Abstract
1. The effects of glucose, pyruvate and lactate on basal metabolism and on contraction-related energy expenditure of thin trabeculae isolated from guinea-pig heart were studied using a microcalorimetric technique. 2. Resting heat rates of cardiac ventricular muscle measured in the presence of substrate-free solution (56 +/- 20 mW (g dry weight)-1), 10 mM-lactate (54 +/- 12 mW (g dry weight)-1) and 10 mM-glucose (63 +/- 24 mW (g dry weight)-1) did not differ significantly. Increasing the external glucose concentration (up to 100 mM) and/or adding insulin (up to 80 units l-1) had virtually no effect on the measured resting heat rate. 3. With 10 mM-pyruvate as substrate resting heat rate was substantially larger (106 +/- 40 mW (g dry weight)-1) than with glucose, lactate or substrate-free solution. The concentrations of pyruvate producing a half-maximal increase in resting heat rate as compared to substrate-free solution ranged between 0.4 and 1.2 mM. 4. In order to test whether the development of an anoxic core contributed to the substrate dependence of resting heat production the critical PO2 (i.e. the PO2 that produced a just-noticeable decrease in heat rate) was determined in cylindrical preparations of various diameters. It was found that none of the preparations had an anoxic core at rest in a solution equilibrated with 100% oxygen. 