Abstract
1. The effects of dihydropyridine (DHP) derivatives on current through the slow Ca2+ channel and on isometric force were investigated in short toe muscle fibres of the frog (Rana temporaria). The experiments were performed under voltage-clamp conditions with two flexible internal microelectrodes. 2. The non-chiral DHP derivative nifedipine was used mainly because it allowed control measurements after the inactivation of the drug with UV light. 3. In a TEA sulphate solution containing 4 mM-free Ca2+, nifedipine (1 microM) caused no relevant alterations in the time course of successive contractures induced by depolarizing steps to 0 mV of 3.5 min duration followed by a restoration time at -90 mV of 1.5 min. 4. When external Ca2+ was replaced by Mg2+, nifedipine caused a dose-dependent shortening of contractures. The effect reached saturation at about 50% of shortening with 1-5 microM-nifedipine. In the absence of divalent cations and with Na+ being the only metallic cation in the solution, shortening became more pronounced and maximum force decreased. 5. The application of 2 microM-nifedipine to a Ca2(+)-free, Mg2(+)-containing solution shifted the voltage dependence of force inactivation by 5-10 mV to more negative potentials. 6. Force activation was facilitated by nifedipine. In the presence of 2 microM-nifedipine in a Ca2(+)-containing solution, threshold potentials (rheobase) as negative as -75 mV were measured under microscopical observation. UV irradiation shifted the threshold potential back to the normal