Abstract
1. We have measured the intracellular pH (pHi) and membrane potential of identified glial cells in the central nervous system of the leech, Hirudo medicinalis, using double-barrelled pH-sensitive microelectrodes. 2. When extracellular K+ concentration was increased, the glial membrane potential decreased and pHi increased; lowering the extracellular K+ concentration hyperpolarized the glial membrane and decreased pHi. These pHi changes were largely dependent upon the presence of CO2-HCO3-; in nominally CO2-HCO3(-)-free saline solution, they were 50-80% smaller. 3. The steady-state pHi of the glial cells in CO2-HCO3(-)-buffered saline solution strongly correlated with the membrane potential between -40 and -90 mV. The slope of this relationship was 60 mV/pH unit. 4. The neurotransmitter 5-hydroxytryptamine (50 microM), which hyperpolarizes the glial membrane, also produced a large, CO2-HCO3(-)-dependent decrease in pHi. The size of the pHi change depended upon the amplitude of the membrane hyperpolarization. 5. The increase in pHi produced by the membrane depolarization in 20 mM-K+ was abolished in Na(+)-free saline. Removal of external Na+ in the presence of 20 mM-K+ reversed the pHi increase. 6. The pHi increase in 20 mM-K+ was also inhibited by the stilbene 4,4-diisothiocyanostilbene-2'-disulphonic acid (DIDS, 0.5 mM). In a DIDS-poisoned preparation a small decrease of pHi was observed in 20 mM-K+ both in the presence and nominal absence of CO2-HCO3-. 