5. From the dependence of the critical PO2 on the diameter of the preparation the diffusion coefficient of oxygen through cardiac muscle was calculated using a modification of Hill's equation (Hill, 1928). The O2 diffusion coefficient was found to be 1.09 X 10(-5) cm2 s-1. 6. Contraction-related heat production was also found to be dependent on the substrate used. In the presence of 10 mM-pyruvate it was about 60% larger than in the presence of 10 mM-glucose, 10 mM-lactate or with substrate-free solution. 7. Isometric force of contraction showed the same substrate dependence as contraction-related heat production and increased with a similar time course during repetitive stimulation. 8. The possible mechanisms underlying the substrate dependence of myocardial energy metabolism are discussed. It is suggested that the increased energy expenditure observed in the presence of pyruvate may be related to a decrease in intracellular phosphate and/or to an increase in intracellular pH.
Full text
PDF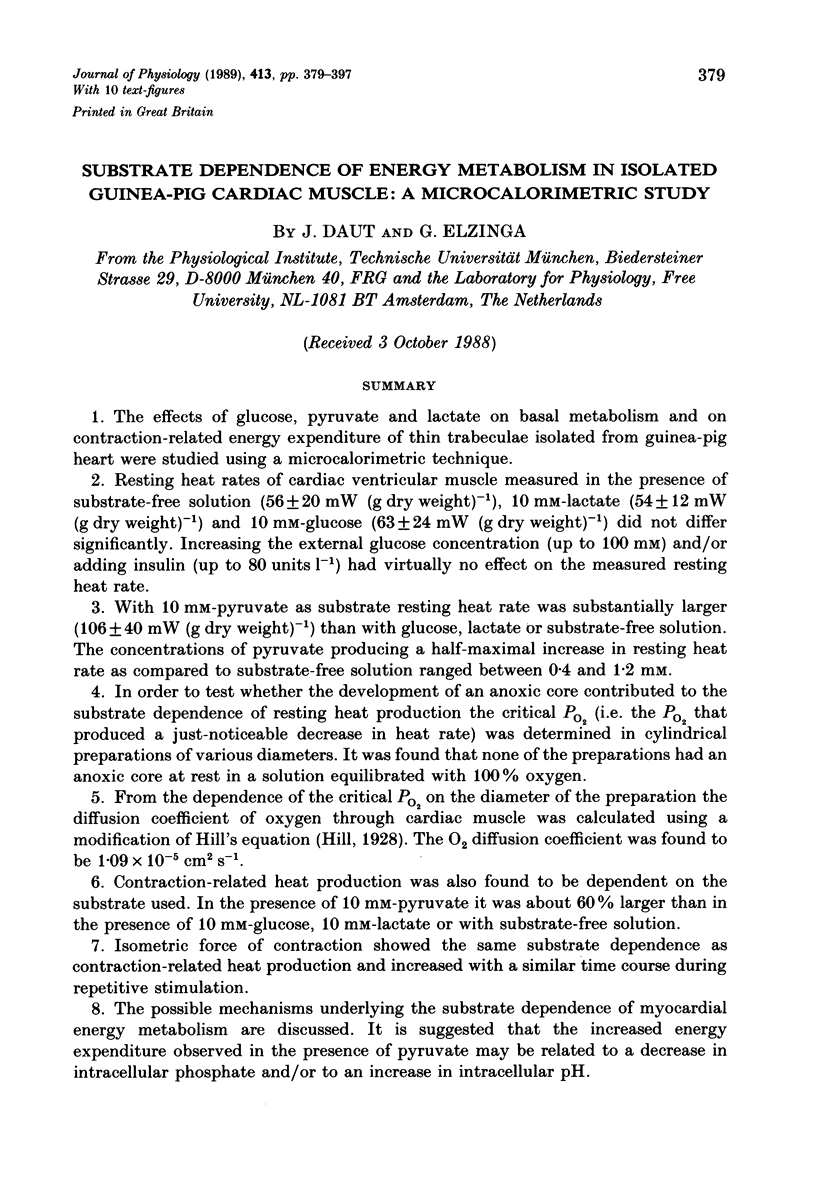
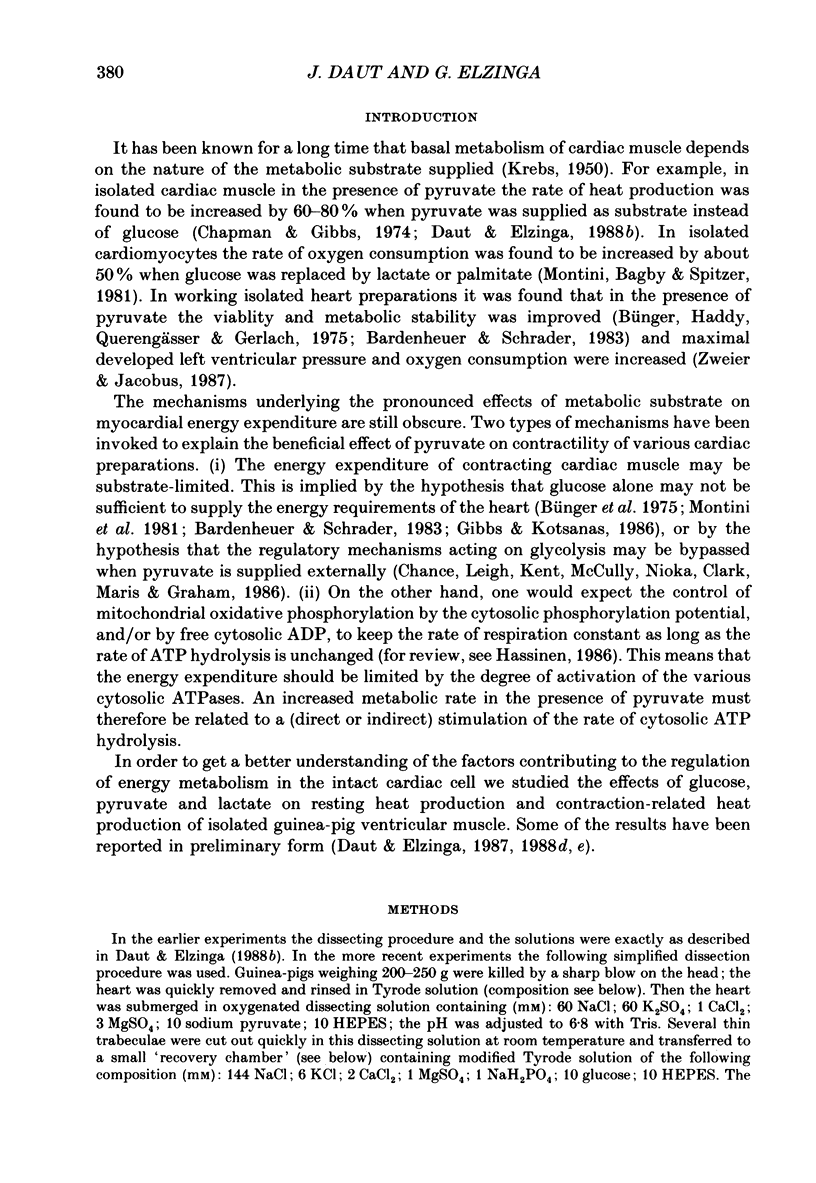
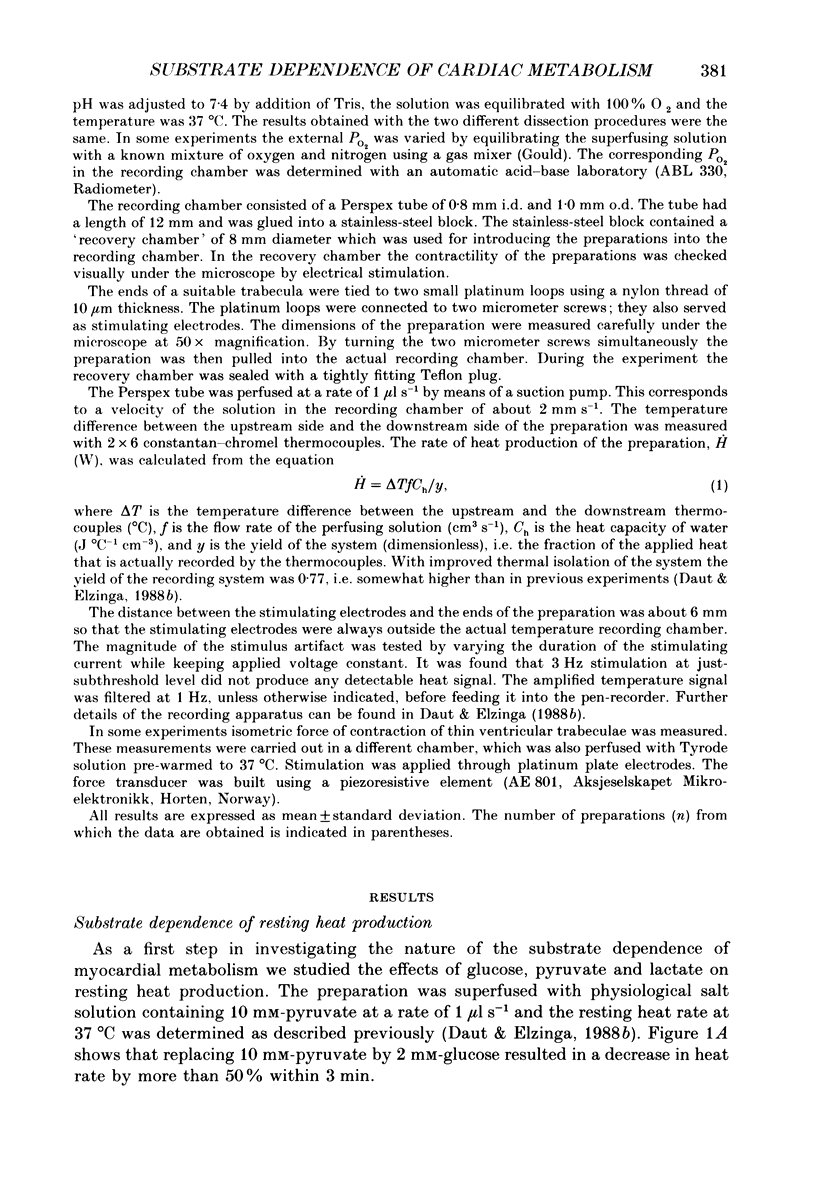
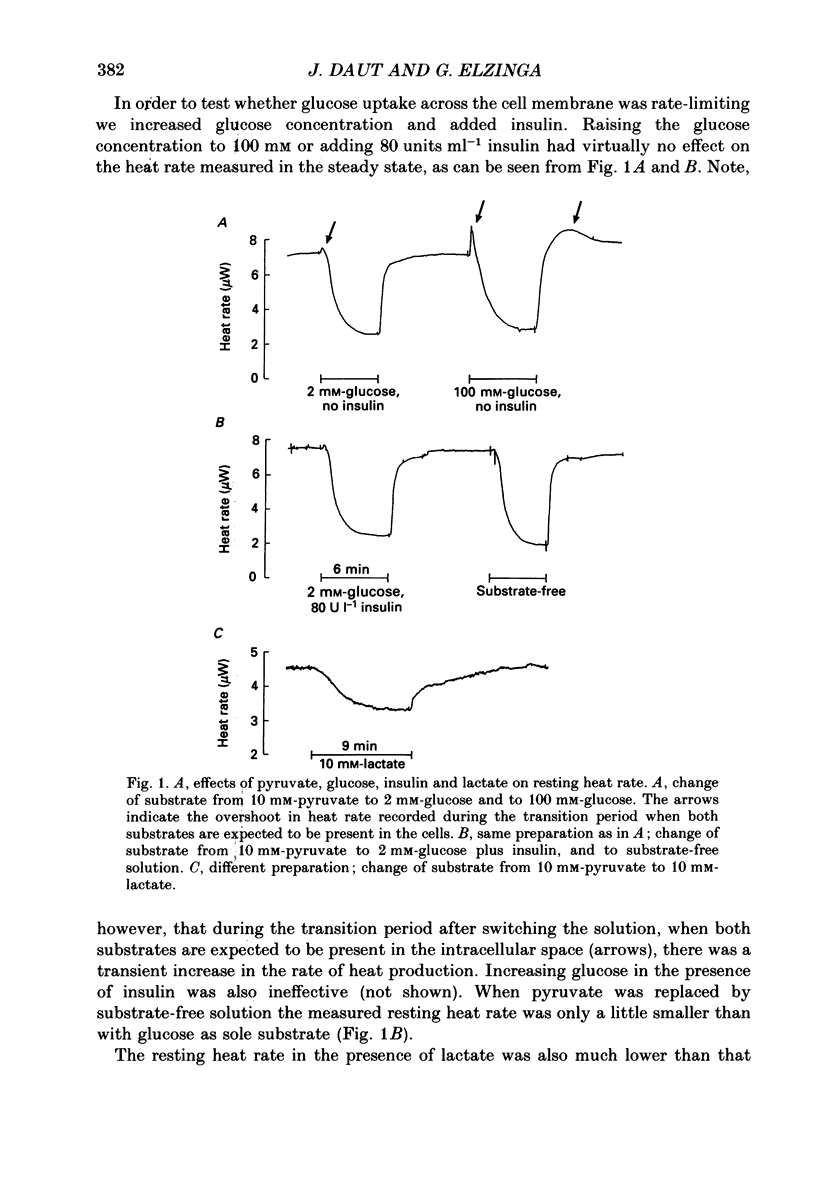
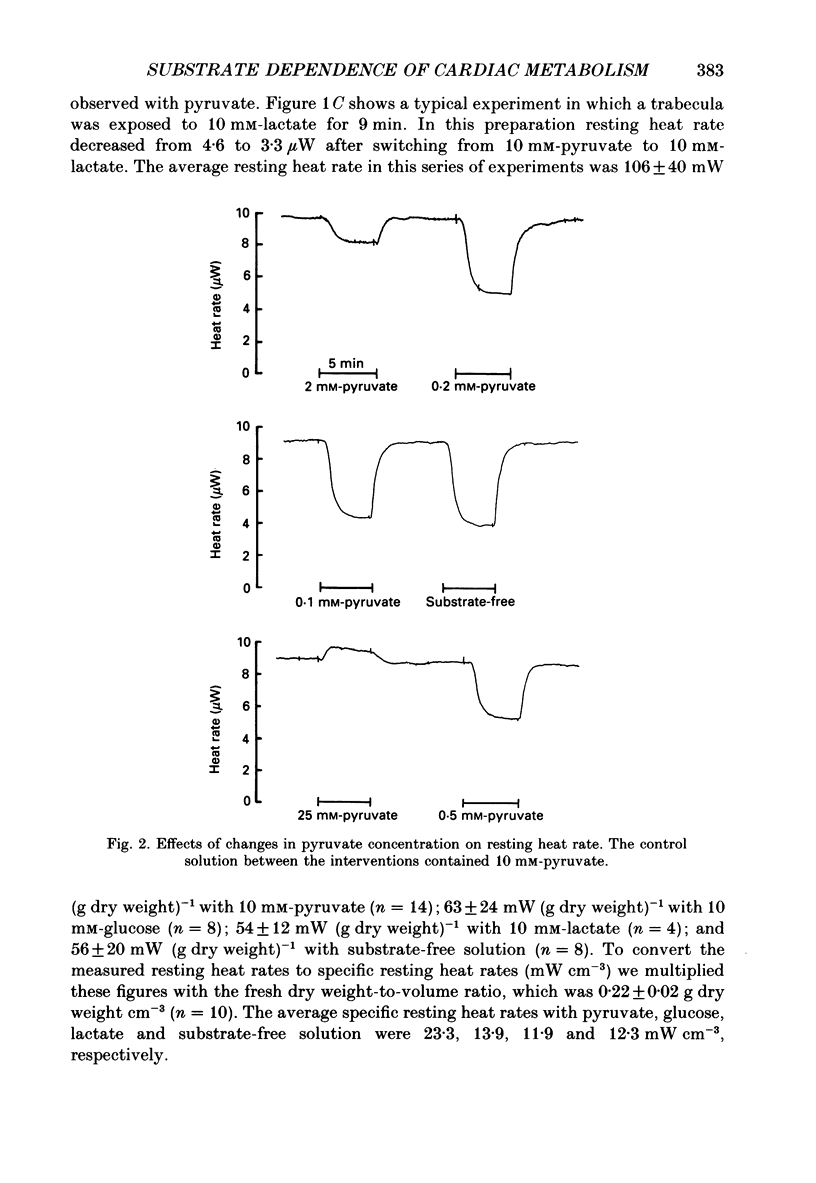
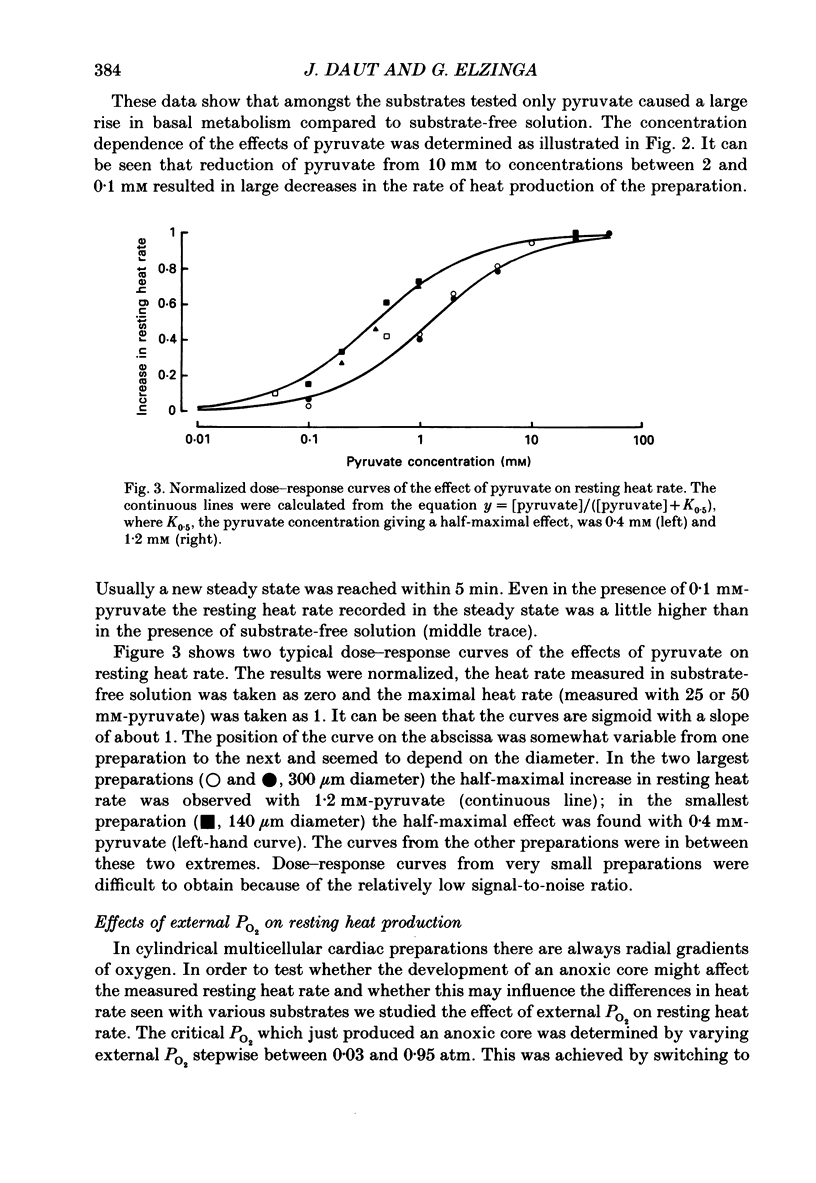
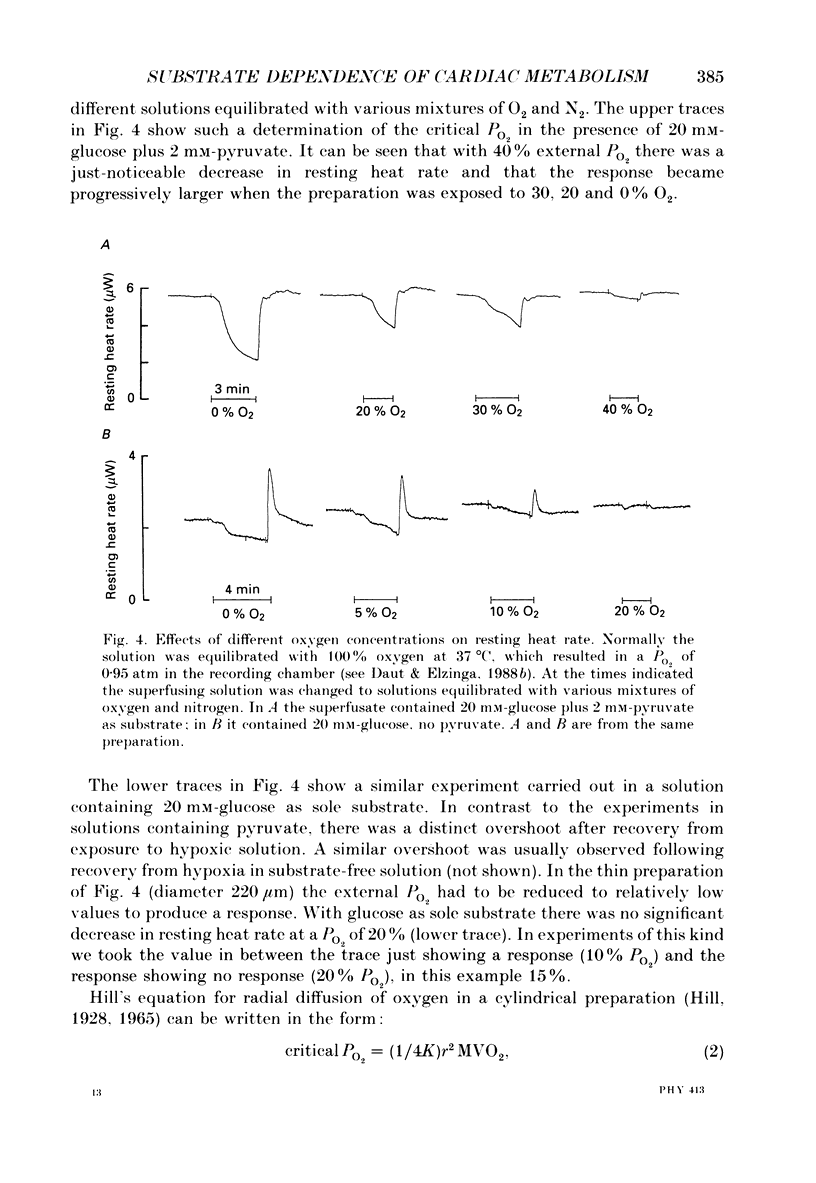
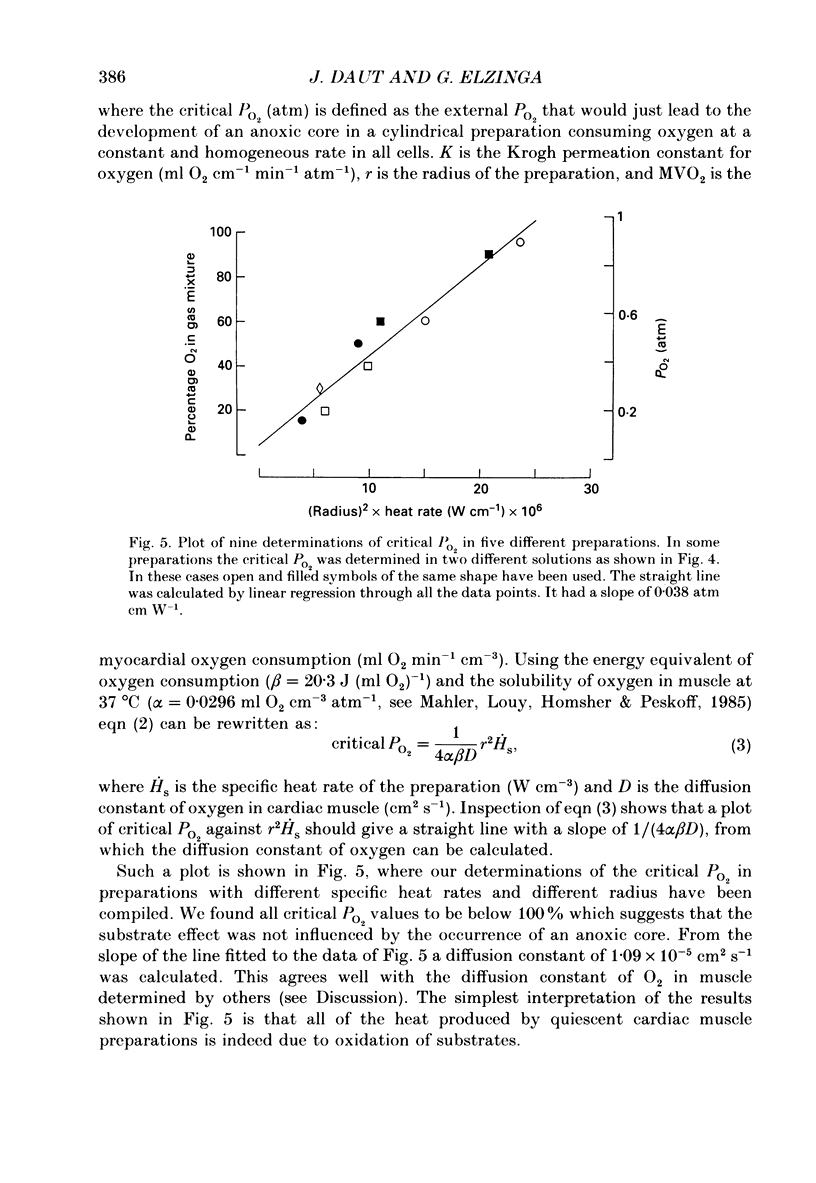
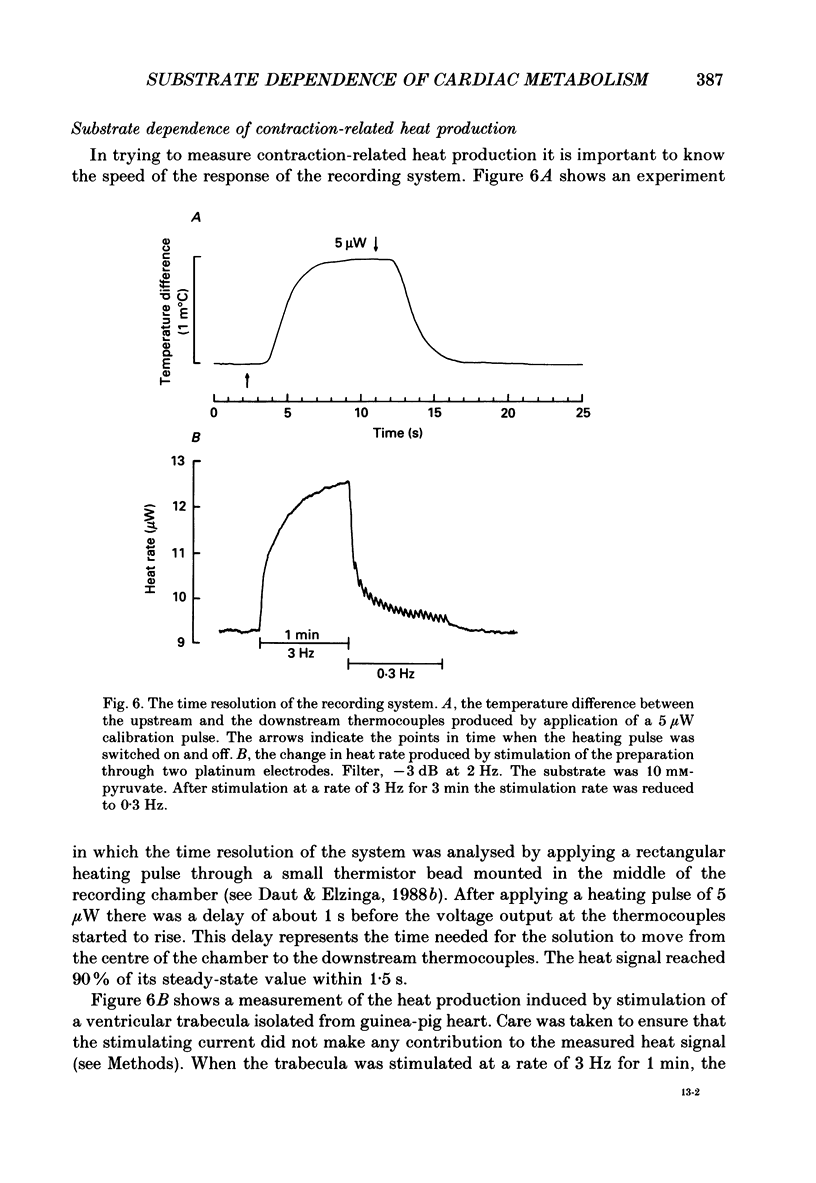
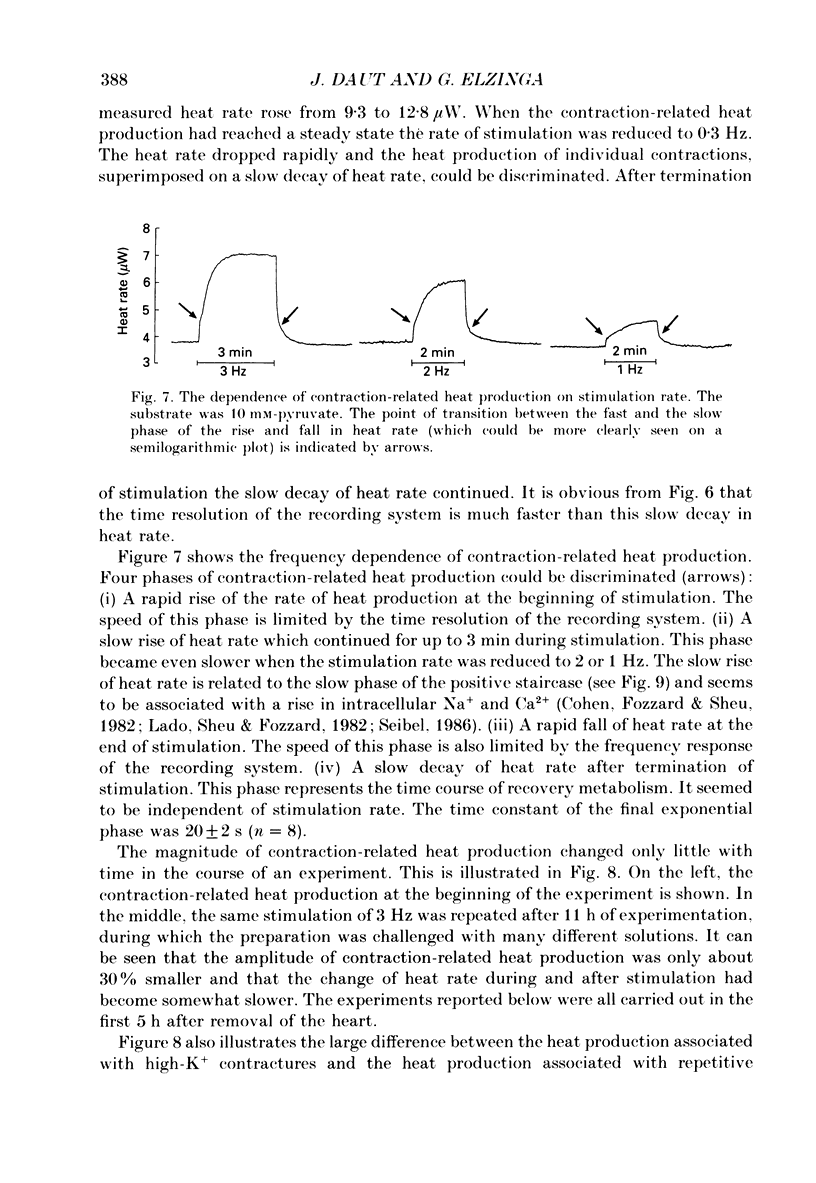
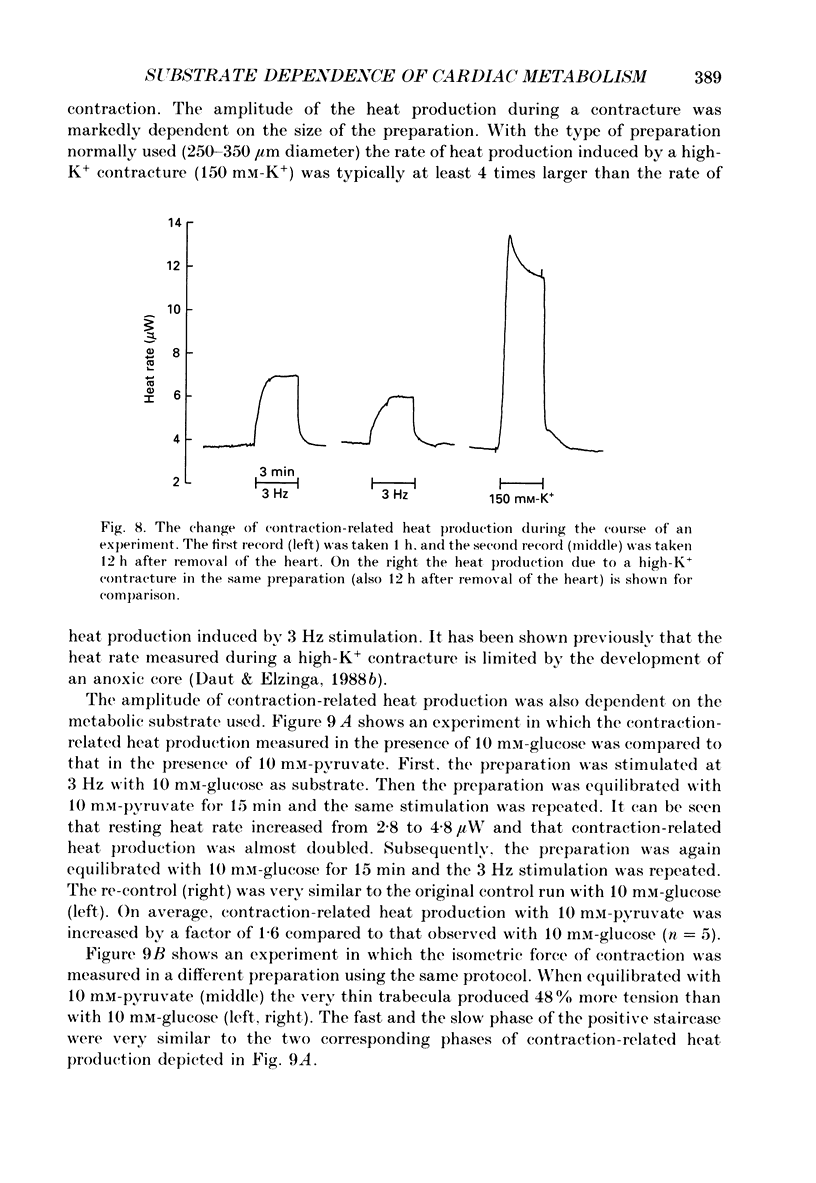
