Abstract
1. Intracellular pH was recorded (double-barrelled pH-selective microelectrodes) in single ventricular myocytes and whole papillary muscles isolated from guinea-pig heart. Both preparations were acid-loaded by various manoeuvres (addition and removal of external NH4Cl or CO2) in order that a comparison could be made of the size and speed of intracellular pH changes and hence of the apparent intracellular buffering power (beta). 2. For the same acid-loading procedure, the size of intracellular pH (pHi) changes was about threefold larger in the isolated myocyte than in whole papillary muscle. The rate of initial acid loading as well as the subsequent rate of pHi recovery (caused by acid extrusion from the cell) were also threefold faster in the myocyte. Estimates of apparent intrinsic (non-CO2) buffering power, based upon the size of pHi changes during acid loading, were 15-20 mmol l-1 for the myocyte and about 70 mmol l-1 for whole muscle. This latter value is similar to previous estimates of beta in heart. 3. When acid extrusion was reduced by applying a high dose of amiloride (1 mmol l-1), then the size of the pHi change during acid loading increased greatly in papillary muscle but changed much less in the myocyte; beta now appeared to be about 30 mmol l-1 in whole muscle but remained essentially unchanged in the myocyte. 4. We conclude that previous values for beta in cardiac muscle have been greatly overestimated because of the presence of sarcolemmal acid