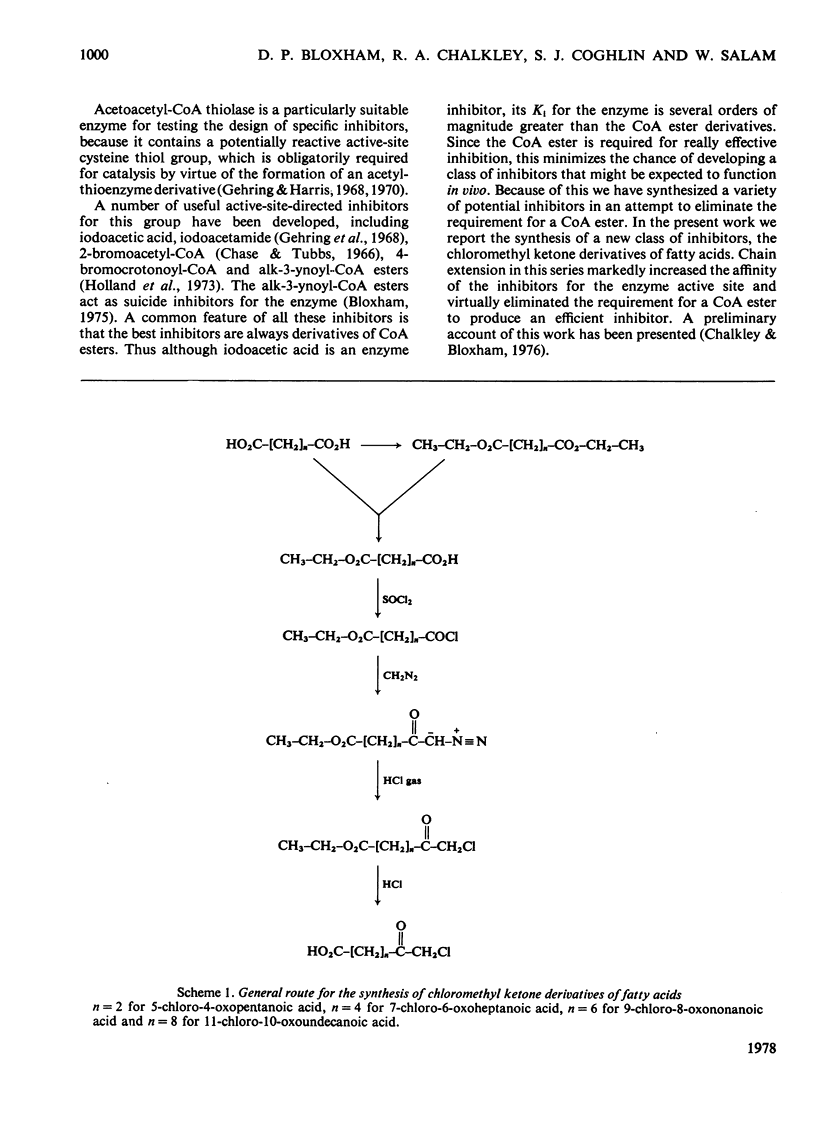
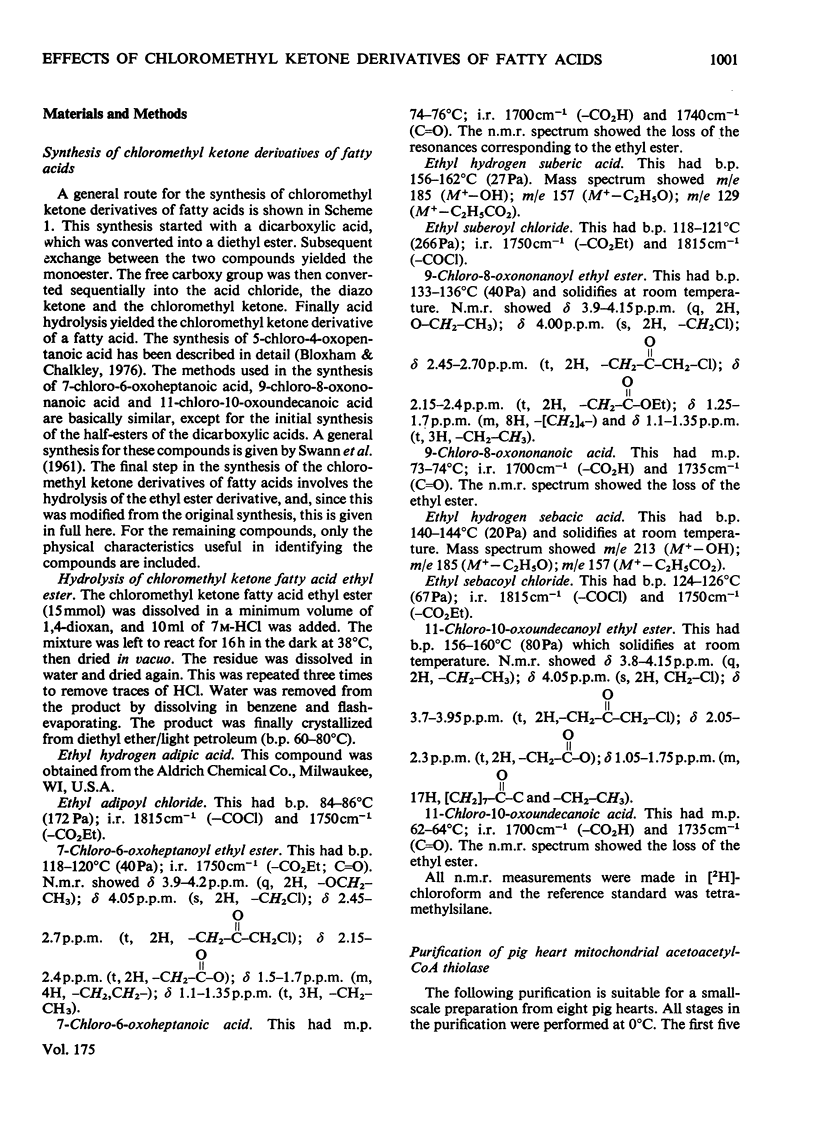
