Abstract
1. Our findings of a low total magnesium content in the dense fraction (over 1.118 g ml-1) of sickle cell anaemia (SS) red cells seemed inconsistent with the low Mg2+ permeability and outward Mg2+ gradient seen in normal red cells, and prompted studies of the Mg2+ permeability and equilibria in the SS cells. 2. Deoxygenation and sickling induced Mg2+ permeabilization in SS cells, supporting non-specificity of the sickling-induced cation permeabilization, previously described for Na+, K+ and Ca2+. The extent of Mg2+ permeabilization was comparable in SS cells with normal or high density. 3. Compared with normal-density SS cells and normal red cells, the dense SS cells showed a much larger increase in the fraction of ionized magnesium ([Mg2+]i) on deoxygenation, resulting in [Mg2+]i levels sufficient to reverse the normal inward direction of the transmembrane Mg2+ gradient. 4. The molar ratio of 2,3-diphosphoglycerate (2,3-DPG) to haemoglobin was markedly reduced in the dense SS cells. Since 2,3-DPG and ATP are the main cytoplasmic Mg2+ buffers, their further reduction upon binding to deoxyhaemoglobin accounts for the high [Mg2+]i in the deoxygenated dense SS cells; the resulting outward electrochemical Mg2+ gradient, together with sickling-induced Mg2+ permeabilization, could explain the decreased total magnesium content of these cells. 5. The above findings suggested that the documented low sodium pump fluxes in dense SS cells may result from an increased Mg2+:ATP ratio, which is known to inhibit Na(+)-K+ exchange fluxes through the sodium pump. If so, deoxygenation, by increasing the Mg2+:ATP ratio, should inhibit the pump further, whereas