Abstract
1. The force produced in single fibres isolated from the anterior tibialis muscle of the frog Rana temporaria has been measured in tetani near 4 degrees C, and then in calcium-activated contractures of segments of the same fibres after chemical demembranation. All measurements were made at a sarcomere length of 2.3 microns. Force was normalized for fibre cross-section by the dry weight per unit length of the segments, which is proportional to cross-sectional area (Elzinga, Howarth, Rall, Wilson & Woledge, 1989). 2. The ratio of the force developed by the skinned segments to that produced by the intact fibres was inversely related to segment cross-section (dry weight per unit length), falling from approximately 1.0 for the thinnest segments to 0.6 for the thickest segments. 3. It is calculated that the accumulation of orthophosphate ion within contracting segments can account for a significant part of the decline in relative force in thicker segments. 4. The absolute forces in intact fibres and their derived segments were strongly correlated, but normalization by segment cross-section removed the correlation. 5. It is concluded that the sources of the approximately twofold variation in normalized force in both intact and skinned preparations are different. The existence of diffusible, force-modulating factors in intact fibres, which may be removed during skinning, is considered.
Full text
PDF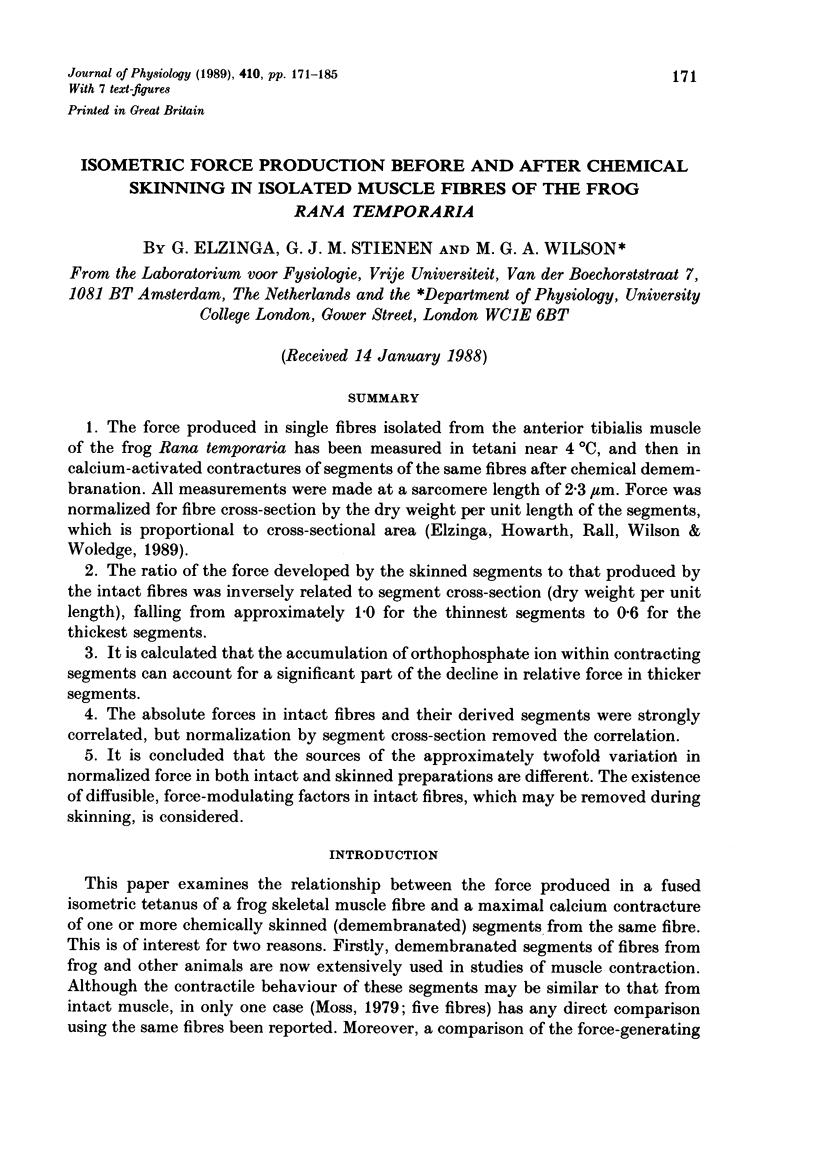
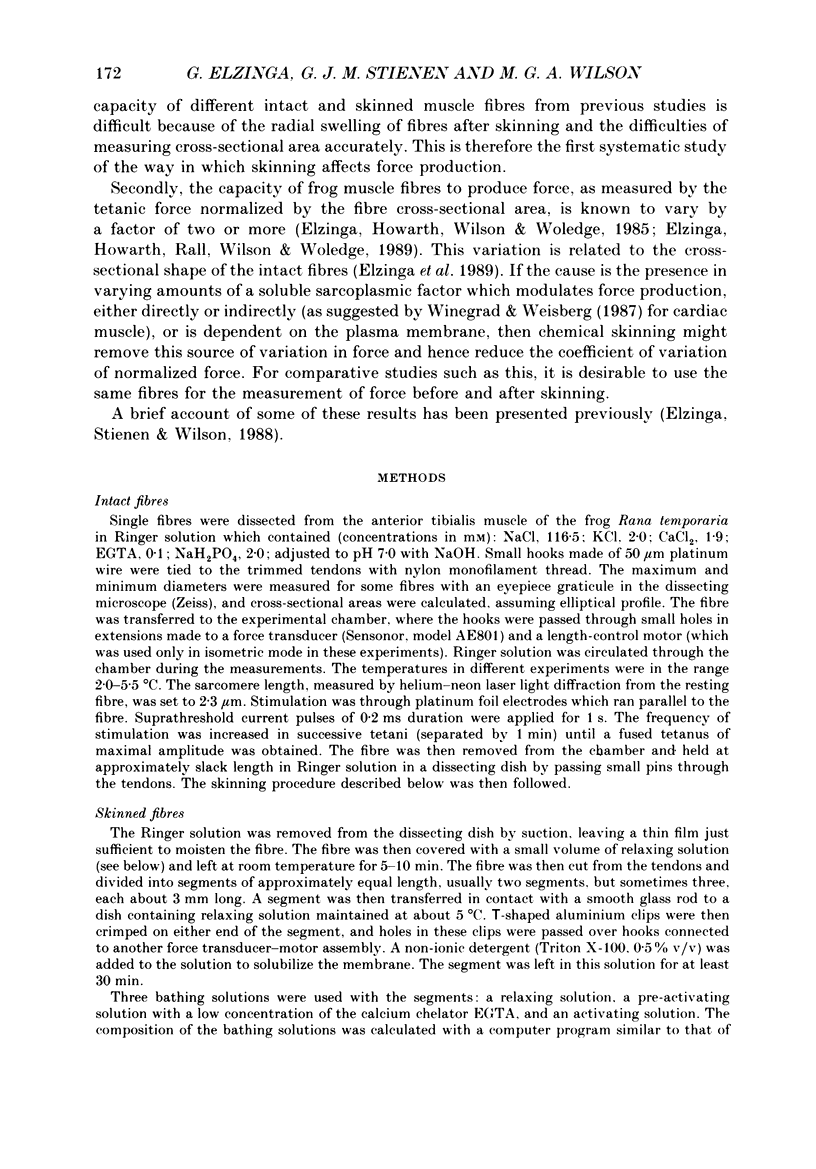
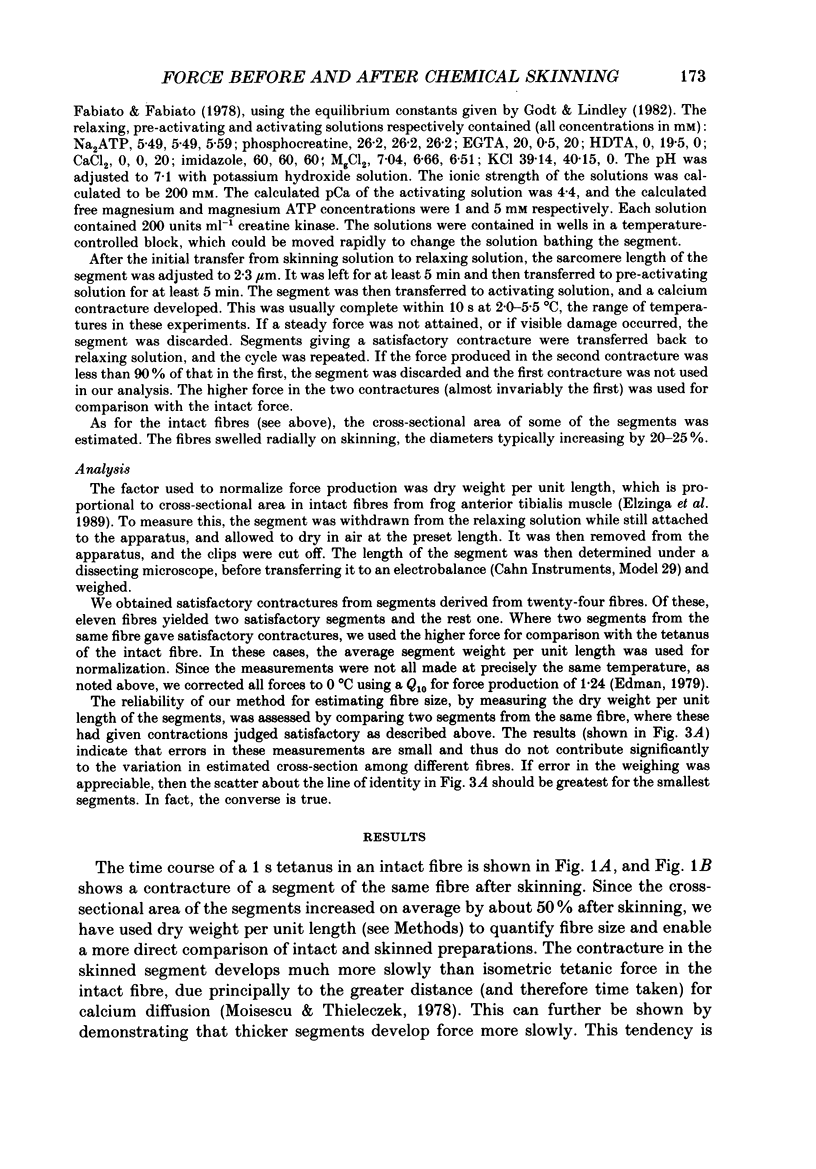
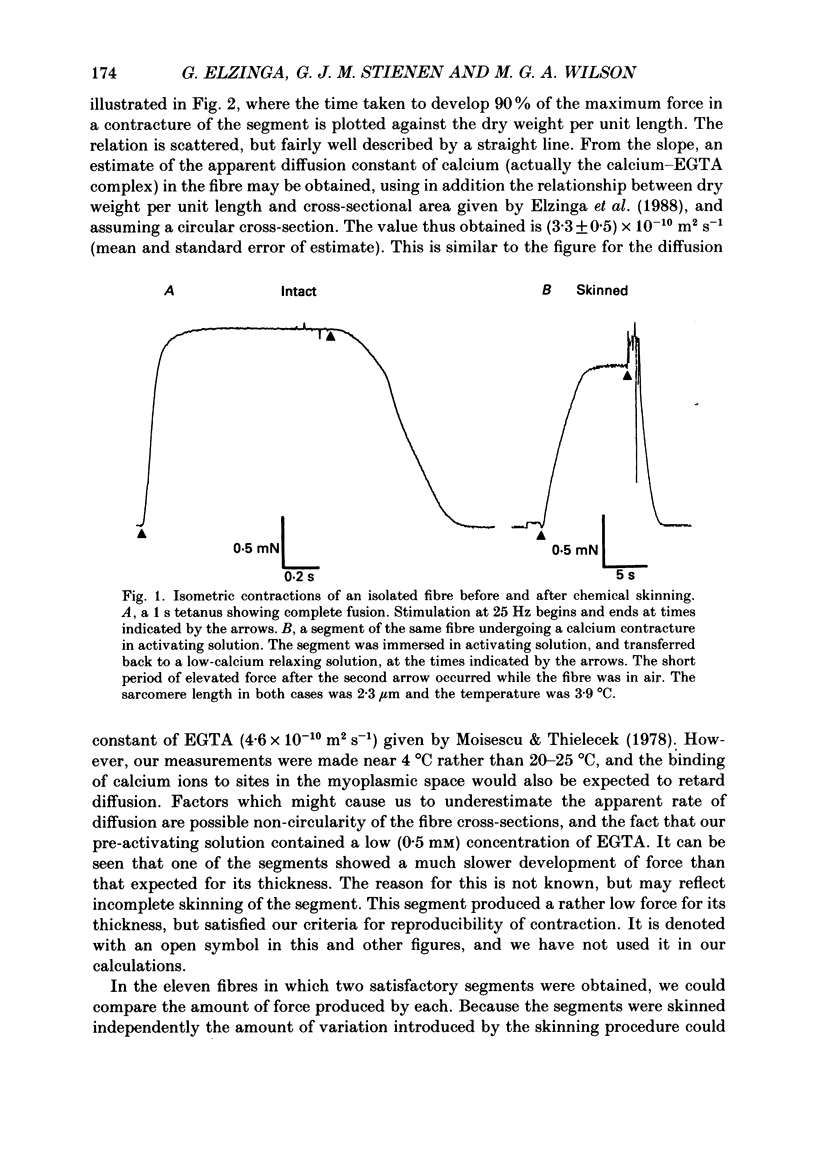
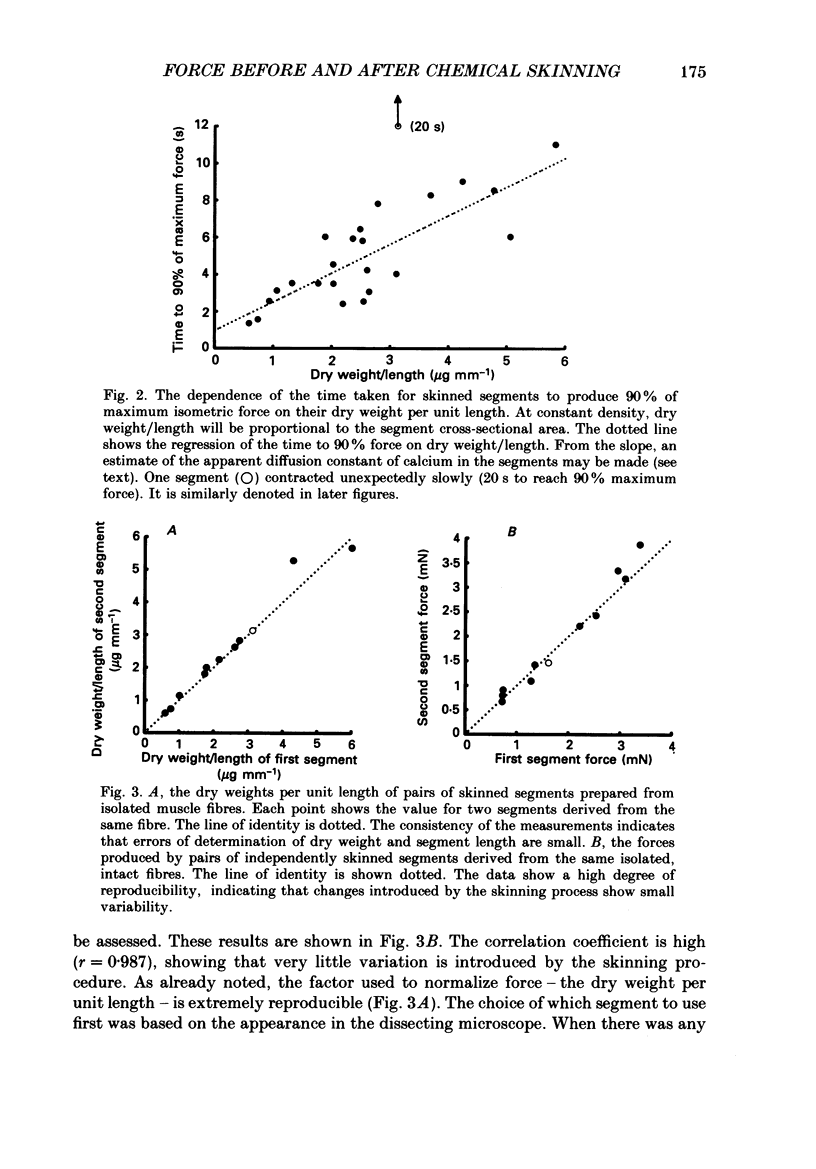
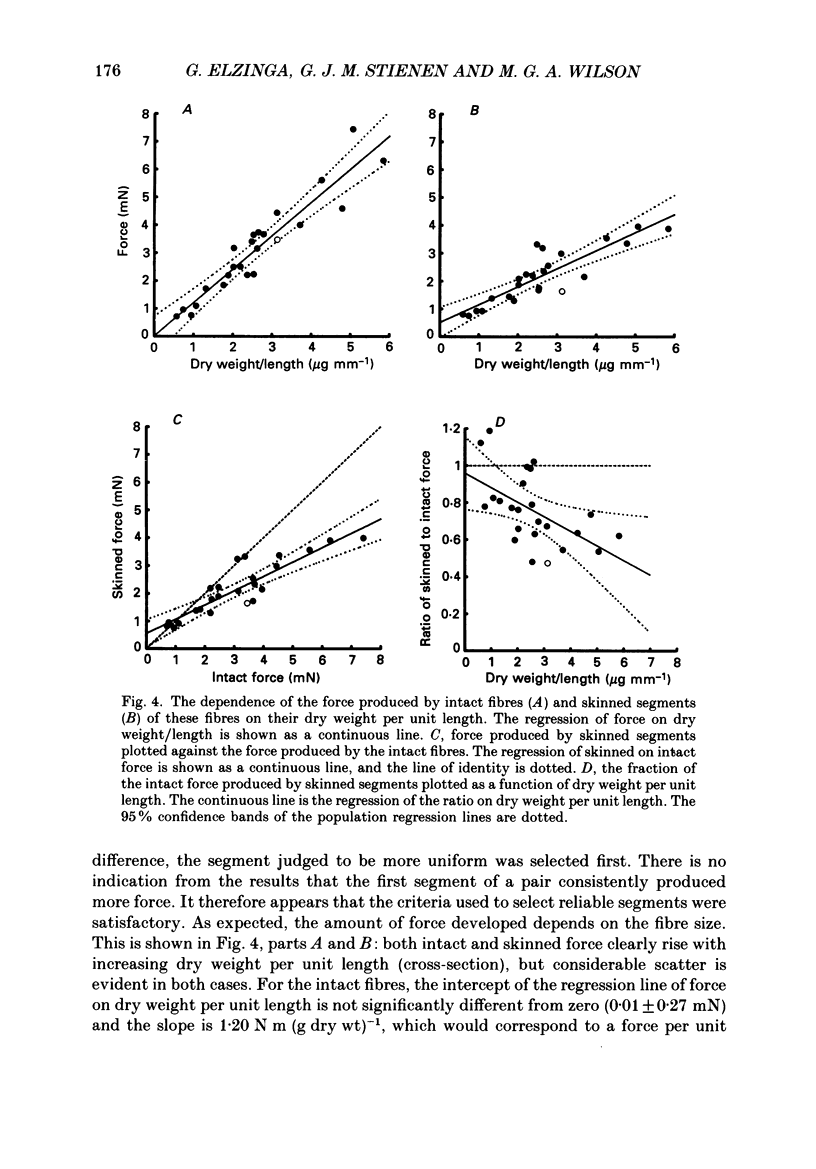
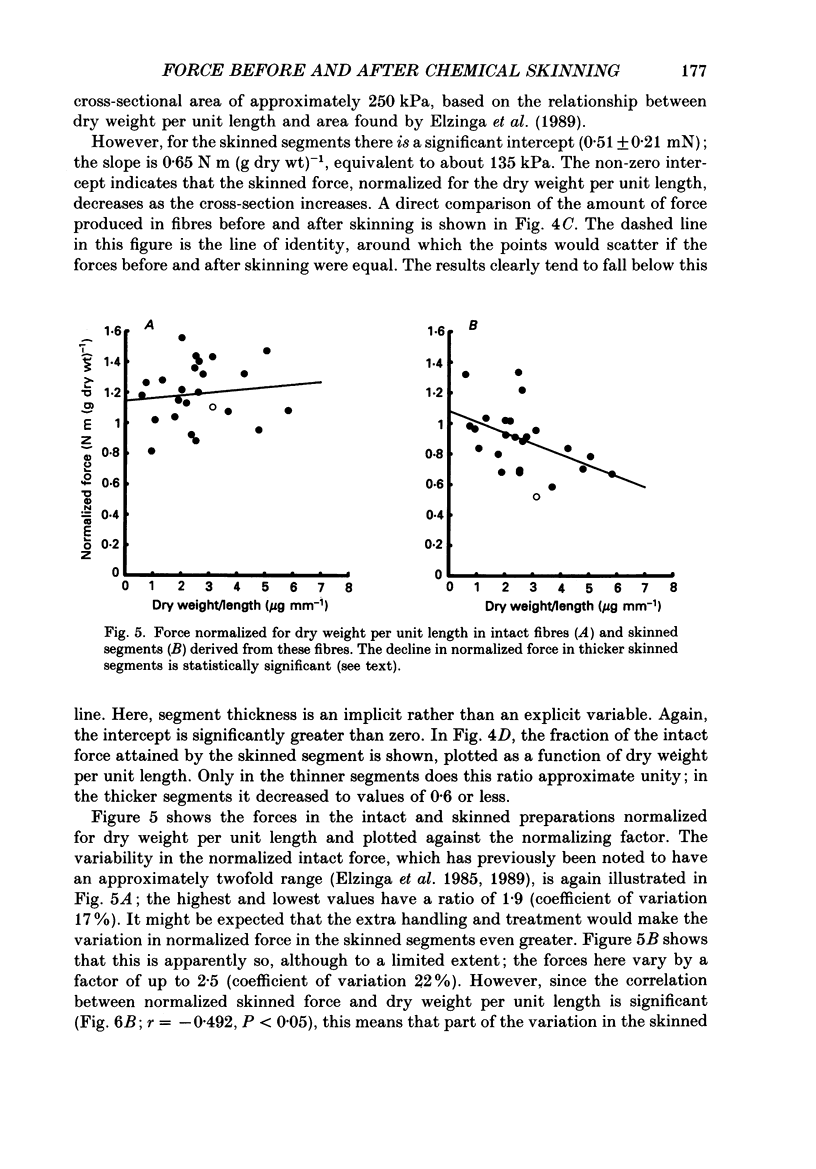
