Abstract
1. Simultaneous whole-cell patch-clamp and Fura-2 microfluorimetric recordings of calcium currents (ICa) and the intracellular free Ca2+ concentration ([Ca2+]i) were made from neurones grown in primary culture from the dorsal root ganglion of the rat. 2. Cells held at -80 mV and depolarized to 0 mV elicited a ICa that resulted in an [Ca2+]i transient which was not significantly buffered during the voltage step and lasted long after the cell had repolarized and the current ceased. The process by which the cell buffered [Ca2+]i back to basal levels could best be described with a single-exponential equation. 3. The membrane potential versus ICa and [Ca2+]i relationship revealed that the peak of the [Ca2+]i transient evoked at a given test potential closely paralleled the magnitude of the ICa suggesting that neither voltage-dependent nor Ca2(+)-induced Ca2+ release from intracellular stores made a significant contribution to the [Ca2+]i transient. 4. When the cell was challenged with Ca2+ loads of different magnitude by varying the duration or potential of the test pulse, [Ca2+]i buffering was more effective for larger Ca2+ loads. The relationship between the integrated ICa and the peak of the [Ca2+]i transient reached an asymptote at large Ca2+ loads indicating that Ca2(+)-dependent processes became more efficient or that low-affinity processes had been recruited. 5. Inhibition of Ca2+ influx with neuropeptide Y demonstrated that inhibition of a large ICa produced minor alterations in the peak of the [Ca2+]i transient, while inhibition of smaller currents produced corresponding decreases in the [Ca2+]i transient. Thus, inhibition of the ICa was reflected by a change in the peak [Ca2+]i only when submaximal Ca2+ loads were applied to the cell, implying that modulation of [Ca2+]i is dependent on the activation state of the cells. 6. Intracellular dialysis with the mitochondrial Ca2+ uptake blocker Ruthenium Red in whole-cell patch-clamp experiments removed the buffering component which was responsible for the more efficient removal of [Ca2+]i observed when large Ca2+ loads were applied to the cell. 7. When cells were superfused with 50 mM-K+, [Ca2+]i transients recorded from the cell soma returned to control levels very slowly. Pharmacological studies indicated that mitochondria were cycling Ca2+ during this sustained elevation in [Ca2+]i. In contrast, [Ca2+]i transients recorded from cell processes returned to basal levels relatively rapidly. 8. Extracellular Na(+)-dependent Ca2+ efflux did not significantly contribute to buffering [Ca2+]i transients in dorsal root ganglion neurone cell bodies.(ABSTRACT TRUNCATED AT 400 WORDS)
Full text
PDF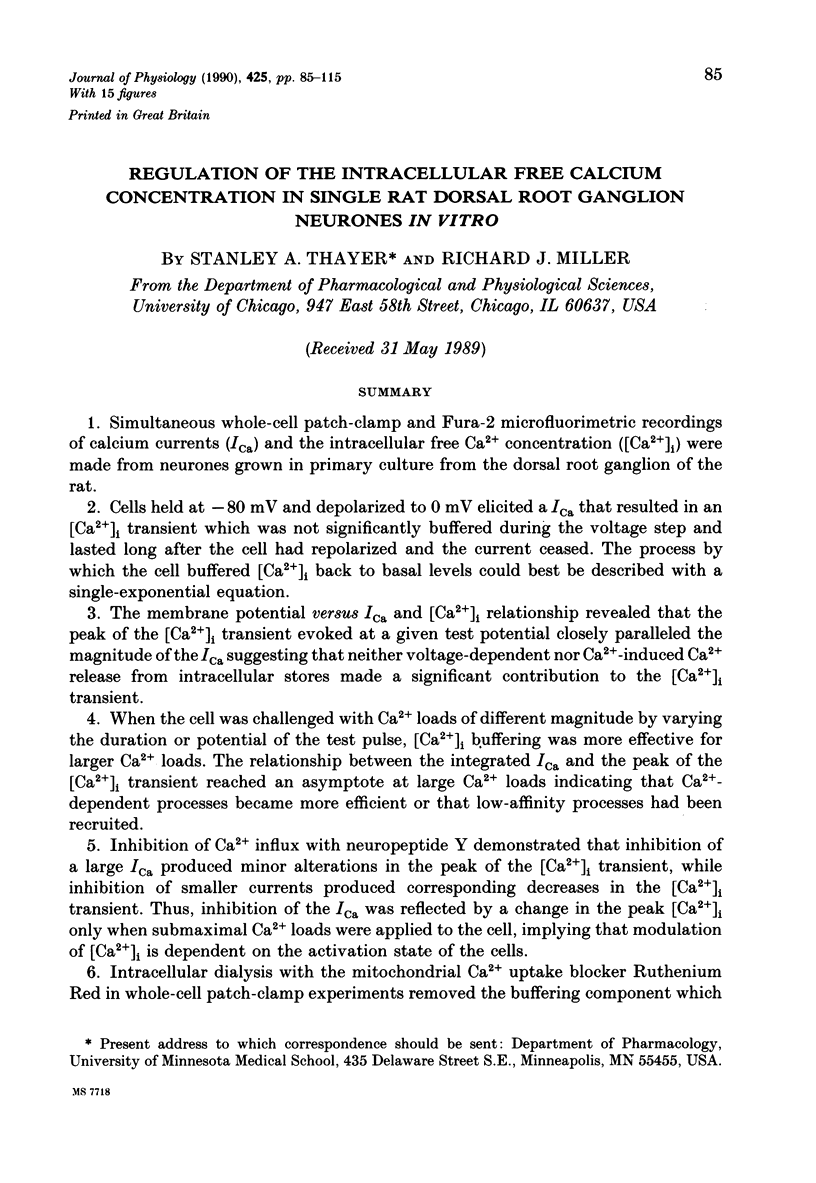
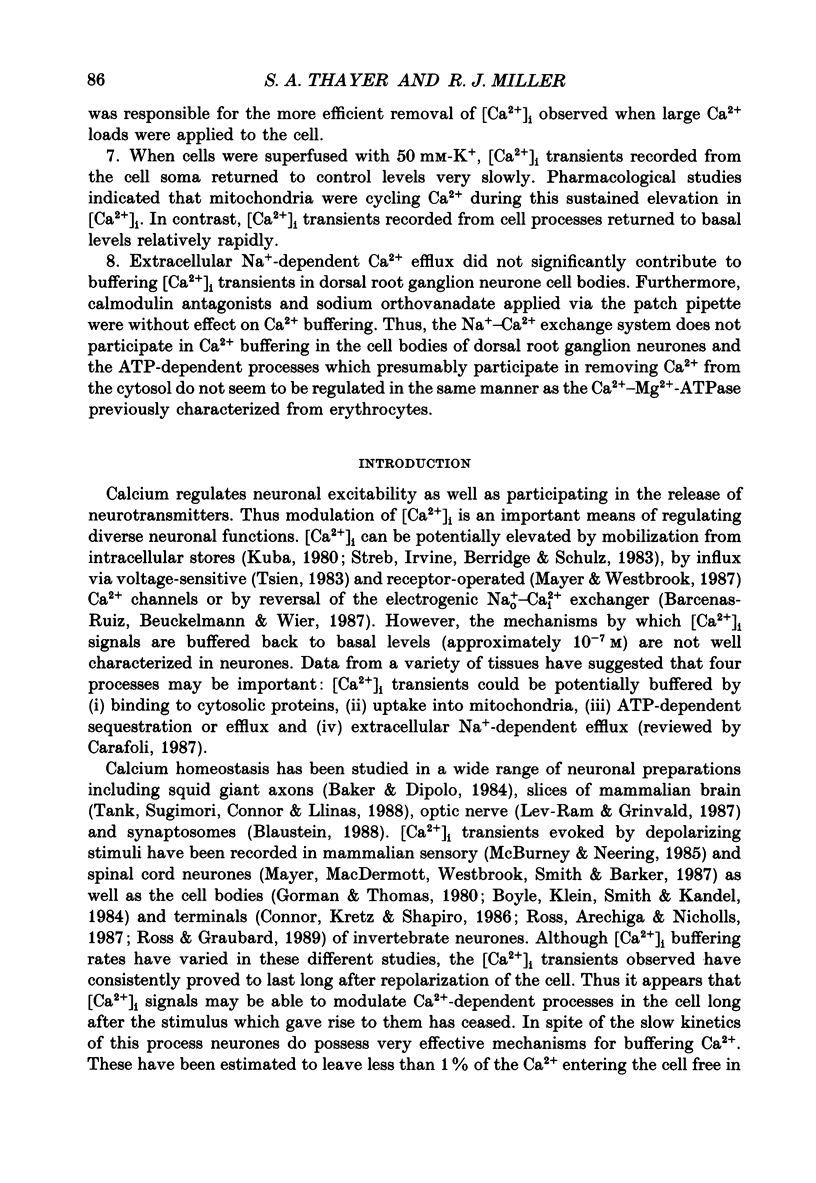
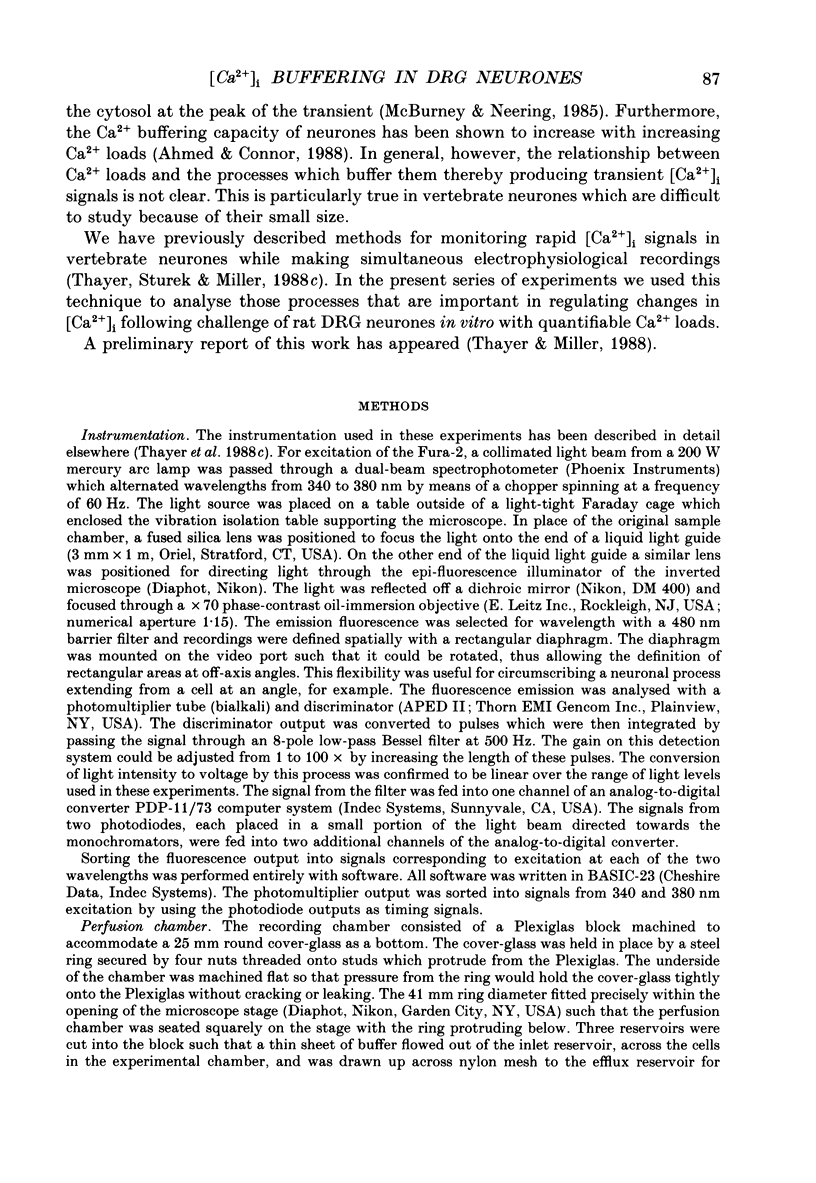
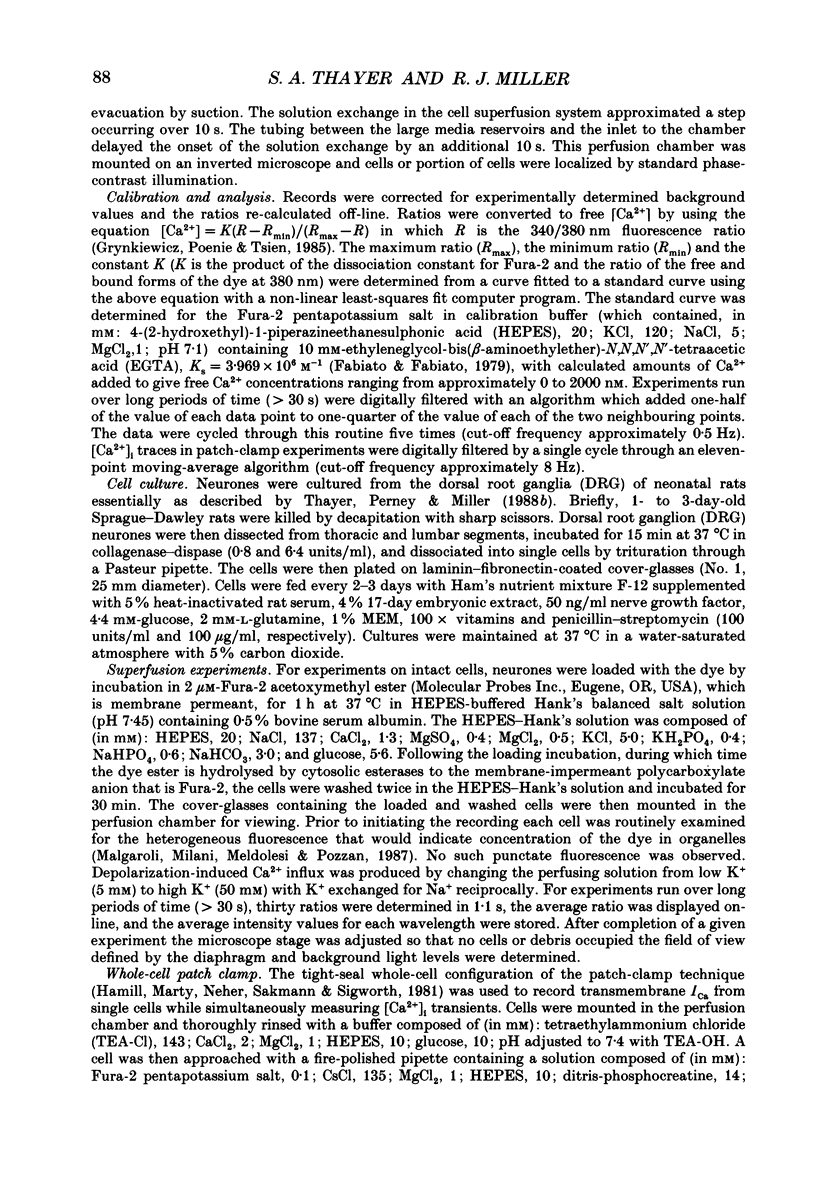
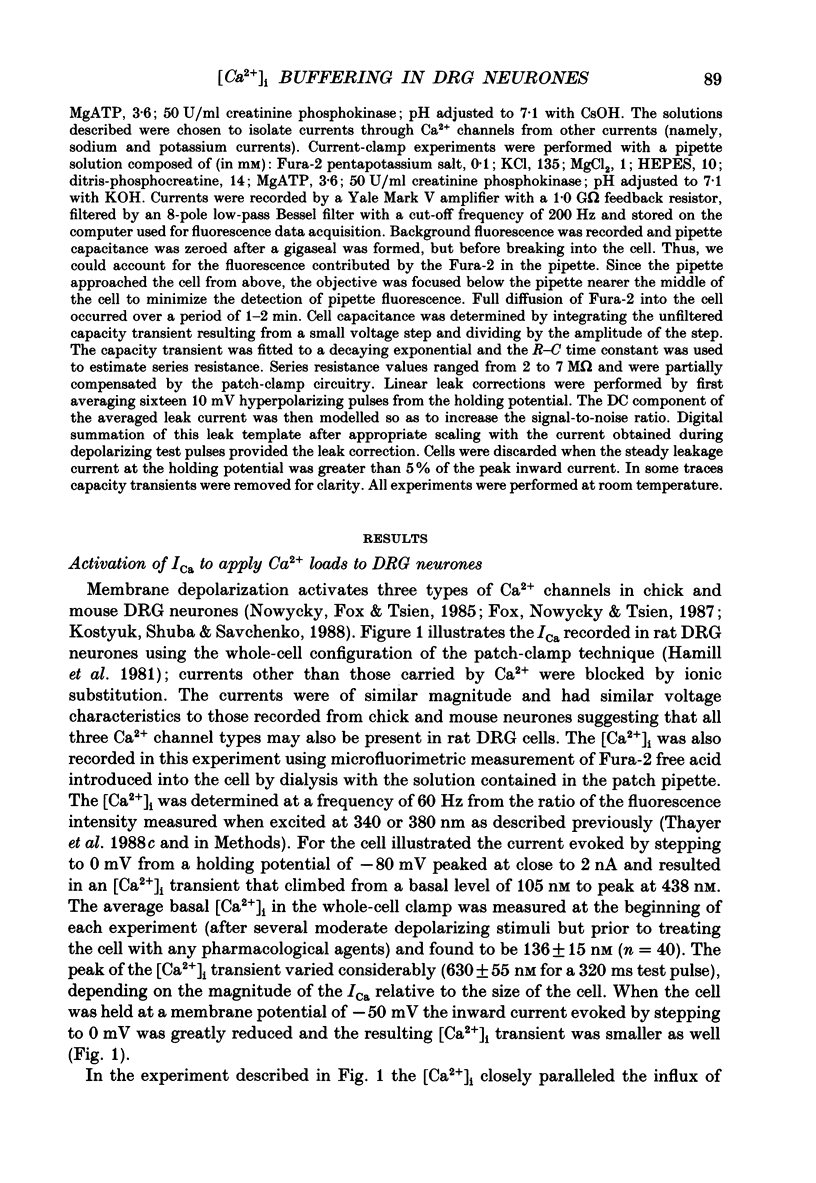
