Abstract
1. The whole-cell and outside-out patch configurations of the patch-clamp technique were used to investigate the effects of extracellular tetraethylammonium ions (TEA+) and quinine on both Ca2(+)-activated and ATP-regulated K+ channels in mouse pancreatic beta-cells. 2. The Ca2(+)-activated K+ channel has a single-channel K+ permeability of 4.7 x 10(-13) cm3 s-1 when recorded with physiological ionic gradients. This value decreased to 2.9 x 10(-13) cm3 s-1 after addition of 0.3 mM-TEA+. 3. Two exponentials with time constants of 0.2 and 4.7 ms were required to describe the distribution of the channel openings suggesting that the Ca2(+)-activated K+ channel has at least two open states. The fast and slow components comprised 16 and 84% of the total number of openings respectively. 4. TEA+ caused a concentration-dependent decrease in the single-channel amplitude and open probability of the Ca2(+)-activated K+ channel. A Kd for the reduction in the mean current of 0.14 mM was observed. The stoichiometry was approximately 1:1. 5. Quinine blocked the Ca2(+)-activated K+ channel in a concentration-dependent manner. Half-maximal block was observed at 0.10 mM and binding was 1:1. Inhibition by 20 microM-quinine was not associated with a decrease in channel amplitude but markedly reduced the lifetime of the channel openings. Two exponentials, with time constants