Abstract
1. Extracellular pH (pHo) was measured in the isolated retina preparation of the toad, Bufo marinus, using H(+)-selective microelectrodes. During superfusion with phosphate-buffered solution (pH 7.8), which had a low buffering capacity, pHo in the inner retina was 7.0-7.2 and there was a pHo gradient throughout the distal retina and into the bathing solution. 2. The retinal acidity appears to be due in part to the combined reactions of glycolysis and ATP hydrolysis, since anoxia greatly increased the pHo gradient, while superfusion with either glucose-free pyruvate solution or strophanthidin decreased this gradient. 3. Maintained illumination evoked both an acidification in the proximal retina and an alkalinization in the distal retina. Blocking synaptic transmission to second-order neurones (1.0 mM-aspartate) decreased the acidification but had little effect on the alkalinization, consistent with the notion that the alkalinization is of receptoral origin, while the acidification is of post-receptoral origin. 4. Retinal neurones extrude a significant amount of acid via Na(+)-H+ exchange, since 2.0 mM-amiloride, a blocker of Na(+)-H+ exchange, caused a sustained alkalinization in darkness and decreased the light-evoked changes in pHo, while 1.0 mM-4-acetamido-4'-isothiocyanatostilbene-2.2'-disulphonic acid (SITS), a blocker of Cl(-)-HCO3- exchange, produced a much smaller alkalinization. 5. Switching to a bicarbonate-buffered solution having a 75 times greater buffering capacity than the phosphate-buffered solution caused retinal pHo to become less acidic and significantly decreased the amplitude of the light-evoked pHo changes. 6. Addition of 2.0 mM-acetazolamide, a carbonic anhydrase inhibitor, to the bicarbonate-buffered solution increased both the pHo gradient and the light-evoked changes in pHo. These data are consistent with the idea that carbonic anhydrase, which is concentrated in Müller (glial) cells and to a lesser extent in horizontal cells, increases the effectiveness of the bicarbonate buffer system. 7. Switching from bicarbonate-buffered to phosphate-buffered solutions attenuated the b-wave of the electroretinogram, most likely by acidifying pHo. Overall, our results emphasize the importance of the bicarbonate buffer system in buffering pHo during periods of variable acid extrusion in light and in darkness.