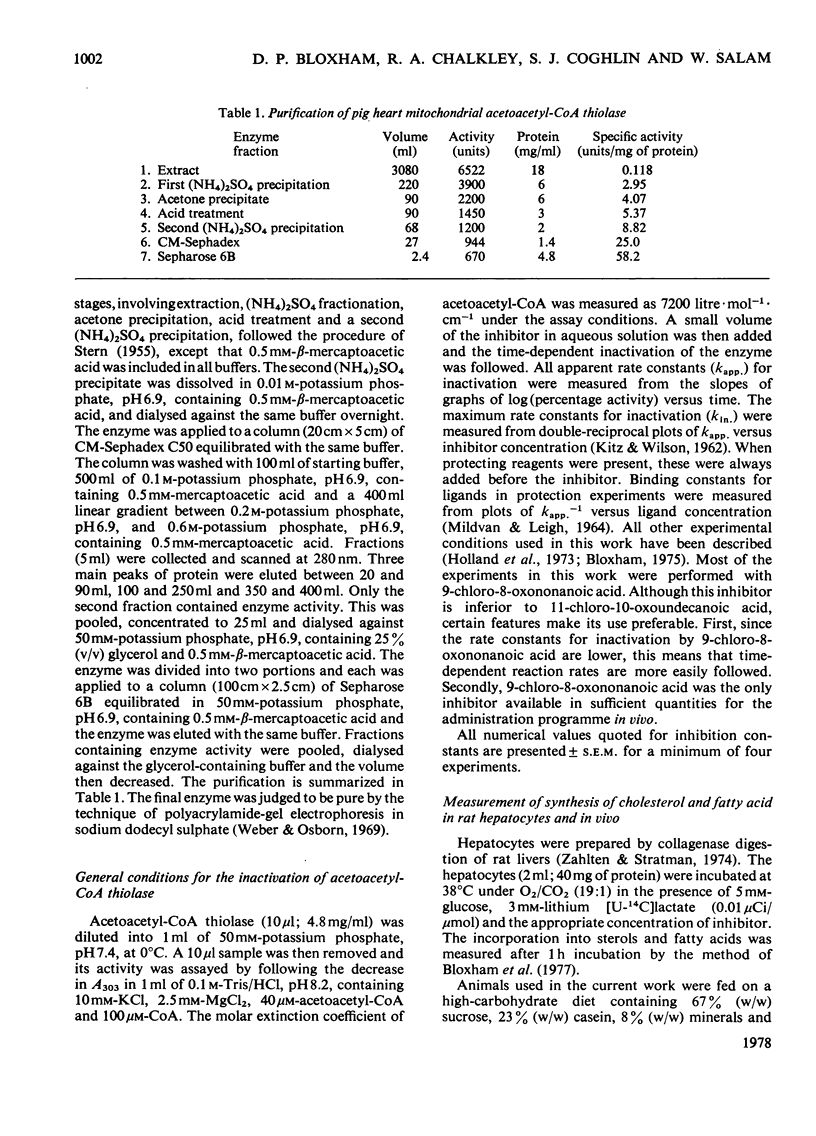
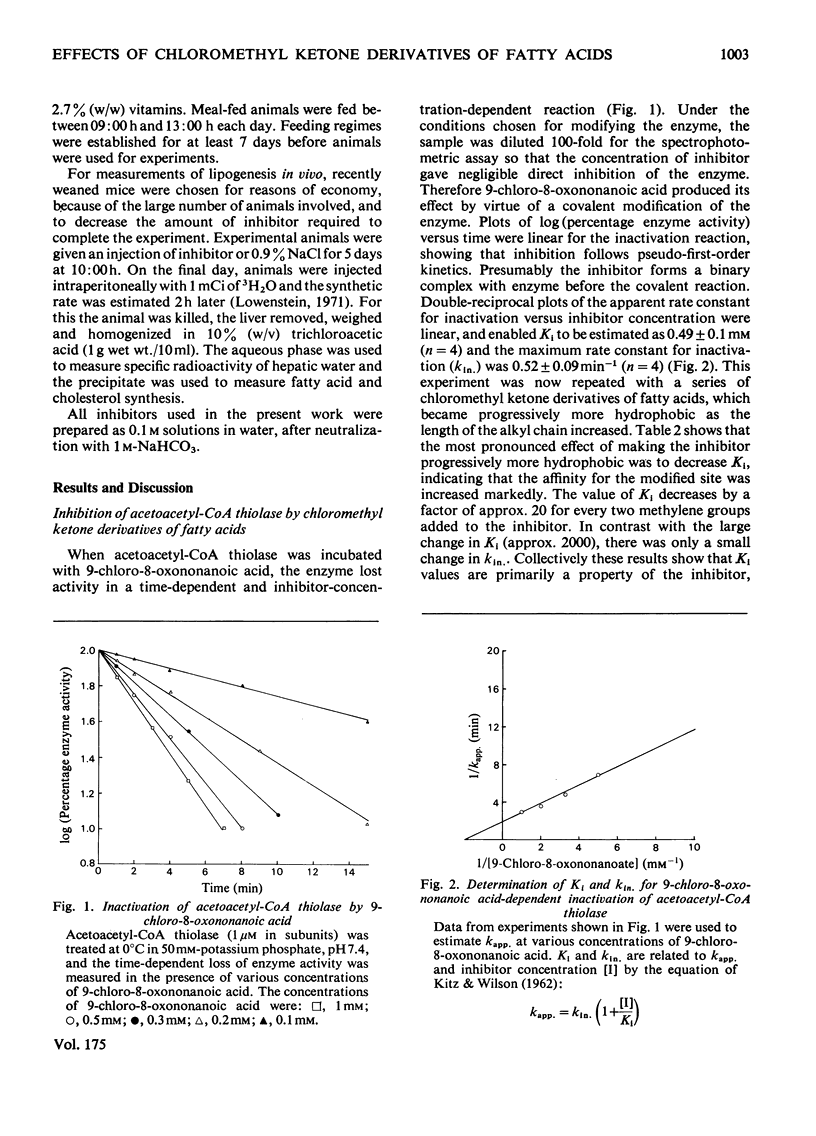
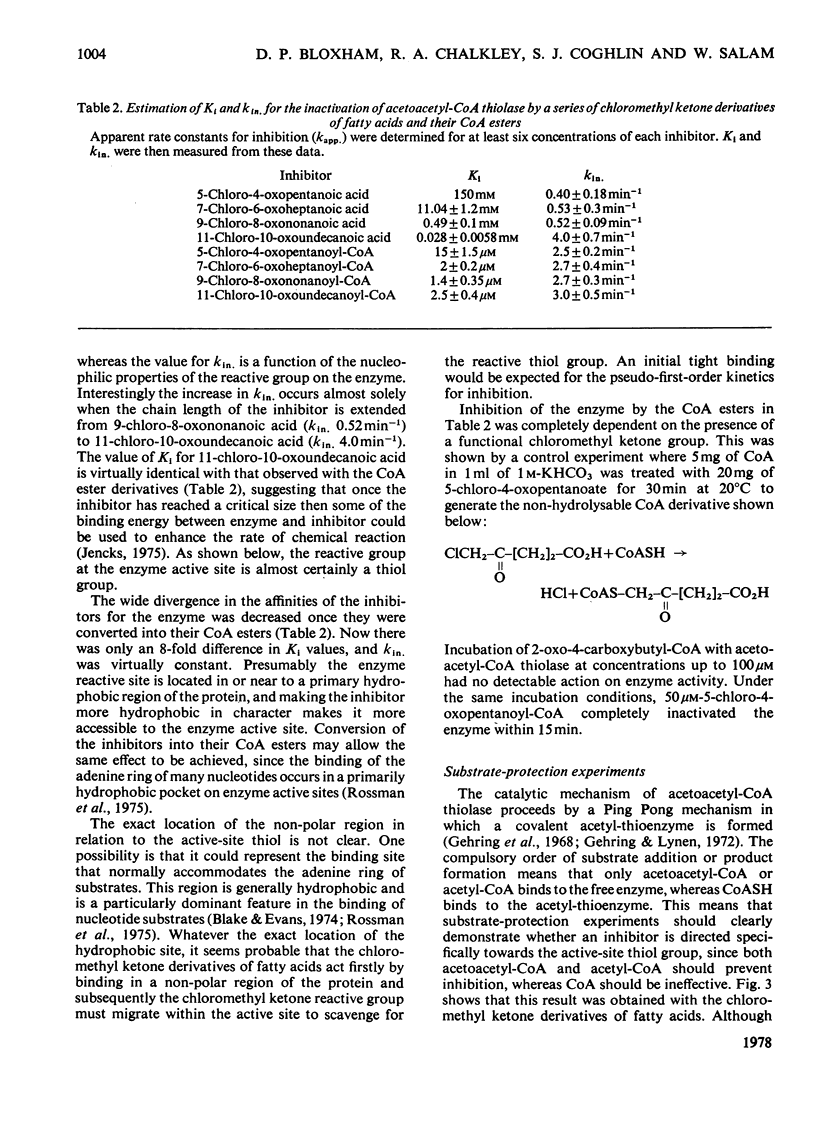