of 0.5 and 1.3 ms, were required to describe the channel openings. The rapid component contained 55% of the events. 6. TEA+ reduced the single-channel amplitude of the ATP-regulated K+ channel in a concentration-dependent manner. Kd for the block was 22 mM and the binding approximately 1:1. The block was not associated with changes in the open probability or channel kinetics. Two exponentials were required to describe the distribution of the open times. The time constants for the fast and slow components were approximately 2 and approximately 20 ms respectively. The rapid component accounted for approximately 35% of the events. 7. Quinine (10-20 microM) almost abolished activity of the ATP-regulated K+ channels. Inhibition was characterized by slow onset and reversibility but not associated with a change in the appearance of the single-channel events. Quinine-induced block could not be reversed by diazoxide. 8. We conclude that TEA+ produces rapid block of both Ca2(+)-activated and ATP-regulated K+ channels.(ABSTRACT TRUNCATED AT 400 WORDS)
Full text
PDF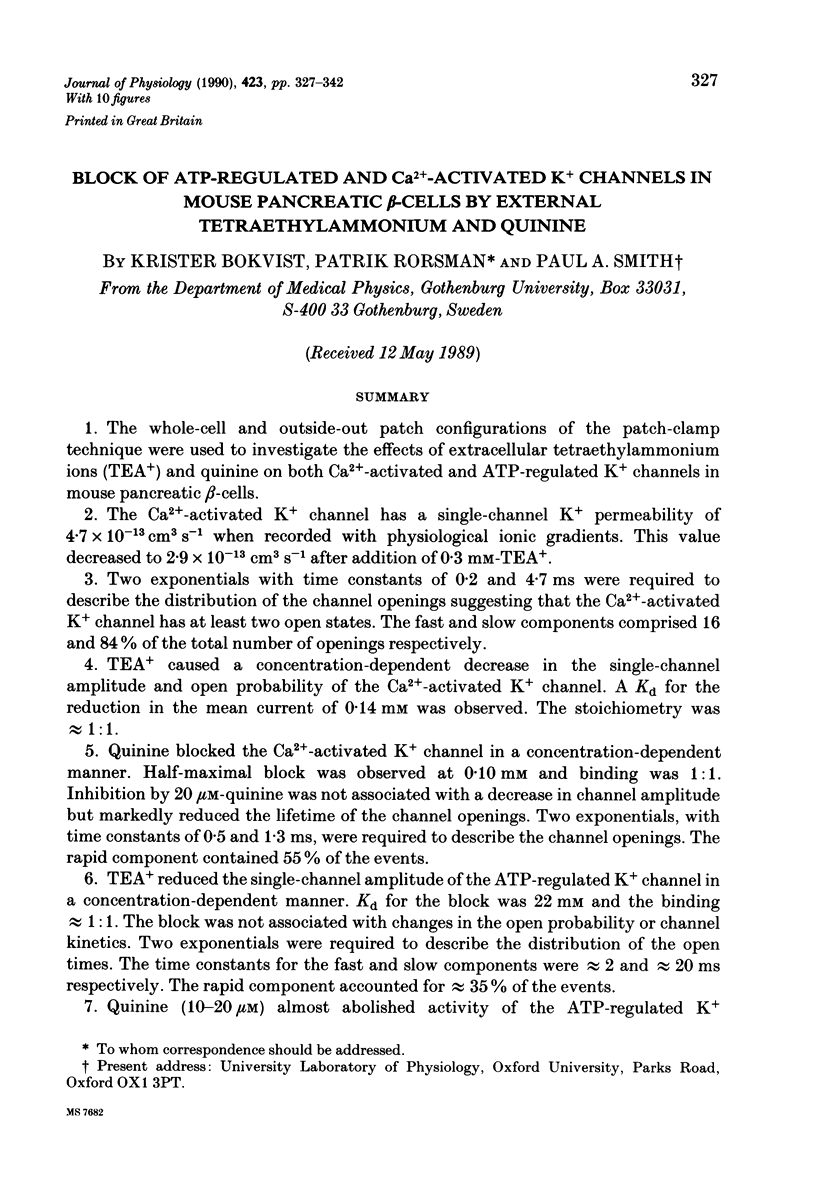
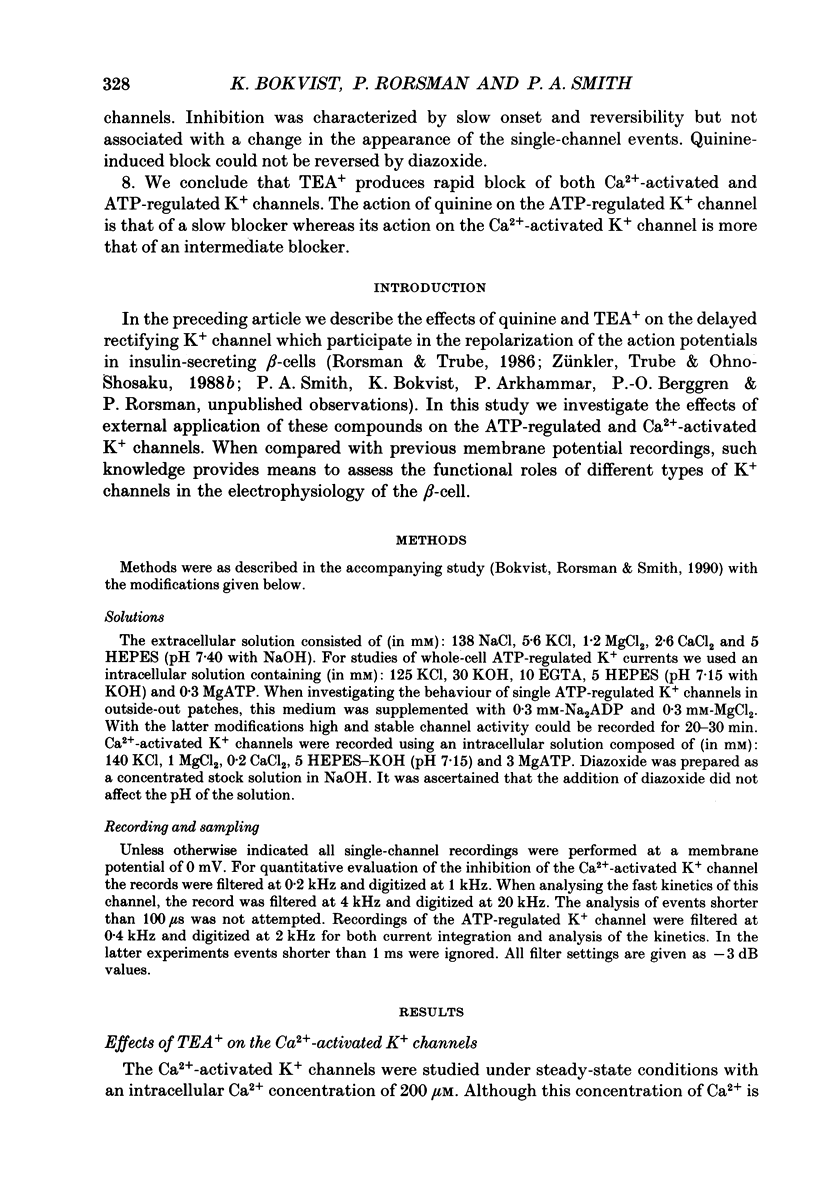
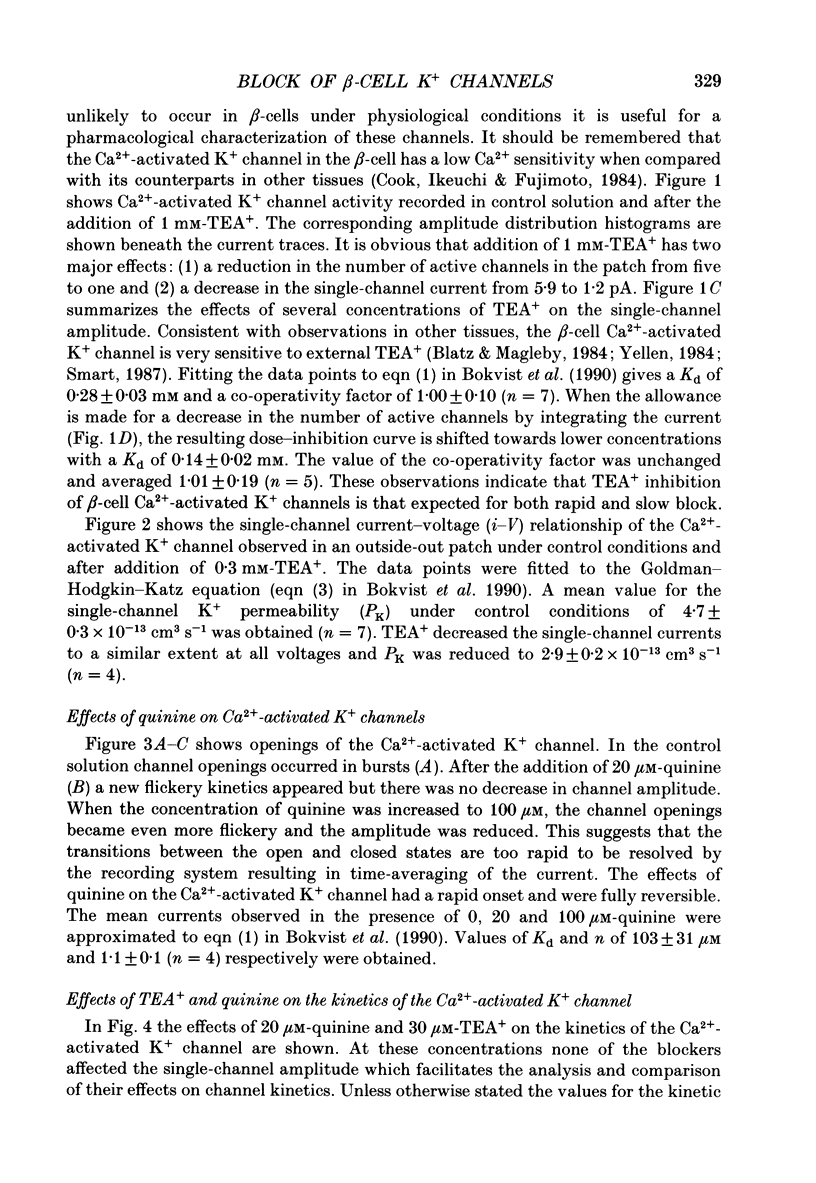
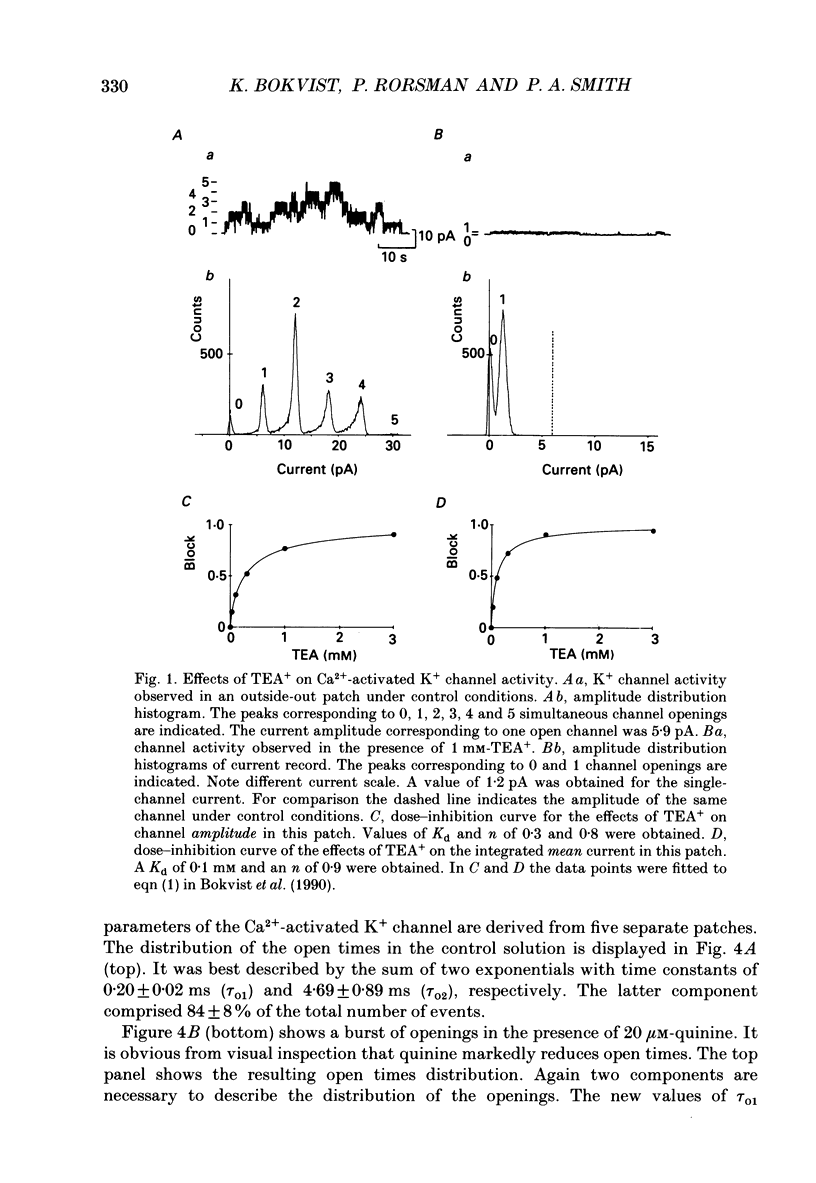
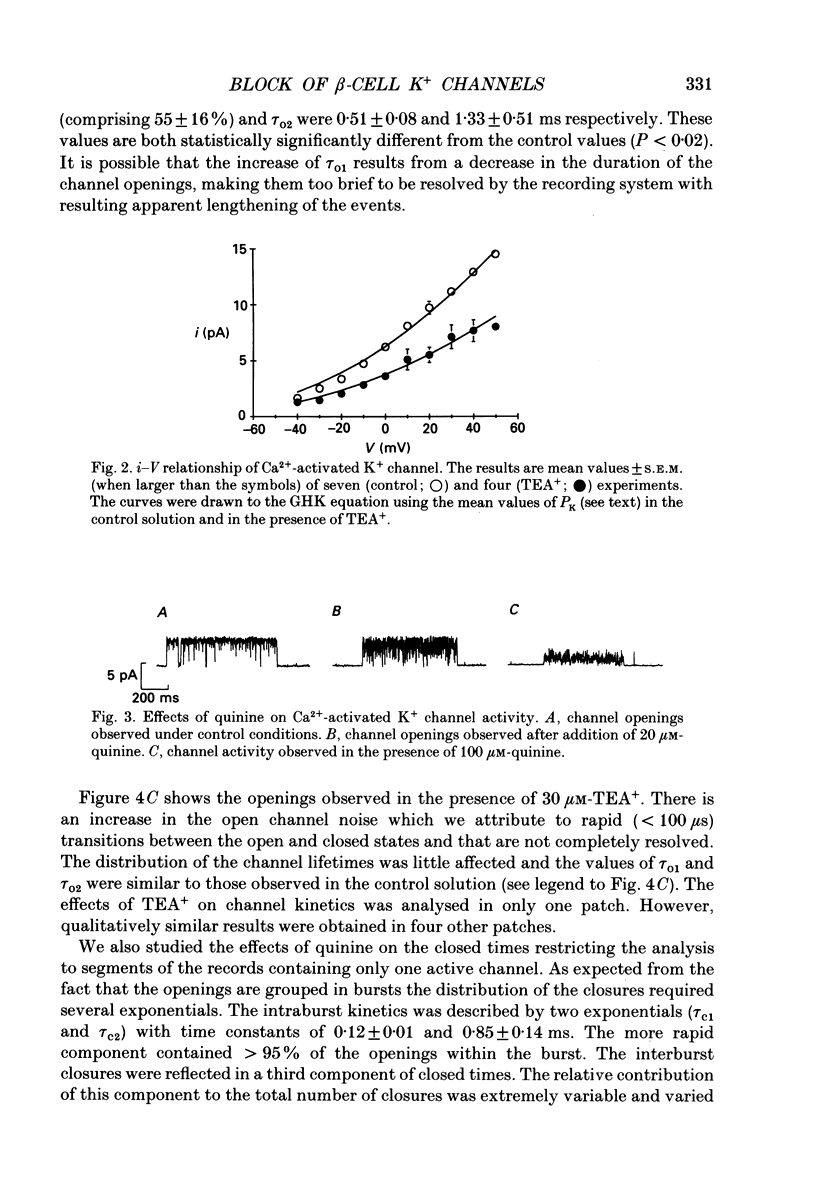
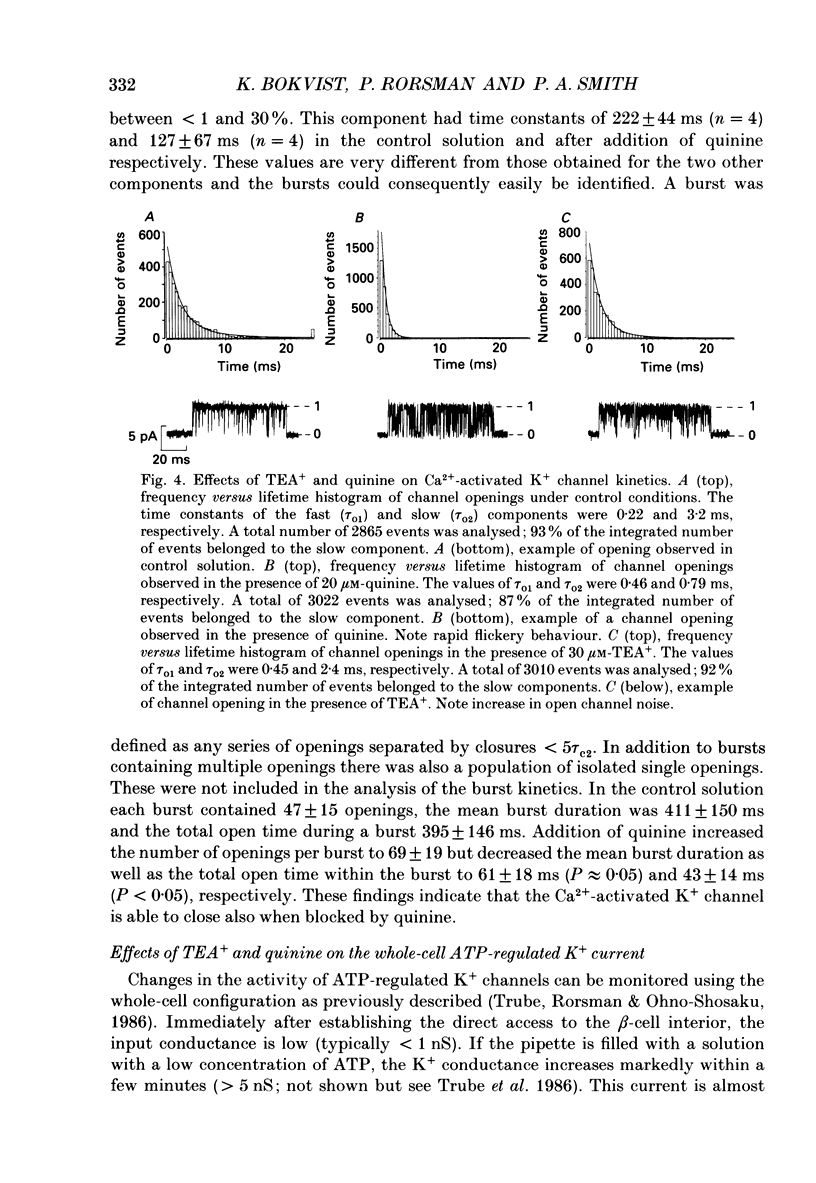
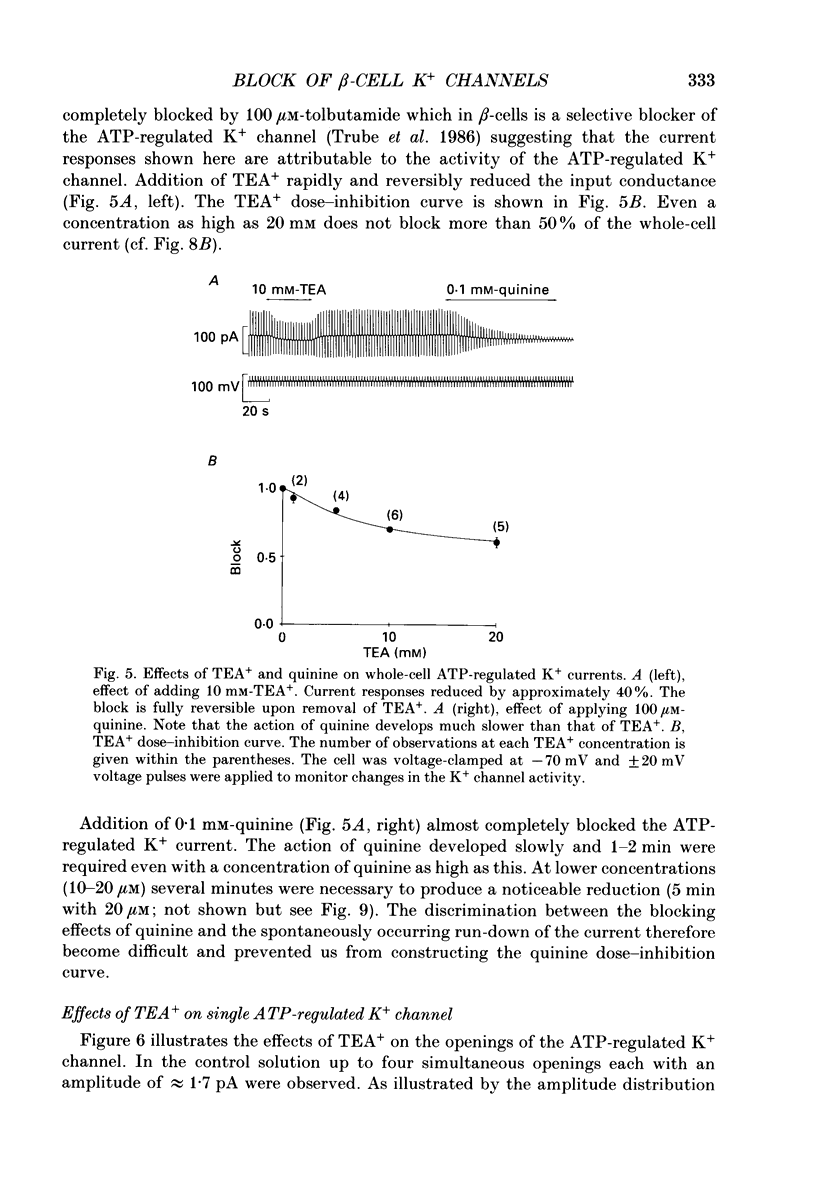
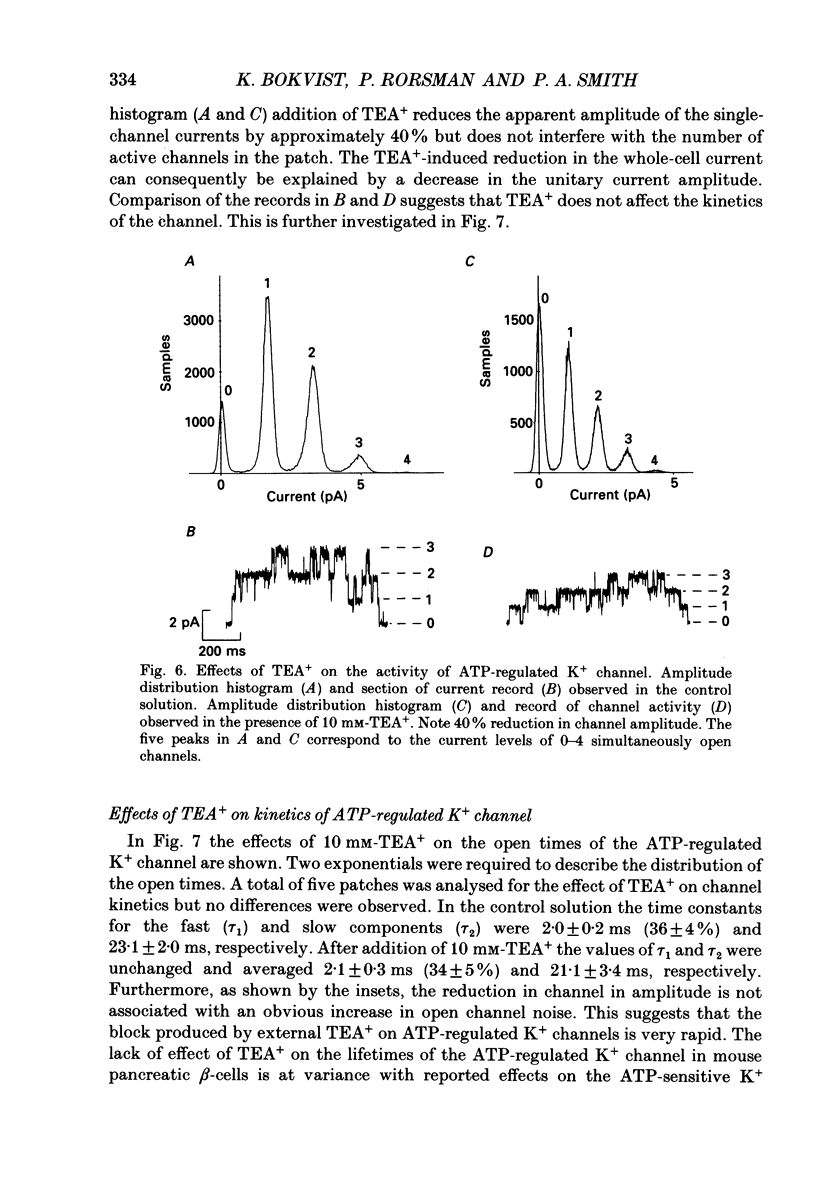
