Abstract
1. We studied the effects of shortening history on isometric force and isotonic velocity in single intact frog fibres. Fibres were isometrically tetanized. When force reached a plateau, shortening was imposed, after which the fibre was held isometric again. Isometric force after shortening could then be compared with controls in which no shortening had taken place. 2. Sarcomere length was measured simultaneously with two independent methods: a laser-diffraction method and a segment-length method that detects the distance between two markers attached to the surface of the fibre, about 800 microns apart. 3. The fibre was mounted between two servomotors. One was used to impose the load clamp while the other cancelled the translation that occurred during this load clamp. Thus, translation of the segment under investigation could be minimized. 4. Initial experiments were performed at the fibre level. We found that active preshortening reduced isometric force considerably, thereby confirming earlier work of others. Force reductions as large as 70% were observed. 5. Under conditions in which there were large effects of shortening at the fibre level, we measured sarcomere length changes in the central region of the fibre. These sarcomeres shortened much less than the fibre's average. In fact, when the load was high, these sarcomeres lengthened while the fibre as a whole shortened. Thus, while the fibre-length signal implied that sarcomeres might have shortened to some intermediate length, in reality some sarcomeres were much longer, others much shorter. 6. Experiments performed at the sarcomere level revealed that isometric force was unaffected by previous sarcomere shortening provided the shortening occurred against either a low load or over