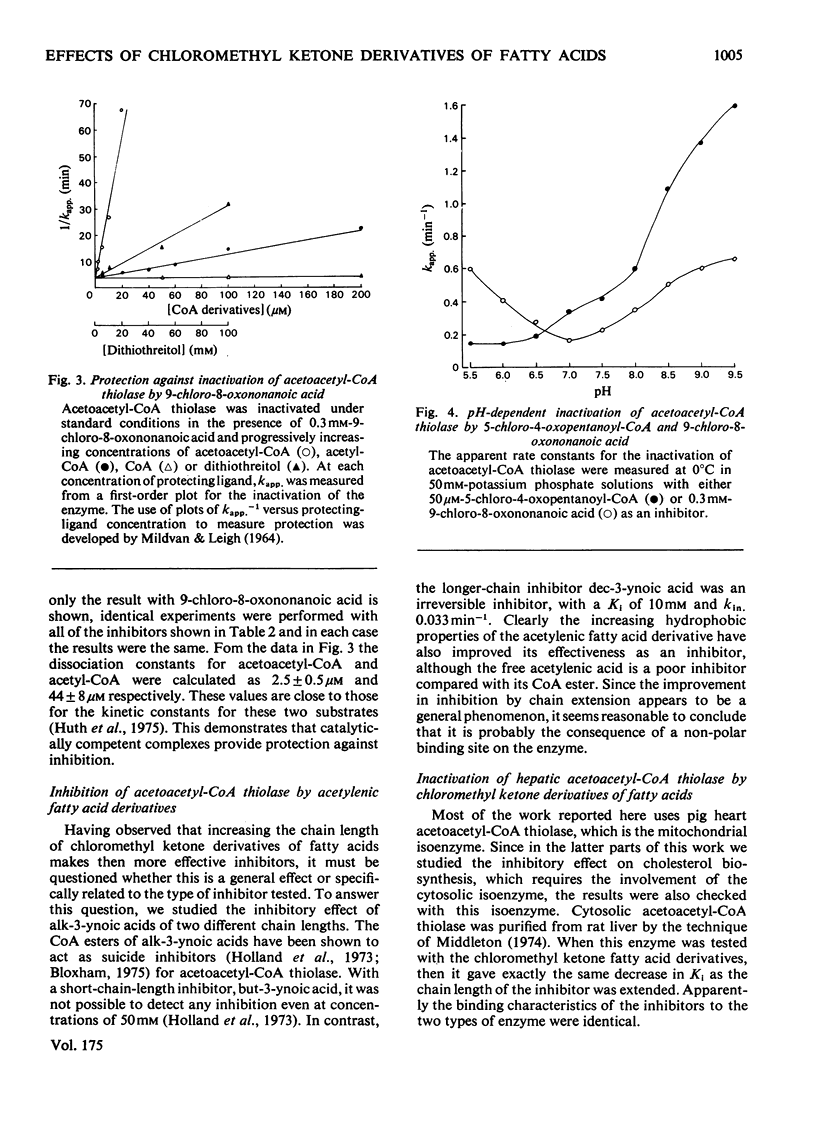
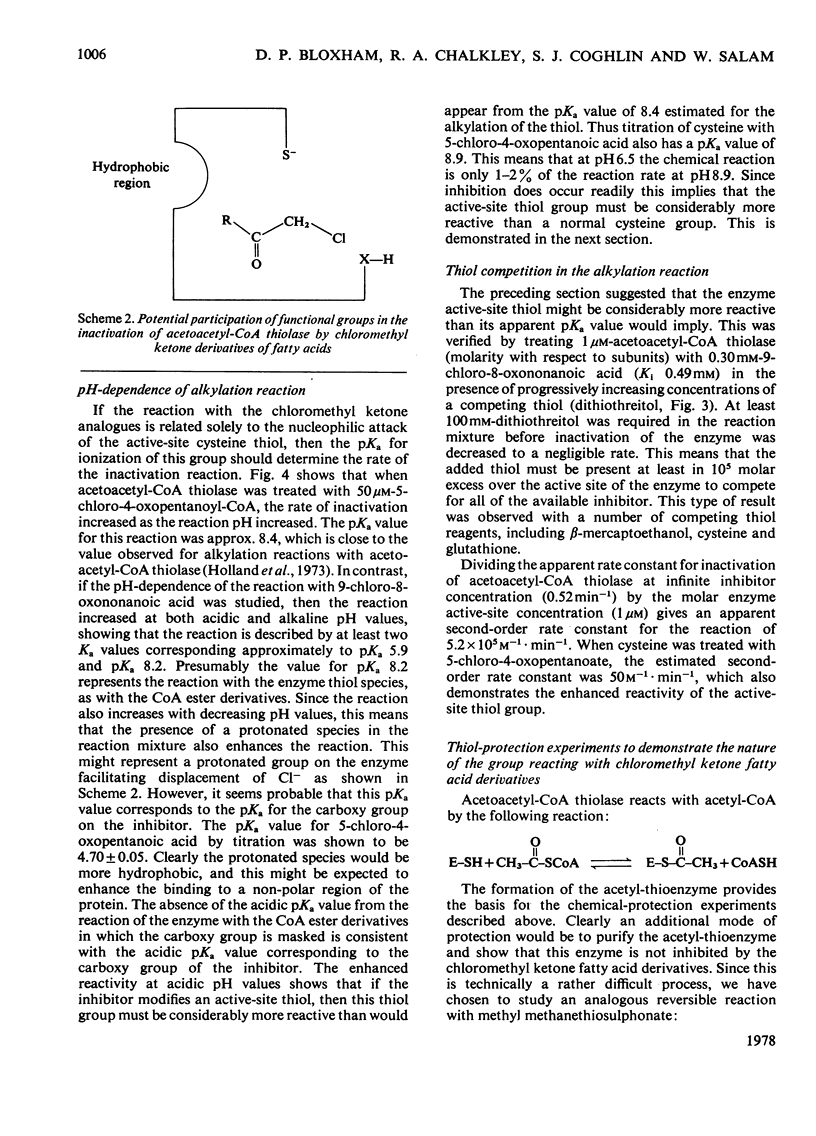
