Abstract
1. Chondrocyte activities within growth plate cartilage are the principal determinants of longitudinal bone growth, and it was the aim of this investigation to assess how these cell activities are modulated under various growth rate conditions. Using proximal tibial growth plates from rats of different ages, growth rate was determined by fluorochrome labelling and incident light fluorescence microscopy. Various cellular parameters contributing to longitudinal bone growth were quantified by light microscopic stereology. The size of the proliferating cell population ('growth fraction') was estimated by autoradiography (using [3H]thymidine labelling). 2. A comparison between data for suckling (21-day-old) and fast-growing (35-day-old) rats revealed that growth acceleration is achieved almost exclusively by cell-shape modelling, namely by an increase in final cell height and a decrease in lateral diameter, whereas final cell volume and surface area are slightly reduced. Cell proliferation rate in the longitudinal direction and net matrix production per cell remain unchanged. The physiological increase in linear growth rate thus appears to be based principally upon a controlled structural modulation of the chondrocyte phenotype. On the other hand, a physiological reduction in growth rate (i.e. growth deceleration) effected during the transition from pre-puberty (35-day-old rats) to maturity (80-day-old rats) is achieved by simultaneous decreases in several chondrocyte parameters, including cell height (i.e. phenotype modulation), cell volume and proliferation rate (in the longitudinal direction). However, chondrocytes continue to produce matrix at a level comparable to that attained during the period characterized by high growth rates (i.e. at 21 and 35 days). Cartilage matrix thus appears to play a subordinate role in regulating longitudinal bone growth rate. The duration of the hypertrophic