a short distance. However, if the work done during shortening was high, force after previous shortening was less than if sarcomeres had remained at the final length throughout contraction. The correlation between the force deficit and the work done during shortening was statistically significant (P = 0.0001). 7. Interrupting the tetanus for 0.5-3.0 s did not reverse the effects of shortening on isometric force; at least 5-10 min of rest were required before force recovered completely. 8. Sarcomeres accelerated during the period of shortening under constant load, indicating that the sarcomeres became progressively stronger. However, the acceleration was less than that predicted from the force-velocity relation applicable at each of the sarcomere lengths transversed during shortening. 9. Velocity of shortening appeared to be much more sensitive to previous shortening than isometric force. 10. Results obtained with the diffraction method were the same as those obtained with the segment method.(ABSTRACT TRUNCATED AT 400 WORDS)
Full text
PDF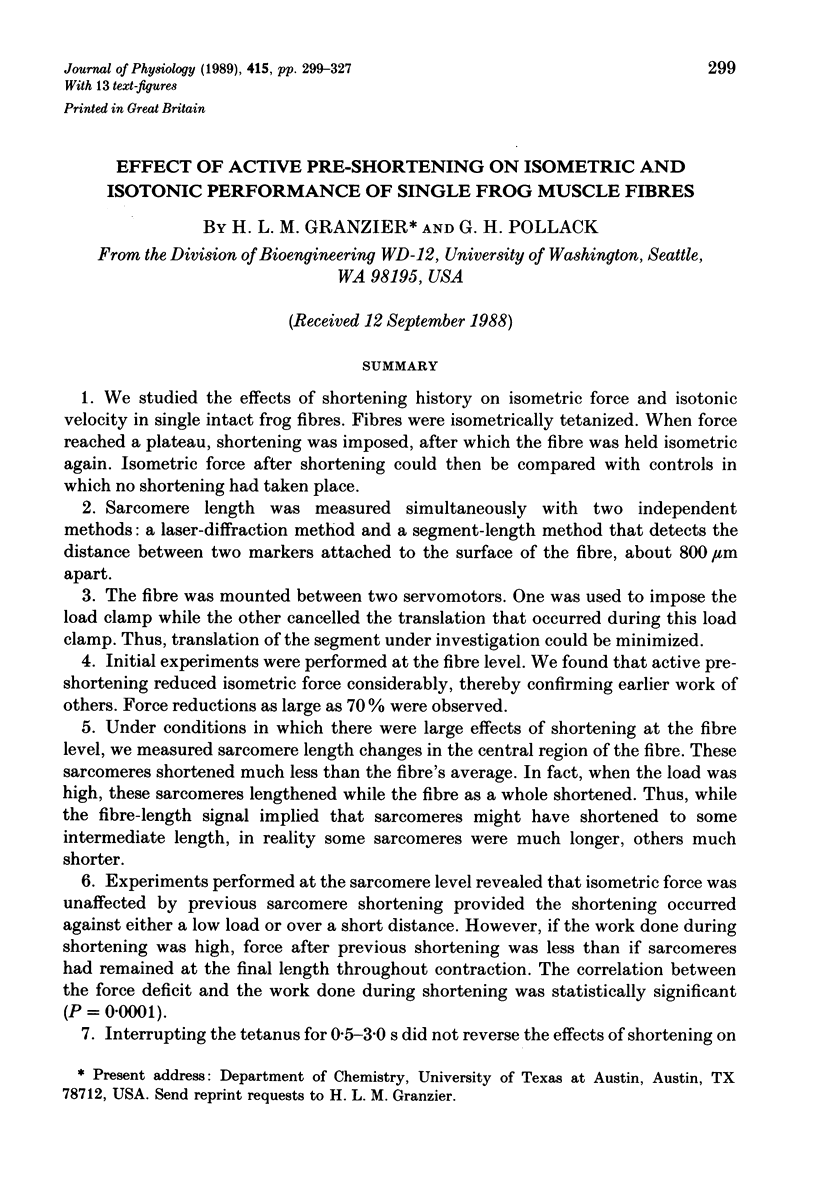
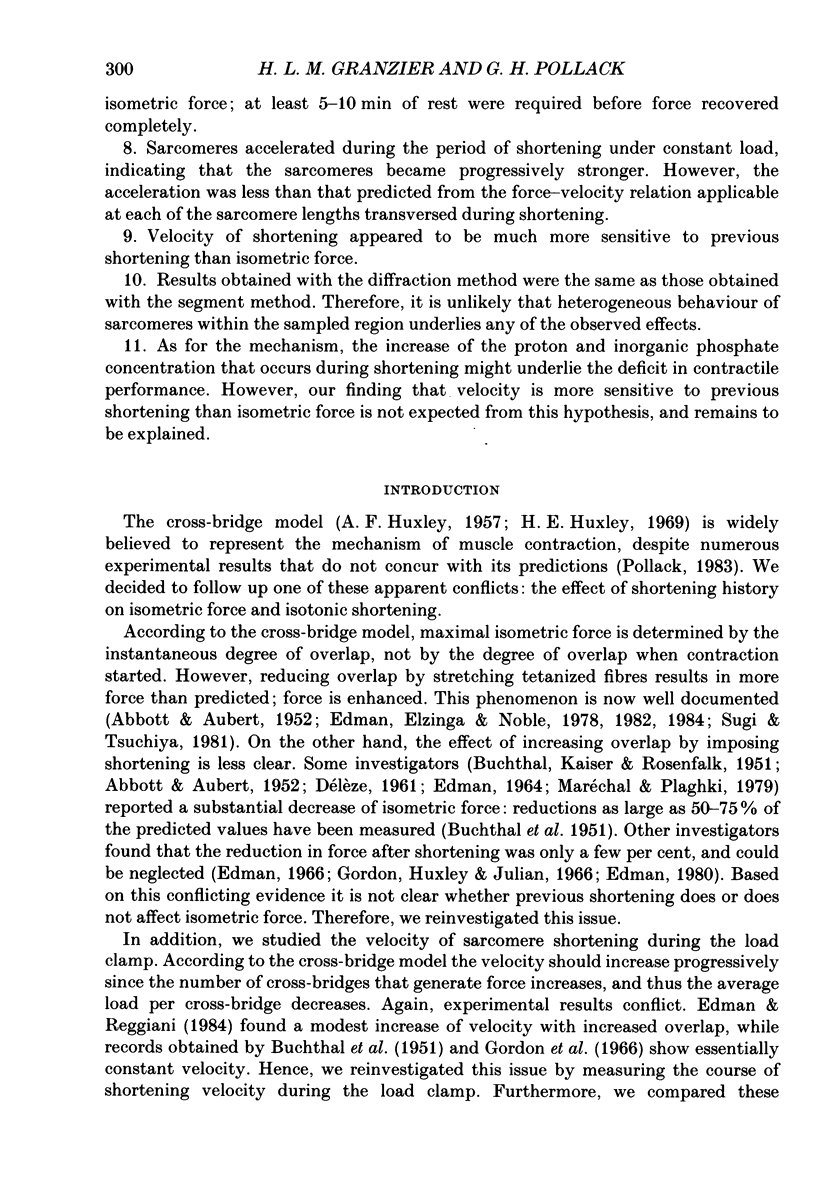
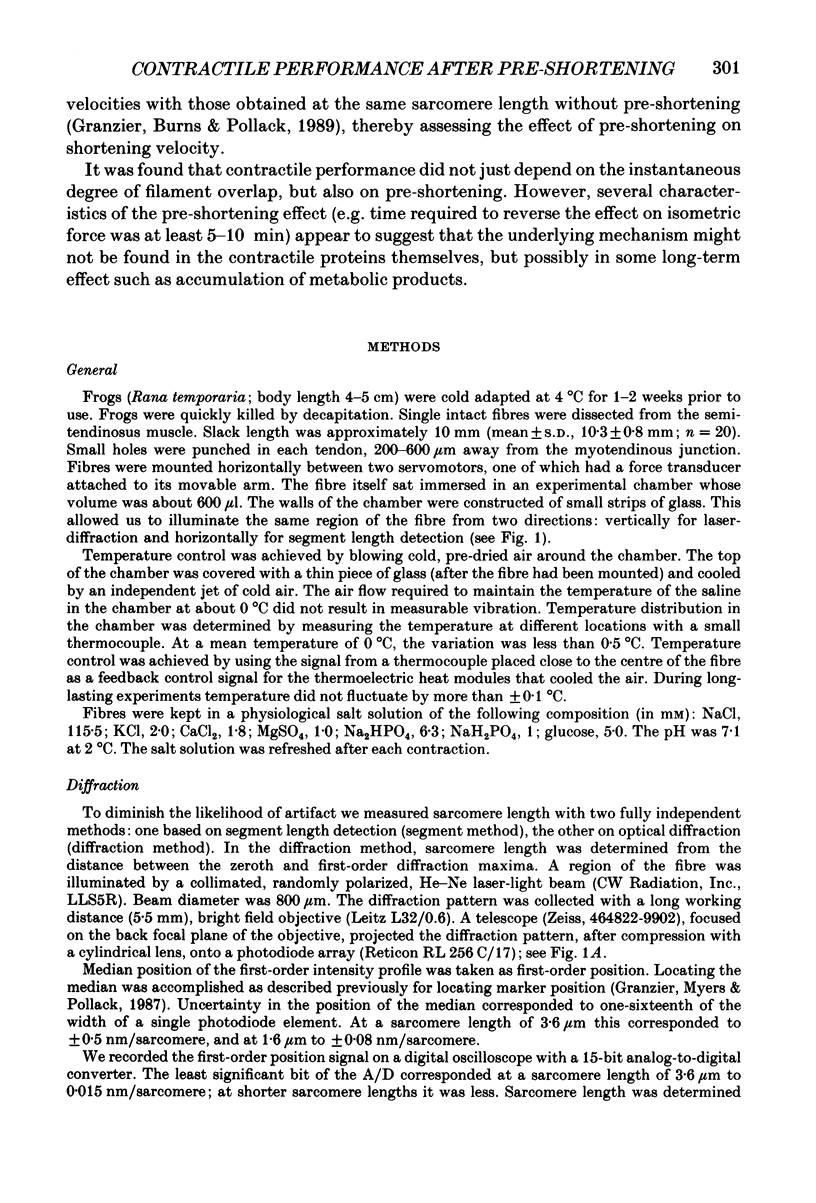