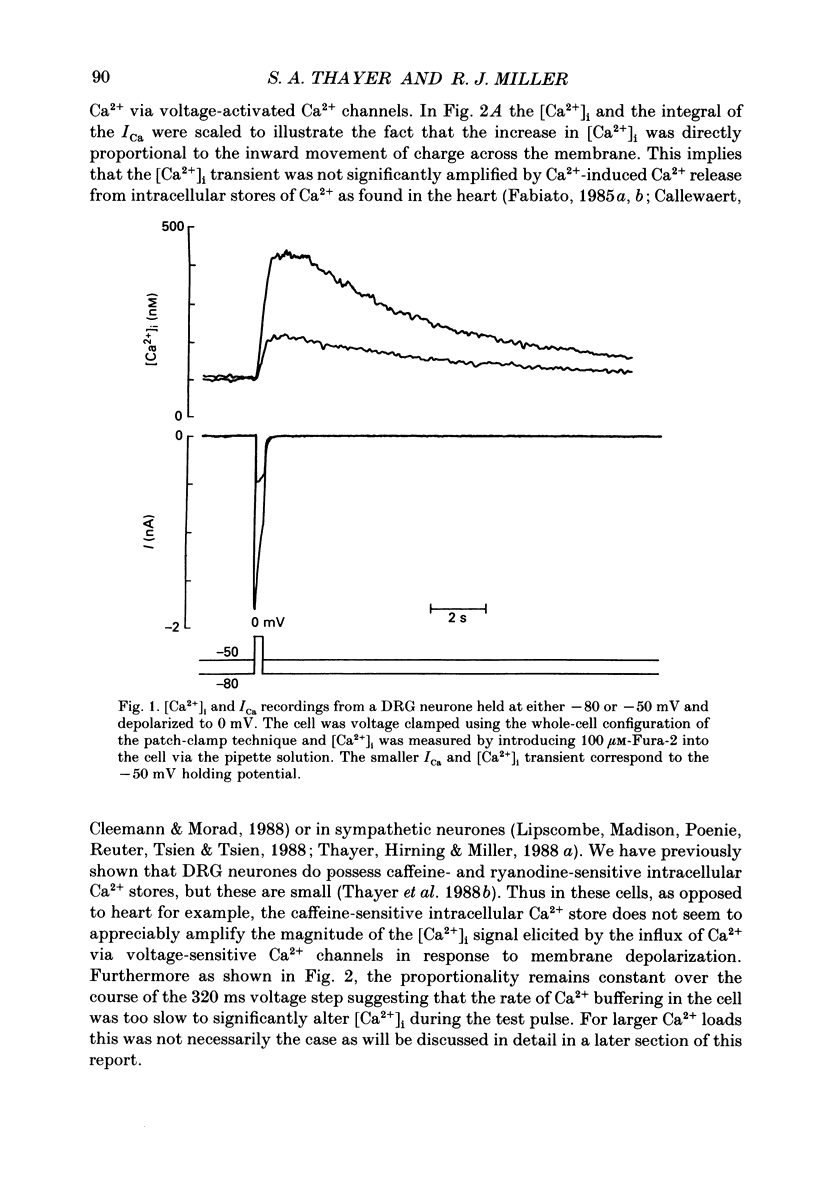
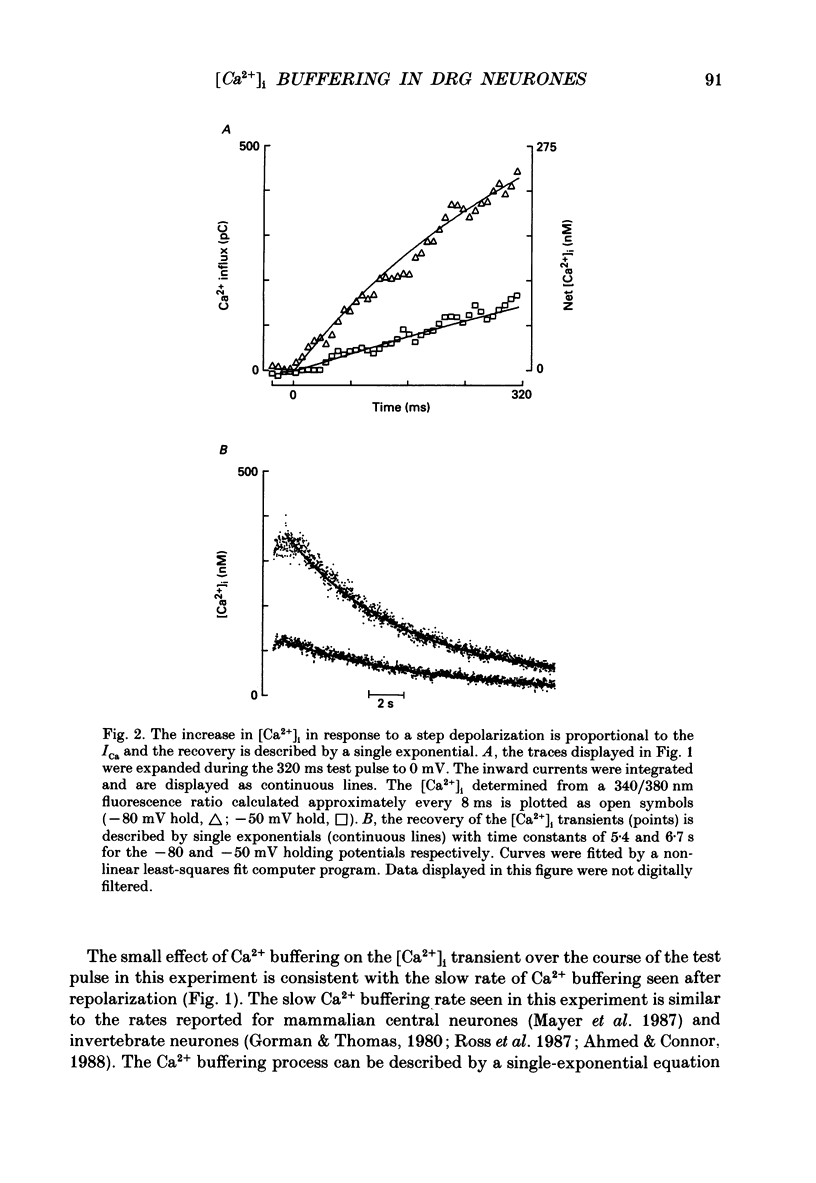
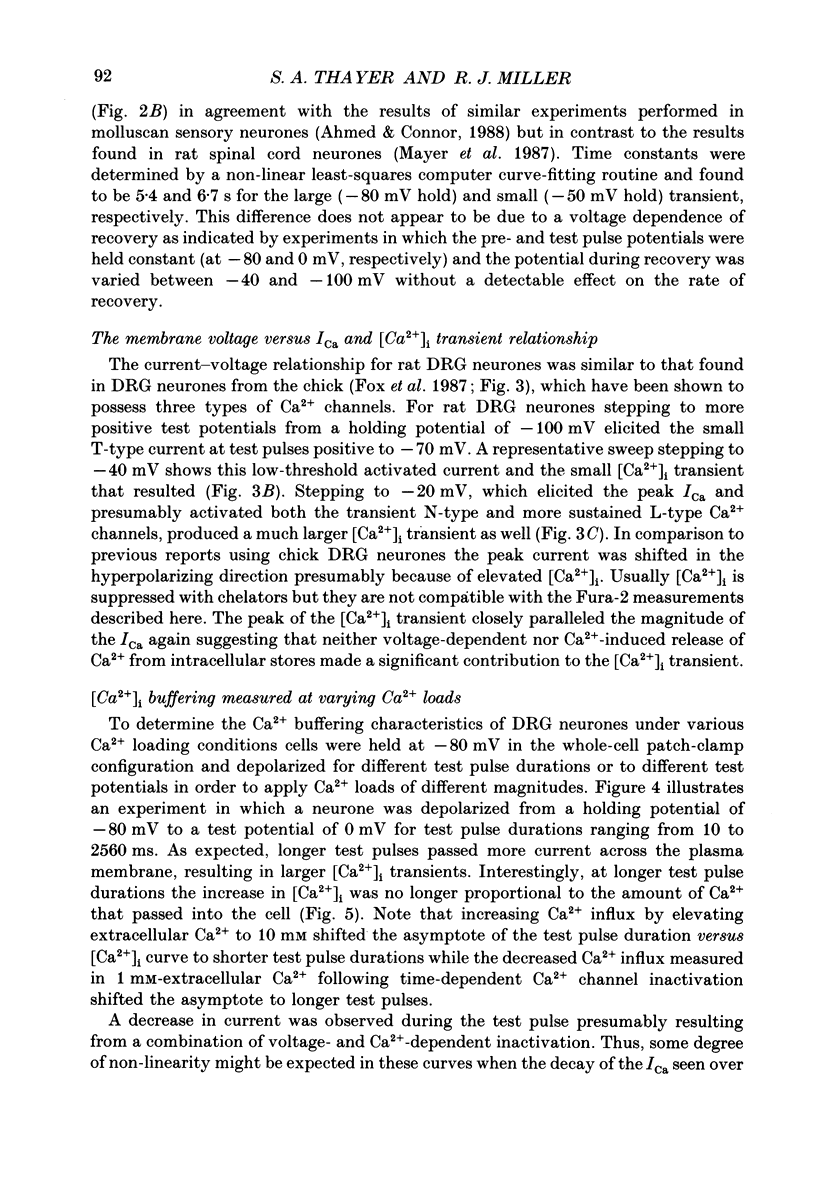
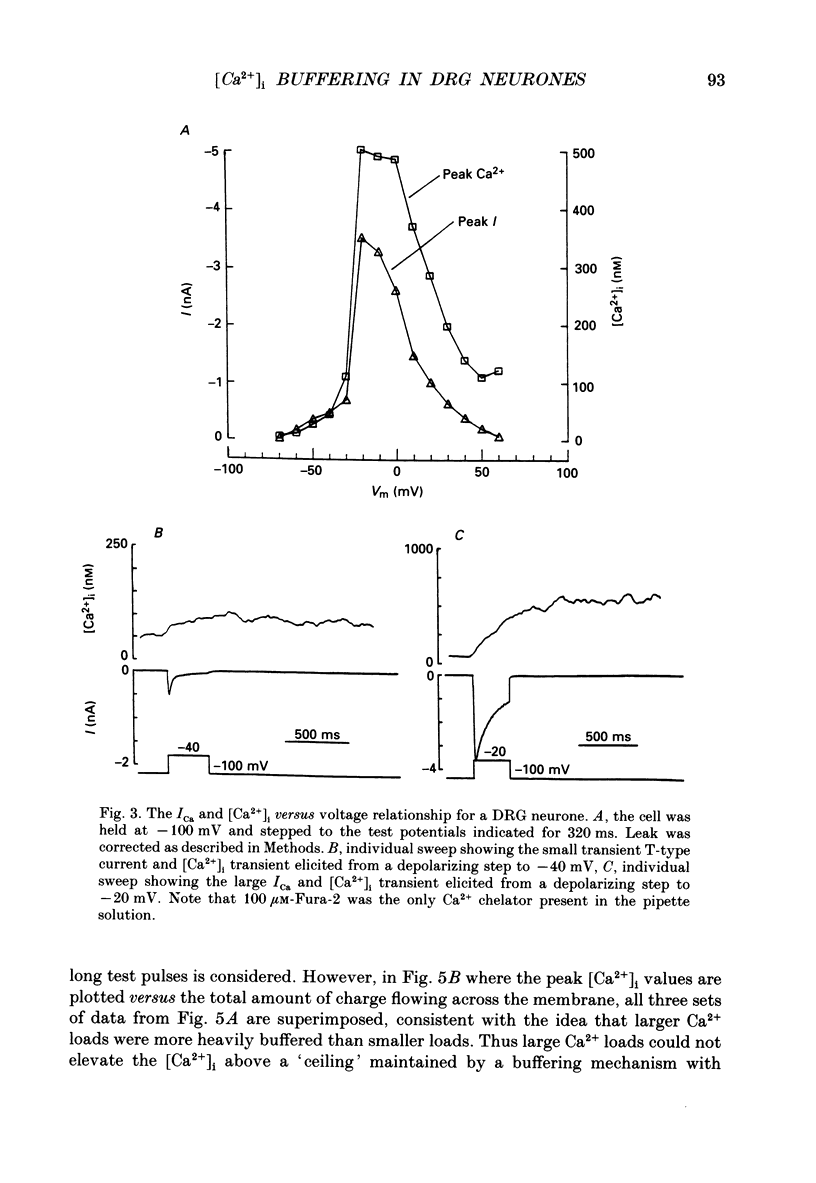
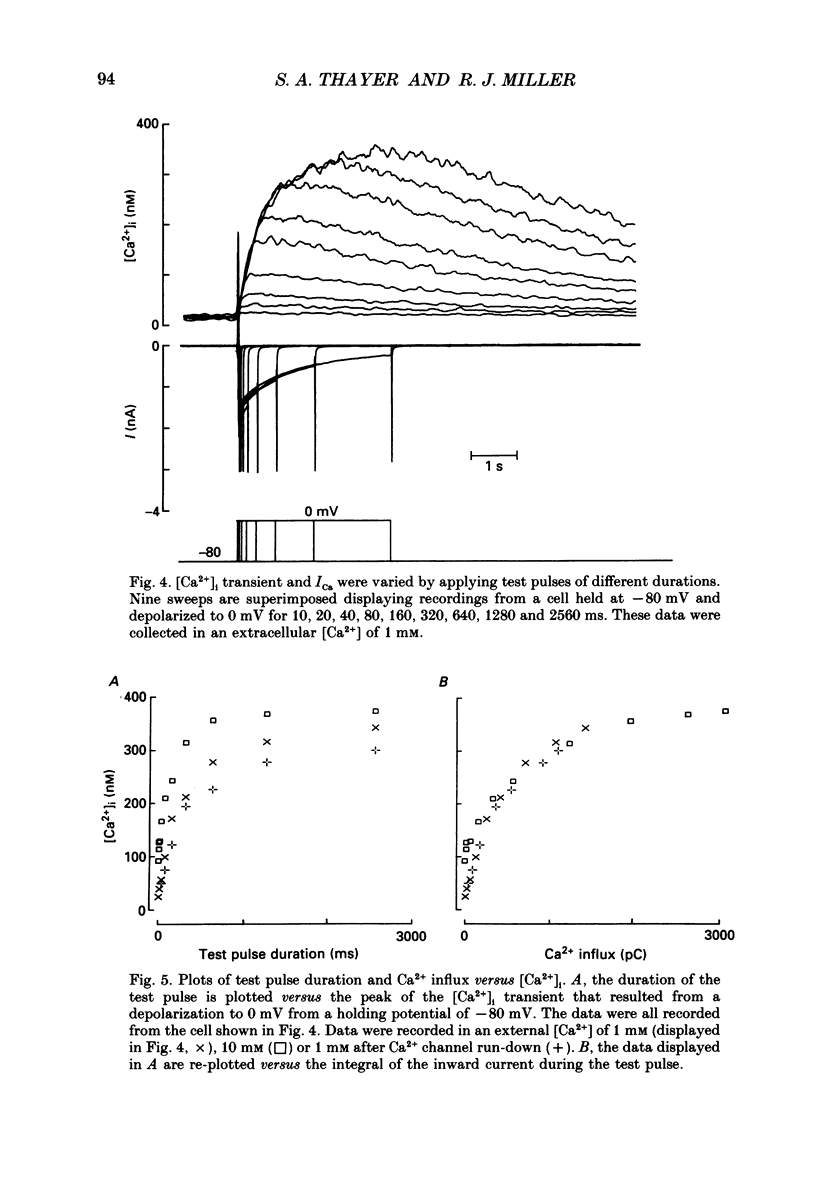
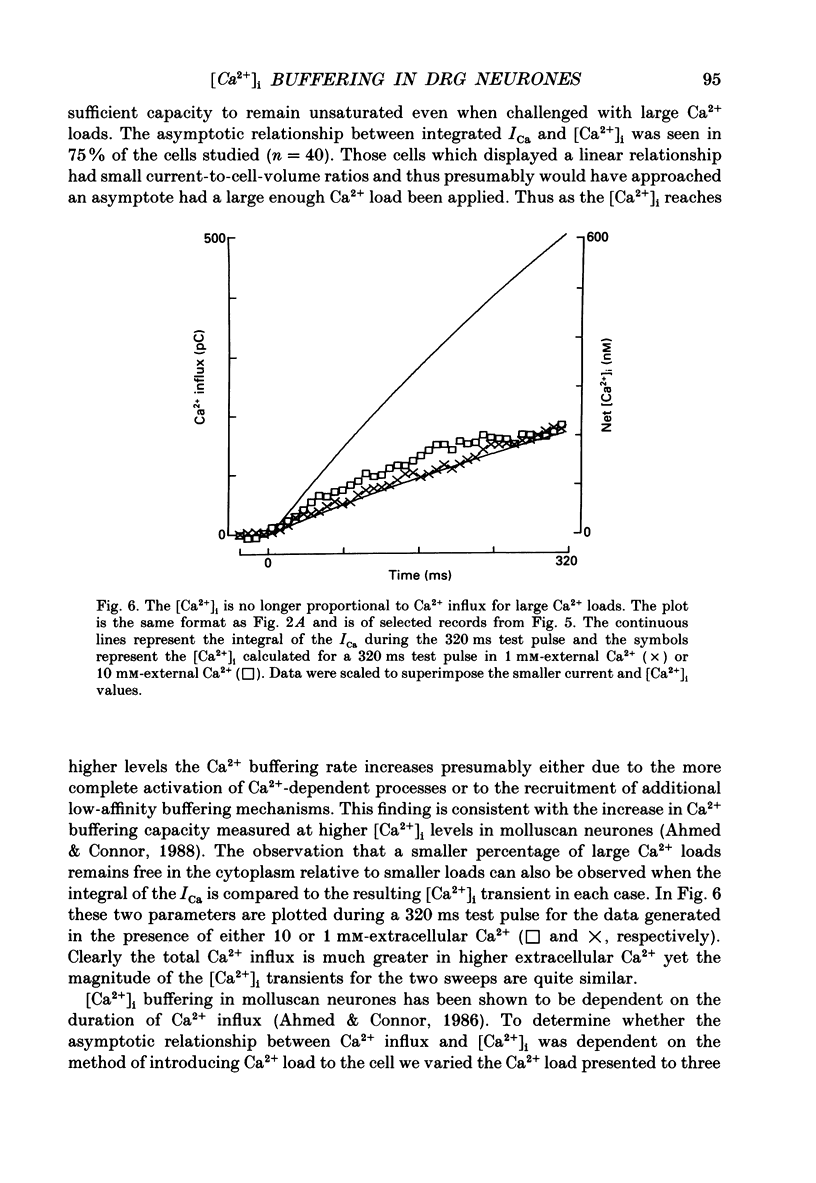
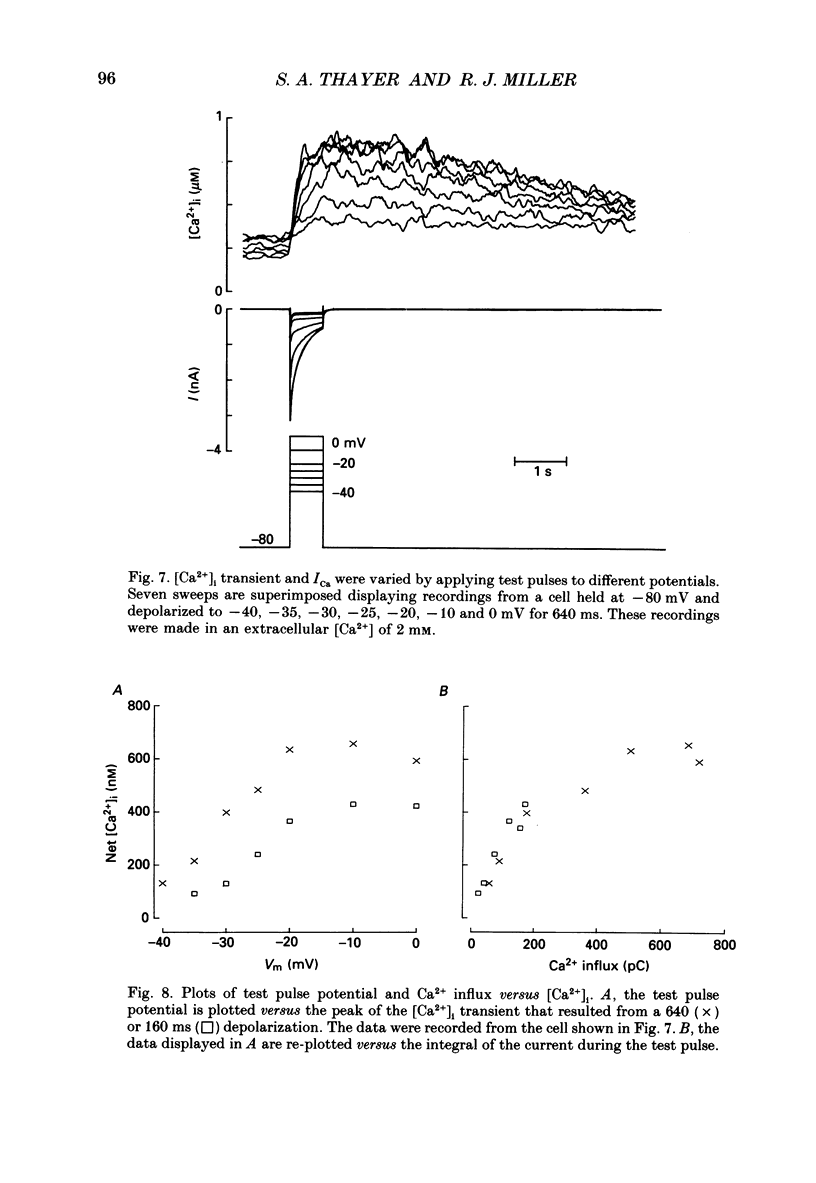
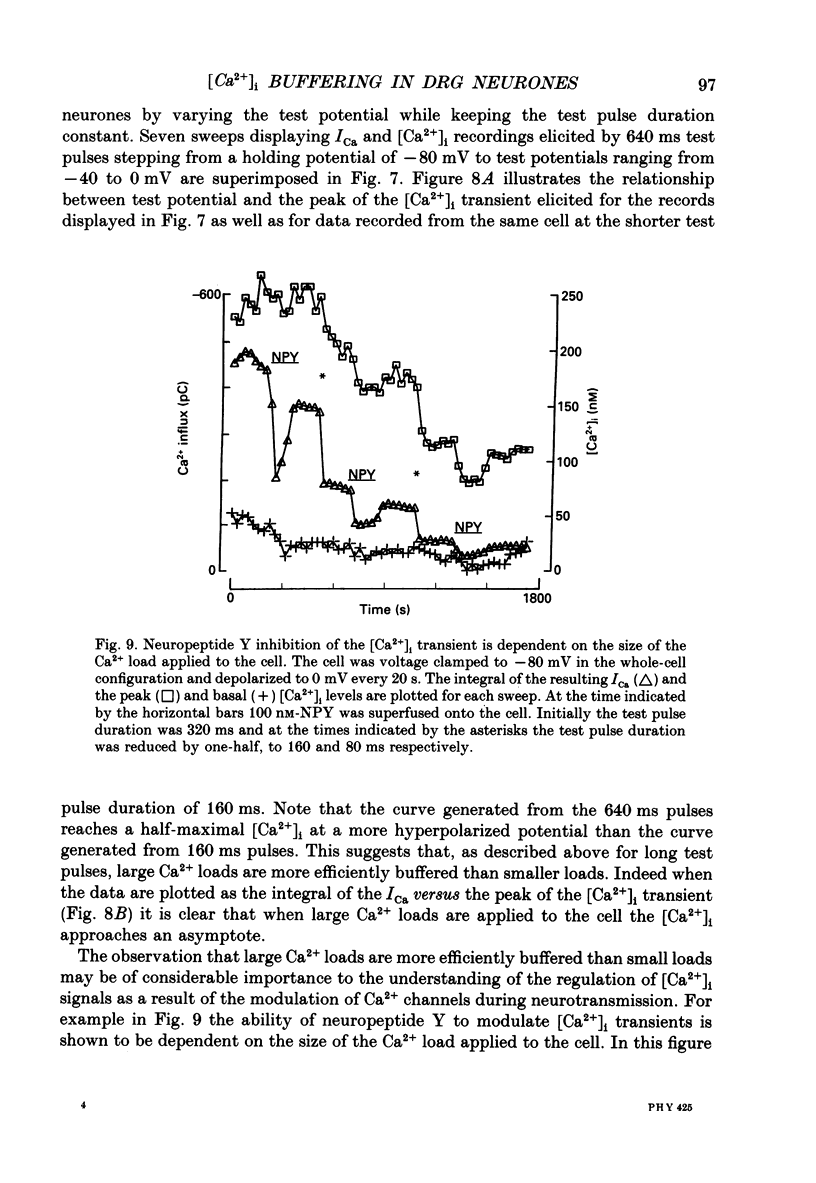
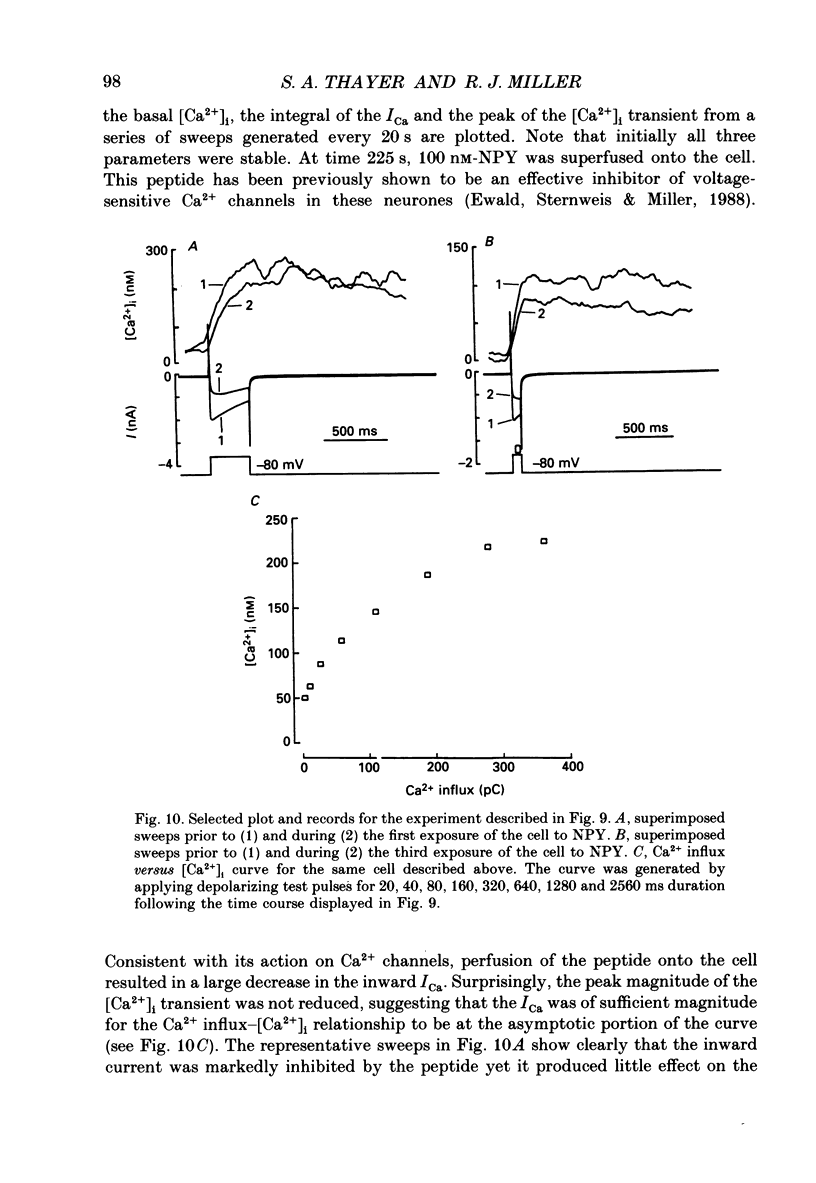
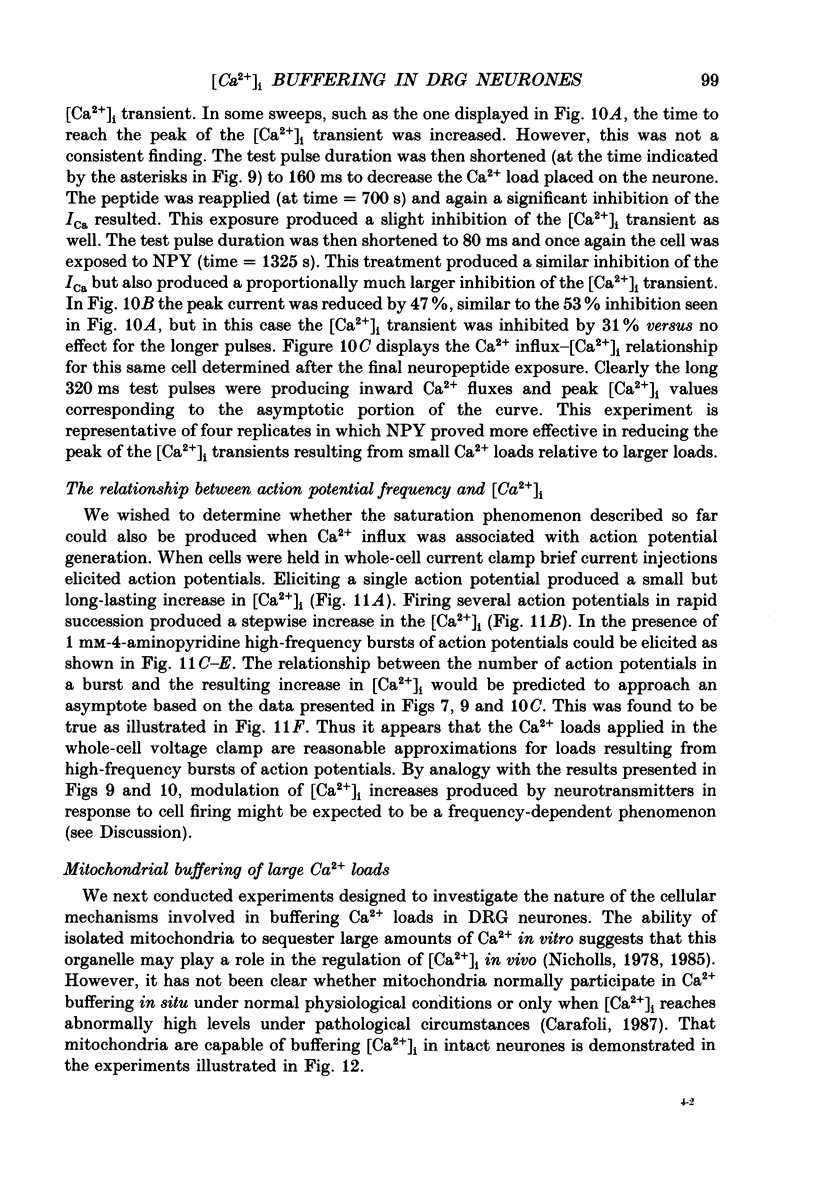
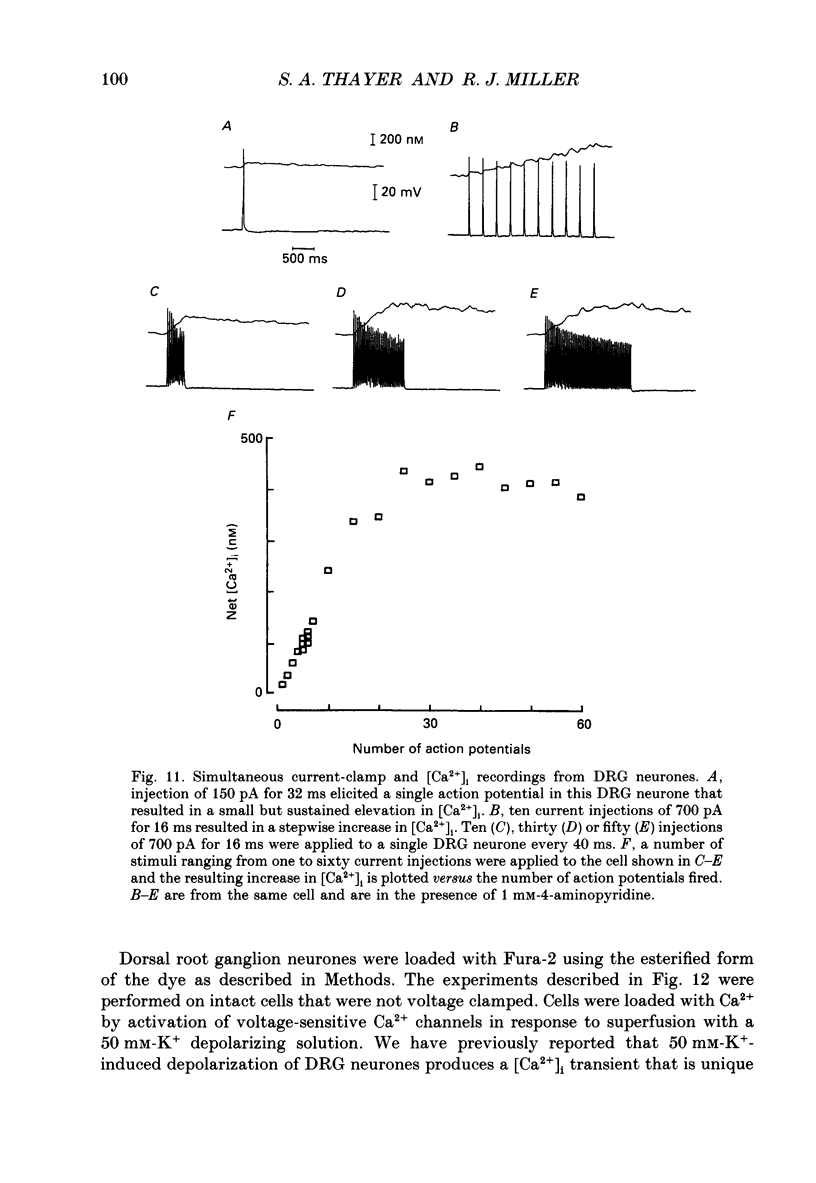
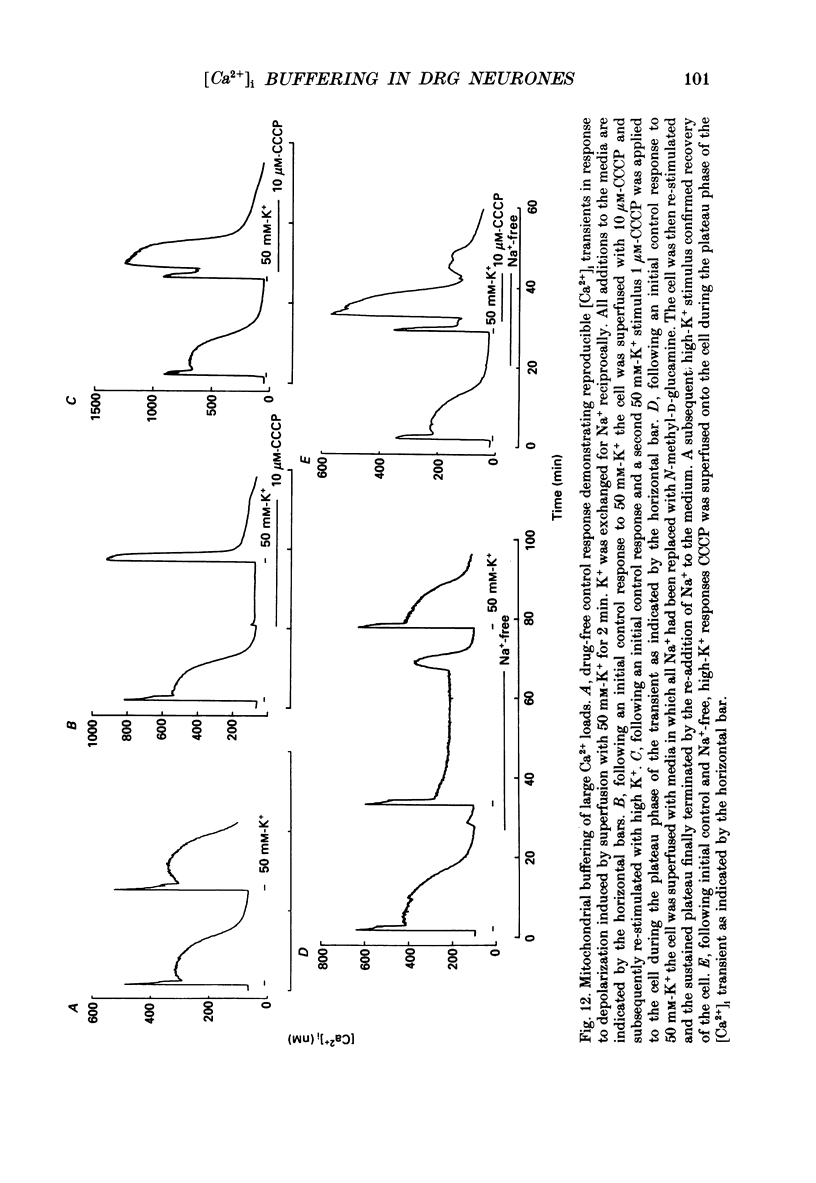
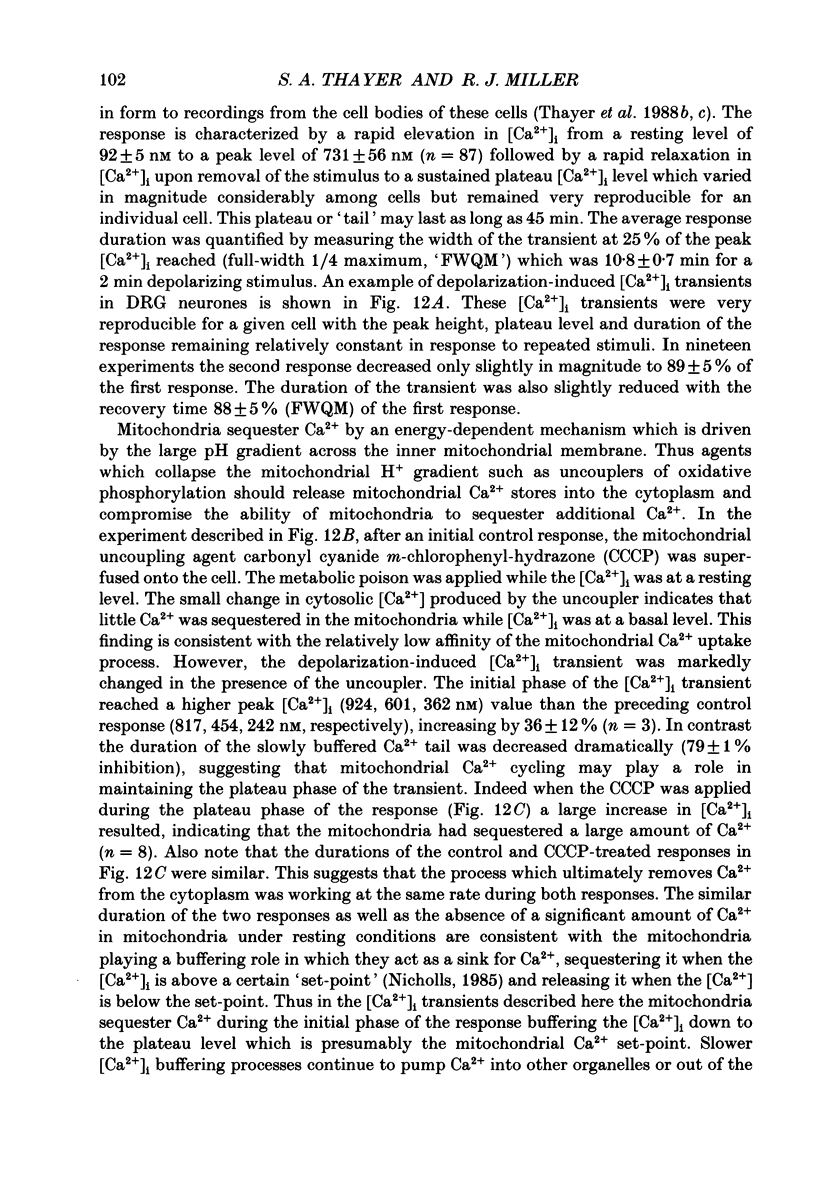
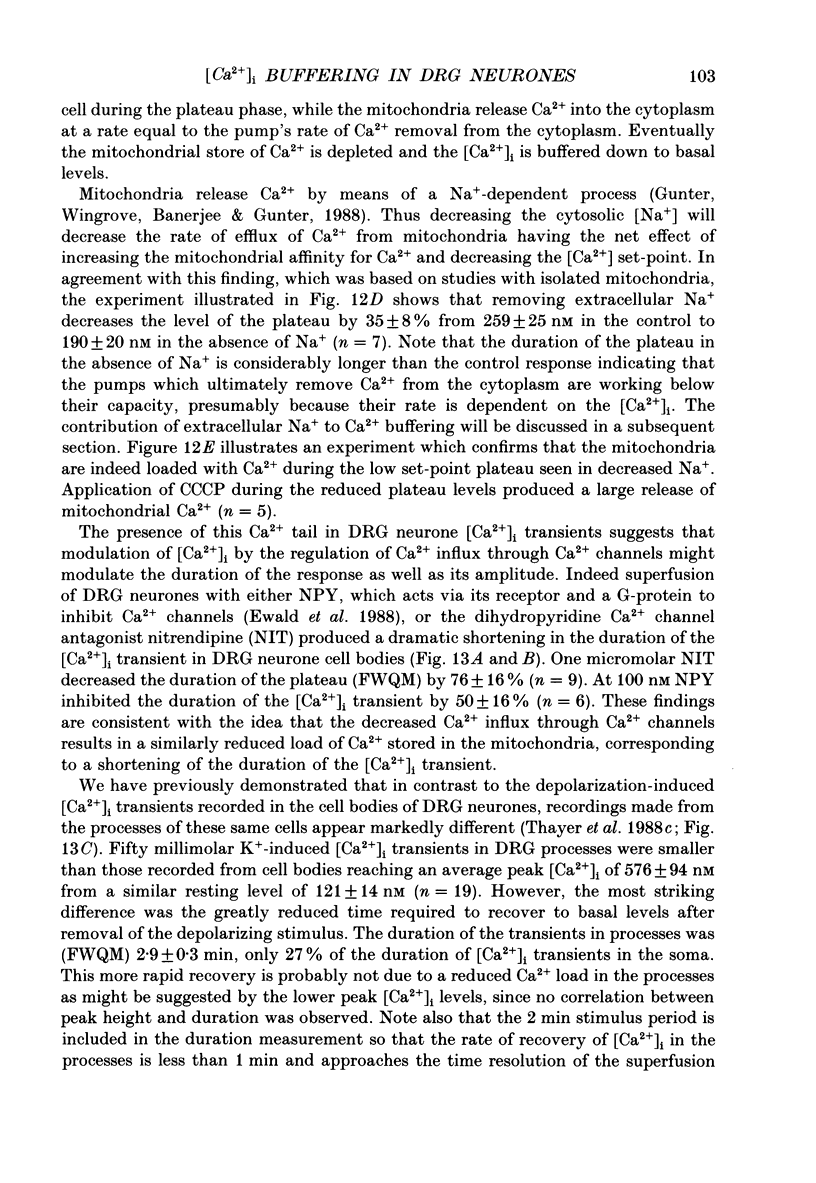
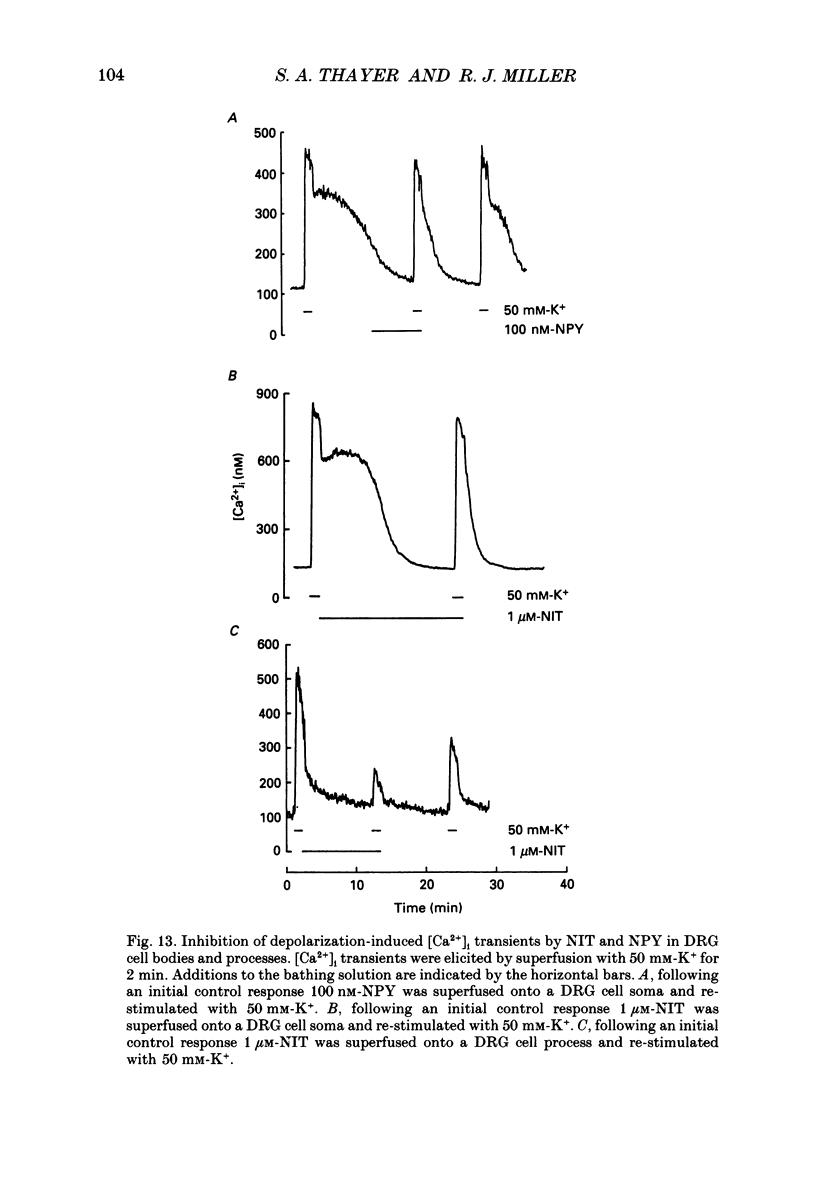