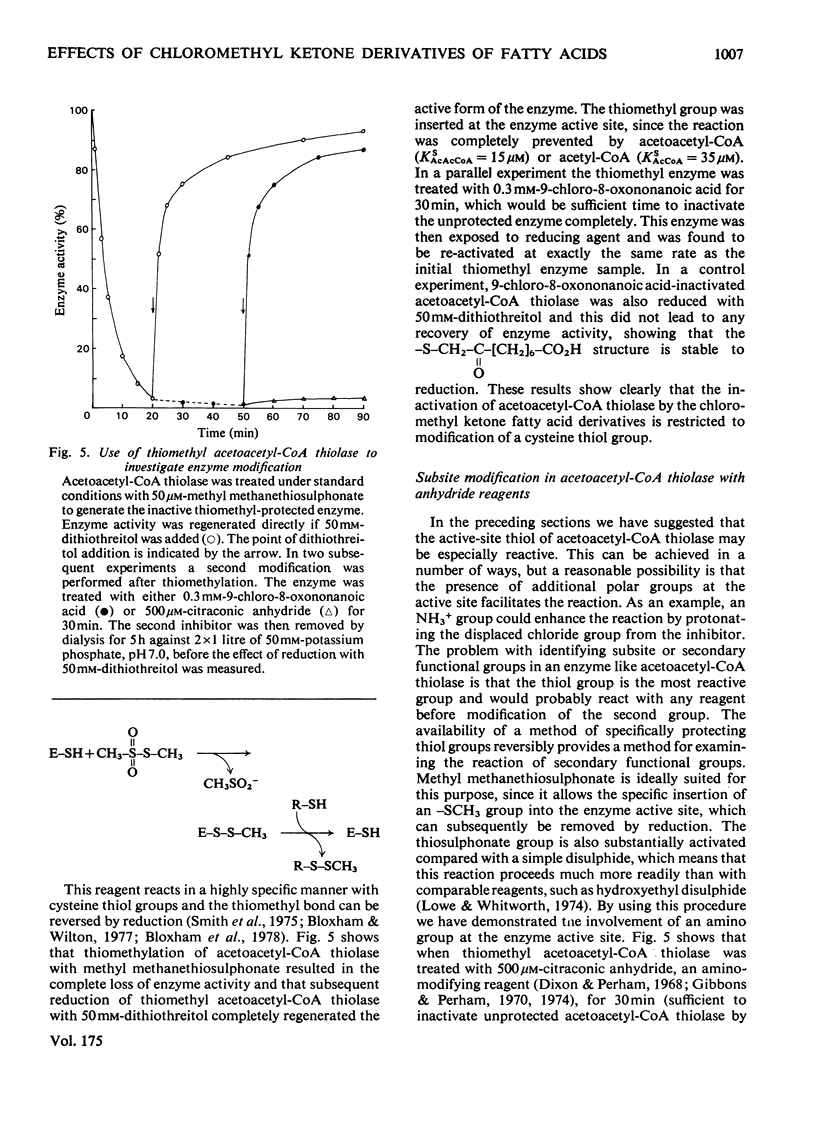
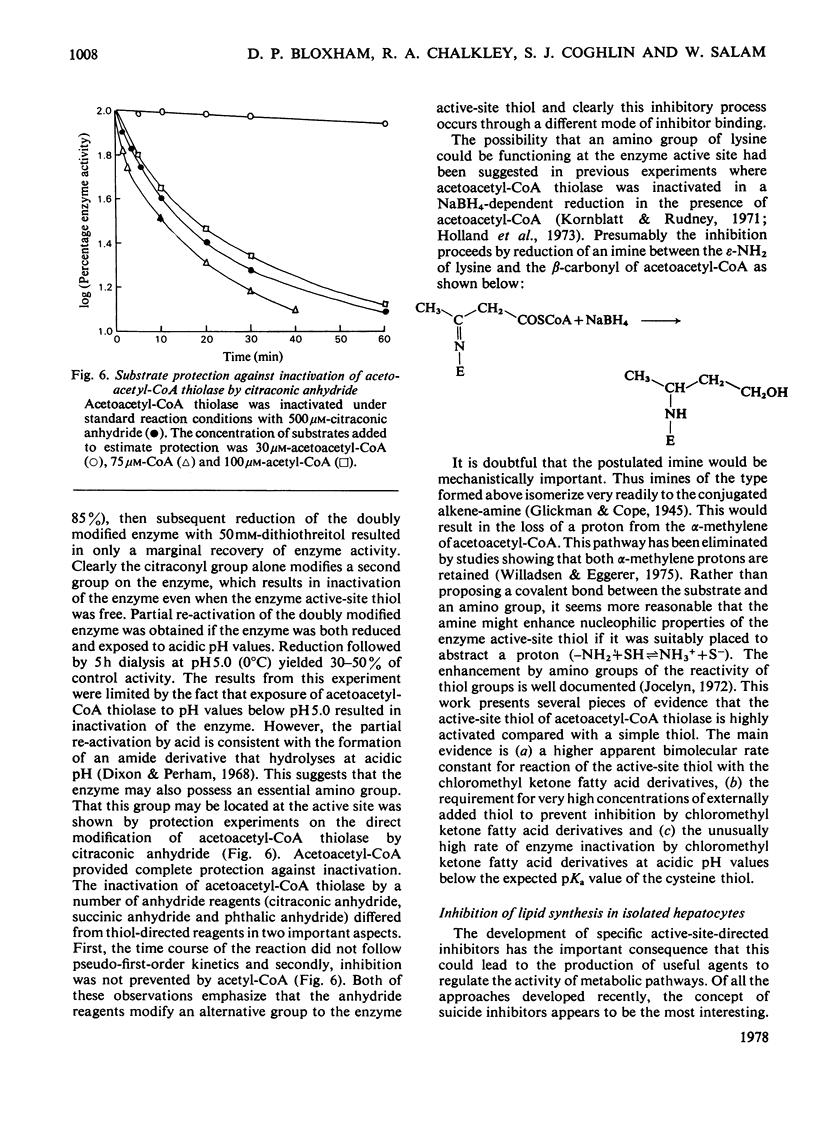
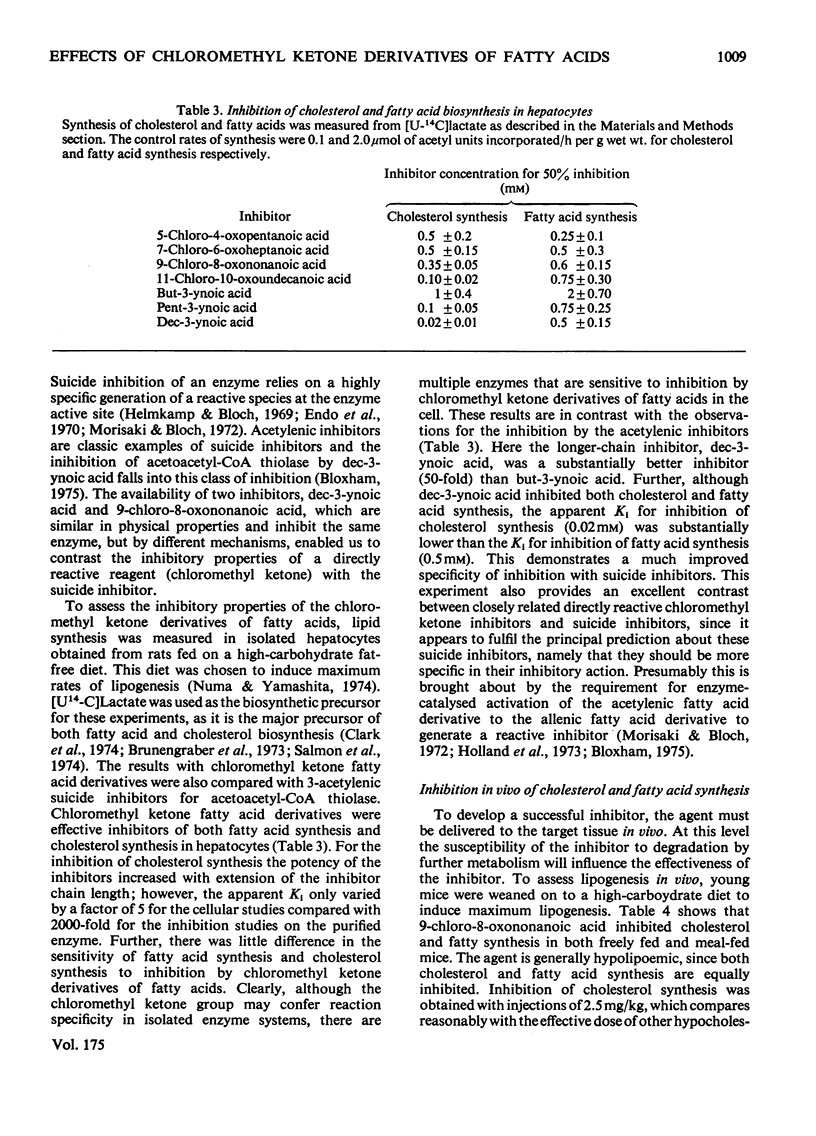