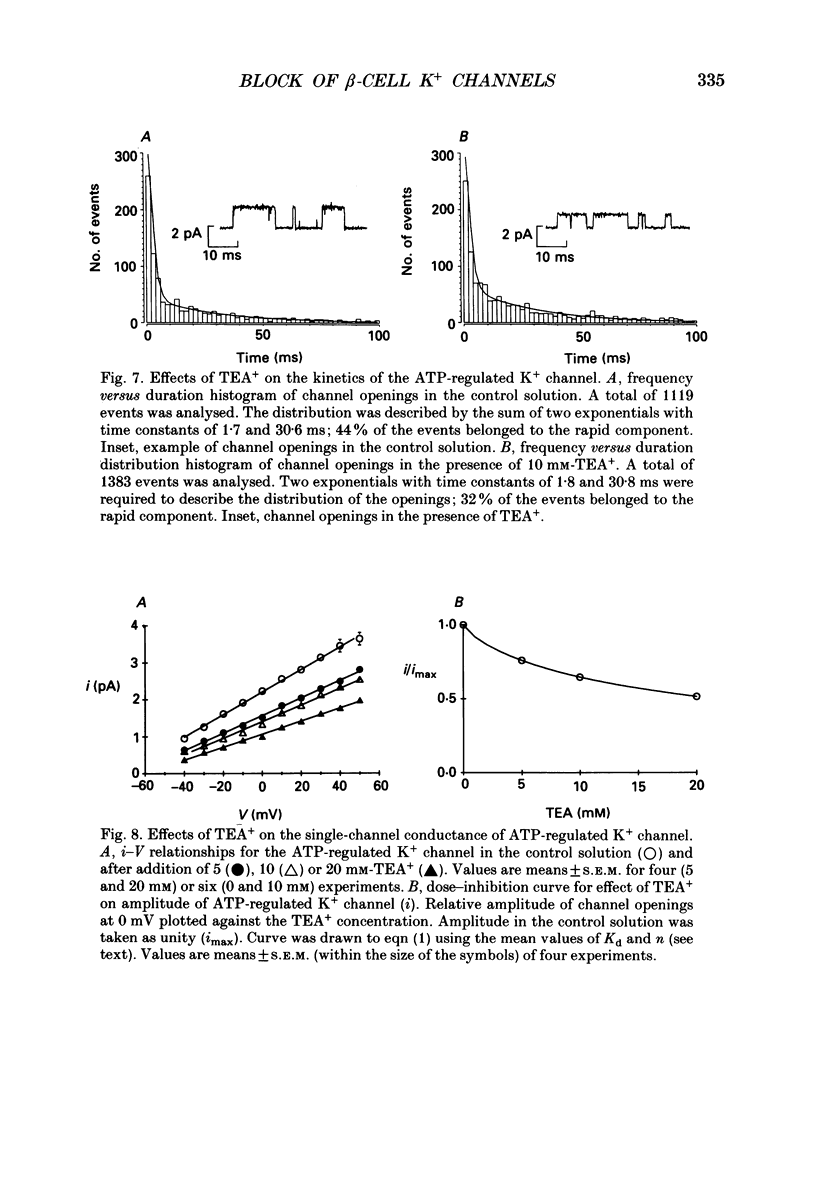
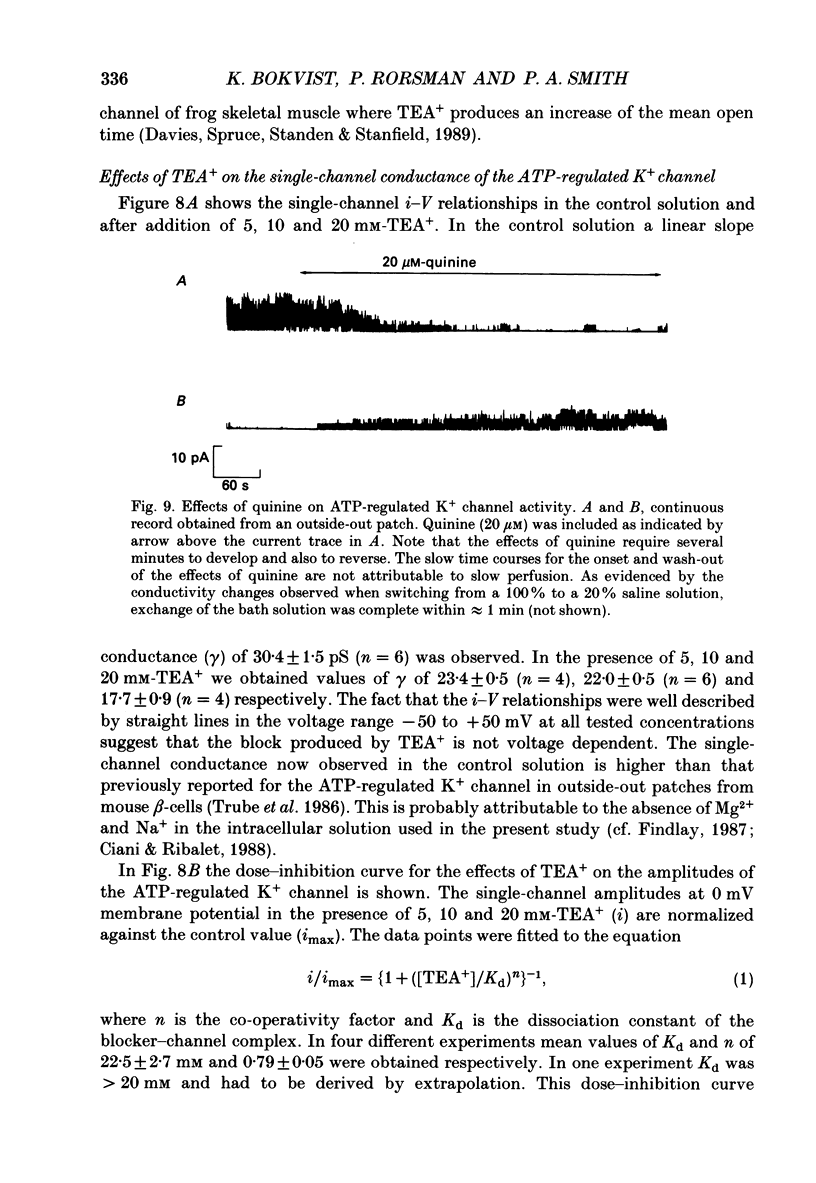
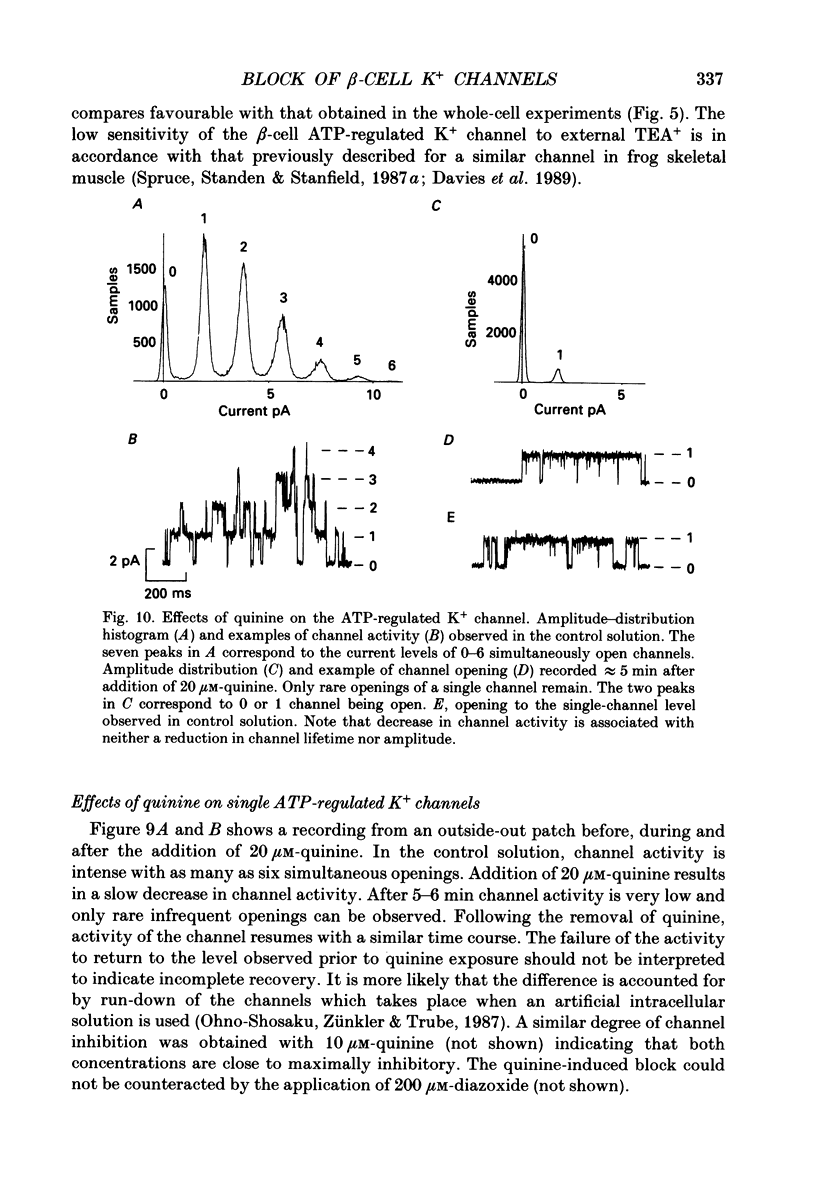
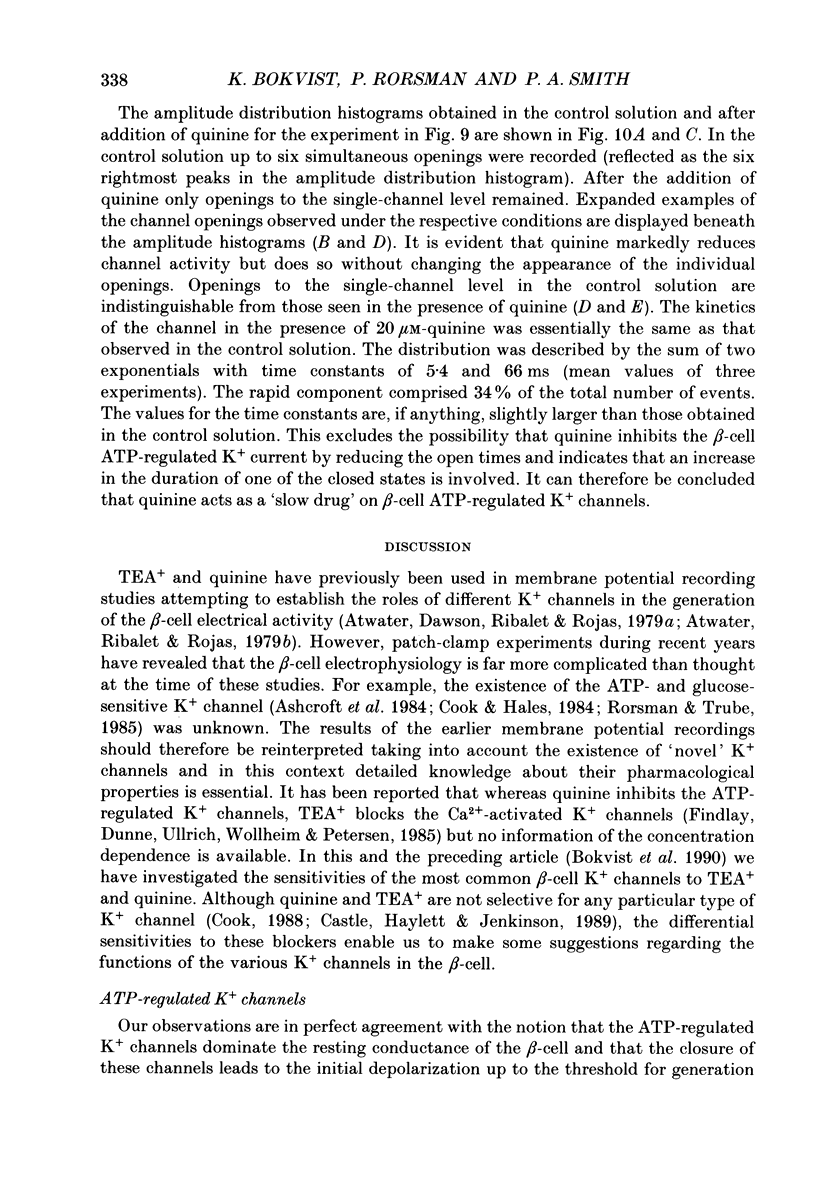
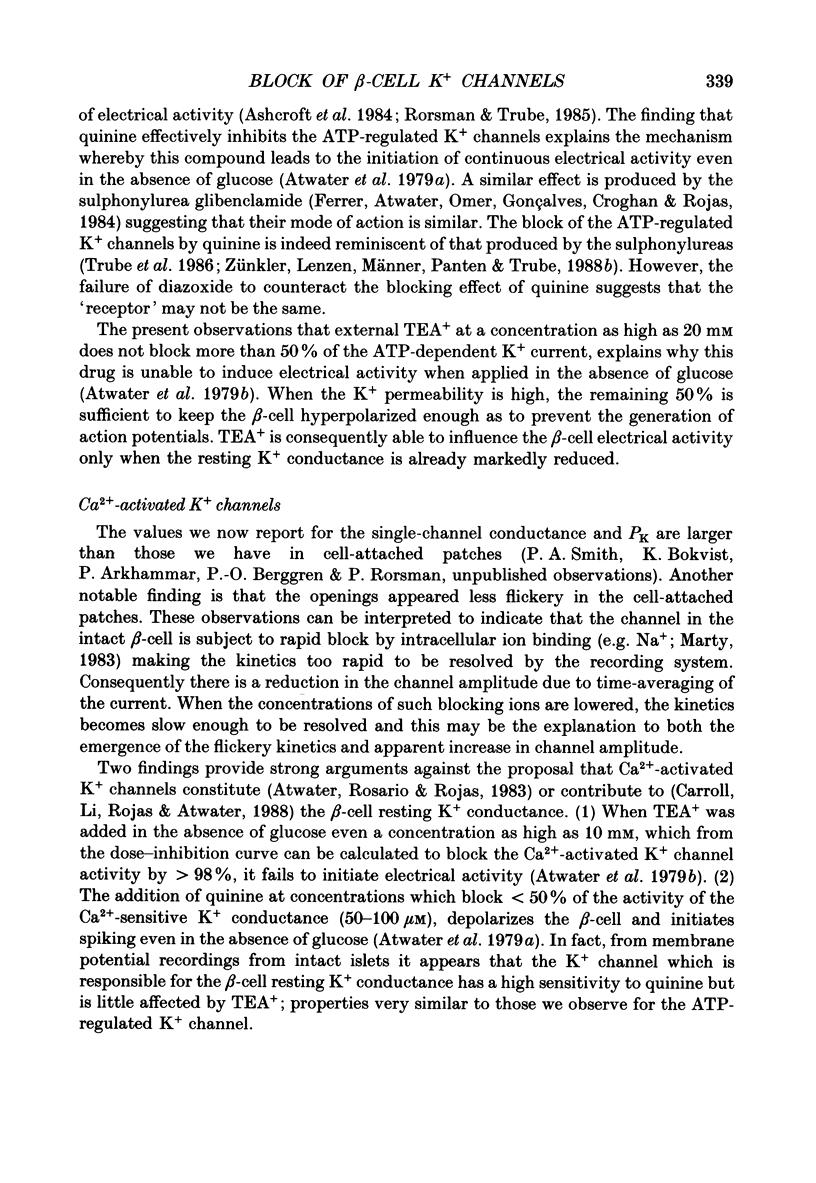
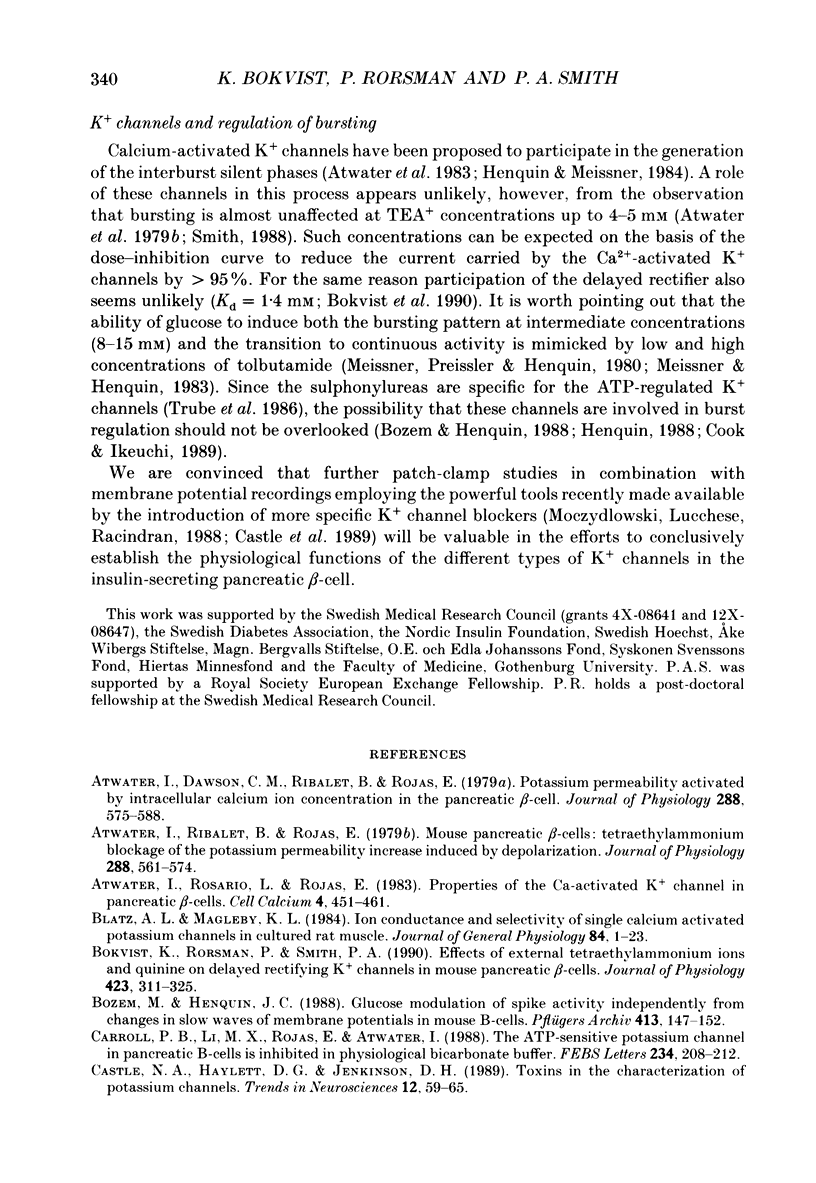
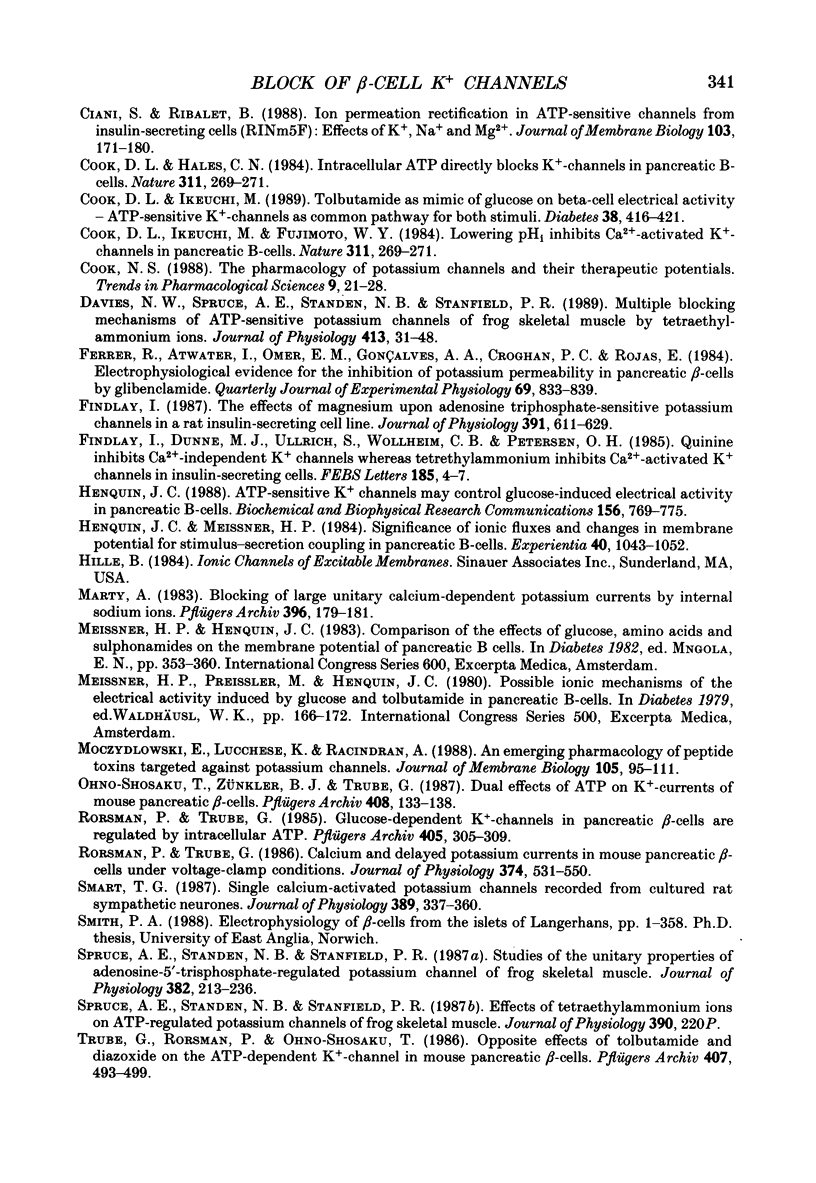
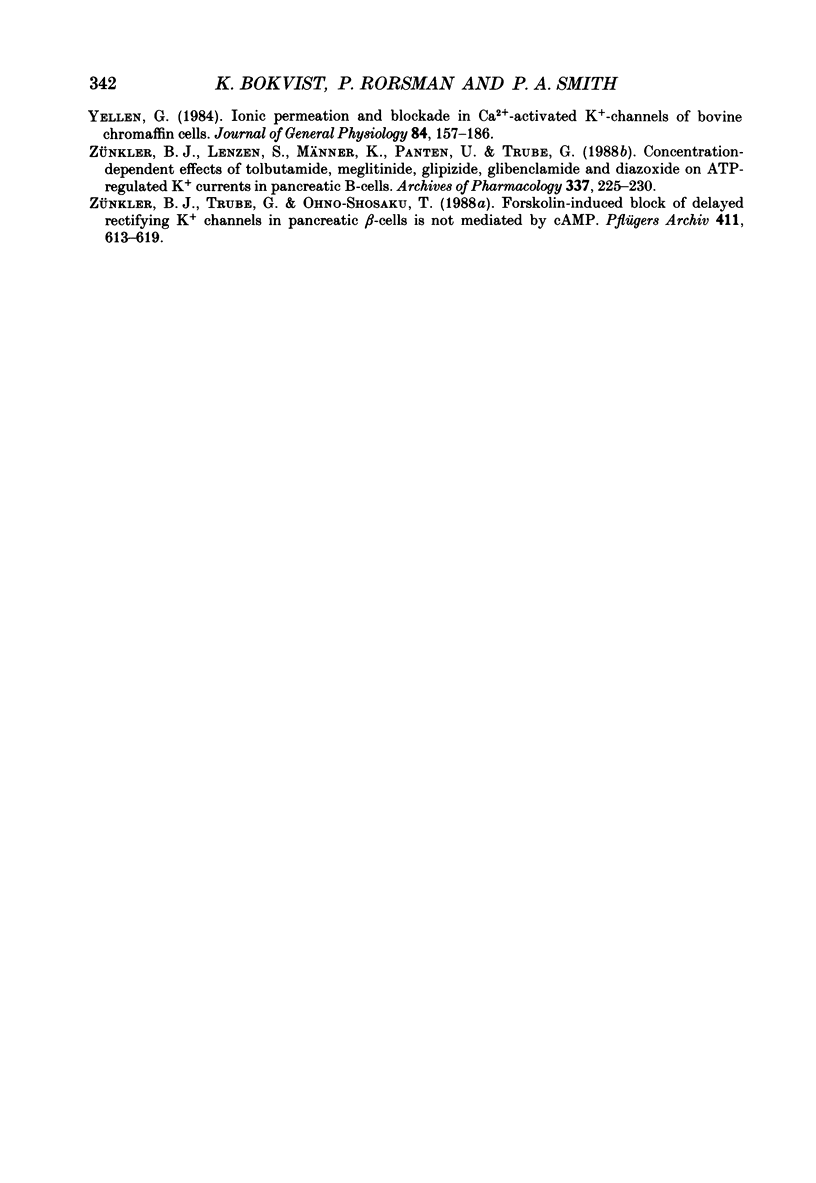
Selected References
These references are in PubMed. This may not be the complete list of references from this article.