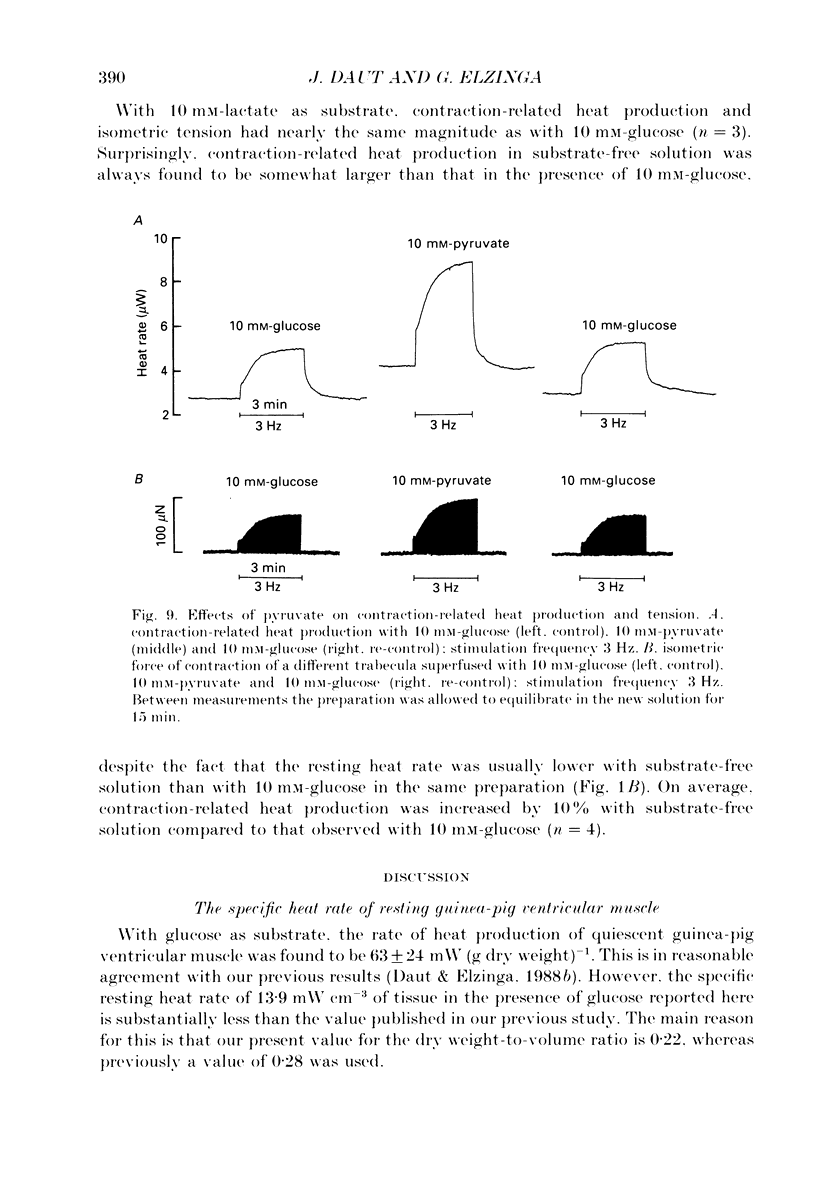
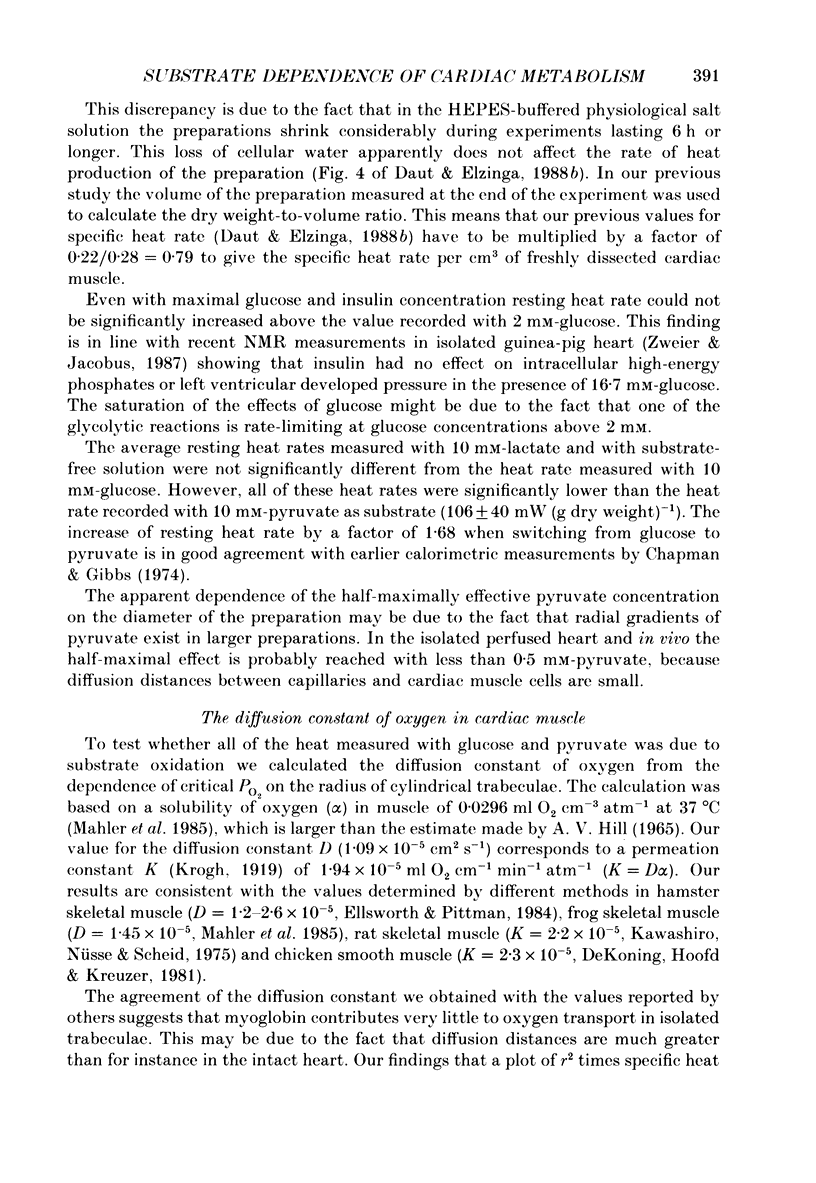
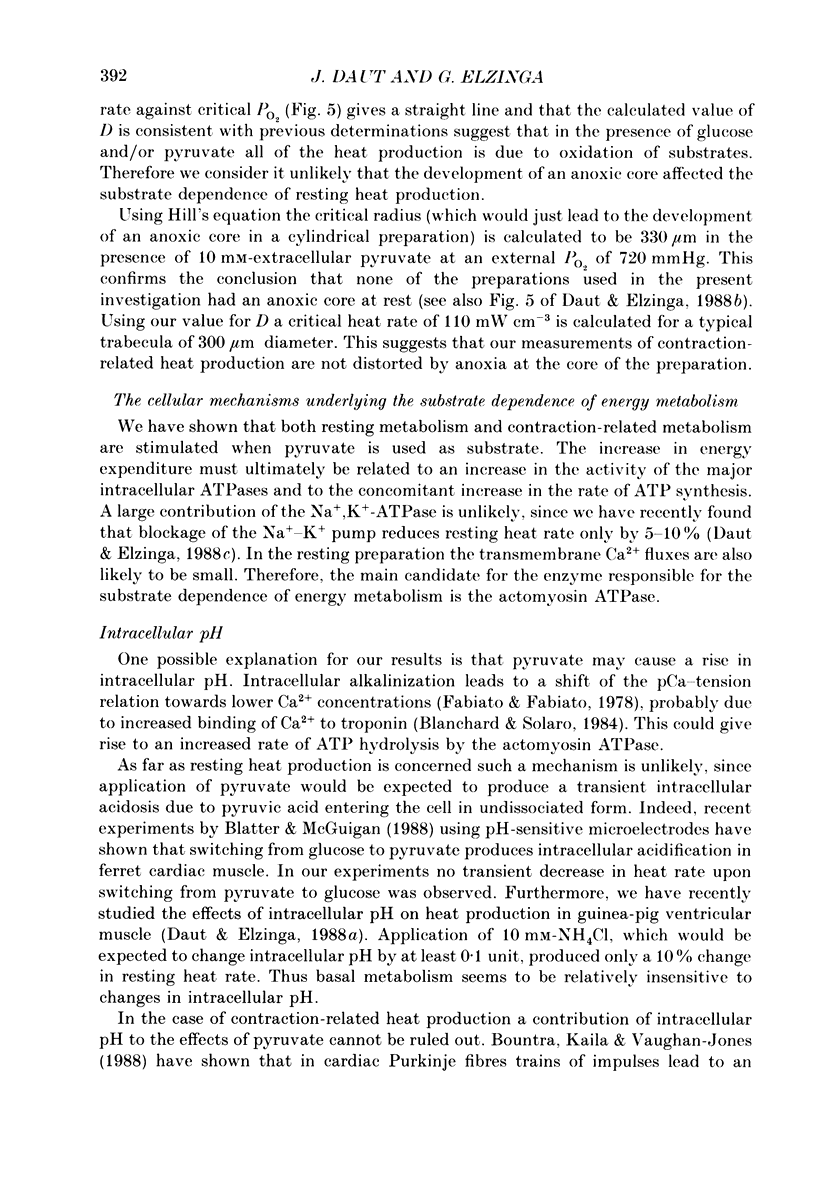
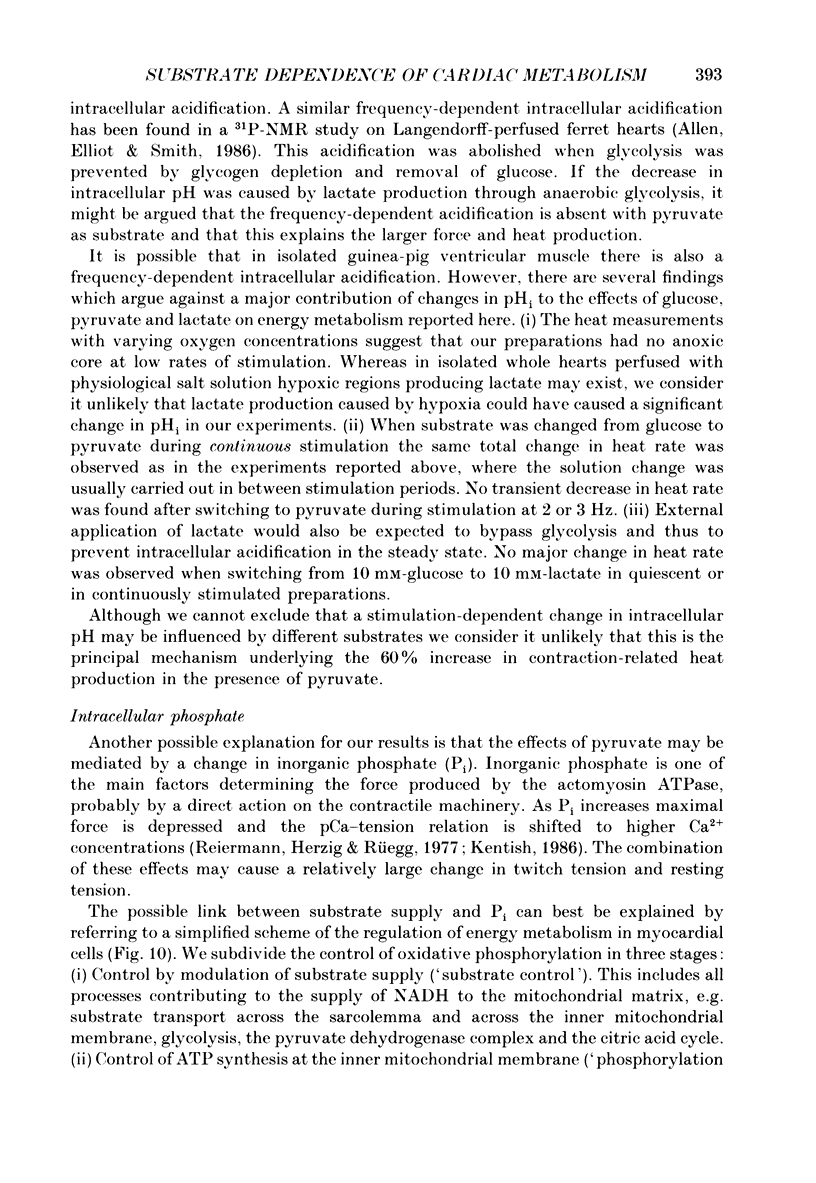
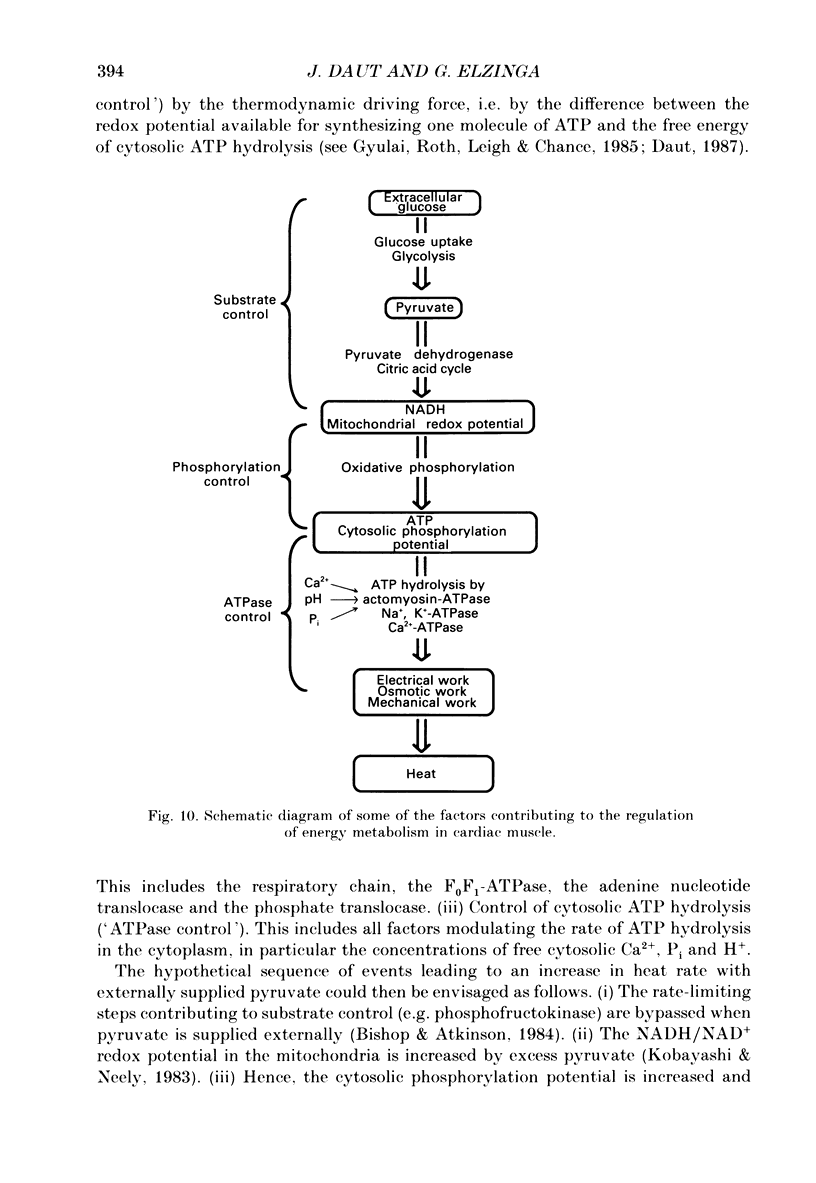
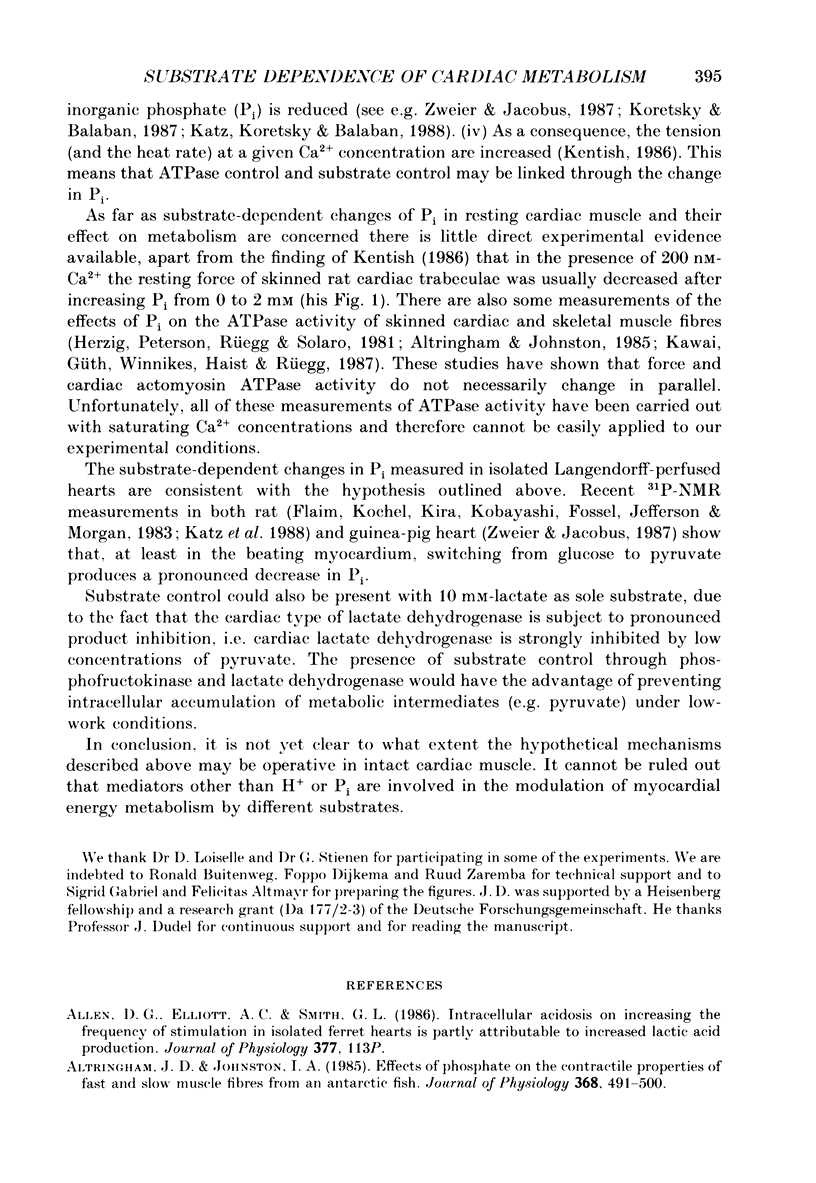
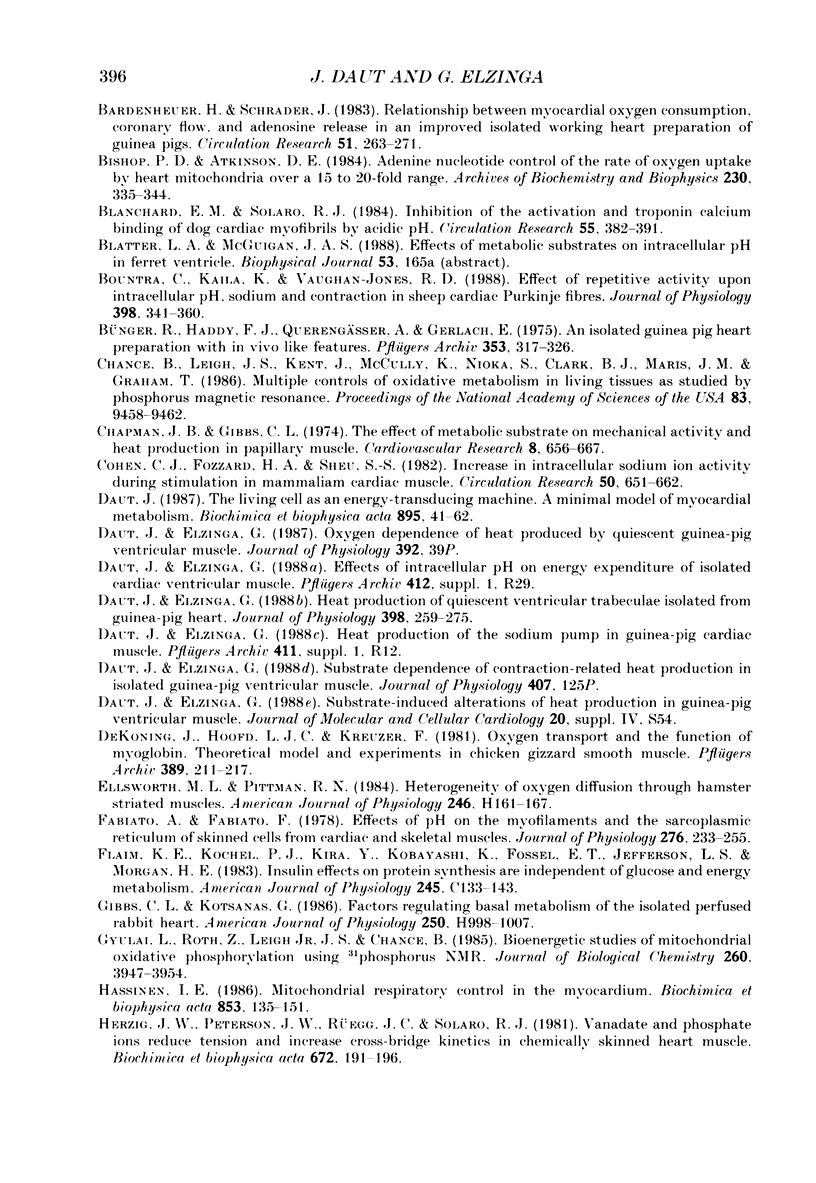
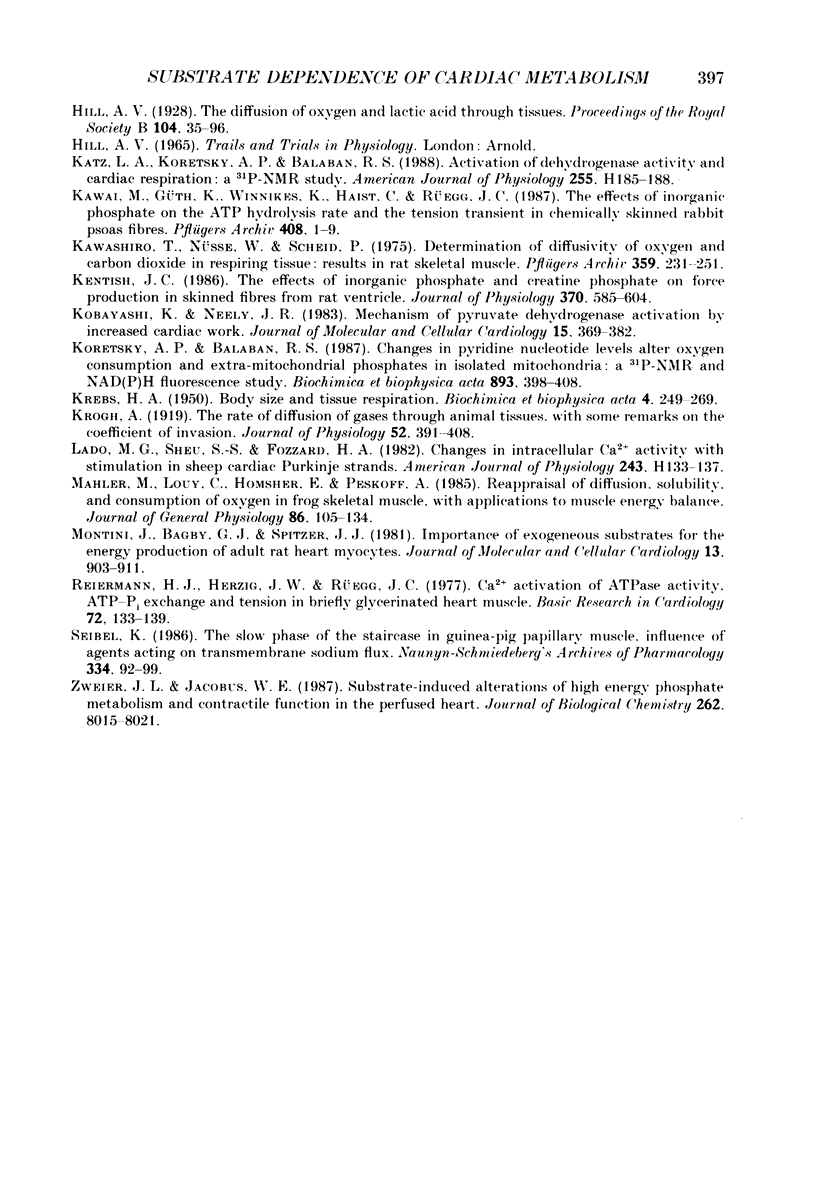
Selected References
These references are in PubMed. This may not be the complete list of references from this article.