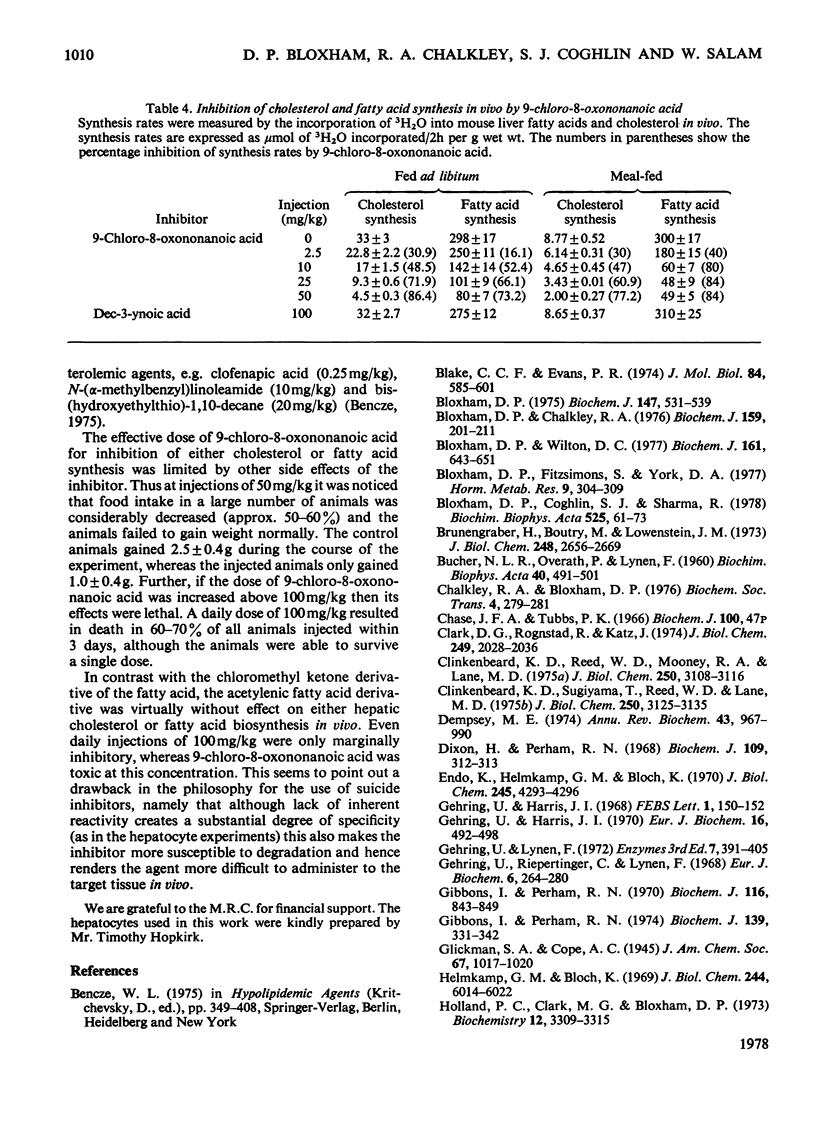
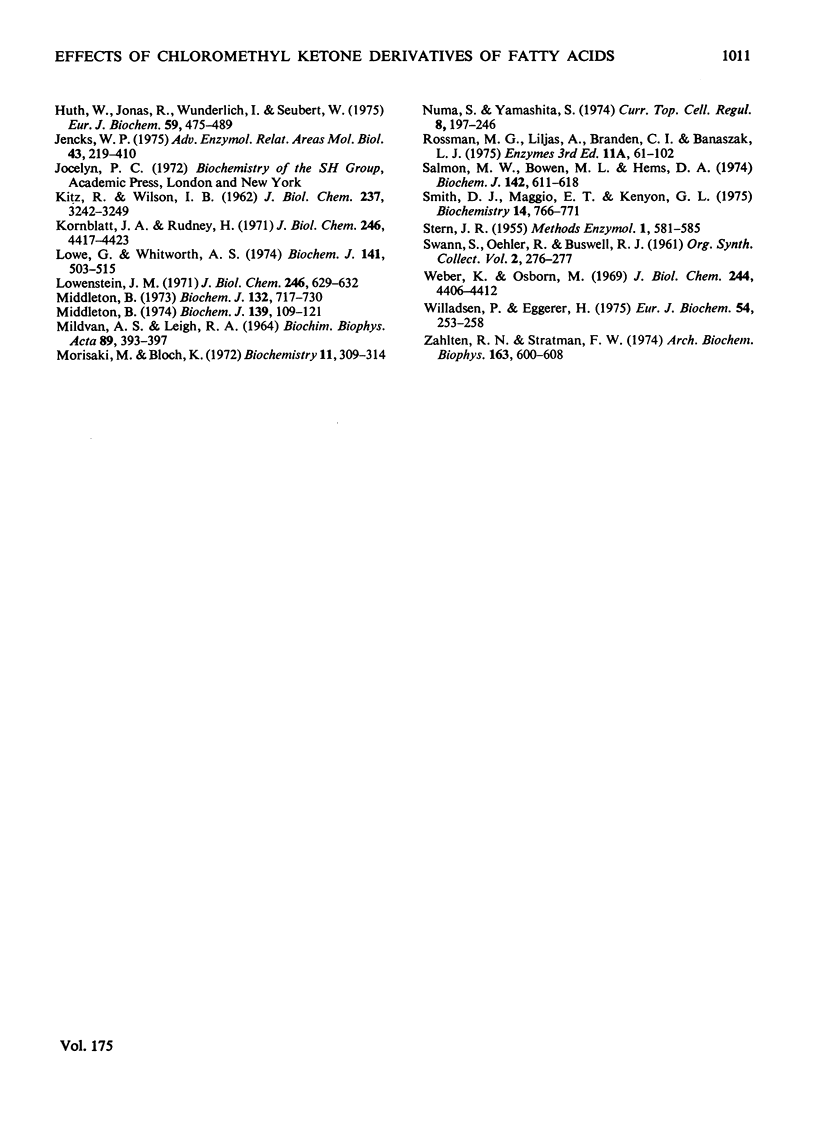
Selected References
These references are in PubMed. This may not be the complete list of references from this article.