extrusion. Paradoxically, this error in estimating beta is far less evident in the isolated myocyte. We suggest that this is because a much more rapid acid loading is achievable in the myocyte so that acid loading will be blunted less by acid extrusion than in whole muscle. We present a simple mathematical model that demonstrates this phenomenon. We conclude that beta in ventricular muscle is likely to resemble that measured in the isolated myocyte, i.e. 15-20 mmol l-1. 5. Slow acid loading in whole ventricular muscle will also affect the kinetics of pHi changes. The model indicates that the rate of pHi recovery from an acid load in papillary muscle does not reflect the pHi dependence of acid extrusion. Instead, it is heavily influenced by the slow rate of acid loading. This emphasises that great care should be taken when interpreting the kinetics of pHi changes in multicellular ventricular preparations.
Full text
PDF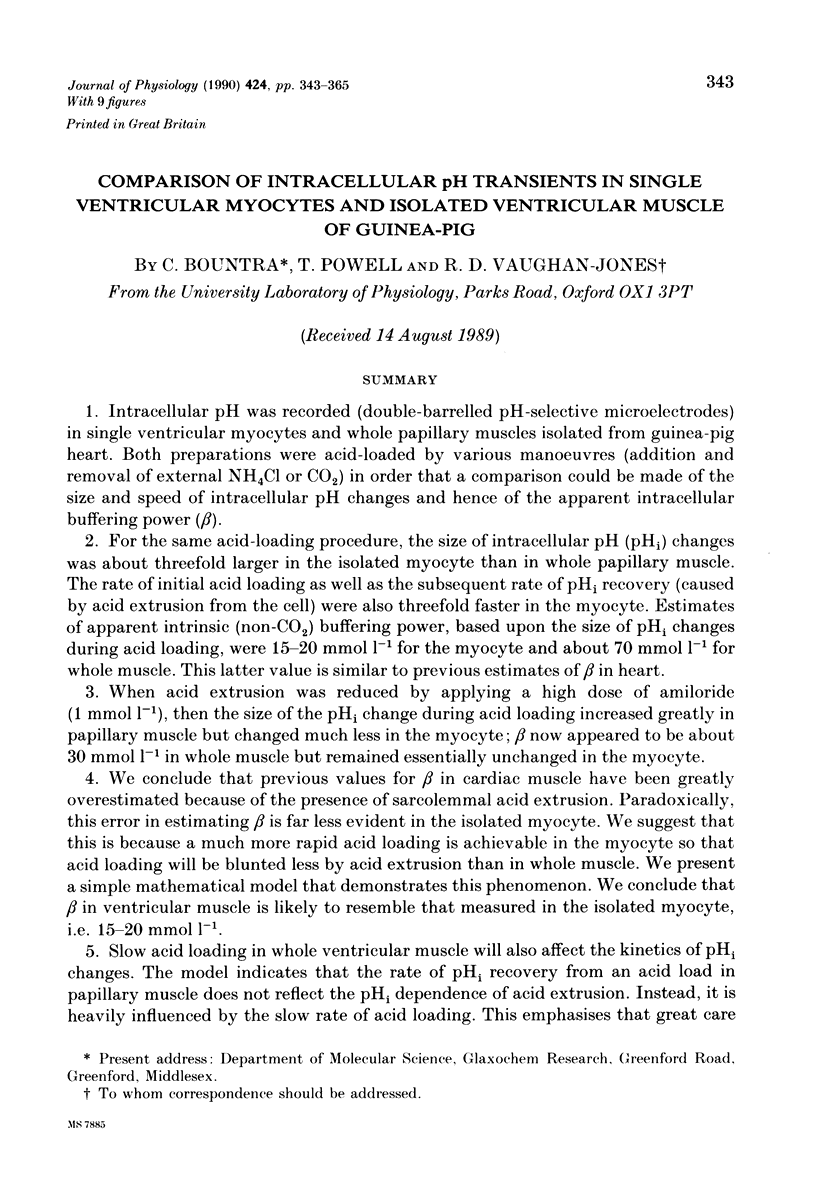
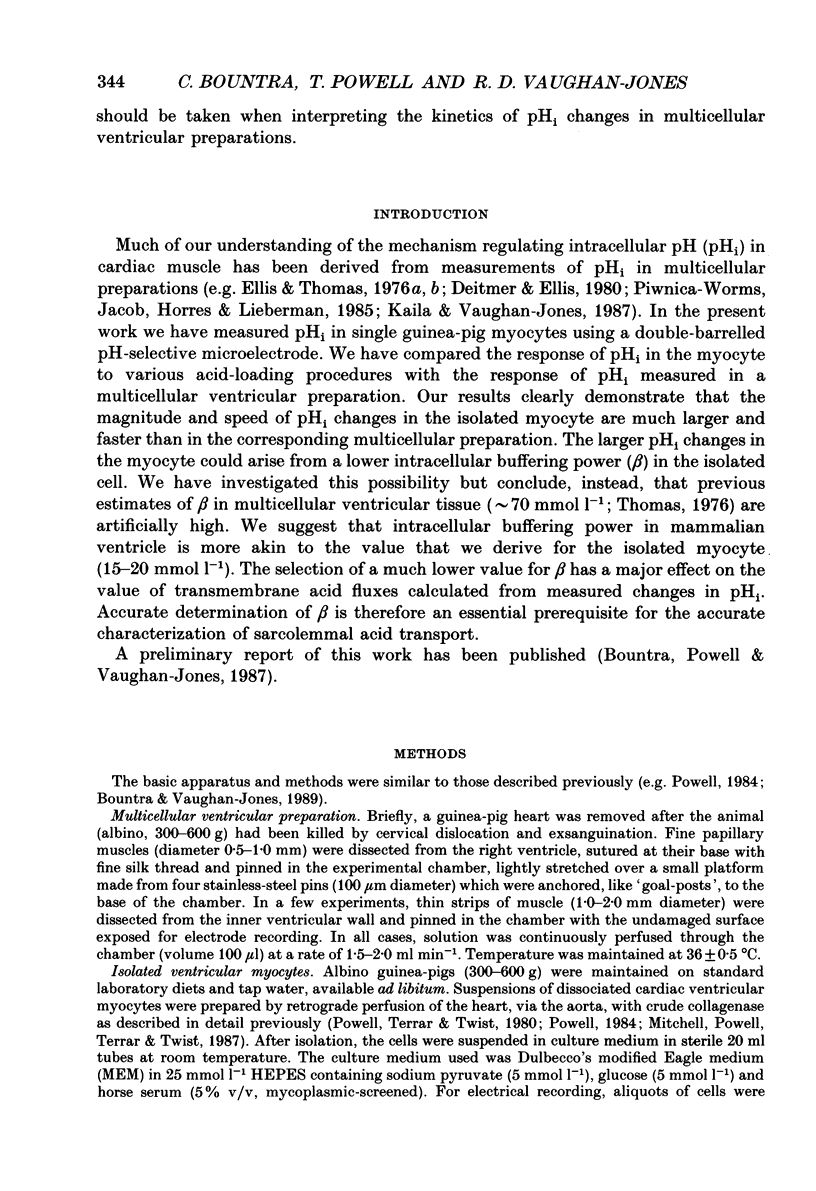