7. In neurones, neither CO2-HCO3- nor 20 mM-K+ produced an intracellular alkanization. The steady-state pHi of several identified neurones was not correlated with the membrane potential. 8. We conclude that in glial cells, but not in neurones, the pHi is dependent upon the membrane potential. This membrane potential dependence is due to the activity of the electrogenic Na(+)-HCO3- co-transporter in the glial cell membrane.
Full text
PDF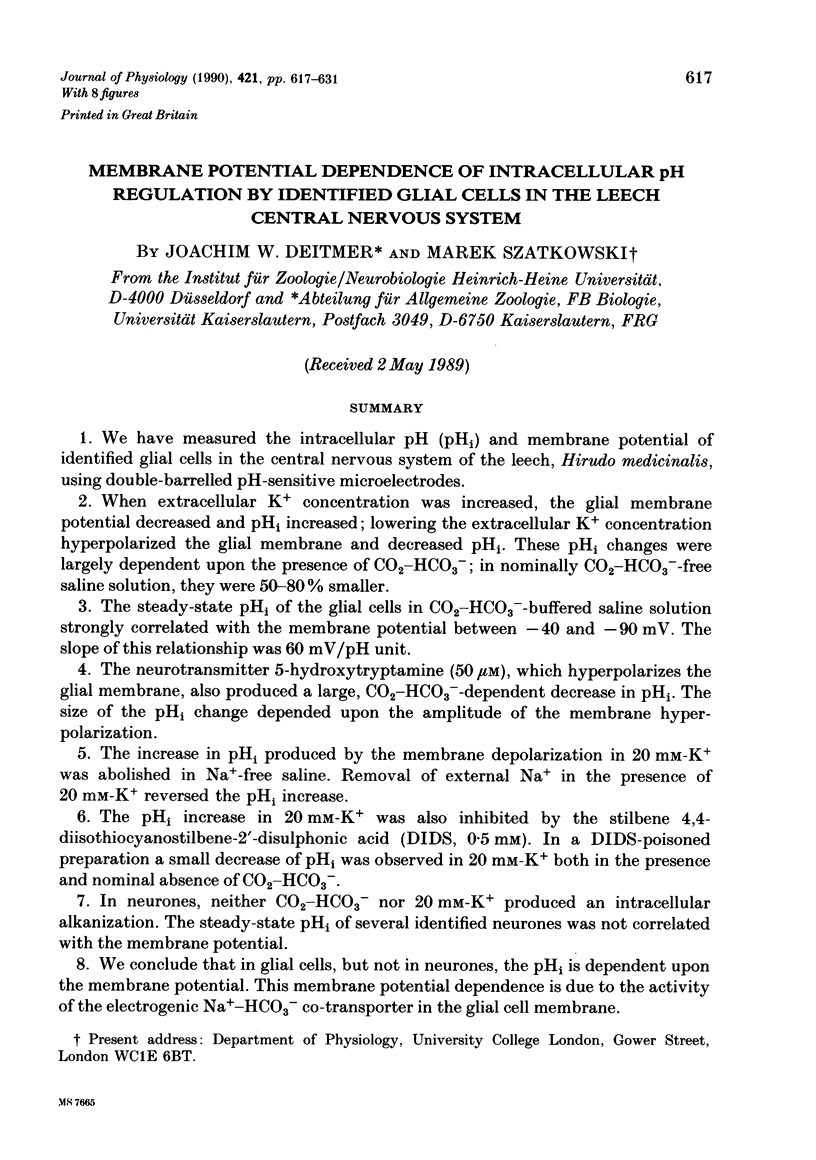
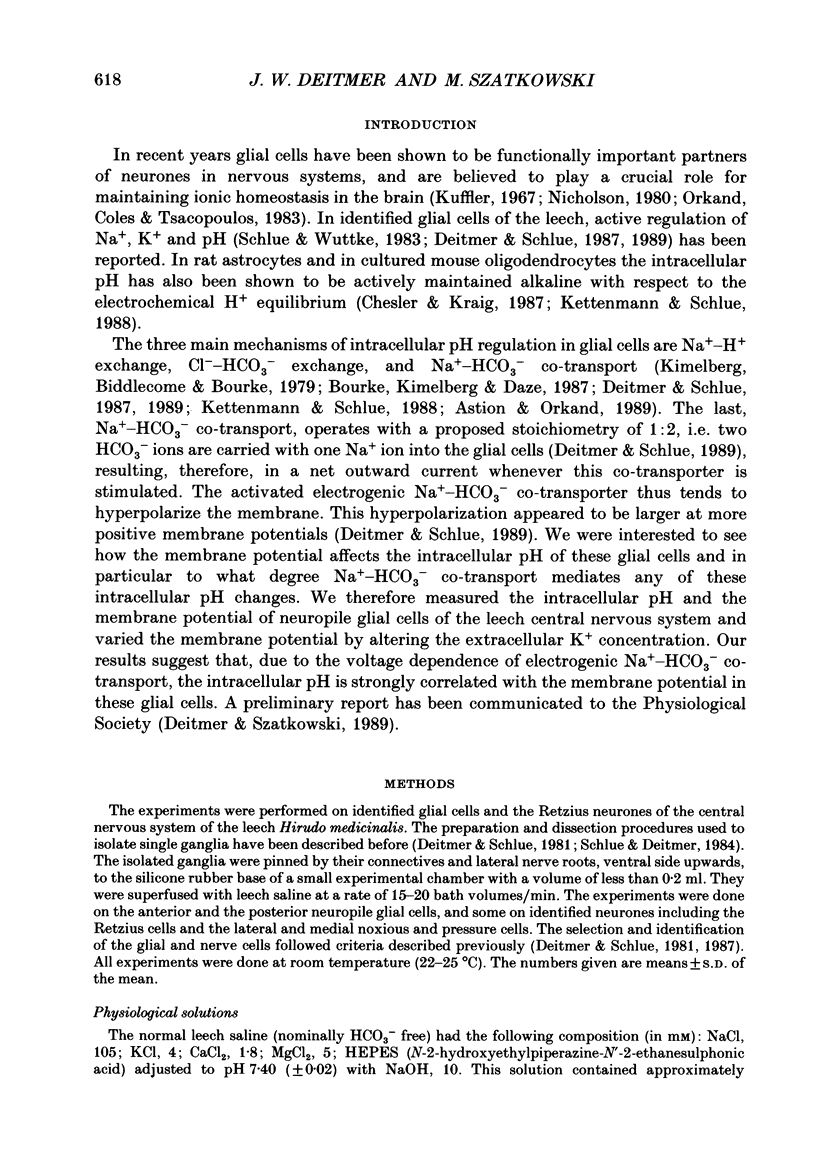
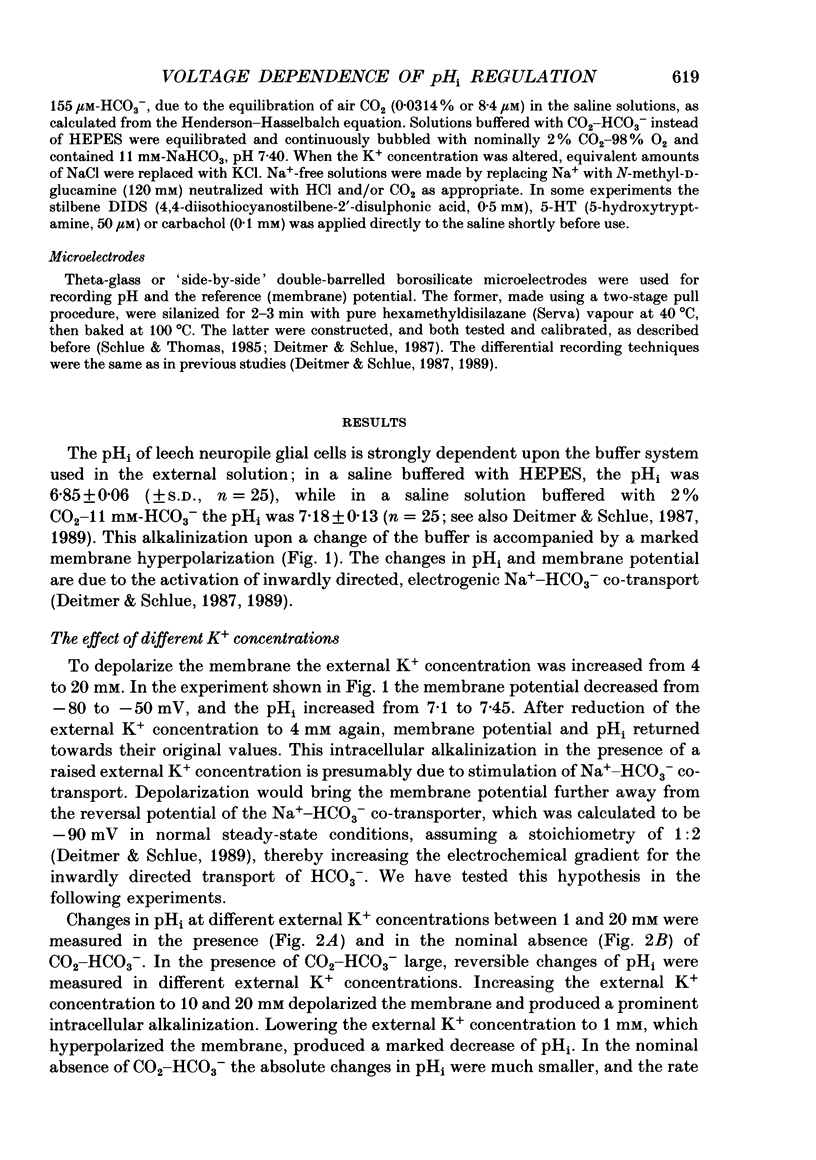
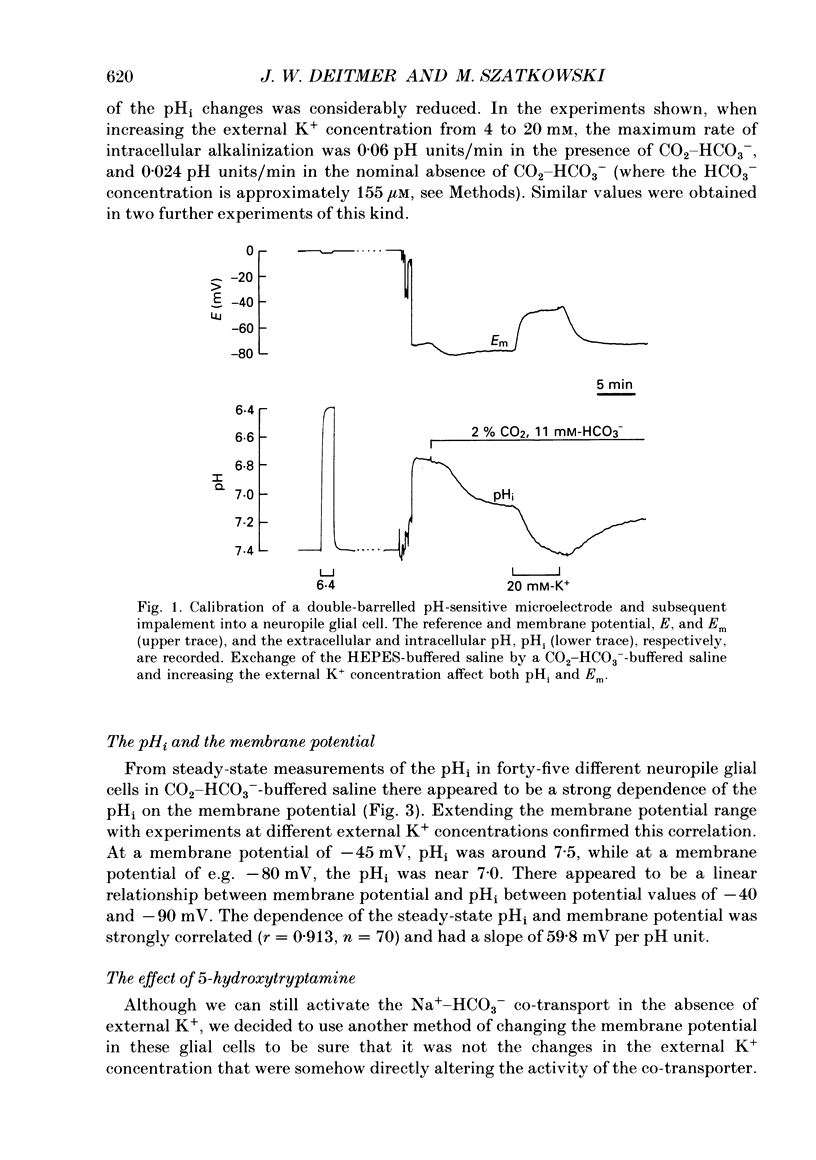
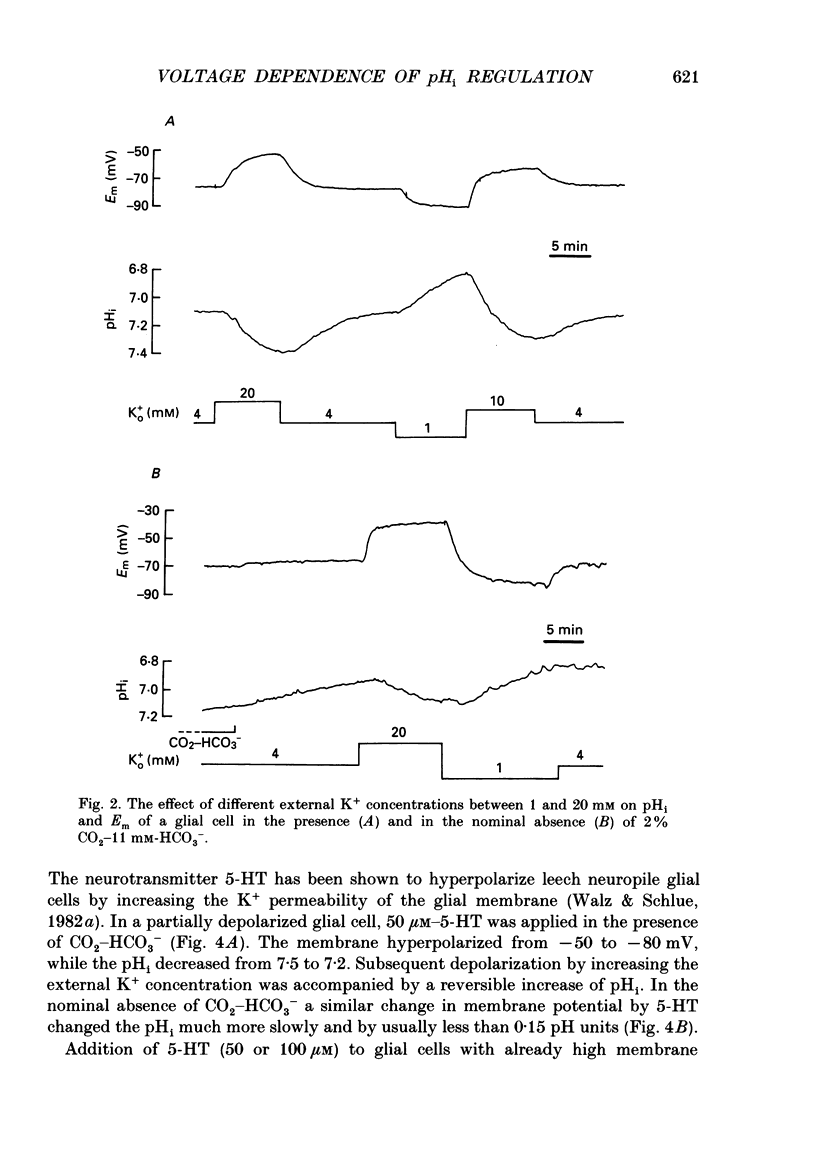
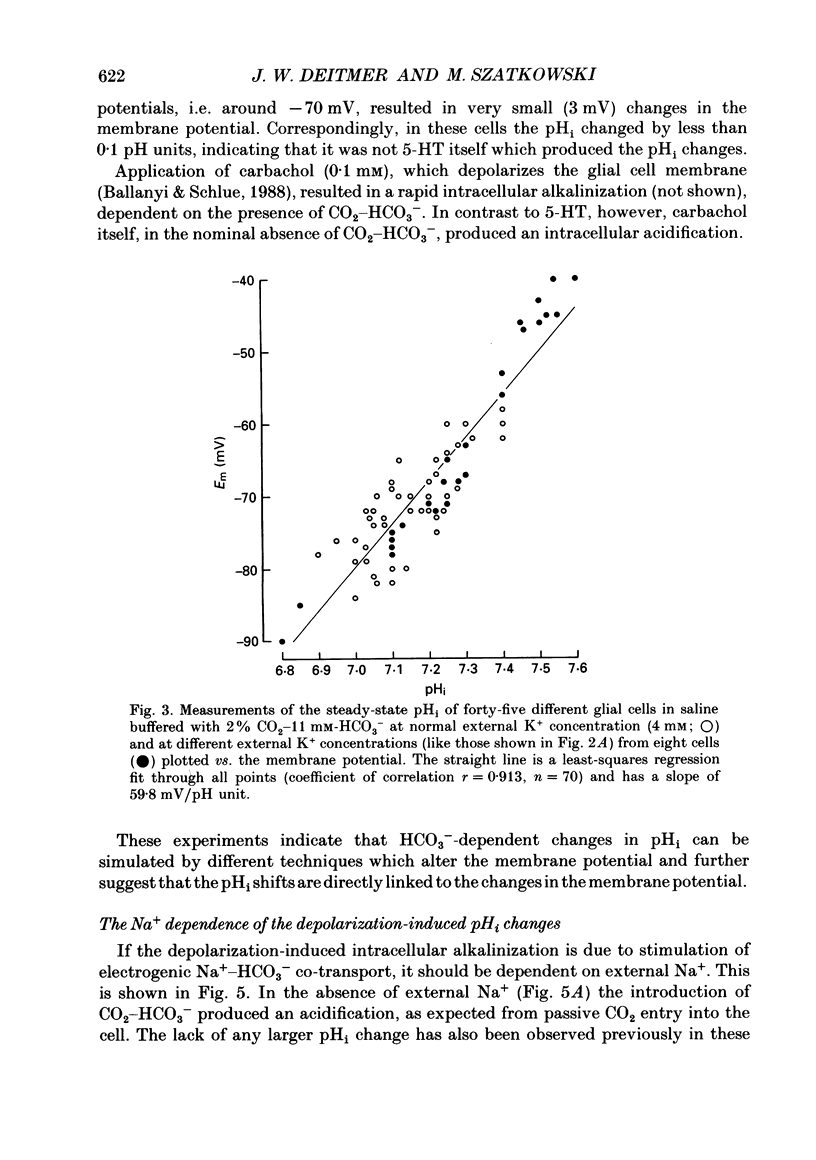
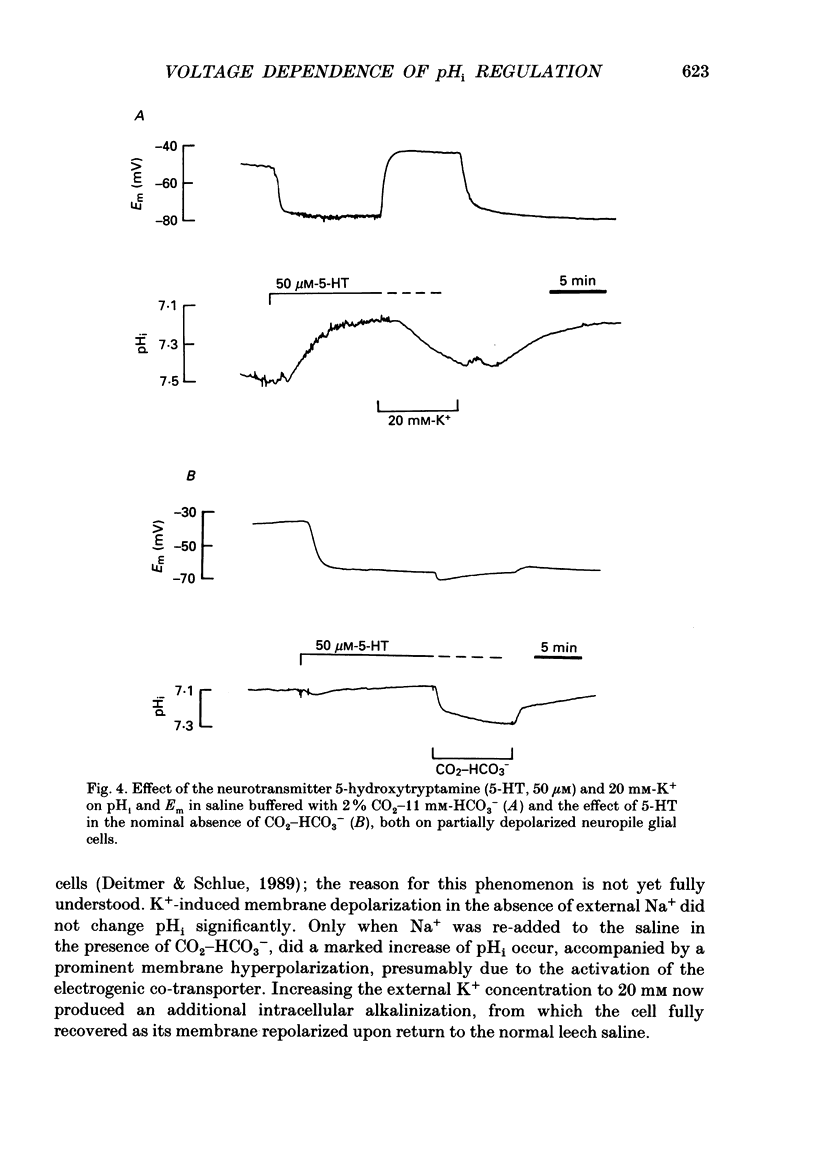
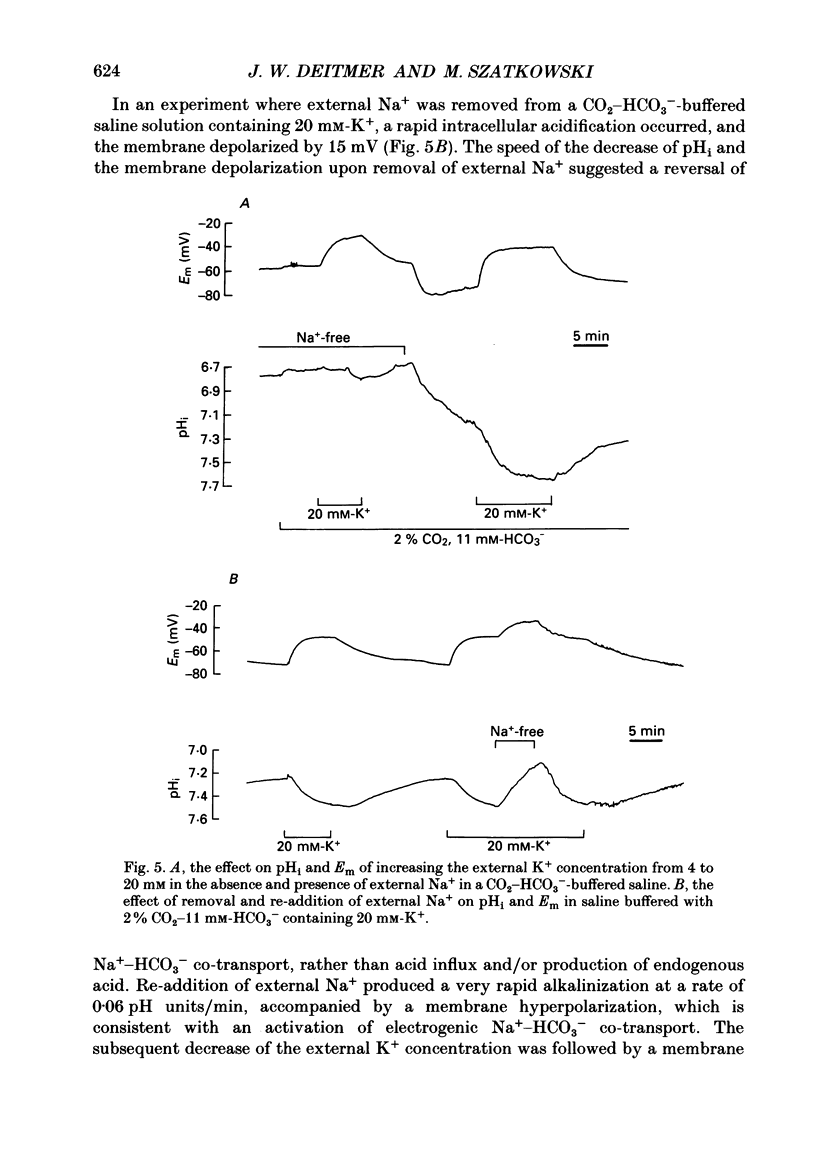
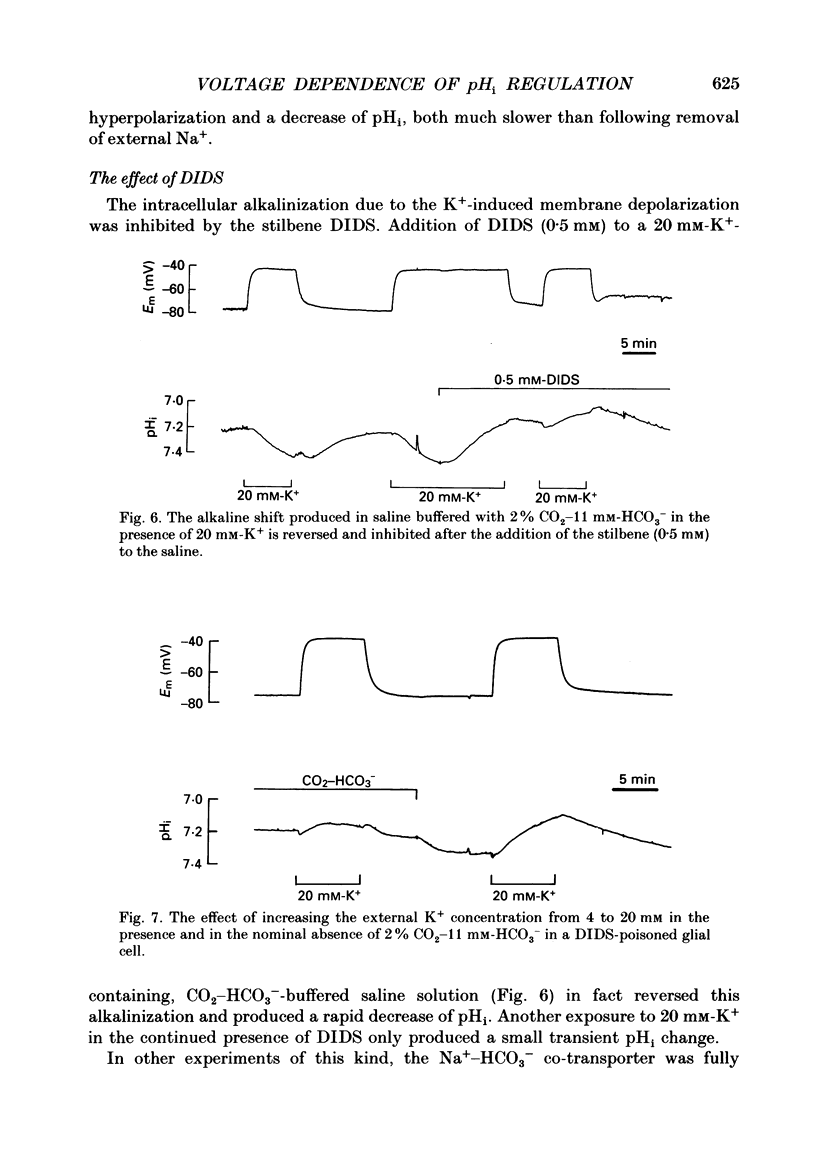