value of about -50 mV. 7. The slow Ca2+ inward current was blocked almost completely by approximately 5 microM-nifedipine, even when induced from negative holding potentials (-90 to -120 mV), i.e. under conditions where normal phasic contractures could still be observed. 8. Nifedipine (0.8 microM) caused a shift of the voltage dependence of current inactivation (V0.5) by 4 mV from -26 to -30 mV and at negative holding potentials (-90 mV), a reduction of maximum current by 35%. 9. The voltage dependence of current activation was not significantly altered by nifedipine (2 microM). 10. It is assumed that nifedipine binds with low affinity to the resting state of the DHP receptor (KD 0.8 microM) and with high affinity (KD 1 nM) to the inactivated and the active state (or to a precursor of this state). These assumptions could explain the relatively small shift of the inactivation curves (points 5 and 8) to more negative potentials and the facilitation of force activation (point 6).
Full text
PDF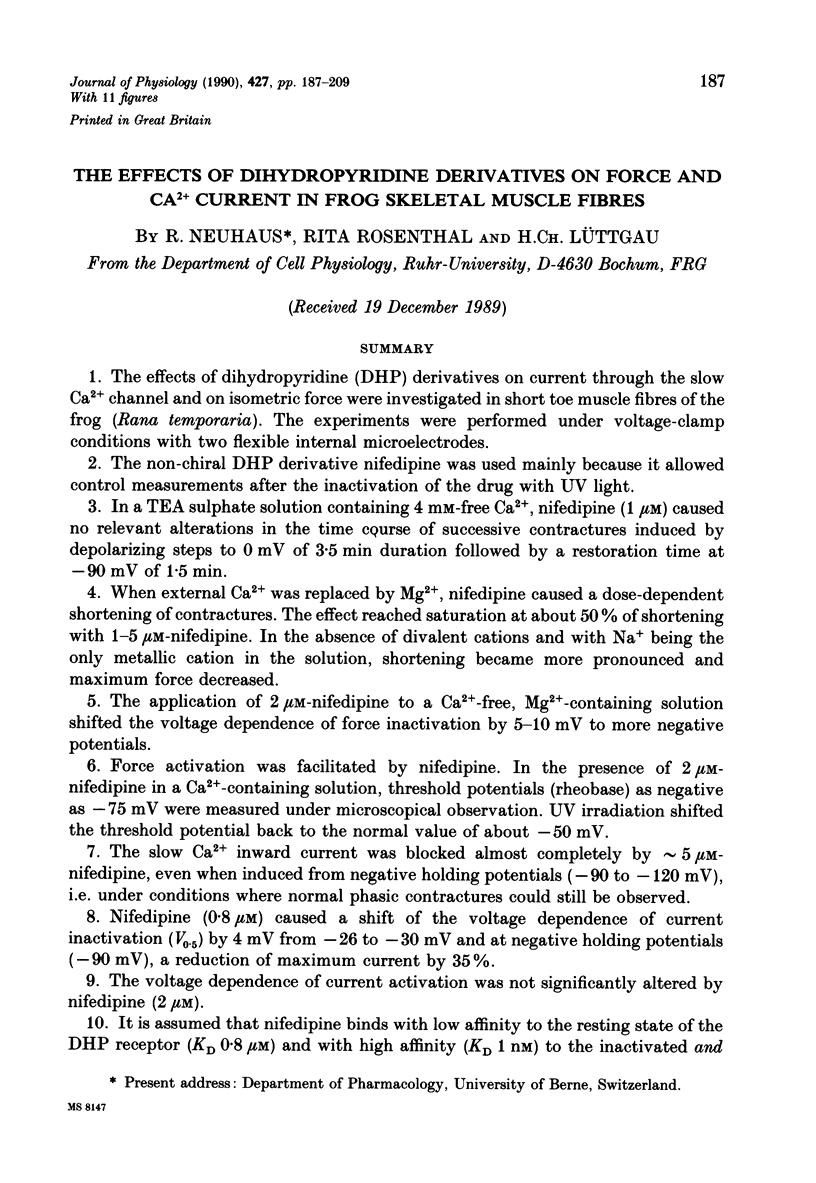