cell activity (i.e. phenotype modulation) phase remains constant (at approximately 2 days) under the various growth rate conditions. 3. The findings presented in this study indicate that measurement of bulk parameters such as [35S]sulphate incorporation into matrix components, [3H]thymidine uptake by cells and growth plate height are of limited value as estimators of longitudinal bone growth, since changes in the parameters that these measurements quantify bear little relationship to changes in linear growth rate, and may be useful only as indicators of total growth plate activity.
Full text
PDF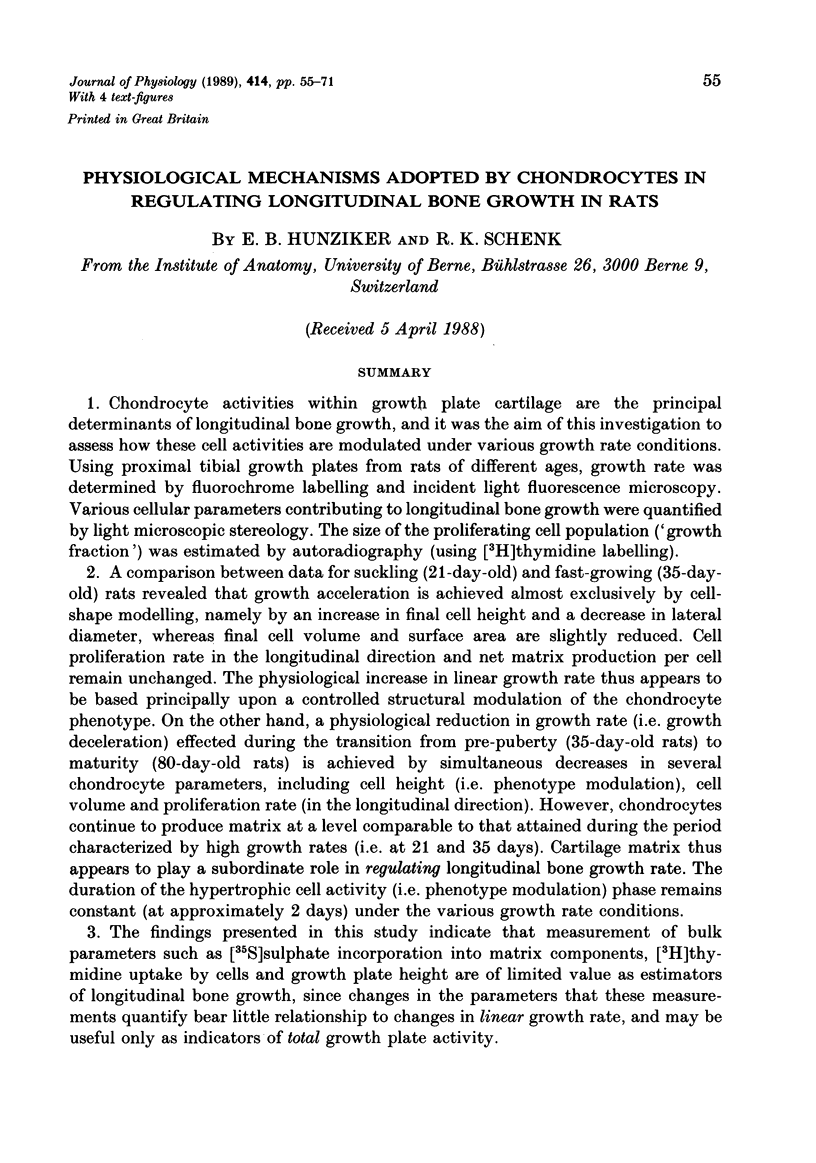
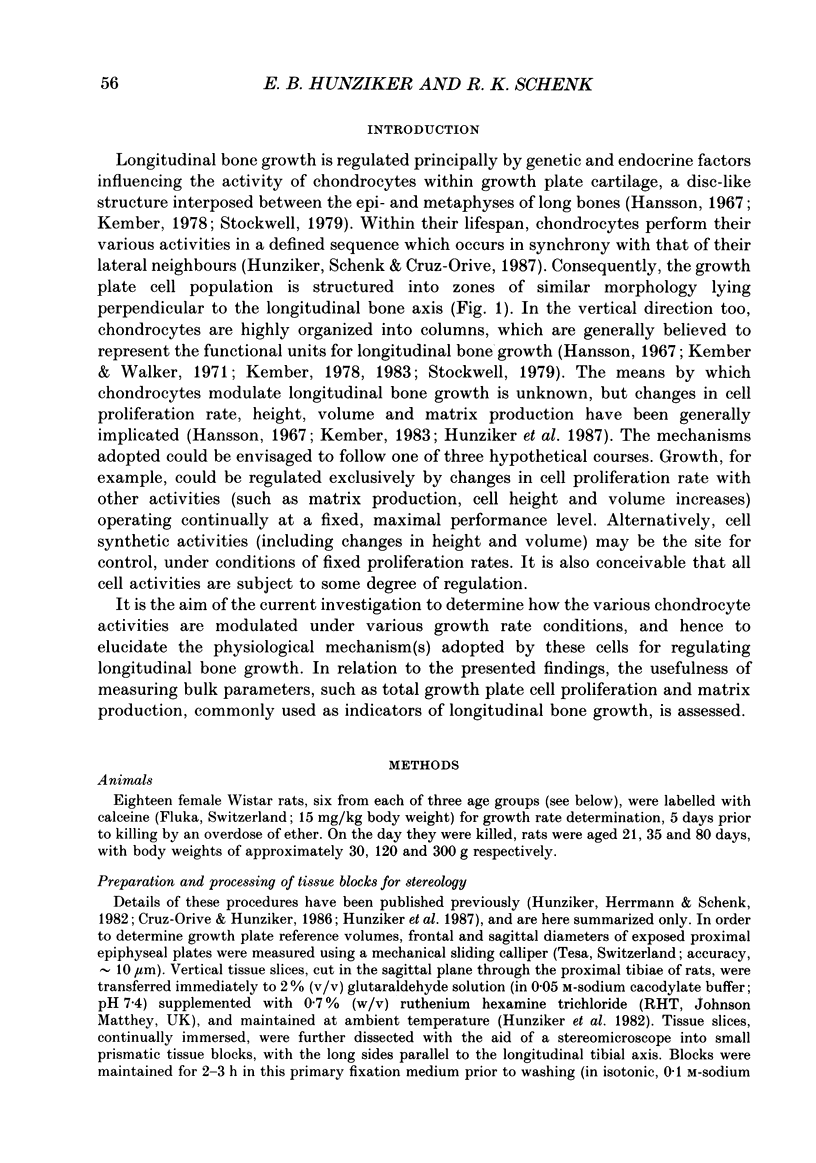
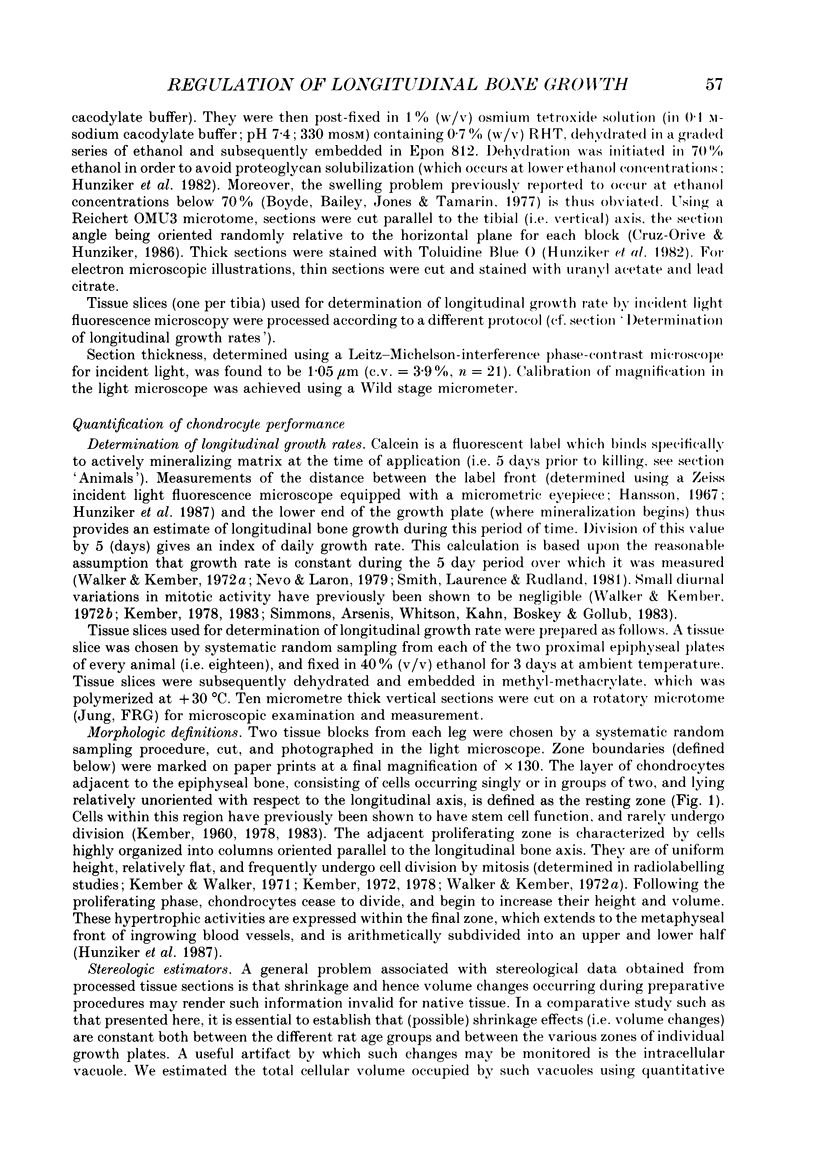
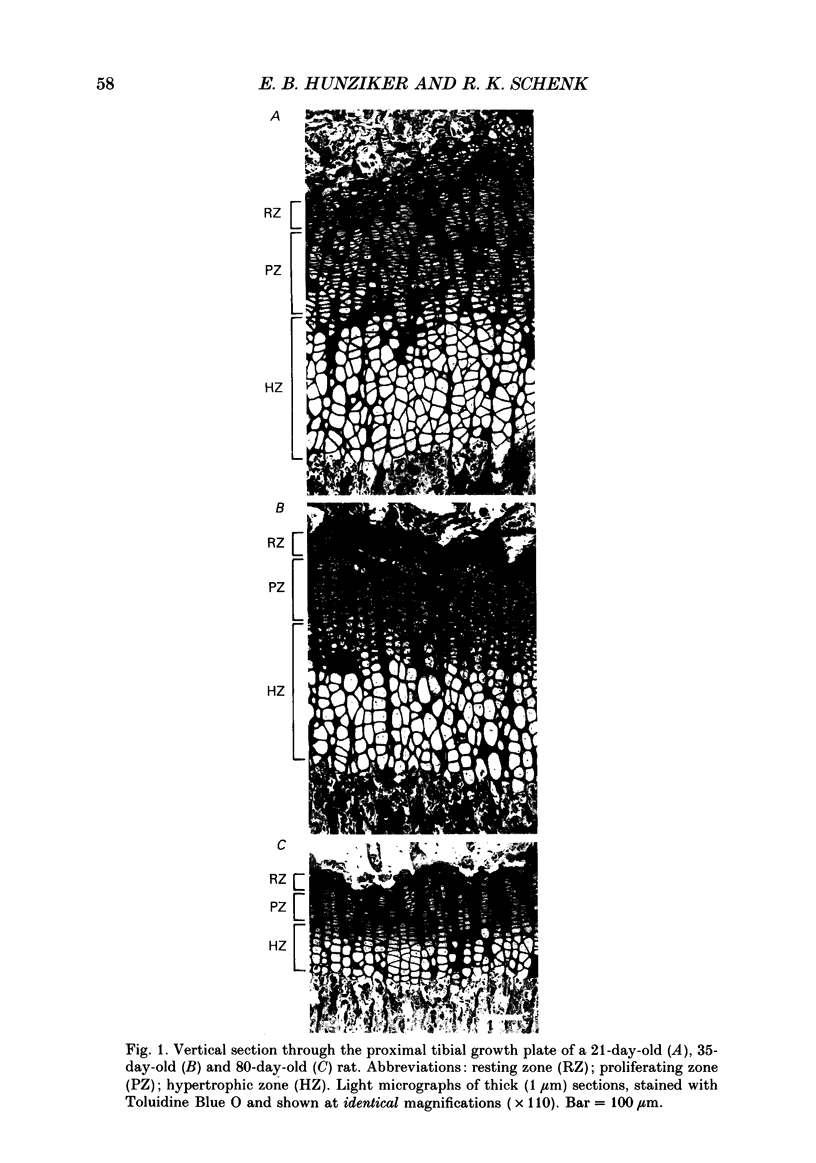
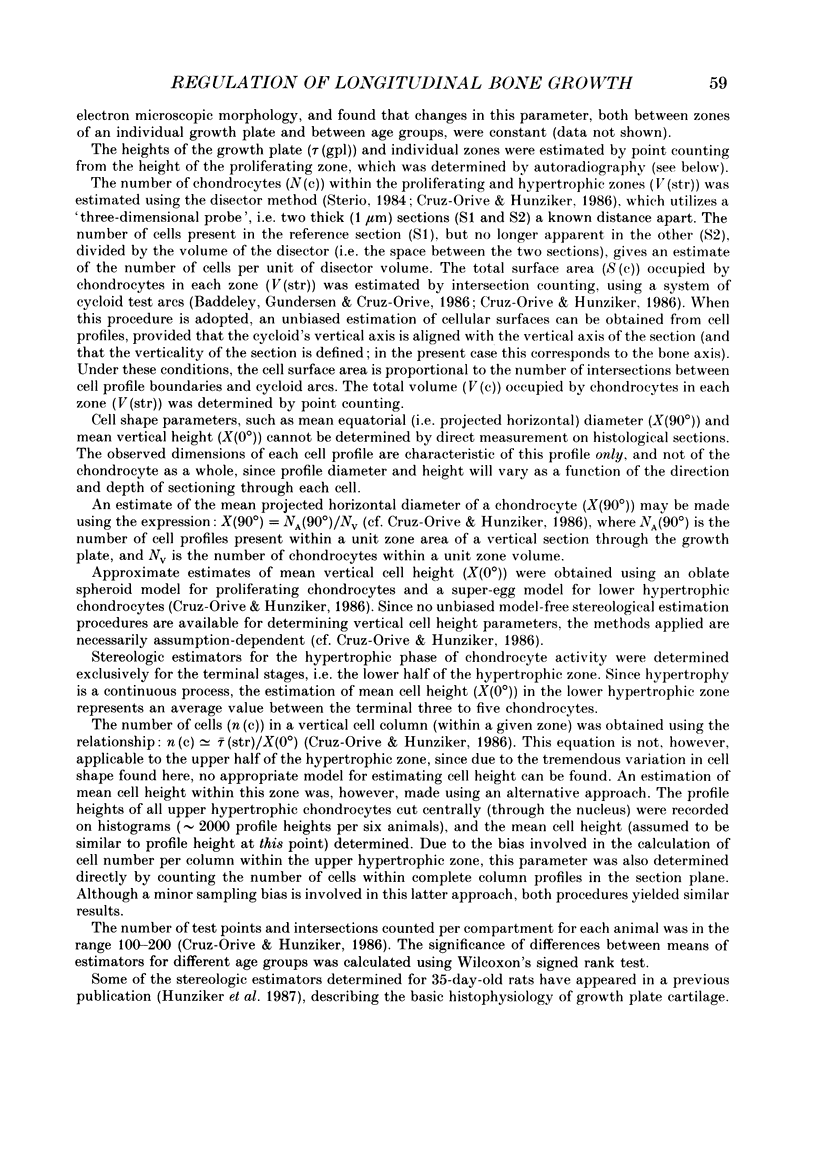
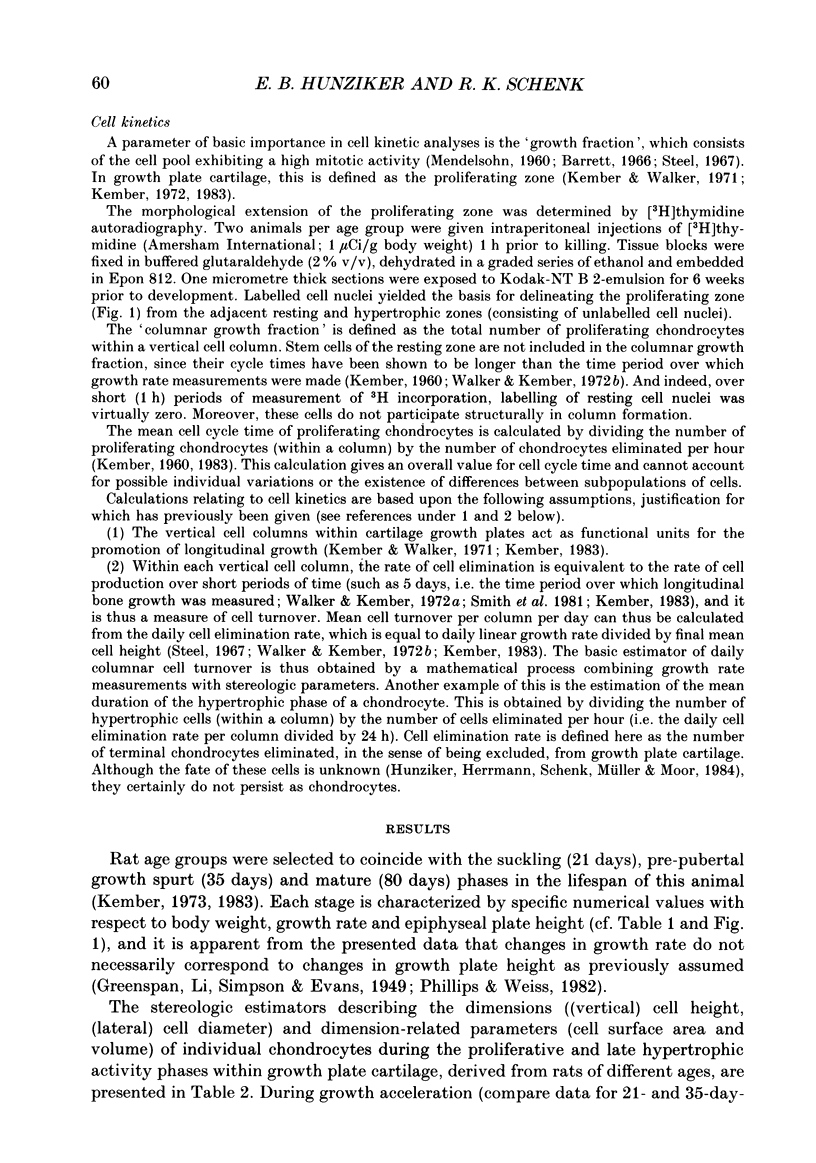
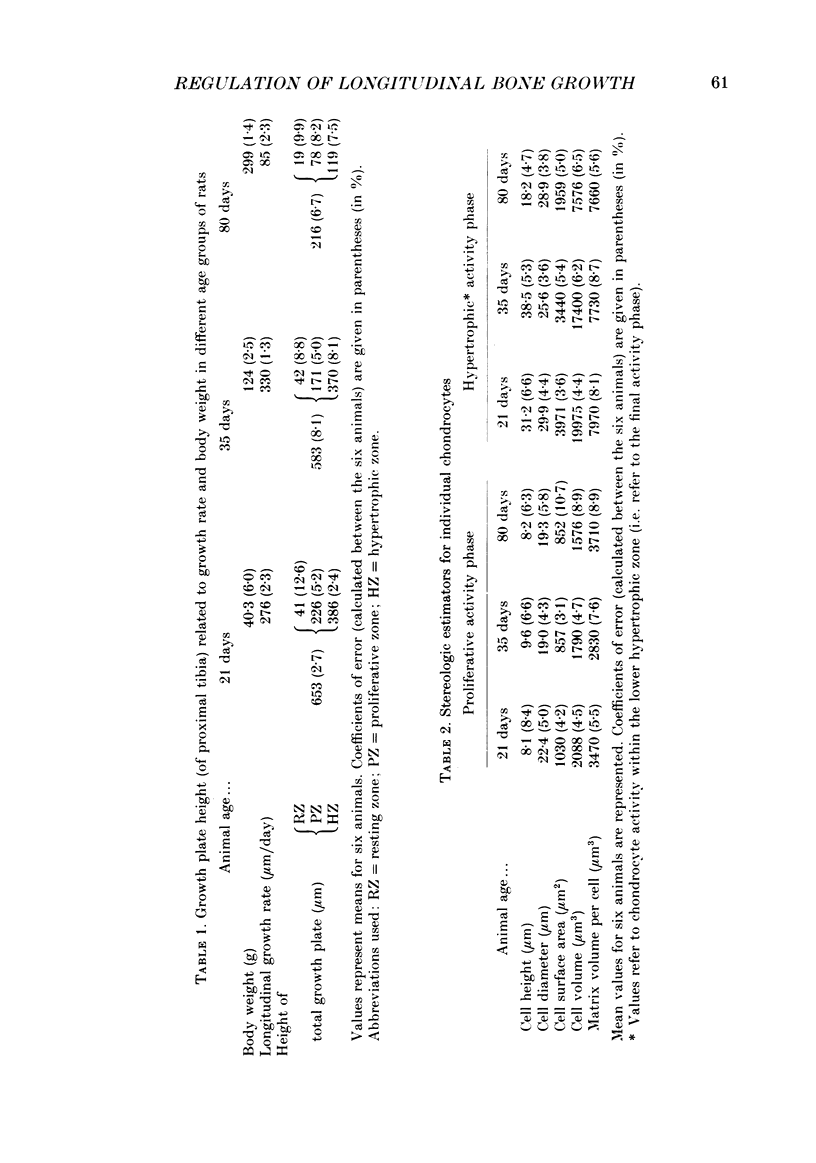
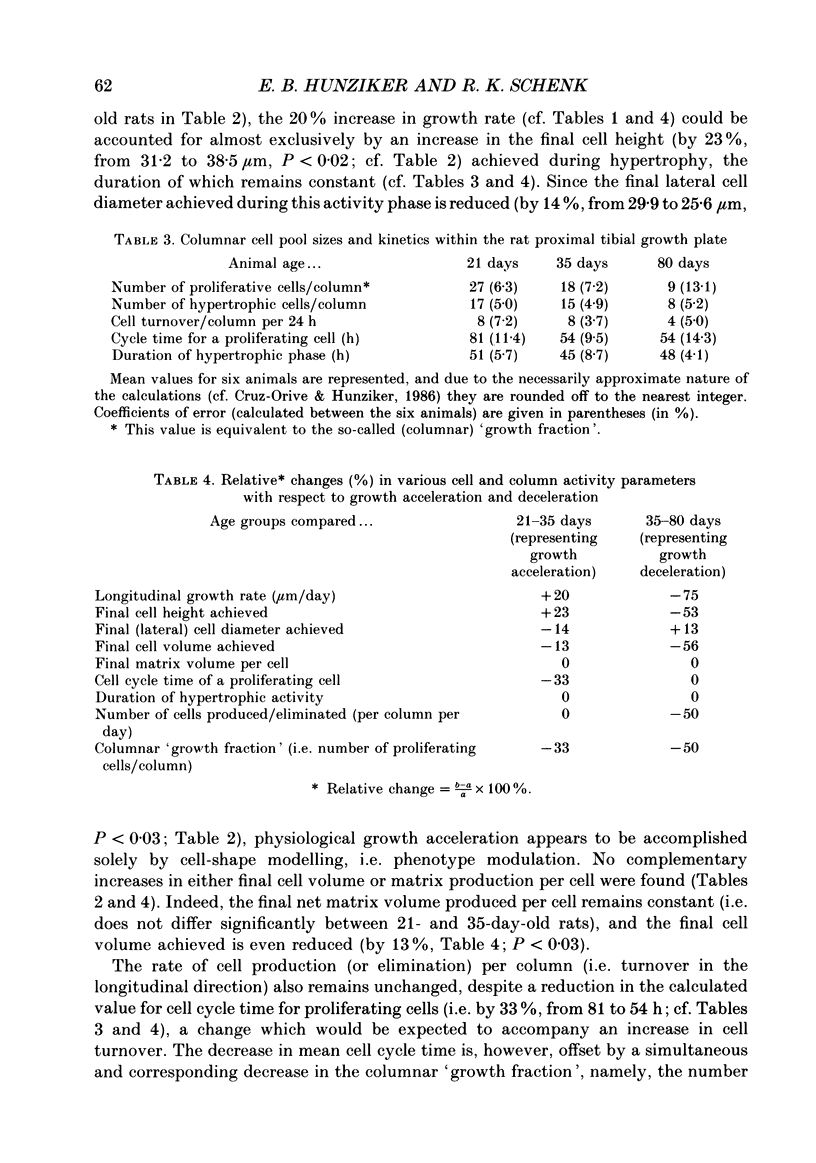
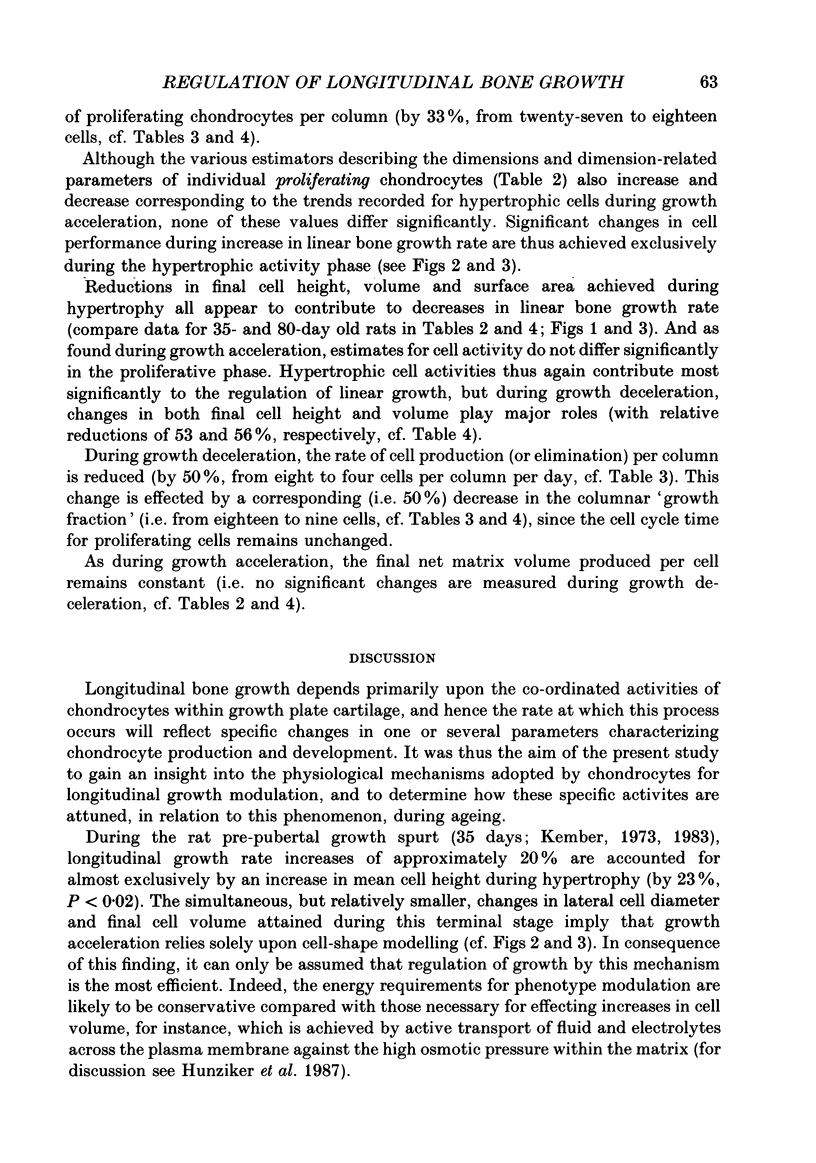