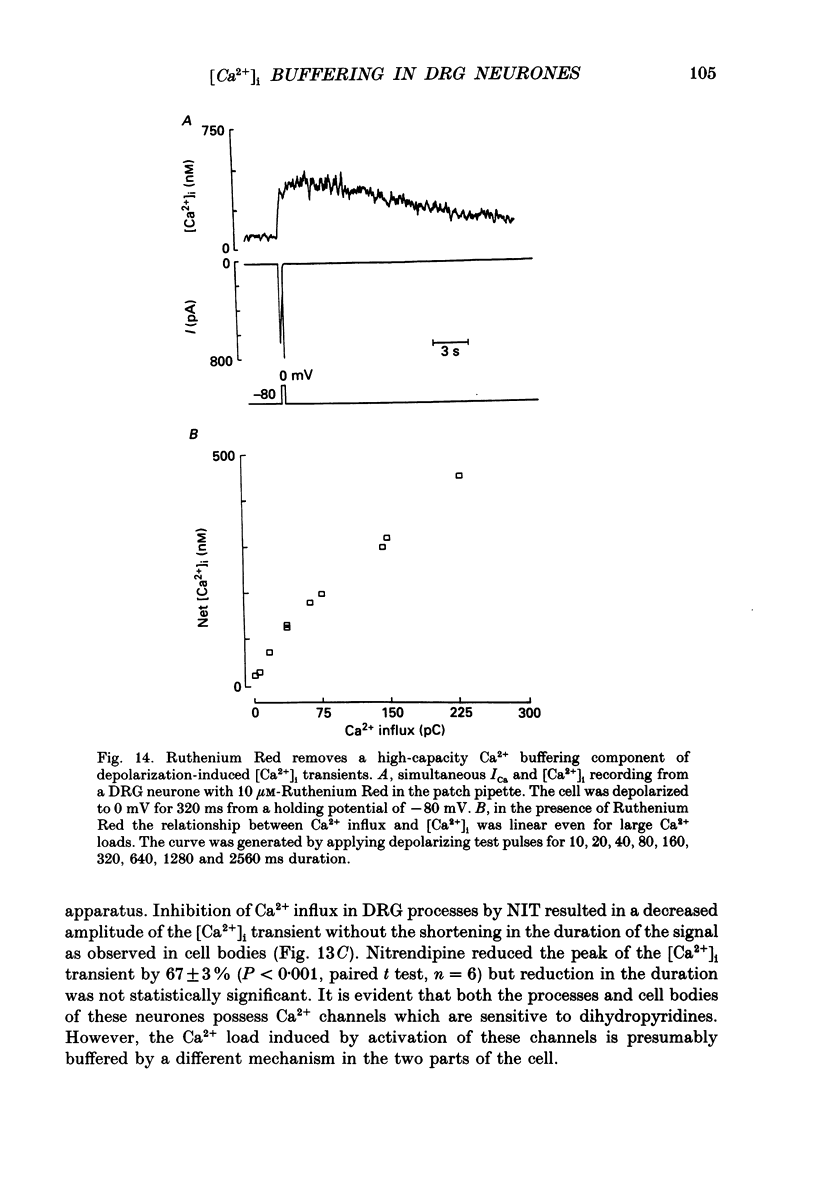
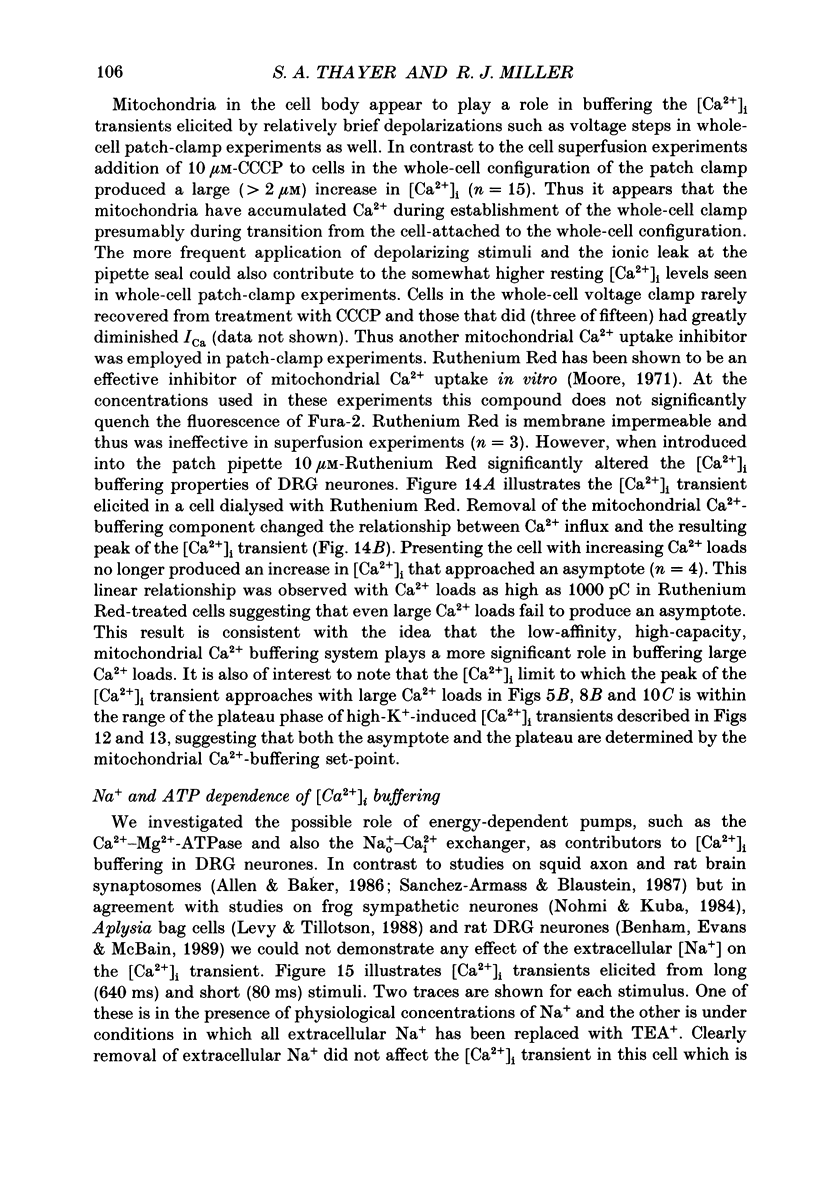
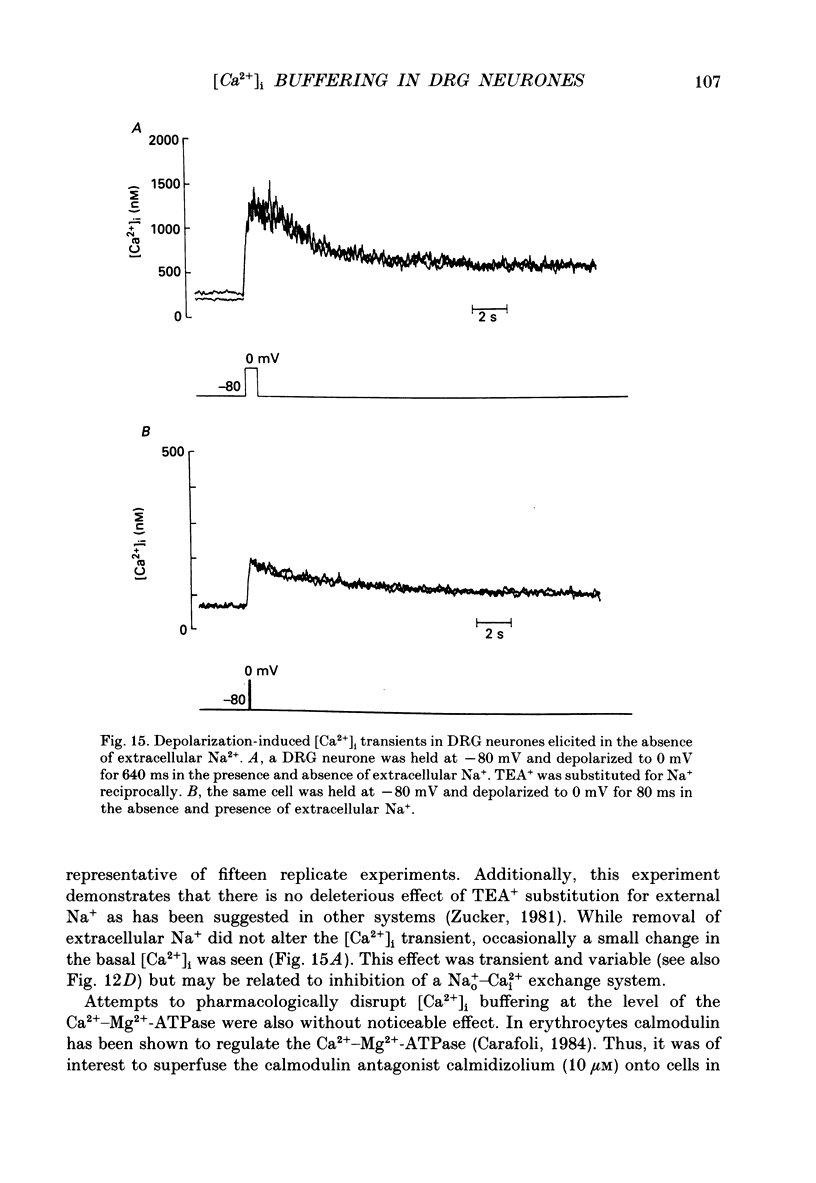
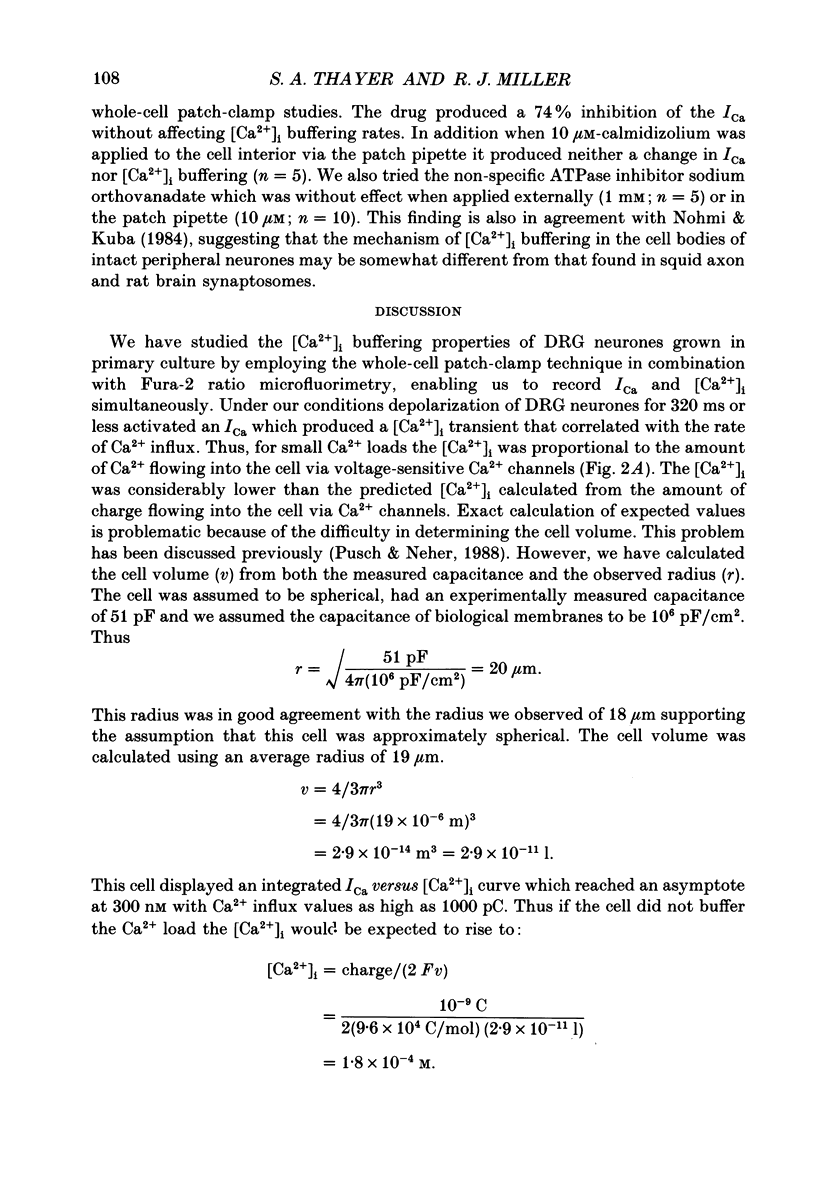
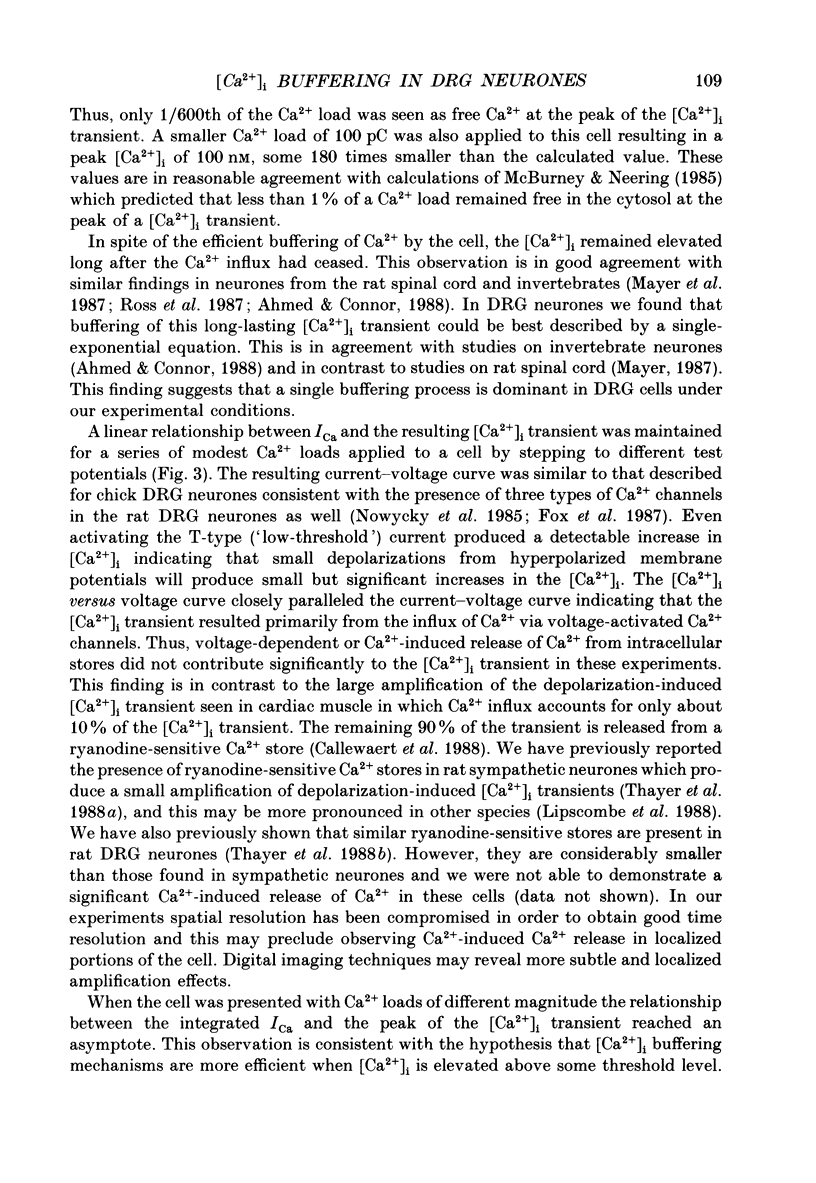
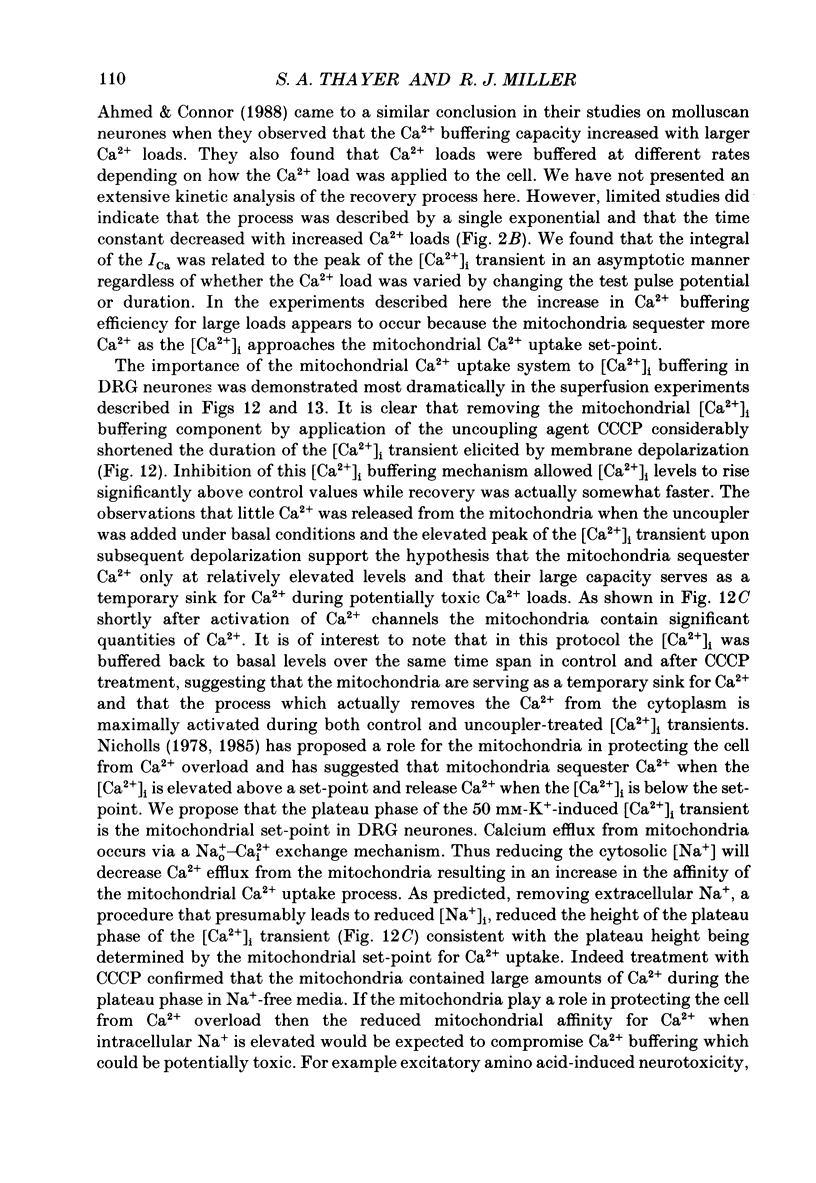
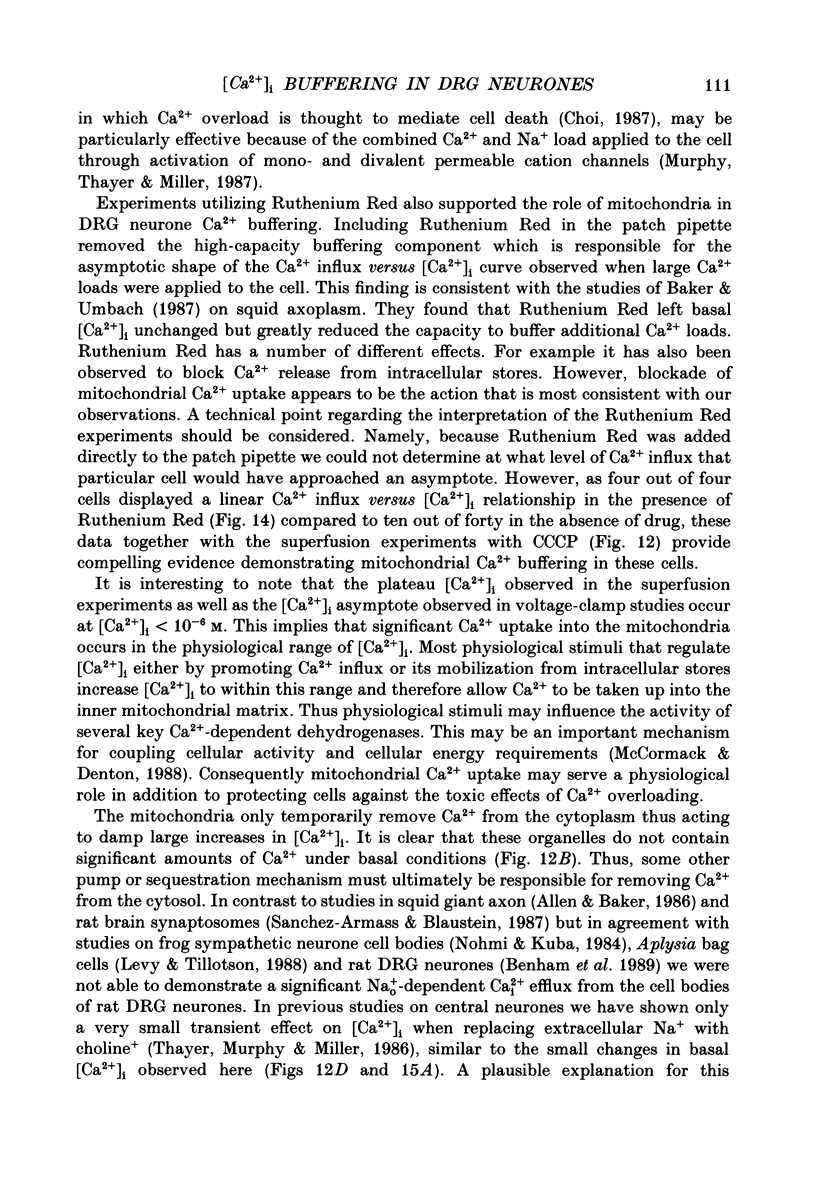
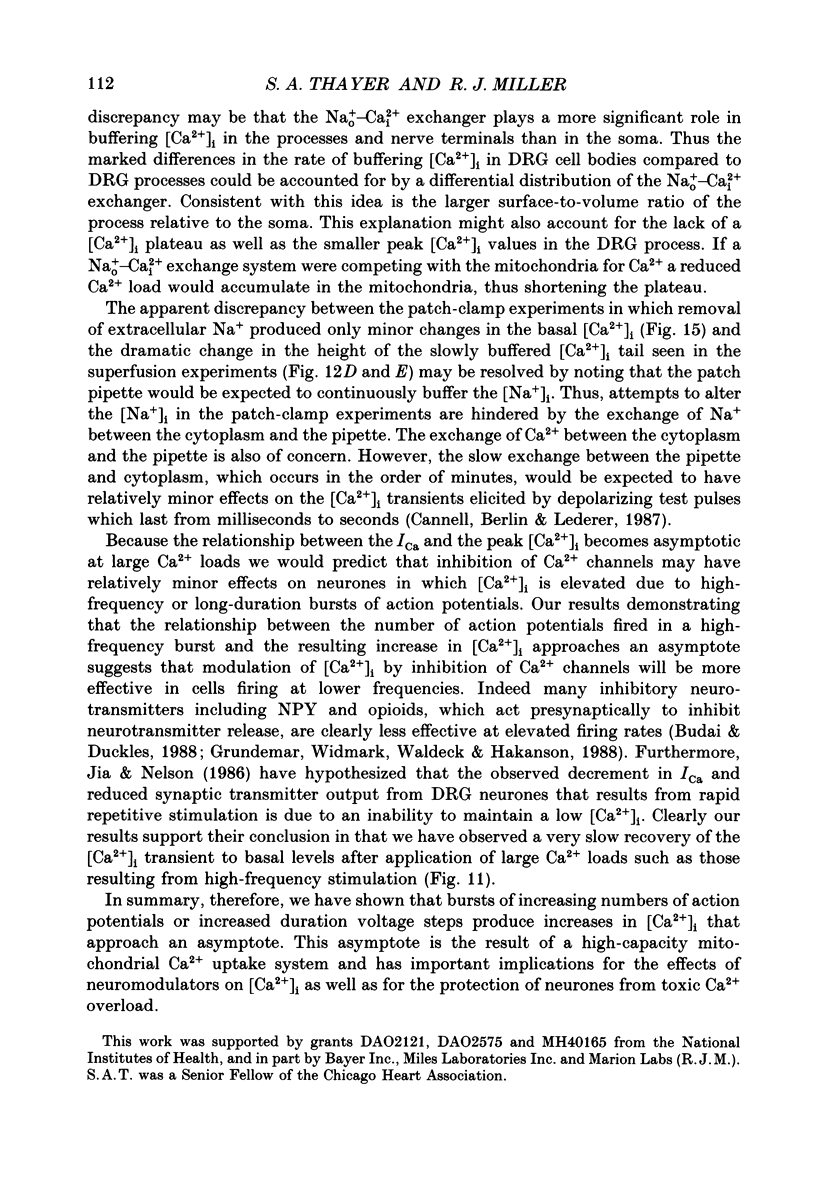
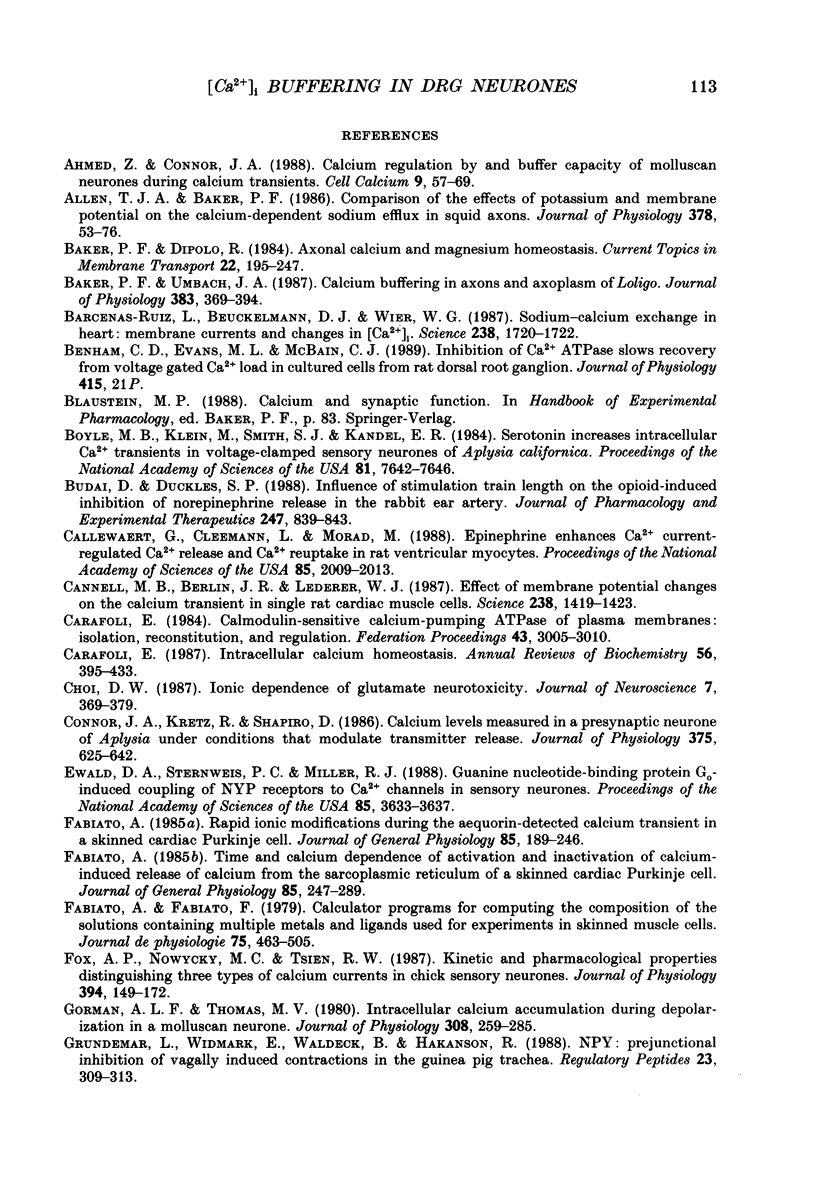
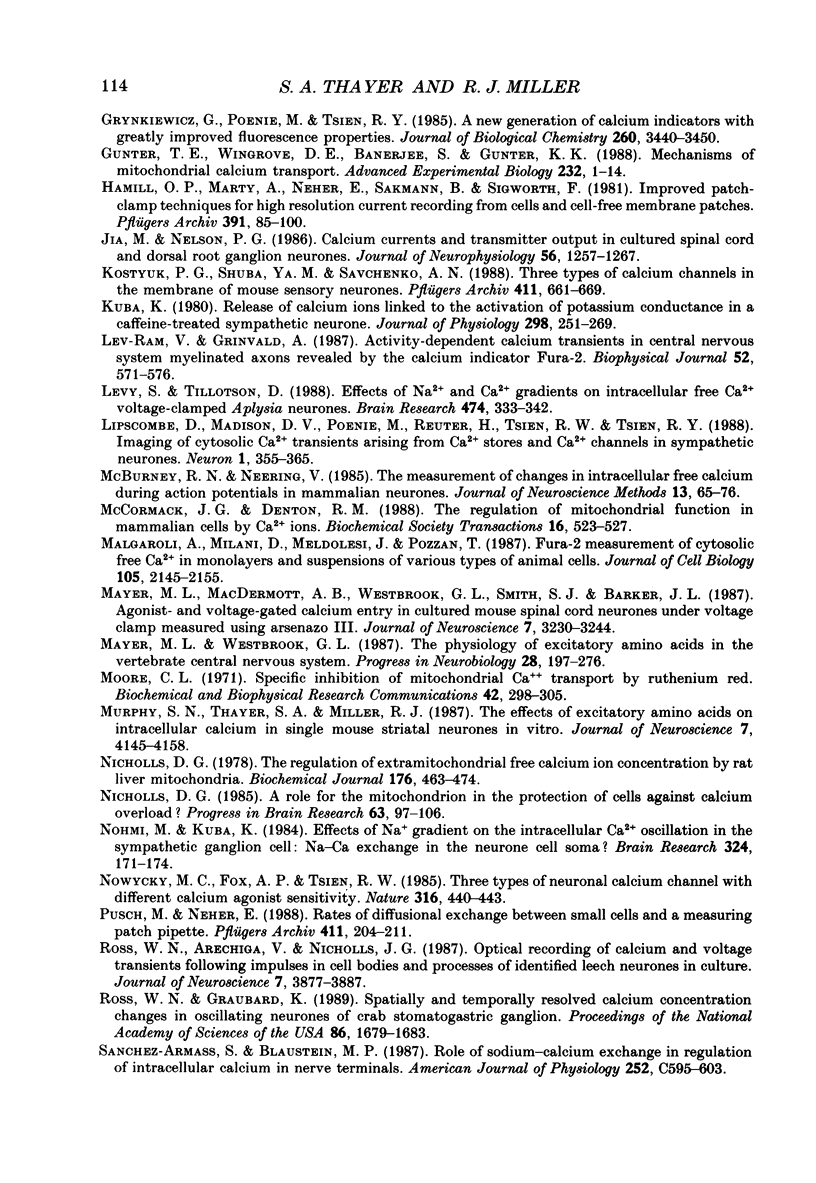
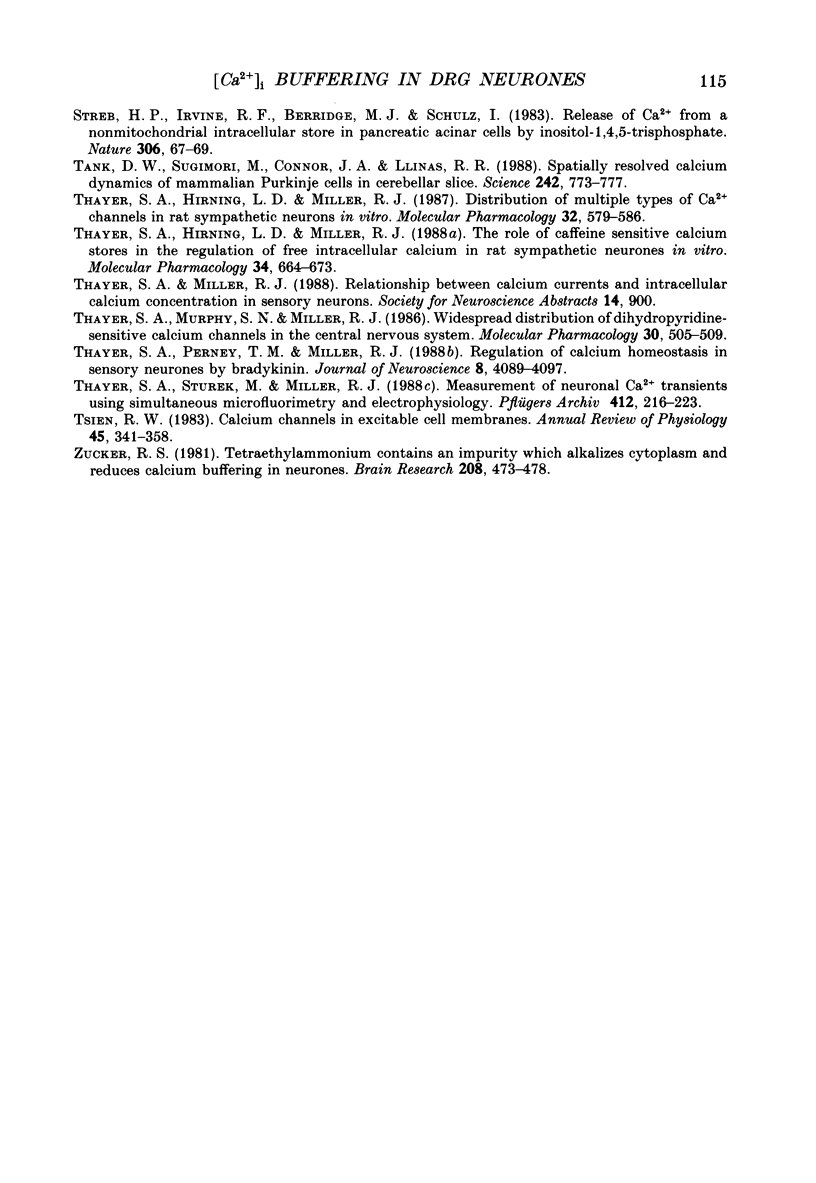
Selected References
These references are in PubMed. This may not be the complete list of references from this article.