increasing ATP should relieve the inhibition. Experiments designed to test this possibility showed that in these dense SS cells, the ouabain-sensitive K(86Rb) influx was low in oxygenated cells, was reduced further by deoxygenation, but was substantially increased after treatment with inosine, pyruvate and phosphate to increase their organic phosphate pool. These results were thus consistent with such a mechanism for Na+ pump inhibition in the dense SS cells.
Full text
PDF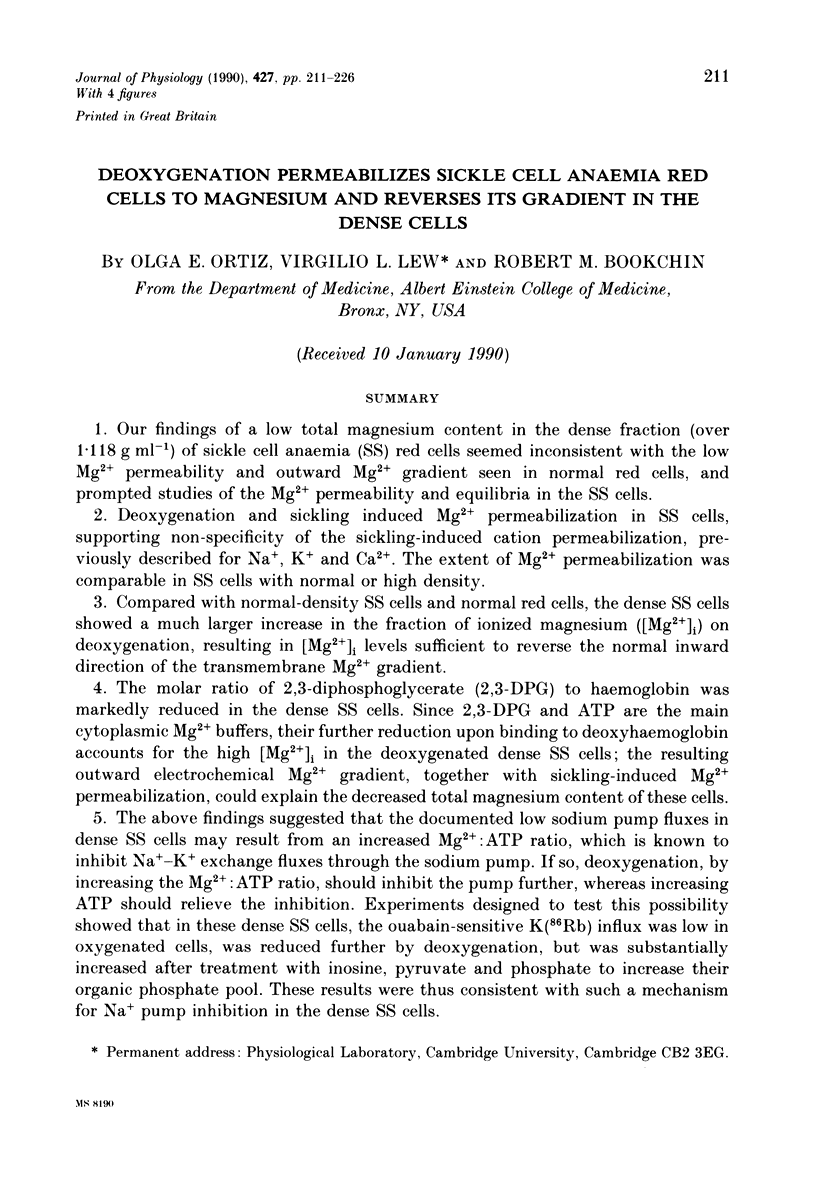
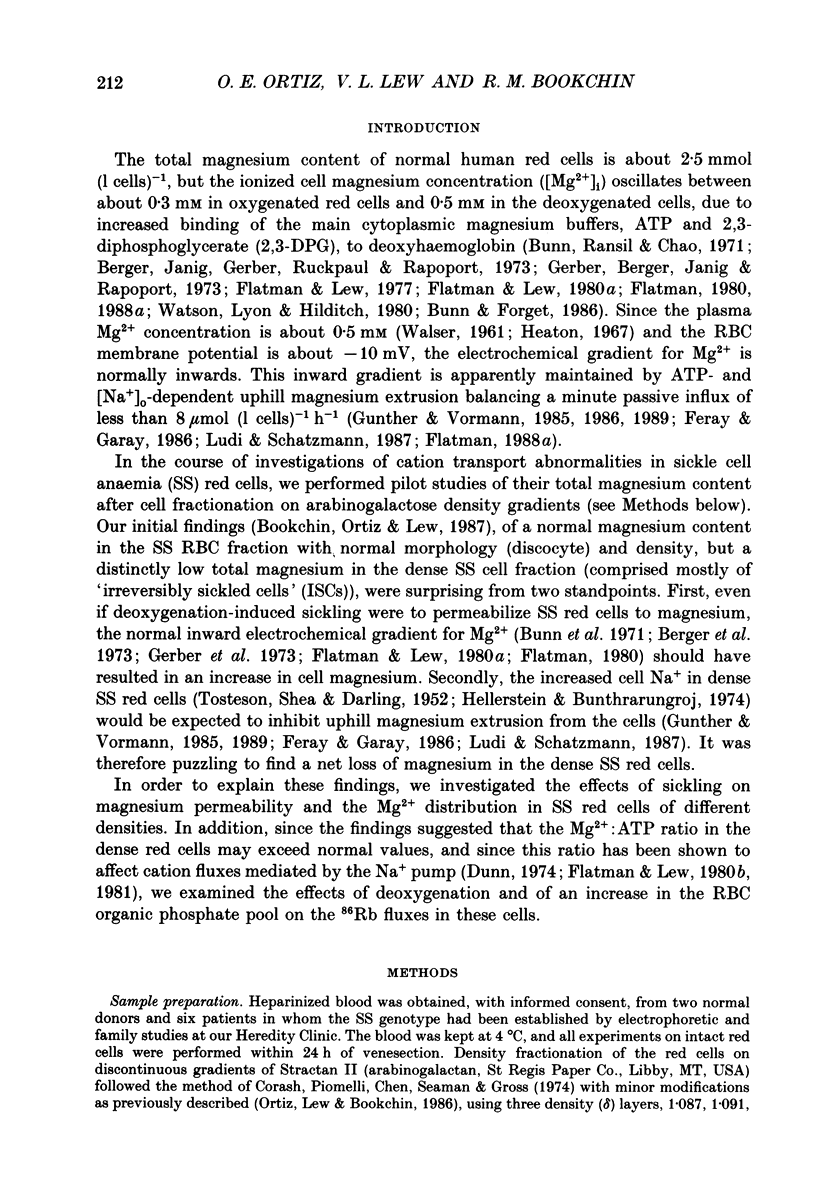
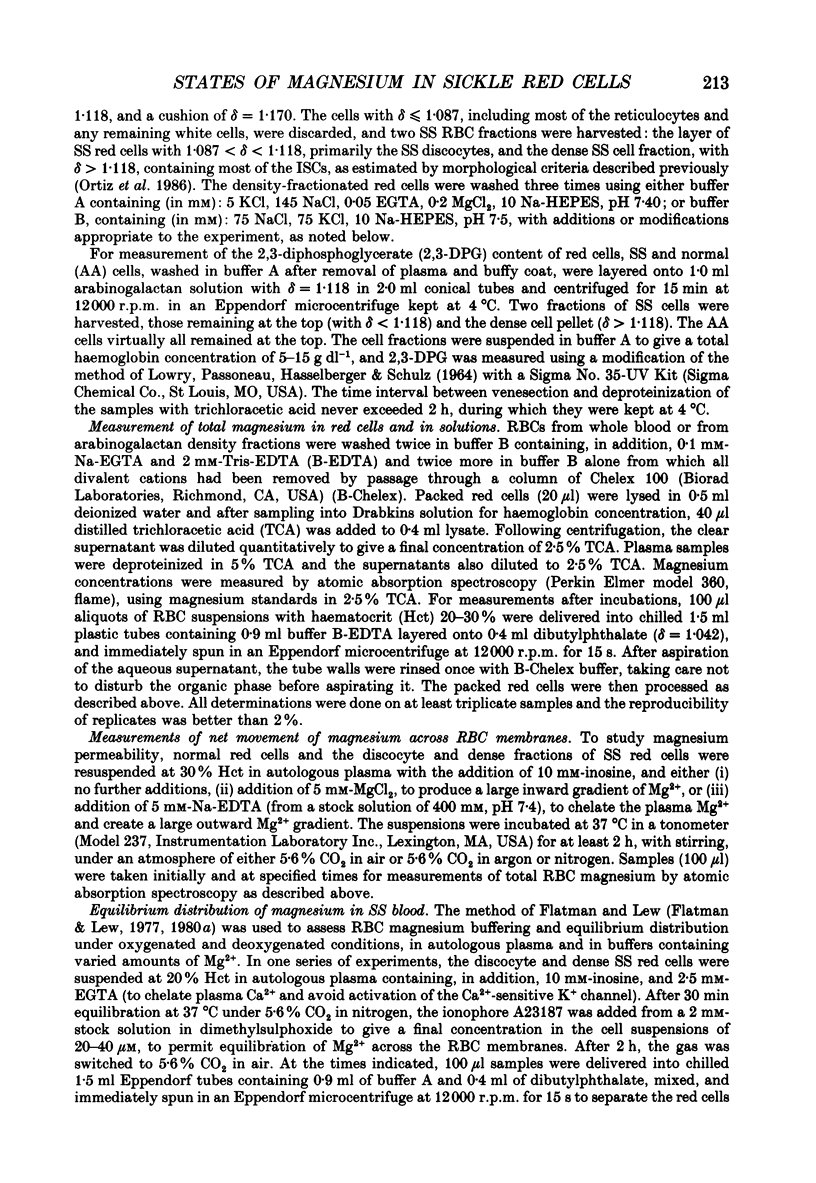
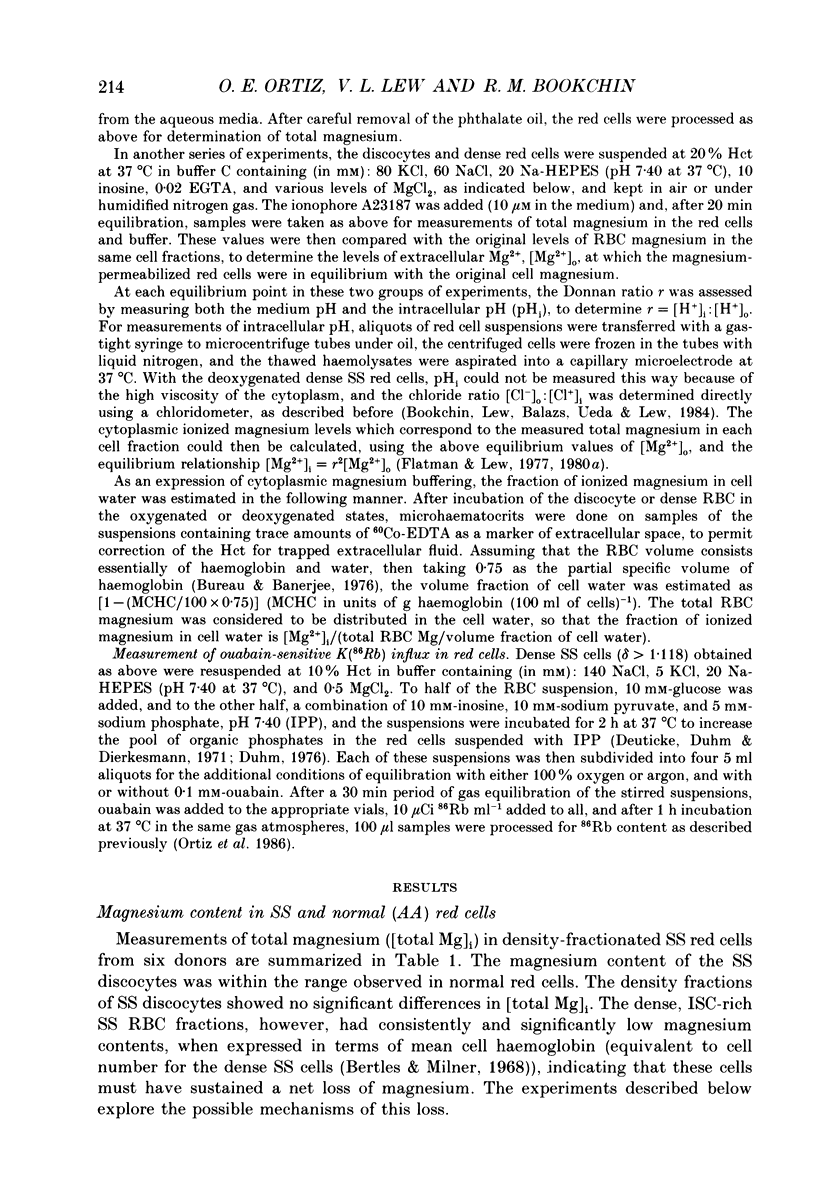
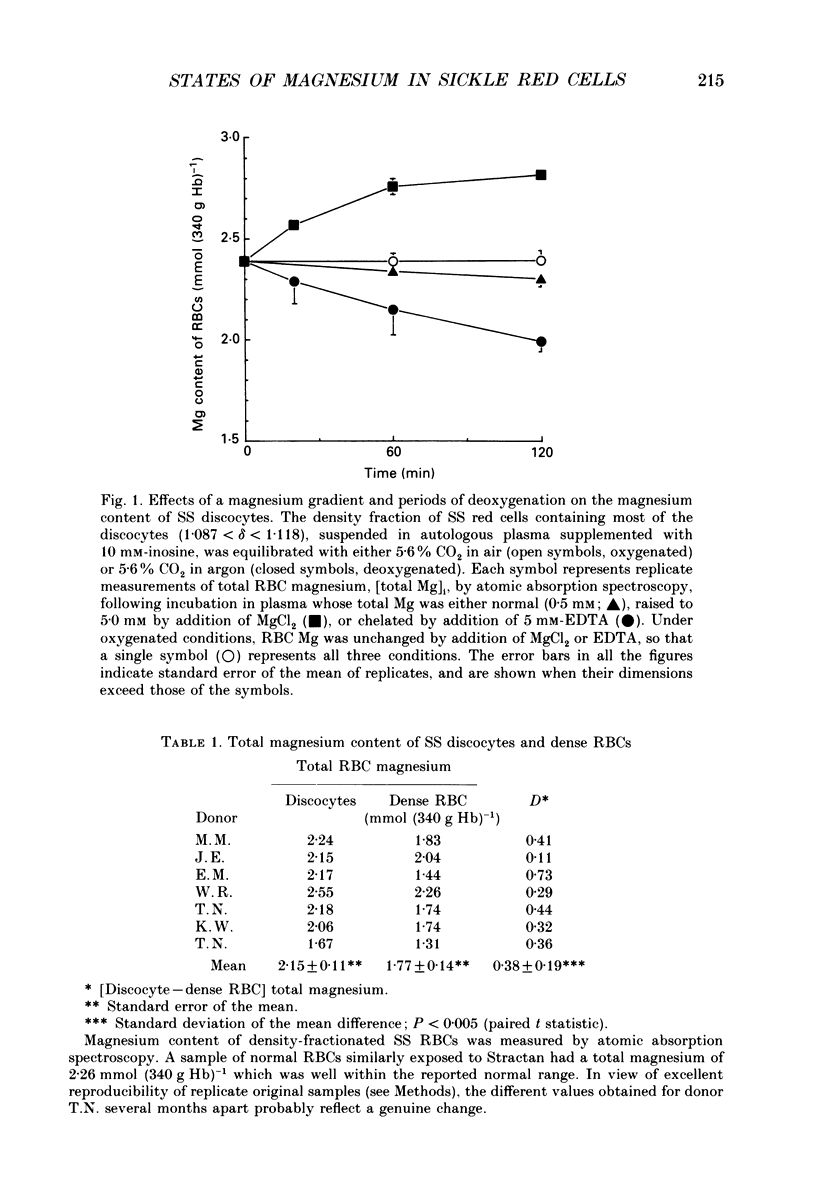
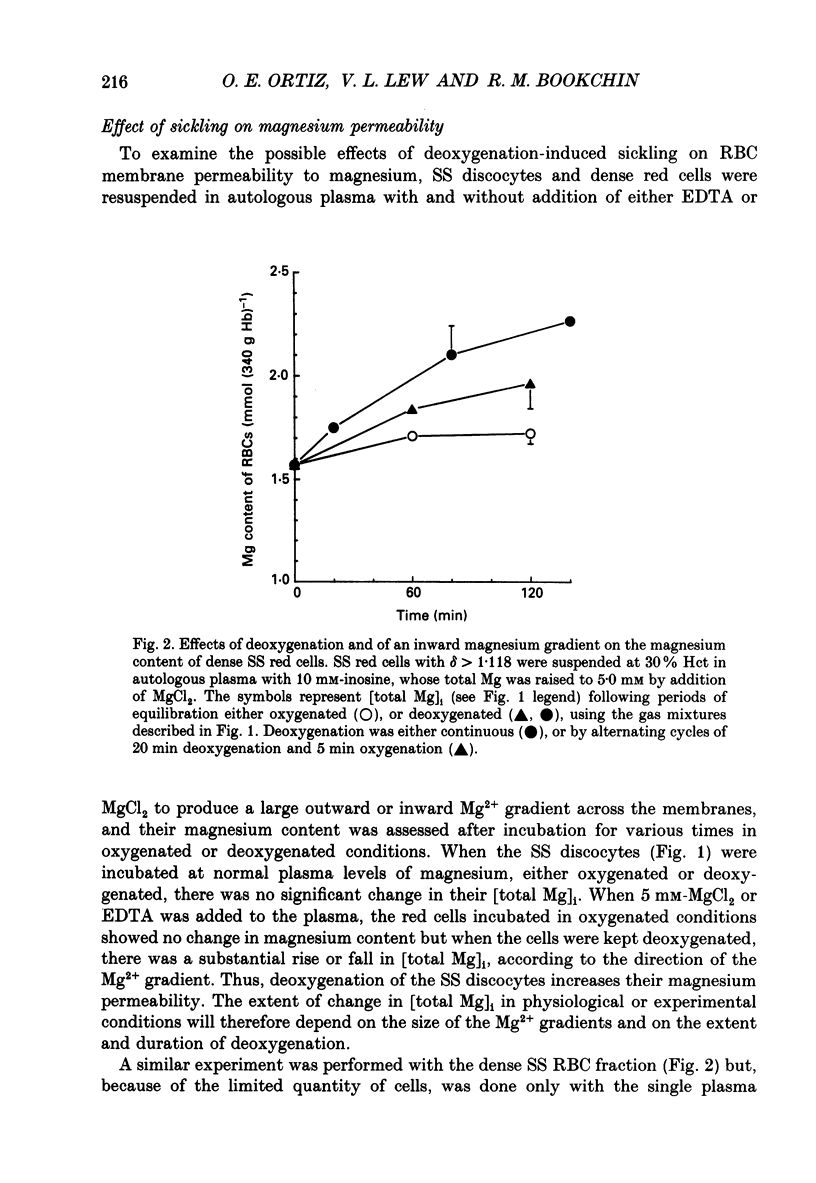
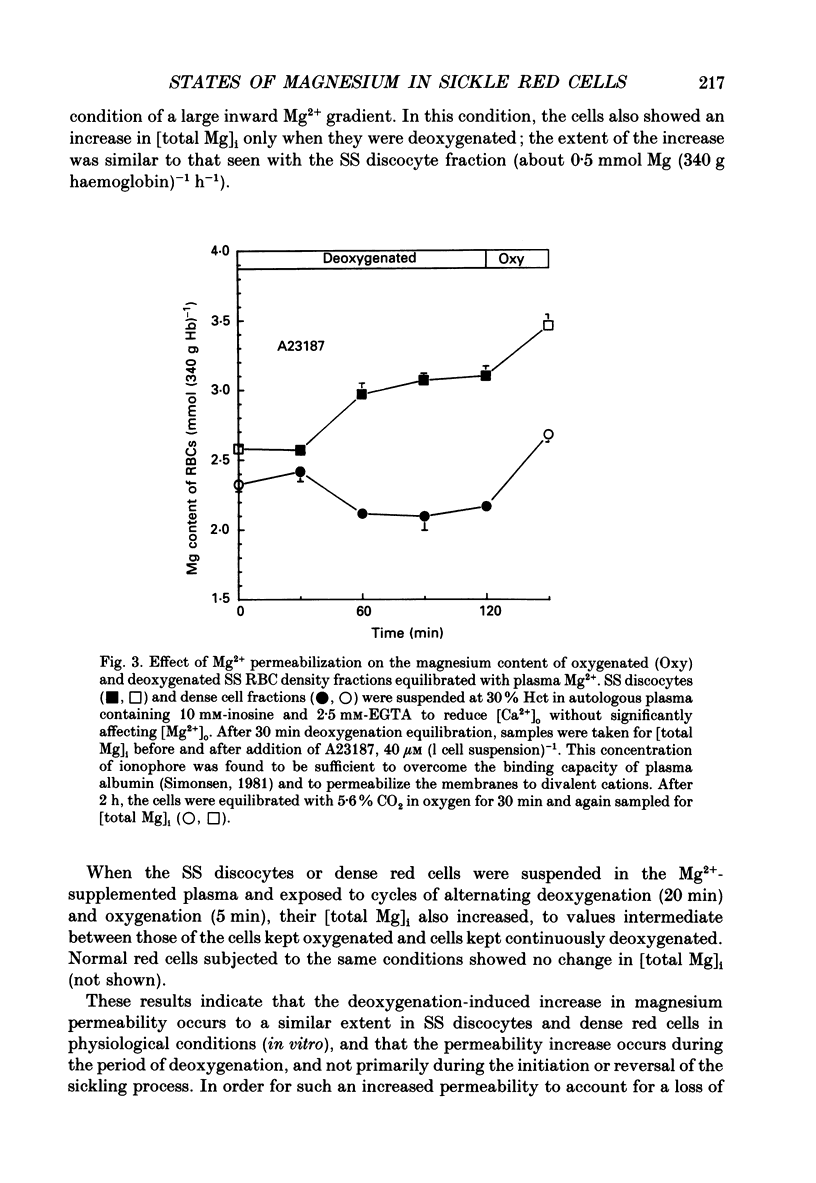
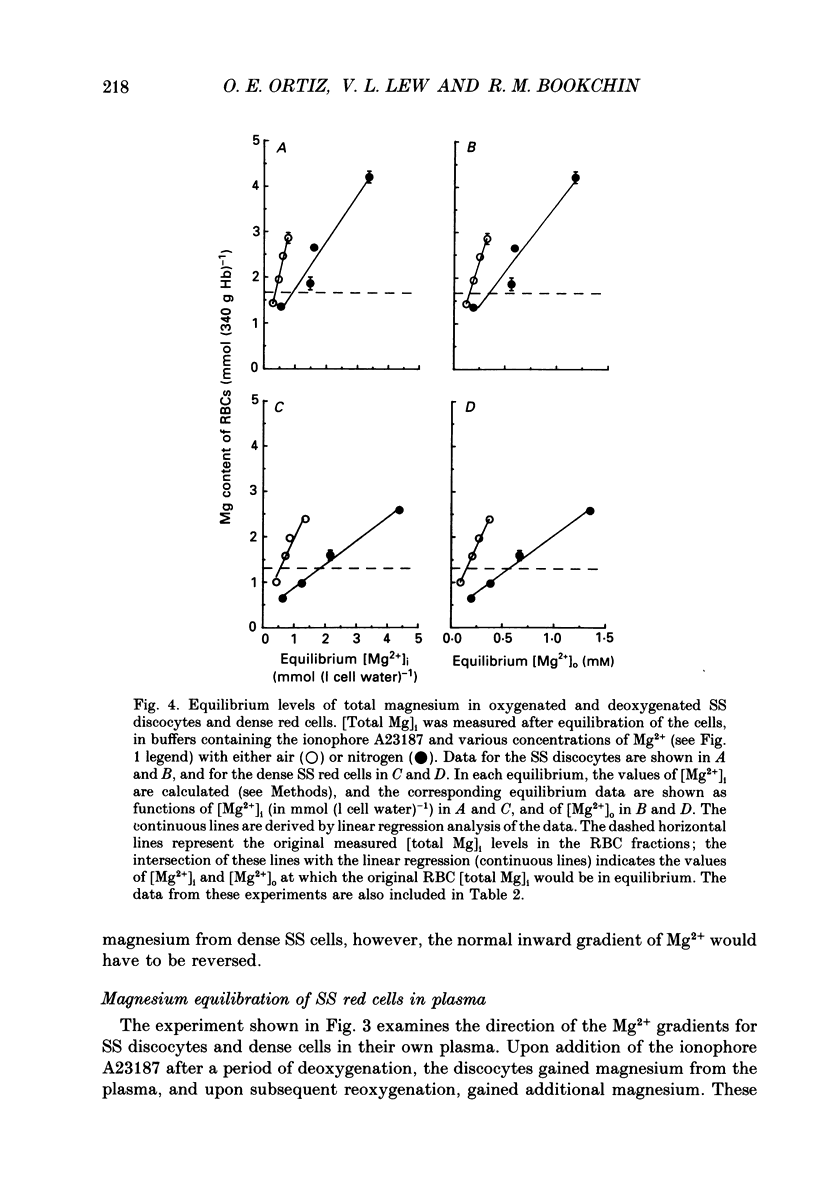
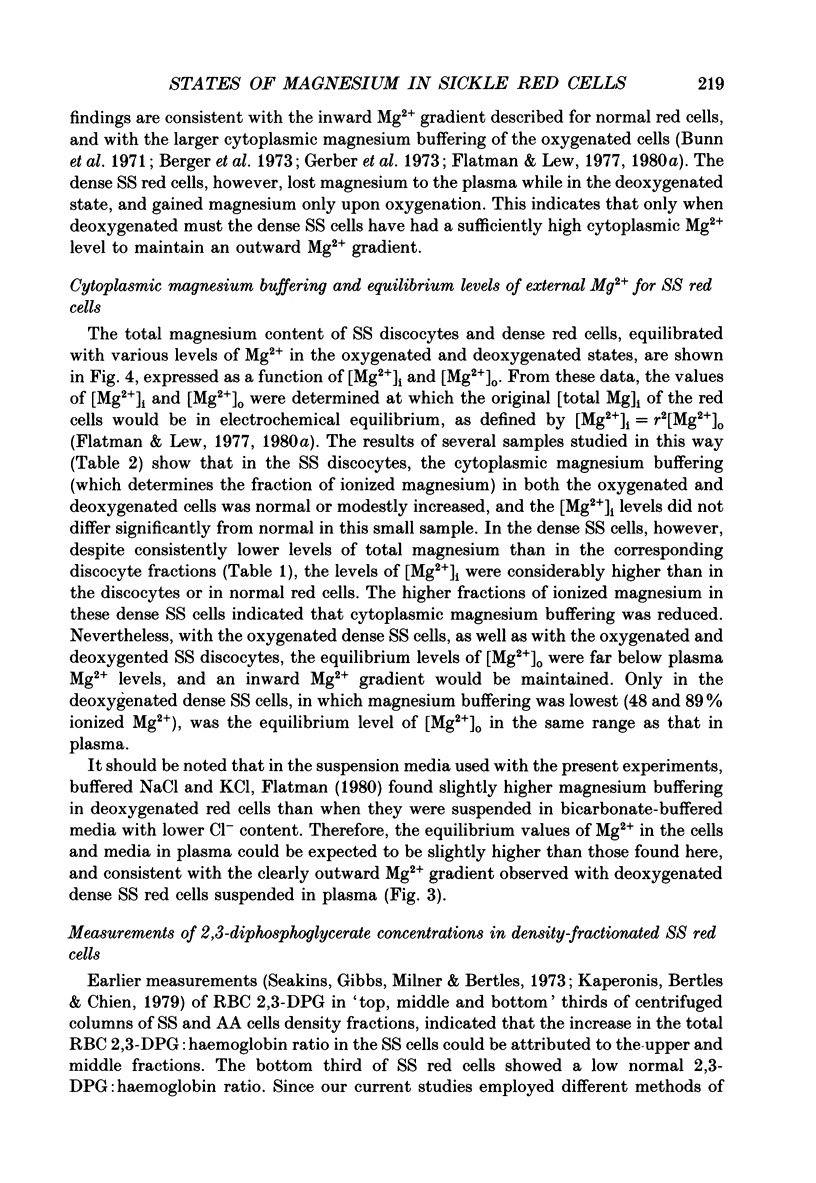
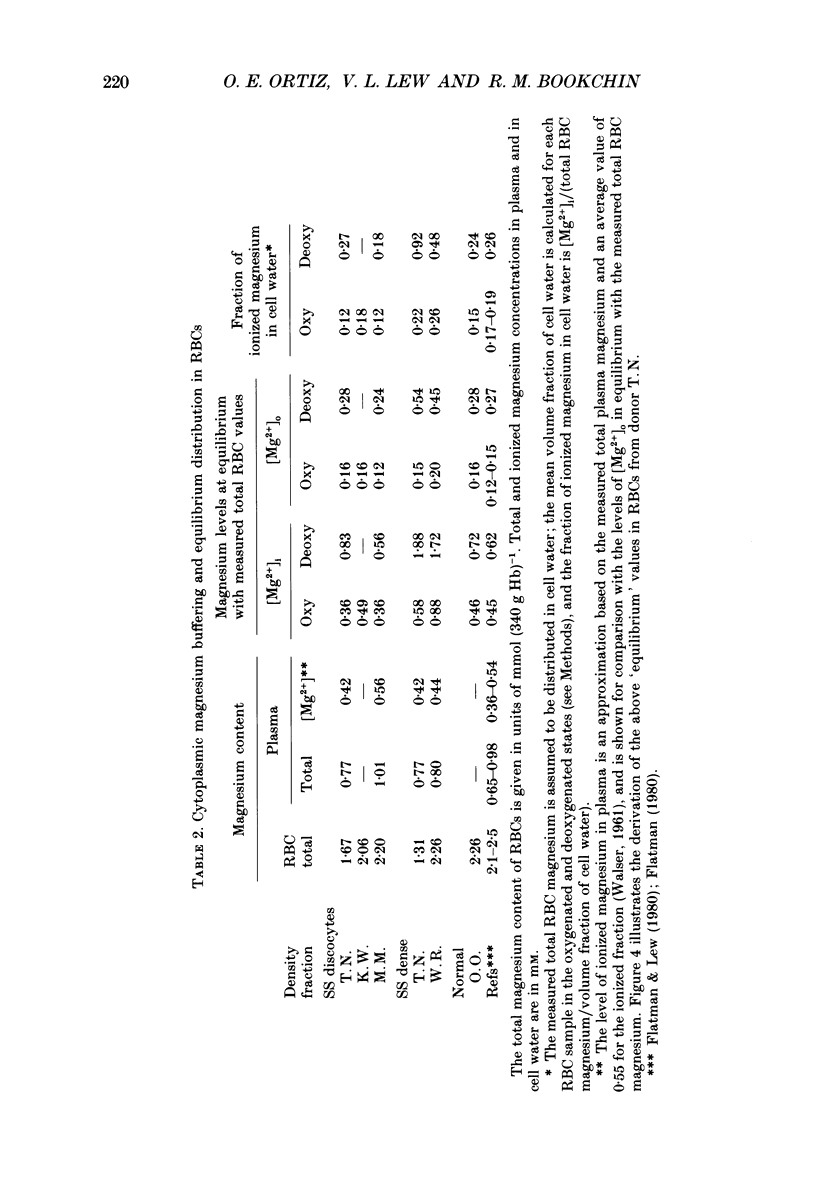
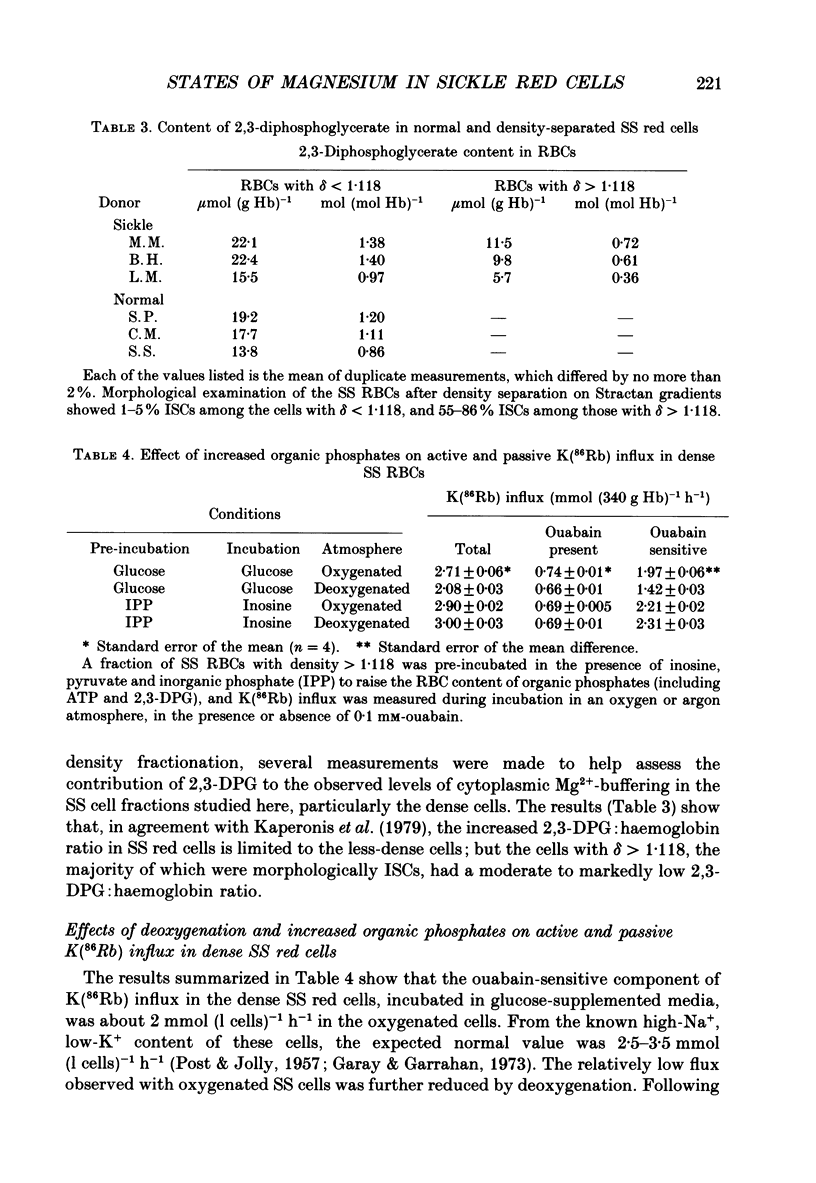
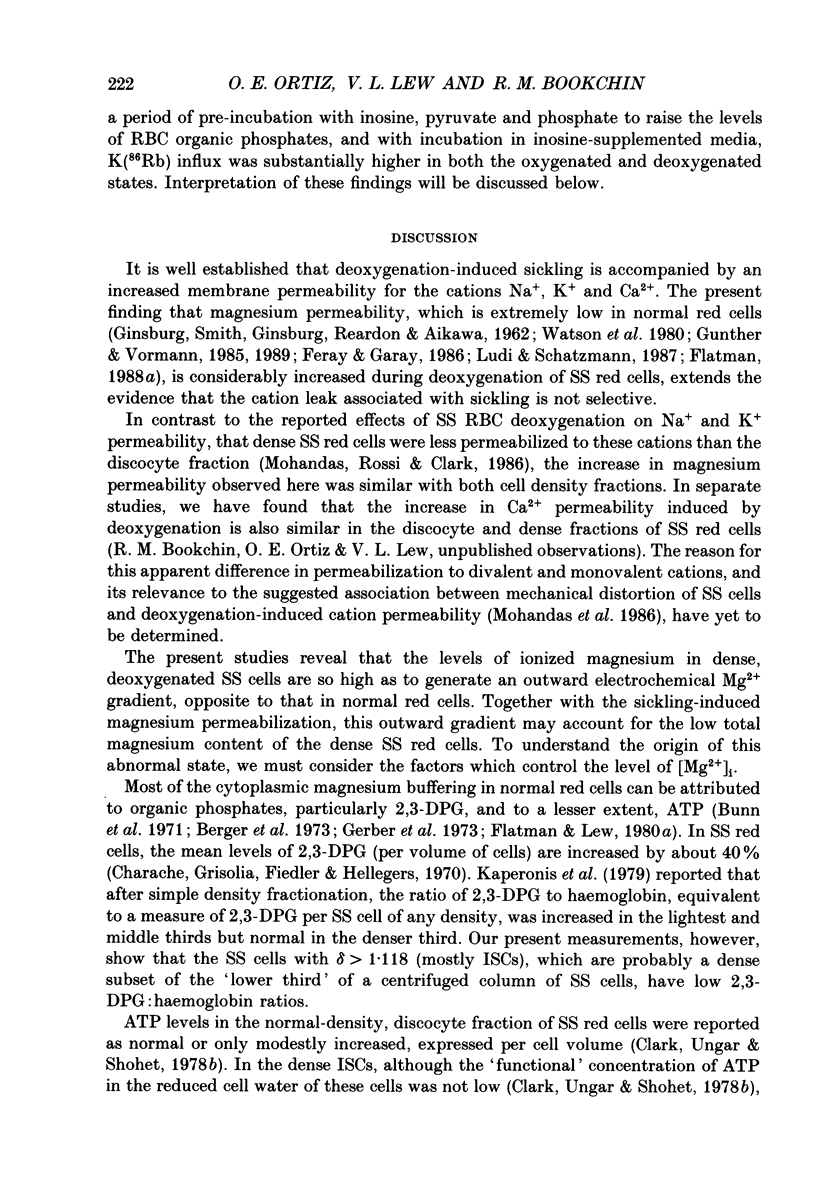
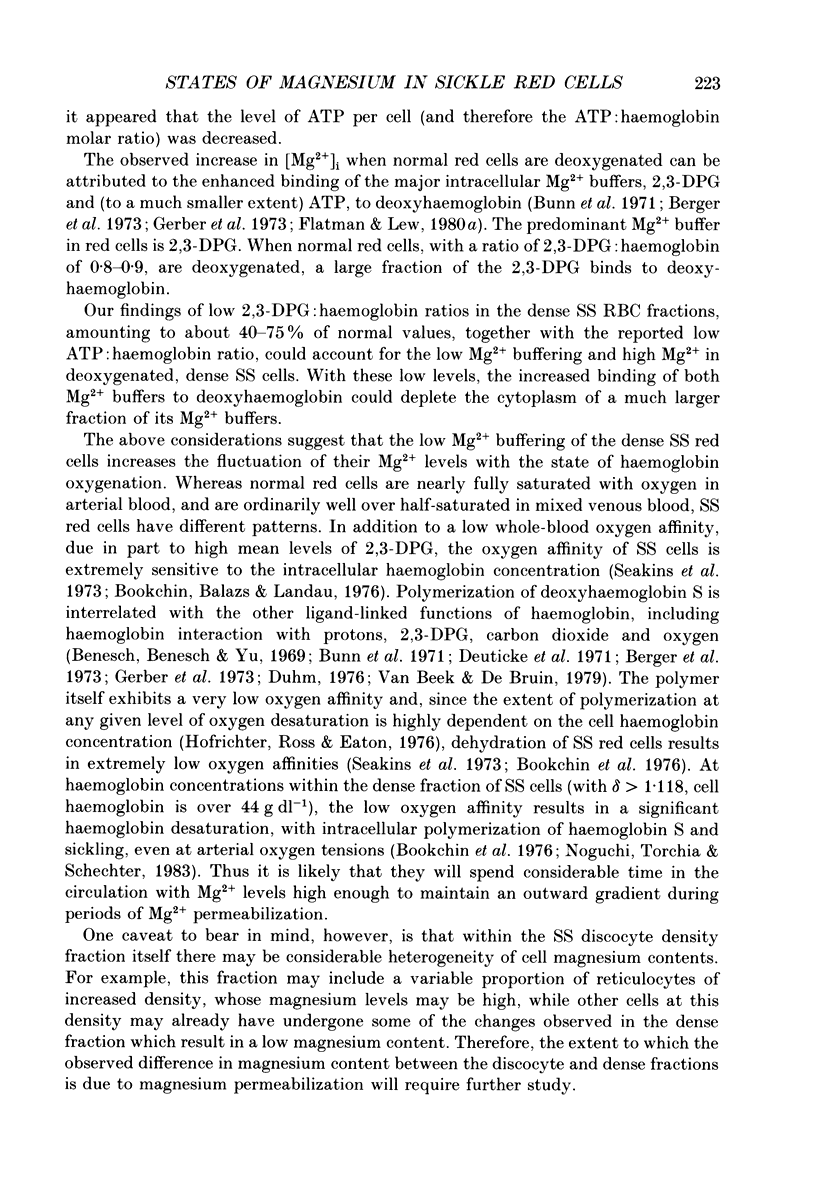
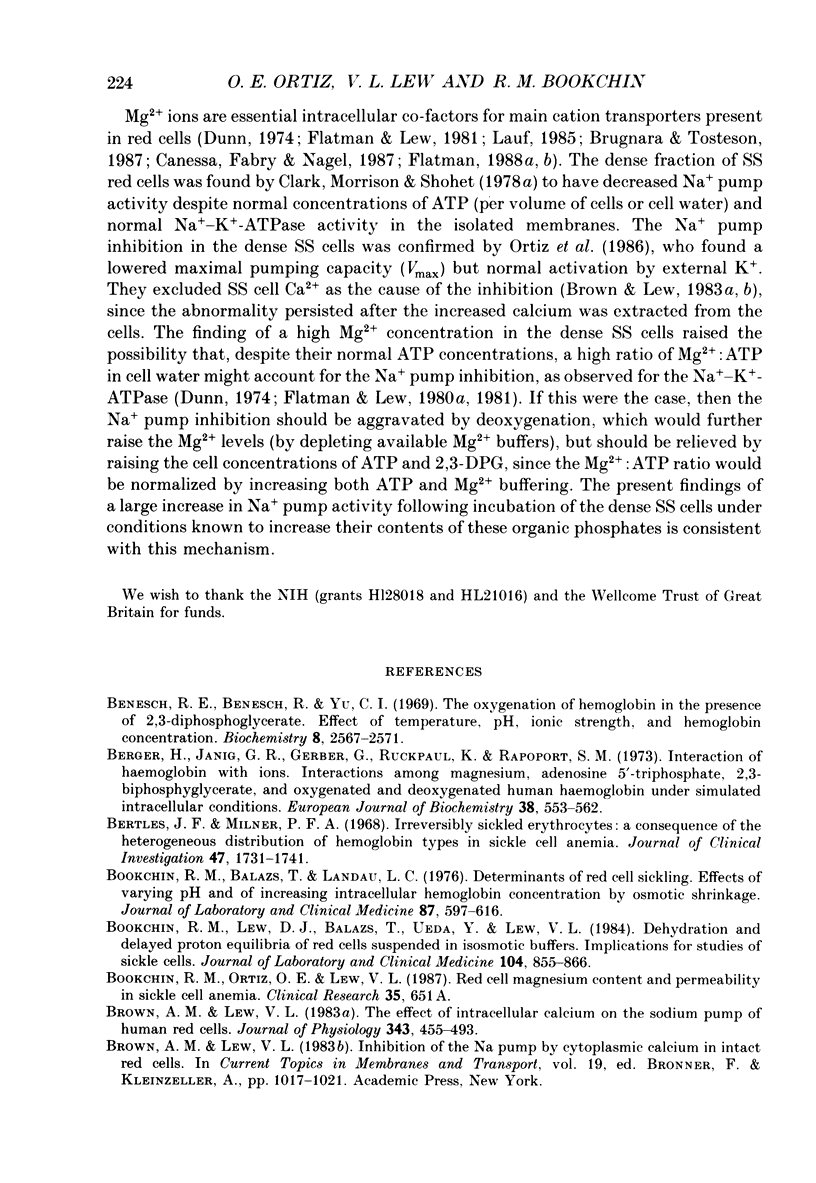
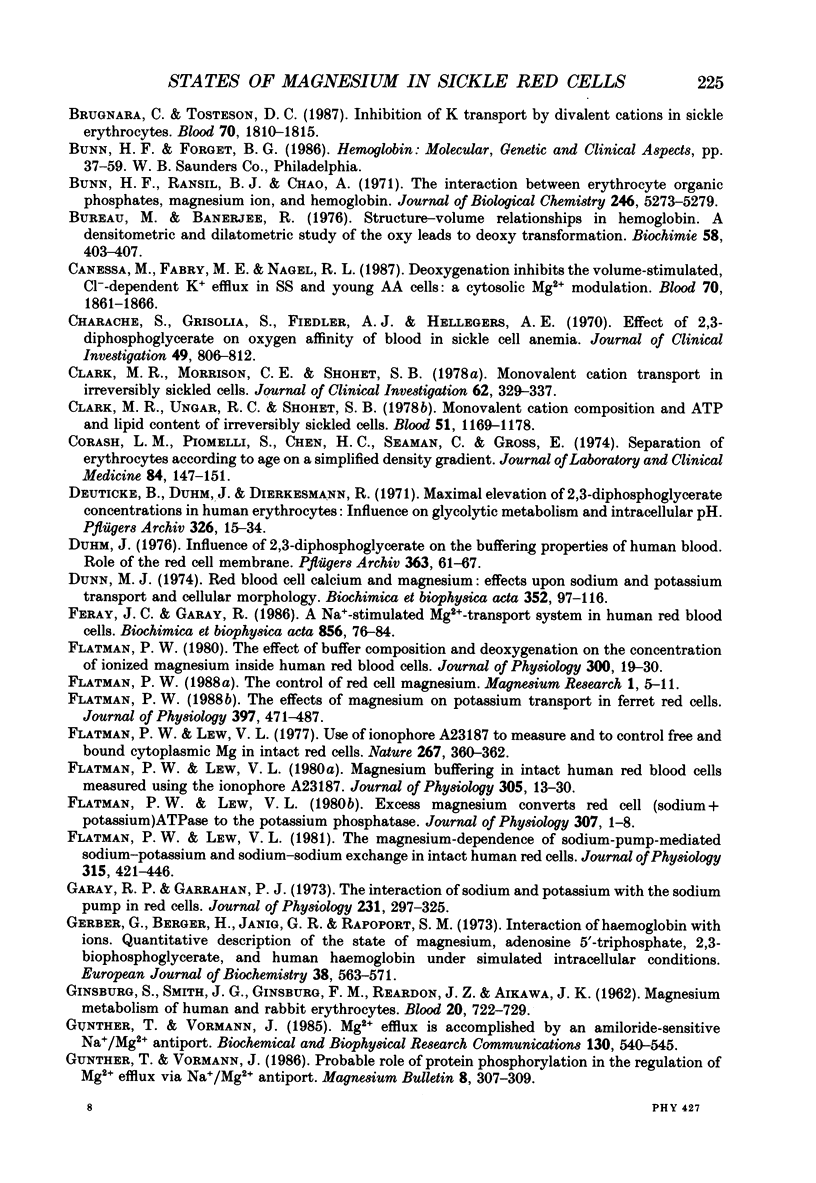
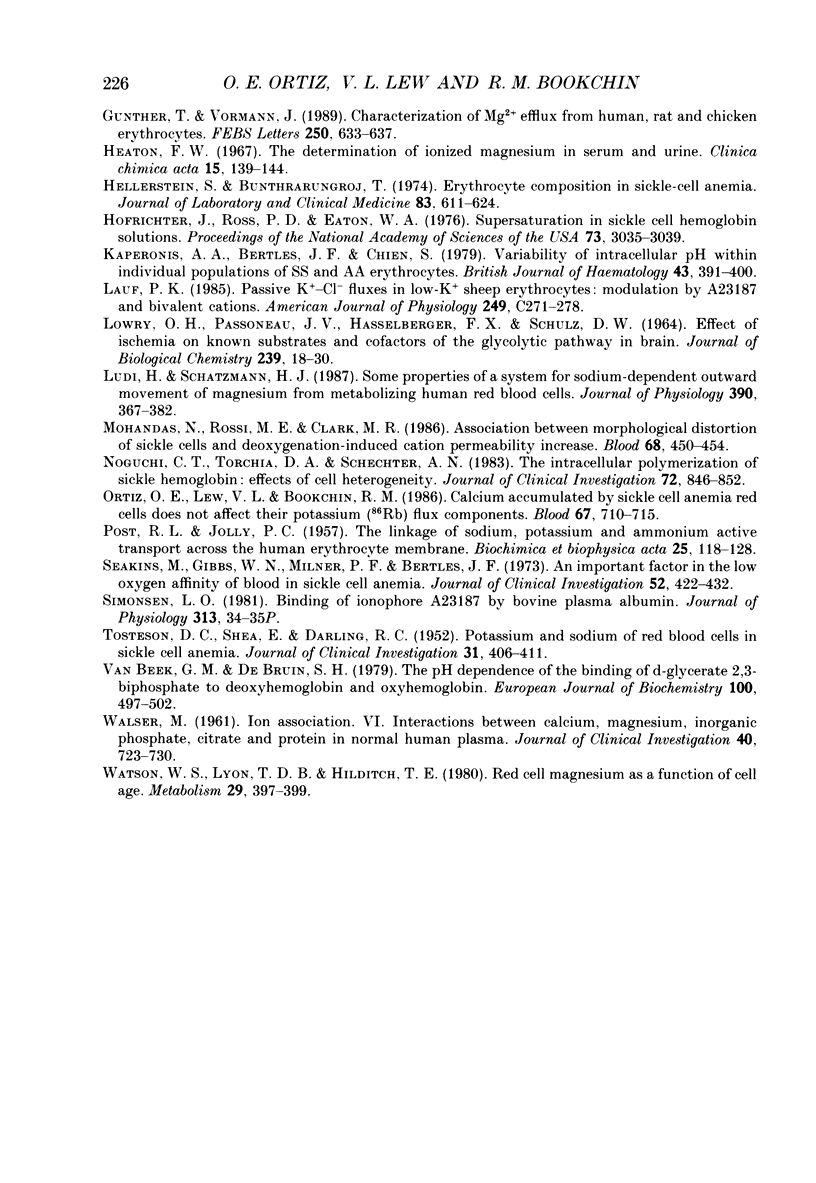
Selected References
These references are in PubMed. This may not be the complete list of references from this article.
- Benesch R. E., Benesch R., Yu C. I. The oxygenation of hemoglobin in the presence of 2,3-diphosphoglycerate. Effect of temperature, pH, ionic strength, and hemoglobin concentration. Biochemistry. 1969 Jun;8(6):2567–2571. doi: 10.1021/bi00834a046. [DOI] [PubMed] [Google Scholar]