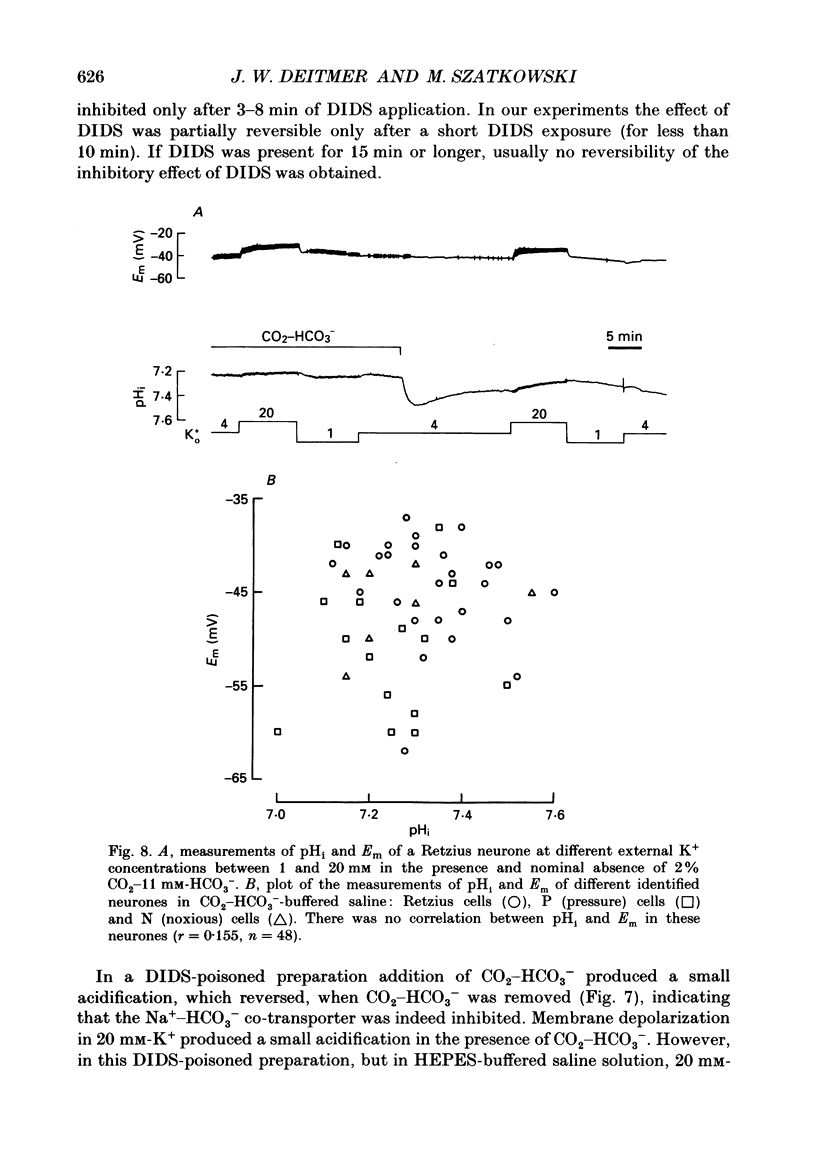
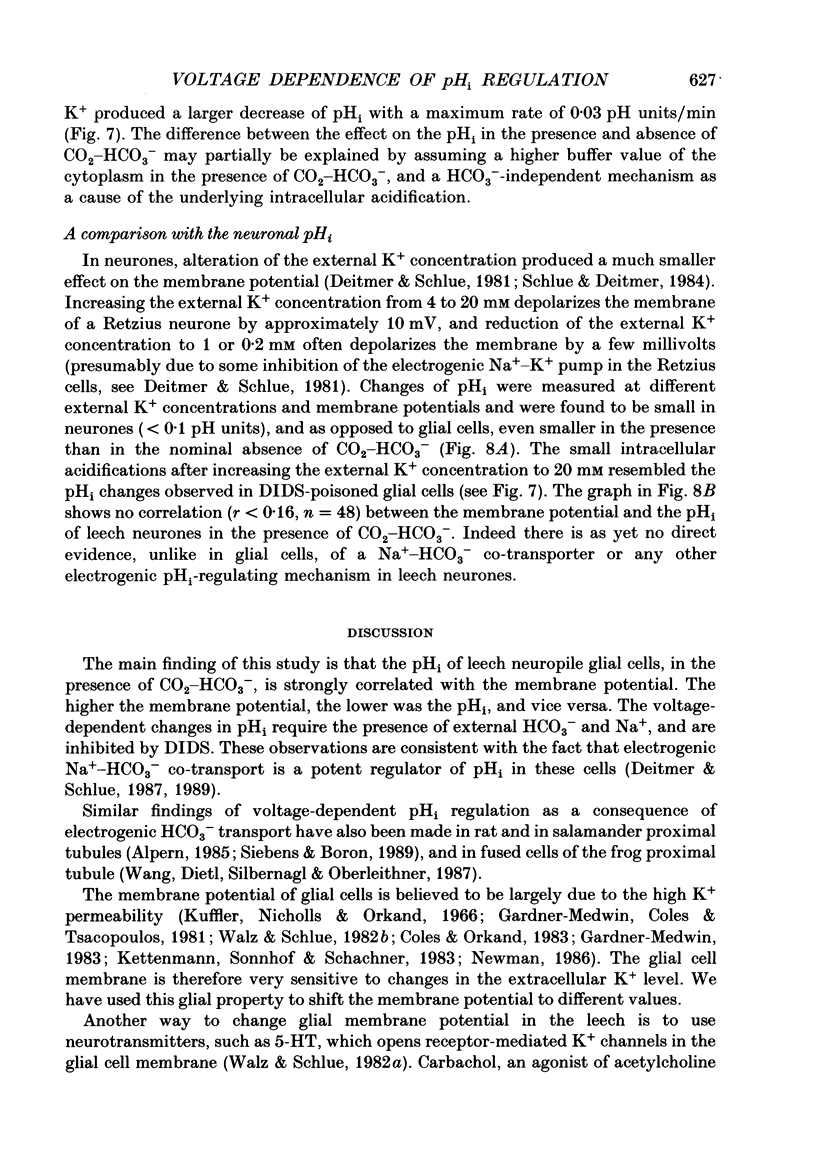
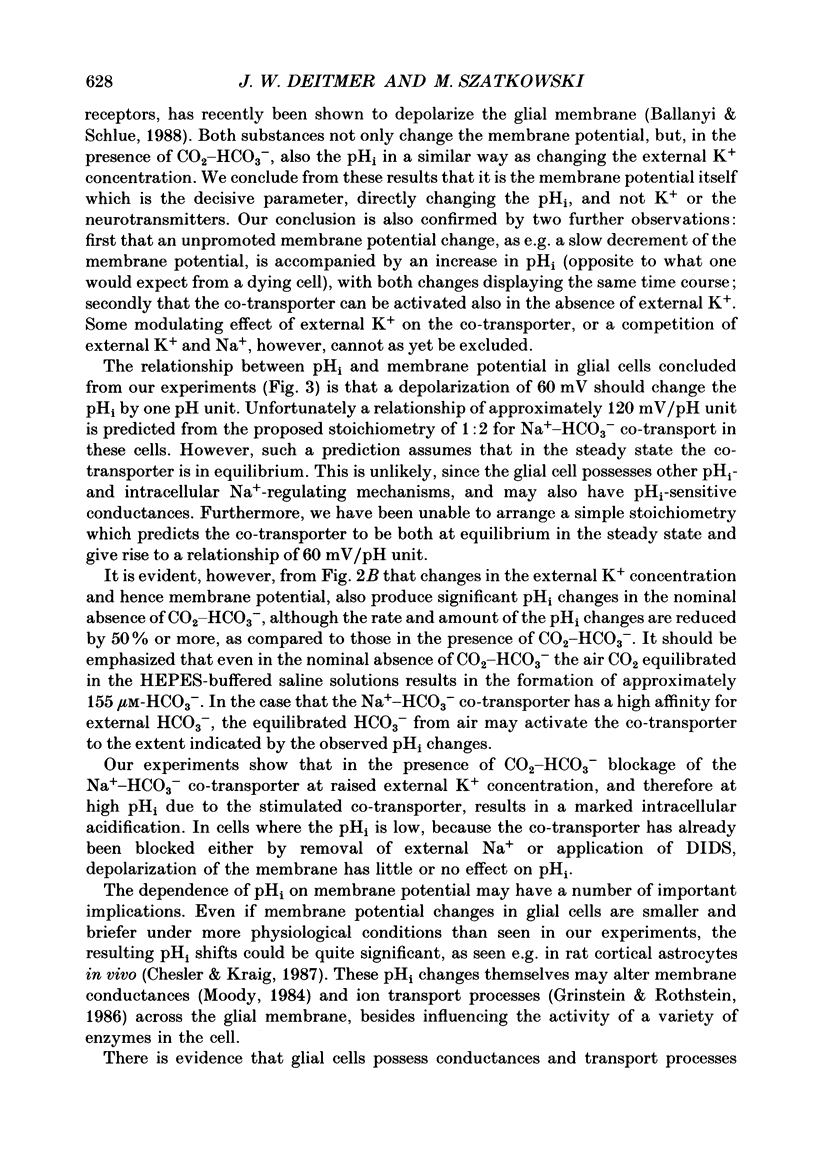
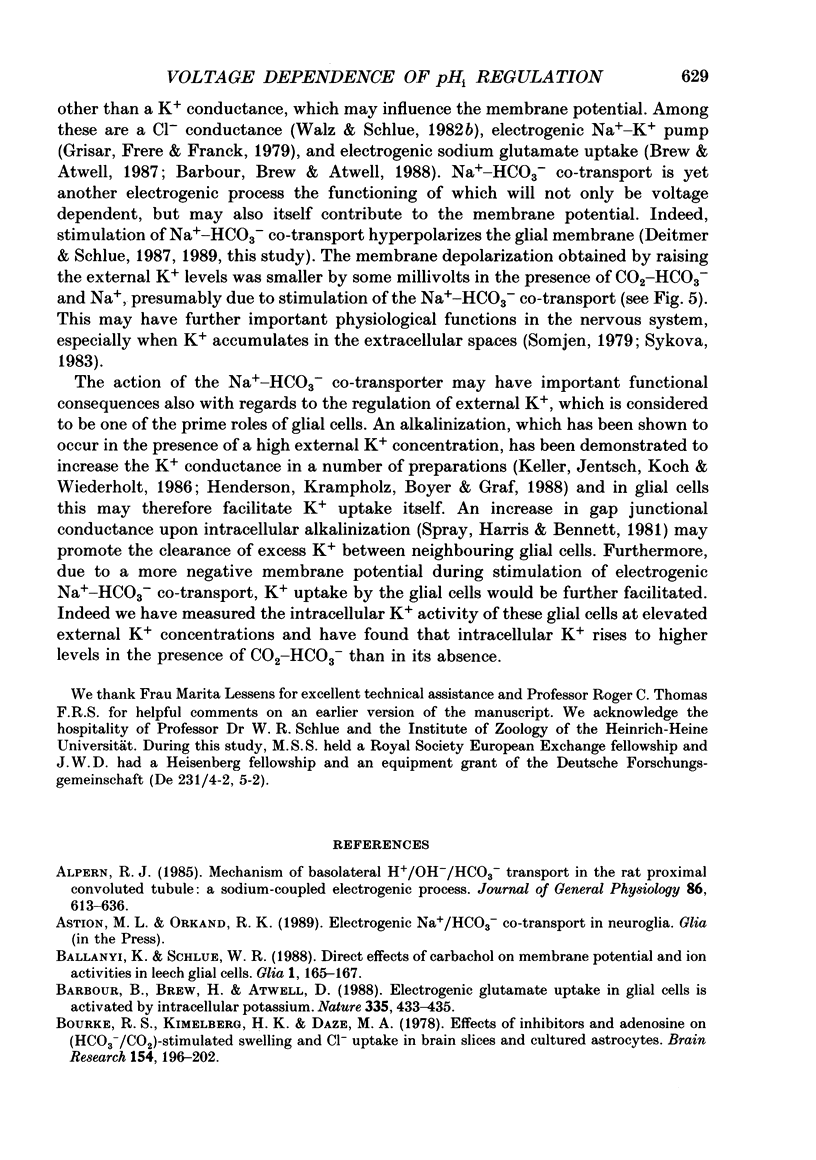
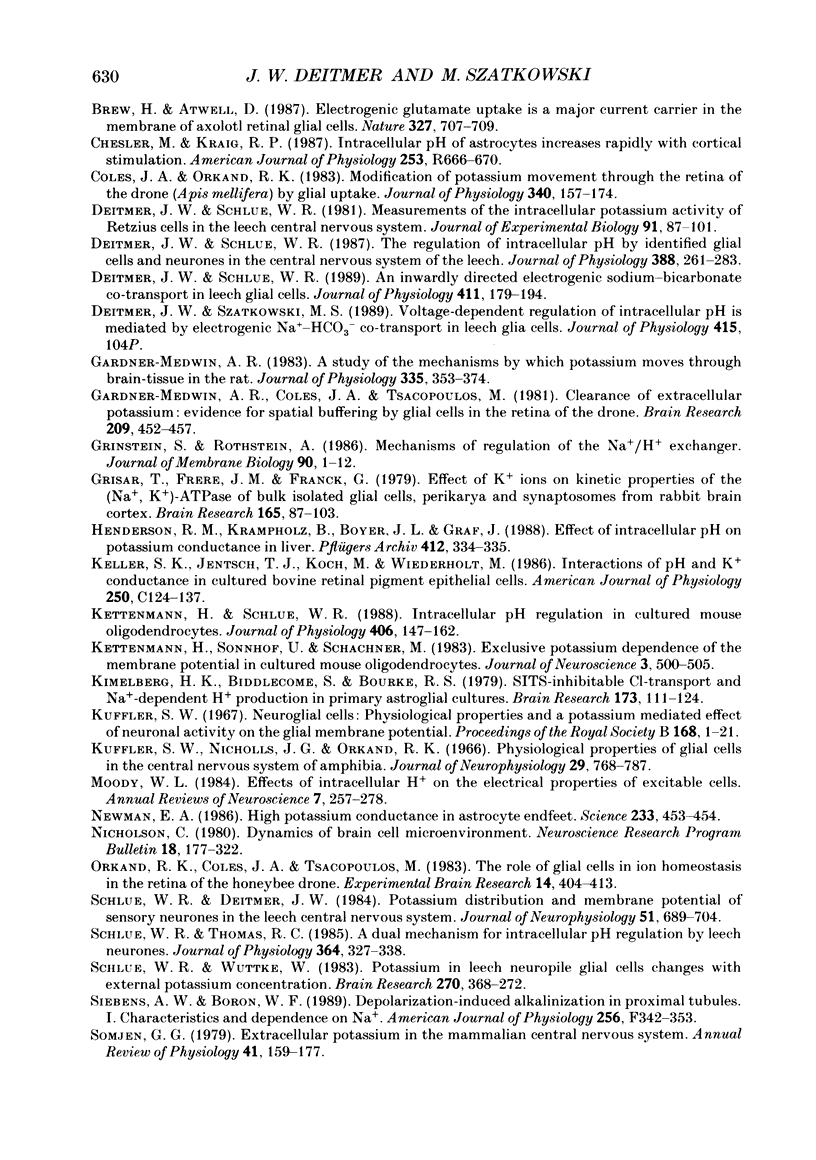
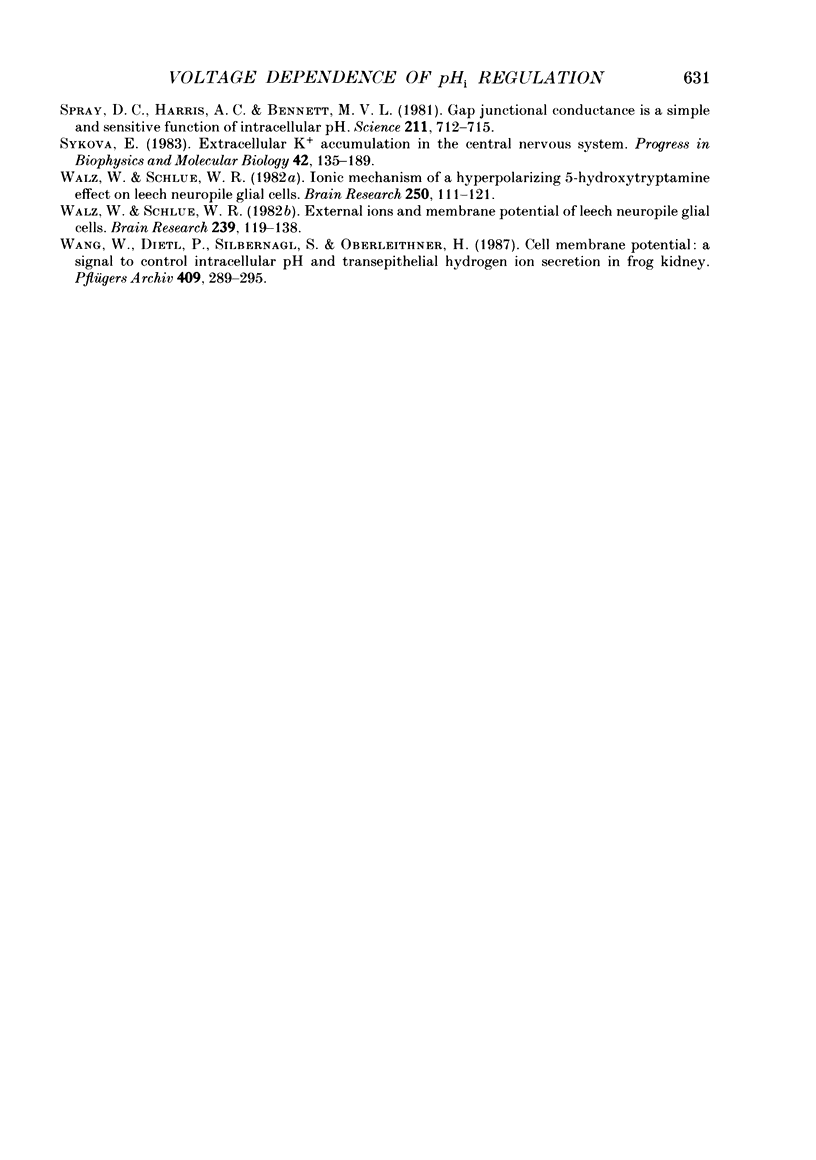
Selected References
These references are in PubMed. This may not be the complete list of references from this article.