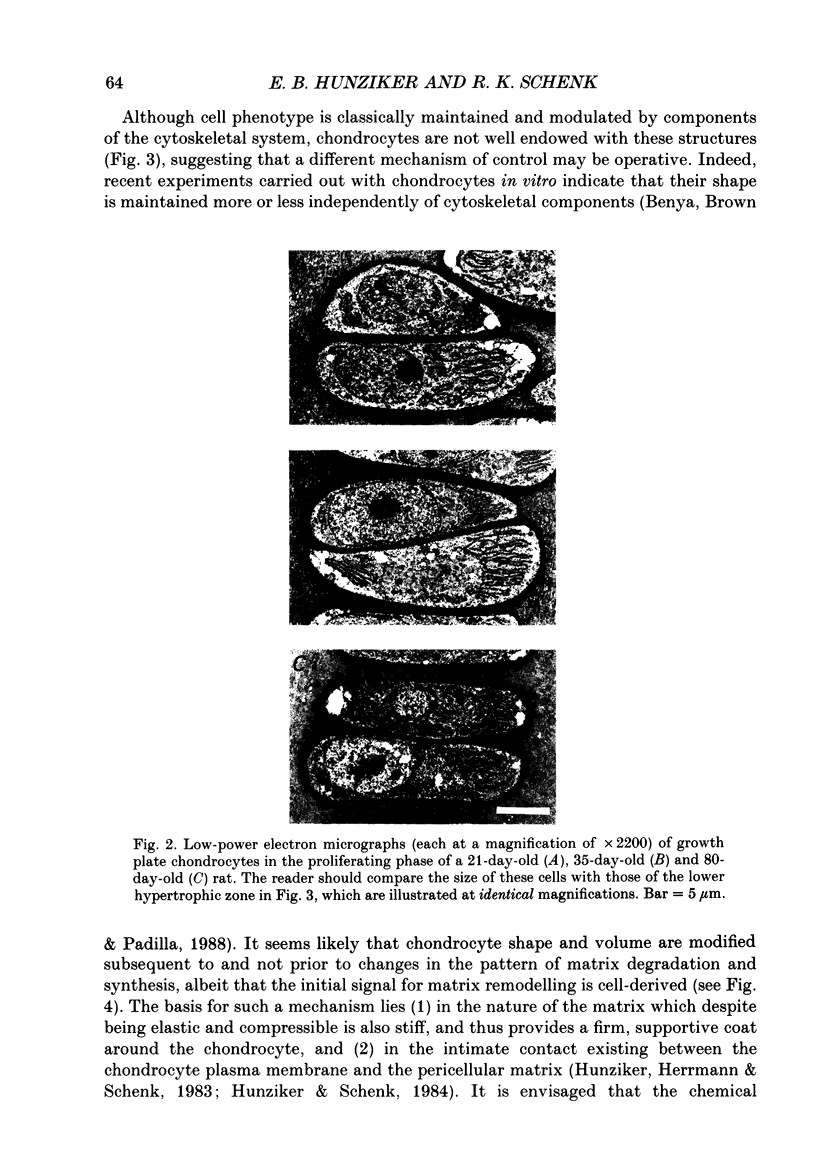
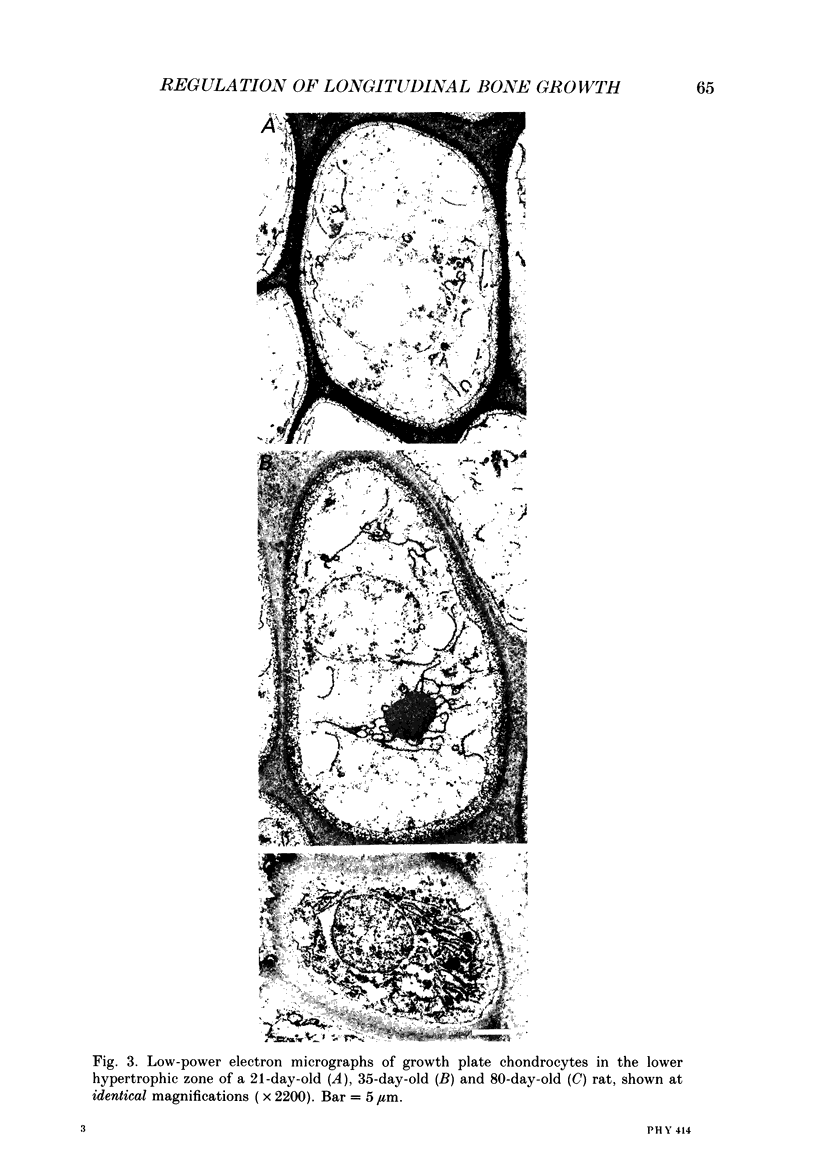
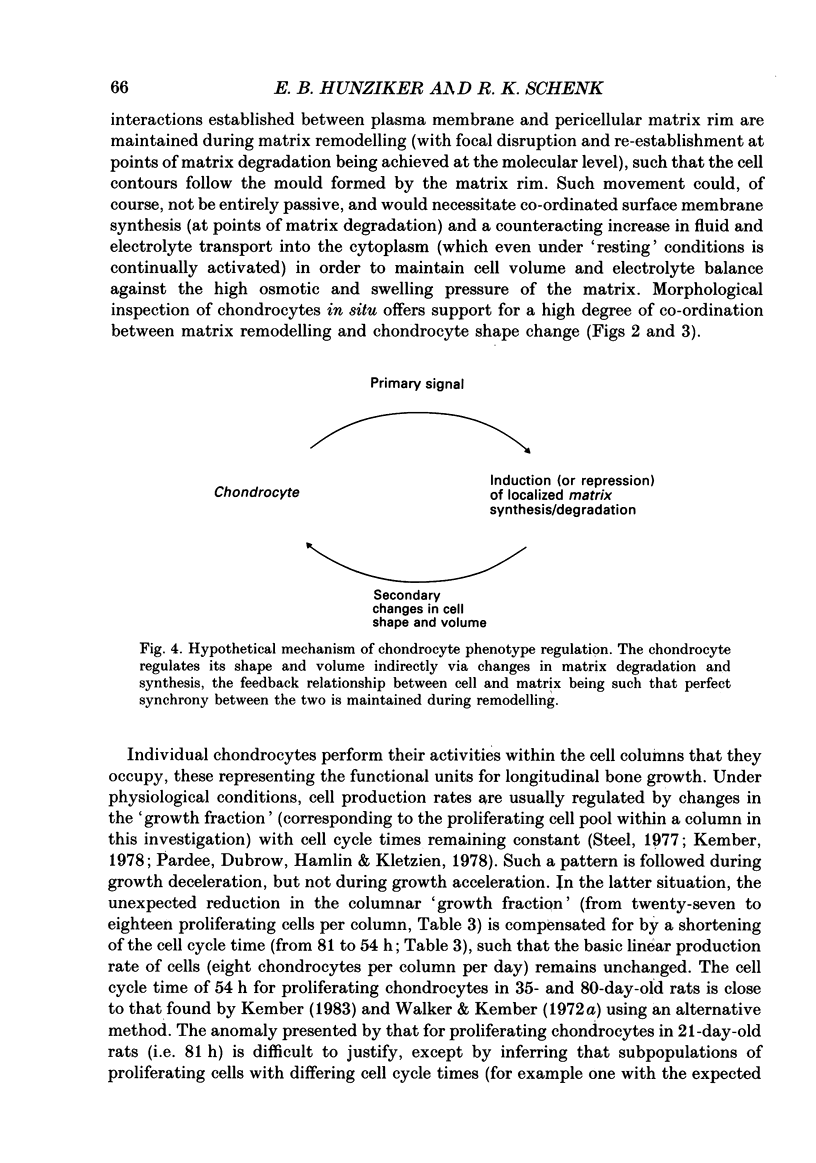
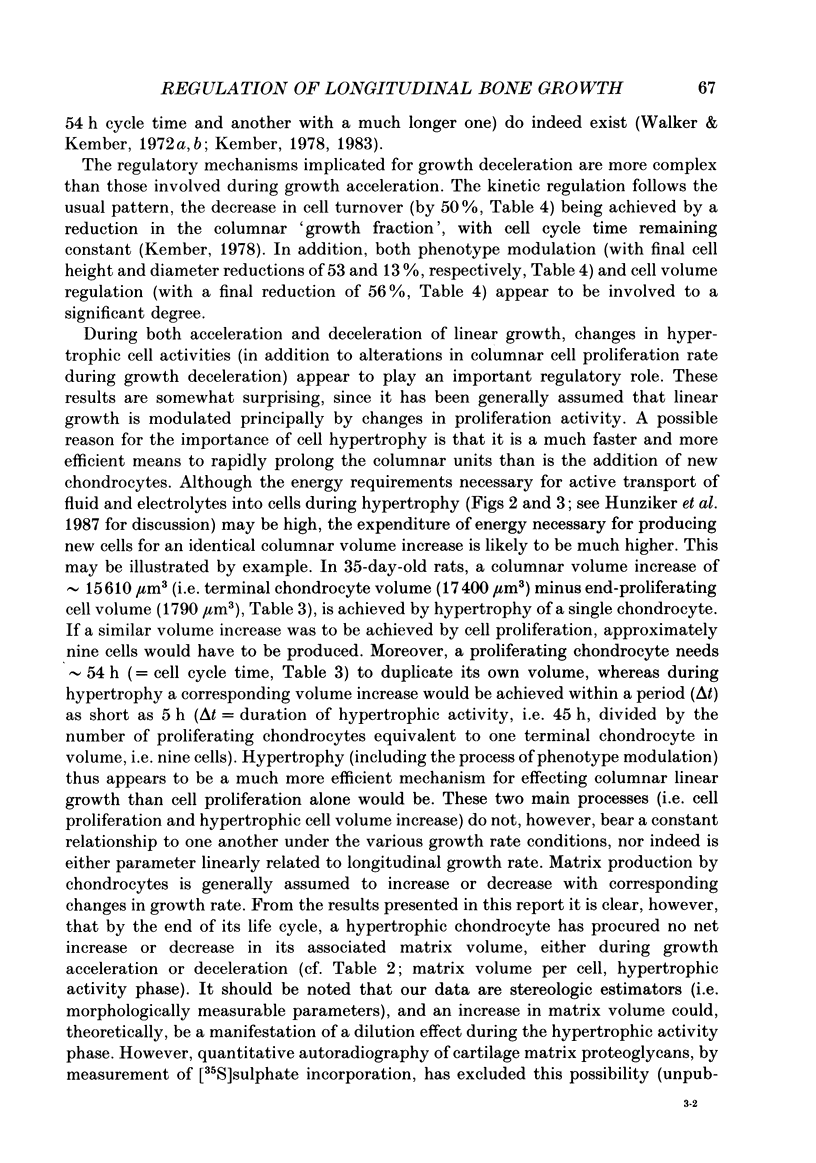
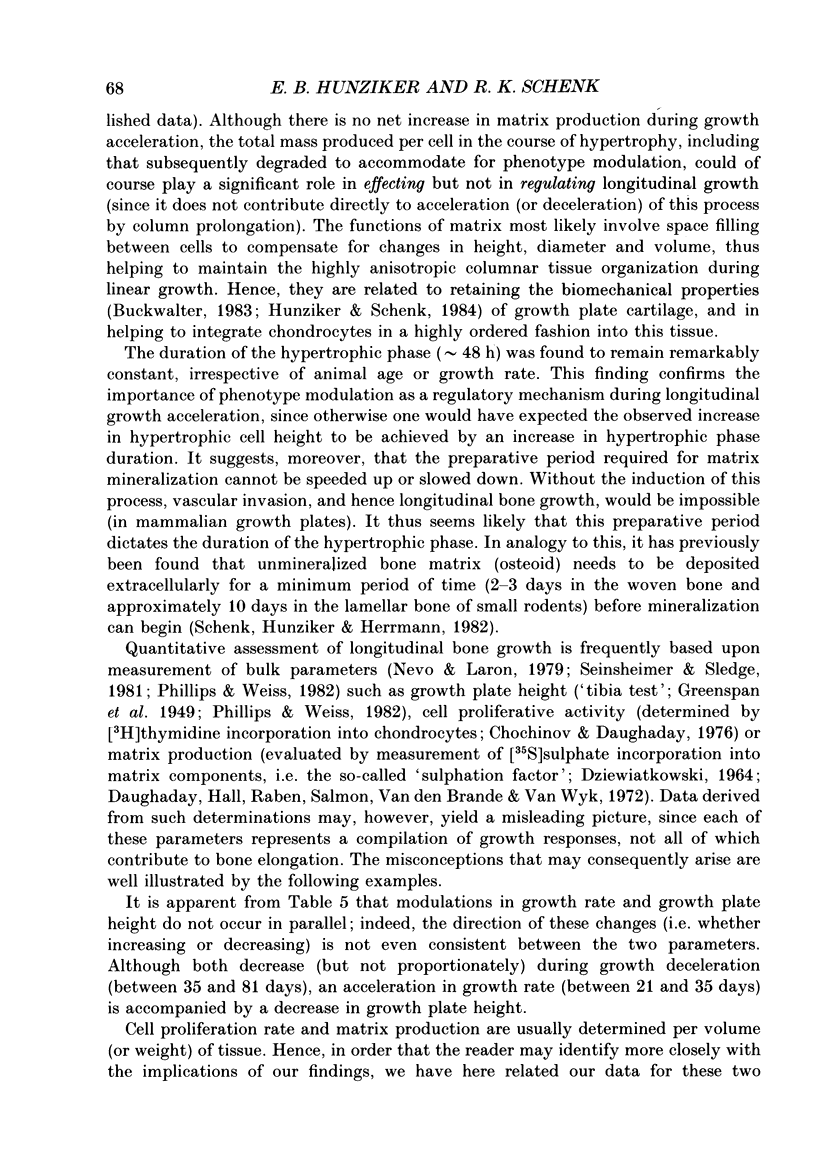
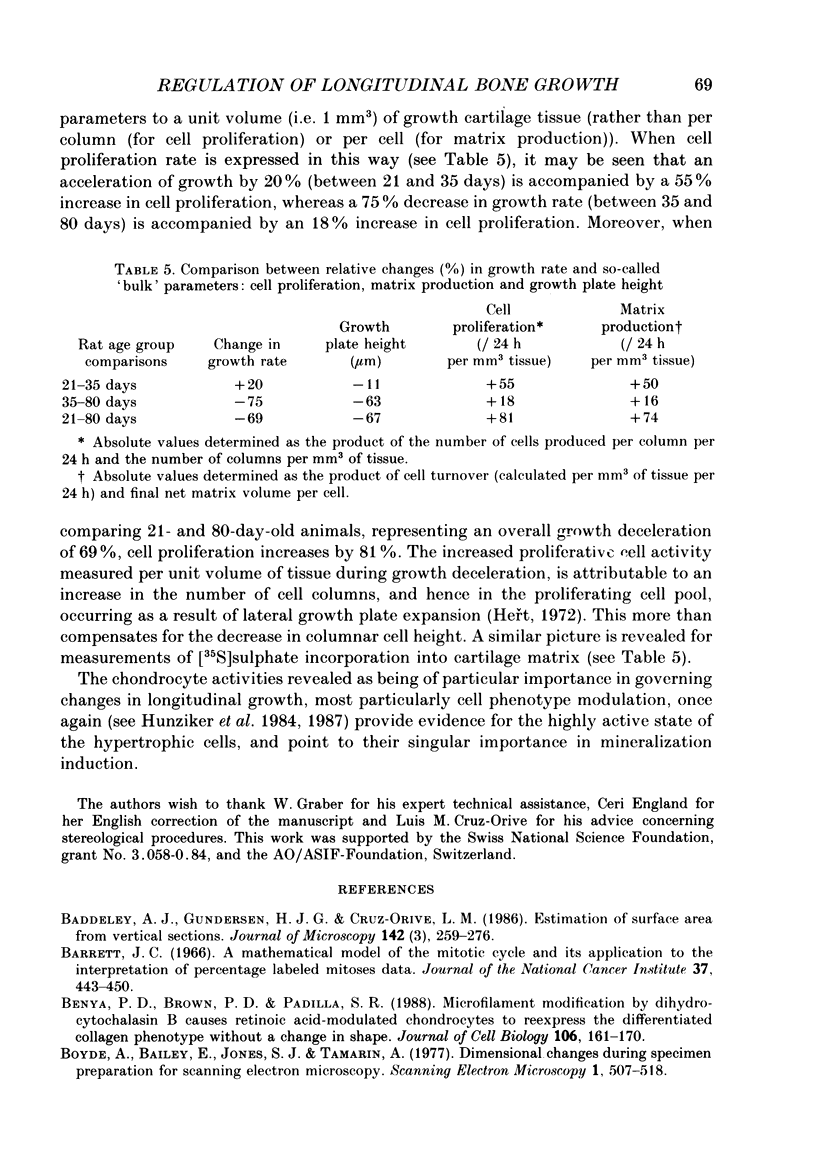
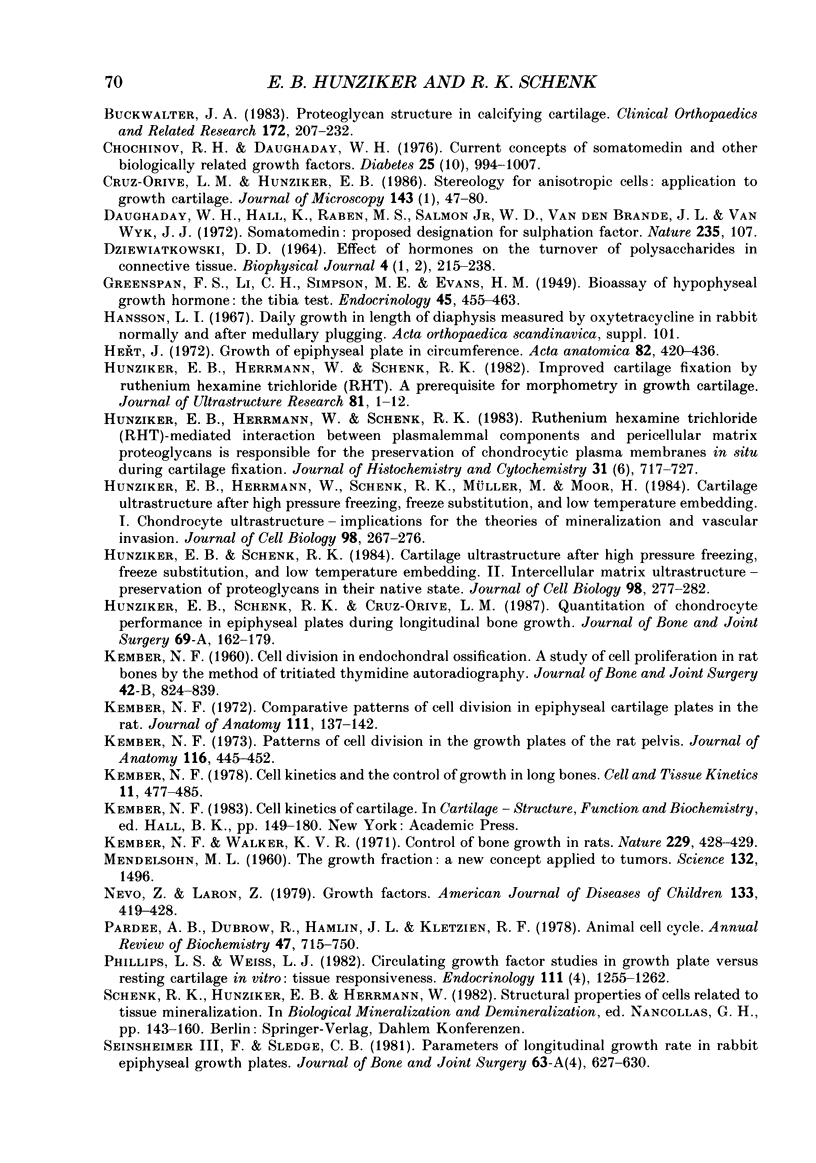
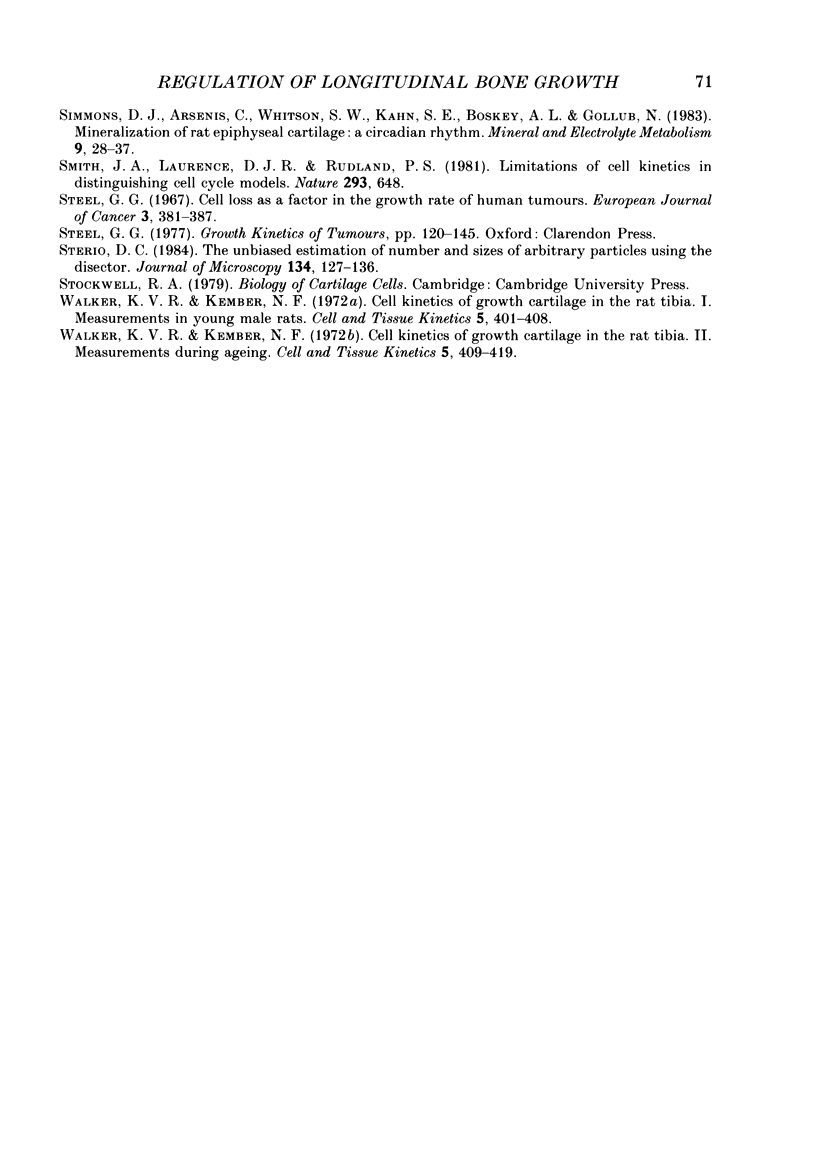
Images in this article
Selected References
These references are in PubMed. This may not be the complete list of references from this article.