Full text
PDF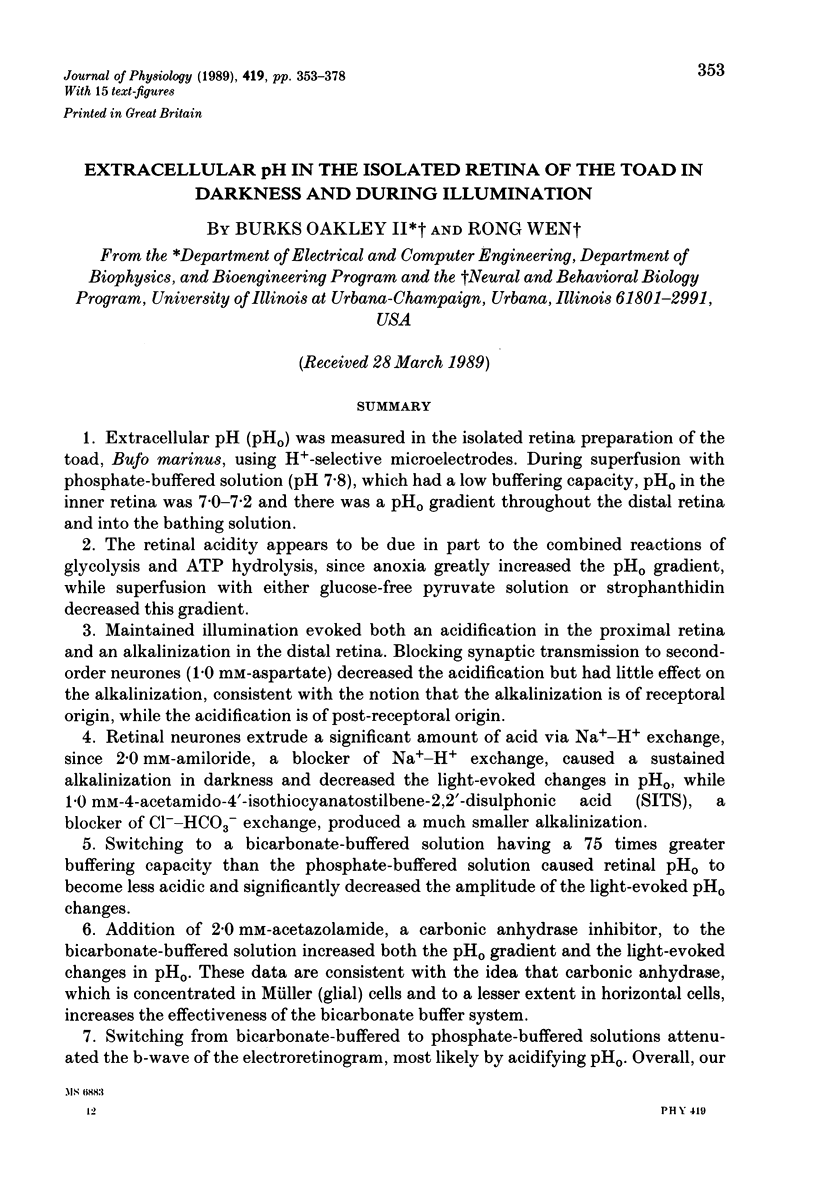
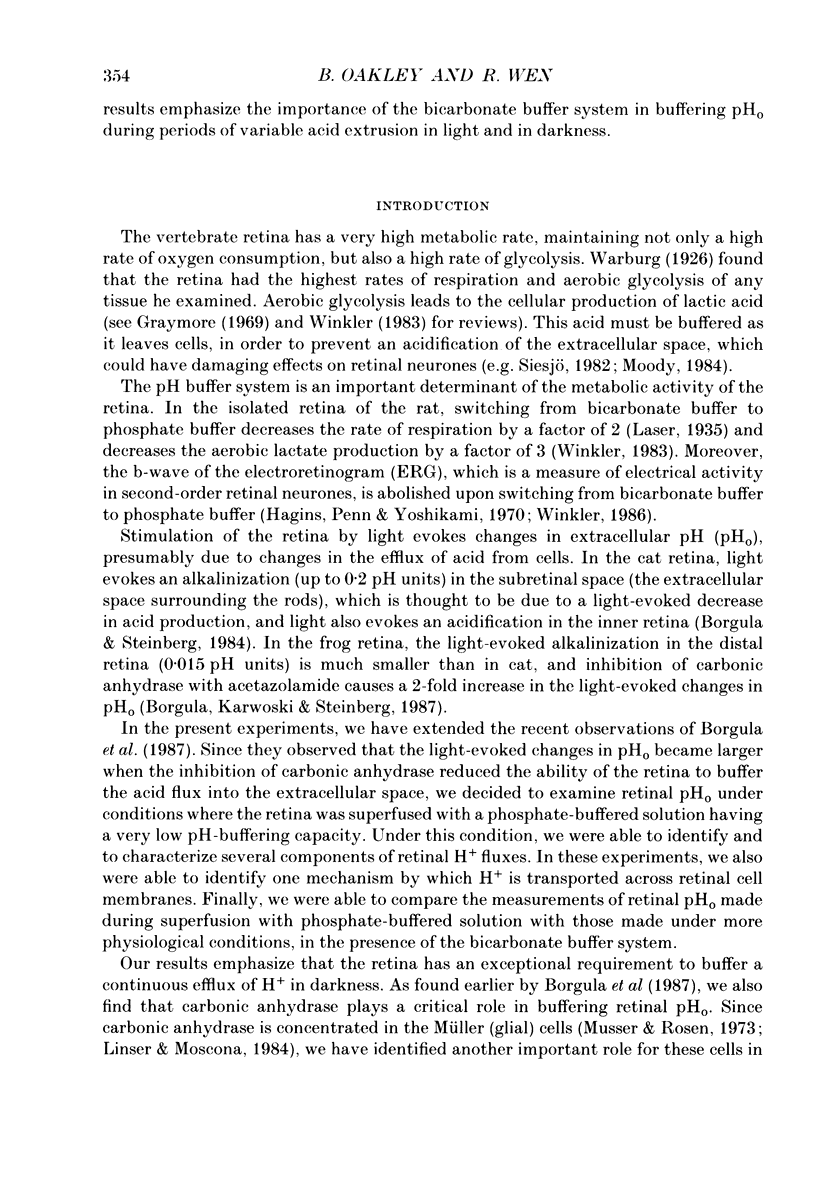
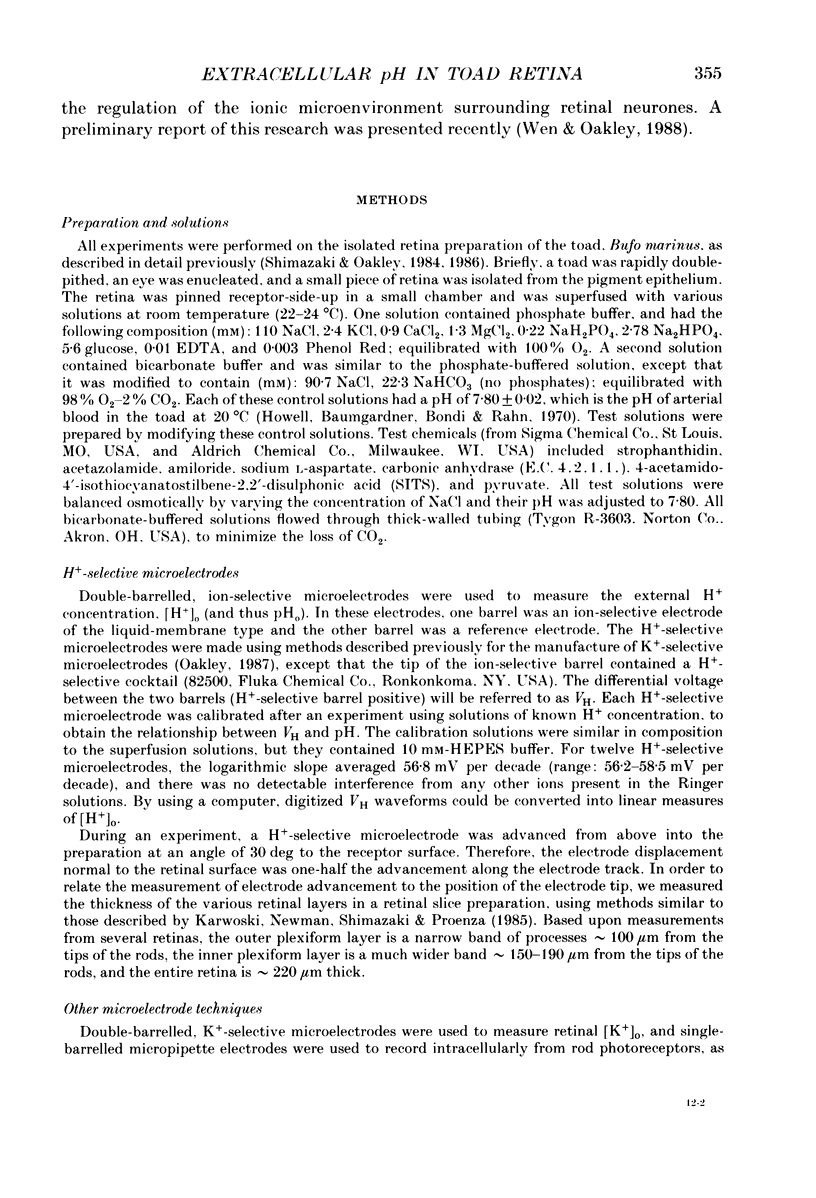
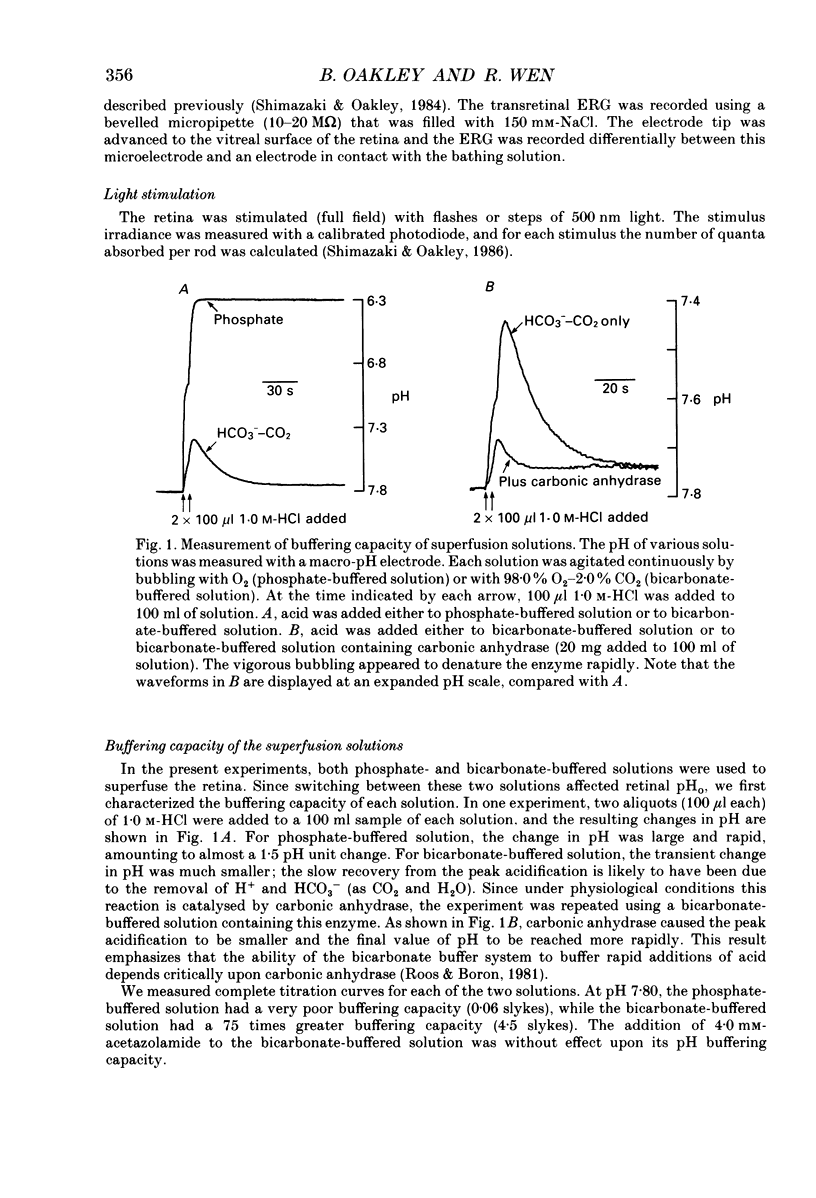
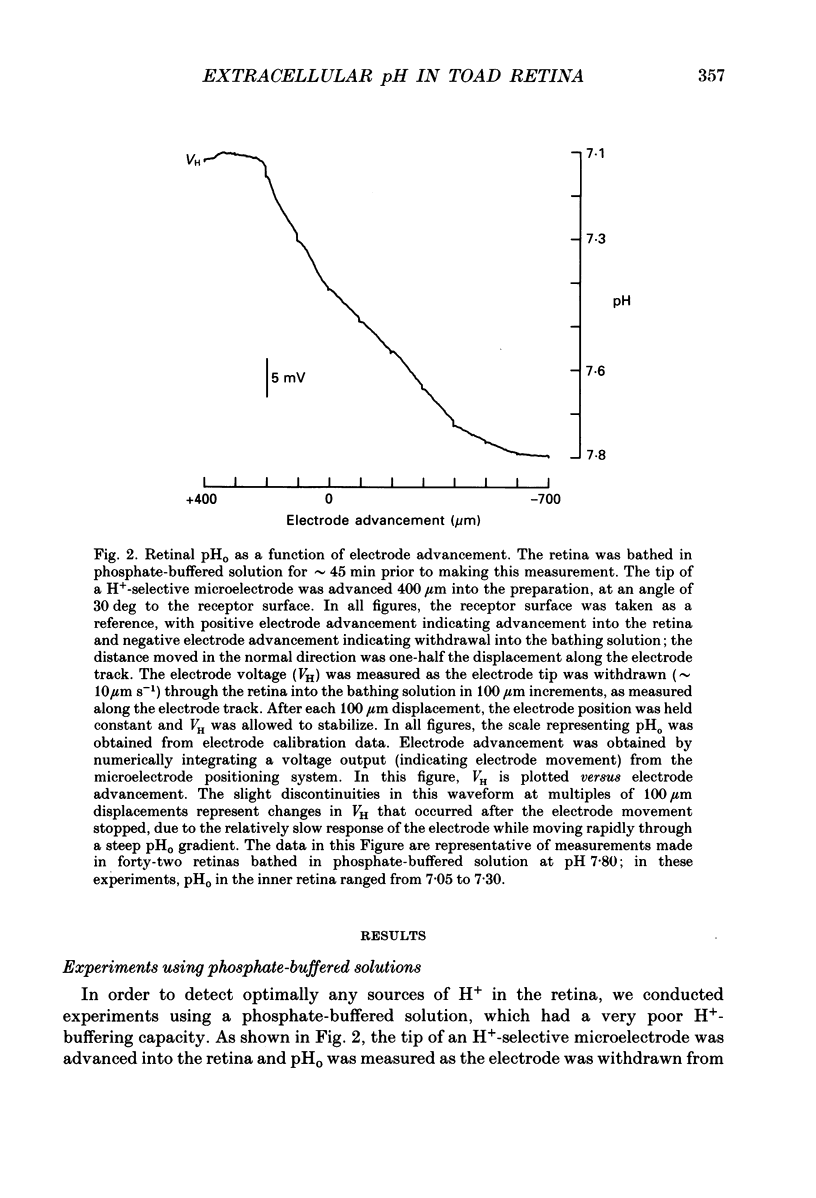
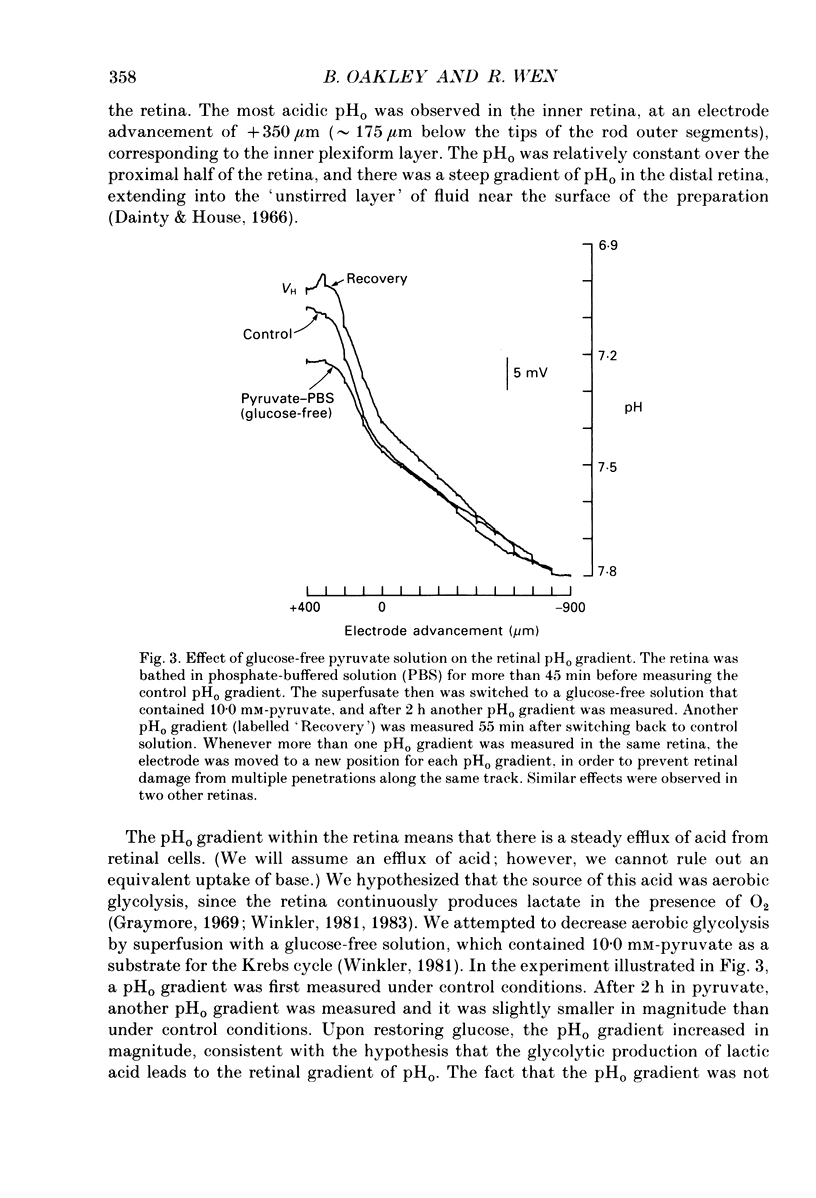
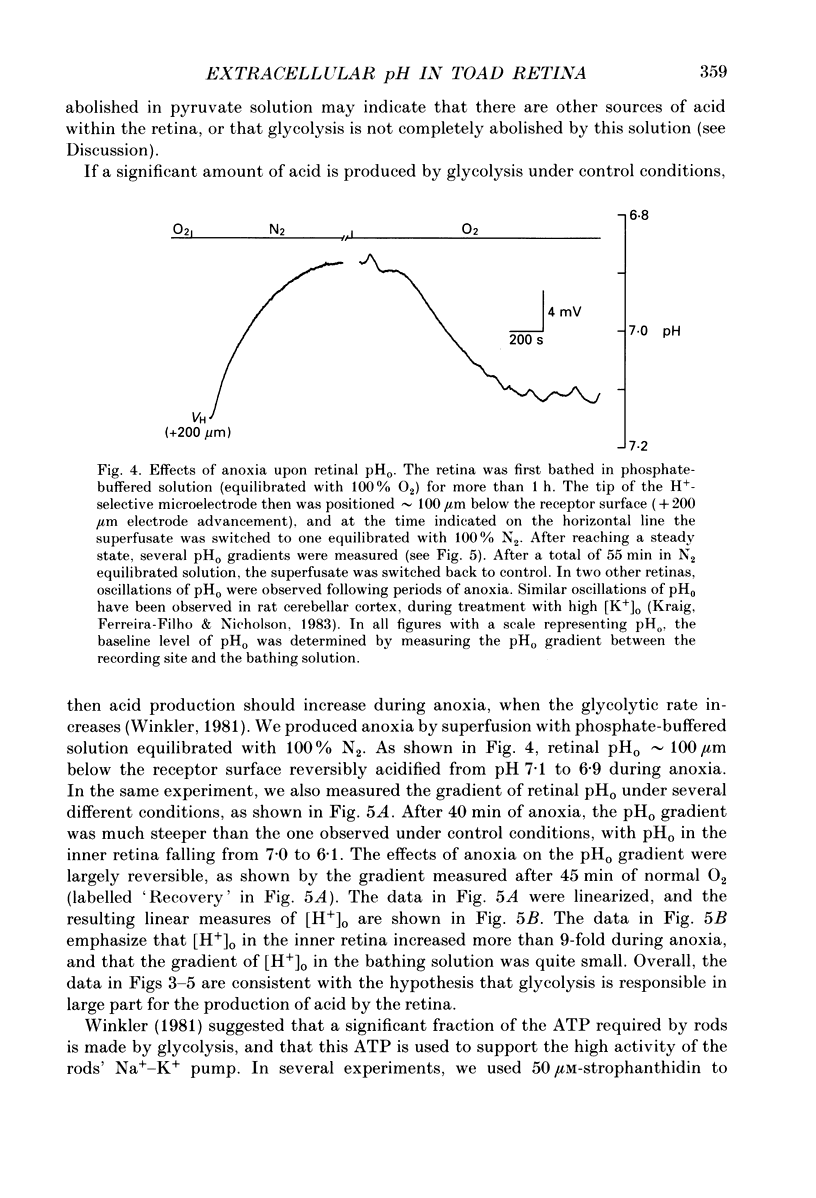
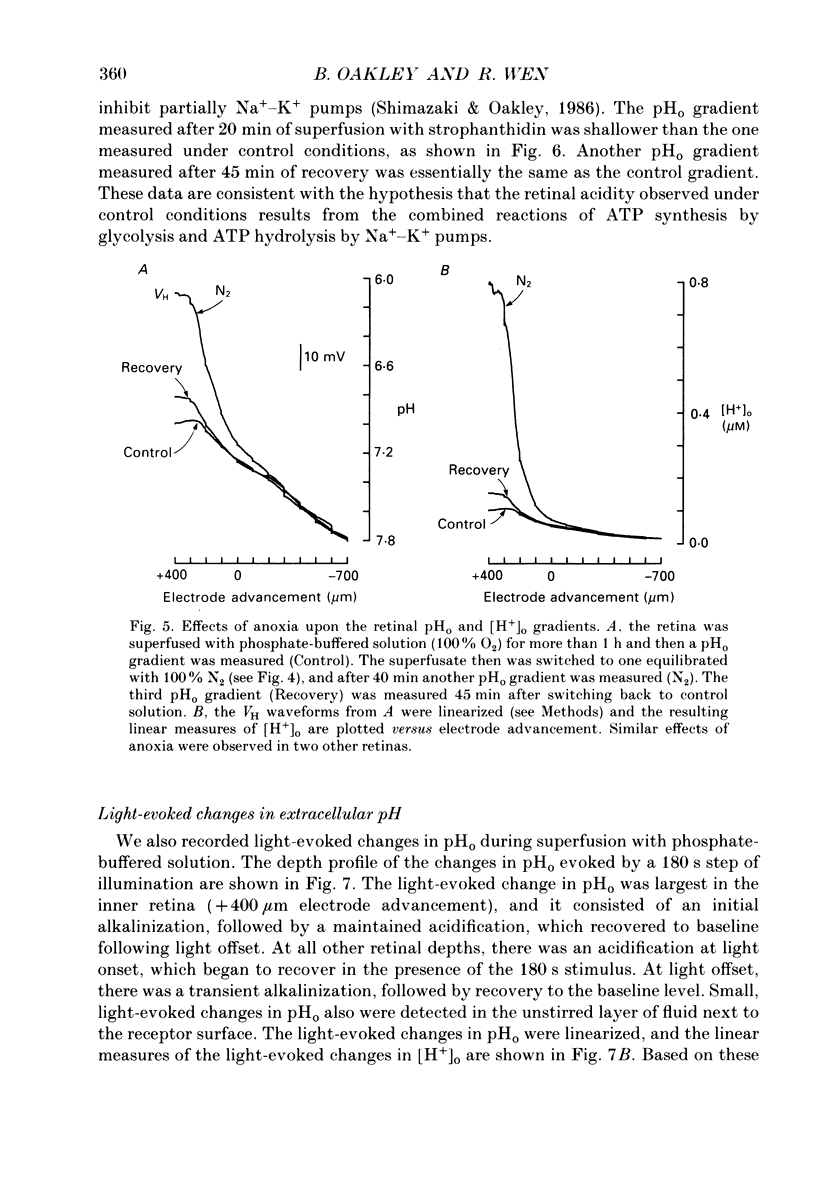
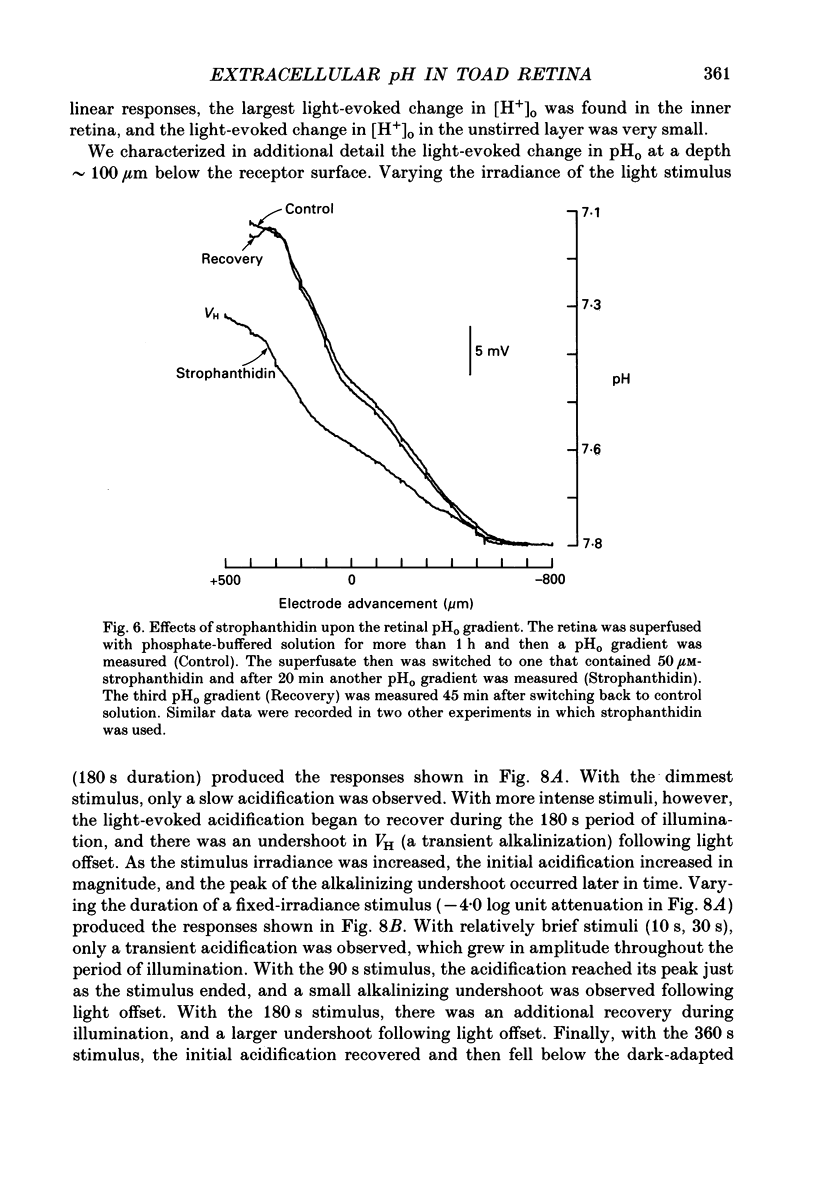
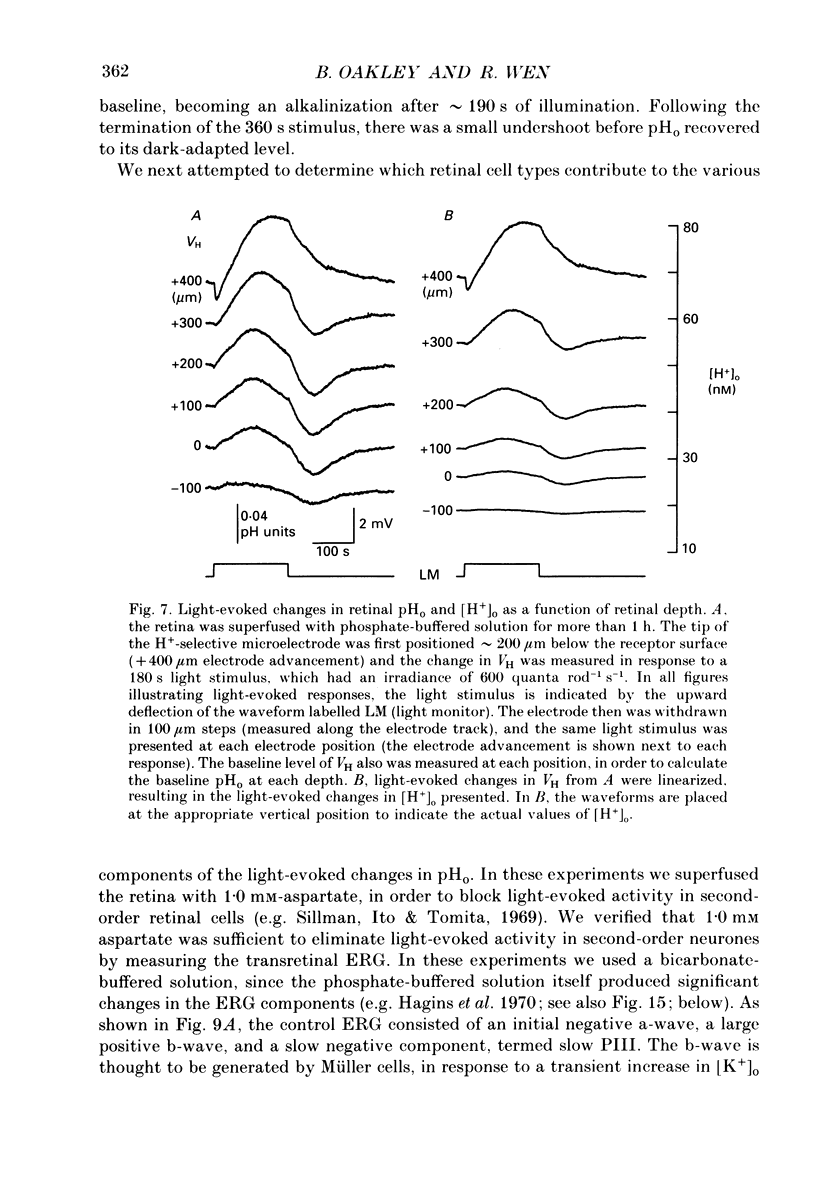
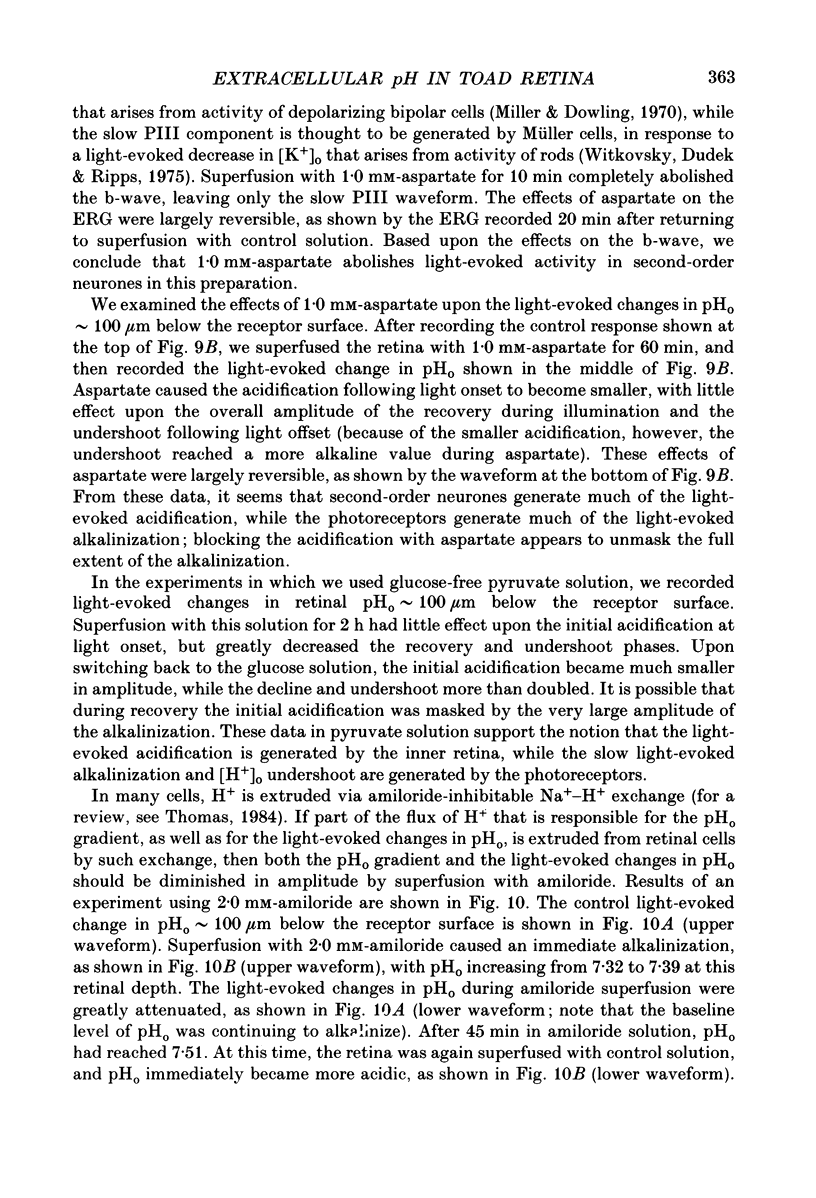
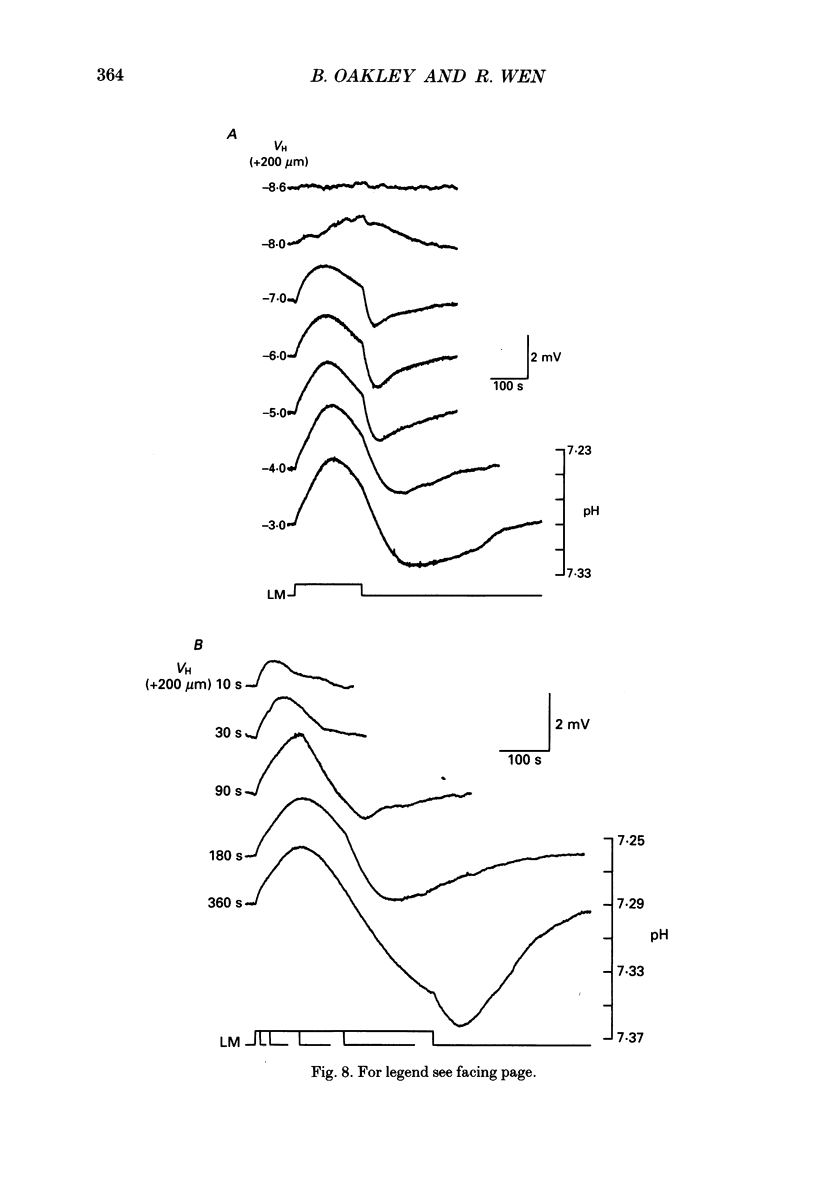
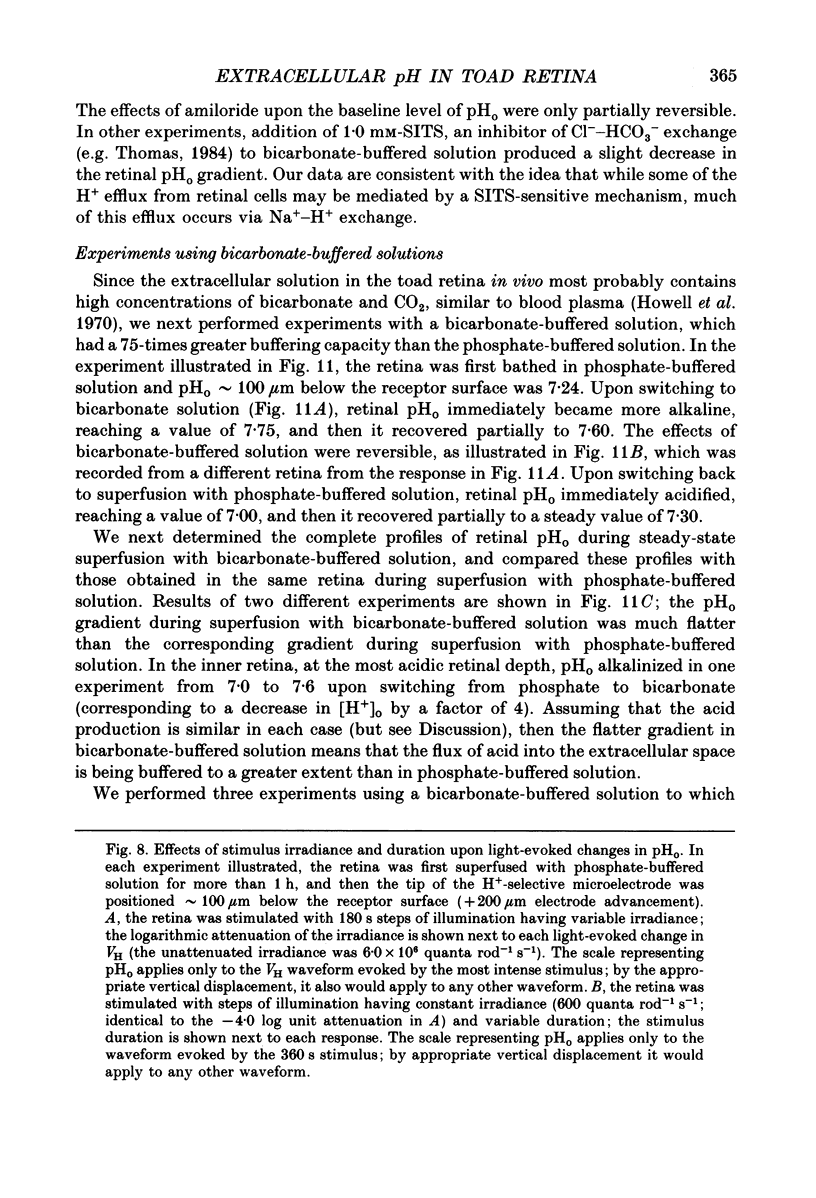
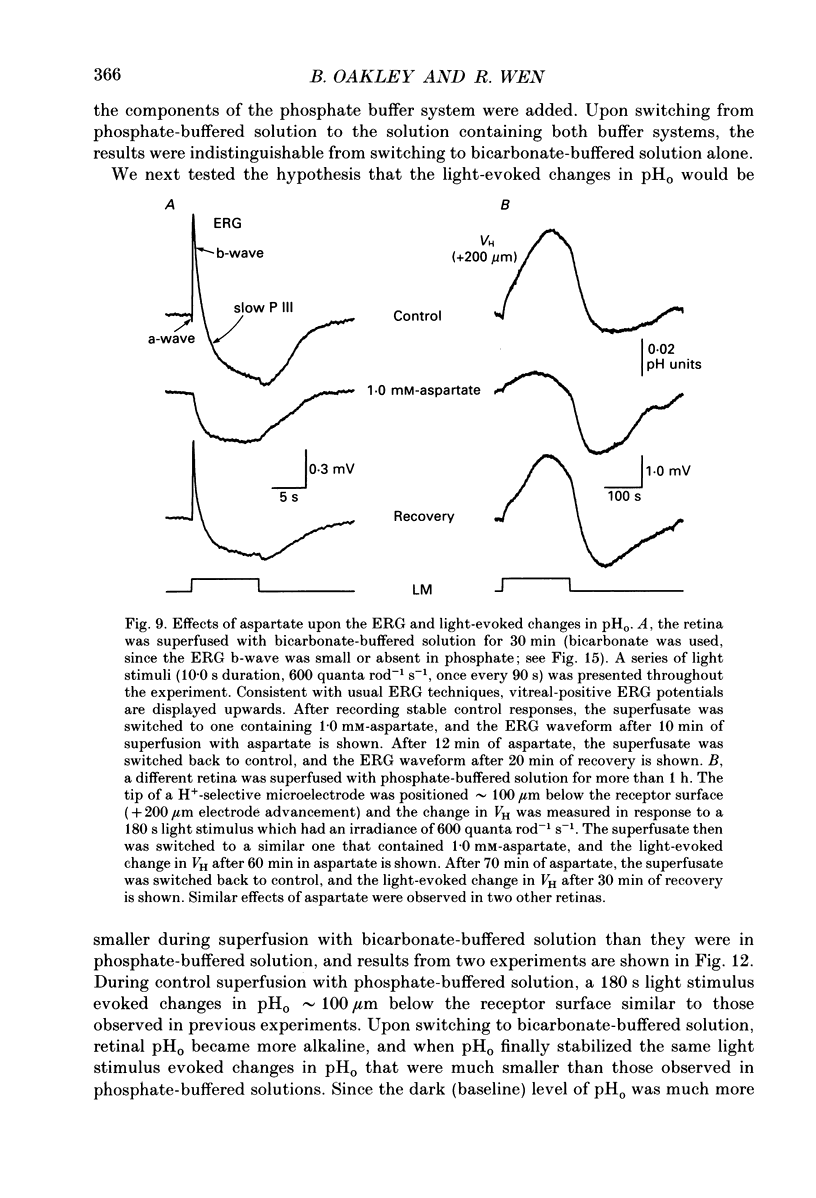
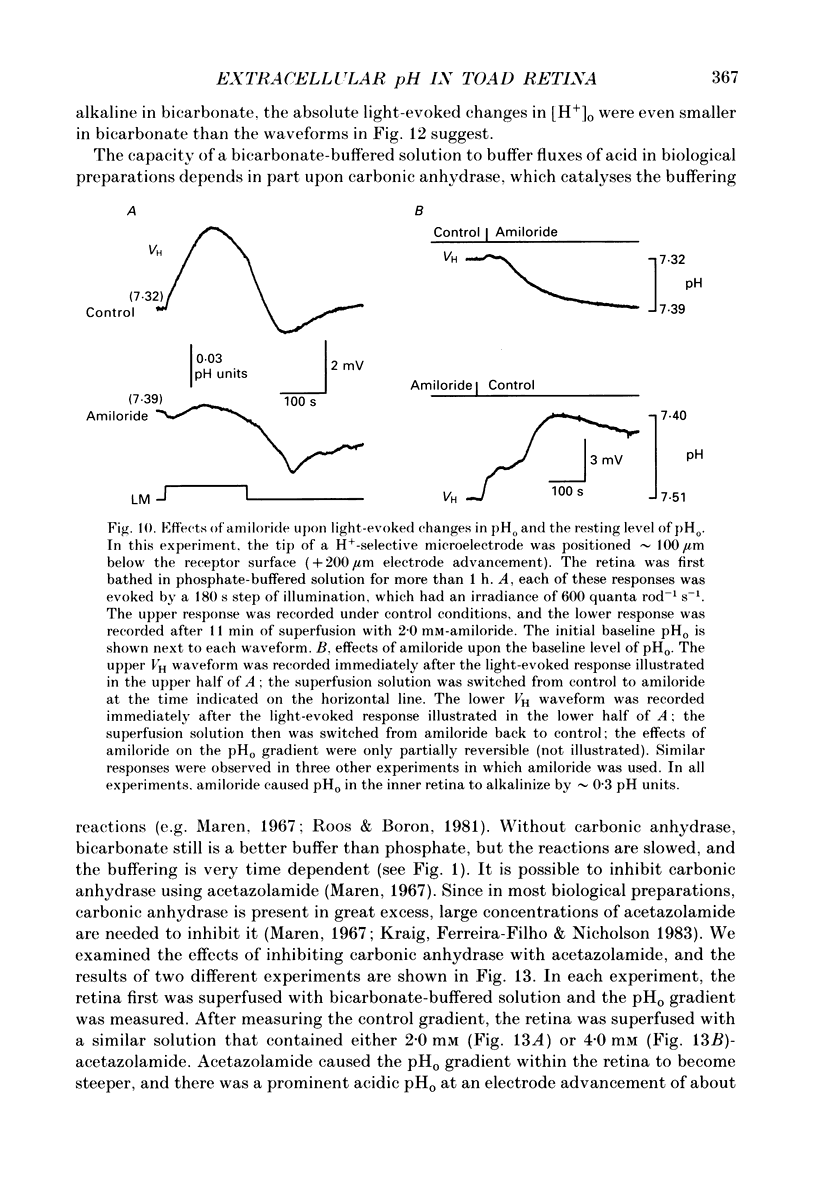
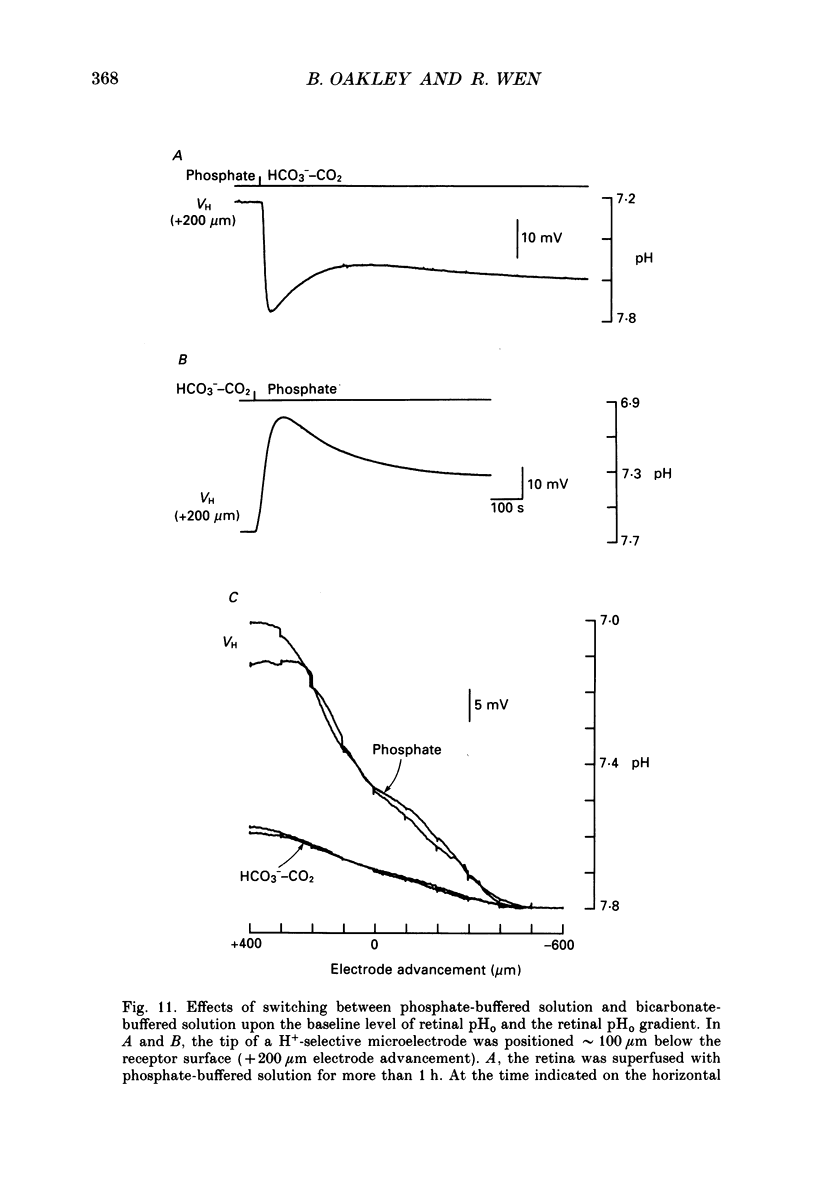
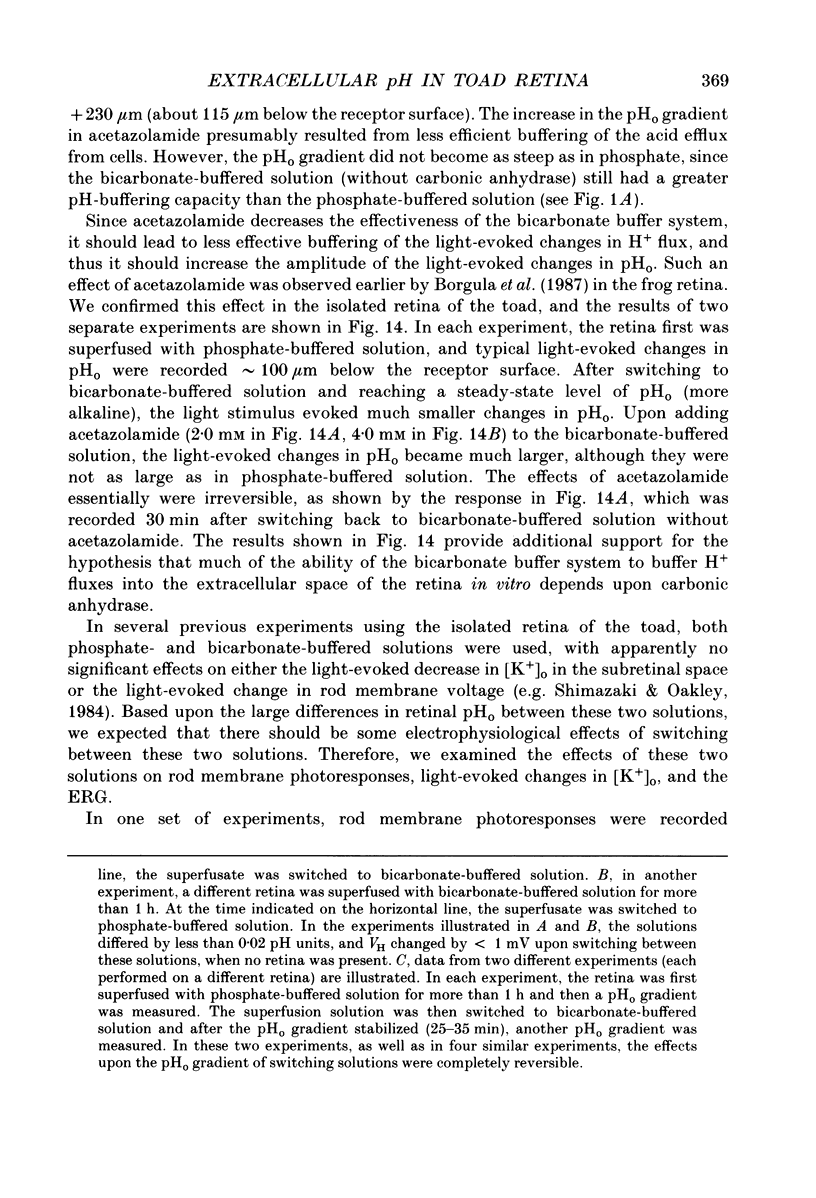
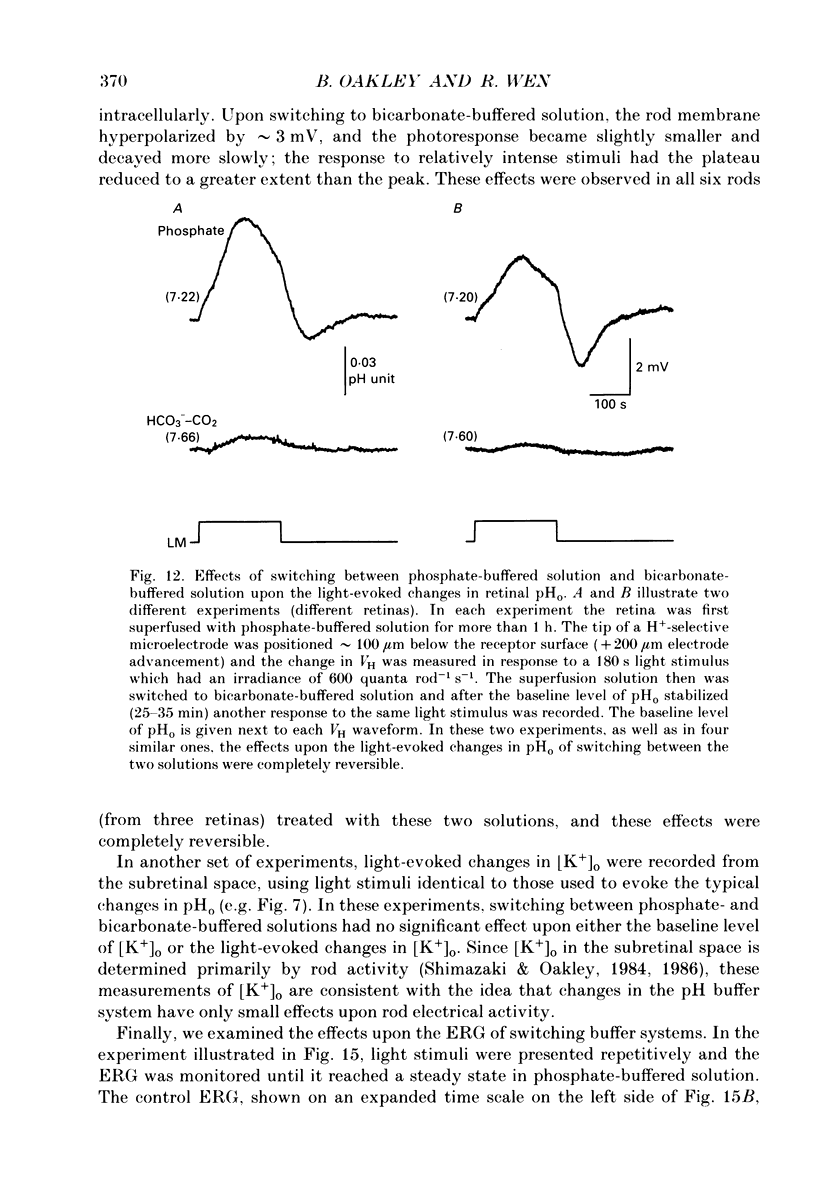
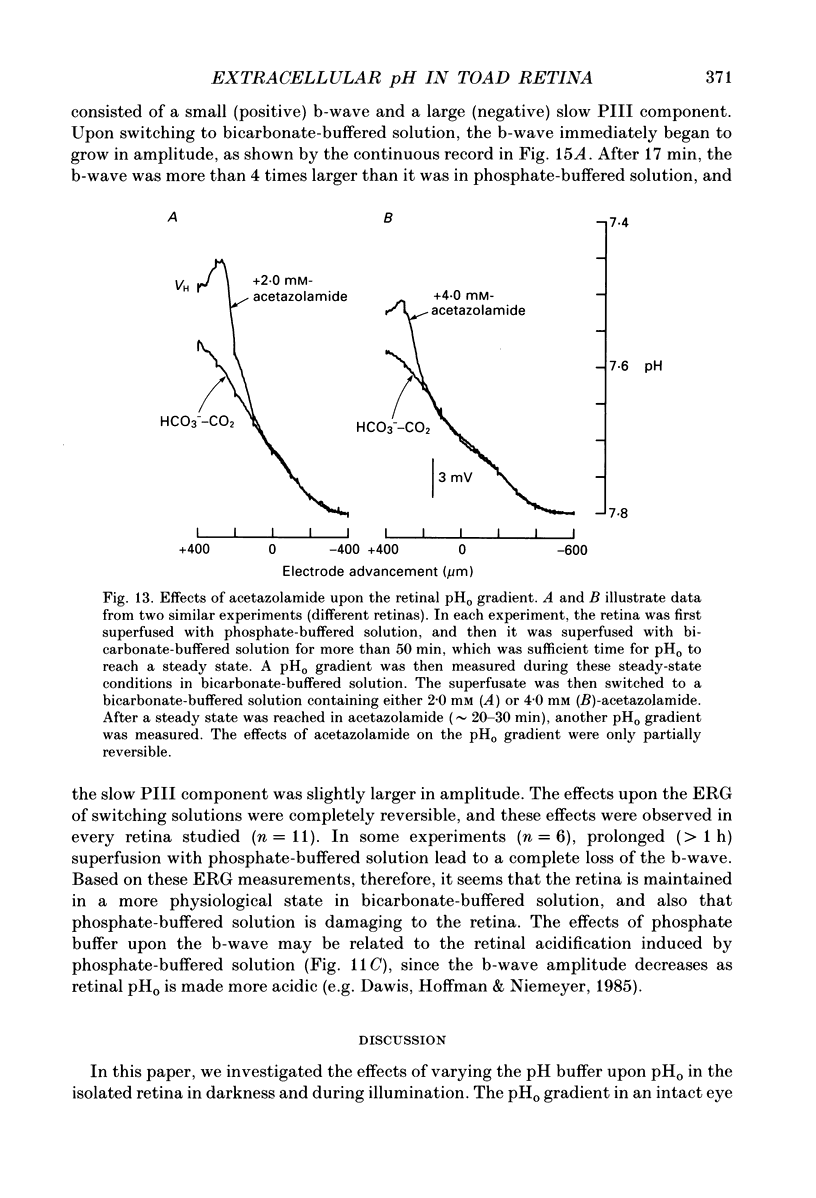
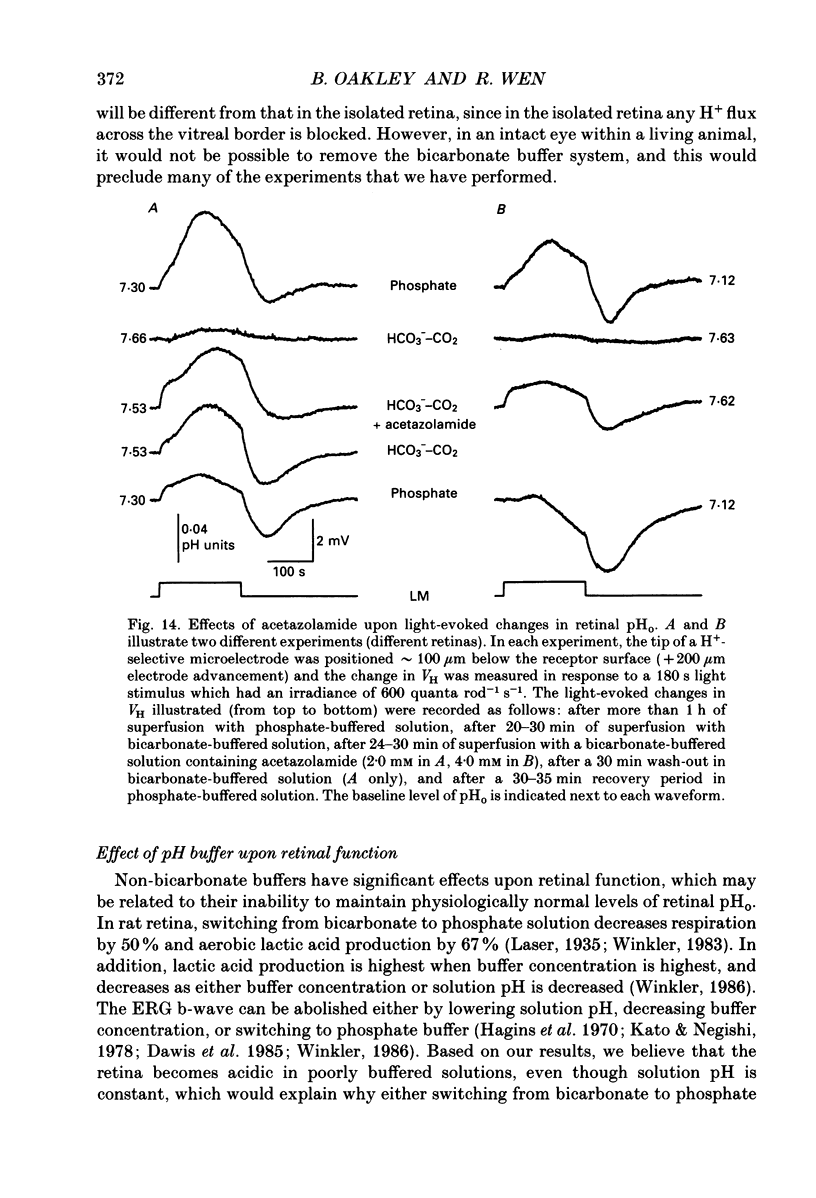
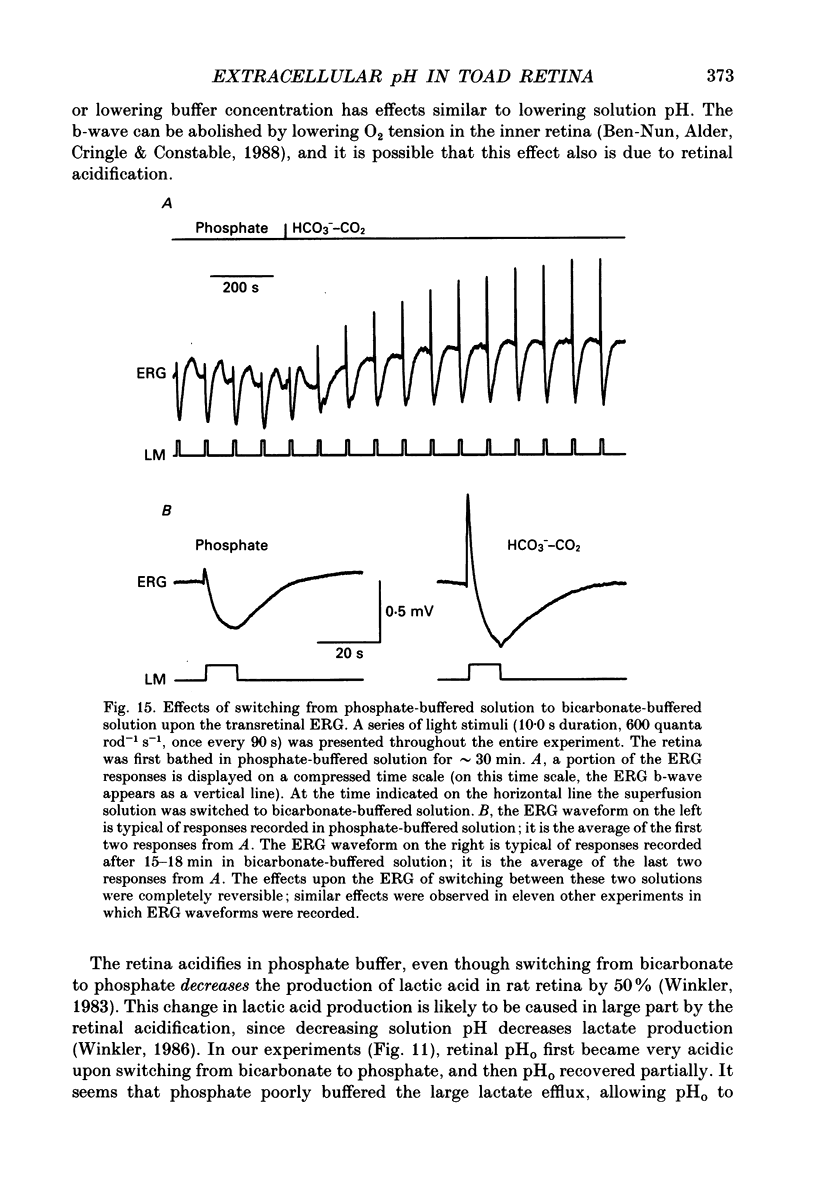
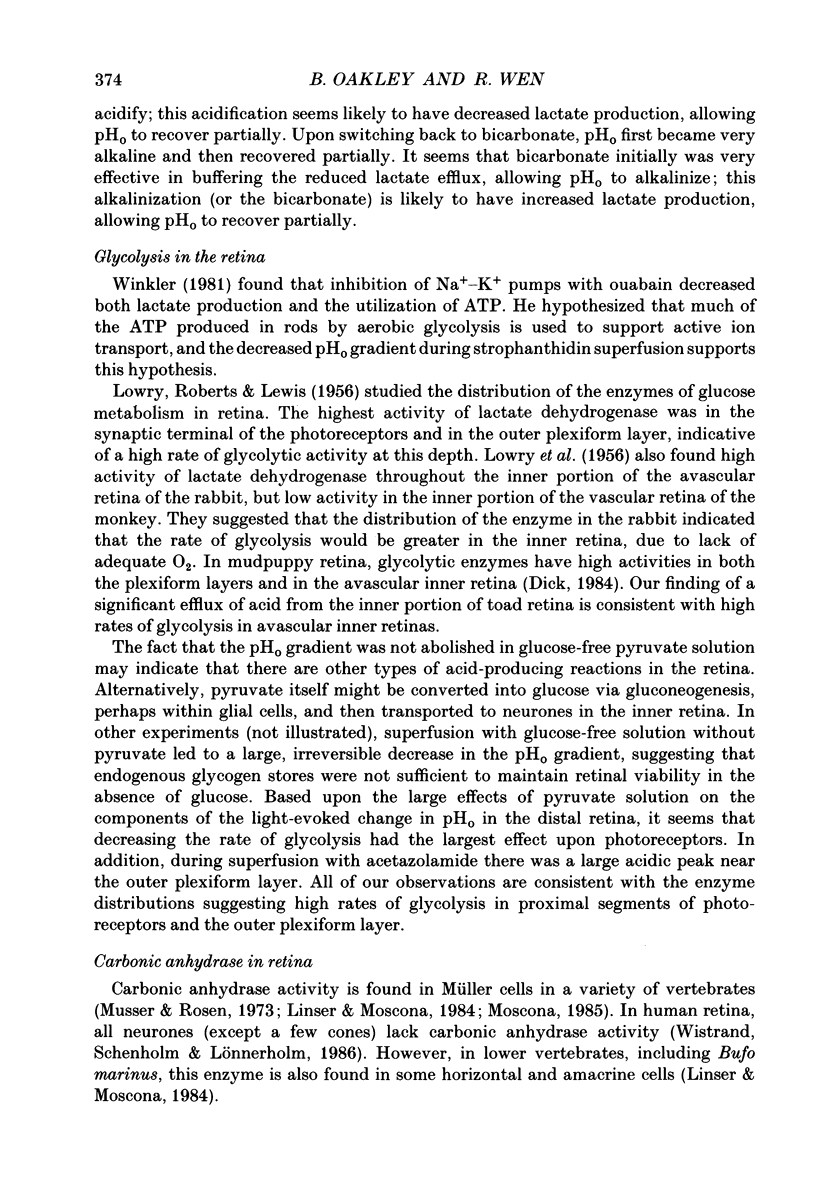
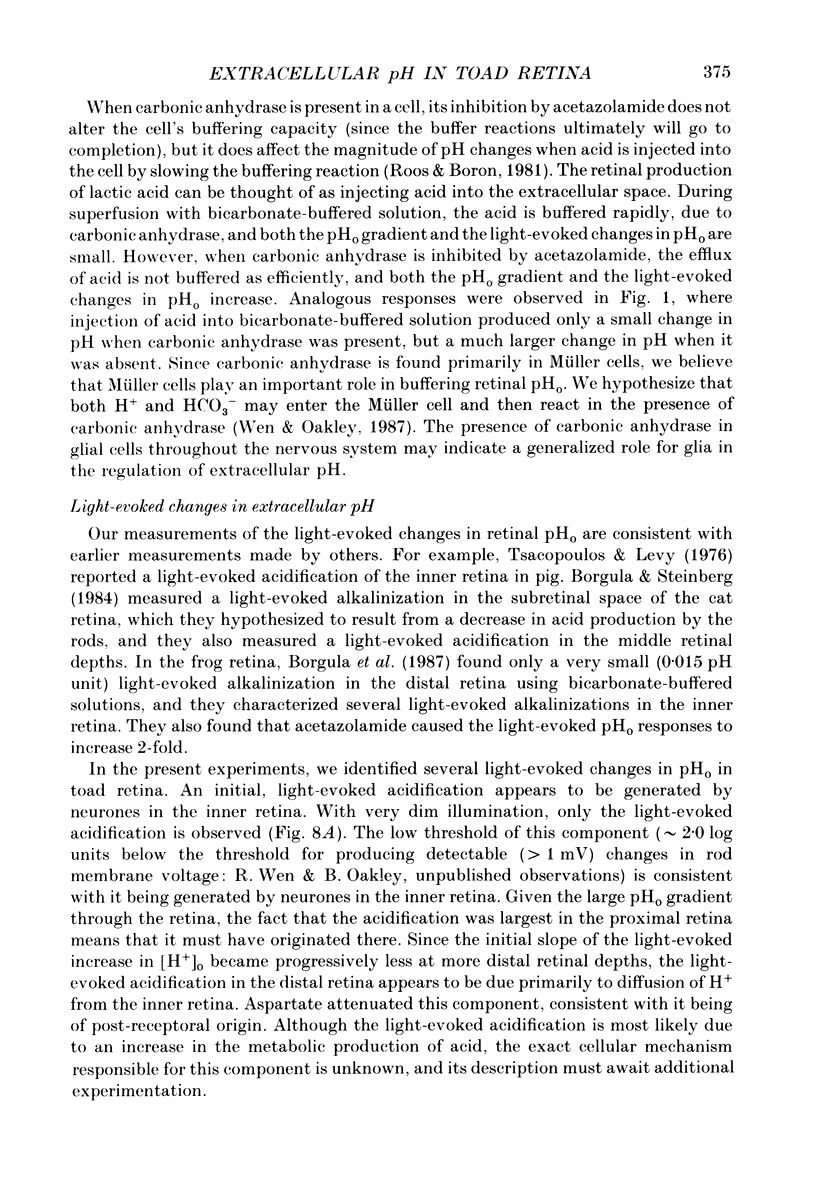
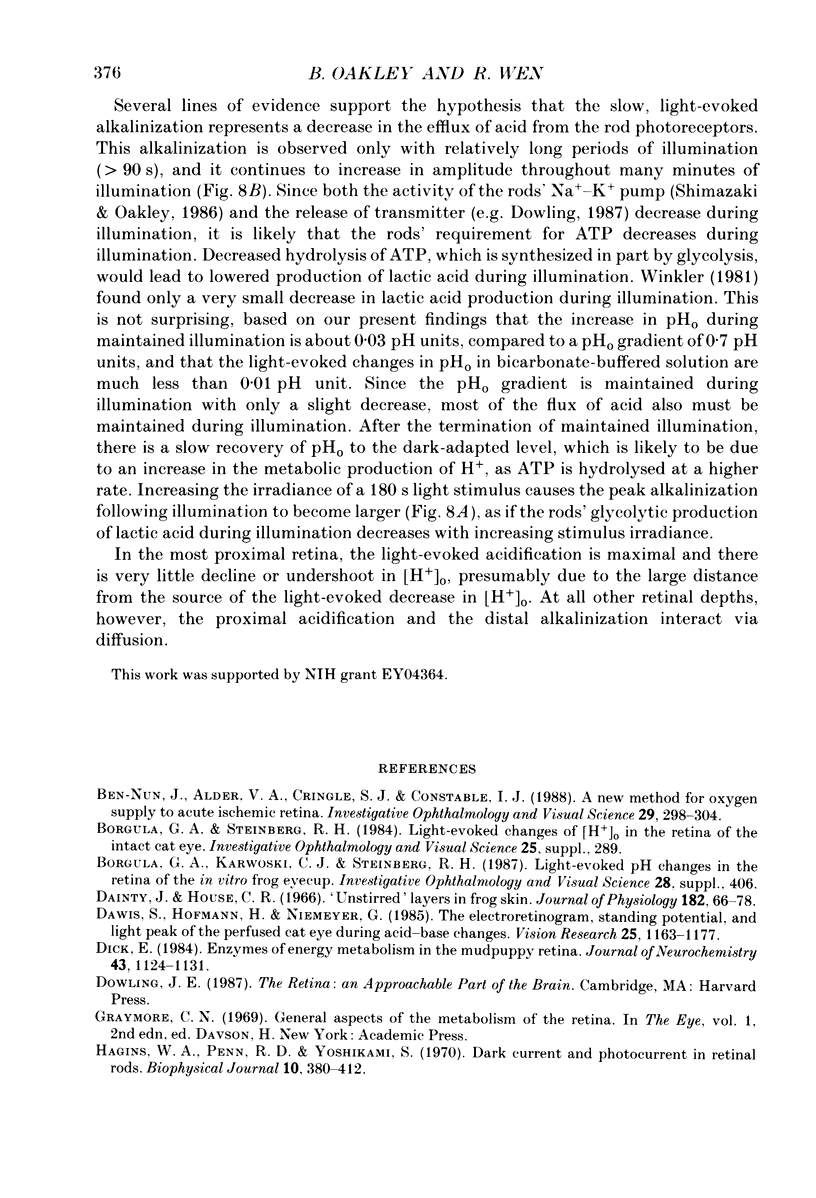
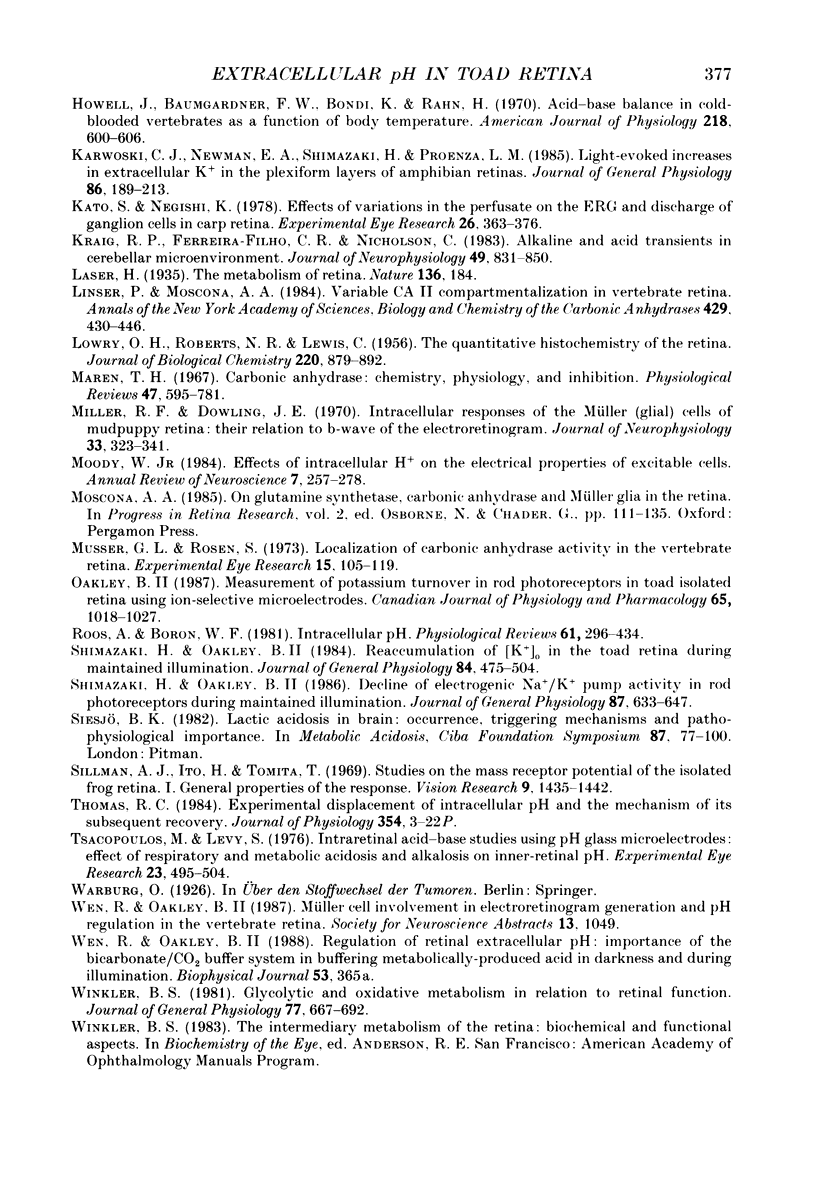