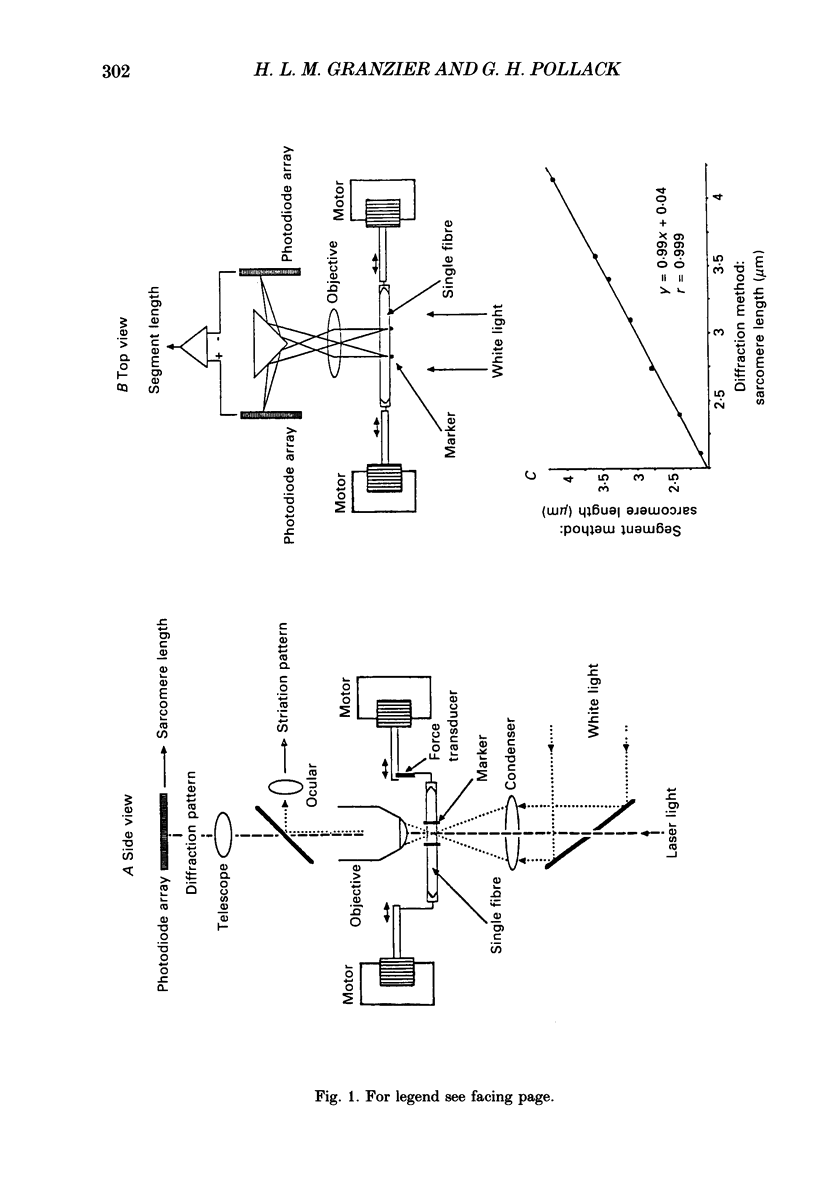
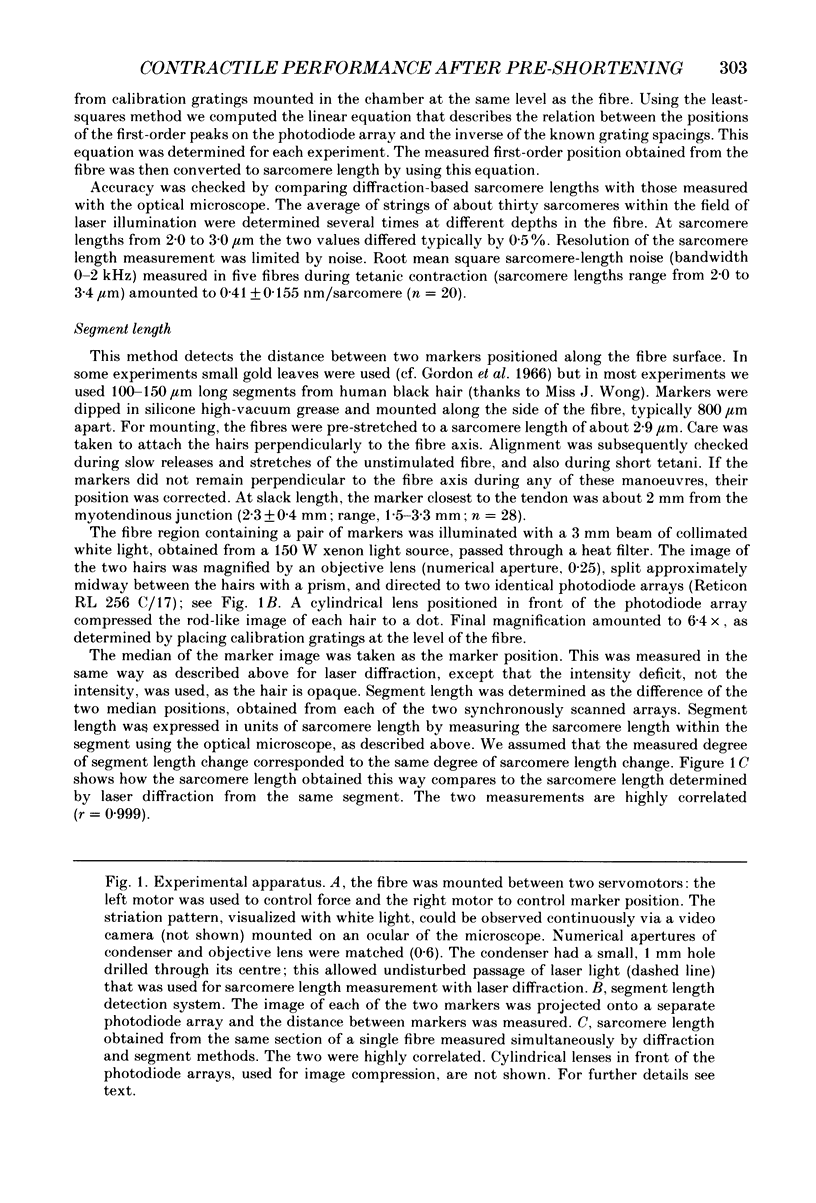
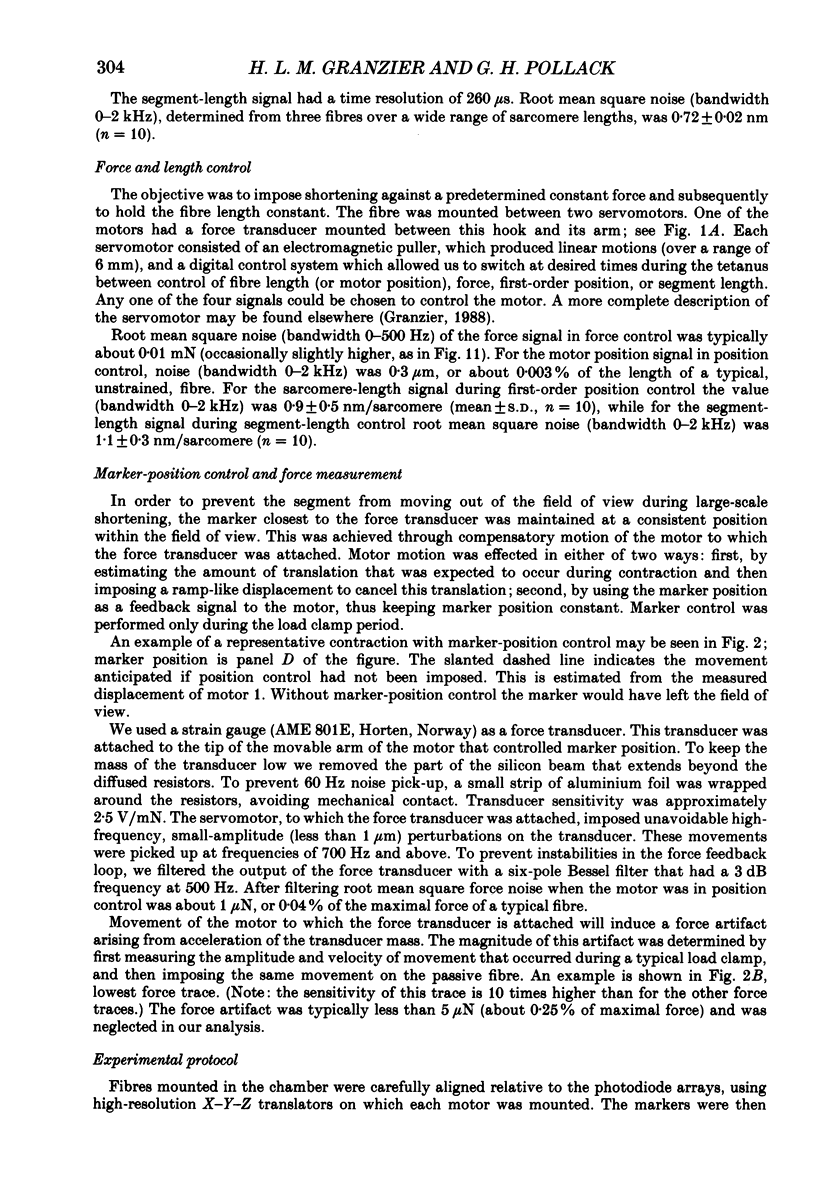
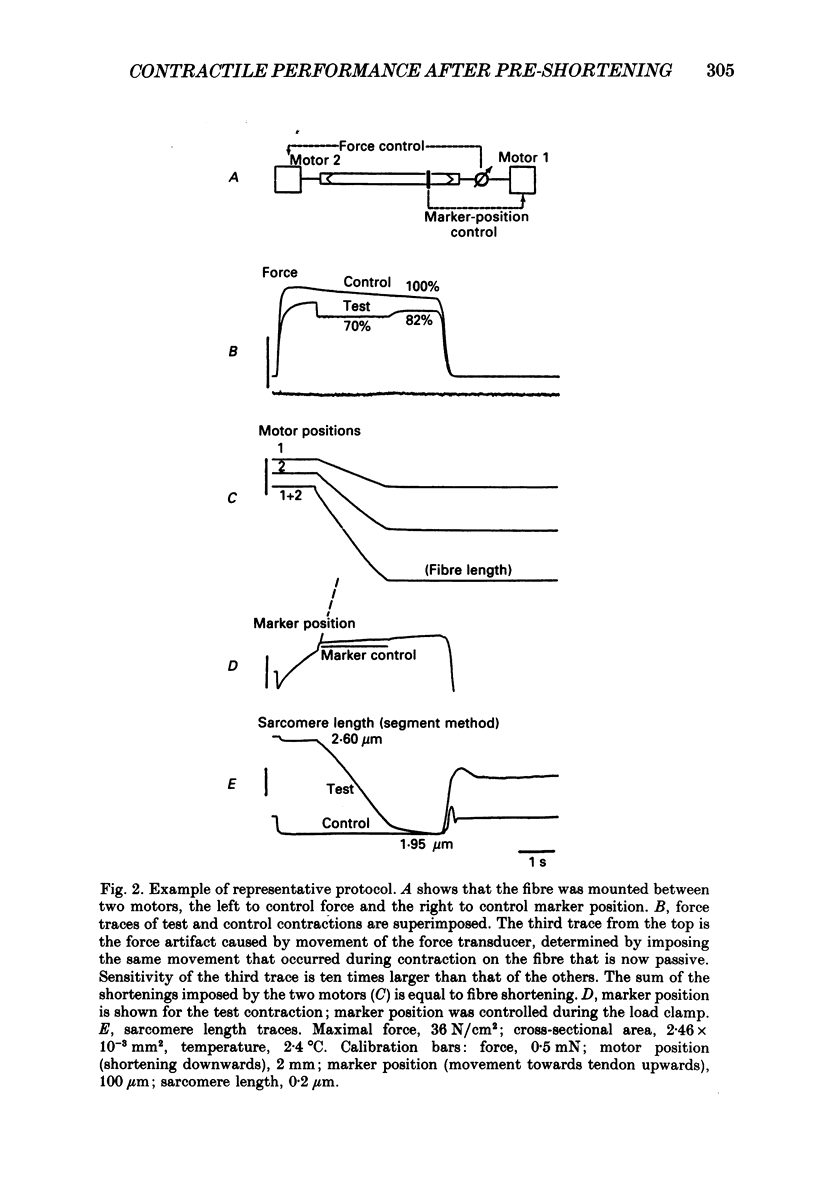
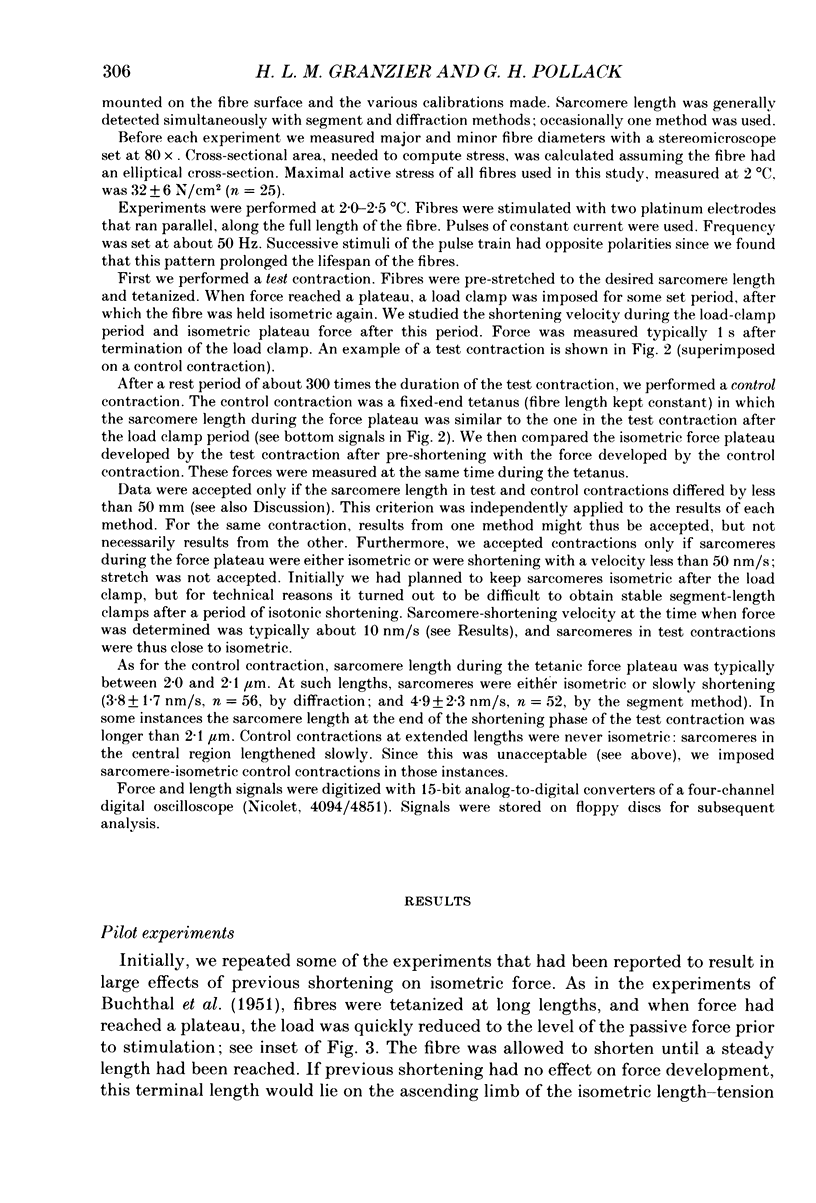
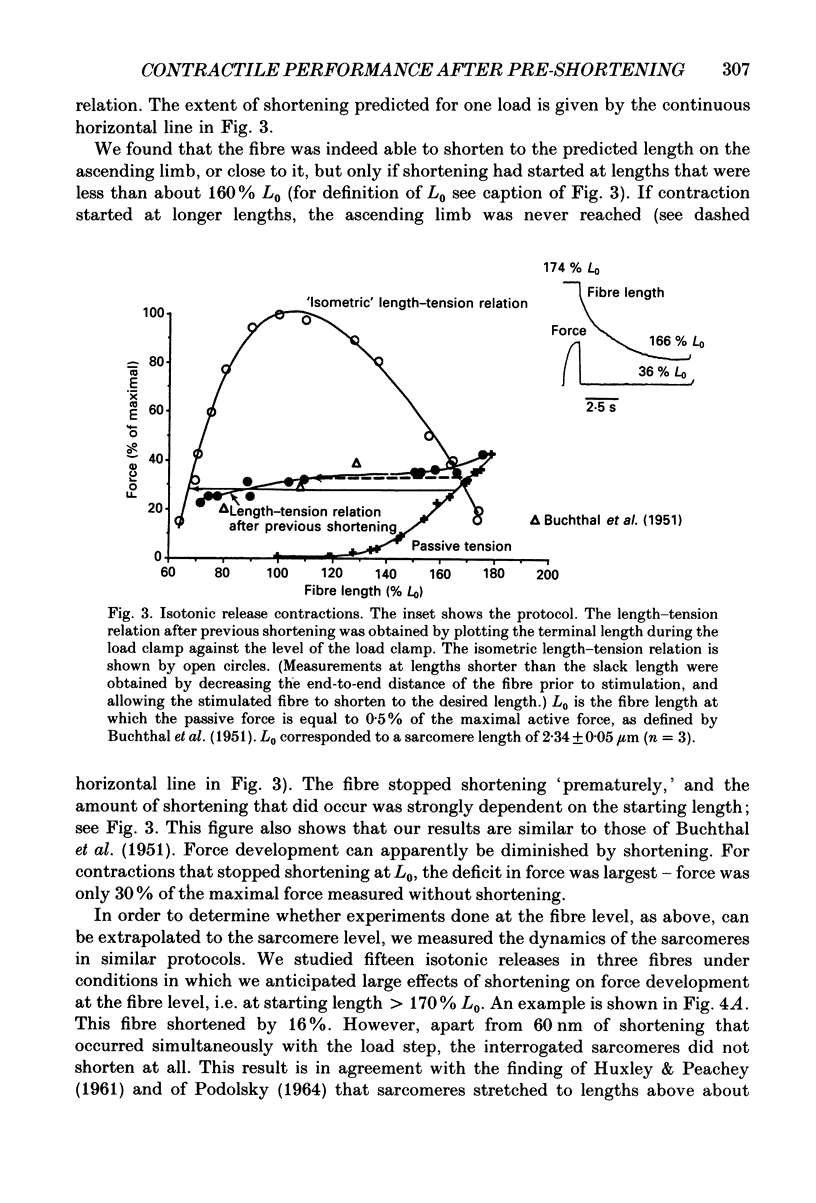
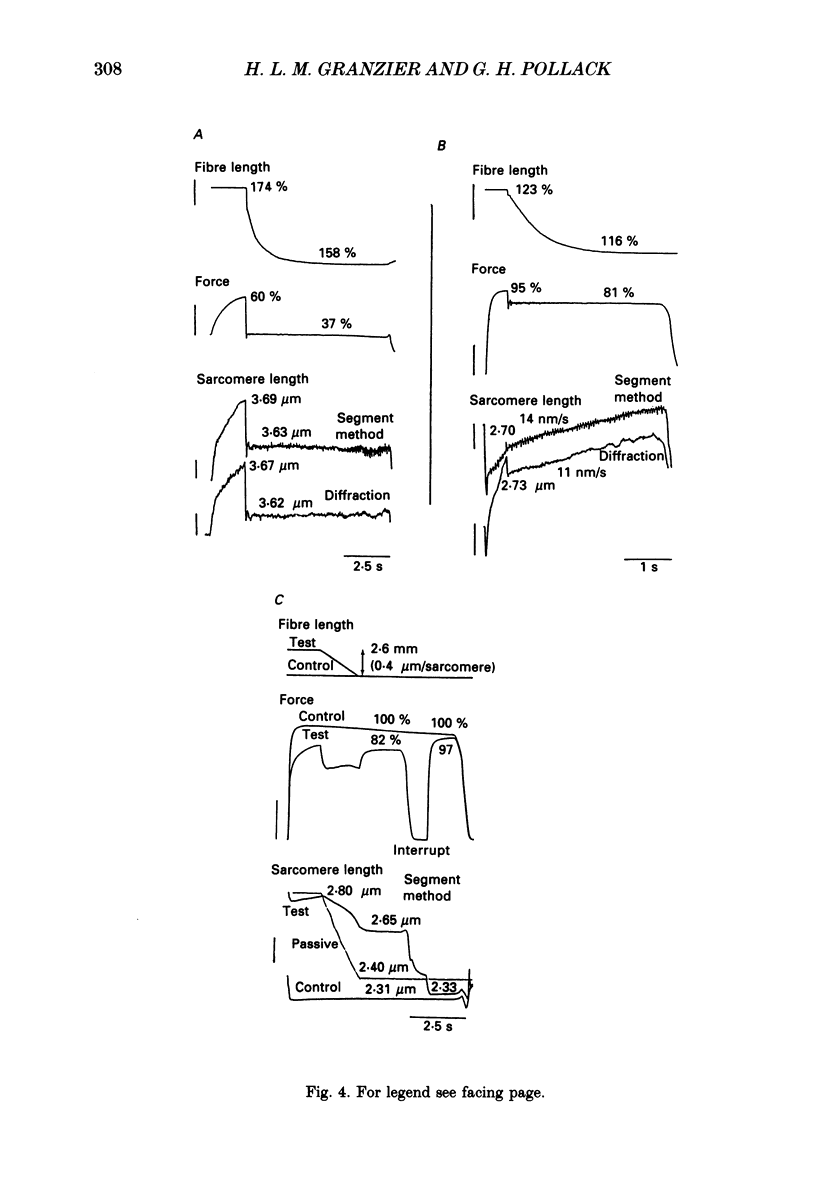
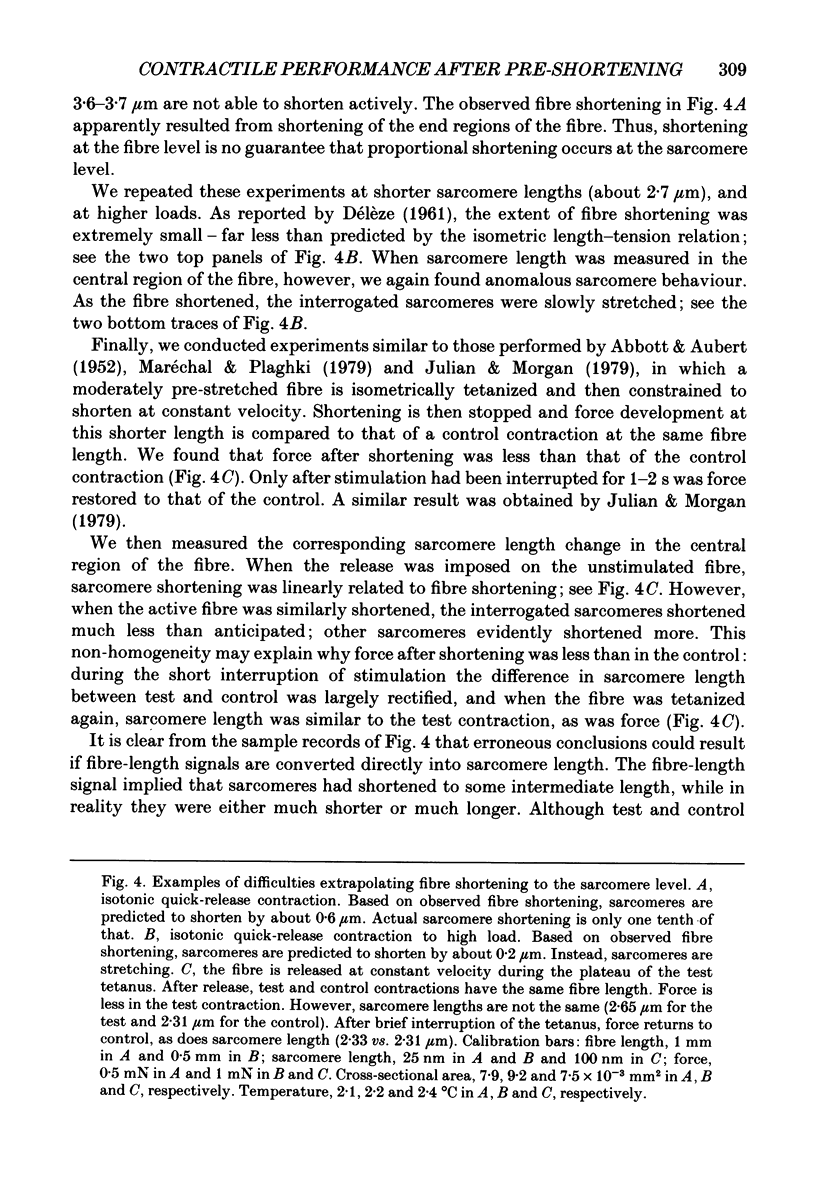
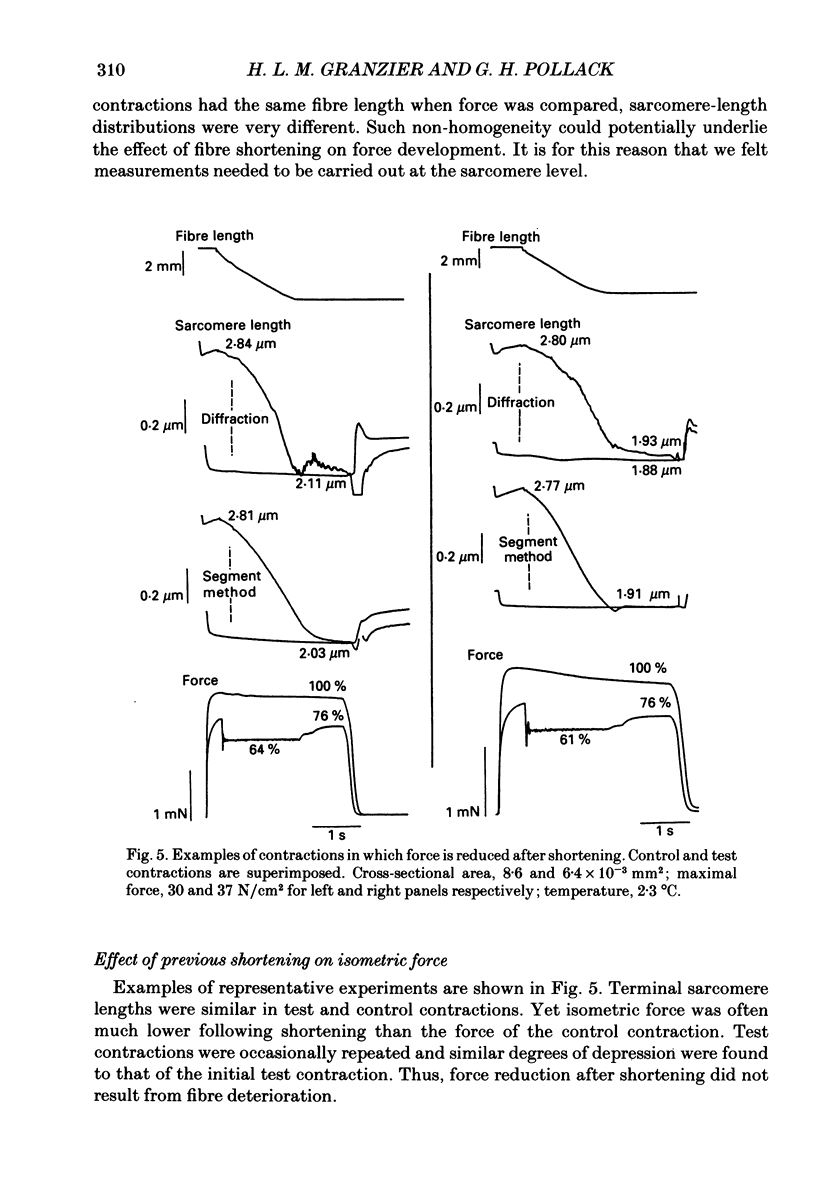
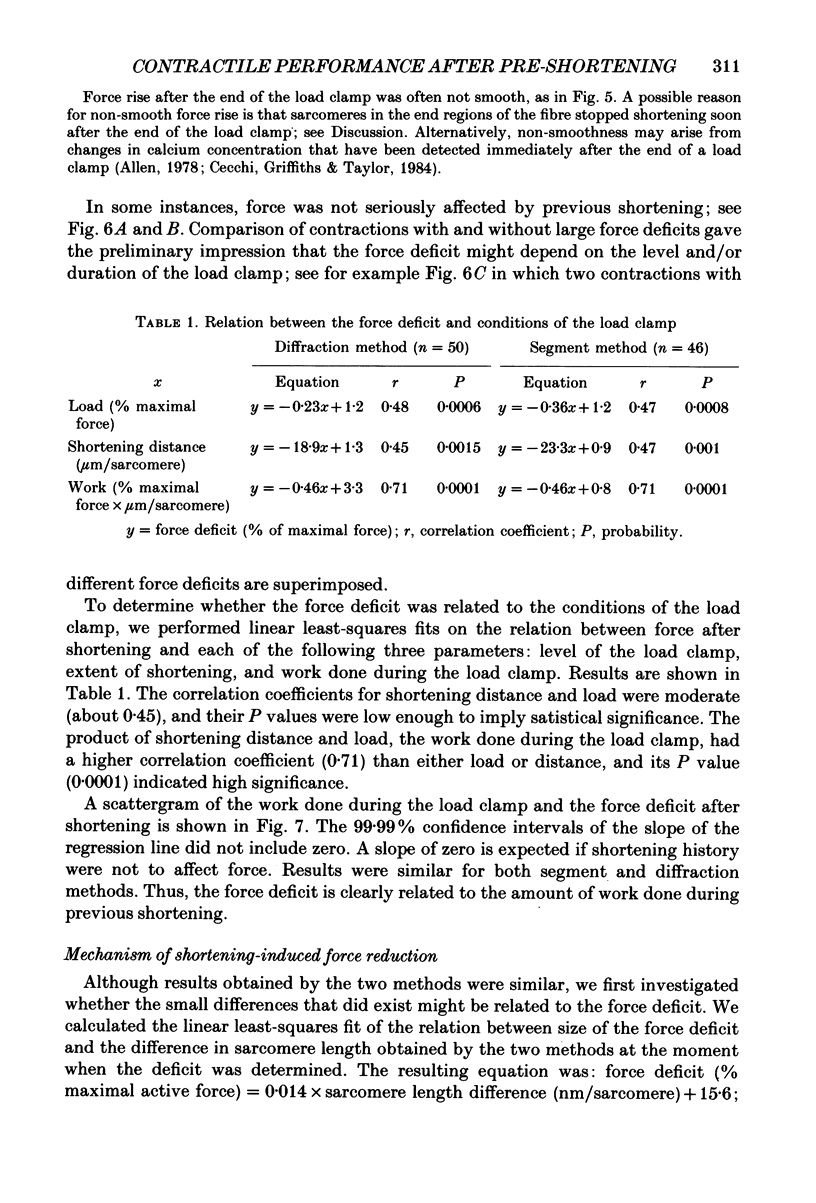
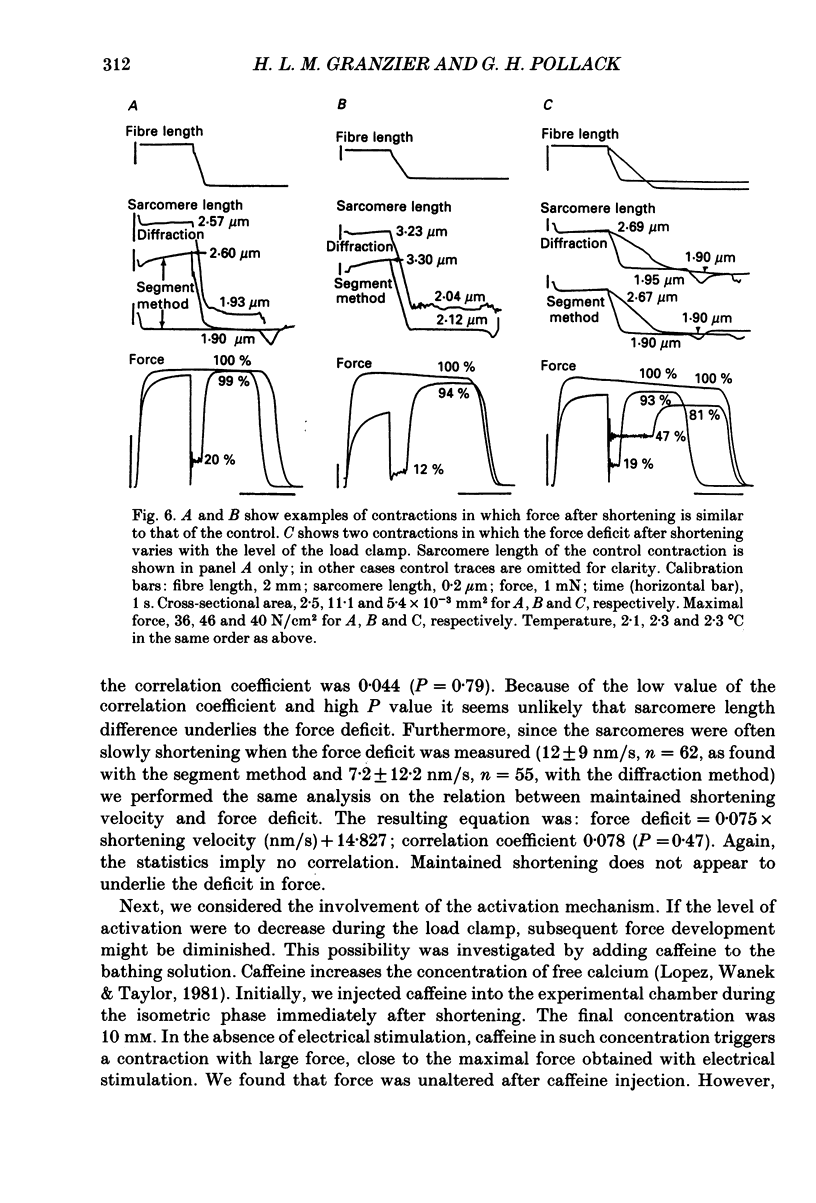
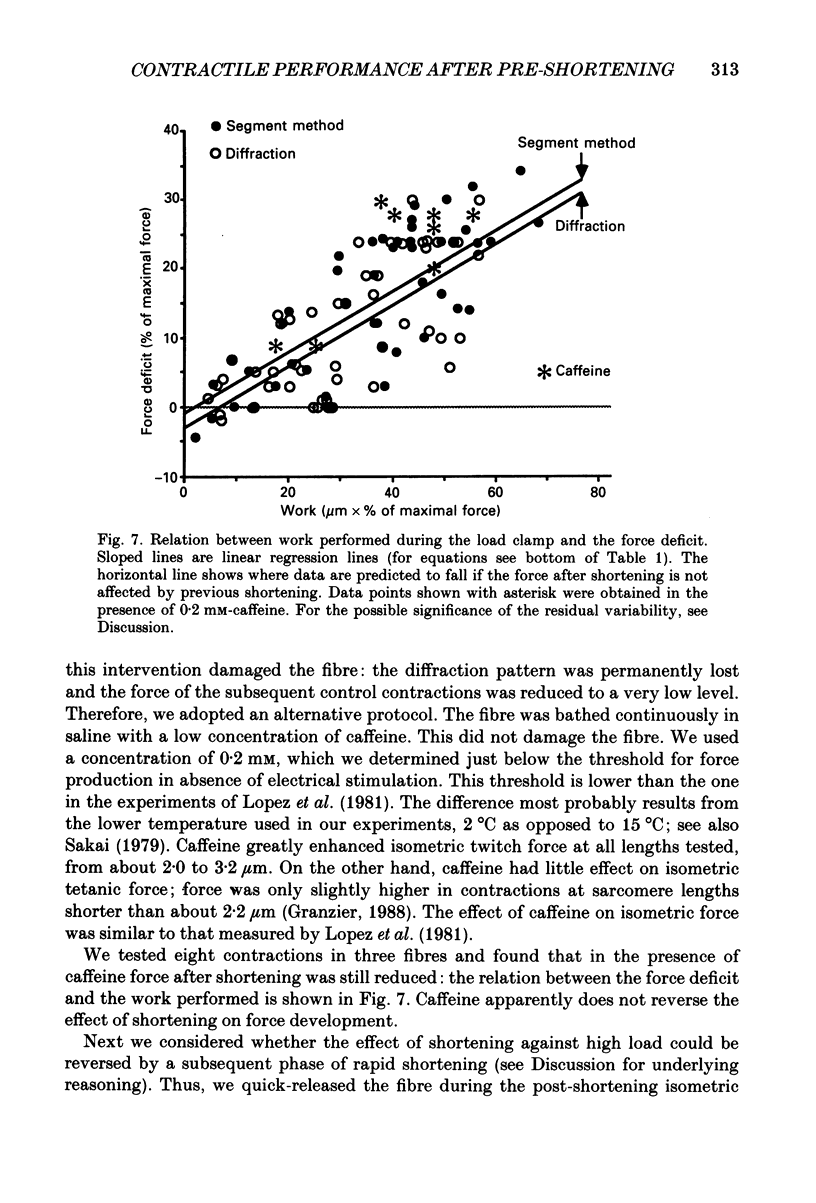
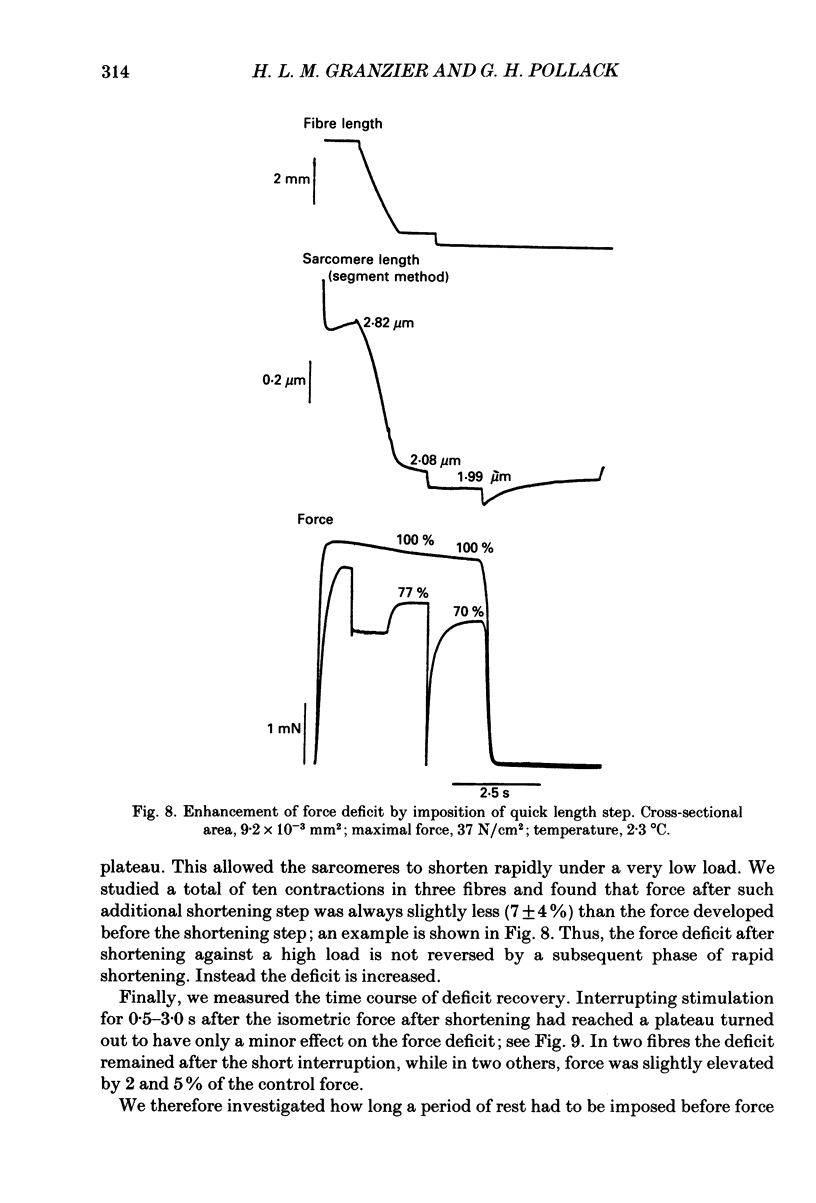
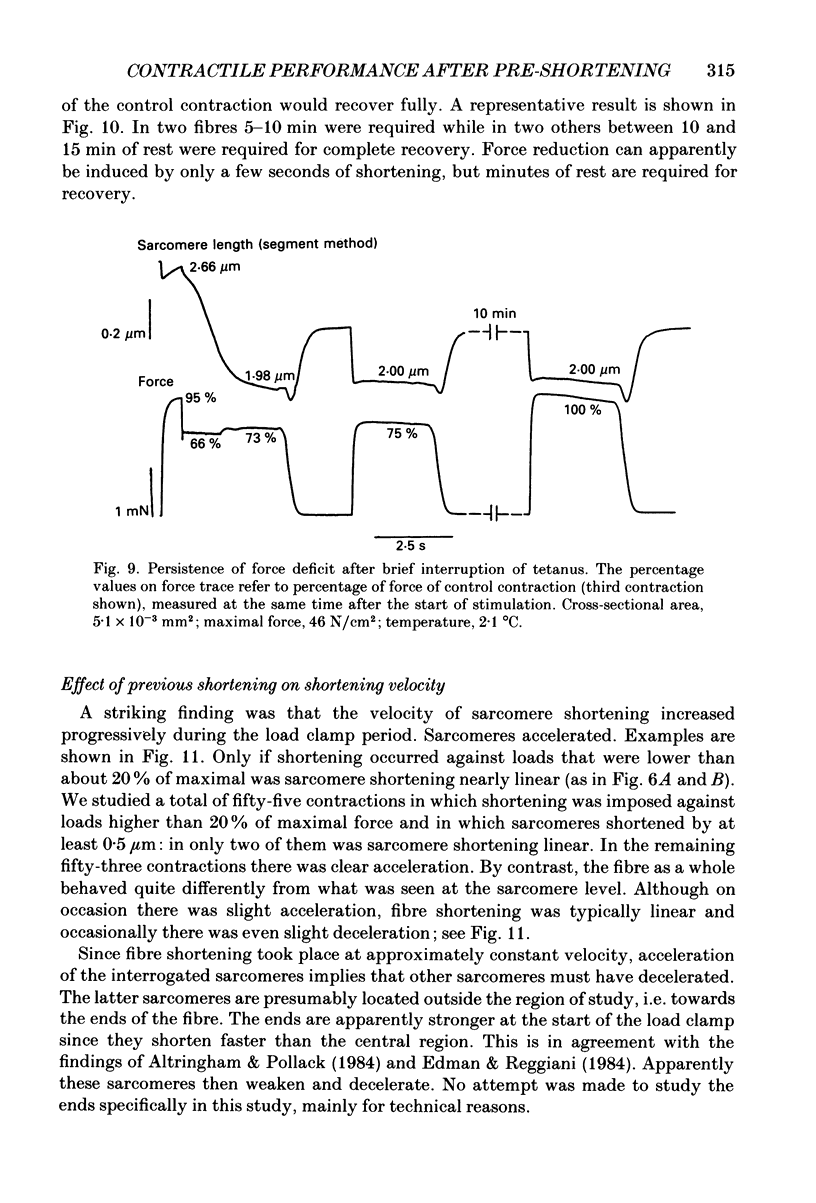