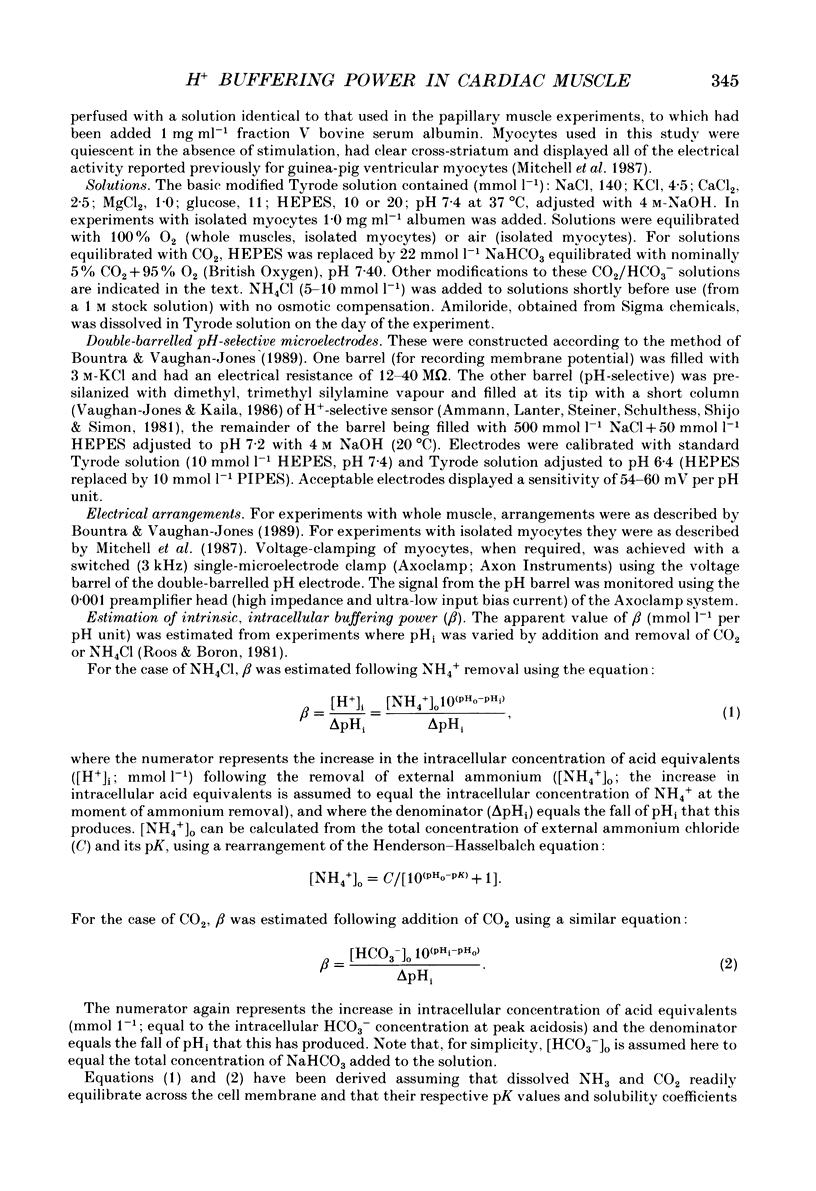
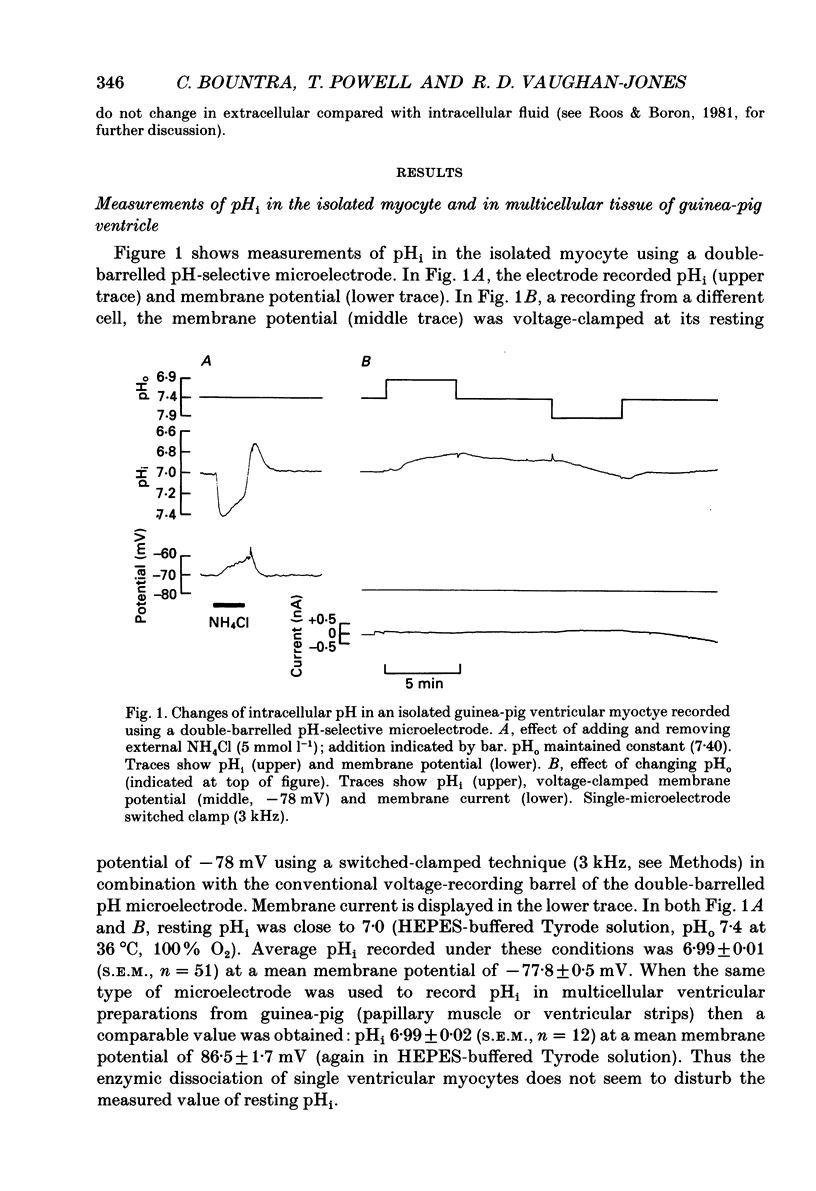
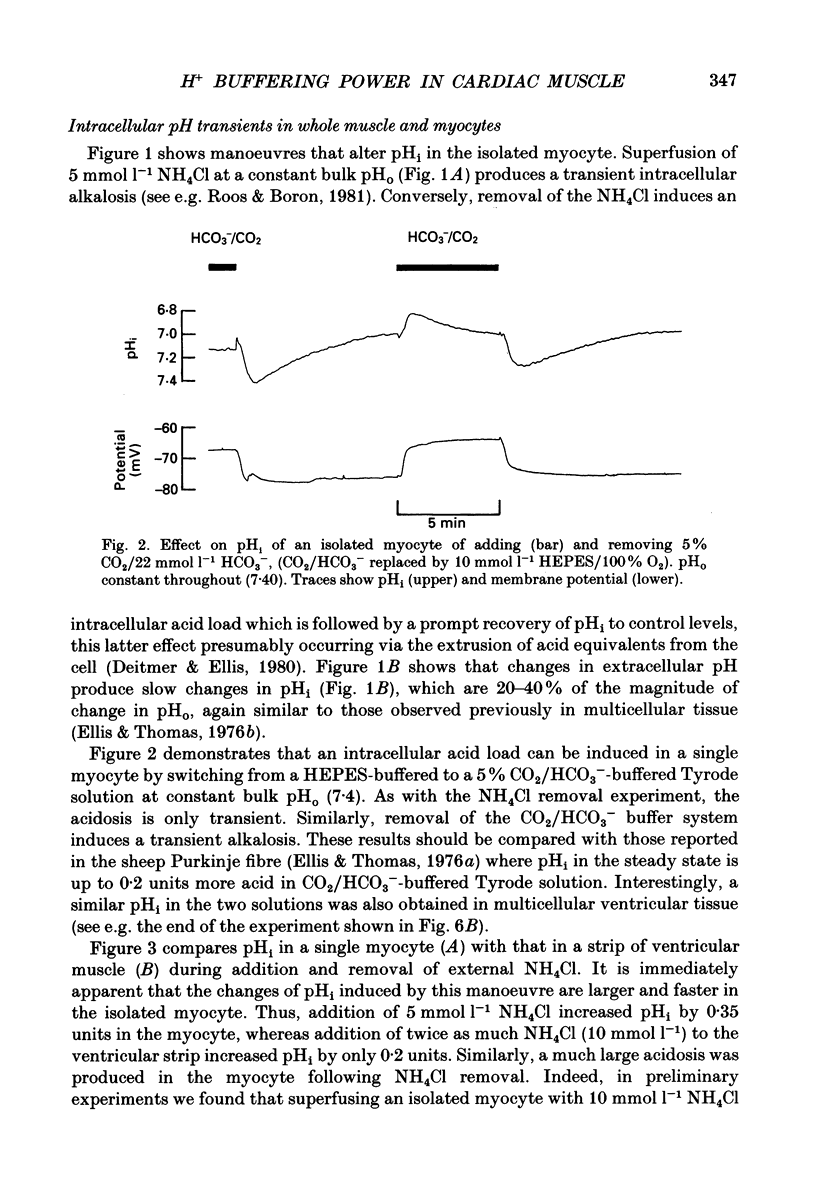
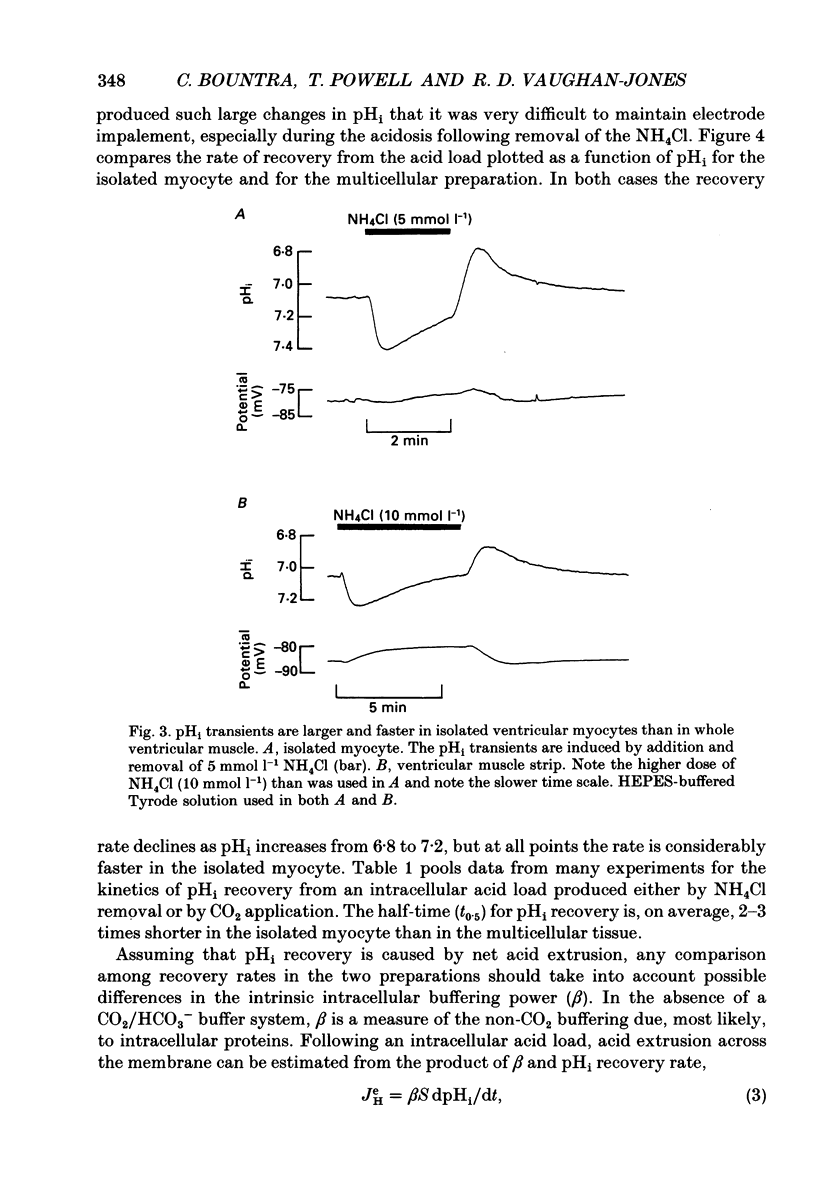
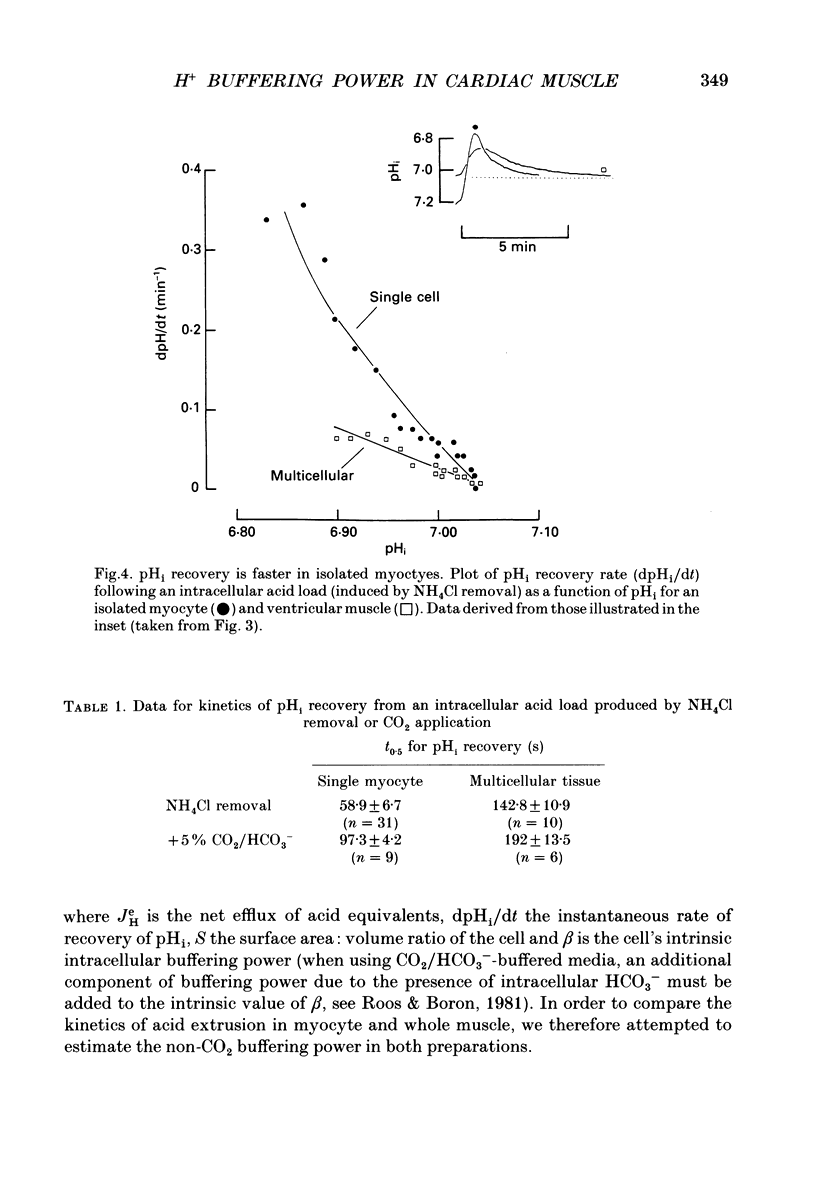
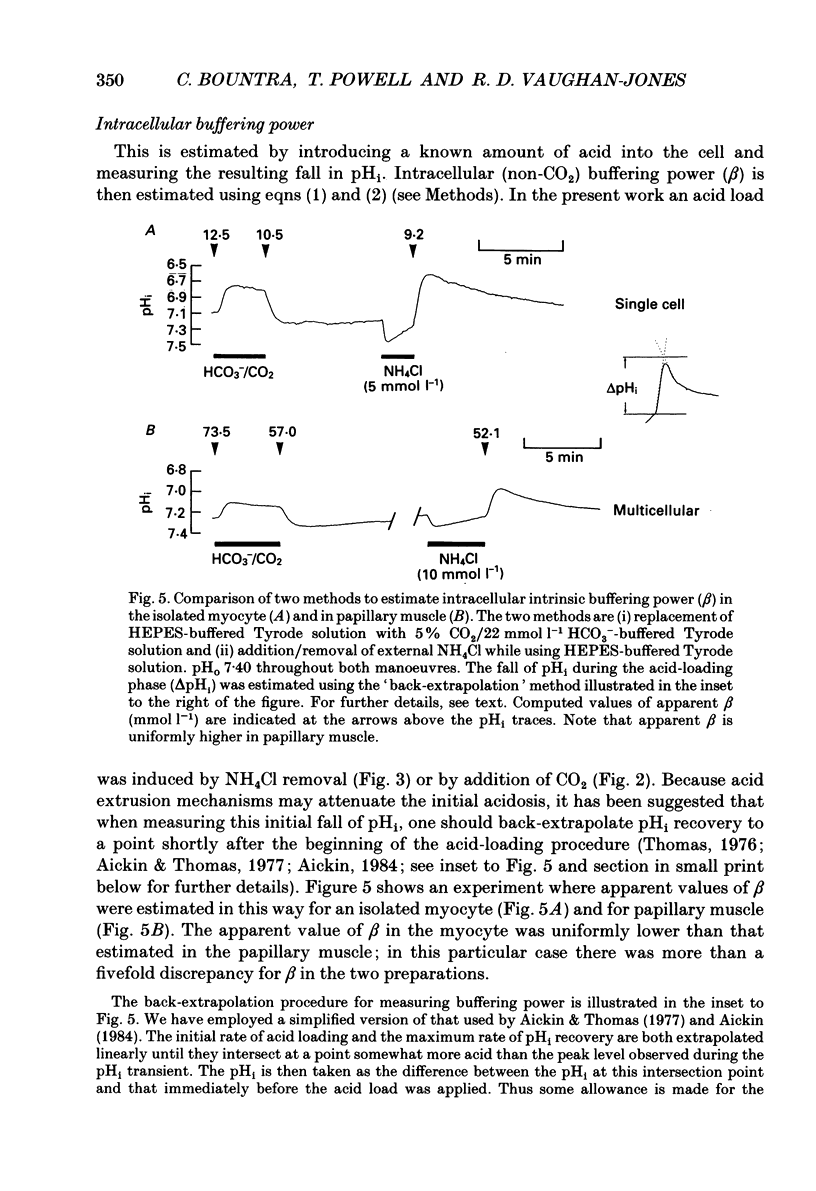
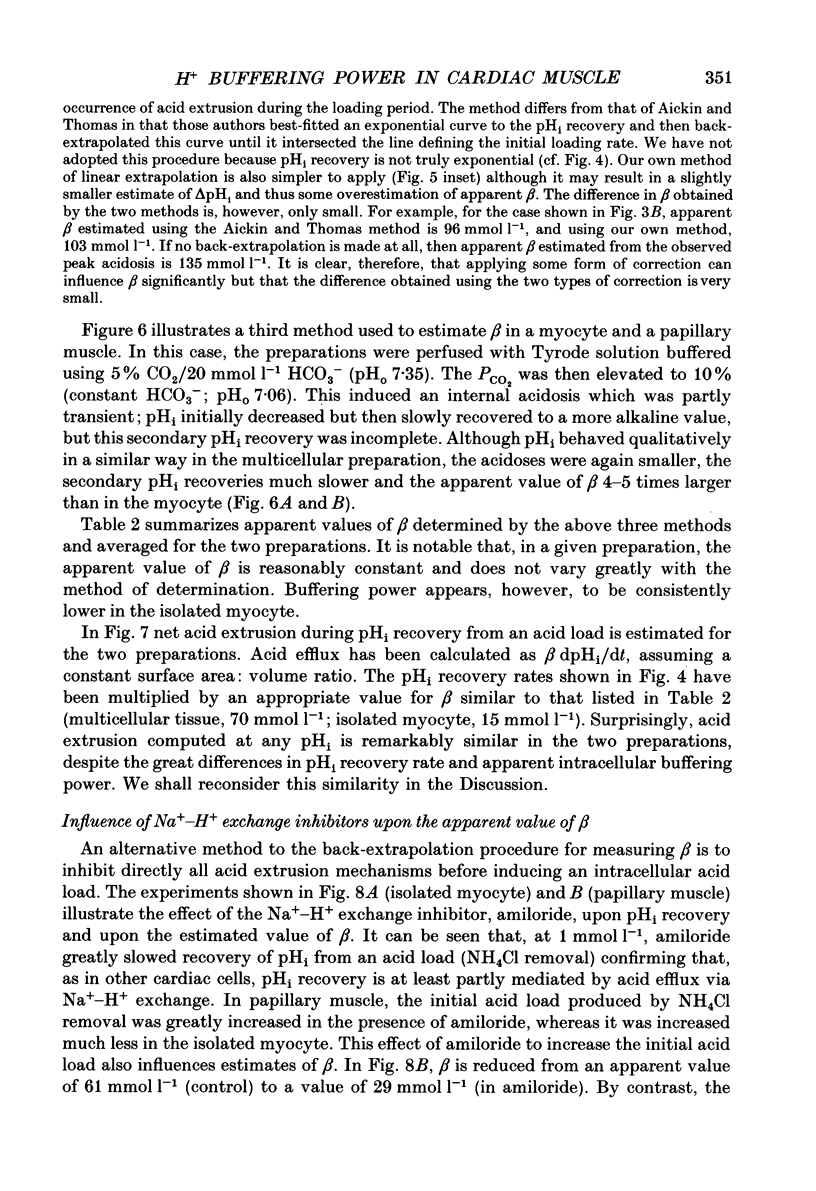
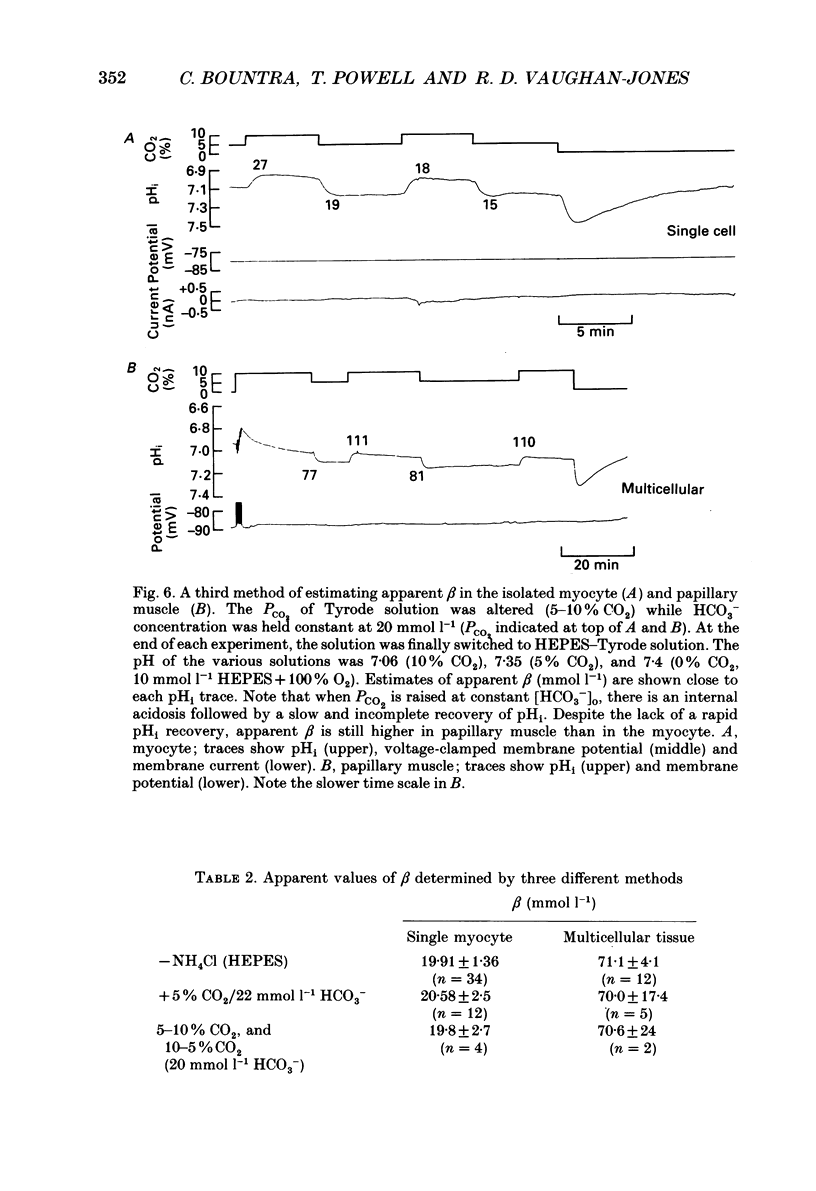
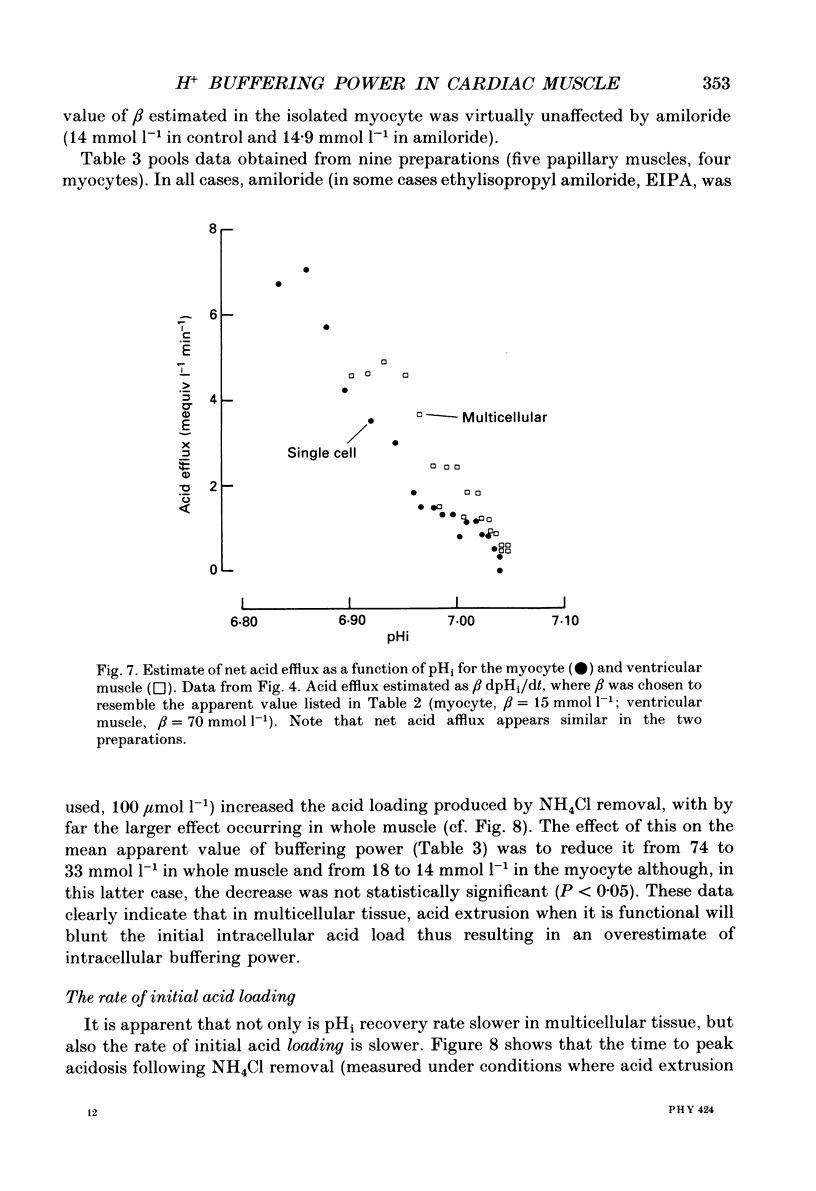
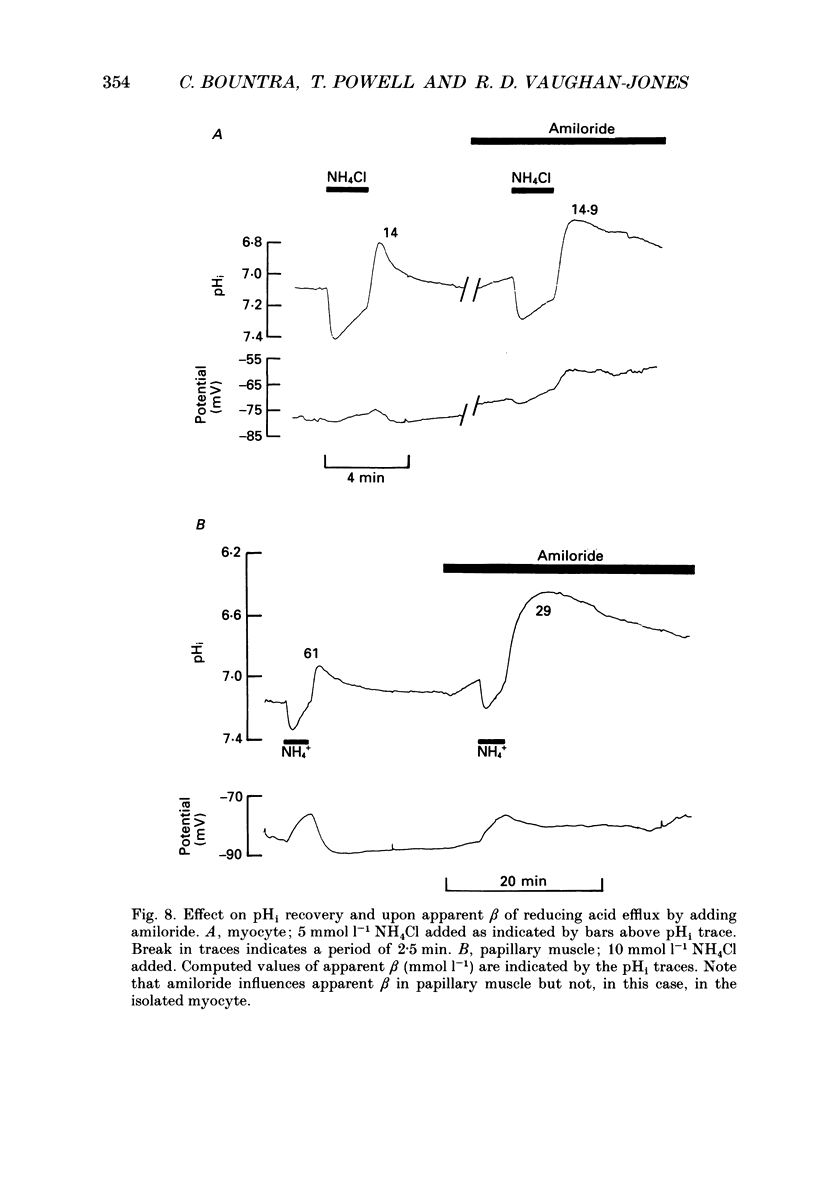
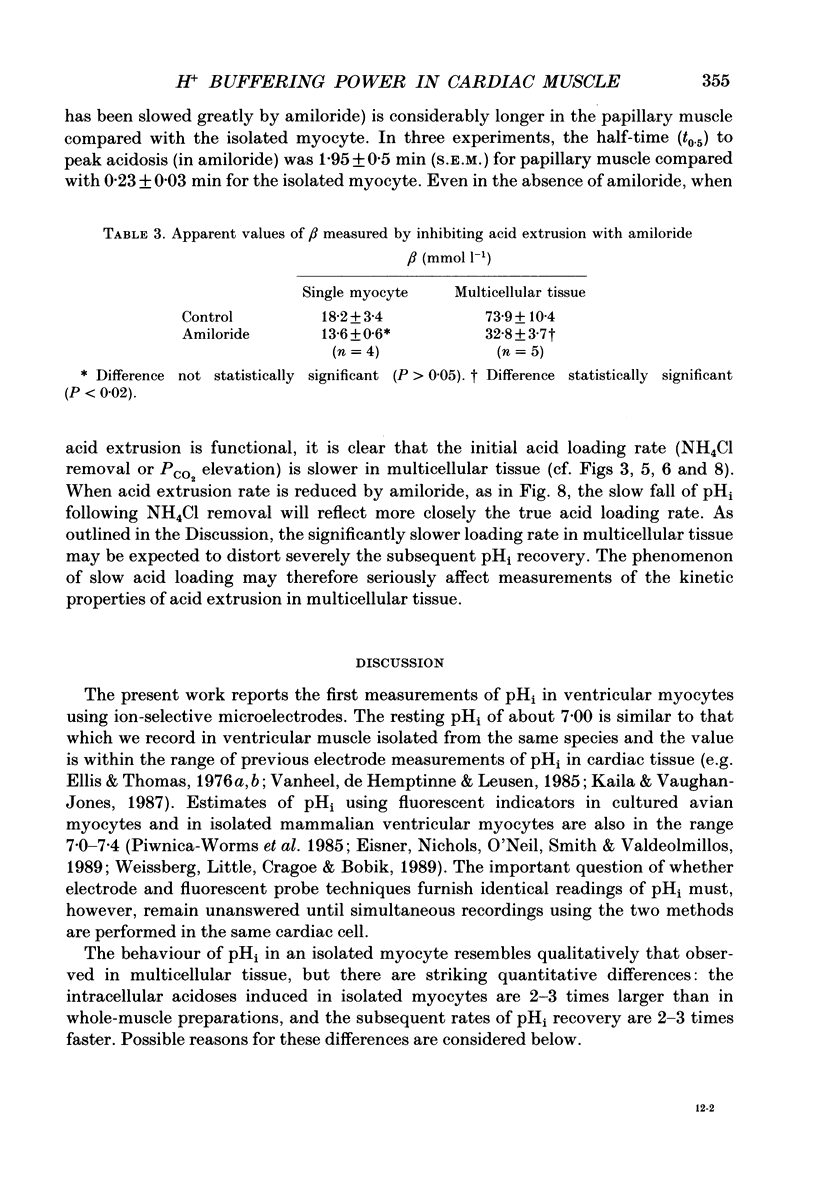
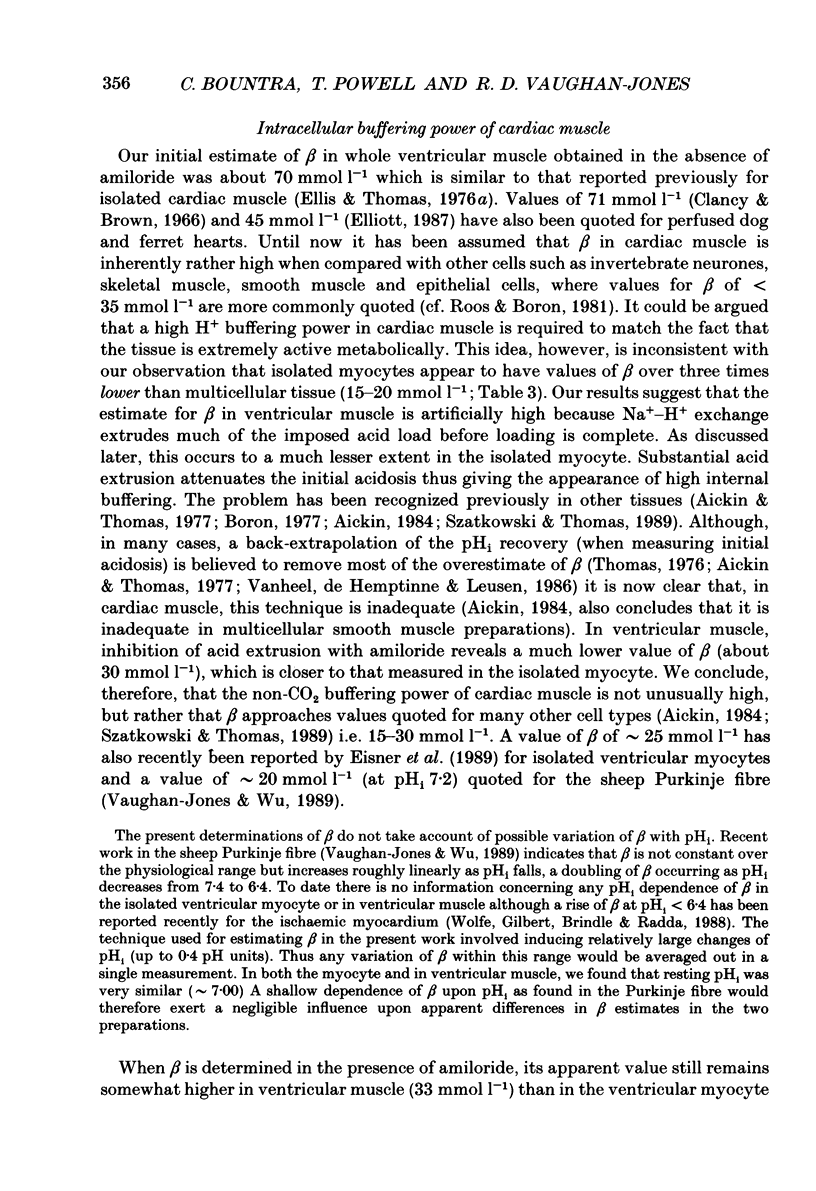