- Ahmed Z., Connor J. A. Calcium regulation by and buffer capacity of molluscan neurons during calcium transients. Cell Calcium. 1988 Apr;9(2):57–69. doi: 10.1016/0143-4160(88)90025-5. [DOI] [PubMed] [Google Scholar]
- Allen T. J., Baker P. F. Comparison of the effects of potassium and membrane potential on the calcium-dependent sodium efflux in squid axons. J Physiol. 1986 Sep;378:53–76. doi: 10.1113/jphysiol.1986.sp016207. [DOI] [PMC free article] [PubMed] [Google Scholar]
- Baker P. F., Umbach J. A. Calcium buffering in axons and axoplasm of Loligo. J Physiol. 1987 Feb;383:369–394. doi: 10.1113/jphysiol.1987.sp016414. [DOI] [PMC free article] [PubMed] [Google Scholar]
- Barcenas-Ruiz L., Beuckelmann D. J., Wier W. G. Sodium-calcium exchange in heart: membrane currents and changes in [Ca2+]i. Science. 1987 Dec 18;238(4834):1720–1722. doi: 10.1126/science.3686010. [DOI] [PubMed] [Google Scholar]
- Boyle M. B., Klein M., Smith S. J., Kandel E. R. Serotonin increases intracellular Ca2+ transients in voltage-clamped sensory neurons of Aplysia californica. Proc Natl Acad Sci U S A. 1984 Dec;81(23):7642–7646. doi: 10.1073/pnas.81.23.7642. [DOI] [PMC free article] [PubMed] [Google Scholar]
- Budai D., Duckles S. P. Influence of stimulation train length on the opioid-induced inhibition of norepinephrine release in the rabbit ear artery. J Pharmacol Exp Ther. 1988 Dec;247(3):839–843. [PubMed] [Google Scholar]
- Callewaert G., Cleemann L., Morad M. Epinephrine enhances Ca2+ current-regulated Ca2+ release and Ca2+ reuptake in rat ventricular myocytes. Proc Natl Acad Sci U S A. 1988 Mar;85(6):2009–2013. doi: 10.1073/pnas.85.6.2009. [DOI] [PMC free article] [PubMed] [Google Scholar]
- Cannell M. B., Berlin J. R., Lederer W. J. Effect of membrane potential changes on the calcium transient in single rat cardiac muscle cells. Science. 1987 Dec 4;238(4832):1419–1423. doi: 10.1126/science.2446391. [DOI] [PubMed] [Google Scholar]
- Carafoli E. Calmodulin-sensitive calcium-pumping ATPase of plasma membranes: isolation, reconstitution, and regulation. Fed Proc. 1984 Dec;43(15):3005–3010. [PubMed] [Google Scholar]
- Carafoli E. Intracellular calcium homeostasis. Annu Rev Biochem. 1987;56:395–433. doi: 10.1146/annurev.bi.56.070187.002143. [DOI] [PubMed] [Google Scholar]
- Choi D. W. Ionic dependence of glutamate neurotoxicity. J Neurosci. 1987 Feb;7(2):369–379. doi: 10.1523/JNEUROSCI.07-02-00369.1987. [DOI] [PMC free article] [PubMed] [Google Scholar]
- Connor J. A., Kretz R., Shapiro E. Calcium levels measured in a presynaptic neurone of Aplysia under conditions that modulate transmitter release. J Physiol. 1986 Jun;375:625–642. doi: 10.1113/jphysiol.1986.sp016137. [DOI] [PMC free article] [PubMed] [Google Scholar]
- Ewald D. A., Sternweis P. C., Miller R. J. Guanine nucleotide-binding protein Go-induced coupling of neuropeptide Y receptors to Ca2+ channels in sensory neurons. Proc Natl Acad Sci U S A. 1988 May;85(10):3633–3637. doi: 10.1073/pnas.85.10.3633. [DOI] [PMC free article] [PubMed] [Google Scholar]
- Fabiato A., Fabiato F. Calculator programs for computing the composition of the solutions containing multiple metals and ligands used for experiments in skinned muscle cells. J Physiol (Paris) 1979;75(5):463–505. [PubMed] [Google Scholar]
- Fabiato A. Rapid ionic modifications during the aequorin-detected calcium transient in a skinned canine cardiac Purkinje cell. J Gen Physiol. 1985 Feb;85(2):189–246. doi: 10.1085/jgp.85.2.189. [DOI] [PMC free article] [PubMed] [Google Scholar]
- Fabiato A. Time and calcium dependence of activation and inactivation of calcium-induced release of calcium from the sarcoplasmic reticulum of a skinned canine cardiac Purkinje cell. J Gen Physiol. 1985 Feb;85(2):247–289. doi: 10.1085/jgp.85.2.247. [DOI] [PMC free article] [PubMed] [Google Scholar]
- Fox A. P., Nowycky M. C., Tsien R. W. Kinetic and pharmacological properties distinguishing three types of calcium currents in chick sensory neurones. J Physiol. 1987 Dec;394:149–172. doi: 10.1113/jphysiol.1987.sp016864. [DOI] [PMC free article] [PubMed] [Google Scholar]
- Gorman A. L., Thomas M. V. Intracellular calcium accumulation during depolarization in a molluscan neurone. J Physiol. 1980 Nov;308:259–285. doi: 10.1113/jphysiol.1980.sp013471. [DOI] [PMC free article] [PubMed] [Google Scholar]
- Grundemar L., Widmark E., Waldeck B., Håkanson R. Neuropeptide Y: prejunctional inhibition of vagally induced contractions in the guinea pig trachea. Regul Pept. 1988 Dec;23(3):309–313. doi: 10.1016/0167-0115(88)90231-5. [DOI] [PubMed] [Google Scholar]
- Grynkiewicz G., Poenie M., Tsien R. Y. A new generation of Ca2+ indicators with greatly improved fluorescence properties. J Biol Chem. 1985 Mar 25;260(6):3440–3450. [PubMed] [Google Scholar]
- Gunter T. E., Wingrove D. E., Banerjee S., Gunter K. K. Mechanisms of mitochondrial calcium transport. Adv Exp Med Biol. 1988;232:1–14. doi: 10.1007/978-1-4757-0007-7_1. [DOI] [PubMed] [Google Scholar]
- Hamill O. P., Marty A., Neher E., Sakmann B., Sigworth F. J. Improved patch-clamp techniques for high-resolution current recording from cells and cell-free membrane patches. Pflugers Arch. 1981 Aug;391(2):85–100. doi: 10.1007/BF00656997. [DOI] [PubMed] [Google Scholar]
- Jia M., Nelson P. G. Calcium currents and transmitter output in cultured spinal cord and dorsal root ganglion neurons. J Neurophysiol. 1986 Nov;56(5):1257–1267. doi: 10.1152/jn.1986.56.5.1257. [DOI] [PubMed] [Google Scholar]
- Kostyuk P. G., Shuba YaM, Savchenko A. N. Three types of calcium channels in the membrane of mouse sensory neurons. Pflugers Arch. 1988 Jun;411(6):661–669. doi: 10.1007/BF00580863. [DOI] [PubMed] [Google Scholar]
- Kuba K. Release of calcium ions linked to the activation of potassium conductance in a caffeine-treated sympathetic neurone. J Physiol. 1980 Jan;298:251–269. doi: 10.1113/jphysiol.1980.sp013079. [DOI] [PMC free article] [PubMed] [Google Scholar]
- Lev-Ram V., Grinvald A. Activity-dependent calcium transients in central nervous system myelinated axons revealed by the calcium indicator Fura-2. Biophys J. 1987 Oct;52(4):571–576. doi: 10.1016/S0006-3495(87)83246-0. [DOI] [PMC free article] [PubMed] [Google Scholar]
- Levy S., Tillotson D. Effects of Na+ and Ca2+ gradients on intracellular free Ca2+ in voltage-clamped Aplysia neurons. Brain Res. 1988 Dec 6;474(2):333–342. doi: 10.1016/0006-8993(88)90447-7. [DOI] [PubMed] [Google Scholar]
- Lipscombe D., Madison D. V., Poenie M., Reuter H., Tsien R. W., Tsien R. Y. Imaging of cytosolic Ca2+ transients arising from Ca2+ stores and Ca2+ channels in sympathetic neurons. Neuron. 1988 Jul;1(5):355–365. doi: 10.1016/0896-6273(88)90185-7. [DOI] [PubMed] [Google Scholar]
- Malgaroli A., Milani D., Meldolesi J., Pozzan T. Fura-2 measurement of cytosolic free Ca2+ in monolayers and suspensions of various types of animal cells. J Cell Biol. 1987 Nov;105(5):2145–2155. doi: 10.1083/jcb.105.5.2145. [DOI] [PMC free article] [PubMed] [Google Scholar]
- Mayer M. L., MacDermott A. B., Westbrook G. L., Smith S. J., Barker J. L. Agonist- and voltage-gated calcium entry in cultured mouse spinal cord neurons under voltage clamp measured using arsenazo III. J Neurosci. 1987 Oct;7(10):3230–3244. doi: 10.1523/JNEUROSCI.07-10-03230.1987. [DOI] [PMC free article] [PubMed] [Google Scholar]
- Mayer M. L., Westbrook G. L. The physiology of excitatory amino acids in the vertebrate central nervous system. Prog Neurobiol. 1987;28(3):197–276. doi: 10.1016/0301-0082(87)90011-6. [DOI] [PubMed] [Google Scholar]
- McBurney R. N., Neering I. R. The measurement of changes in intracellular free calcium during action potentials in mammalian neurones. J Neurosci Methods. 1985 Mar;13(1):65–76. doi: 10.1016/0165-0270(85)90044-5. [DOI] [PubMed] [Google Scholar]
- McCormack J. G., Denton R. M. The regulation of mitochondrial function in mammalian cells by Ca2+ ions. Biochem Soc Trans. 1988 Aug;16(4):523–527. doi: 10.1042/bst0160523. [DOI] [PubMed] [Google Scholar]
- Moore C. L. Specific inhibition of mitochondrial Ca++ transport by ruthenium red. Biochem Biophys Res Commun. 1971 Jan 22;42(2):298–305. doi: 10.1016/0006-291x(71)90102-1. [DOI] [PubMed] [Google Scholar]
- Murphy S. N., Thayer S. A., Miller R. J. The effects of excitatory amino acids on intracellular calcium in single mouse striatal neurons in vitro. J Neurosci. 1987 Dec;7(12):4145–4158. doi: 10.1523/JNEUROSCI.07-12-04145.1987. [DOI] [PMC free article] [PubMed] [Google Scholar]
- Nicholls D. G. A role for the mitochondrion in the protection of cells against calcium overload? Prog Brain Res. 1985;63:97–106. doi: 10.1016/S0079-6123(08)61978-0. [DOI] [PubMed] [Google Scholar]
- Nicholls D. G. The regulation of extramitochondrial free calcium ion concentration by rat liver mitochondria. Biochem J. 1978 Nov 15;176(2):463–474. doi: 10.1042/bj1760463. [DOI] [PMC free article] [PubMed] [Google Scholar]
- Nohmi M., Kuba K. Effects of Na+ gradient on the intracellular Ca2+ oscillation in the sympathetic ganglion cell: Na-Ca exchange in the neurone cell soma? Brain Res. 1984 Dec 17;324(1):171–174. doi: 10.1016/0006-8993(84)90638-3. [DOI] [PubMed] [Google Scholar]
- Nowycky M. C., Fox A. P., Tsien R. W. Three types of neuronal calcium channel with different calcium agonist sensitivity. Nature. 1985 Aug 1;316(6027):440–443. doi: 10.1038/316440a0. [DOI] [PubMed] [Google Scholar]
- Pusch M., Neher E. Rates of diffusional exchange between small cells and a measuring patch pipette. Pflugers Arch. 1988 Feb;411(2):204–211. doi: 10.1007/BF00582316. [DOI] [PubMed] [Google Scholar]
- Ross W. N., Arechiga H., Nicholls J. G. Optical recording of calcium and voltage transients following impulses in cell bodies and processes of identified leech neurons in culture. J Neurosci. 1987 Dec;7(12):3877–3887. doi: 10.1523/JNEUROSCI.07-12-03877.1987. [DOI] [PMC free article] [PubMed] [Google Scholar]
- Ross W. N., Graubard K. Spatially and temporally resolved calcium concentration changes in oscillating neurons of crab stomatogastric ganglion. Proc Natl Acad Sci U S A. 1989 Mar;86(5):1679–1683. doi: 10.1073/pnas.86.5.1679. [DOI] [PMC free article] [PubMed] [Google Scholar]
- Sanchez-Armass S., Blaustein M. P. Role of sodium-calcium exchange in regulation of intracellular calcium in nerve terminals. Am J Physiol. 1987 Jun;252(6 Pt 1):C595–C603. doi: 10.1152/ajpcell.1987.252.6.C595. [DOI] [PubMed] [Google Scholar]
- Streb H., Irvine R. F., Berridge M. J., Schulz I. Release of Ca2+ from a nonmitochondrial intracellular store in pancreatic acinar cells by inositol-1,4,5-trisphosphate. Nature. 1983 Nov 3;306(5938):67–69. doi: 10.1038/306067a0. [DOI] [PubMed] [Google Scholar]
- Tank D. W., Sugimori M., Connor J. A., Llinás R. R. Spatially resolved calcium dynamics of mammalian Purkinje cells in cerebellar slice. Science. 1988 Nov 4;242(4879):773–777. doi: 10.1126/science.2847315. [DOI] [PubMed] [Google Scholar]
- Thayer S. A., Hirning L. D., Miller R. J. Distribution of multiple types of Ca2+ channels in rat sympathetic neurons in vitro. Mol Pharmacol. 1987 Nov;32(5):579–586. [PubMed] [Google Scholar]
- Thayer S. A., Hirning L. D., Miller R. J. The role of caffeine-sensitive calcium stores in the regulation of the intracellular free calcium concentration in rat sympathetic neurons in vitro. Mol Pharmacol. 1988 Nov;34(5):664–673. [PubMed] [Google Scholar]
- Thayer S. A., Murphy S. N., Miller R. J. Widespread distribution of dihydropyridine-sensitive calcium channels in the central nervous system. Mol Pharmacol. 1986 Dec;30(6):505–509. [PubMed] [Google Scholar]
- Thayer S. A., Perney T. M., Miller R. J. Regulation of calcium homeostasis in sensory neurons by bradykinin. J Neurosci. 1988 Nov;8(11):4089–4097. doi: 10.1523/JNEUROSCI.08-11-04089.1988. [DOI] [PMC free article] [PubMed] [Google Scholar]
- Thayer S. A., Sturek M., Miller R. J. Measurement of neuronal Ca2+ transients using simultaneous microfluorimetry and electrophysiology. Pflugers Arch. 1988 Jul;412(1-2):216–223. doi: 10.1007/BF00583753. [DOI] [PubMed] [Google Scholar]
- Tsien R. W. Calcium channels in excitable cell membranes. Annu Rev Physiol. 1983;45:341–358. doi: 10.1146/annurev.ph.45.030183.002013. [DOI] [PubMed] [Google Scholar]
- Zucker R. S. Tetraethylammonium contains an impurity which alkalizes cytoplasm and reduce calcium buffering in neurons. Brain Res. 1981 Mar 16;208(2):473–478. doi: 10.1016/0006-8993(81)90580-1. [DOI] [PubMed] [Google Scholar]