- Atwater I., Dawson C. M., Ribalet B., Rojas E. Potassium permeability activated by intracellular calcium ion concentration in the pancreatic beta-cell. J Physiol. 1979 Mar;288:575–588. [PMC free article] [PubMed] [Google Scholar]
- Atwater I., Ribalet B., Rojas E. Mouse pancreatic beta-cells: tetraethylammonium blockage of the potassium permeability increase induced by depolarization. J Physiol. 1979 Mar;288:561–574. [PMC free article] [PubMed] [Google Scholar]
- Atwater I., Rosario L., Rojas E. Properties of the Ca-activated K+ channel in pancreatic beta-cells. Cell Calcium. 1983 Dec;4(5-6):451–461. doi: 10.1016/0143-4160(83)90021-0. [DOI] [PubMed] [Google Scholar]
- Blatz A. L., Magleby K. L. Ion conductance and selectivity of single calcium-activated potassium channels in cultured rat muscle. J Gen Physiol. 1984 Jul;84(1):1–23. doi: 10.1085/jgp.84.1.1. [DOI] [PMC free article] [PubMed] [Google Scholar]
- Bokvist K., Rorsman P., Smith P. A. Effects of external tetraethylammonium ions and quinine on delayed rectifying K+ channels in mouse pancreatic beta-cells. J Physiol. 1990 Apr;423:311–325. doi: 10.1113/jphysiol.1990.sp018024. [DOI] [PMC free article] [PubMed] [Google Scholar]
- Bozem M., Henquin J. C. Glucose modulation of spike activity independently from changes in slow waves of membrane potential in mouse B-cells. Pflugers Arch. 1988 Dec;413(2):147–152. doi: 10.1007/BF00582524. [DOI] [PubMed] [Google Scholar]
- Carroll P. B., Li M. X., Rojas E., Atwater I. The ATP-sensitive potassium channel in pancreatic B-cells is inhibited in physiological bicarbonate buffer. FEBS Lett. 1988 Jul 4;234(1):208–212. doi: 10.1016/0014-5793(88)81335-8. [DOI] [PubMed] [Google Scholar]
- Castle N. A., Haylett D. G., Jenkinson D. H. Toxins in the characterization of potassium channels. Trends Neurosci. 1989 Feb;12(2):59–65. doi: 10.1016/0166-2236(89)90137-9. [DOI] [PubMed] [Google Scholar]
- Ciani S., Ribalet B. Ion permeation and rectification in ATP-sensitive channels from insulin-secreting cells (RINm5F): effects of K+, Na+ and Mg2+. J Membr Biol. 1988 Jul;103(2):171–180. doi: 10.1007/BF01870947. [DOI] [PubMed] [Google Scholar]
- Cook D. L., Hales C. N. Intracellular ATP directly blocks K+ channels in pancreatic B-cells. Nature. 1984 Sep 20;311(5983):271–273. doi: 10.1038/311271a0. [DOI] [PubMed] [Google Scholar]
- Cook D. L., Ikeuchi M., Fujimoto W. Y. Lowering of pHi inhibits Ca2+-activated K+ channels in pancreatic B-cells. Nature. 1984 Sep 20;311(5983):269–271. doi: 10.1038/311269a0. [DOI] [PubMed] [Google Scholar]
- Cook D. L., Ikeuchi M. Tolbutamide as mimic of glucose on beta-cell electrical activity. ATP-sensitive K+ channels as common pathway for both stimuli. Diabetes. 1989 Apr;38(4):416–421. doi: 10.2337/diab.38.4.416. [DOI] [PubMed] [Google Scholar]
- Cook N. S. The pharmacology of potassium channels and their therapeutic potential. Trends Pharmacol Sci. 1988 Jan;9(1):21–28. doi: 10.1016/0165-6147(88)90238-6. [DOI] [PubMed] [Google Scholar]
- Davies N. W., Spruce A. E., Standen N. B., Stanfield P. R. Multiple blocking mechanisms of ATP-sensitive potassium channels of frog skeletal muscle by tetraethylammonium ions. J Physiol. 1989 Jun;413:31–48. doi: 10.1113/jphysiol.1989.sp017640. [DOI] [PMC free article] [PubMed] [Google Scholar]
- Ferrer R., Atwater I., Omer E. M., Gonçalves A. A., Croghan P. C., Rojas E. Electrophysiological evidence for the inhibition of potassium permeability in pancreatic beta-cells by glibenclamide. Q J Exp Physiol. 1984 Oct;69(4):831–839. doi: 10.1113/expphysiol.1984.sp002872. [DOI] [PubMed] [Google Scholar]
- Findlay I., Dunne M. J., Ullrich S., Wollheim C. B., Petersen O. H. Quinine inhibits Ca2+-independent K+ channels whereas tetraethylammonium inhibits Ca2+-activated K+ channels in insulin-secreting cells. FEBS Lett. 1985 Jun 3;185(1):4–8. doi: 10.1016/0014-5793(85)80729-8. [DOI] [PubMed] [Google Scholar]
- Findlay I. The effects of magnesium upon adenosine triphosphate-sensitive potassium channels in a rat insulin-secreting cell line. J Physiol. 1987 Oct;391:611–629. doi: 10.1113/jphysiol.1987.sp016759. [DOI] [PMC free article] [PubMed] [Google Scholar]
- Henquin J. C. ATP-sensitive K+ channels may control glucose-induced electrical activity in pancreatic B-cells. Biochem Biophys Res Commun. 1988 Oct 31;156(2):769–775. doi: 10.1016/s0006-291x(88)80910-0. [DOI] [PubMed] [Google Scholar]
- Henquin J. C., Meissner H. P. Significance of ionic fluxes and changes in membrane potential for stimulus-secretion coupling in pancreatic B-cells. Experientia. 1984 Oct 15;40(10):1043–1052. doi: 10.1007/BF01971450. [DOI] [PubMed] [Google Scholar]
- Marty A. Blocking of large unitary calcium-dependent potassium currents by internal sodium ions. Pflugers Arch. 1983 Feb;396(2):179–181. doi: 10.1007/BF00615524. [DOI] [PubMed] [Google Scholar]
- Moczydlowski E., Lucchesi K., Ravindran A. An emerging pharmacology of peptide toxins targeted against potassium channels. J Membr Biol. 1988 Oct;105(2):95–111. doi: 10.1007/BF02009164. [DOI] [PubMed] [Google Scholar]
- Ohno-Shosaku T., Zünkler B. J., Trube G. Dual effects of ATP on K+ currents of mouse pancreatic beta-cells. Pflugers Arch. 1987 Feb;408(2):133–138. doi: 10.1007/BF00581342. [DOI] [PubMed] [Google Scholar]
- Rorsman P., Trube G. Calcium and delayed potassium currents in mouse pancreatic beta-cells under voltage-clamp conditions. J Physiol. 1986 May;374:531–550. doi: 10.1113/jphysiol.1986.sp016096. [DOI] [PMC free article] [PubMed] [Google Scholar]
- Rorsman P., Trube G. Glucose dependent K+-channels in pancreatic beta-cells are regulated by intracellular ATP. Pflugers Arch. 1985 Dec;405(4):305–309. doi: 10.1007/BF00595682. [DOI] [PubMed] [Google Scholar]
- Smart T. G. Single calcium-activated potassium channels recorded from cultured rat sympathetic neurones. J Physiol. 1987 Aug;389:337–360. doi: 10.1113/jphysiol.1987.sp016660. [DOI] [PMC free article] [PubMed] [Google Scholar]
- Spruce A. E., Standen N. B., Stanfield P. R. Studies of the unitary properties of adenosine-5'-triphosphate-regulated potassium channels of frog skeletal muscle. J Physiol. 1987 Jan;382:213–236. doi: 10.1113/jphysiol.1987.sp016364. [DOI] [PMC free article] [PubMed] [Google Scholar]
- Trube G., Rorsman P., Ohno-Shosaku T. Opposite effects of tolbutamide and diazoxide on the ATP-dependent K+ channel in mouse pancreatic beta-cells. Pflugers Arch. 1986 Nov;407(5):493–499. doi: 10.1007/BF00657506. [DOI] [PubMed] [Google Scholar]
- Yellen G. Ionic permeation and blockade in Ca2+-activated K+ channels of bovine chromaffin cells. J Gen Physiol. 1984 Aug;84(2):157–186. doi: 10.1085/jgp.84.2.157. [DOI] [PMC free article] [PubMed] [Google Scholar]
- Zünkler B. J., Lenzen S., Männer K., Panten U., Trube G. Concentration-dependent effects of tolbutamide, meglitinide, glipizide, glibenclamide and diazoxide on ATP-regulated K+ currents in pancreatic B-cells. Naunyn Schmiedebergs Arch Pharmacol. 1988 Feb;337(2):225–230. doi: 10.1007/BF00169252. [DOI] [PubMed] [Google Scholar]
- Zünkler B. J., Trube G., Ohno-Shosaku T. Forskolin-induced block of delayed rectifying K+ channels in pancreatic beta-cells is not mediated by cAMP. Pflugers Arch. 1988 Jun;411(6):613–619. doi: 10.1007/BF00580856. [DOI] [PubMed] [Google Scholar]