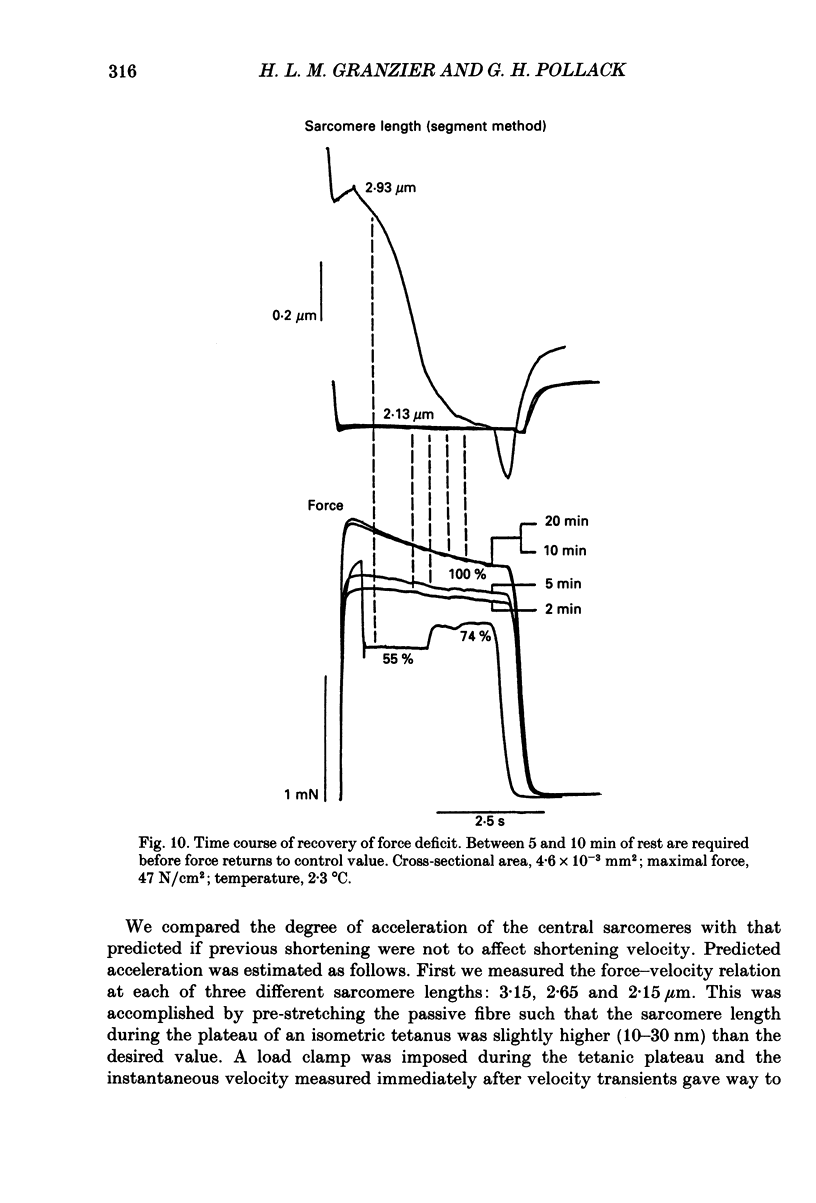
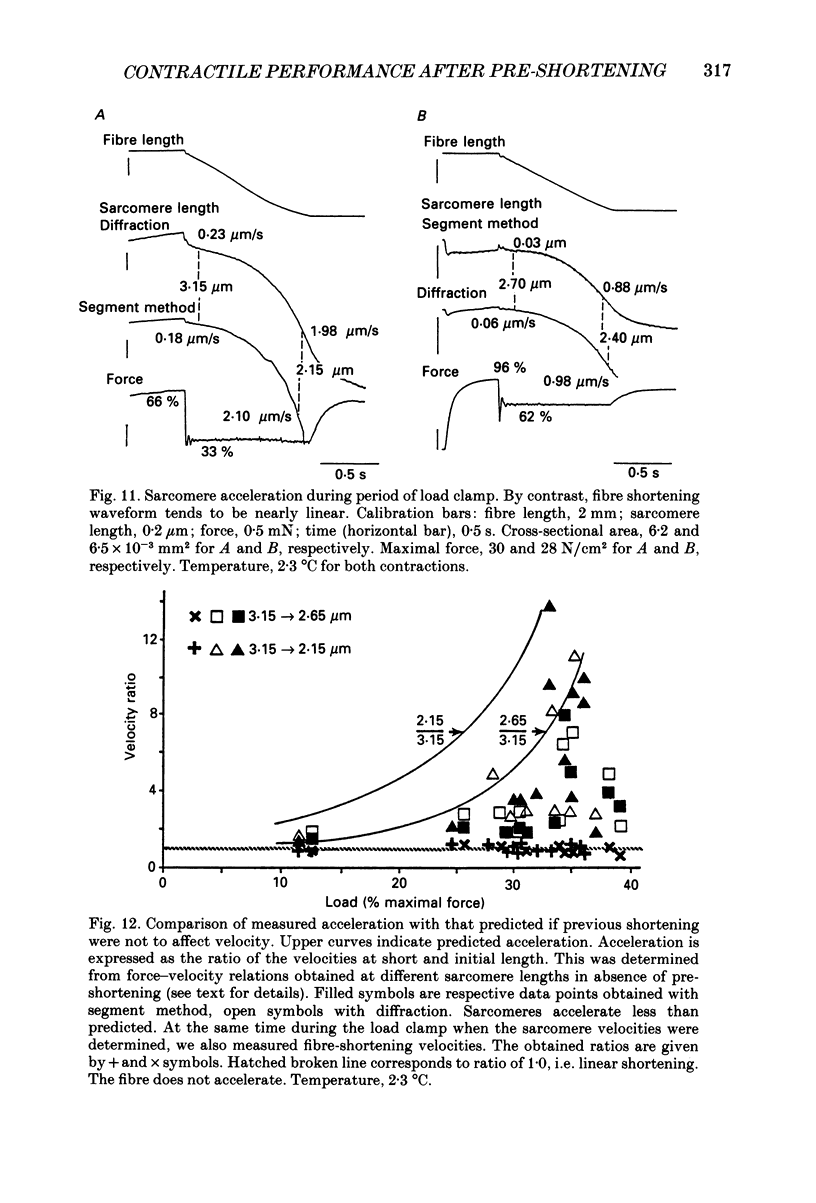
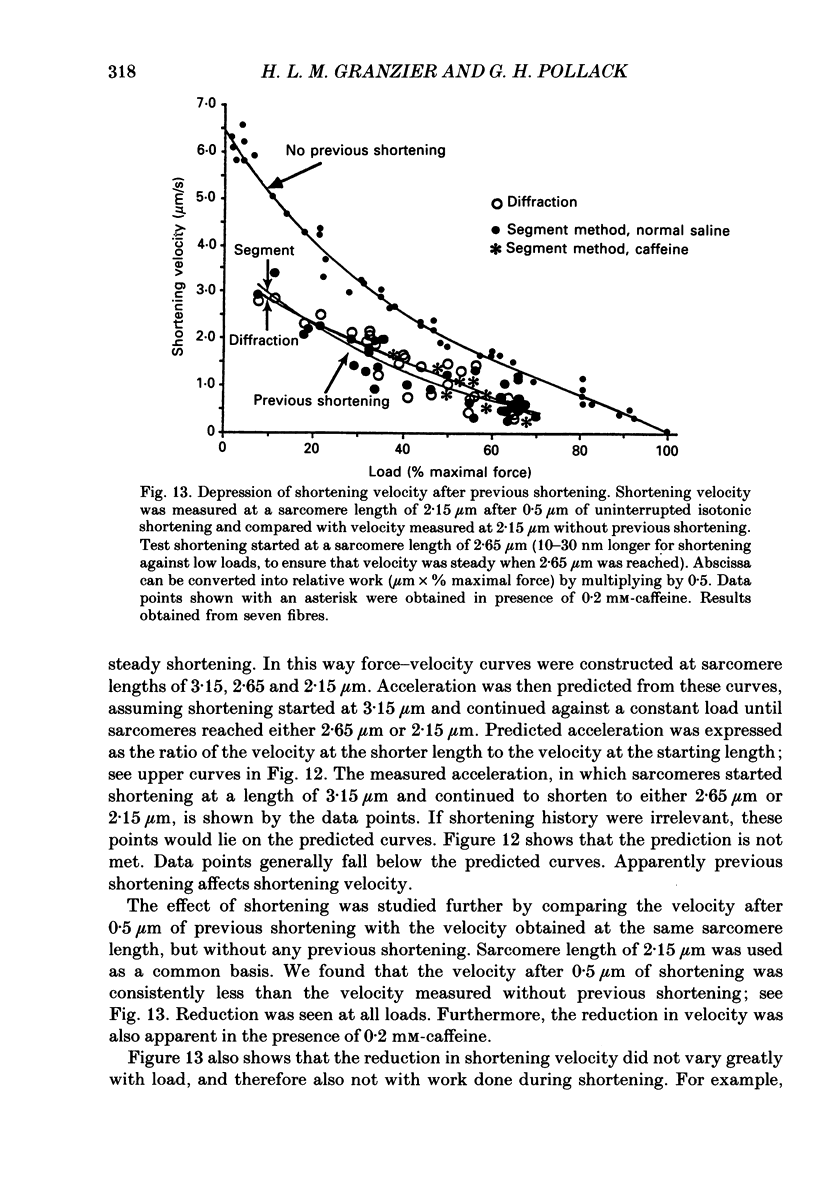
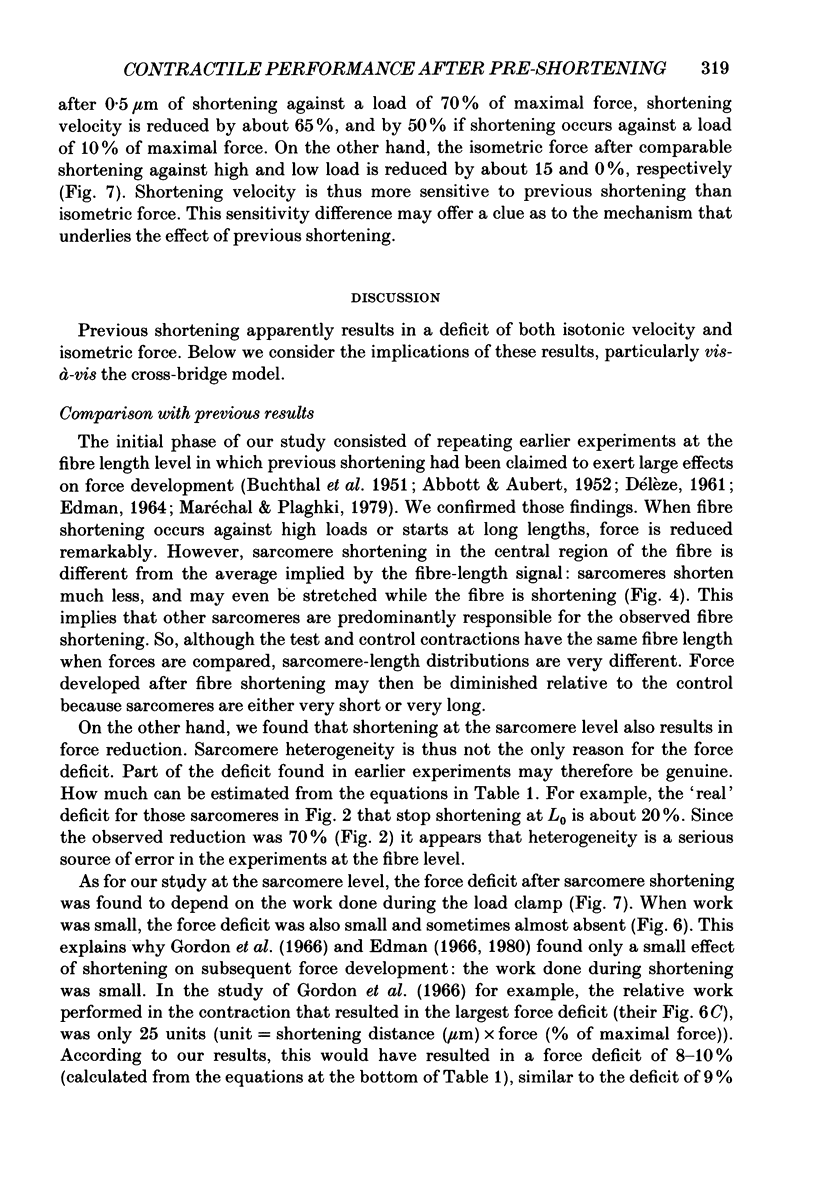
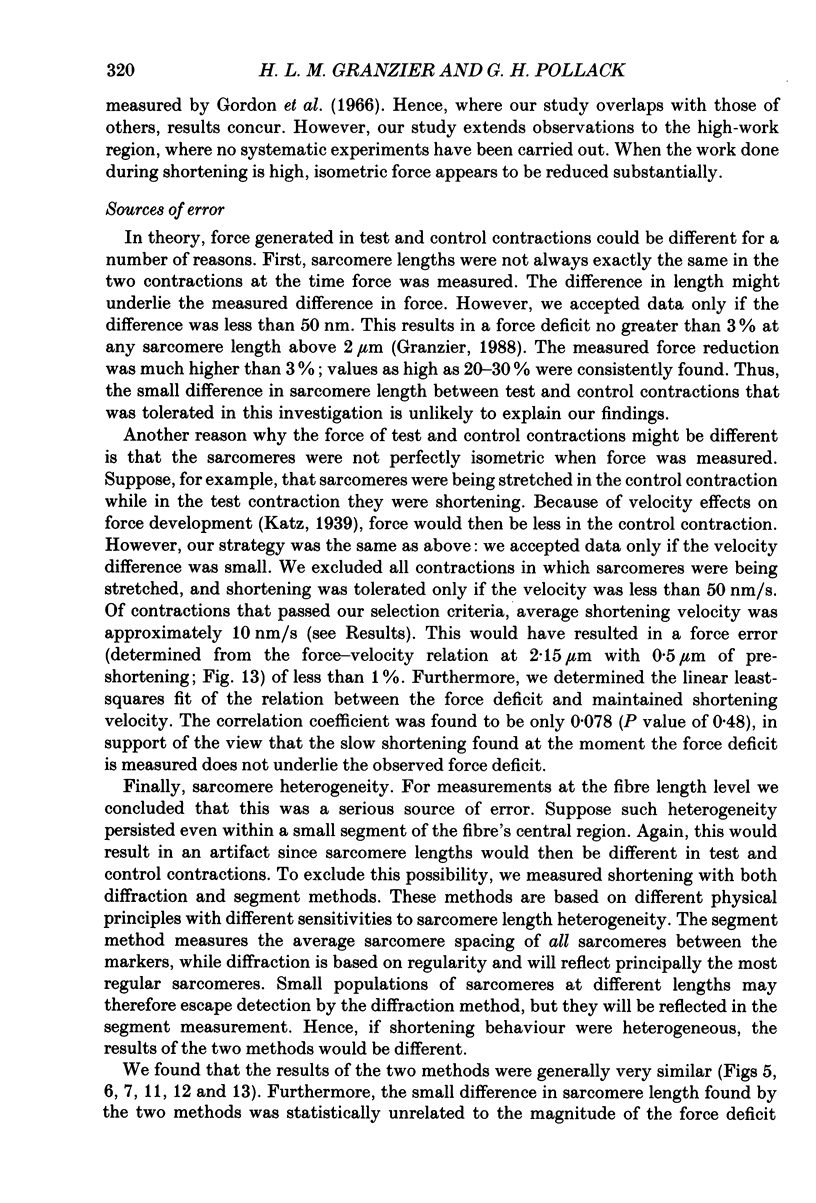
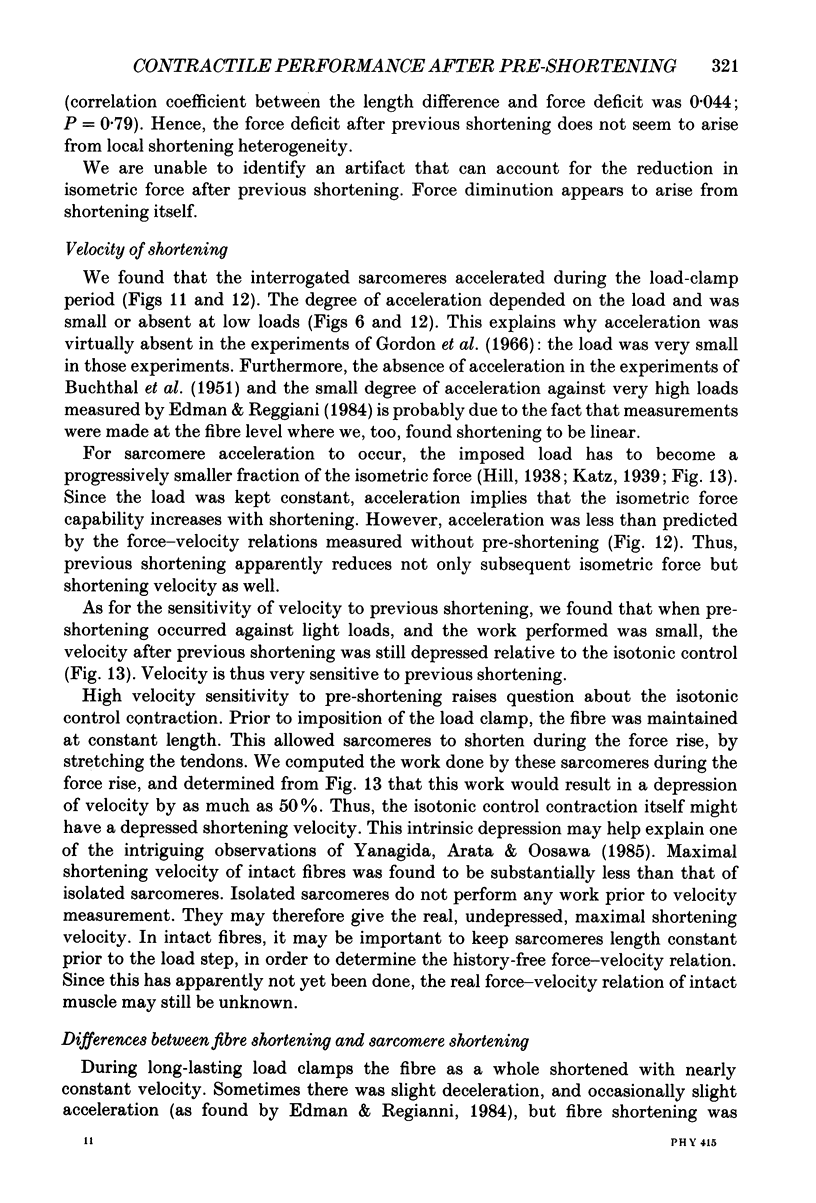
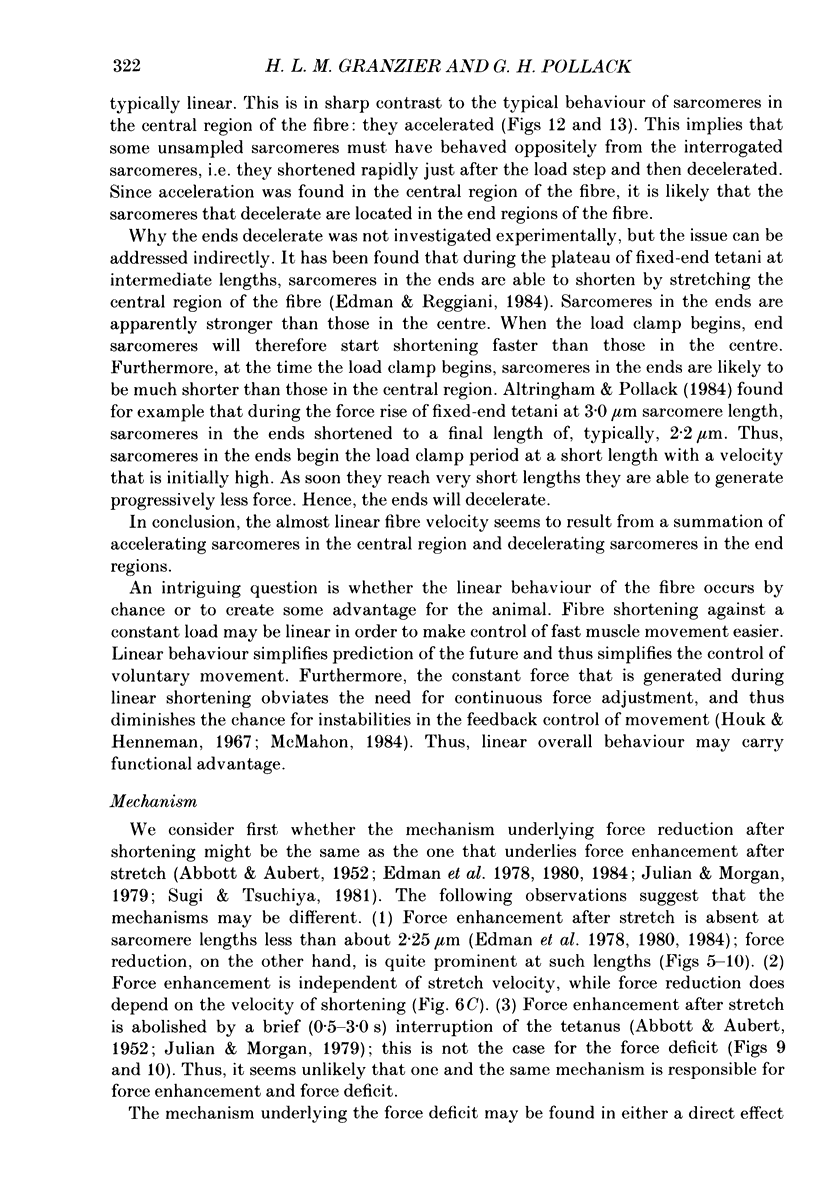
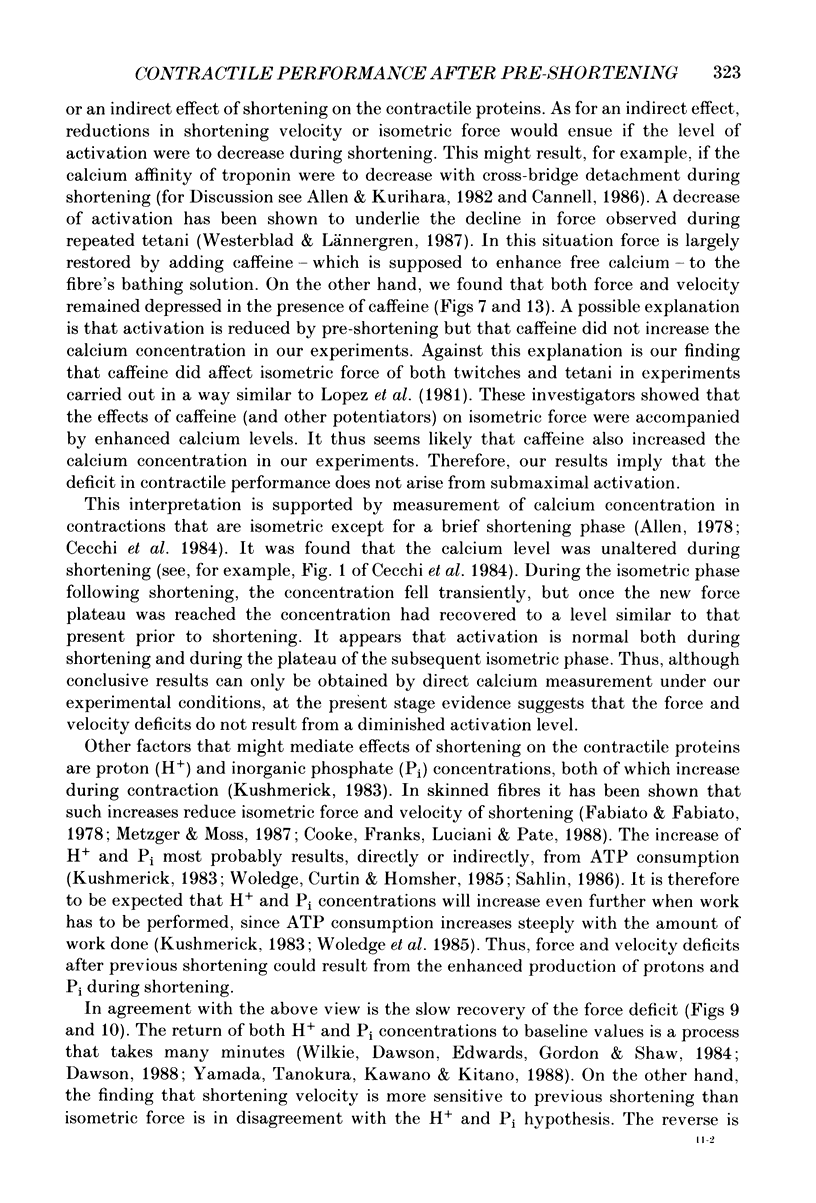
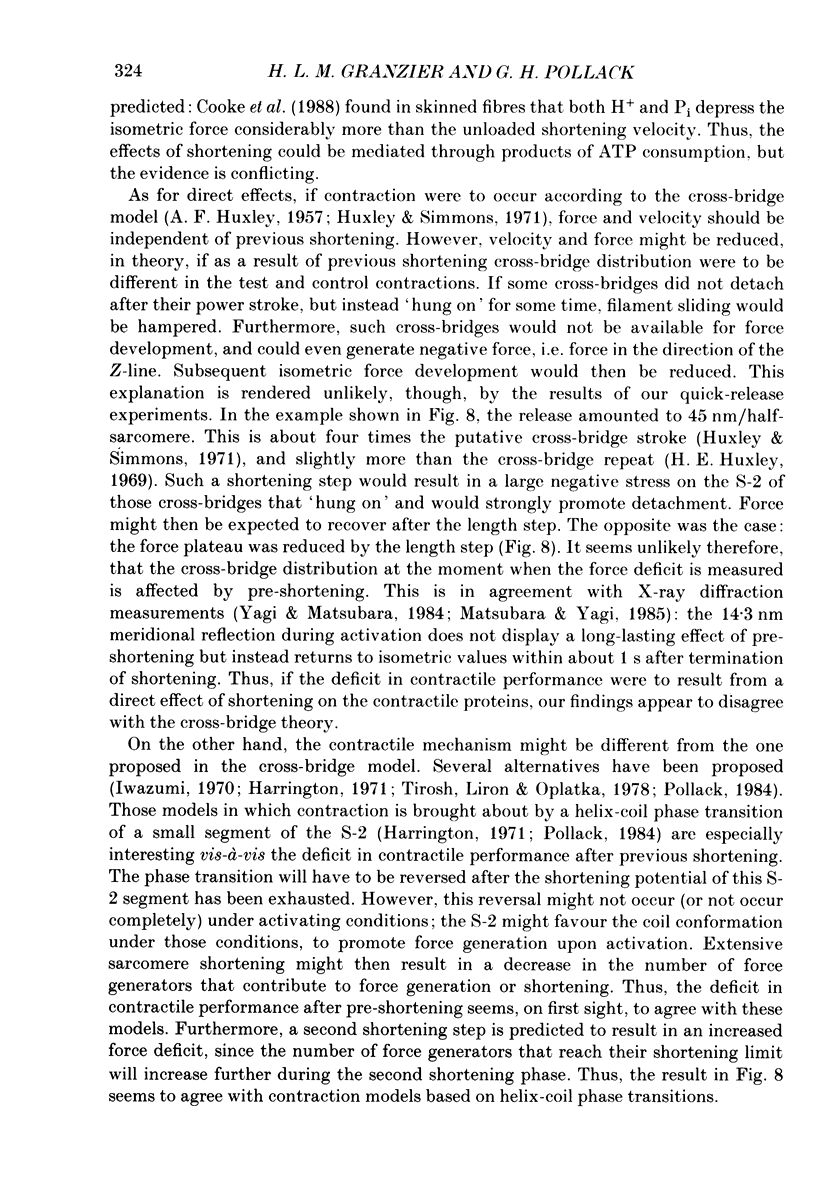
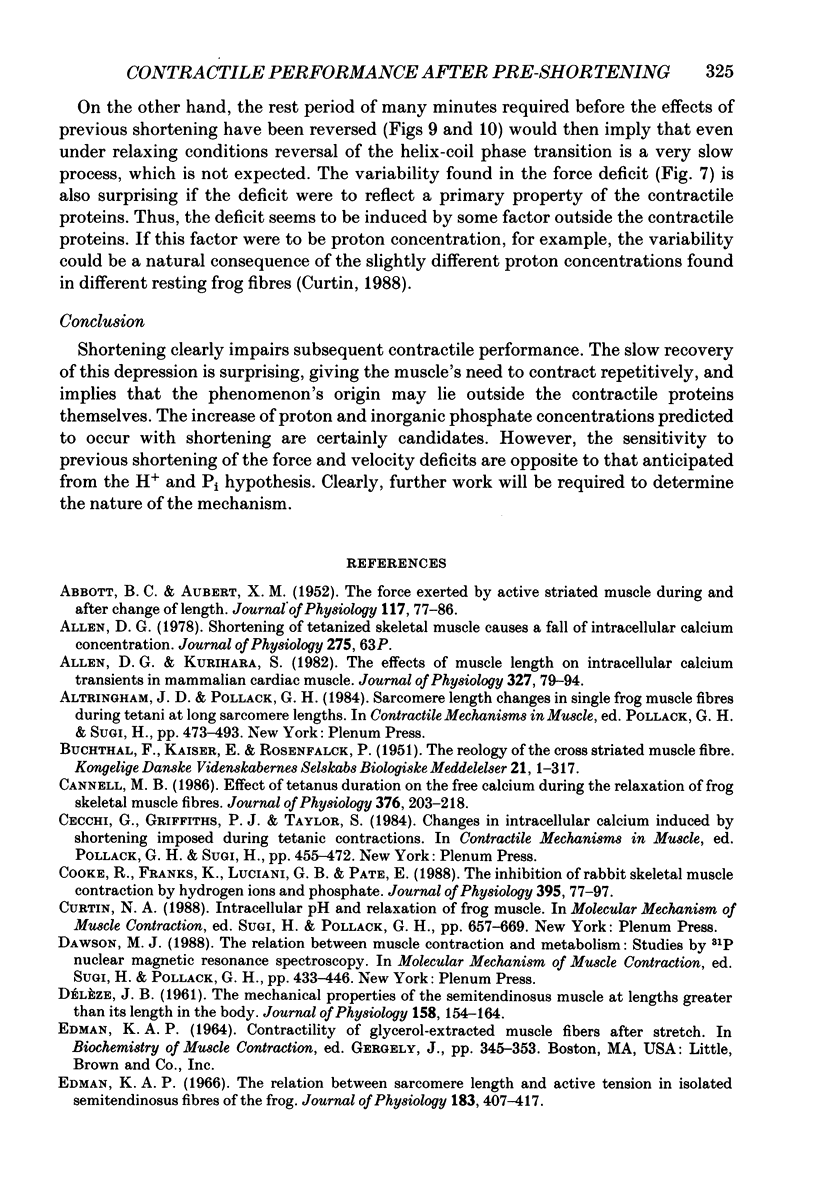
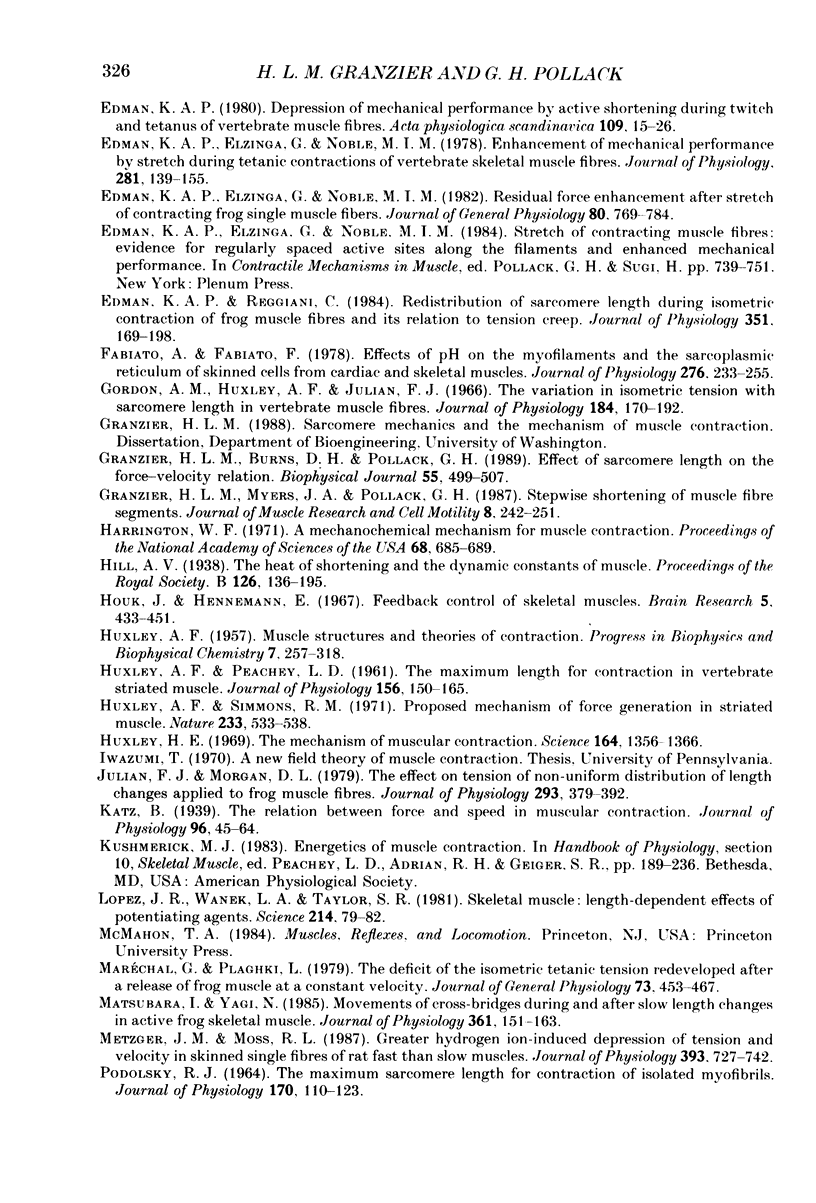
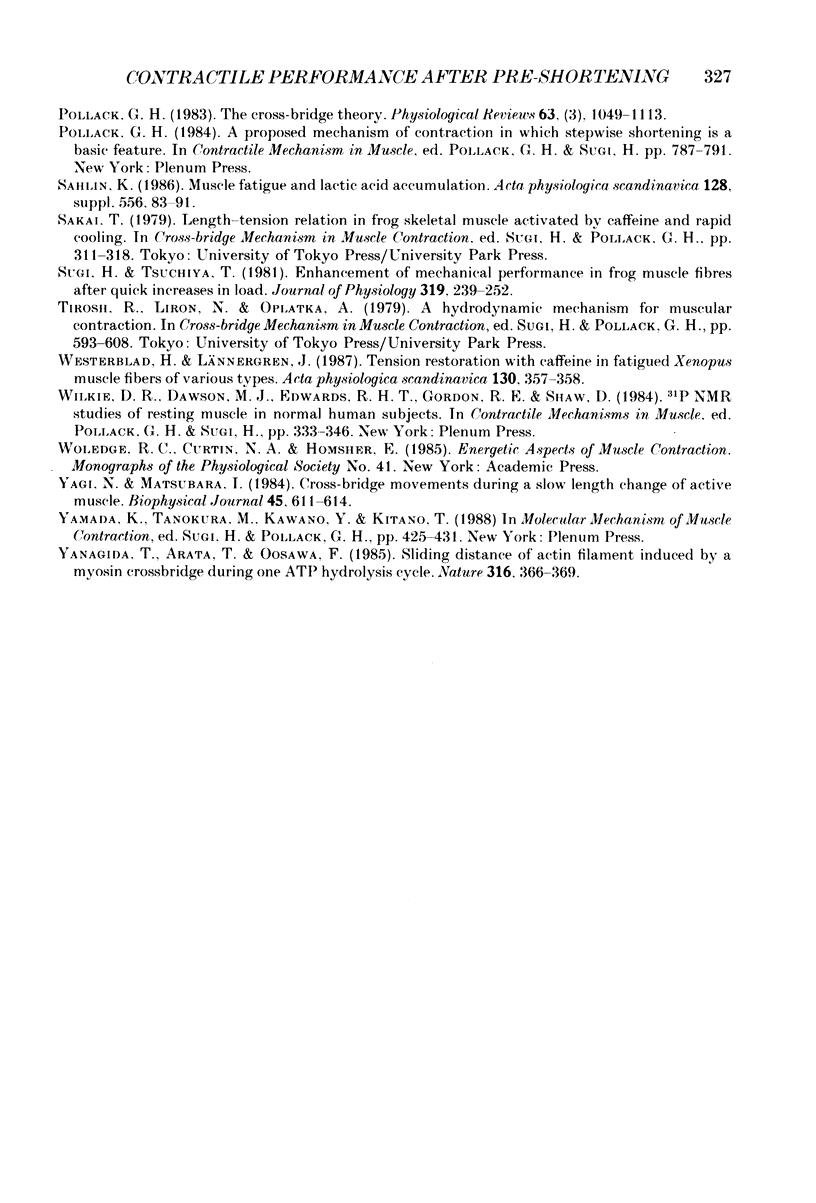
Selected References
These references are in PubMed. This may not be the complete list of references from this article.