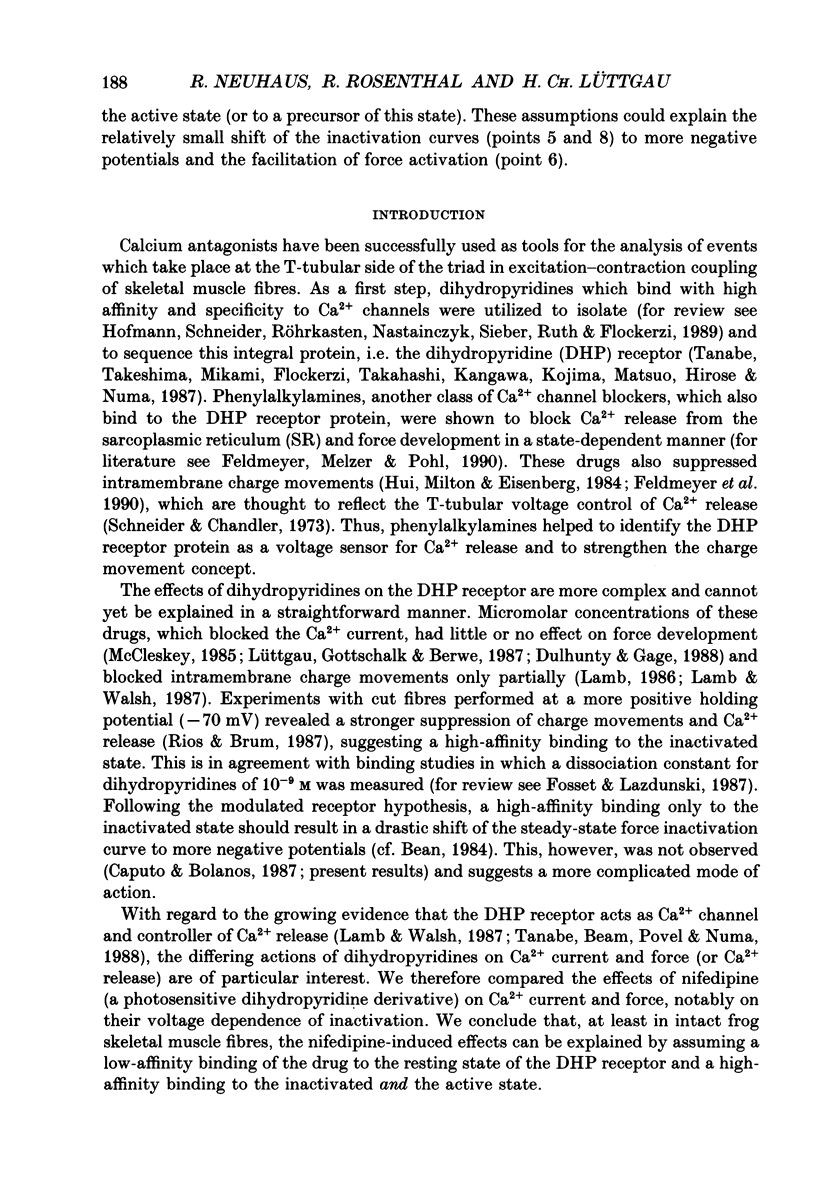
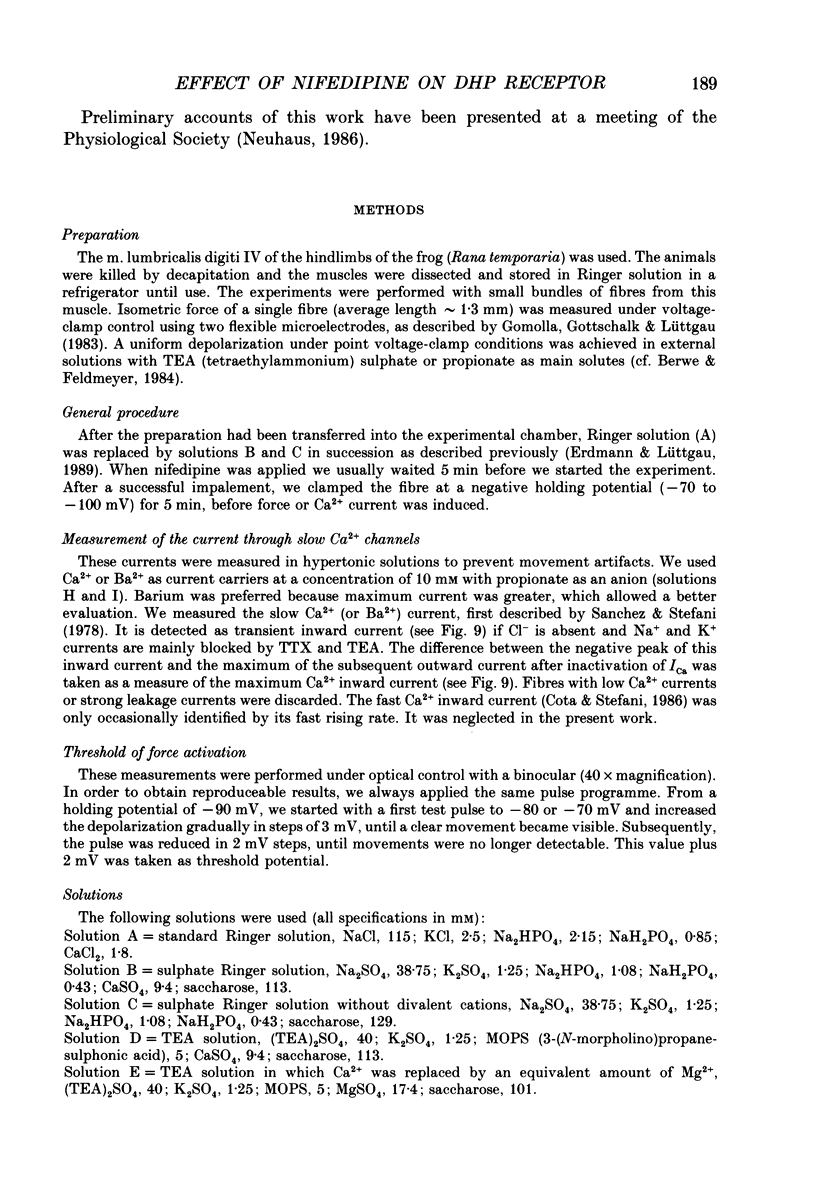
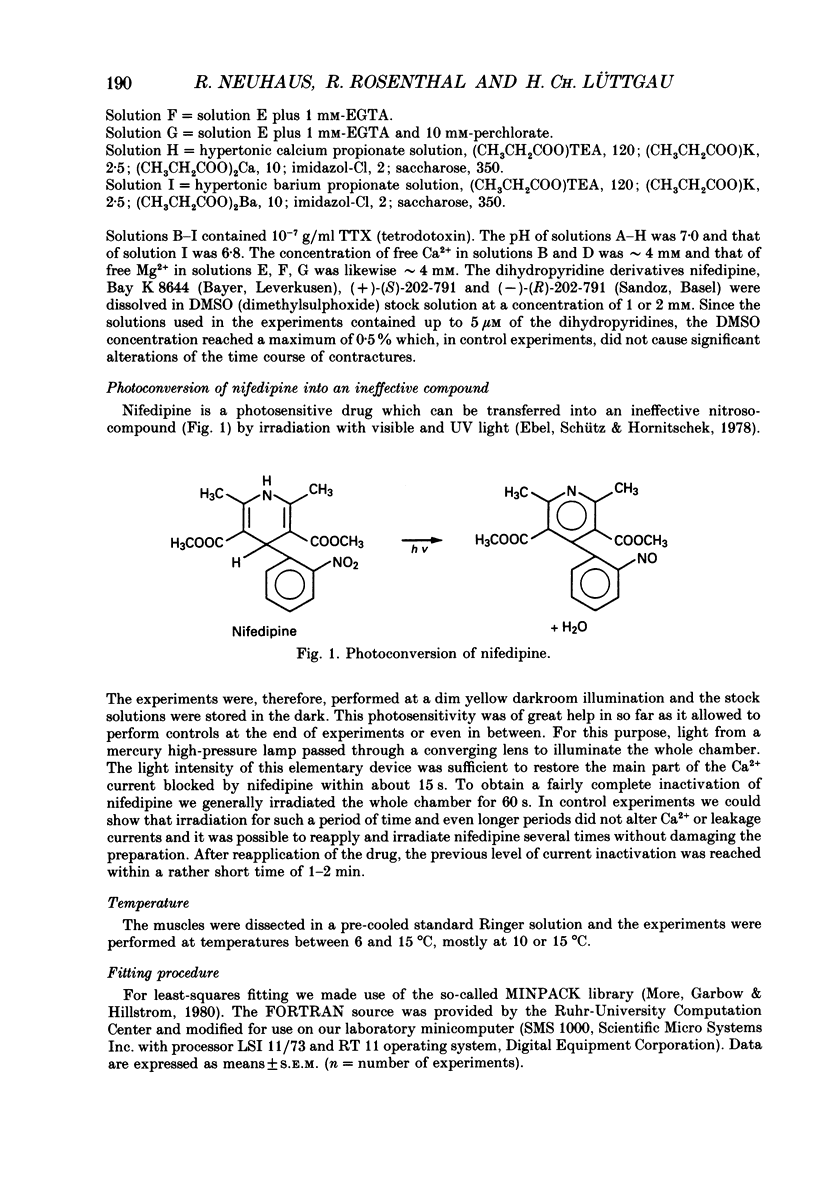
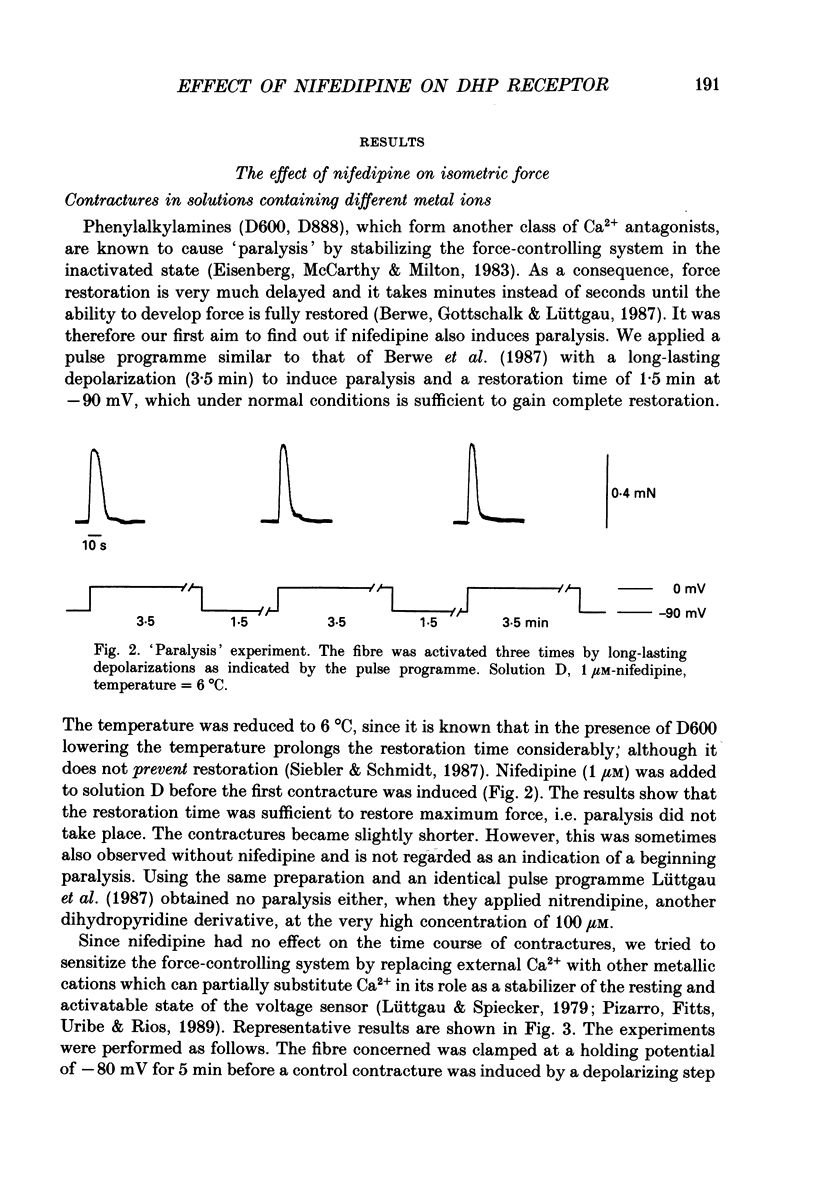
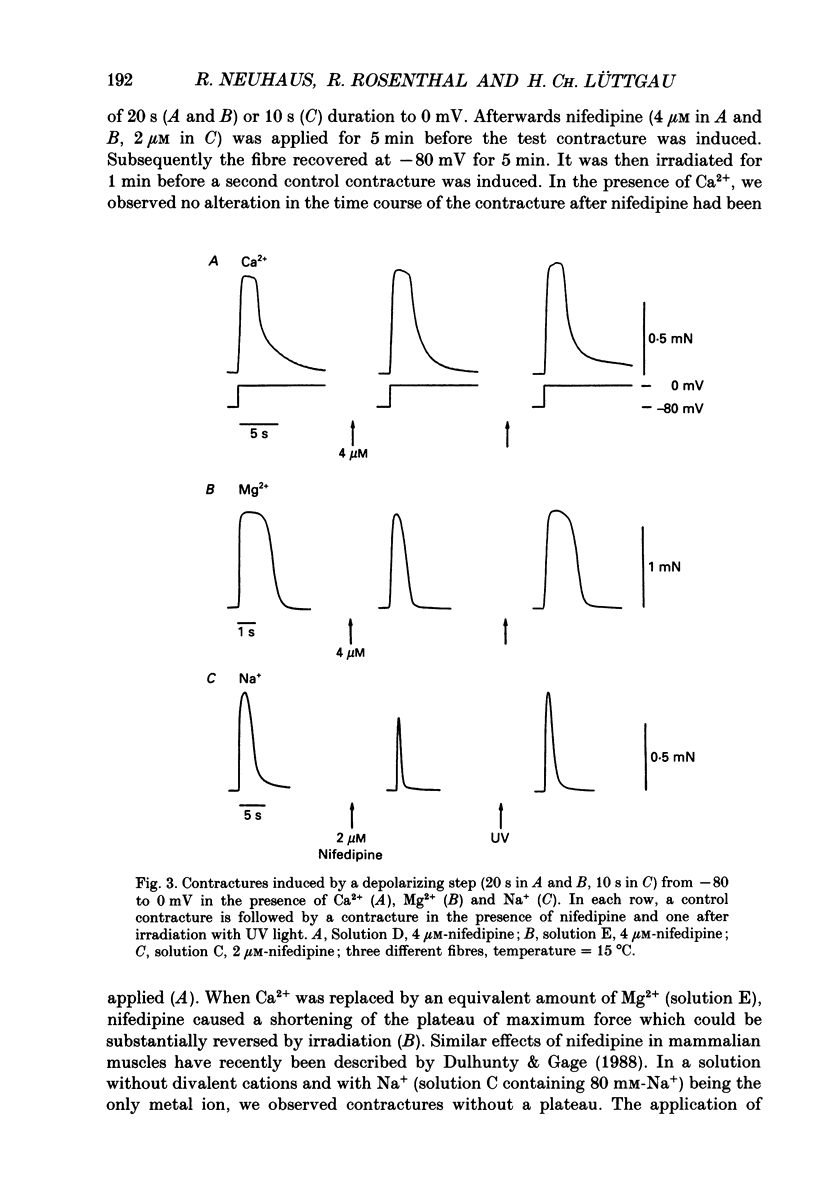
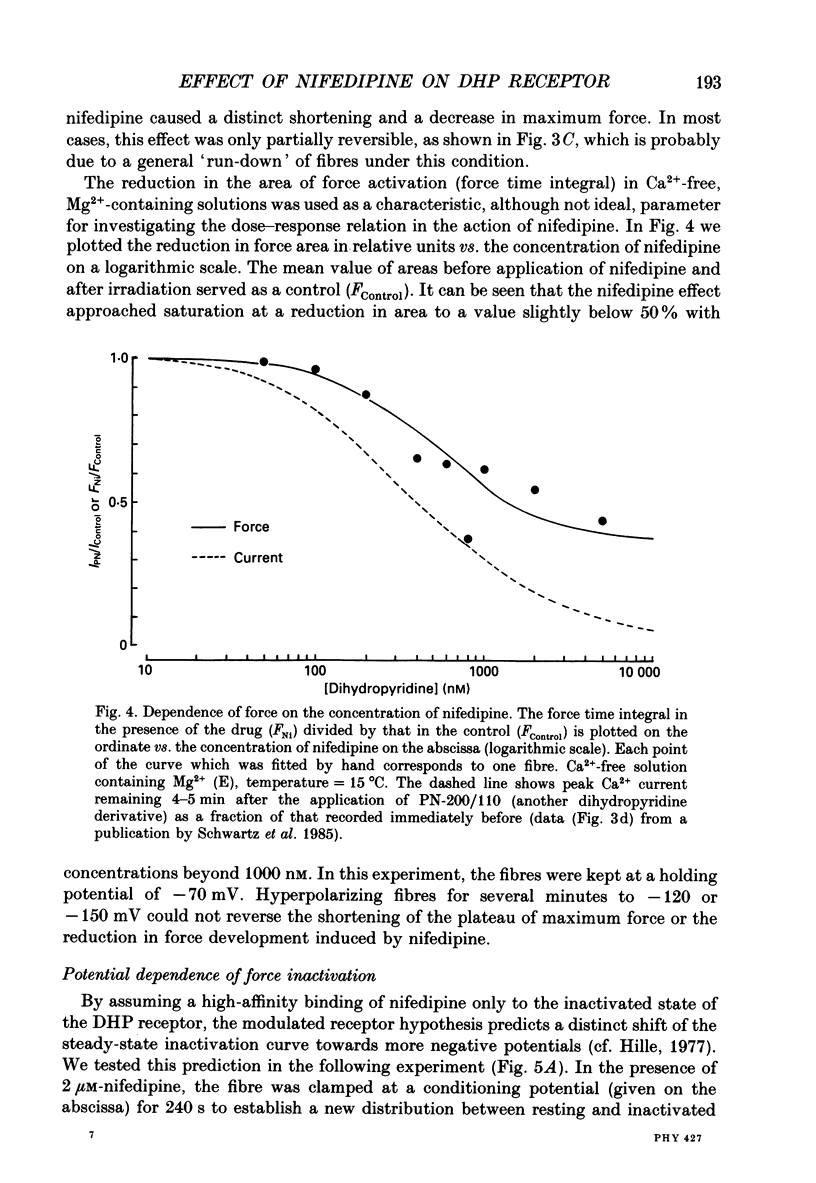
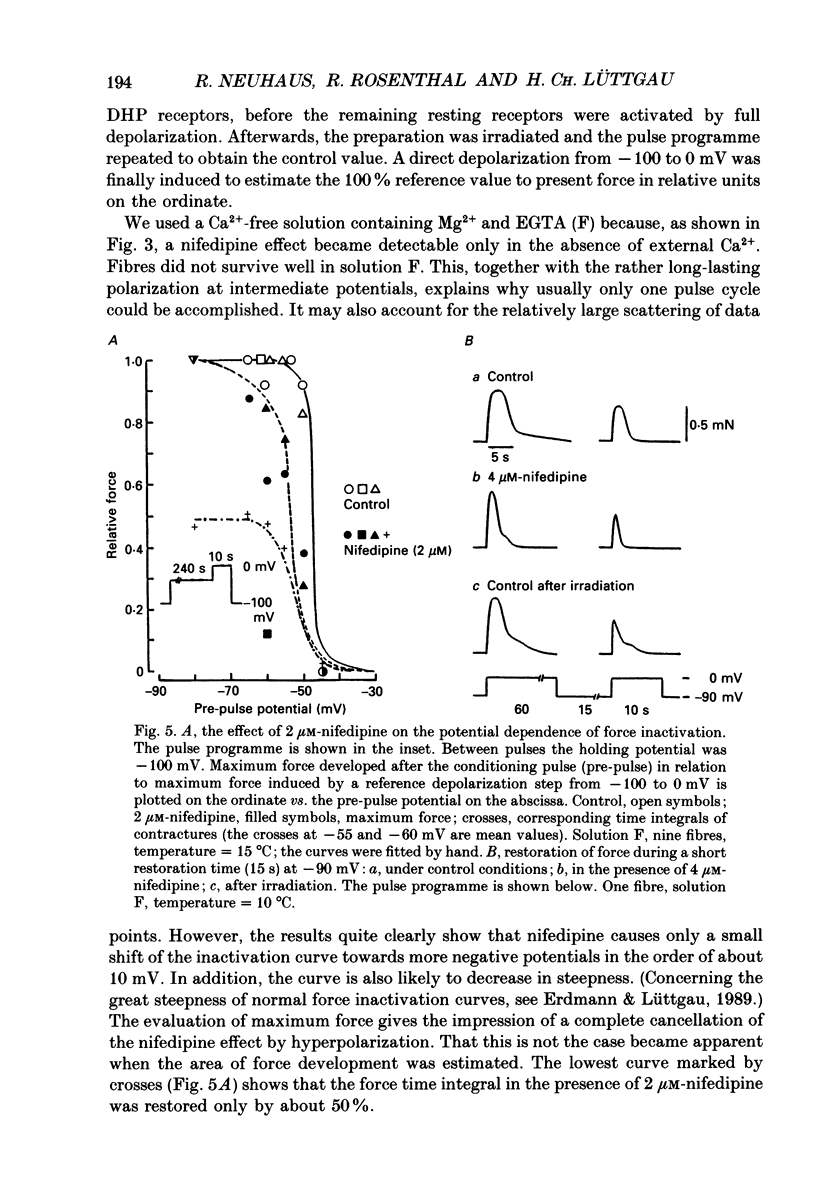
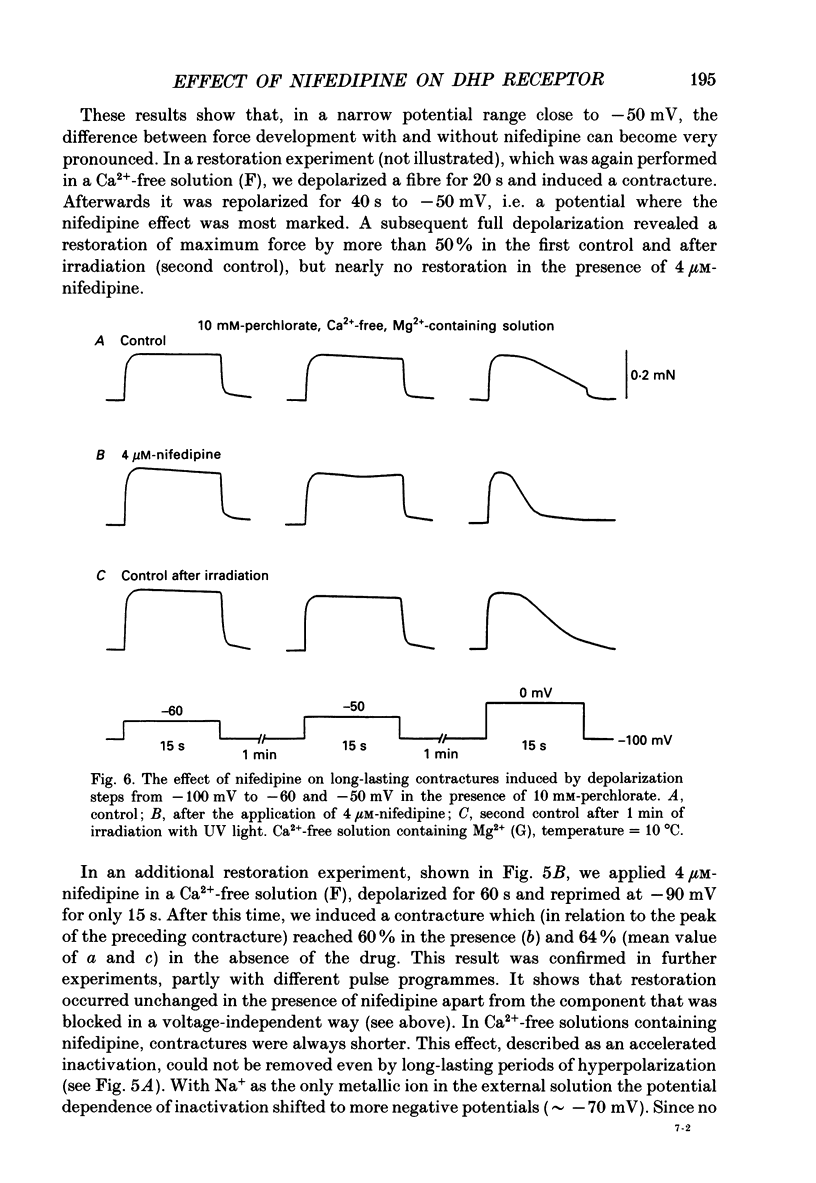
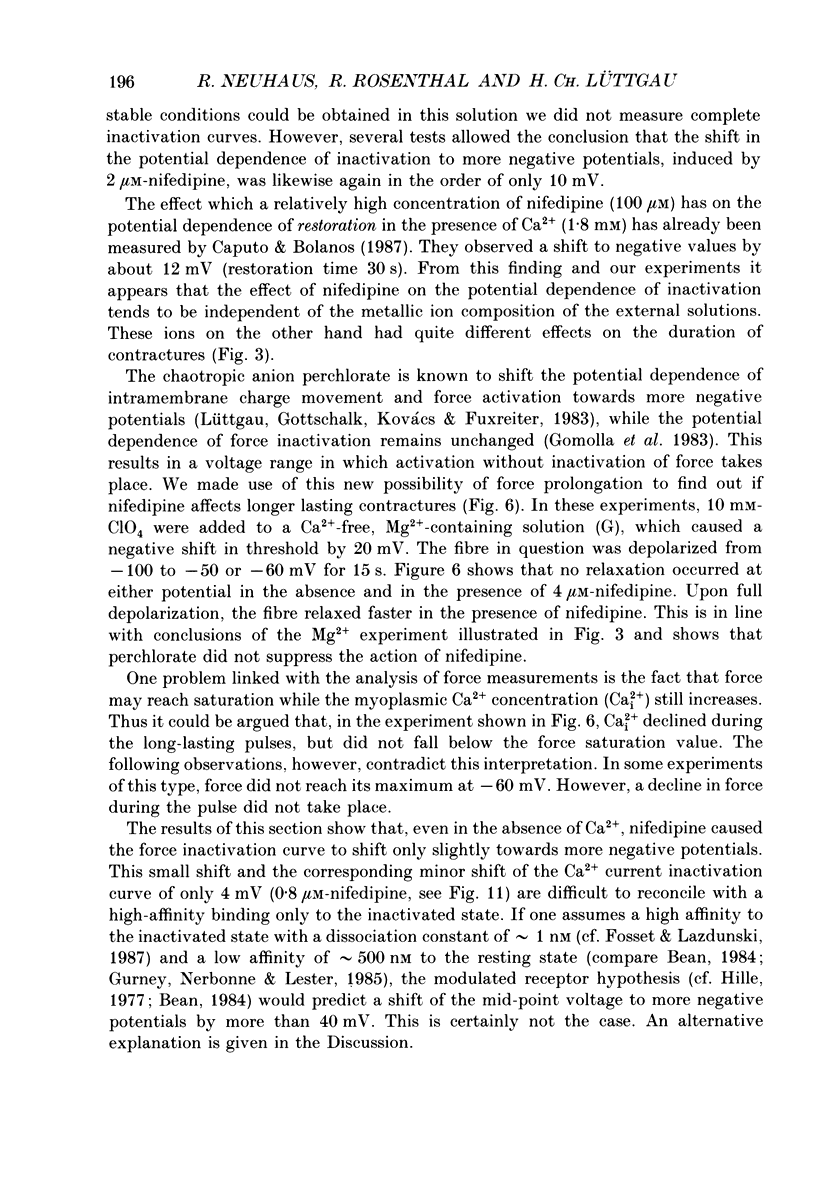
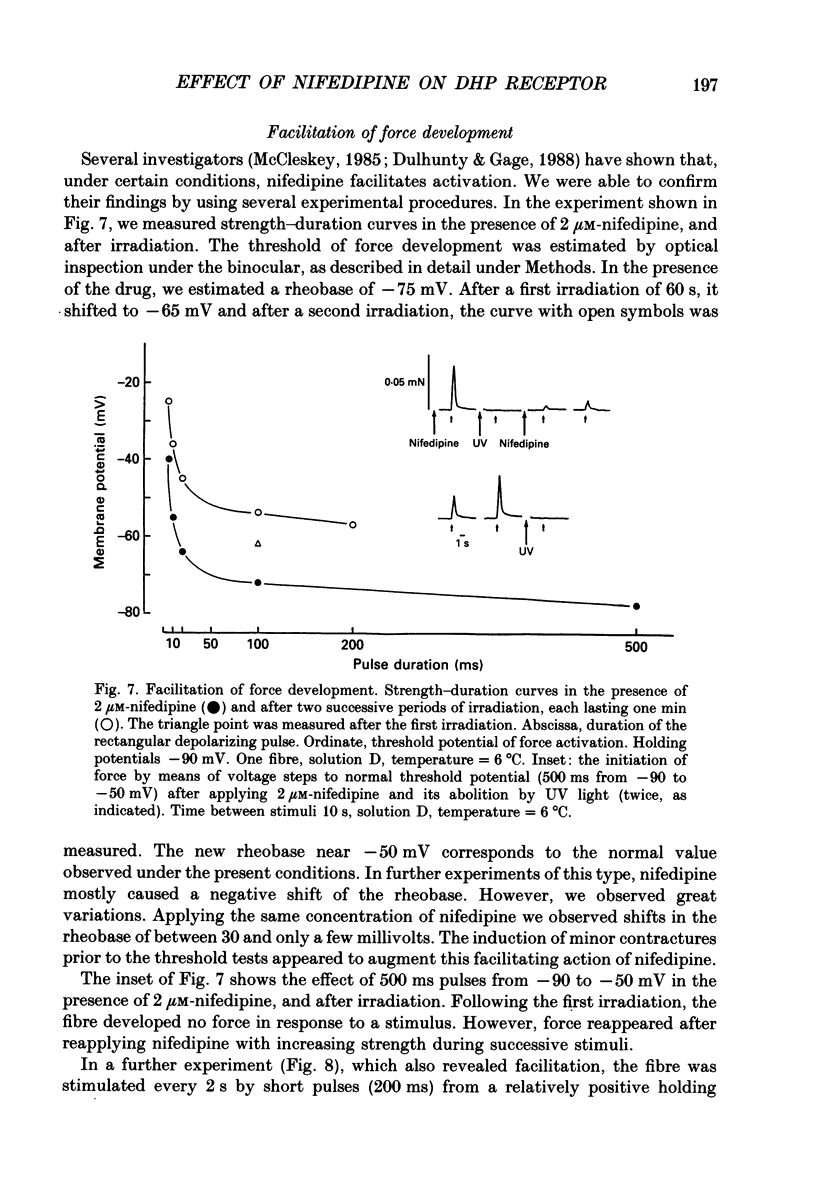
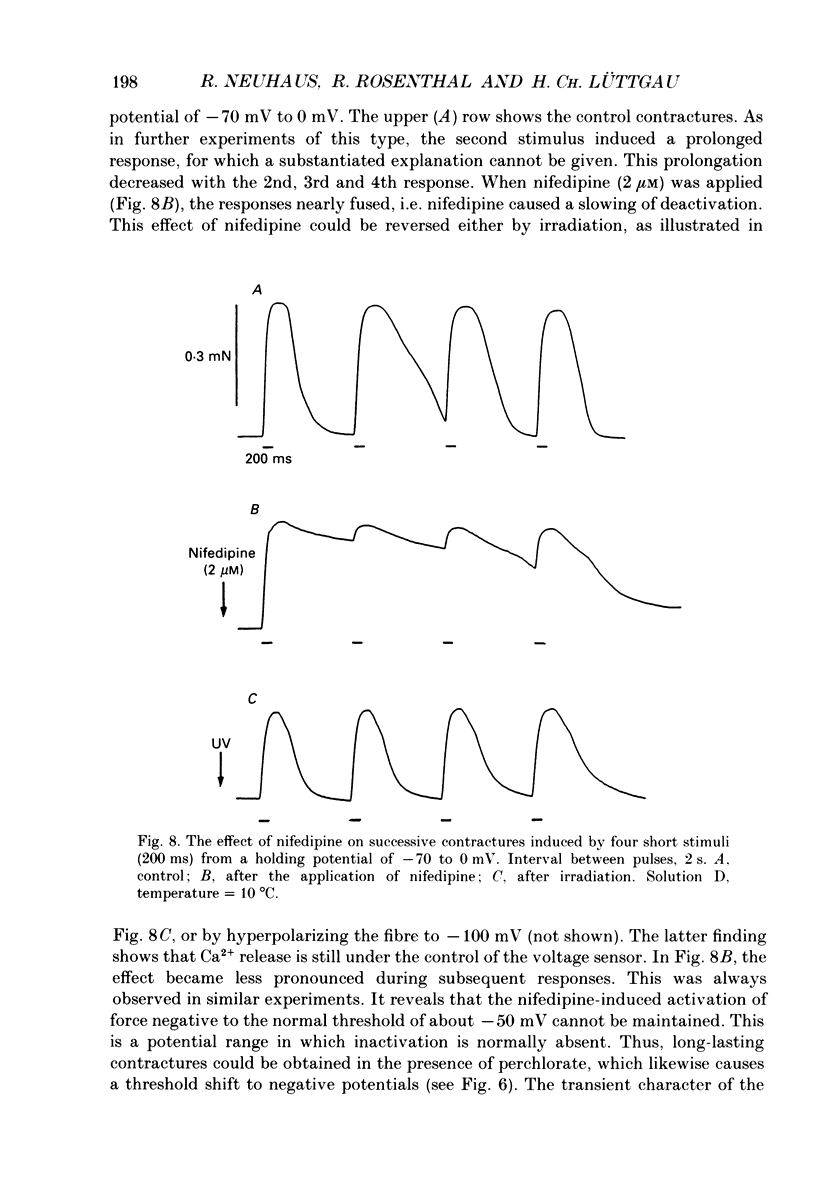
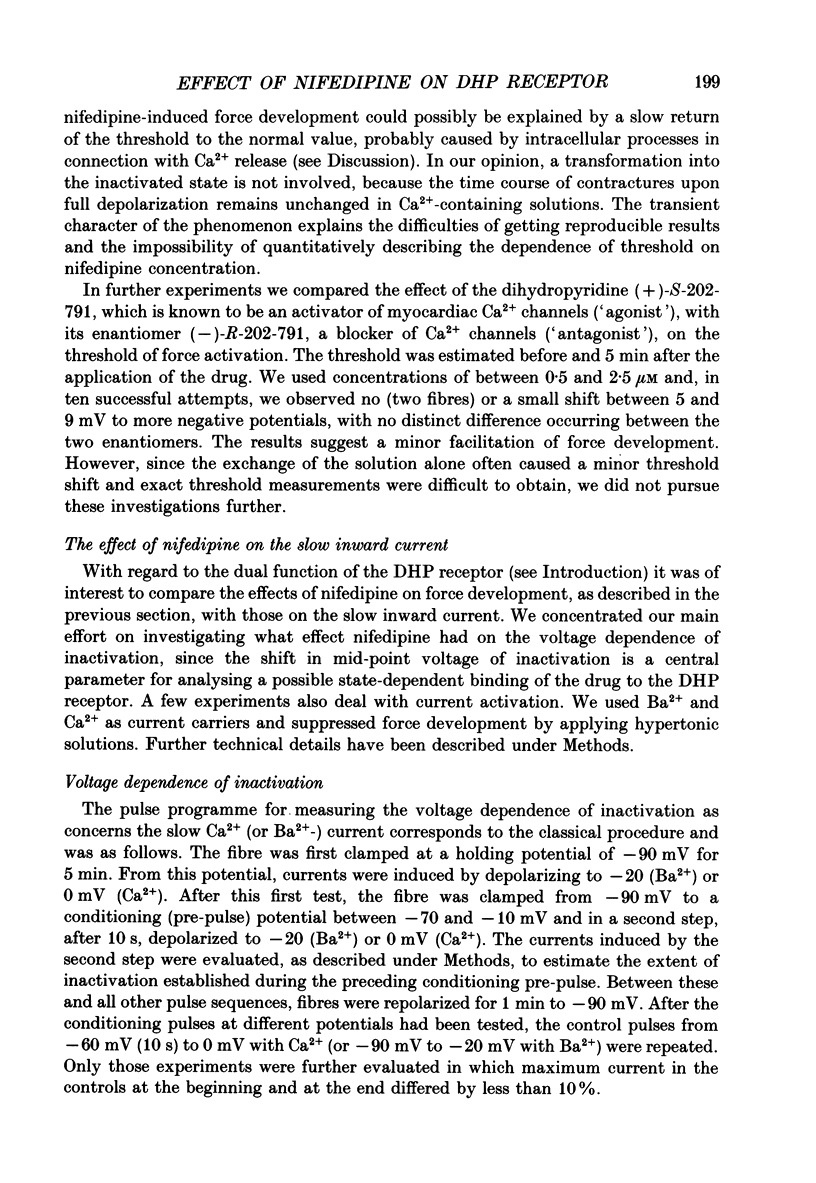