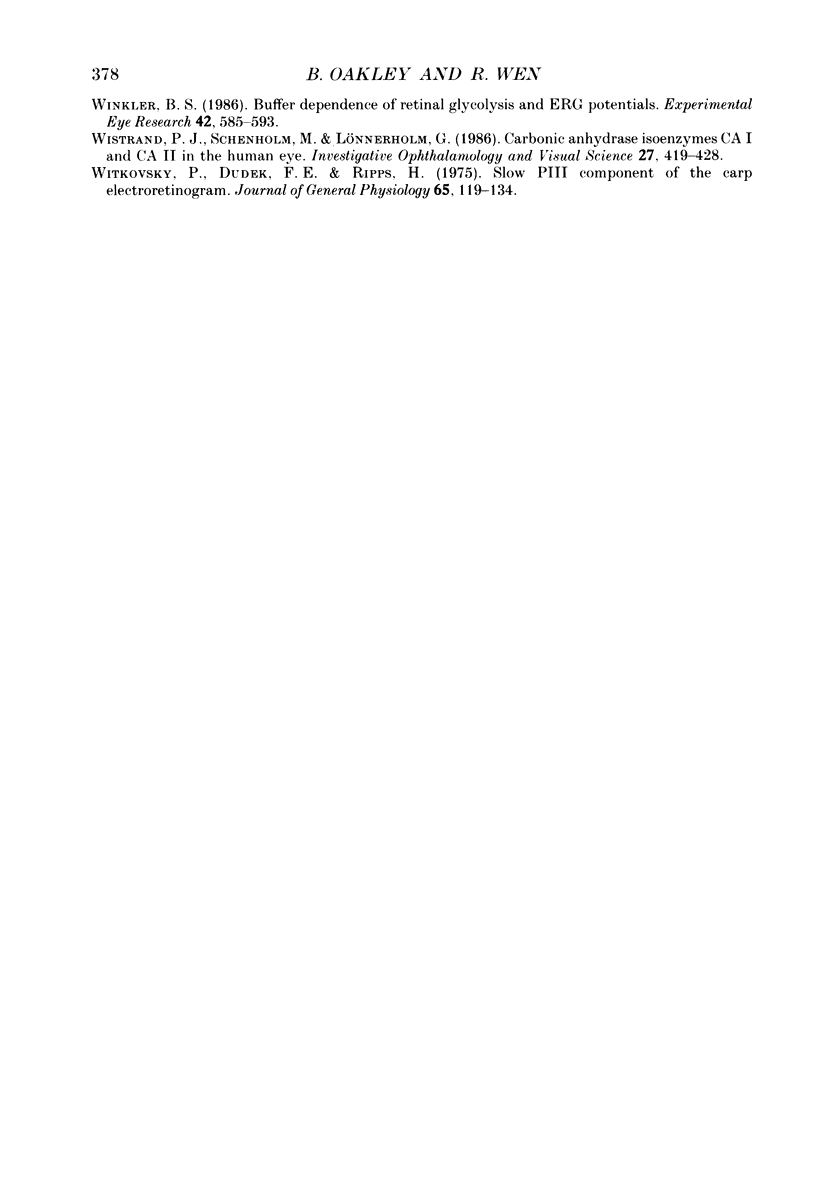
Selected References
These references are in PubMed. This may not be the complete list of references from this article.
- Ben-Nun J., Alder V. A., Cringle S. J., Constable I. J. A new method for oxygen supply to acute ischemic retina. Invest Ophthalmol Vis Sci. 1988 Feb;29(2):298–304. [PubMed] [Google Scholar]
- Dainty J., House C. R. Unstirred layers in frog skin. J Physiol. 1966 Jan;182(1):66–78. doi: 10.1113/jphysiol.1966.sp007809. [DOI] [PMC free article] [PubMed] [Google Scholar]
- Dawis S., Hofmann H., Niemeyer G. The electroretinogram, standing potential, and light peak of the perfused cat eye during acid-base changes. Vision Res. 1985;25(9):1163–1177. doi: 10.1016/0042-6989(85)90030-6. [DOI] [PubMed] [Google Scholar]
- Dick E. Enzymes of energy metabolism in the mudpuppy retina. J Neurochem. 1984 Oct;43(4):1124–1131. doi: 10.1111/j.1471-4159.1984.tb12852.x. [DOI] [PubMed] [Google Scholar]
- Hagins W. A., Penn R. D., Yoshikami S. Dark current and photocurrent in retinal rods. Biophys J. 1970 May;10(5):380–412. doi: 10.1016/S0006-3495(70)86308-1. [DOI] [PMC free article] [PubMed] [Google Scholar]