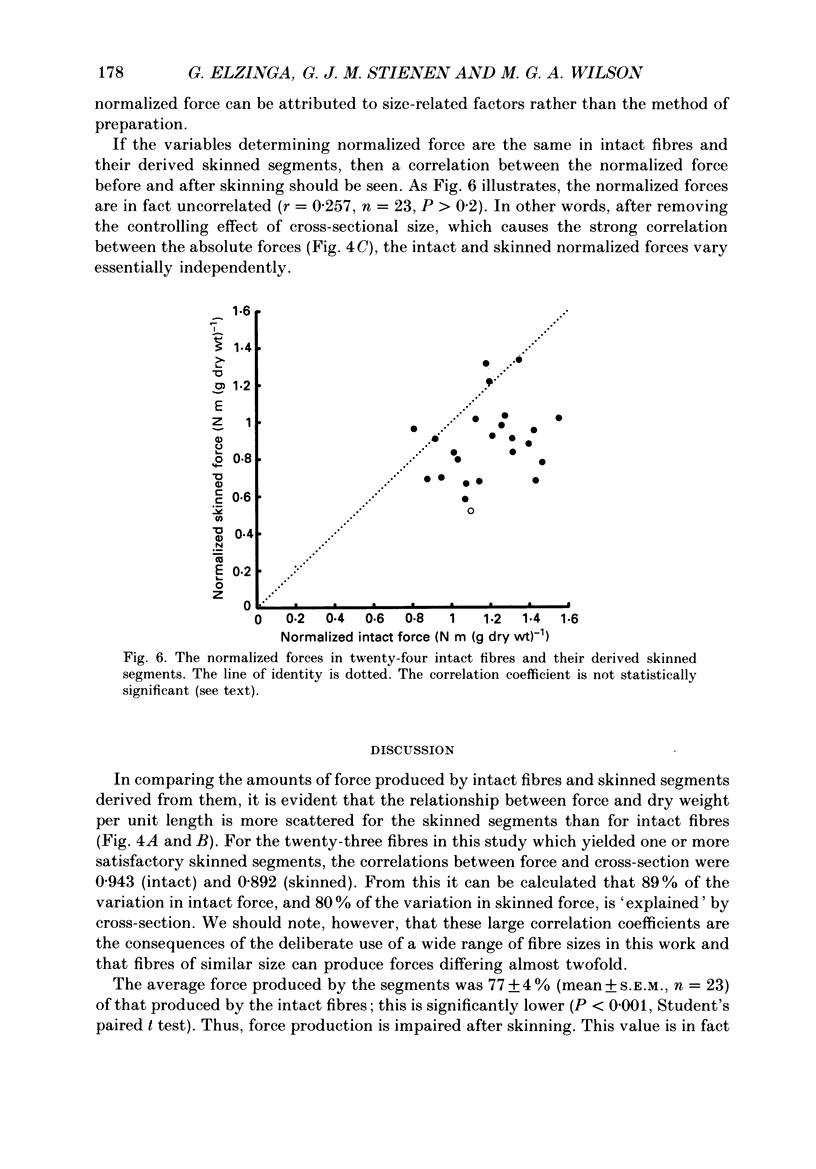
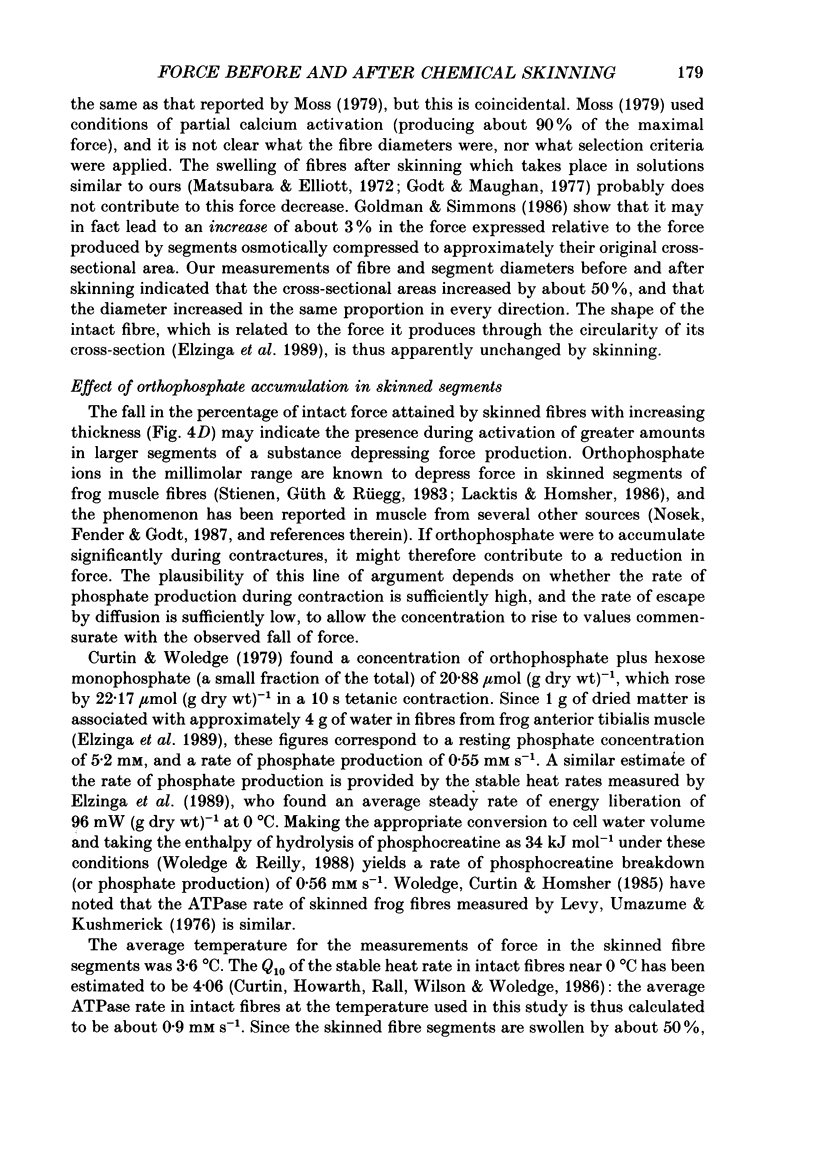
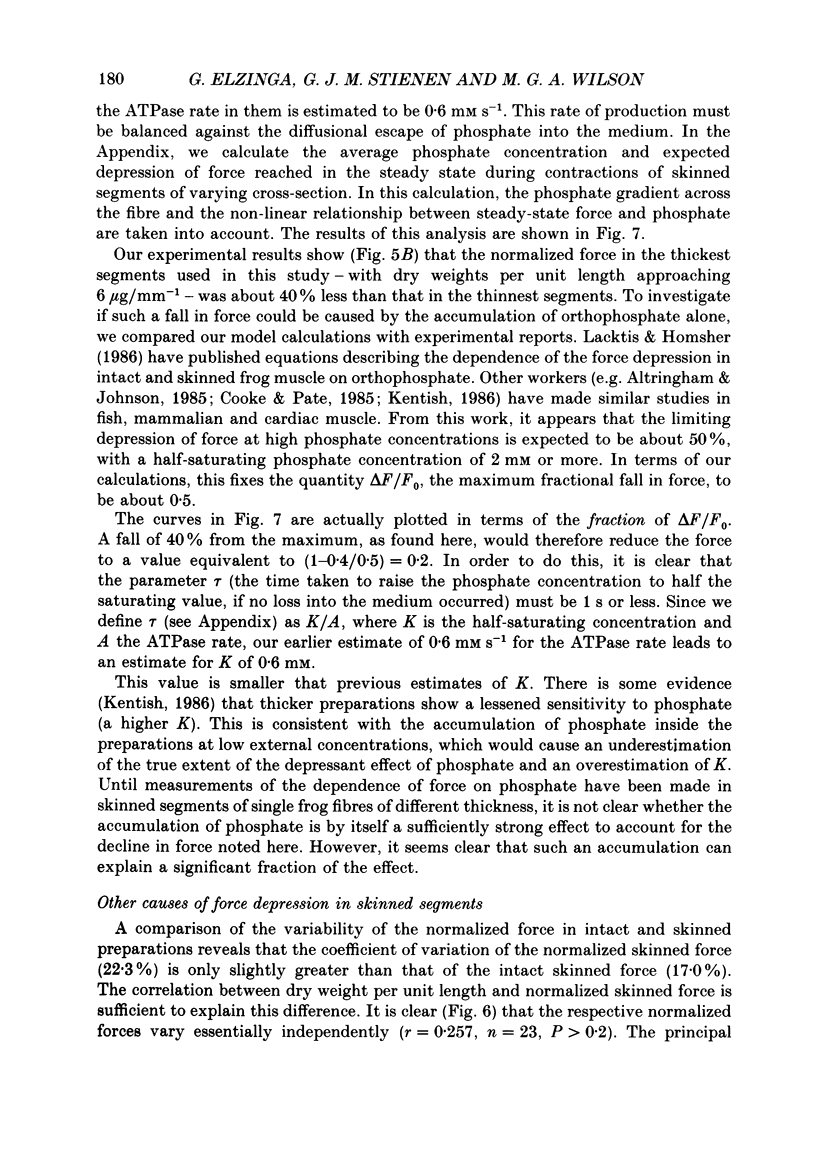
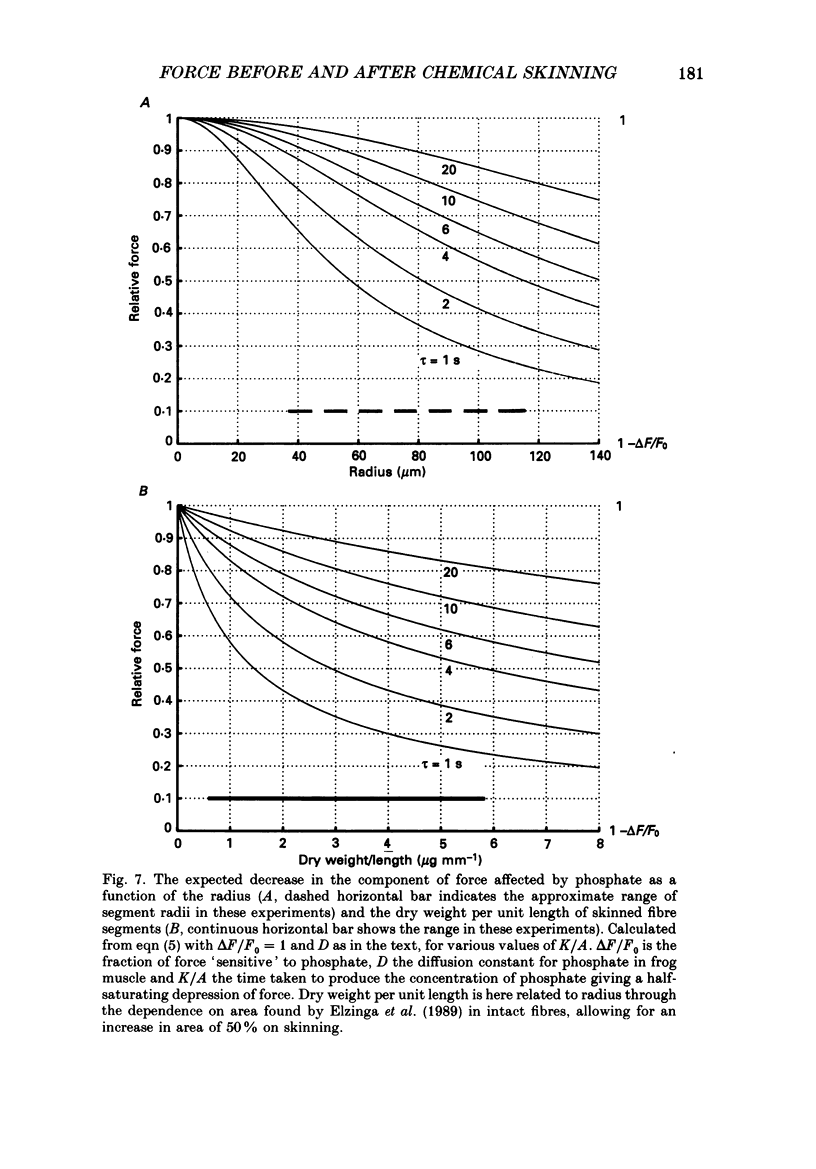
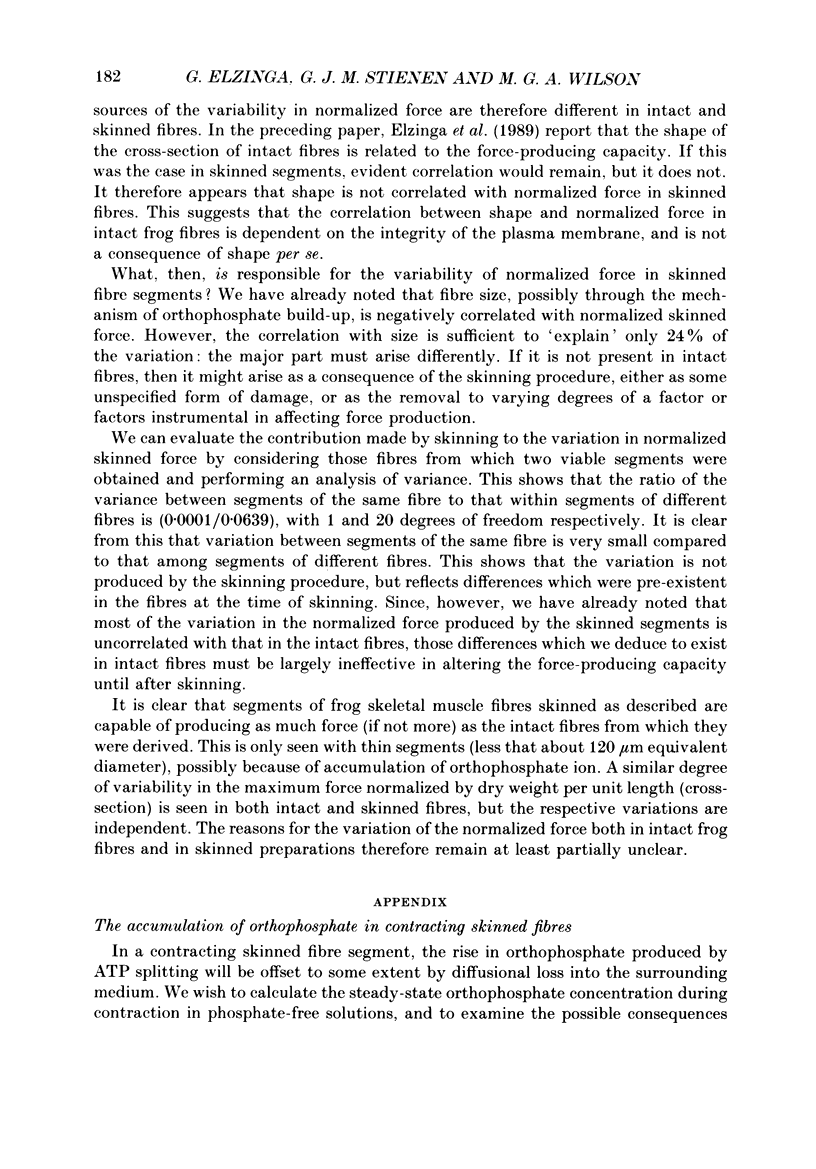
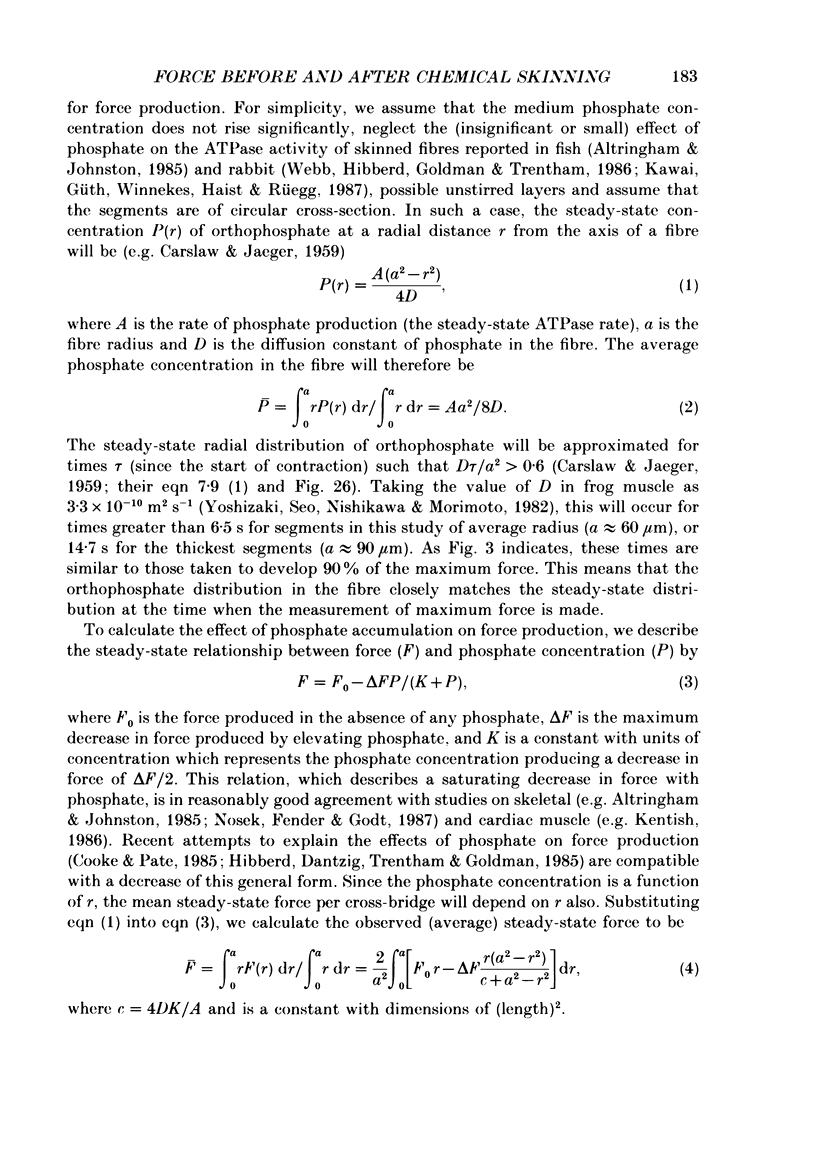
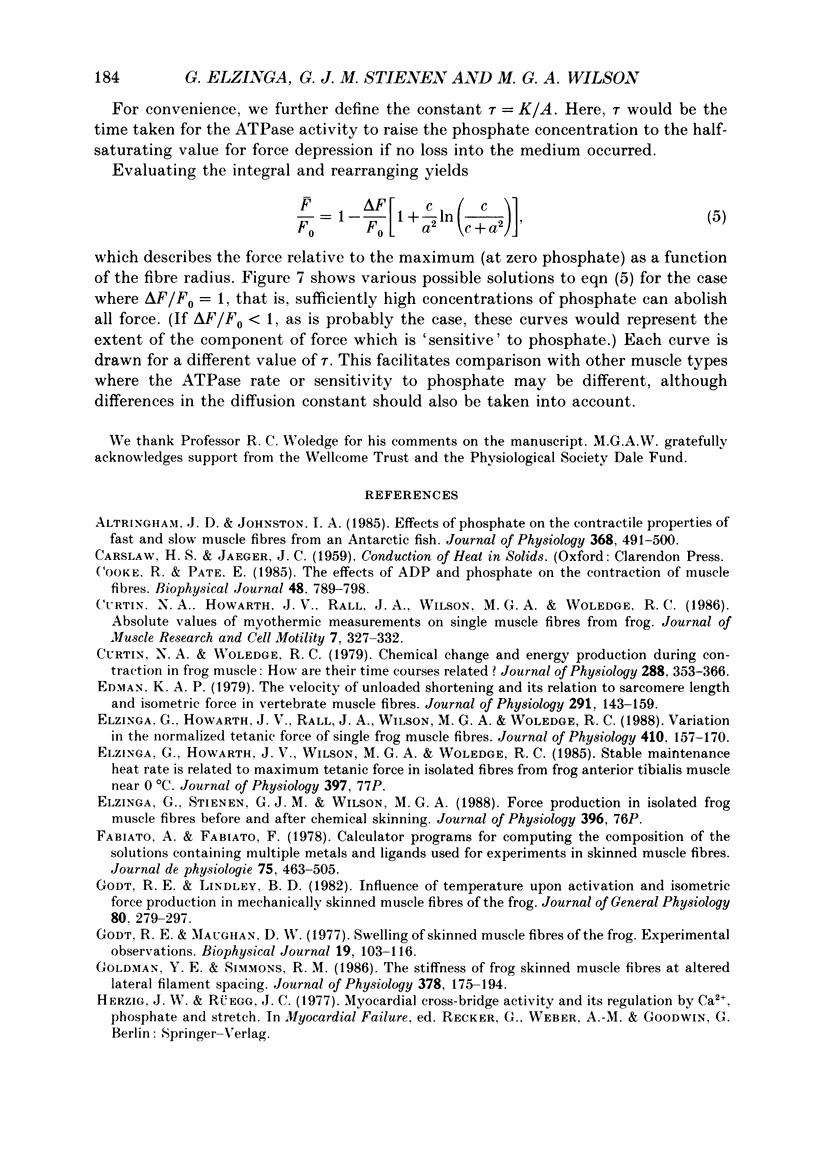
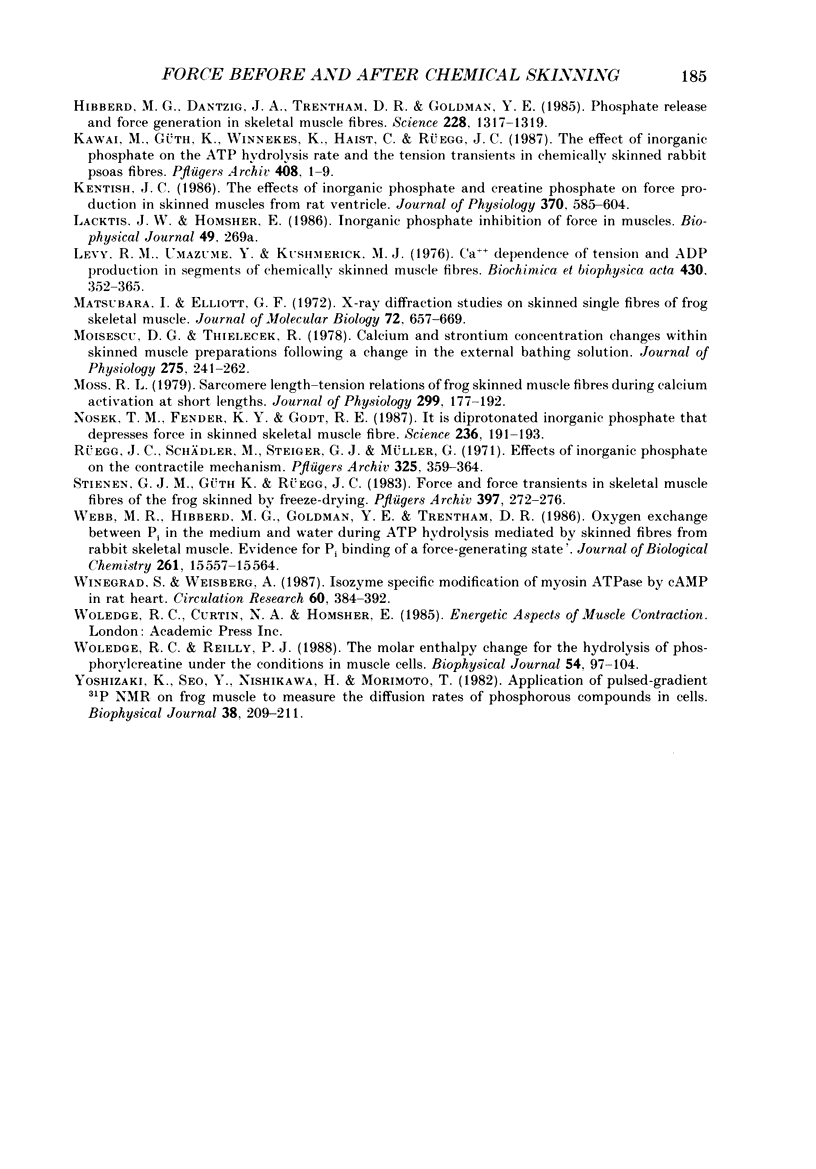
Selected References
These references are in PubMed. This may not be the complete list of references from this article.