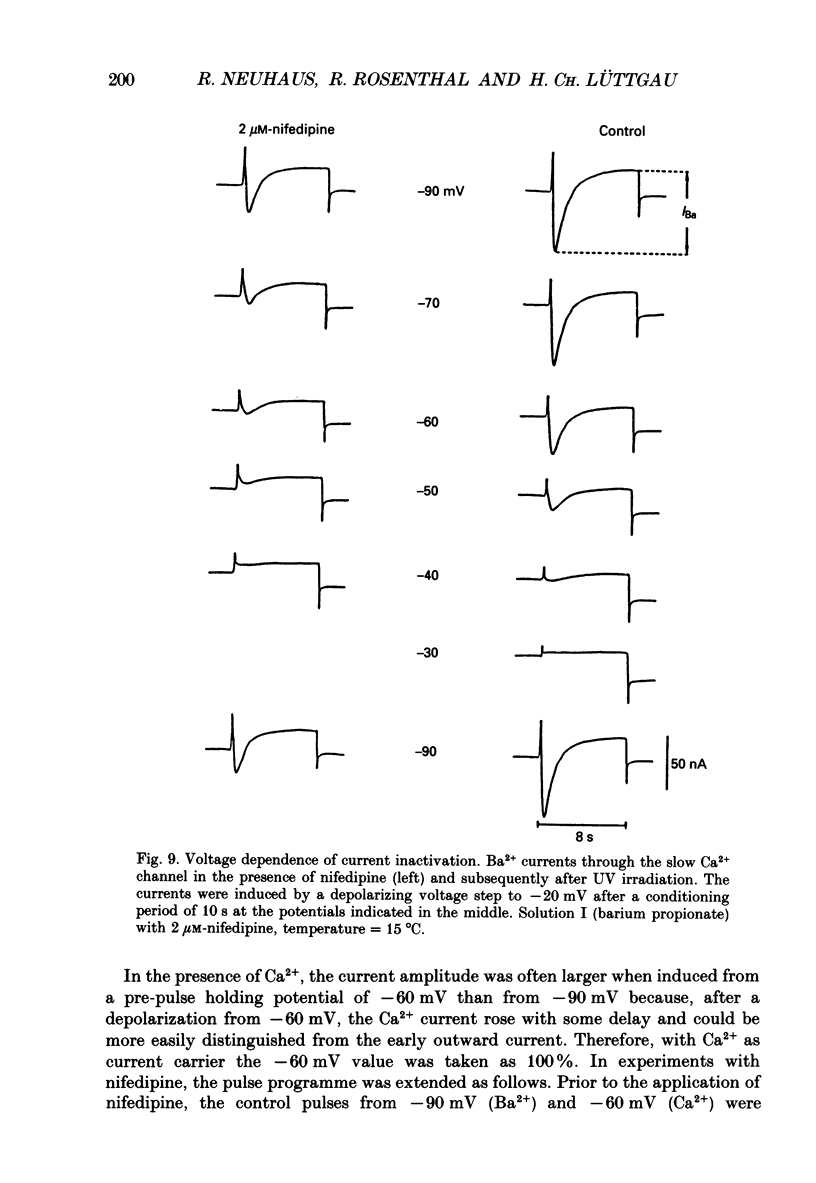
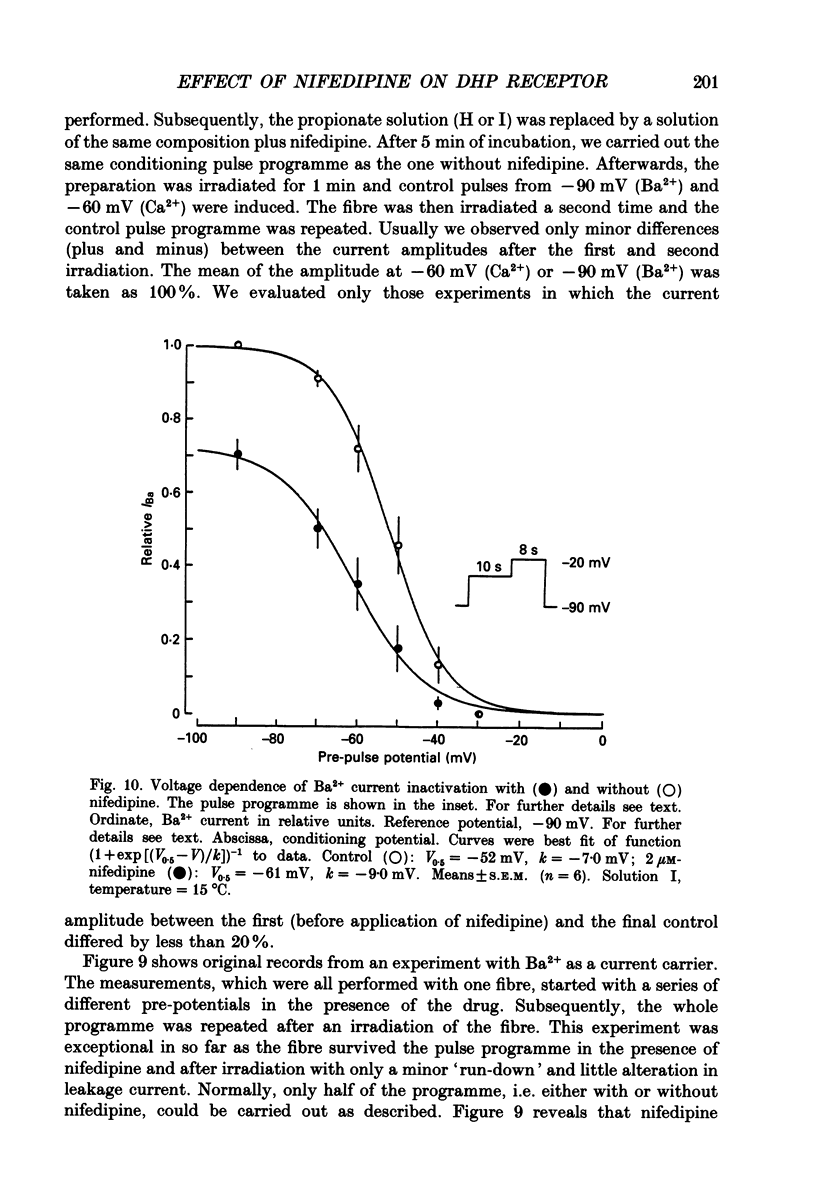
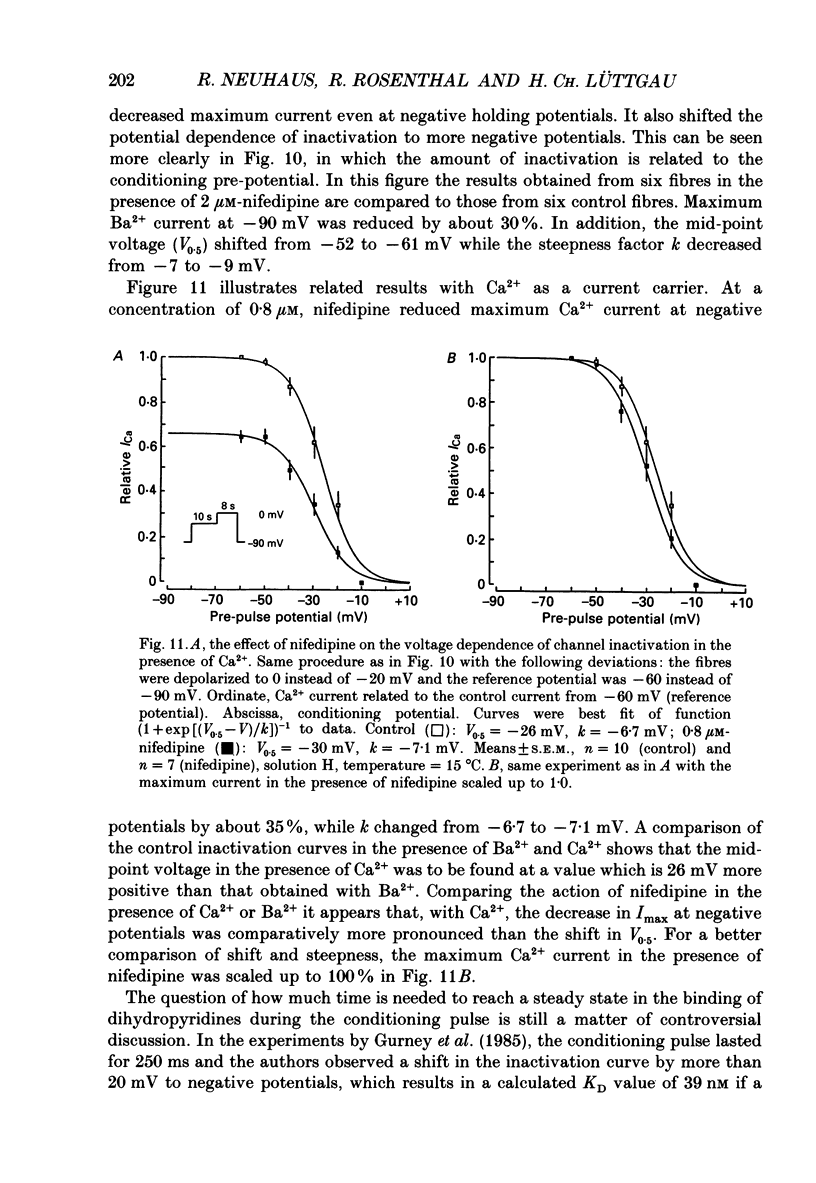
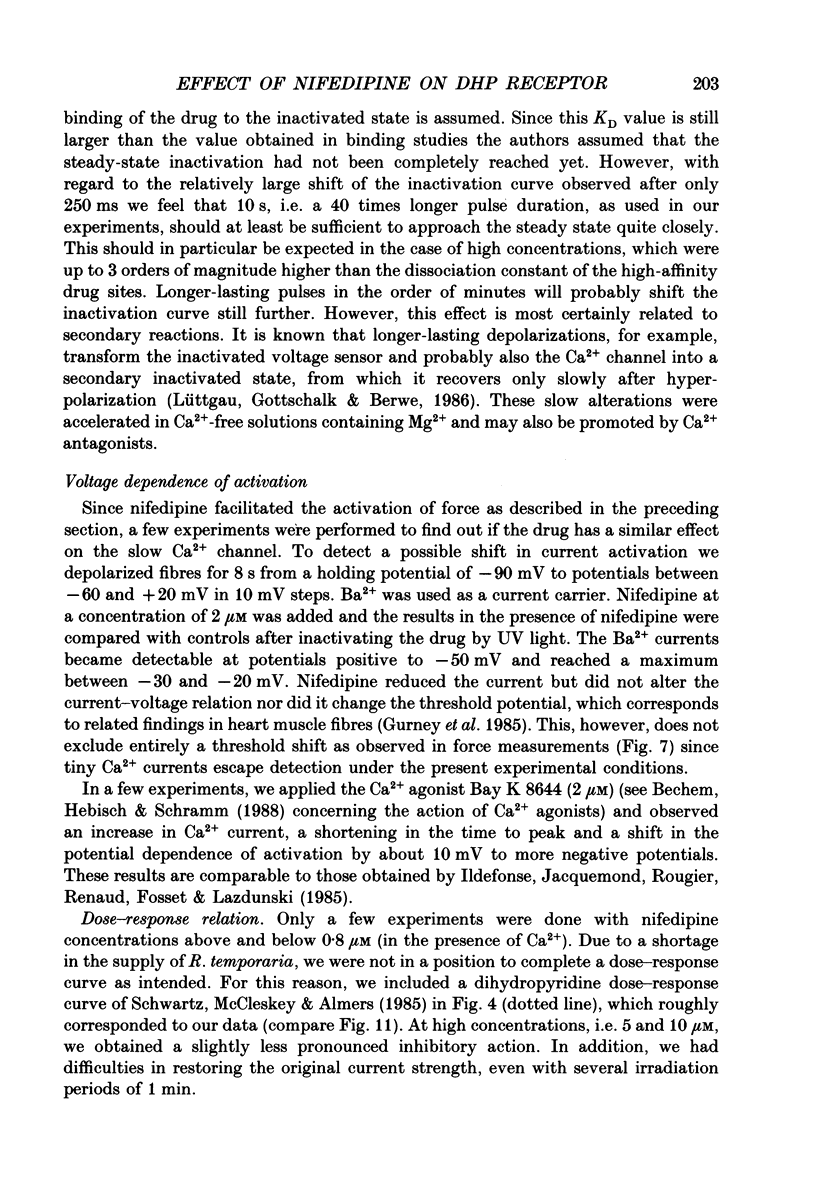
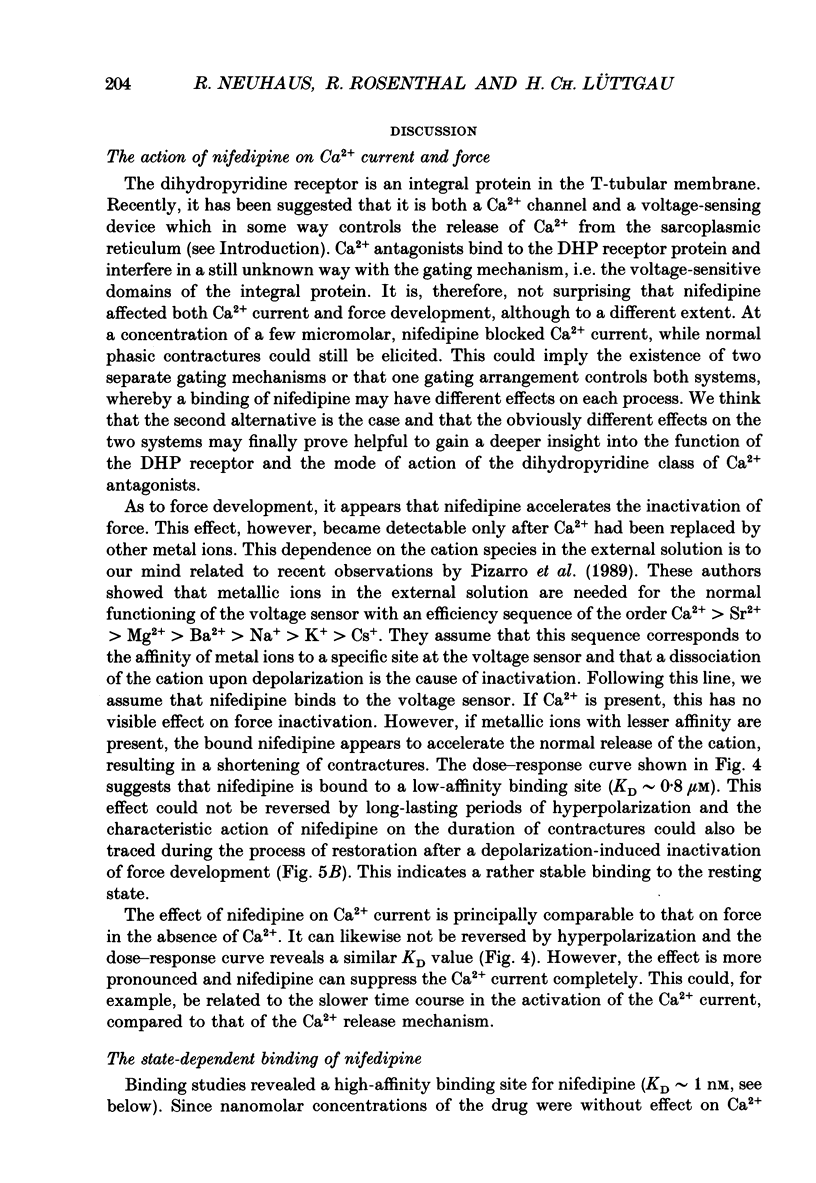
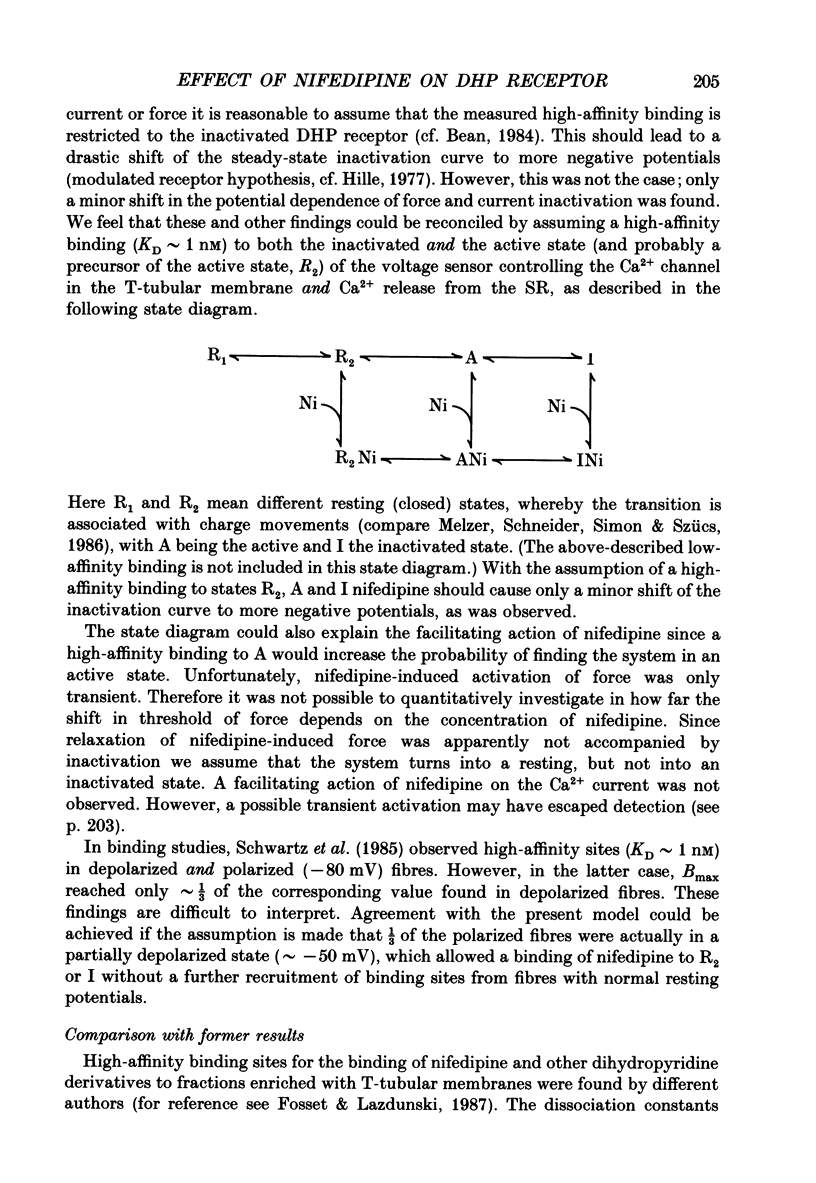
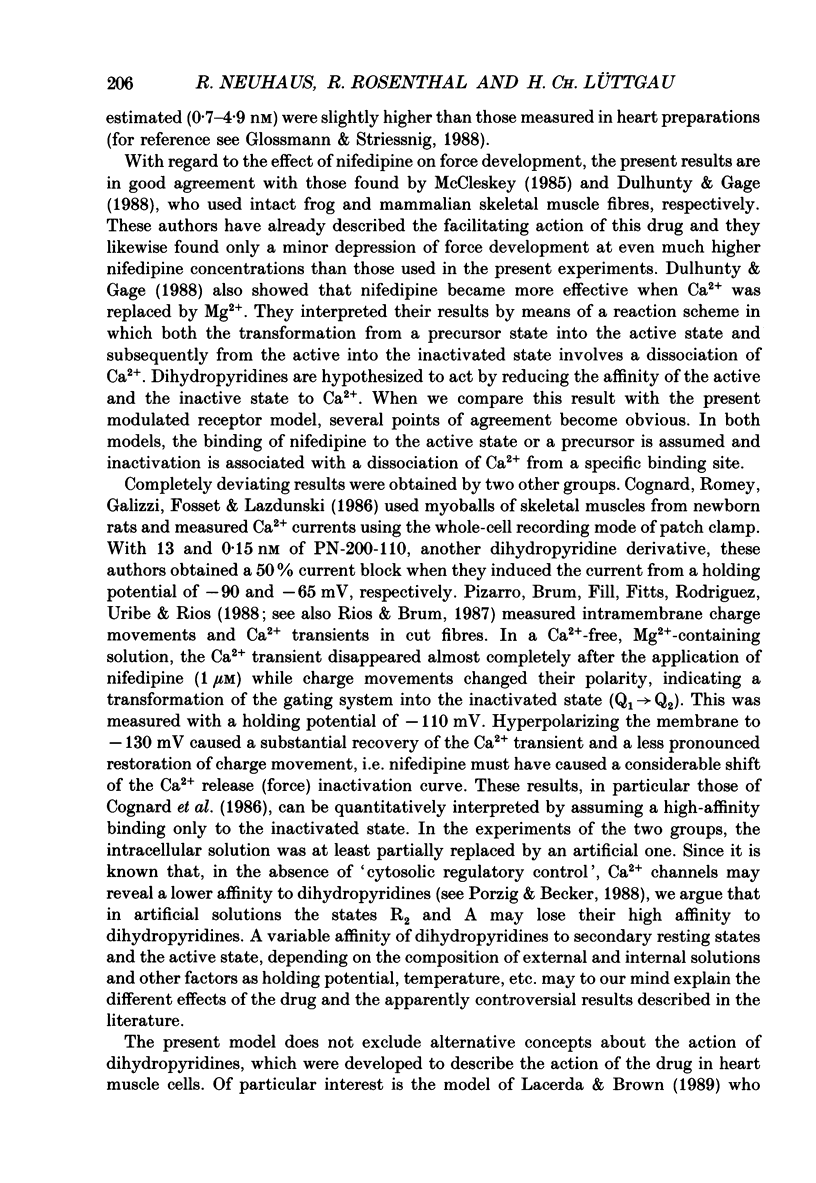
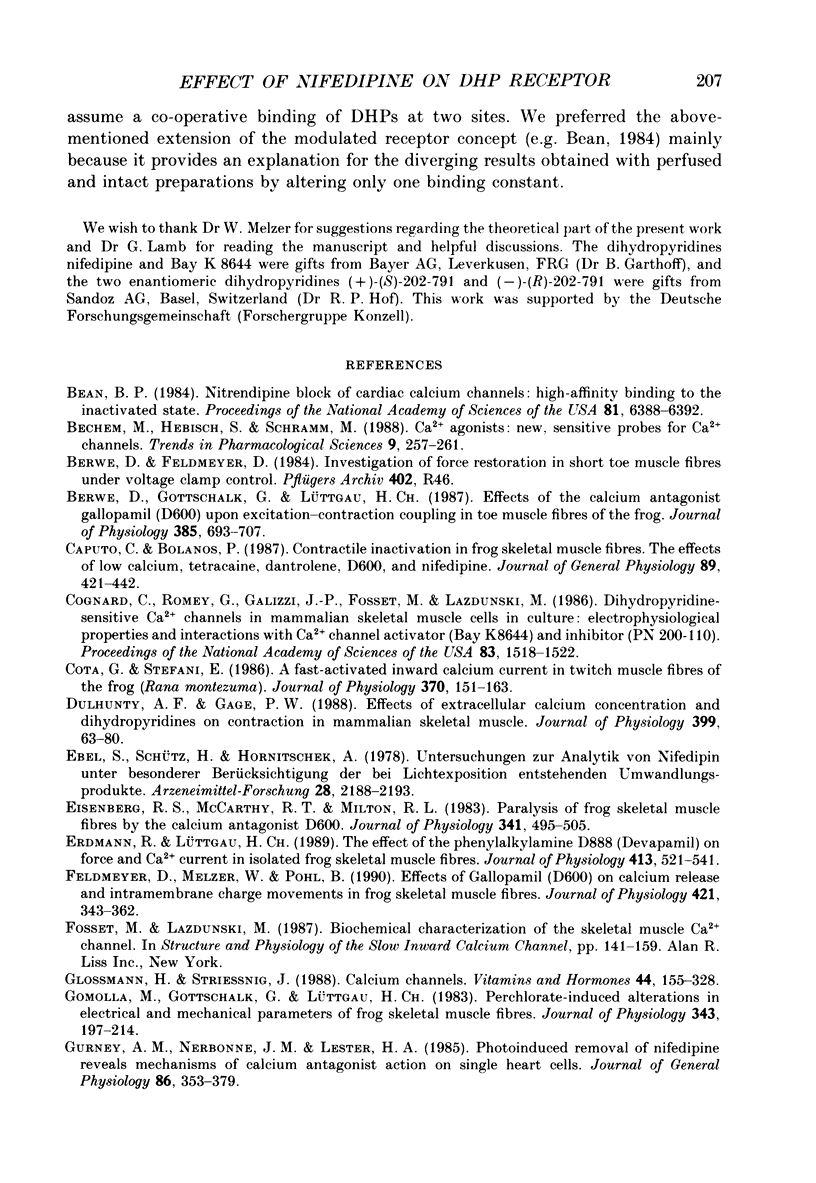
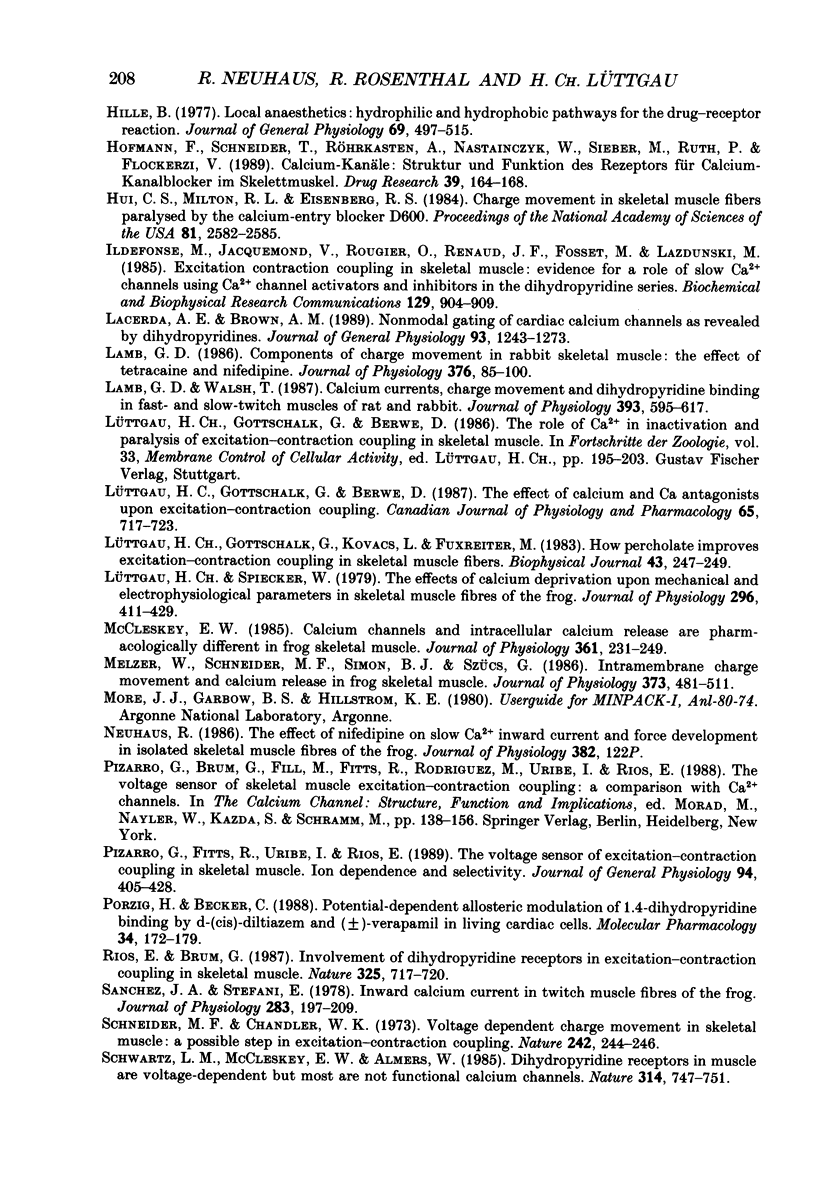
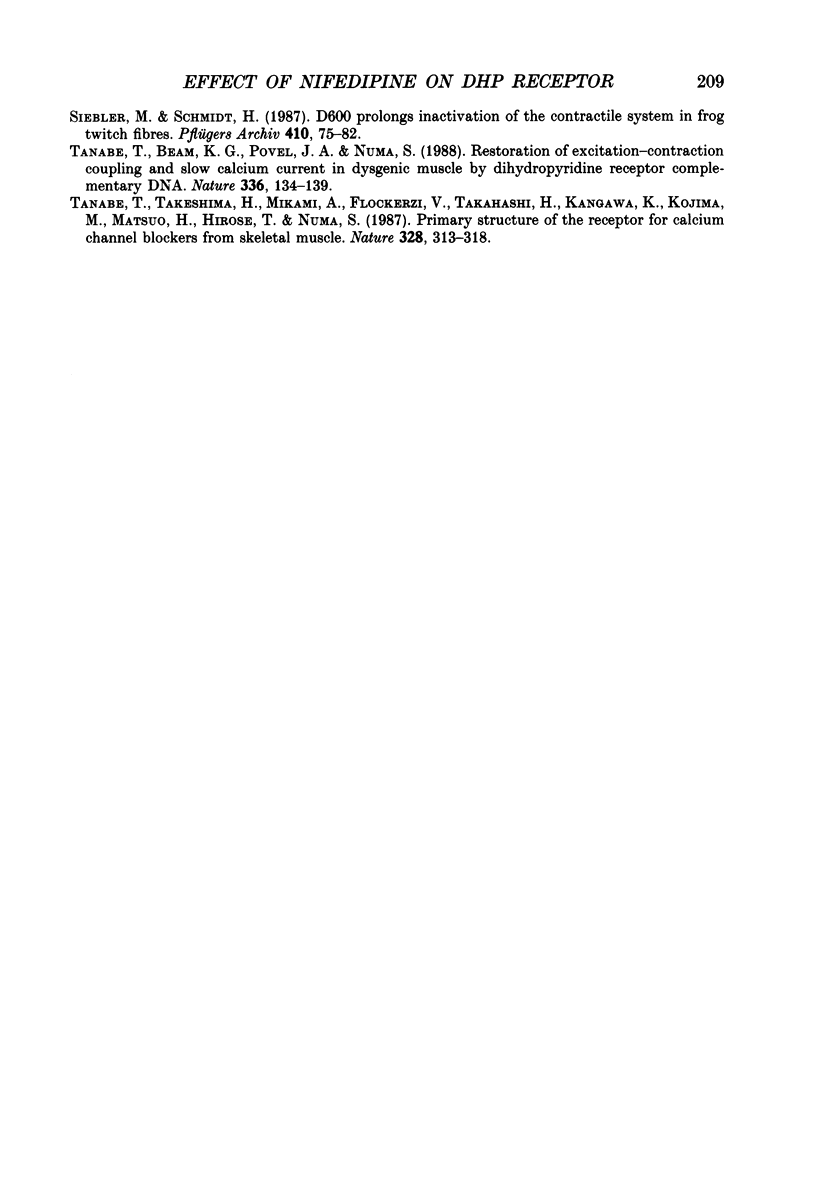
Selected References
These references are in PubMed. This may not be the complete list of references from this article.