- BUCHER N. L., OVERATH P., LYNEN F. beta-Hydroxy-beta-methyl-glutaryl coenzyme A reductase, cleavage and condensing enzymes in relation to cholesterol formation in rat liver. Biochim Biophys Acta. 1960 Jun 3;40:491–501. doi: 10.1016/0006-3002(60)91390-1. [DOI] [PubMed] [Google Scholar]
- Blake C. C., Evans P. R. Structure of horse muscle phosphoglycerate kinase. Some results on the chain conformation, substrate binding and evolution of the molecule from a 3 angstrom Fourier map. J Mol Biol. 1974 Apr 25;84(4):585–601. doi: 10.1016/0022-2836(74)90118-1. [DOI] [PubMed] [Google Scholar]
- Bloxham D. P., Chalkley R. A. Selective modification of rabbit muscle pyruvate kinase by 5-chloro-4-oxopentanoic acid. Biochem J. 1976 Nov;159(2):201–211. doi: 10.1042/bj1590201. [DOI] [PMC free article] [PubMed] [Google Scholar]
- Bloxham D. P., Coghlin S. J., Sharma R. P. Use of methanethiolation to investigate the catalytic role of sulphydryl groups in rabbit skeletal muscle pyruvate kinase. Biochim Biophys Acta. 1978 Jul 7;525(1):61–73. doi: 10.1016/0005-2744(78)90200-0. [DOI] [PubMed] [Google Scholar]
- Bloxham D. P., Fitzsimons J. T., York D. A. Lipogenesis in hepatocytes of genetically obese rats. Horm Metab Res. 1977 Jul;9(4):304–309. doi: 10.1055/s-0028-1093518. [DOI] [PubMed] [Google Scholar]
- Bloxham D. P. Selective inhibition of cholesterol synthesis by cell-free preparations of rat liver by using inhibitors of cytoplasmic acetoacetyl-coenzyme A thiolase. Biochem J. 1975 Jun;147(3):531–539. doi: 10.1042/bj1470531. [DOI] [PMC free article] [PubMed] [Google Scholar]
- Bloxham D. P., Wilton D. C. Modification of pig heart lactate dehydrogenase with methyl methanethiosulphonate to produce an enzyme with altered catalytic activity. Biochem J. 1977 Mar 1;161(3):643–651. doi: 10.1042/bj1610643. [DOI] [PMC free article] [PubMed] [Google Scholar]
- Brunengraber H., Boutry M., Lowenstein J. M. Fatty acid and 3- -hydroxysterol synthesis in the perfused rat liver. Including measurements on the production of lactate, pyruvate, -hydroxy-butyrate, and acetoacetate by the fed liver. J Biol Chem. 1973 Apr 25;248(8):2656–2669. [PubMed] [Google Scholar]
- Chalkley R. A., Bloxham D. P. Improved alkylation of acetoacetyl-coenzyme A thiolase by extension of chain length in chloromethyl ketone fatty acids. Biochem Soc Trans. 1976;4(2):279–281. doi: 10.1042/bst0040279. [DOI] [PubMed] [Google Scholar]
- Clark D. G., Rognstad R., Katz J. Lipogenesis in rat hepatocytes. J Biol Chem. 1974 Apr 10;249(7):2028–2036. [PubMed] [Google Scholar]
- Clinkenbeard K. D., Reed W. D., Mooney R. A., Lane M. D. Intracellular localization of the 3-hydroxy-3-methylglutaryl coenzme A cycle enzymes in liver. Separate cytoplasmic and mitochondrial 3-hydroxy-3-methylglutaryl coenzyme A generating systems for cholesterogenesis and ketogenesis. J Biol Chem. 1975 Apr 25;250(8):3108–3116. [PubMed] [Google Scholar]
- Dempsey M. E. Regulation of steroid biosynthesis. Annu Rev Biochem. 1974;43(0):967–990. doi: 10.1146/annurev.bi.43.070174.004535. [DOI] [PubMed] [Google Scholar]
- Dixon H. B., Perham R. N. Reversible blocking of amino groups with citraconic anhydride. Biochem J. 1968 Sep;109(2):312–314. doi: 10.1042/bj1090312. [DOI] [PMC free article] [PubMed] [Google Scholar]
- Endo K., Helmkamp G. M., Jr, Bloch K. Mode of inhibition of beta-hydroxydecanoyl thioester dehydrase by 3-decynoyl-N-acetylcysteamine. J Biol Chem. 1970 Sep 10;245(17):4293–4296. [PubMed] [Google Scholar]
- Gehring U., Harris J. I. Amino acid sequence around the reactive cysteine residues in thiolase. FEBS Lett. 1968 Aug;1(3):150–152. doi: 10.1016/0014-5793(68)80044-4. [DOI] [PubMed] [Google Scholar]
- Gehring U., Harris J. I. The active site cysteines of thiolase. Eur J Biochem. 1970 Nov;16(3):492–498. doi: 10.1111/j.1432-1033.1970.tb01108.x. [DOI] [PubMed] [Google Scholar]
- Gehring U., Riepertinger C., Lynen F. Reinigung und Kristallisation der Thiolase, Untersuchungen zum Wirkungsmechanismus. Eur J Biochem. 1968 Nov;6(2):264–280. doi: 10.1111/j.1432-1033.1968.tb00446.x. [DOI] [PubMed] [Google Scholar]
- Gibbons I., Perham R. N. Kinetic and molecular properties of citraconyl-aldolase. The reversible denaturation and hybridization of the native and modified enzymes. Biochem J. 1974 May;139(2):331–342. doi: 10.1042/bj1390331. [DOI] [PMC free article] [PubMed] [Google Scholar]