- Bardenheuer H., Schrader J. Relationship between myocardial oxygen consumption, coronary flow, and adenosine release in an improved isolated working heart preparation of guinea pigs. Circ Res. 1983 Mar;52(3):263–271. doi: 10.1161/01.res.52.3.263. [DOI] [PubMed] [Google Scholar]
- Bishop P. D., Atkinson D. E. Adenine nucleotide control of the rate of oxygen uptake by rat heart mitochondria over a 15- to 20-fold range. Arch Biochem Biophys. 1984 Apr;230(1):335–344. doi: 10.1016/0003-9861(84)90116-4. [DOI] [PubMed] [Google Scholar]
- Blanchard E. M., Solaro R. J. Inhibition of the activation and troponin calcium binding of dog cardiac myofibrils by acidic pH. Circ Res. 1984 Sep;55(3):382–391. doi: 10.1161/01.res.55.3.382. [DOI] [PubMed] [Google Scholar]
- Bountra C., Kaila K., Vaughan-Jones R. D. Effect of repetitive activity upon intracellular pH, sodium and contraction in sheep cardiac Purkinje fibres. J Physiol. 1988 Apr;398:341–360. doi: 10.1113/jphysiol.1988.sp017046. [DOI] [PMC free article] [PubMed] [Google Scholar]
- Bünger R., Haddy F. J., Querengässer A., Gerlach E. An isolated guinea pig heart preparation with in vivo like features. Pflugers Arch. 1975;353(4):317–326. doi: 10.1007/BF00587028. [DOI] [PubMed] [Google Scholar]
- Cohen C. J., Fozzard H. A., Sheu S. S. Increase in intracellular sodium ion activity during stimulation in mammalian cardiac muscle. Circ Res. 1982 May;50(5):651–662. doi: 10.1161/01.res.50.5.651. [DOI] [PubMed] [Google Scholar]
- Daut J., Elzinga G. Heat production of quiescent ventricular trabeculae isolated from guinea-pig heart. J Physiol. 1988 Apr;398:259–275. doi: 10.1113/jphysiol.1988.sp017041. [DOI] [PMC free article] [PubMed] [Google Scholar]
- Daut J. The living cell as an energy-transducing machine. A minimal model of myocardial metabolism. Biochim Biophys Acta. 1987;895(1):41–62. doi: 10.1016/s0304-4173(87)80016-2. [DOI] [PubMed] [Google Scholar]
- Fabiato A., Fabiato F. Effects of pH on the myofilaments and the sarcoplasmic reticulum of skinned cells from cardiace and skeletal muscles. J Physiol. 1978 Mar;276:233–255. doi: 10.1113/jphysiol.1978.sp012231. [DOI] [PMC free article] [PubMed] [Google Scholar]
- Flaim K. E., Kochel P. J., Kira Y., Kobayashi K., Fossel E. T., Jefferson L. S., Morgan H. E. Insulin effects on protein synthesis are independent of glucose and energy metabolism. Am J Physiol. 1983 Jul;245(1):C133–C143. doi: 10.1152/ajpcell.1983.245.1.C133. [DOI] [PubMed] [Google Scholar]
- Gibbs C. L., Kotsanas G. Factors regulating basal metabolism of the isolated perfused rabbit heart. Am J Physiol. 1986 Jun;250(6 Pt 2):H998–1007. doi: 10.1152/ajpheart.1986.250.6.H998. [DOI] [PubMed] [Google Scholar]
- Gyulai L., Roth Z., Leigh J. S., Jr, Chance B. Bioenergetic studies of mitochondrial oxidative phosphorylation using 31phosphorus NMR. J Biol Chem. 1985 Apr 10;260(7):3947–3954. [PubMed] [Google Scholar]
- Hassinen I. E. Mitochondrial respiratory control in the myocardium. Biochim Biophys Acta. 1986;853(2):135–151. doi: 10.1016/0304-4173(86)90008-x. [DOI] [PubMed] [Google Scholar]
- Herzig J. W., Peterson J. W., Rüegg J. C., Solaro R. J. Vanadate and phosphate ions reduce tension and increase cross-bridge kinetics in chemically skinned heart muscle. Biochim Biophys Acta. 1981 Jan 21;672(2):191–196. doi: 10.1016/0304-4165(81)90392-5. [DOI] [PubMed] [Google Scholar]
- KREBS H. A. Body size and tissue respiration. Biochim Biophys Acta. 1950 Jan;4(1-3):249–269. doi: 10.1016/0006-3002(50)90032-1. [DOI] [PubMed] [Google Scholar]
- Katz L. A., Koretsky A. P., Balaban R. S. Activation of dehydrogenase activity and cardiac respiration: a 31P-NMR study. Am J Physiol. 1988 Jul;255(1 Pt 2):H185–H188. doi: 10.1152/ajpheart.1988.255.1.H185. [DOI] [PubMed] [Google Scholar]
- Kawashiro T., Nüsse W., Scheid P. Determination of diffusivity of oxygen and carbon dioxide in respiring tissue: results in rat skeletal muscle. Pflugers Arch. 1975 Sep 9;359(3):231–251. doi: 10.1007/BF00587382. [DOI] [PubMed] [Google Scholar]
- Kentish J. C. The effects of inorganic phosphate and creatine phosphate on force production in skinned muscles from rat ventricle. J Physiol. 1986 Jan;370:585–604. doi: 10.1113/jphysiol.1986.sp015952. [DOI] [PMC free article] [PubMed] [Google Scholar]
- Kobayashi K., Neely J. R. Mechanism of pyruvate dehydrogenase activation by increased cardiac work. J Mol Cell Cardiol. 1983 Jun;15(6):369–382. doi: 10.1016/0022-2828(83)90321-8. [DOI] [PubMed] [Google Scholar]
- Koretsky A. P., Balaban R. S. Changes in pyridine nucleotide levels alter oxygen consumption and extra-mitochondrial phosphates in isolated mitochondria: a 31P-NMR and NAD(P)H fluorescence study. Biochim Biophys Acta. 1987 Oct 7;893(3):398–408. doi: 10.1016/0005-2728(87)90092-2. [DOI] [PubMed] [Google Scholar]
- Krogh A. The rate of diffusion of gases through animal tissues, with some remarks on the coefficient of invasion. J Physiol. 1919 May 20;52(6):391–408. doi: 10.1113/jphysiol.1919.sp001838. [DOI] [PMC free article] [PubMed] [Google Scholar]
- Lado M. G., Sheu S. S., Fozzard H. A. Changes in intracellular Ca2+ activity with stimulation in sheep cardiac Purkinje strands. Am J Physiol. 1982 Jul;243(1):H133–H137. doi: 10.1152/ajpheart.1982.243.1.H133. [DOI] [PubMed] [Google Scholar]
- Mahler M., Louy C., Homsher E., Peskoff A. Reappraisal of diffusion, solubility, and consumption of oxygen in frog skeletal muscle, with applications to muscle energy balance. J Gen Physiol. 1985 Jul;86(1):105–134. doi: 10.1085/jgp.86.1.105. [DOI] [PMC free article] [PubMed] [Google Scholar]
- Montini J., Bagby G. J., Spitzer J. J. Importance of exogenous substrates for the energy production of adult rat heart myocytes. J Mol Cell Cardiol. 1981 Oct;13(10):903–911. doi: 10.1016/0022-2828(81)90289-3. [DOI] [PubMed] [Google Scholar]
- Reiermann H. J., Herzig J. W., Rüegg J. C. Ca++ activation of ATPase activity, ATP-Pi exchange, and tension in briefly glycerinated heart muscle. Basic Res Cardiol. 1977 Mar-Jun;72(2-3):133–139. doi: 10.1007/BF01906351. [DOI] [PubMed] [Google Scholar]
- Seibel K. The slow phase of the staircase in guinea-pig papillary muscle, influence of agents acting on transmembrane sodium flux. Naunyn Schmiedebergs Arch Pharmacol. 1986 Sep;334(1):92–99. doi: 10.1007/BF00498745. [DOI] [PubMed] [Google Scholar]
- Zweier J. L., Jacobus W. E. Substrate-induced alterations of high energy phosphate metabolism and contractile function in the perfused heart. J Biol Chem. 1987 Jun 15;262(17):8015–8021. [PubMed] [Google Scholar]
- de Koning J., Hoofd L. J., Kreuzer F. Oxygen transport and the function of myoglobin. Theoretical model and experiments in chicken gizzard smooth muscle. Pflugers Arch. 1981 Mar;389(3):211–217. doi: 10.1007/BF00584781. [DOI] [PubMed] [Google Scholar]