- Bean B. P. Nitrendipine block of cardiac calcium channels: high-affinity binding to the inactivated state. Proc Natl Acad Sci U S A. 1984 Oct;81(20):6388–6392. doi: 10.1073/pnas.81.20.6388. [DOI] [PMC free article] [PubMed] [Google Scholar]
- Berwe D., Gottschalk G., Lüttgau H. C. Effects of the calcium antagonist gallopamil (D600) upon excitation-contraction coupling in toe muscle fibres of the frog. J Physiol. 1987 Apr;385:693–707. doi: 10.1113/jphysiol.1987.sp016515. [DOI] [PMC free article] [PubMed] [Google Scholar]
- Caputo C., Bolaños P. Contractile inactivation in frog skeletal muscle fibers. The effects of low calcium, tetracaine, dantrolene, D-600, and nifedipine. J Gen Physiol. 1987 Mar;89(3):421–442. doi: 10.1085/jgp.89.3.421. [DOI] [PMC free article] [PubMed] [Google Scholar]
- Cognard C., Romey G., Galizzi J. P., Fosset M., Lazdunski M. Dihydropyridine-sensitive Ca2+ channels in mammalian skeletal muscle cells in culture: electrophysiological properties and interactions with Ca2+ channel activator (Bay K8644) and inhibitor (PN 200-110). Proc Natl Acad Sci U S A. 1986 Mar;83(5):1518–1522. doi: 10.1073/pnas.83.5.1518. [DOI] [PMC free article] [PubMed] [Google Scholar]
- Cota G., Stefani E. A fast-activated inward calcium current in twitch muscle fibres of the frog (Rana montezume). J Physiol. 1986 Jan;370:151–163. doi: 10.1113/jphysiol.1986.sp015927. [DOI] [PMC free article] [PubMed] [Google Scholar]
- Dulhunty A. F., Gage P. W. Effects of extracellular calcium concentration and dihydropyridines on contraction in mammalian skeletal muscle. J Physiol. 1988 May;399:63–80. doi: 10.1113/jphysiol.1988.sp017068. [DOI] [PMC free article] [PubMed] [Google Scholar]
- Ebel S., Schütz H., Hornitschek A. Untersuchungen zur Analytik von Nifedipin unter besonderer Berücksichtigung der bei Lichtexposition entstehenden Umwandlungsprodukte. Arzneimittelforschung. 1978;28(12):2188–2193. [PubMed] [Google Scholar]
- Eisenberg R. S., McCarthy R. T., Milton R. L. Paralysis of frog skeletal muscle fibres by the calcium antagonist D-600. J Physiol. 1983 Aug;341:495–505. doi: 10.1113/jphysiol.1983.sp014819. [DOI] [PMC free article] [PubMed] [Google Scholar]
- Erdmann R., Lüttgau H. C. The effect of the phenylalkylamine D888 (devapamil) on force and Ca2+ current in isolated frog skeletal muscle fibres. J Physiol. 1989 Jun;413:521–541. doi: 10.1113/jphysiol.1989.sp017667. [DOI] [PMC free article] [PubMed] [Google Scholar]
- Feldmeyer D., Melzer W., Pohl B. Effects of gallopamil on calcium release and intramembrane charge movements in frog skeletal muscle fibres. J Physiol. 1990 Feb;421:343–362. doi: 10.1113/jphysiol.1990.sp017948. [DOI] [PMC free article] [PubMed] [Google Scholar]
- Glossmann H., Striessnig J. Calcium channels. Vitam Horm. 1988;44:155–328. doi: 10.1016/s0083-6729(08)60695-0. [DOI] [PubMed] [Google Scholar]
- Gomolla M., Gottschalk G., Lüttgau H. C. Perchlorate-induced alterations in electrical and mechanical parameters of frog skeletal muscle fibres. J Physiol. 1983 Oct;343:197–214. doi: 10.1113/jphysiol.1983.sp014888. [DOI] [PMC free article] [PubMed] [Google Scholar]
- Gurney A. M., Nerbonne J. M., Lester H. A. Photoinduced removal of nifedipine reveals mechanisms of calcium antagonist action on single heart cells. J Gen Physiol. 1985 Sep;86(3):353–379. doi: 10.1085/jgp.86.3.353. [DOI] [PMC free article] [PubMed] [Google Scholar]
- Hille B. Local anesthetics: hydrophilic and hydrophobic pathways for the drug-receptor reaction. J Gen Physiol. 1977 Apr;69(4):497–515. doi: 10.1085/jgp.69.4.497. [DOI] [PMC free article] [PubMed] [Google Scholar]
- Hofmann F., Schneider T., Röhrkasten A., Nastainczyk W., Sieber M., Ruth P., Flockerzi V. Calcium-Kanäle: Struktur und Funktion des Rezeptors für Calcium-Kanalblocker im Skelettmuskel. Arzneimittelforschung. 1989 Jan;39(1A):164–168. [PubMed] [Google Scholar]
- Hui C. S., Milton R. L., Eisenberg R. S. Charge movement in skeletal muscle fibers paralyzed by the calcium-entry blocker D600. Proc Natl Acad Sci U S A. 1984 Apr;81(8):2582–2585. doi: 10.1073/pnas.81.8.2582. [DOI] [PMC free article] [PubMed] [Google Scholar]
- Ildefonse M., Jacquemond V., Rougier O., Renaud J. F., Fosset M., Lazdunski M. Excitation contraction coupling in skeletal muscle: evidence for a role of slow Ca2+ channels using Ca2+ channel activators and inhibitors in the dihydropyridine series. Biochem Biophys Res Commun. 1985 Jun 28;129(3):904–909. doi: 10.1016/0006-291x(85)91977-1. [DOI] [PubMed] [Google Scholar]
- Lacerda A. E., Brown A. M. Nonmodal gating of cardiac calcium channels as revealed by dihydropyridines. J Gen Physiol. 1989 Jun;93(6):1243–1273. doi: 10.1085/jgp.93.6.1243. [DOI] [PMC free article] [PubMed] [Google Scholar]
- Lamb G. D. Components of charge movement in rabbit skeletal muscle: the effect of tetracaine and nifedipine. J Physiol. 1986 Jul;376:85–100. doi: 10.1113/jphysiol.1986.sp016143. [DOI] [PMC free article] [PubMed] [Google Scholar]
- Lamb G. D., Walsh T. Calcium currents, charge movement and dihydropyridine binding in fast- and slow-twitch muscles of rat and rabbit. J Physiol. 1987 Dec;393:595–617. doi: 10.1113/jphysiol.1987.sp016843. [DOI] [PMC free article] [PubMed] [Google Scholar]
- Lüttgau H. C., Gottschalk G., Berwe D. The effect of calcium and Ca antagonists upon excitation-contraction coupling. Can J Physiol Pharmacol. 1987 Apr;65(4):717–723. doi: 10.1139/y87-118. [DOI] [PubMed] [Google Scholar]
- Lüttgau H. C., Gottschalk G., Kovács L., Fuxreiter M. How perchlorate improves excitation-contraction coupling in skeletal muscle fibers. Biophys J. 1983 Aug;43(2):247–249. doi: 10.1016/S0006-3495(83)84346-X. [DOI] [PMC free article] [PubMed] [Google Scholar]
- Lüttgau H. C., Spiecker W. The effects of calcium deprivation upon mechanical and electrophysiological parameters in skeletal muscle fibres of the frog. J Physiol. 1979 Nov;296:411–429. doi: 10.1113/jphysiol.1979.sp013013. [DOI] [PMC free article] [PubMed] [Google Scholar]
- McCleskey E. W. Calcium channels and intracellular calcium release are pharmacologically different in frog skeletal muscle. J Physiol. 1985 Apr;361:231–249. doi: 10.1113/jphysiol.1985.sp015643. [DOI] [PMC free article] [PubMed] [Google Scholar]
- Melzer W., Schneider M. F., Simon B. J., Szucs G. Intramembrane charge movement and calcium release in frog skeletal muscle. J Physiol. 1986 Apr;373:481–511. doi: 10.1113/jphysiol.1986.sp016059. [DOI] [PMC free article] [PubMed] [Google Scholar]
- Pizarro G., Fitts R., Uribe I., Ríos E. The voltage sensor of excitation-contraction coupling in skeletal muscle. Ion dependence and selectivity. J Gen Physiol. 1989 Sep;94(3):405–428. doi: 10.1085/jgp.94.3.405. [DOI] [PMC free article] [PubMed] [Google Scholar]
- Porzig H., Becker C. Potential-dependent allosteric modulation of 1,4-dihydropyridine binding by d-(cis)-diltiazem and (+/-)-verapamil in living cardiac cells. Mol Pharmacol. 1988 Aug;34(2):172–179. [PubMed] [Google Scholar]
- Rios E., Brum G. Involvement of dihydropyridine receptors in excitation-contraction coupling in skeletal muscle. Nature. 1987 Feb 19;325(6106):717–720. doi: 10.1038/325717a0. [DOI] [PubMed] [Google Scholar]
- Sanchez J. A., Stefani E. Inward calcium current in twitch muscle fibres of the frog. J Physiol. 1978 Oct;283:197–209. doi: 10.1113/jphysiol.1978.sp012496. [DOI] [PMC free article] [PubMed] [Google Scholar]
- Schneider M. F., Chandler W. K. Voltage dependent charge movement of skeletal muscle: a possible step in excitation-contraction coupling. Nature. 1973 Mar 23;242(5395):244–246. doi: 10.1038/242244a0. [DOI] [PubMed] [Google Scholar]
- Schwartz L. M., McCleskey E. W., Almers W. Dihydropyridine receptors in muscle are voltage-dependent but most are not functional calcium channels. 1985 Apr 25-May 1Nature. 314(6013):747–751. doi: 10.1038/314747a0. [DOI] [PubMed] [Google Scholar]
- Siebler M., Schmidt H. D600 prolongs inactivation of the contractile system in frog twitch fibres. Pflugers Arch. 1987 Sep;410(1-2):75–82. doi: 10.1007/BF00581899. [DOI] [PubMed] [Google Scholar]
- Tanabe T., Beam K. G., Powell J. A., Numa S. Restoration of excitation-contraction coupling and slow calcium current in dysgenic muscle by dihydropyridine receptor complementary DNA. Nature. 1988 Nov 10;336(6195):134–139. doi: 10.1038/336134a0. [DOI] [PubMed] [Google Scholar]
- Tanabe T., Takeshima H., Mikami A., Flockerzi V., Takahashi H., Kangawa K., Kojima M., Matsuo H., Hirose T., Numa S. Primary structure of the receptor for calcium channel blockers from skeletal muscle. Nature. 1987 Jul 23;328(6128):313–318. doi: 10.1038/328313a0. [DOI] [PubMed] [Google Scholar]