- ABBOTT B. C., AUBERT X. M. The force exerted by active striated muscle during and after change of length. J Physiol. 1952 May;117(1):77–86. [PMC free article] [PubMed] [Google Scholar]
- Allen D. G., Kurihara S. The effects of muscle length on intracellular calcium transients in mammalian cardiac muscle. J Physiol. 1982 Jun;327:79–94. doi: 10.1113/jphysiol.1982.sp014221. [DOI] [PMC free article] [PubMed] [Google Scholar]
- Allen D. G. Shortening of tetanized skeletal muscle causes a fall of intracellular calcium concentration [proceedings]. J Physiol. 1978 Feb;275:63P–63P. [PubMed] [Google Scholar]
- Altringham J. D., Pollack G. H. Sarcomere length changes in single frog muscle fibres during tetani at long sarcomere lengths. Adv Exp Med Biol. 1984;170:473–493. doi: 10.1007/978-1-4684-4703-3_42. [DOI] [PubMed] [Google Scholar]
- Cannell M. B. Effect of tetanus duration on the free calcium during the relaxation of frog skeletal muscle fibres. J Physiol. 1986 Jul;376:203–218. doi: 10.1113/jphysiol.1986.sp016149. [DOI] [PMC free article] [PubMed] [Google Scholar]
- Cecchi G., Griffiths P. J., Taylor S. Changes in intracellular Ca2+ induced by shortening imposed during tetanic contractions. Adv Exp Med Biol. 1984;170:455–472. doi: 10.1007/978-1-4684-4703-3_41. [DOI] [PubMed] [Google Scholar]
- Cooke R., Franks K., Luciani G. B., Pate E. The inhibition of rabbit skeletal muscle contraction by hydrogen ions and phosphate. J Physiol. 1988 Jan;395:77–97. doi: 10.1113/jphysiol.1988.sp016909. [DOI] [PMC free article] [PubMed] [Google Scholar]
- Curtin N. A. Intracellular pH and relaxation of frog muscle. Adv Exp Med Biol. 1988;226:657–669. [PubMed] [Google Scholar]
- Dawson M. J. The relation between muscle contraction and metabolism: studies by 31P nuclear magnetic resonance spectroscopy. Adv Exp Med Biol. 1988;226:433–448. [PubMed] [Google Scholar]
- Edman K. A. Depression of mechanical performance by active shortening during twitch and tetanus of vertebrate muscle fibres. Acta Physiol Scand. 1980 May;109(1):15–26. doi: 10.1111/j.1748-1716.1980.tb06559.x. [DOI] [PubMed] [Google Scholar]
- Edman K. A., Elzinga G., Noble M. I. Enhancement of mechanical performance by stretch during tetanic contractions of vertebrate skeletal muscle fibres. J Physiol. 1978 Aug;281:139–155. doi: 10.1113/jphysiol.1978.sp012413. [DOI] [PMC free article] [PubMed] [Google Scholar]
- Edman K. A., Elzinga G., Noble M. I. Residual force enhancement after stretch of contracting frog single muscle fibers. J Gen Physiol. 1982 Nov;80(5):769–784. doi: 10.1085/jgp.80.5.769. [DOI] [PMC free article] [PubMed] [Google Scholar]
- Edman K. A., Elzinga G., Noble M. I. Stretch of contracting muscle fibres: evidence for regularly spaced active sites along the filaments and enhanced mechanical performance. Adv Exp Med Biol. 1984;170:739–751. doi: 10.1007/978-1-4684-4703-3_71. [DOI] [PubMed] [Google Scholar]
- Edman K. A., Reggiani C. Redistribution of sarcomere length during isometric contraction of frog muscle fibres and its relation to tension creep. J Physiol. 1984 Jun;351:169–198. doi: 10.1113/jphysiol.1984.sp015240. [DOI] [PMC free article] [PubMed] [Google Scholar]
- Edman K. A. The relation between sarcomere length and active tension in isolated semitendinosus fibres of the frog. J Physiol. 1966 Mar;183(2):407–417. doi: 10.1113/jphysiol.1966.sp007873. [DOI] [PMC free article] [PubMed] [Google Scholar]
- Fabiato A., Fabiato F. Effects of pH on the myofilaments and the sarcoplasmic reticulum of skinned cells from cardiace and skeletal muscles. J Physiol. 1978 Mar;276:233–255. doi: 10.1113/jphysiol.1978.sp012231. [DOI] [PMC free article] [PubMed] [Google Scholar]
- Gordon A. M., Huxley A. F., Julian F. J. The variation in isometric tension with sarcomere length in vertebrate muscle fibres. J Physiol. 1966 May;184(1):170–192. doi: 10.1113/jphysiol.1966.sp007909. [DOI] [PMC free article] [PubMed] [Google Scholar]
- Granzier H. L., Burns D. H., Pollack G. H. Sarcomere length dependence of the force-velocity relation in single frog muscle fibers. Biophys J. 1989 Mar;55(3):499–507. doi: 10.1016/S0006-3495(89)82843-7. [DOI] [PMC free article] [PubMed] [Google Scholar]
- Granzier H. L., Myers J. A., Pollack G. H. Stepwise shortening of muscle fibre segments. J Muscle Res Cell Motil. 1987 Jun;8(3):242–251. doi: 10.1007/BF01574592. [DOI] [PubMed] [Google Scholar]
- HUXLEY A. F., PEACHEY L. D. The maximum length for contraction in vertebrate straiated muscle. J Physiol. 1961 Apr;156:150–165. doi: 10.1113/jphysiol.1961.sp006665. [DOI] [PMC free article] [PubMed] [Google Scholar]
- Harrington W. F. A mechanochemical mechanism for muscle contraction. Proc Natl Acad Sci U S A. 1971 Mar;68(3):685–689. doi: 10.1073/pnas.68.3.685. [DOI] [PMC free article] [PubMed] [Google Scholar]
- Houk J., Henneman E. Feedback control of skeletal muscles. Brain Res. 1967 Aug;5(4):433–451. doi: 10.1016/0006-8993(67)90014-5. [DOI] [PubMed] [Google Scholar]
- Huxley A. F., Simmons R. M. Proposed mechanism of force generation in striated muscle. Nature. 1971 Oct 22;233(5321):533–538. doi: 10.1038/233533a0. [DOI] [PubMed] [Google Scholar]
- Julian F. J., Morgan D. L. The effect on tension of non-uniform distribution of length changes applied to frog muscle fibres. J Physiol. 1979 Aug;293:379–392. doi: 10.1113/jphysiol.1979.sp012895. [DOI] [PMC free article] [PubMed] [Google Scholar]
- Katz B. The relation between force and speed in muscular contraction. J Physiol. 1939 Jun 14;96(1):45–64. doi: 10.1113/jphysiol.1939.sp003756. [DOI] [PMC free article] [PubMed] [Google Scholar]
- Lopez J. R., Wanek L. A., Taylor S. R. Skeletal muscle: length-dependent effects of potentiating agents. Science. 1981 Oct 2;214(4516):79–82. doi: 10.1126/science.6974399. [DOI] [PubMed] [Google Scholar]
- Maréchal G., Plaghki L. The deficit of the isometric tetanic tension redeveloped after a release of frog muscle at a constant velocity. J Gen Physiol. 1979 Apr;73(4):453–467. doi: 10.1085/jgp.73.4.453. [DOI] [PMC free article] [PubMed] [Google Scholar]
- Matsubara I., Yagi N. Movements of cross-bridges during and after slow length changes in active frog skeletal muscle. J Physiol. 1985 Apr;361:151–163. doi: 10.1113/jphysiol.1985.sp015638. [DOI] [PMC free article] [PubMed] [Google Scholar]
- Metzger J. M., Moss R. L. Greater hydrogen ion-induced depression of tension and velocity in skinned single fibres of rat fast than slow muscles. J Physiol. 1987 Dec;393:727–742. doi: 10.1113/jphysiol.1987.sp016850. [DOI] [PMC free article] [PubMed] [Google Scholar]
- PODOLSKY R. J. THE MAXIMUM SARCOMERE LENGTH FOR CONTRACTION OF ISOLATED MYOFIBRILS. J Physiol. 1964 Jan;170:110–123. doi: 10.1113/jphysiol.1964.sp007317. [DOI] [PMC free article] [PubMed] [Google Scholar]
- Pollack G. H. A proposed mechanism of contraction in which stepwise shortening is a basic feature. Adv Exp Med Biol. 1984;170:787–792. doi: 10.1007/978-1-4684-4703-3_75. [DOI] [PubMed] [Google Scholar]
- Sugi H., Tsuchiya T. Enhancement of mechanical performance in frog muscle fibres after quick increases in load. J Physiol. 1981;319:239–252. doi: 10.1113/jphysiol.1981.sp013904. [DOI] [PMC free article] [PubMed] [Google Scholar]
- Westerblad H., Lännergren J. Tension restoration with caffeine in fatigued Xenopus muscle fibres of various types. Acta Physiol Scand. 1987 Jun;130(2):357–358. doi: 10.1111/j.1748-1716.1987.tb08148.x. [DOI] [PubMed] [Google Scholar]
- Wilkie D. R., Dawson M. J., Edwards R. H., Gordon R. E., Shaw D. 31P NMR studies of resting muscle in normal human subjects. Adv Exp Med Biol. 1984;170:333–347. doi: 10.1007/978-1-4684-4703-3_28. [DOI] [PubMed] [Google Scholar]
- Yagi N., Matsubara I. Cross-bridge movements during a slow length change of active muscle. Biophys J. 1984 Mar;45(3):611–614. doi: 10.1016/S0006-3495(84)84199-5. [DOI] [PMC free article] [PubMed] [Google Scholar]
- Yamada K., Tanokura M., Kawano Y., Kitano T. Time-resolved 31P nuclear magnetic resonance studies on isometric contraction of frog skeletal muscle. Adv Exp Med Biol. 1988;226:425–432. [PubMed] [Google Scholar]
- Yanagida T., Arata T., Oosawa F. Sliding distance of actin filament induced by a myosin crossbridge during one ATP hydrolysis cycle. Nature. 1985 Jul 25;316(6026):366–369. doi: 10.1038/316366a0. [DOI] [PubMed] [Google Scholar]