- Gibbons I., Perham R. N. The reaction of aldolase with 2-methylmaleic anhydride. Biochem J. 1970 Mar;116(5):843–849. doi: 10.1042/bj1160843. [DOI] [PMC free article] [PubMed] [Google Scholar]
- Helmkamp G. M., Jr, Bloch K. Beta-hydroxydecanoyl thioester dehydrase. Studies on molecular structure and active site. J Biol Chem. 1969 Nov 10;244(21):6014–6022. [PubMed] [Google Scholar]
- Holland P. C., Clark M. G., Bloxham D. P. Inactivation of pig heart thiolase by 3-butynoyl coenzyme A, 3-pentynoyl coenzyme A, and 4-bromocrotonyl coenzyme A. Biochemistry. 1973 Aug 14;12(17):3309–3315. doi: 10.1021/bi00741a024. [DOI] [PubMed] [Google Scholar]
- Huth W., Jonas R., Wunderlich I., Seubert W. On the mechanism of ketogenesis and its control. Purification, kinetic mechanism and regulation of different forms of mitochondrial acetoacetyl-CoA thiolases from ox liver. Eur J Biochem. 1975 Nov 15;59(2):475–489. doi: 10.1111/j.1432-1033.1975.tb02476.x. [DOI] [PubMed] [Google Scholar]
- Jencks W. P. Binding energy, specificity, and enzymic catalysis: the circe effect. Adv Enzymol Relat Areas Mol Biol. 1975;43:219–410. doi: 10.1002/9780470122884.ch4. [DOI] [PubMed] [Google Scholar]
- KITZ R., WILSON I. B. Esters of methanesulfonic acid as irreversible inhibitors of acetylcholinesterase. J Biol Chem. 1962 Oct;237:3245–3249. [PubMed] [Google Scholar]
- Kornblatt J. A., Rudney H. Two forms of acetoacetyl coenzyme A thiolase in yeast. I. Separation and properties. J Biol Chem. 1971 Jul 25;246(14):4417–4423. [PubMed] [Google Scholar]
- Lowe G., Whitworth A. S. A kinetic and fluorimetric investigation of papain modified at tryptophan-69 and -177 by N-bromosuccinimide. Biochem J. 1974 Aug;141(2):503–515. doi: 10.1042/bj1410503. [DOI] [PMC free article] [PubMed] [Google Scholar]
- Lowenstein J. M. Effect of (-)-hydroxycitrate on fatty acid synthesis by rat liver in vivo. J Biol Chem. 1971 Feb 10;246(3):629–632. [PubMed] [Google Scholar]
- MILDVAN A. S., LEIGH R. A. DETERNATION OF CO-FACTOR DISSOCIATION CONSTANTS FROM THE KINETICS OF INHIBITION OF ENZYMES. Biochim Biophys Acta. 1964 Sep 18;89:393–397. doi: 10.1016/0926-6569(64)90065-3. [DOI] [PubMed] [Google Scholar]
- Middleton B. The kinetic mechanism and properties of the cytoplasmic acetoacetyl-coenzyme A thiolase from rat liver. Biochem J. 1974 Apr;139(1):109–121. doi: 10.1042/bj1390109. [DOI] [PMC free article] [PubMed] [Google Scholar]
- Middleton B. The oxoacyl-coenzyme A thiolases of animal tissues. Biochem J. 1973 Apr;132(4):717–730. doi: 10.1042/bj1320717. [DOI] [PMC free article] [PubMed] [Google Scholar]
- Morisaki M., Bloch K. On the mode of interaction of -hydroxydecanoyl thioester dehydrase with allenic acid derivatives. Biochemistry. 1972 Feb 1;11(3):309–314. doi: 10.1021/bi00753a001. [DOI] [PubMed] [Google Scholar]
- Numa S., Yamashita S. Regulation of lipogenesis in animal tissues. Curr Top Cell Regul. 1974;8(0):197–246. doi: 10.1016/b978-0-12-152808-9.50012-2. [DOI] [PubMed] [Google Scholar]
- Salmon D. M., Bowen N. L., Hems D. A. Synthesis of fatty acids in the perused mouse liver. Biochem J. 1974 Sep;142(3):611–618. doi: 10.1042/bj1420611. [DOI] [PMC free article] [PubMed] [Google Scholar]
- Smith D. J., Maggio E. T., Kenyon G. L. Simple alkanethiol groups for temporary blocking of sulfhydryl groups of enzymes. Biochemistry. 1975 Feb 25;14(4):766–771. doi: 10.1021/bi00675a019. [DOI] [PubMed] [Google Scholar]
- Weber K., Osborn M. The reliability of molecular weight determinations by dodecyl sulfate-polyacrylamide gel electrophoresis. J Biol Chem. 1969 Aug 25;244(16):4406–4412. [PubMed] [Google Scholar]
- Wheather D. W., Snow G. A. Assay of the mycobactins by measurement of the growth of Mycobacterium johnei. Biochem J. 1966 Jul;100(1):47–49. doi: 10.1042/bj1000047. [DOI] [PMC free article] [PubMed] [Google Scholar]
- Willadsen P., Eggerer H. Substrate stereochemistry of the acetyl-CoA acetyltransferase reaction. Eur J Biochem. 1975 May;54(1):253–258. doi: 10.1111/j.1432-1033.1975.tb04135.x. [DOI] [PubMed] [Google Scholar]
- Zahlten R. N., Stratman F. W. The isolation of hormone-sensitive rat hepatocytes by a modified enzymatic technique. Arch Biochem Biophys. 1974 Aug;163(2):600–608. doi: 10.1016/0003-9861(74)90519-0. [DOI] [PubMed] [Google Scholar]