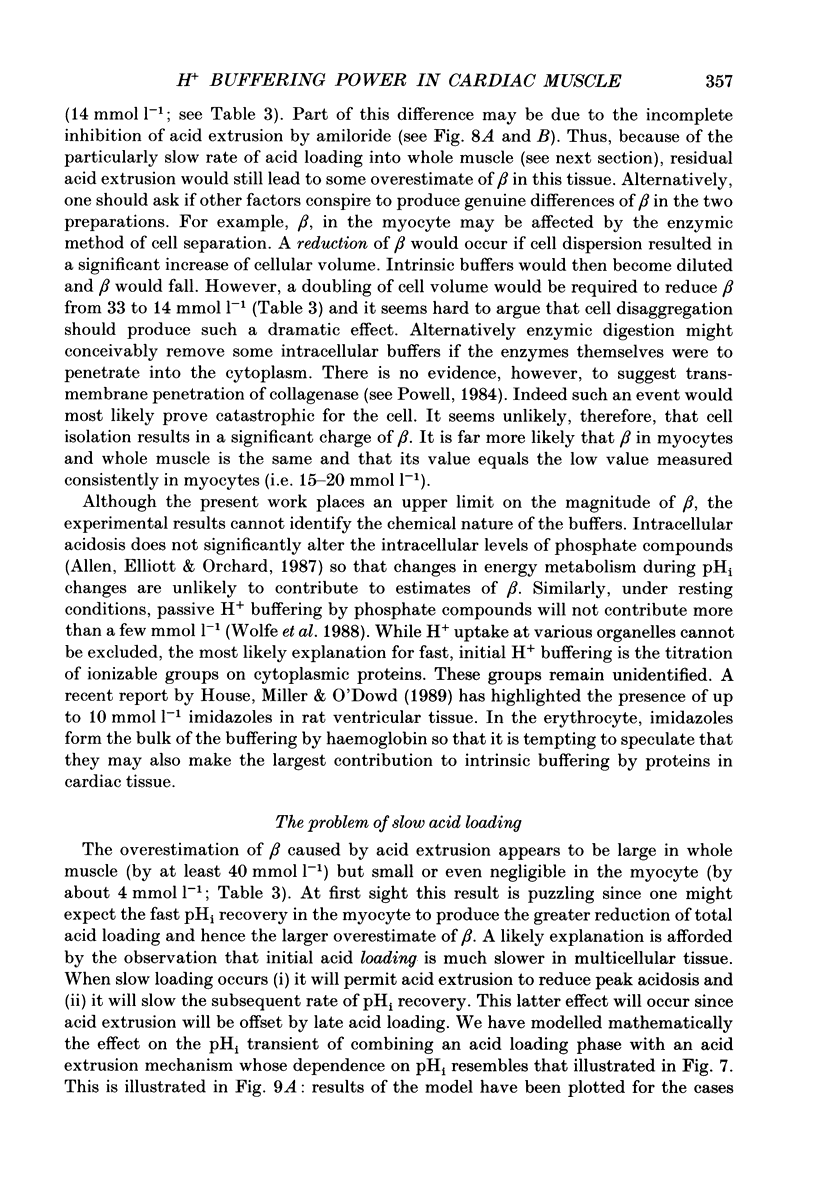
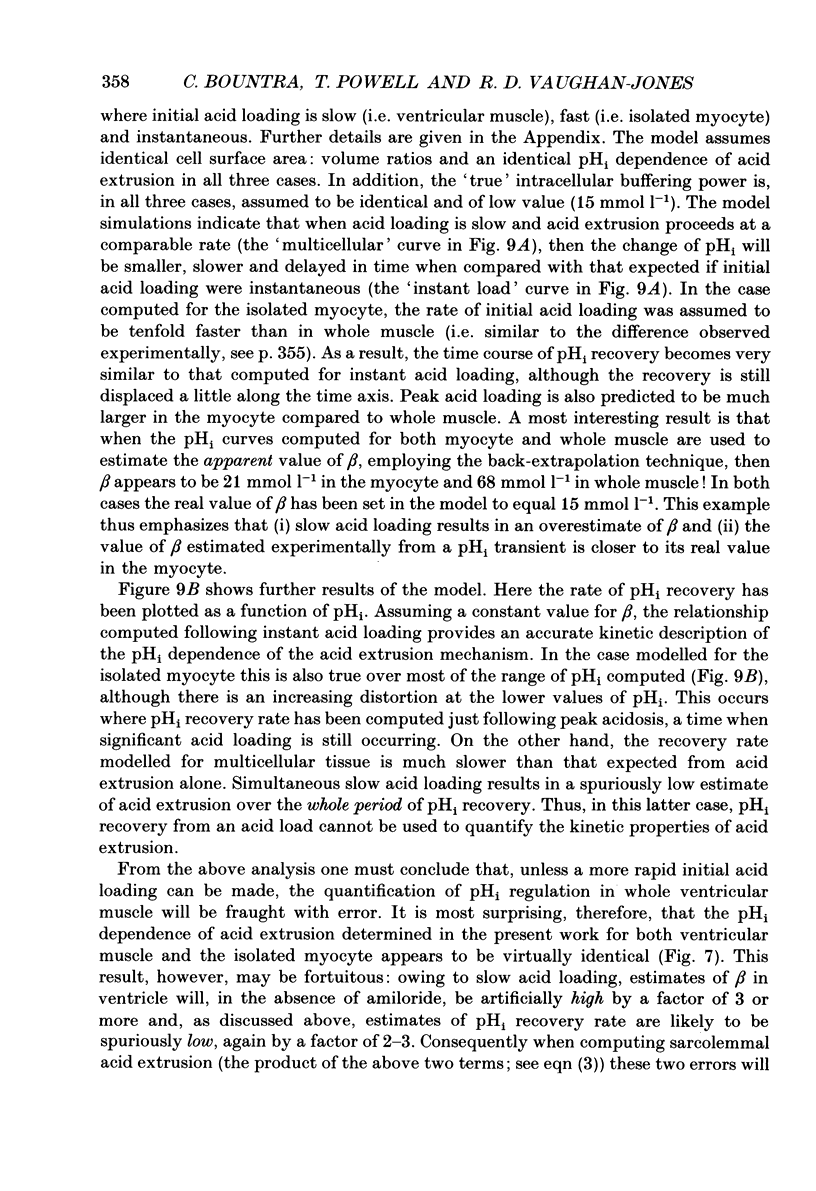
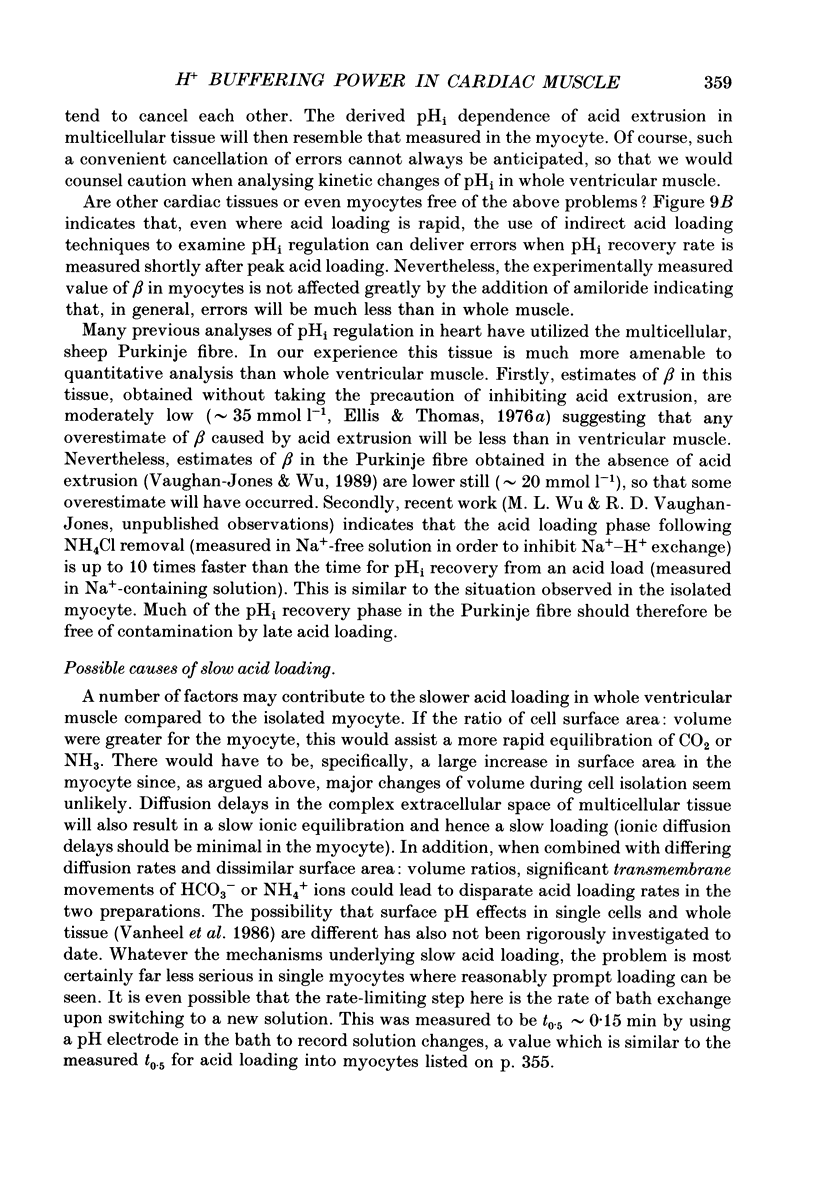
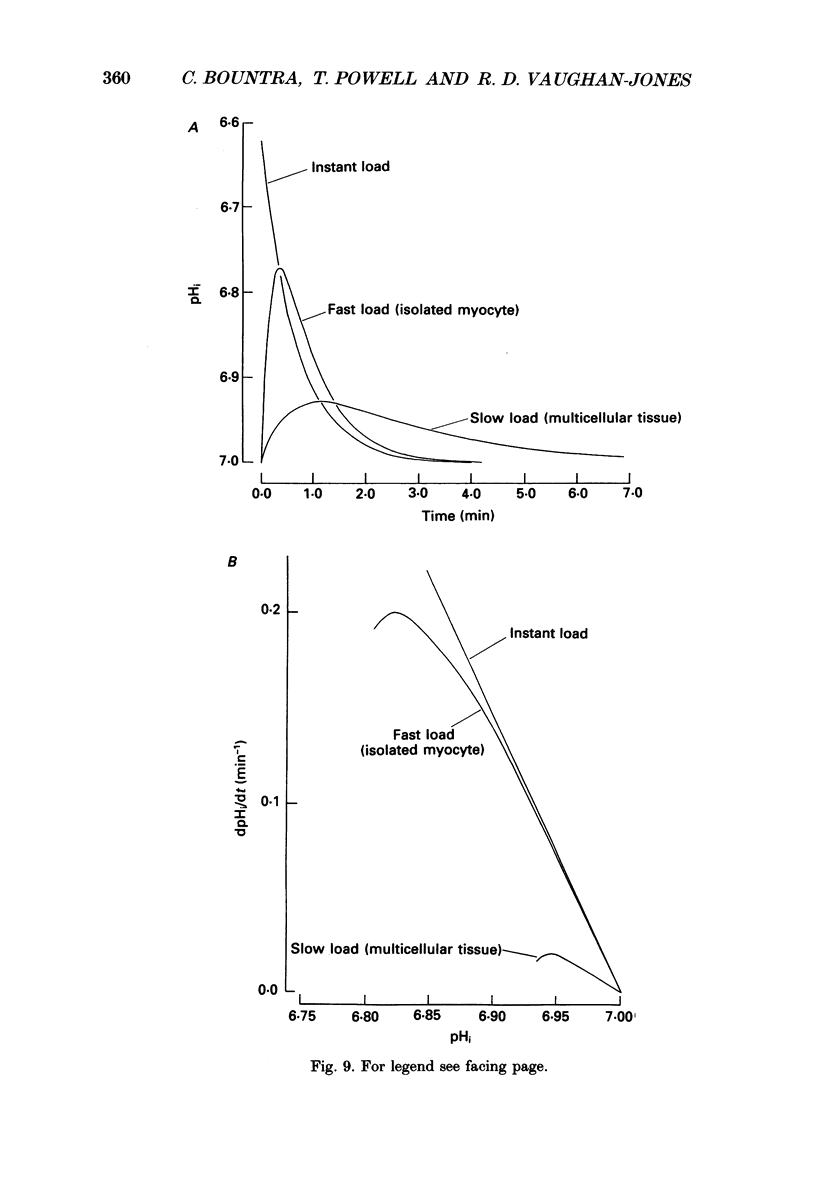
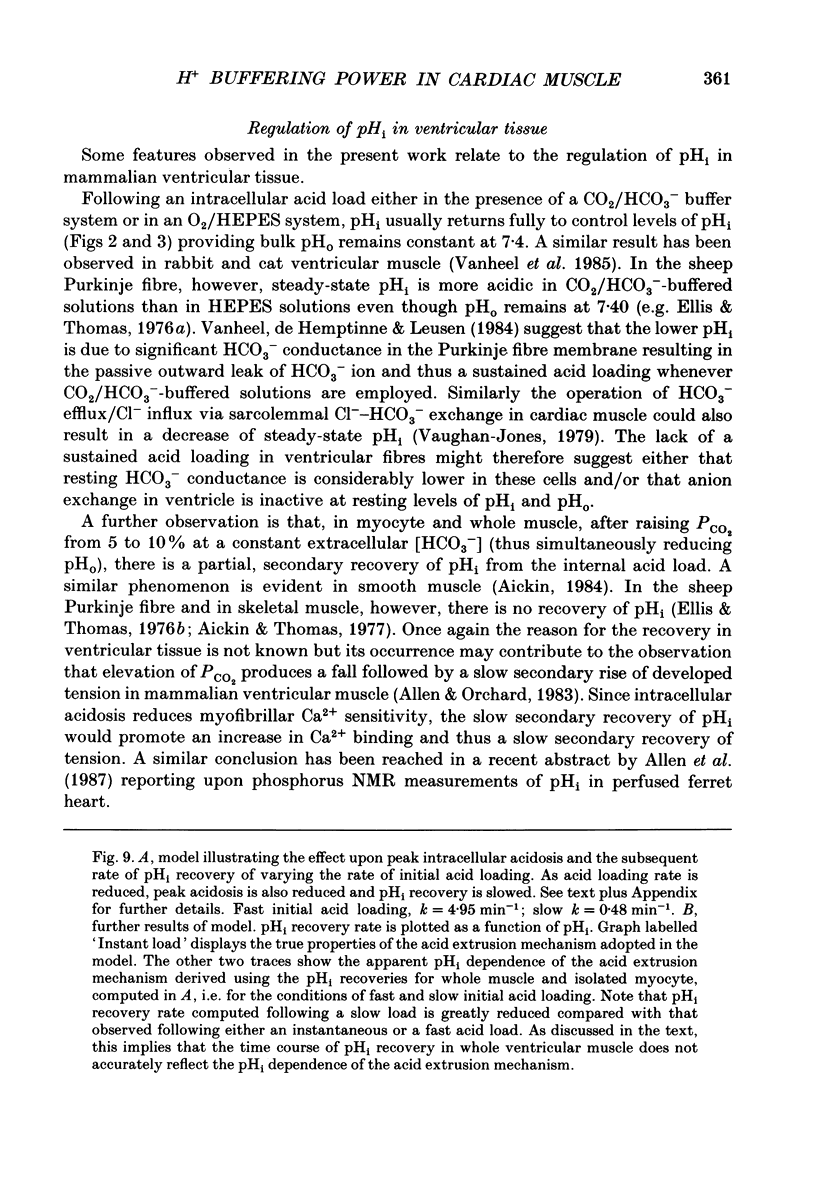
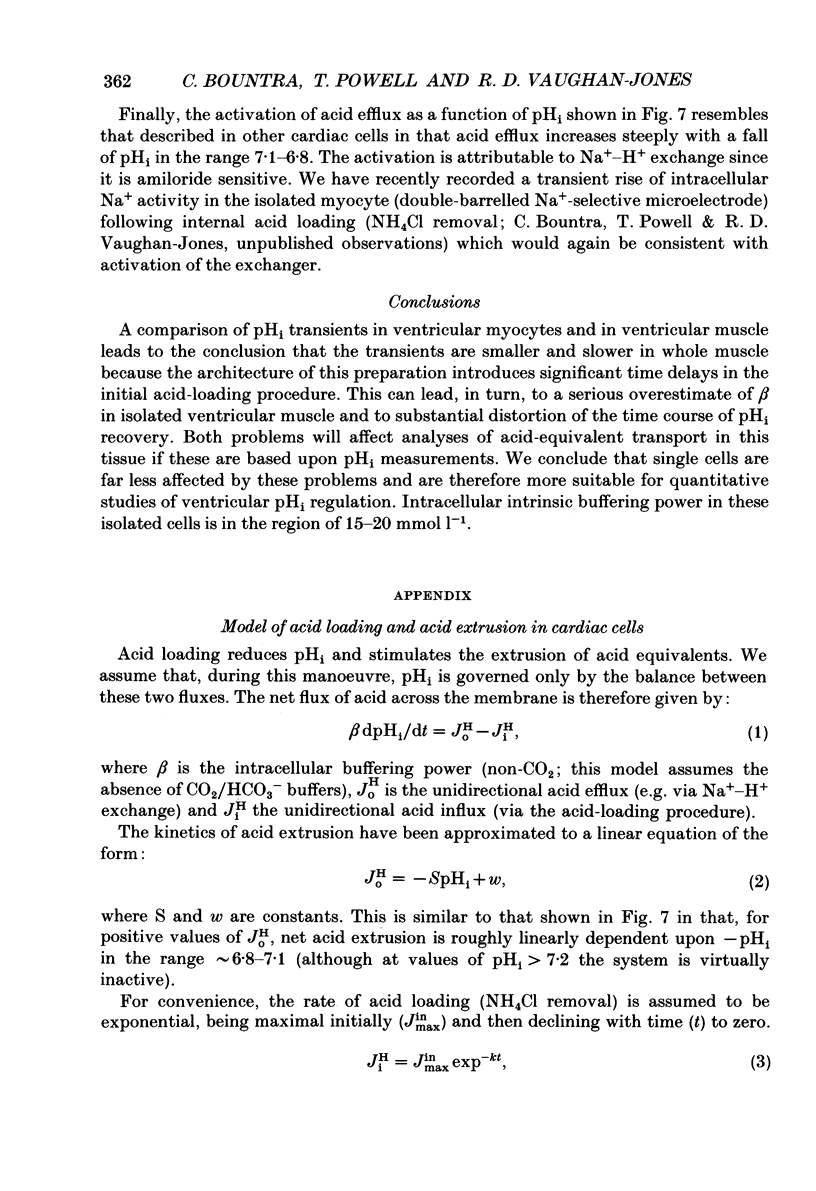
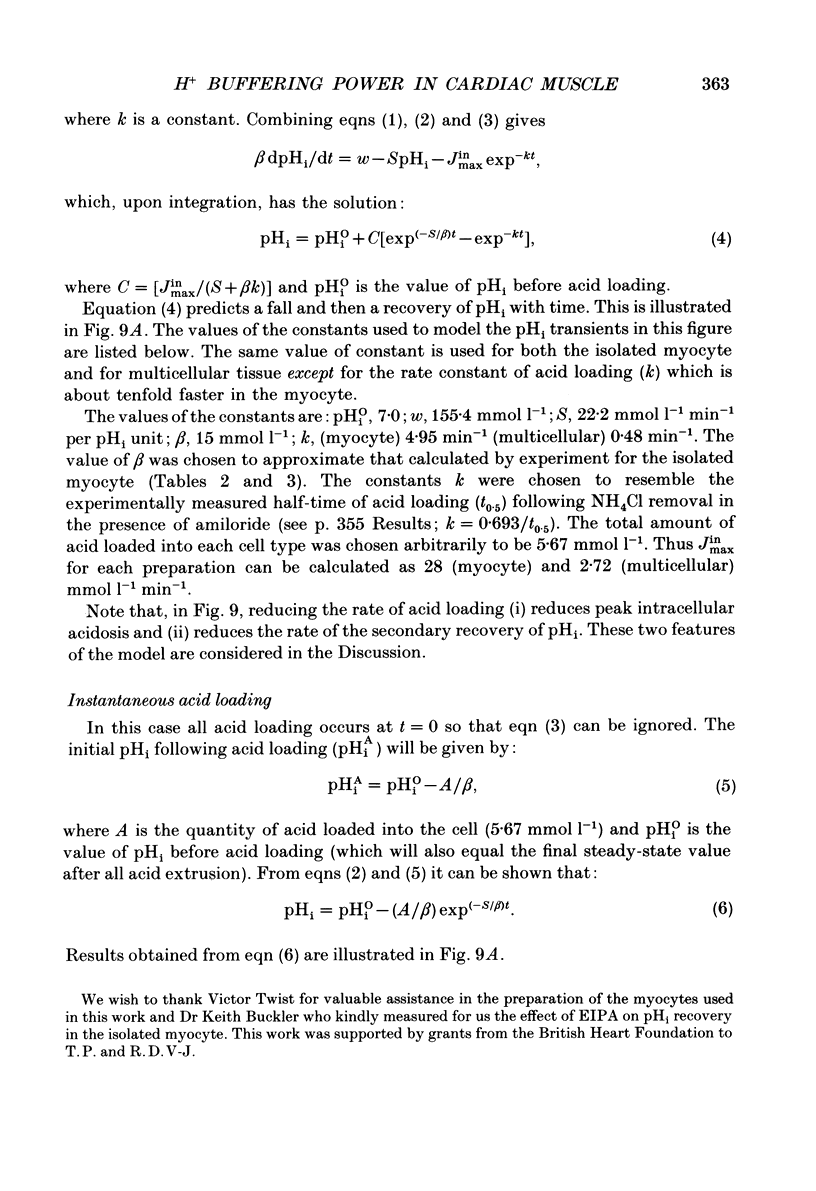
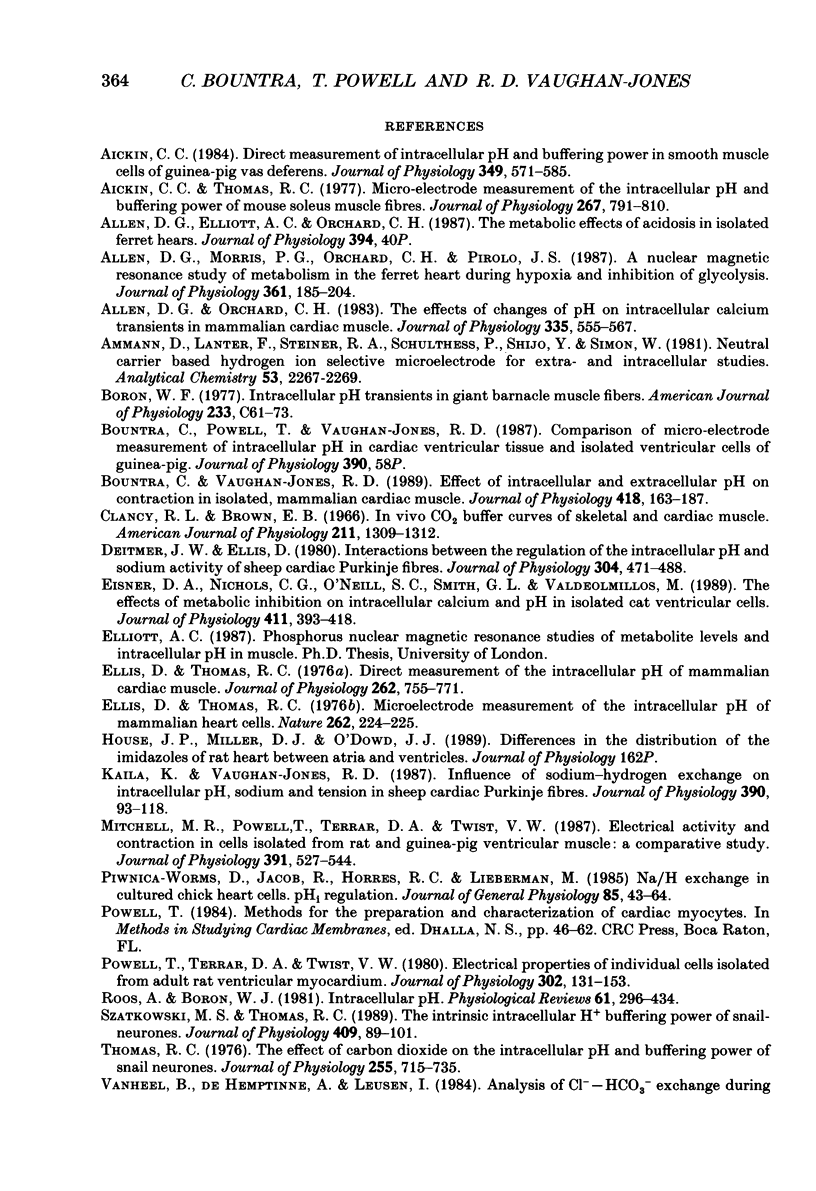
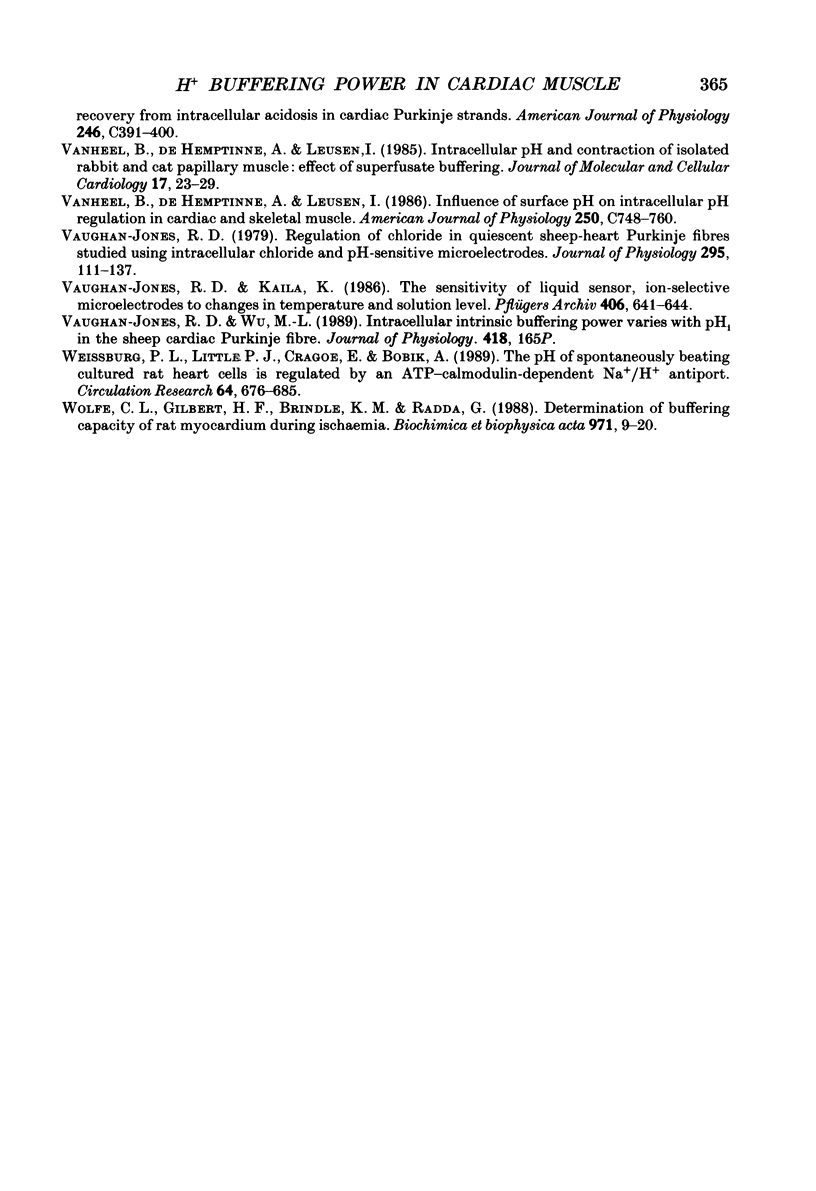
Selected References
These references are in PubMed. This may not be the complete list of references from this article.