- Berger H., Jänig G. R., Gerber G., Ruckpaul K., Rapoport S. M. Interaction of haemoglobin with ions. Interactions among magnesium, adenosine 5'-triphosphate, 2,3-bisphosphoglycerate, and oxygenated and deoxygenated human haemoglobin under simulated intracellular conditions. Eur J Biochem. 1973 Oct 18;38(3):553–562. doi: 10.1111/j.1432-1033.1973.tb03090.x. [DOI] [PubMed] [Google Scholar]
- Bertles J. F., Milner P. F. Irreversibly sickled erythrocytes: a consequence of the heterogeneous distribution of hemoglobin types in sickle-cell anemia. J Clin Invest. 1968 Aug;47(8):1731–1741. doi: 10.1172/JCI105863. [DOI] [PMC free article] [PubMed] [Google Scholar]
- Bookchin R. M., Balazs T., Landau L. C. Determinants of red cell sickling. Effects of varying pH and of increasing intracellular hemoglobin concentration by osmotic shrinkage. J Lab Clin Med. 1976 Apr;87(4):597–616. [PubMed] [Google Scholar]
- Bookchin R. M., Lew D. J., Balazs T., Ueda Y., Lew V. L. Dehydration and delayed proton equilibria of red blood cells suspended in isosmotic phosphate buffers. Implications for studies of sickled cells. J Lab Clin Med. 1984 Dec;104(6):855–866. [PubMed] [Google Scholar]
- Brown A. M., Lew V. L. The effect of intracellular calcium on the sodium pump of human red cells. J Physiol. 1983 Oct;343:455–493. doi: 10.1113/jphysiol.1983.sp014904. [DOI] [PMC free article] [PubMed] [Google Scholar]
- Brugnara C., Tosteson D. C. Inhibition of K transport by divalent cations in sickle erythrocytes. Blood. 1987 Dec;70(6):1810–1815. [PubMed] [Google Scholar]
- Bunn H. F., Ransil B. J., Chao A. The interaction between erythrocyte organic phosphates, magnesium ion, and hemoglobin. J Biol Chem. 1971 Sep 10;246(17):5273–5279. [PubMed] [Google Scholar]