- Alpern R. J. Mechanism of basolateral membrane H+/OH-/HCO-3 transport in the rat proximal convoluted tubule. A sodium-coupled electrogenic process. J Gen Physiol. 1985 Nov;86(5):613–636. doi: 10.1085/jgp.86.5.613. [DOI] [PMC free article] [PubMed] [Google Scholar]
- Ballanyi K., Schlue W. R. Direct effects of carbachol on membrane potential and ion activities in leech glial cells. Glia. 1988;1(2):165–167. doi: 10.1002/glia.440010209. [DOI] [PubMed] [Google Scholar]
- Barbour B., Brew H., Attwell D. Electrogenic glutamate uptake in glial cells is activated by intracellular potassium. Nature. 1988 Sep 29;335(6189):433–435. doi: 10.1038/335433a0. [DOI] [PubMed] [Google Scholar]
- Bourke R. S., Kimelberg H. K., Dazé M. A. Effects of inhibitors and adenosine on (HCO3-/CO2)-stimulated swelling and Cl- uptake in brain slices and cultured astrocytes. Brain Res. 1978 Oct 6;154(1):196–202. doi: 10.1016/0006-8993(78)91072-7. [DOI] [PubMed] [Google Scholar]
- Brew H., Attwell D. Electrogenic glutamate uptake is a major current carrier in the membrane of axolotl retinal glial cells. 1987 Jun 25-Jul 1Nature. 327(6124):707–709. doi: 10.1038/327707a0. [DOI] [PubMed] [Google Scholar]
- Chesler M., Kraig R. P. Intracellular pH of astrocytes increases rapidly with cortical stimulation. Am J Physiol. 1987 Oct;253(4 Pt 2):R666–R670. doi: 10.1152/ajpregu.1987.253.4.R666. [DOI] [PMC free article] [PubMed] [Google Scholar]
- Coles J. A., Orkand R. K. Modification of potassium movement through the retina of the drone (Apis mellifera male) by glial uptake. J Physiol. 1983 Jul;340:157–174. doi: 10.1113/jphysiol.1983.sp014756. [DOI] [PMC free article] [PubMed] [Google Scholar]
- Deitmer J. W., Schlue W. R. An inwardly directed electrogenic sodium-bicarbonate co-transport in leech glial cells. J Physiol. 1989 Apr;411:179–194. doi: 10.1113/jphysiol.1989.sp017567. [DOI] [PMC free article] [PubMed] [Google Scholar]
- Deitmer J. W., Schlue W. R. The regulation of intracellular pH by identified glial cells and neurones in the central nervous system of the leech. J Physiol. 1987 Jul;388:261–283. doi: 10.1113/jphysiol.1987.sp016614. [DOI] [PMC free article] [PubMed] [Google Scholar]
- Gardner-Medwin A. R. A study of the mechanisms by which potassium moves through brain tissue in the rat. J Physiol. 1983 Feb;335:353–374. doi: 10.1113/jphysiol.1983.sp014539. [DOI] [PMC free article] [PubMed] [Google Scholar]
- Gardner-Medwin A. R., Coles J. A., Tsacopoulos M. Clearance of extracellular potassium: evidence for spatial buffering by glial cells in the retina of the drone. Brain Res. 1981 Mar 30;209(2):452–457. doi: 10.1016/0006-8993(81)90169-4. [DOI] [PubMed] [Google Scholar]
- Grinstein S., Rothstein A. Mechanisms of regulation of the Na+/H+ exchanger. J Membr Biol. 1986;90(1):1–12. doi: 10.1007/BF01869680. [DOI] [PubMed] [Google Scholar]
- Grisar T., Frere J. M., Franck G. Effect of K+ ions on kinetic properties of the (Na+, K+)-ATPase (EC 3.6.1.3) of bulk isolated glial cells, perikarya and synaptosomes from rabbit brain cortex. Brain Res. 1979 Apr 6;165(1):87–103. doi: 10.1016/0006-8993(79)90047-7. [DOI] [PubMed] [Google Scholar]
- Henderson R. M., Krumpholz B., Boyer J. L., Graf J. Effect of intracellular pH on potassium conductance in liver. Pflugers Arch. 1988 Aug;412(3):334–335. doi: 10.1007/BF00582518. [DOI] [PubMed] [Google Scholar]
- Keller S. K., Jentsch T. J., Koch M., Wiederholt M. Interactions of pH and K+ conductance in cultured bovine retinal pigment epithelial cells. Am J Physiol. 1986 Jan;250(1 Pt 1):C124–C137. doi: 10.1152/ajpcell.1986.250.1.C124. [DOI] [PubMed] [Google Scholar]
- Kettenmann H., Schlue W. R. Intracellular pH regulation in cultured mouse oligodendrocytes. J Physiol. 1988 Dec;406:147–162. doi: 10.1113/jphysiol.1988.sp017373. [DOI] [PMC free article] [PubMed] [Google Scholar]
- Kettenmann H., Sonnhof U., Schachner M. Exclusive potassium dependence of the membrane potential in cultured mouse oligodendrocytes. J Neurosci. 1983 Mar;3(3):500–505. doi: 10.1523/JNEUROSCI.03-03-00500.1983. [DOI] [PMC free article] [PubMed] [Google Scholar]
- Kimelberg H. K., Biddlecome S., Bourke R. S. SITS-inhibitable Cl- transport and Na+-dependent H+ production in primary astroglial cultures. Brain Res. 1979 Sep 7;173(1):111–124. [PubMed] [Google Scholar]
- Kuffler S. W. Neuroglial cells: physiological properties and a potassium mediated effect of neuronal activity on the glial membrane potential. Proc R Soc Lond B Biol Sci. 1967 Jun 6;168(1010):1–21. doi: 10.1098/rspb.1967.0047. [DOI] [PubMed] [Google Scholar]
- Kuffler S. W., Nicholls J. G., Orkand R. K. Physiological properties of glial cells in the central nervous system of amphibia. J Neurophysiol. 1966 Jul;29(4):768–787. doi: 10.1152/jn.1966.29.4.768. [DOI] [PubMed] [Google Scholar]
- Moody W., Jr Effects of intracellular H+ on the electrical properties of excitable cells. Annu Rev Neurosci. 1984;7:257–278. doi: 10.1146/annurev.ne.07.030184.001353. [DOI] [PubMed] [Google Scholar]
- Newman E. A. High potassium conductance in astrocyte endfeet. Science. 1986 Jul 25;233(4762):453–454. doi: 10.1126/science.3726539. [DOI] [PMC free article] [PubMed] [Google Scholar]
- Nicholson C. Dynamics of the brain cell microenvironment. Neurosci Res Program Bull. 1980 Apr;18(2):175–322. [PubMed] [Google Scholar]
- Schlue W. R., Deitmer J. W. Potassium distribution and membrane potential of sensory neurons in the leech nervous system. J Neurophysiol. 1984 Apr;51(4):689–704. doi: 10.1152/jn.1984.51.4.689. [DOI] [PubMed] [Google Scholar]
- Schlue W. R., Thomas R. C. A dual mechanism for intracellular pH regulation by leech neurones. J Physiol. 1985 Jul;364:327–338. doi: 10.1113/jphysiol.1985.sp015748. [DOI] [PMC free article] [PubMed] [Google Scholar]
- Schlue W. R., Wuttke W. Potassium activity in leech neuropile glial cells changes with external potassium concentration. Brain Res. 1983 Jul 4;270(2):368–372. doi: 10.1016/0006-8993(83)90616-9. [DOI] [PubMed] [Google Scholar]
- Siebens A. W., Boron W. F. Depolarization-induced alkalinization in proximal tubules. I. Characteristics and dependence on Na+. Am J Physiol. 1989 Feb;256(2 Pt 2):F342–F353. doi: 10.1152/ajprenal.1989.256.2.F342. [DOI] [PubMed] [Google Scholar]
- Somjen G. G. Extracellular potassium in the mammalian central nervous system. Annu Rev Physiol. 1979;41:159–177. doi: 10.1146/annurev.ph.41.030179.001111. [DOI] [PubMed] [Google Scholar]
- Spray D. C., Harris A. L., Bennett M. V. Gap junctional conductance is a simple and sensitive function of intracellular pH. Science. 1981 Feb 13;211(4483):712–715. doi: 10.1126/science.6779379. [DOI] [PubMed] [Google Scholar]
- Syková E. Extracellular K+ accumulation in the central nervous system. Prog Biophys Mol Biol. 1983;42(2-3):135–189. doi: 10.1016/0079-6107(83)90006-8. [DOI] [PubMed] [Google Scholar]
- Walz W., Schlue W. R. External ions and membrane potential of leech neuropile glial cells. Brain Res. 1982 May 6;239(1):119–138. doi: 10.1016/0006-8993(82)90837-x. [DOI] [PubMed] [Google Scholar]
- Walz W., Schlue W. R. Ionic mechanism of a hyperpolarizing 5-hydroxytryptamine effect on leech neuropile glial cells. Brain Res. 1982 Oct 28;250(1):111–121. doi: 10.1016/0006-8993(82)90957-x. [DOI] [PubMed] [Google Scholar]
- Wang W., Dietl P., Silbernagl S., Oberleithner H. Cell membrane potential: a signal to control intracellular pH and transepithelial hydrogen ion secretion in frog kidney. Pflugers Arch. 1987 Jul;409(3):289–295. doi: 10.1007/BF00583478. [DOI] [PubMed] [Google Scholar]