- Aickin C. C. Direct measurement of intracellular pH and buffering power in smooth muscle cells of guinea-pig vas deferens. J Physiol. 1984 Apr;349:571–585. doi: 10.1113/jphysiol.1984.sp015174. [DOI] [PMC free article] [PubMed] [Google Scholar]
- Allen D. G., Morris P. G., Orchard C. H., Pirolo J. S. A nuclear magnetic resonance study of metabolism in the ferret heart during hypoxia and inhibition of glycolysis. J Physiol. 1985 Apr;361:185–204. doi: 10.1113/jphysiol.1985.sp015640. [DOI] [PMC free article] [PubMed] [Google Scholar]
- Allen D. G., Orchard C. H. The effects of changes of pH on intracellular calcium transients in mammalian cardiac muscle. J Physiol. 1983 Feb;335:555–567. doi: 10.1113/jphysiol.1983.sp014550. [DOI] [PMC free article] [PubMed] [Google Scholar]
- Ammann D., Lanter F., Steiner R. A., Schulthess P., Shijo Y., Simon W. Neutral carrier based hydrogen ion selective microelectrode for extra- and intracellular studies. Anal Chem. 1981 Dec;53(14):2267–2269. doi: 10.1021/ac00237a031. [DOI] [PubMed] [Google Scholar]
- Boron W. F. Intracellular pH transients in giant barnacle muscle fibers. Am J Physiol. 1977 Sep;233(3):C61–C73. doi: 10.1152/ajpcell.1977.233.3.C61. [DOI] [PubMed] [Google Scholar]
- Bountra C., Vaughan-Jones R. D. Effect of intracellular and extracellular pH on contraction in isolated, mammalian cardiac muscle. J Physiol. 1989 Nov;418:163–187. doi: 10.1113/jphysiol.1989.sp017833. [DOI] [PMC free article] [PubMed] [Google Scholar]
- Clancy R. L., Brown E. B., Jr In vivo CO-2 buffer curves of skeletal and cardiac muscle. Am J Physiol. 1966 Dec;211(6):1309–1312. doi: 10.1152/ajplegacy.1966.211.6.1309. [DOI] [PubMed] [Google Scholar]
- Deitmer J. W., Ellis D. Interactions between the regulation of the intracellular pH and sodium activity of sheep cardiac Purkinje fibres. J Physiol. 1980 Jul;304:471–488. doi: 10.1113/jphysiol.1980.sp013337. [DOI] [PMC free article] [PubMed] [Google Scholar]
- Eisner D. A., Nichols C. G., O'Neill S. C., Smith G. L., Valdeolmillos M. The effects of metabolic inhibition on intracellular calcium and pH in isolated rat ventricular cells. J Physiol. 1989 Apr;411:393–418. doi: 10.1113/jphysiol.1989.sp017580. [DOI] [PMC free article] [PubMed] [Google Scholar]
- Ellis D., Thomas R. C. Direct measurement of the intracellular pH of mammalian cardiac muscle. J Physiol. 1976 Nov;262(3):755–771. doi: 10.1113/jphysiol.1976.sp011619. [DOI] [PMC free article] [PubMed] [Google Scholar]
- Ellis D., Thomas R. C. Microelectrode measurement of the intracellular pH of mammalian heart cells. Nature. 1976 Jul 15;262(5565):224–225. doi: 10.1038/262224a0. [DOI] [PubMed] [Google Scholar]
- Kaila K., Vaughan-Jones R. D. Influence of sodium-hydrogen exchange on intracellular pH, sodium and tension in sheep cardiac Purkinje fibres. J Physiol. 1987 Sep;390:93–118. doi: 10.1113/jphysiol.1987.sp016688. [DOI] [PMC free article] [PubMed] [Google Scholar]
- Micro-electrode measurement of the intracellular pH and buffering power of mouse soleus muscle fibres. J Physiol. 1977 Jun;267(3):791–810. doi: 10.1113/jphysiol.1977.sp011838. [DOI] [PMC free article] [PubMed] [Google Scholar]
- Mitchell M. R., Powell T., Terrar D. A., Twist V. W. Electrical activity and contraction in cells isolated from rat and guinea-pig ventricular muscle: a comparative study. J Physiol. 1987 Oct;391:527–544. doi: 10.1113/jphysiol.1987.sp016754. [DOI] [PMC free article] [PubMed] [Google Scholar]
- Piwnica-Worms D., Jacob R., Horres C. R., Lieberman M. Na/H exchange in cultured chick heart cells. pHi regulation. J Gen Physiol. 1985 Jan;85(1):43–64. doi: 10.1085/jgp.85.1.43. [DOI] [PMC free article] [PubMed] [Google Scholar]
- Powell T., Terrar D. A., Twist V. W. Electrical properties of individual cells isolated from adult rat ventricular myocardium. J Physiol. 1980 May;302:131–153. doi: 10.1113/jphysiol.1980.sp013234. [DOI] [PMC free article] [PubMed] [Google Scholar]
- Roos A., Boron W. F. Intracellular pH. Physiol Rev. 1981 Apr;61(2):296–434. doi: 10.1152/physrev.1981.61.2.296. [DOI] [PubMed] [Google Scholar]
- Szatkowski M. S., Thomas R. C. The intrinsic intracellular H+ buffering power of snail neurones. J Physiol. 1989 Feb;409:89–101. doi: 10.1113/jphysiol.1989.sp017486. [DOI] [PMC free article] [PubMed] [Google Scholar]
- Thomas R. C. The effect of carbon dioxide on the intracellular pH and buffering power of snail neurones. J Physiol. 1976 Mar;255(3):715–735. doi: 10.1113/jphysiol.1976.sp011305. [DOI] [PMC free article] [PubMed] [Google Scholar]
- Vanheel B., de Hemptinne A., Leusen I. Analysis of Cl- -HCO3(-) exchange during recovery from intracellular acidosis in cardiac Purkinje strands. Am J Physiol. 1984 May;246(5 Pt 1):C391–C400. doi: 10.1152/ajpcell.1984.246.5.C391. [DOI] [PubMed] [Google Scholar]
- Vanheel B., de Hemptinne A., Leusen I. Influence of surface pH on intracellular pH regulation in cardiac and skeletal muscle. Am J Physiol. 1986 May;250(5 Pt 1):C748–C760. doi: 10.1152/ajpcell.1986.250.5.C748. [DOI] [PubMed] [Google Scholar]
- Vanheel B., de Hemptinne A., Leusen I. Intracellular pH and contraction of isolated rabbit and cat papillary muscle: effect of superfusate buffering. J Mol Cell Cardiol. 1985 Jan;17(1):23–29. doi: 10.1016/s0022-2828(85)80089-4. [DOI] [PubMed] [Google Scholar]
- Vaughan-Jones R. D., Kaila K. The sensitivity of liquid sensor, ion-selective microelectrodes to changes in temperature and solution level. Pflugers Arch. 1986 Jun;406(6):641–644. doi: 10.1007/BF00584033. [DOI] [PubMed] [Google Scholar]
- Vaughan-Jones R. D. Regulation of chloride in quiescent sheep-heart Purkinje fibres studied using intracellular chloride and pH-sensitive micro-electrodes. J Physiol. 1979 Oct;295:111–137. doi: 10.1113/jphysiol.1979.sp012957. [DOI] [PMC free article] [PubMed] [Google Scholar]
- Weissberg P. L., Little P. J., Cragoe E. J., Jr, Bobik A. The pH of spontaneously beating cultured rat heart cells is regulated by an ATP-calmodulin-dependent Na+/H+ antiport. Circ Res. 1989 Apr;64(4):676–685. doi: 10.1161/01.res.64.4.676. [DOI] [PubMed] [Google Scholar]
- Wolfe C. L., Gilbert H. F., Brindle K. M., Radda G. K. Determination of buffering capacity of rat myocardium during ischemia. Biochim Biophys Acta. 1988 Aug 19;971(1):9–20. doi: 10.1016/0167-4889(88)90156-5. [DOI] [PubMed] [Google Scholar]