- Bureau M., Banerjee R. Structure-volume relationships in hemoglobin. A densitometric and dilatometric study of the oxy leads to deoxy transformation. Biochimie. 1976;58(4):403–407. doi: 10.1016/s0300-9084(76)80249-0. [DOI] [PubMed] [Google Scholar]
- Canessa M., Fabry M. E., Nagel R. L. Deoxygenation inhibits the volume-stimulated, Cl(-)-dependent K+ efflux in SS and young AA cells: a cytosolic Mg2+ modulation. Blood. 1987 Dec;70(6):1861–1866. [PubMed] [Google Scholar]
- Charache S., Grisolia S., Fiedler A. J., Hellegers A. E. Effect of 2,3-diphosphoglycerate on oxygen affinity of blood in sickle cell anemia. J Clin Invest. 1970 Apr;49(4):806–812. doi: 10.1172/JCI106294. [DOI] [PMC free article] [PubMed] [Google Scholar]
- Clark M. R., Morrison C. E., Shohet S. B. Monovalent cation transport in irreversibly sickled cells. J Clin Invest. 1978 Aug;62(2):329–337. doi: 10.1172/JCI109133. [DOI] [PMC free article] [PubMed] [Google Scholar]
- Clark M. R., Unger R. C., Shohet S. B. Monovalent cation composition and ATP and lipid content of irreversibly sickled cells. Blood. 1978 Jun;51(6):1169–1178. [PubMed] [Google Scholar]
- Corash L. M., Piomelli S., Chen H. C., Seaman C., Gross E. Separation of erythrocytes according to age on a simplified density gradient. J Lab Clin Med. 1974 Jul;84(1):147–151. [PubMed] [Google Scholar]
- Deuticke B., Duhm J., Dierkesmann R. Maximal elevation of 2,3-diphosphoglycerate concentrations in human erythrocytes: influence on glycolytic metabolism and intracellular pH. Pflugers Arch. 1971;326(1):15–34. doi: 10.1007/BF00586792. [DOI] [PubMed] [Google Scholar]
- Duhm J. Influence of 2,3-diphosphoglycerate on the buffering properties of human blood: role of the red cell membrane. Pflugers Arch. 1976 May 6;363(1):61–67. doi: 10.1007/BF00587403. [DOI] [PubMed] [Google Scholar]