- Howell B. J., Baumgardner F. W., Bondi K., Rahn H. Acid-base balance in cold-blooded vertebrates as a function of body temperature. Am J Physiol. 1970 Feb;218(2):600–606. doi: 10.1152/ajplegacy.1970.218.2.600. [DOI] [PubMed] [Google Scholar]
- Karwoski C. J., Newman E. A., Shimazaki H., Proenza L. M. Light-evoked increases in extracellular K+ in the plexiform layers of amphibian retinas. J Gen Physiol. 1985 Aug;86(2):189–213. doi: 10.1085/jgp.86.2.189. [DOI] [PMC free article] [PubMed] [Google Scholar]
- Kato S., Negishi K. Effects of variations in the perfusate on the ERG and discharge of ganglion cells in carp retina. Exp Eye Res. 1978 Apr;26(4):363–376. doi: 10.1016/0014-4835(78)90124-0. [DOI] [PubMed] [Google Scholar]
- Kraig R. P., Ferreira-Filho C. R., Nicholson C. Alkaline and acid transients in cerebellar microenvironment. J Neurophysiol. 1983 Mar;49(3):831–850. doi: 10.1152/jn.1983.49.3.831. [DOI] [PubMed] [Google Scholar]
- LOWRY O. H., ROBERTS N. R., LEWIS C. The quantitative histochemistry of the retina. J Biol Chem. 1956 Jun;220(2):879–892. [PubMed] [Google Scholar]