- Baddeley A. J., Gundersen H. J., Cruz-Orive L. M. Estimation of surface area from vertical sections. J Microsc. 1986 Jun;142(Pt 3):259–276. doi: 10.1111/j.1365-2818.1986.tb04282.x. [DOI] [PubMed] [Google Scholar]
- Barrett J. C. A mathematical model of the mitotic cycle and its application to the interpretation of percentage labeled mitoses data. J Natl Cancer Inst. 1966 Oct;37(4):443–450. [PubMed] [Google Scholar]
- Benya P. D., Brown P. D., Padilla S. R. Microfilament modification by dihydrocytochalasin B causes retinoic acid-modulated chondrocytes to reexpress the differentiated collagen phenotype without a change in shape. J Cell Biol. 1988 Jan;106(1):161–170. doi: 10.1083/jcb.106.1.161. [DOI] [PMC free article] [PubMed] [Google Scholar]
- Buckwalter J. A. Proteoglycan structure in calcifying cartilage. Clin Orthop Relat Res. 1983 Jan-Feb;(172):207–232. [PubMed] [Google Scholar]
- Chochinov R. H., Daughaday W. H. Current concepts of somatomedin and other biologically related growth factors. Diabetes. 1976 Oct;25(10):994–1004. doi: 10.2337/diab.25.10.994. [DOI] [PubMed] [Google Scholar]
- Cruz-Orive L. M., Hunziker E. B. Stereology for anisotropic cells: application to growth cartilage. J Microsc. 1986 Jul;143(Pt 1):47–80. doi: 10.1111/j.1365-2818.1986.tb02765.x. [DOI] [PubMed] [Google Scholar]
- DZIEWIATKOWSKI D. D. EFFECT OF HORMONES ON THE TURNOVER OF POLYSACCHARIDES IN CONNECTIVE TISSUES. Biophys J. 1964 Jan;4:SUPPL–237. doi: 10.1016/s0006-3495(64)86940-x. [DOI] [PMC free article] [PubMed] [Google Scholar]
- Daughaday W. H., Hall K., Raben M. S., Salmon W. D., Jr, van den Brande J. L., van Wyk J. J. Somatomedin: proposed designation for sulphation factor. Nature. 1972 Jan 14;235(5333):107–107. doi: 10.1038/235107a0. [DOI] [PubMed] [Google Scholar]
- GREENSPAN F. S., LI C. H. Bioassay of hypophyseal growth hormone; the tibia test. Endocrinology. 1949 Nov;45(5):455-63, illust. doi: 10.1210/endo-45-5-455. [DOI] [PubMed] [Google Scholar]
- Hansson L. I. Daily growth in length of diaphysis measured by oxytetracycline in rabbit normally and after medullary plugging. Acta Orthop Scand. 1967;(Suppl):1+–1+. doi: 10.3109/ort.1967.38.suppl-101.01. [DOI] [PubMed] [Google Scholar]
- Hunziker E. B., Herrmann W., Schenk R. K. Improved cartilage fixation by ruthenium hexammine trichloride (RHT). A prerequisite for morphometry in growth cartilage. J Ultrastruct Res. 1982 Oct;81(1):1–12. doi: 10.1016/s0022-5320(82)90036-3. [DOI] [PubMed] [Google Scholar]
- Hunziker E. B., Herrmann W., Schenk R. K., Mueller M., Moor H. Cartilage ultrastructure after high pressure freezing, freeze substitution, and low temperature embedding. I. Chondrocyte ultrastructure--implications for the theories of mineralization and vascular invasion. J Cell Biol. 1984 Jan;98(1):267–276. doi: 10.1083/jcb.98.1.267. [DOI] [PMC free article] [PubMed] [Google Scholar]
- Hunziker E. B., Herrmann W., Schenk R. K. Ruthenium hexammine trichloride (RHT)-mediated interaction between plasmalemmal components and pericellular matrix proteoglycans is responsible for the preservation of chondrocytic plasma membranes in situ during cartilage fixation. J Histochem Cytochem. 1983 Jun;31(6):717–727. doi: 10.1177/31.6.6341460. [DOI] [PubMed] [Google Scholar]
- Hunziker E. B., Schenk R. K. Cartilage ultrastructure after high pressure freezing, freeze substitution, and low temperature embedding. II. Intercellular matrix ultrastructure - preservation of proteoglycans in their native state. J Cell Biol. 1984 Jan;98(1):277–282. doi: 10.1083/jcb.98.1.277. [DOI] [PMC free article] [PubMed] [Google Scholar]
- Hunziker E. B., Schenk R. K., Cruz-Orive L. M. Quantitation of chondrocyte performance in growth-plate cartilage during longitudinal bone growth. J Bone Joint Surg Am. 1987 Feb;69(2):162–173. [PubMed] [Google Scholar]
- KEMBER N. F. Cell division in endochondral ossification. A study of cell proliferation in rat bones by the method of tritiated thymidine autoradiography. J Bone Joint Surg Br. 1960 Nov;42B:824–839. [PubMed] [Google Scholar]
- Kember N. F. Cell kinetics and the control of growth in long bones. Cell Tissue Kinet. 1978 Sep;11(5):477–485. doi: 10.1111/j.1365-2184.1978.tb00820.x. [DOI] [PubMed] [Google Scholar]
- Kember N. F. Comparative patterns of cell division in epiphyseal cartilage plates in the rat. J Anat. 1972 Jan;111(Pt 1):137–142. [PMC free article] [PubMed] [Google Scholar]
- Kember N. F. Patterns of cell division in the growth plates of the rat pelvis. J Anat. 1973 Dec;116(Pt 3):445–452. [PMC free article] [PubMed] [Google Scholar]
- Kember N. F., Walker K. V. Control of bone growth in rats. Nature. 1971 Feb 5;229(5284):428–429. doi: 10.1038/229428a0. [DOI] [PubMed] [Google Scholar]
- Nevo Z., Laron Z. Growth factors. Am J Dis Child. 1979 Apr;133(4):419–428. doi: 10.1001/archpedi.1979.02130040073016. [DOI] [PubMed] [Google Scholar]
- Pardee A. B., Dubrow R., Hamlin J. L., Kletzien R. F. Animal cell cycle. Annu Rev Biochem. 1978;47:715–750. doi: 10.1146/annurev.bi.47.070178.003435. [DOI] [PubMed] [Google Scholar]
- Phillips L. S., Weiss L. J. Circulating growth factor studies in growth plate versus resting cartilage in vitro: tissue responsiveness. Endocrinology. 1982 Oct;111(4):1255–1262. doi: 10.1210/endo-111-4-1255. [DOI] [PubMed] [Google Scholar]
- Seinsheimer F., 3rd, Sledge C. B. Parameters of longitudinal growth rate in rabbit epiphyseal growth plates. J Bone Joint Surg Am. 1981 Apr;63(4):627–630. [PubMed] [Google Scholar]
- Simmons D. J., Arsenis C., Whitson S. W., Kahn S. E., Boskey A. L., Gollub N. Mineralization of rat epiphyseal cartilage: a circadian rhythm. Miner Electrolyte Metab. 1983 Jan-Feb;9(1):28–37. [PubMed] [Google Scholar]
- Smith J. A., Laurence D. J., Rudland P. S. Limitations of cell kinetics in distinguishing cell cycle models. Nature. 1981 Oct 22;293(5834):648–650. doi: 10.1038/293648a0. [DOI] [PubMed] [Google Scholar]
- Steel G. G. Cell loss as a factor in the growth rate of human tumours. Eur J Cancer. 1967 Nov;3(4):381–387. doi: 10.1016/0014-2964(67)90022-9. [DOI] [PubMed] [Google Scholar]
- Sterio D. C. The unbiased estimation of number and sizes of arbitrary particles using the disector. J Microsc. 1984 May;134(Pt 2):127–136. doi: 10.1111/j.1365-2818.1984.tb02501.x. [DOI] [PubMed] [Google Scholar]
- Walker K. V., Kember N. F. Cell kinetics of growth cartilage in the rat tibia. I. Measurements in young male rats. Cell Tissue Kinet. 1972 Sep;5(5):401–408. doi: 10.1111/j.1365-2184.1972.tb00378.x. [DOI] [PubMed] [Google Scholar]
- Walker K. V., Kember N. F. Cell kinetics of growth cartilage in the rat tibia. II. Measurements during ageing. Cell Tissue Kinet. 1972 Sep;5(5):409–419. doi: 10.1111/j.1365-2184.1972.tb00379.x. [DOI] [PubMed] [Google Scholar]