- Dunn M. J. Red blood cell calcium and magnesium: effects upon sodium and potassium transport and cellular morphology. Biochim Biophys Acta. 1974 May 30;352(1):97–116. doi: 10.1016/0005-2736(74)90182-5. [DOI] [PubMed] [Google Scholar]
- Flatman P. W., Lew V. L. Excess magnesium converts red cell (sodium+potassium) ATPase to the potassium phosphatase. J Physiol. 1980 Oct;307:1–8. doi: 10.1113/jphysiol.1980.sp013419. [DOI] [PMC free article] [PubMed] [Google Scholar]
- Flatman P. W., Lew V. L. Magnesium buffering in intact human red blood cells measured using the ionophore A23187. J Physiol. 1980 Aug;305:13–30. doi: 10.1113/jphysiol.1980.sp013346. [DOI] [PMC free article] [PubMed] [Google Scholar]
- Flatman P. W., Lew V. L. The magnesium dependence of sodium-pump-mediated sodium-potassium and sodium-sodium exchange in intact human red cells. J Physiol. 1981 Jun;315:421–446. doi: 10.1113/jphysiol.1981.sp013756. [DOI] [PMC free article] [PubMed] [Google Scholar]
- Flatman P. W. The control of red cell magnesium. Magnes Res. 1988 Jul;1(1-2):5–11. [PubMed] [Google Scholar]
- Flatman P. W. The effect of buffer composition and deoxygenation on the concentration of ionized magnesium inside human red blood cells. J Physiol. 1980 Mar;300:19–30. doi: 10.1113/jphysiol.1980.sp013148. [DOI] [PMC free article] [PubMed] [Google Scholar]
- Flatman P. W. The effects of magnesium on potassium transport in ferret red cells. J Physiol. 1988 Mar;397:471–487. doi: 10.1113/jphysiol.1988.sp017013. [DOI] [PMC free article] [PubMed] [Google Scholar]