- Maren T. H. Carbonic anhydrase: chemistry, physiology, and inhibition. Physiol Rev. 1967 Oct;47(4):595–781. doi: 10.1152/physrev.1967.47.4.595. [DOI] [PubMed] [Google Scholar]
- Miller R. F., Dowling J. E. Intracellular responses of the Müller (glial) cells of mudpuppy retina: their relation to b-wave of the electroretinogram. J Neurophysiol. 1970 May;33(3):323–341. doi: 10.1152/jn.1970.33.3.323. [DOI] [PubMed] [Google Scholar]
- Moody W., Jr Effects of intracellular H+ on the electrical properties of excitable cells. Annu Rev Neurosci. 1984;7:257–278. doi: 10.1146/annurev.ne.07.030184.001353. [DOI] [PubMed] [Google Scholar]
- Musser G. L., Rosen S. Localization of carbonic anhydrase activity in the vertebrate retina. Exp Eye Res. 1973 Jan 1;15(1):105–119. doi: 10.1016/0014-4835(73)90195-4. [DOI] [PubMed] [Google Scholar]
- Oakley B., 2nd Measurement of potassium turnover in rod photoreceptors in toad isolated retina using ion-selective microelectrodes. Can J Physiol Pharmacol. 1987 May;65(5):1018–1027. doi: 10.1139/y87-161. [DOI] [PubMed] [Google Scholar]
- Roos A., Boron W. F. Intracellular pH. Physiol Rev. 1981 Apr;61(2):296–434. doi: 10.1152/physrev.1981.61.2.296. [DOI] [PubMed] [Google Scholar]