- Flatman P., Lew V. L. Use of ionophore A23187 to measure and to control free and bound cytoplasmic Mg in intact red cells. Nature. 1977 May 26;267(5609):360–362. doi: 10.1038/267360a0. [DOI] [PubMed] [Google Scholar]
- Féray J. C., Garay R. An Na+-stimulated Mg2+-transport system in human red blood cells. Biochim Biophys Acta. 1986 Mar 27;856(1):76–84. doi: 10.1016/0005-2736(86)90012-x. [DOI] [PubMed] [Google Scholar]
- GINSBURG S., SMITH J. G., GINSBURG F. M., REARDON J. Z., AIKAWA J. K. Magnesium metabolism of human and rabbit erythrocytes. Blood. 1962 Dec;20:722–729. [PubMed] [Google Scholar]
- Garay R. P., Garrahan P. J. The interaction of sodium and potassium with the sodium pump in red cells. J Physiol. 1973 Jun;231(2):297–325. doi: 10.1113/jphysiol.1973.sp010234. [DOI] [PMC free article] [PubMed] [Google Scholar]
- Gerber G., Berger H., Jänig G. R., Rapoport S. M. Interaction of haemoglobin with ions. Quantitative description of the state of magnesium, adenosine 5'-triphosphate, 2,3-bisphosphoglycerate, and human haemoglobin under simulated intracellular conditions. Eur J Biochem. 1973 Oct 18;38(3):563–571. doi: 10.1111/j.1432-1033.1973.tb03091.x. [DOI] [PubMed] [Google Scholar]
- Günther T., Vormann J. Characterization of Mg2+ efflux from human, rat and chicken erythrocytes. FEBS Lett. 1989 Jul 3;250(2):633–637. doi: 10.1016/0014-5793(89)80812-9. [DOI] [PubMed] [Google Scholar]
- Günther T., Vormann J. Mg2+ efflux is accomplished by an amiloride-sensitive Na+/Mg2+ antiport. Biochem Biophys Res Commun. 1985 Jul 31;130(2):540–545. doi: 10.1016/0006-291x(85)90450-4. [DOI] [PubMed] [Google Scholar]
- Hellerstein S., Bunthrarungroj T. Erythrocyte composition in sickle-cell anemia. J Lab Clin Med. 1974 Apr;83(4):611–624. [PubMed] [Google Scholar]
- Hofrichter J., Ross P. D., Eaton W. A. Supersaturation in sickle cell hemoglobin solutions. Proc Natl Acad Sci U S A. 1976 Sep;73(9):3035–3039. doi: 10.1073/pnas.73.9.3035. [DOI] [PMC free article] [PubMed] [Google Scholar]
- Kaperonis A. A., Bertles J. F., Chien S. Variability of intracellular pH within individual populations of SS and AA erythrocytes. Br J Haematol. 1979 Nov;43(3):391–400. doi: 10.1111/j.1365-2141.1979.tb03766.x. [DOI] [PubMed] [Google Scholar]
- LOWRY O. H., PASSONNEAU J. V., HASSELBERGER F. X., SCHULZ D. W. EFFECT OF ISCHEMIA ON KNOWN SUBSTRATES AND COFACTORS OF THE GLYCOLYTIC PATHWAY IN BRAIN. J Biol Chem. 1964 Jan;239:18–30. [PubMed] [Google Scholar]
- Lauf P. K. Passive K+-Cl- fluxes in low-K+ sheep erythrocytes: modulation by A23187 and bivalent cations. Am J Physiol. 1985 Sep;249(3 Pt 1):C271–C278. doi: 10.1152/ajpcell.1985.249.3.C271. [DOI] [PubMed] [Google Scholar]
- Lüdi H., Schatzmann H. J. Some properties of a system for sodium-dependent outward movement of magnesium from metabolizing human red blood cells. J Physiol. 1987 Sep;390:367–382. doi: 10.1113/jphysiol.1987.sp016706. [DOI] [PMC free article] [PubMed] [Google Scholar]
- Mohandas N., Rossi M. E., Clark M. R. Association between morphologic distortion of sickle cells and deoxygenation-induced cation permeability increase. Blood. 1986 Aug;68(2):450–454. [PubMed] [Google Scholar]
- Noguchi C. T., Torchia D. A., Schechter A. N. Intracellular polymerization of sickle hemoglobin. Effects of cell heterogeneity. J Clin Invest. 1983 Sep;72(3):846–852. doi: 10.1172/JCI111055. [DOI] [PMC free article] [PubMed] [Google Scholar]