- Shimazaki H., Oakley B., 2nd Decline of electrogenic Na+/K+ pump activity in rod photoreceptors during maintained illumination. J Gen Physiol. 1986 Apr;87(4):633–647. doi: 10.1085/jgp.87.4.633. [DOI] [PMC free article] [PubMed] [Google Scholar]
- Shimazaki H., Oakley B., 2nd Reaccumulation of [K+]o in the toad retina during maintained illumination. J Gen Physiol. 1984 Sep;84(3):475–504. doi: 10.1085/jgp.84.3.475. [DOI] [PMC free article] [PubMed] [Google Scholar]
- Siesjö B. K. Lactic acidosis in the brain: occurrence, triggering mechanisms and pathophysiological importance. Ciba Found Symp. 1982;87:77–100. doi: 10.1002/9780470720691.ch5. [DOI] [PubMed] [Google Scholar]
- Sillman A. J., Ito H., Tomita T. Studies on the mass receptor potential of the isolated frog retina. I. General properties of the response. Vision Res. 1969 Dec;9(12):1435–1442. doi: 10.1016/0042-6989(69)90059-5. [DOI] [PubMed] [Google Scholar]
- Thomas R. C. Experimental displacement of intracellular pH and the mechanism of its subsequent recovery. J Physiol. 1984 Sep;354:3P–22P. doi: 10.1113/jphysiol.1984.sp015397. [DOI] [PMC free article] [PubMed] [Google Scholar]