- Ortiz O. E., Lew V. L., Bookchin R. M. Calcium accumulated by sickle cell anemia red cells does not affect their potassium (86Rb+) flux components. Blood. 1986 Mar;67(3):710–715. [PubMed] [Google Scholar]
- POST R. L., JOLLY P. C. The linkage of sodium, potassium, and ammonium active transport across the human erythrocyte membrane. Biochim Biophys Acta. 1957 Jul;25(1):118–128. doi: 10.1016/0006-3002(57)90426-2. [DOI] [PubMed] [Google Scholar]
- Seakins M., Gibbs W. N., Milner P. F., Bertles J. F. Erythrocyte Hb-S concentration. An important factor in the low oxygen affinity of blood in sickle cell anemia. J Clin Invest. 1973 Feb;52(2):422–432. doi: 10.1172/JCI107199. [DOI] [PMC free article] [PubMed] [Google Scholar]
- TOSTESON D. C., SHEA E., DARLING R. C. Potassium and sodium of red blood cells in sickle cell anemia. J Clin Invest. 1952 Apr;31(4):406–411. doi: 10.1172/JCI102623. [DOI] [PMC free article] [PubMed] [Google Scholar]
- Van Beek G. G., De Bruin S. H. The pH dependence of the binding of D-glycerate 2,3-bisphosphate to deoxyhemoglobin and oxyhemoglobin. Determination of the number of binding sites in oxyhemoglobin. Eur J Biochem. 1979 Oct 15;100(2):497–502. doi: 10.1111/j.1432-1033.1979.tb04194.x. [DOI] [PubMed] [Google Scholar]
- WALSER M. Ion association. VI. Interactions between calcium, magnesium, inorganic phosphate, citrate and protein in normal human plasma. J Clin Invest. 1961 Apr;40:723–730. doi: 10.1172/JCI104306. [DOI] [PMC free article] [PubMed] [Google Scholar]
- Watson W. S., Lyon T. D., Hilditch T. E. Red cell magnesium as a function of cell age. Metabolism. 1980 May;29(5):397–399. doi: 10.1016/0026-0495(80)90162-6. [DOI] [PubMed] [Google Scholar]