- Tsacopoulos M., Levy S. Intraretinal acid-base studies using pH glass microelectrodes: effect of respiratory and metabolic acidosis and alkalosis on inner-retinal pH. Exp Eye Res. 1976 Nov;23(5):495–504. doi: 10.1016/0014-4835(76)90158-5. [DOI] [PubMed] [Google Scholar]
- Winkler B. S. Buffer dependence of retinal glycolysis and ERG potentials. Exp Eye Res. 1986 Jun;42(6):585–593. doi: 10.1016/0014-4835(86)90048-5. [DOI] [PubMed] [Google Scholar]
- Winkler B. S. Glycolytic and oxidative metabolism in relation to retinal function. J Gen Physiol. 1981 Jun;77(6):667–692. doi: 10.1085/jgp.77.6.667. [DOI] [PMC free article] [PubMed] [Google Scholar]
- Wistrand P. J., Schenholm M., Lönnerholm G. Carbonic anhydrase isoenzymes CA I and CA II in the human eye. Invest Ophthalmol Vis Sci. 1986 Mar;27(3):419–428. [PubMed] [Google Scholar]
- Witkovsky P., Dudek F. E., Ripps H. Slow PIII component of the carp electroretinogram. J Gen Physiol. 1975 Feb;65(2):119–134. doi: 10.1085/jgp.65.2.119. [DOI] [PMC free article] [PubMed] [Google Scholar]