- Altringham J. D., Johnston I. A. Effects of phosphate on the contractile properties of fast and slow muscle fibres from an Antarctic fish. J Physiol. 1985 Nov;368:491–500. doi: 10.1113/jphysiol.1985.sp015871. [DOI] [PMC free article] [PubMed] [Google Scholar]
- Cooke R., Pate E. The effects of ADP and phosphate on the contraction of muscle fibers. Biophys J. 1985 Nov;48(5):789–798. doi: 10.1016/S0006-3495(85)83837-6. [DOI] [PMC free article] [PubMed] [Google Scholar]
- Curtin N. A., Howarth J. V., Rall J. A., Wilson M. G., Woledge R. C. Absolute values of myothermic measurements on single muscle fibres from frog. J Muscle Res Cell Motil. 1986 Aug;7(4):327–332. doi: 10.1007/BF01753653. [DOI] [PubMed] [Google Scholar]
- Curtin N. A., Woledge R. C. Chemical change and energy production during contraction of frog muscle: how are their time courses related? J Physiol. 1979 Mar;288:353–366. [PMC free article] [PubMed] [Google Scholar]
- Edman K. A. The velocity of unloaded shortening and its relation to sarcomere length and isometric force in vertebrate muscle fibres. J Physiol. 1979 Jun;291:143–159. doi: 10.1113/jphysiol.1979.sp012804. [DOI] [PMC free article] [PubMed] [Google Scholar]
- Elzinga G., Howarth J. V., Rall J. A., Wilson M. G., Woledge R. C. Variation in the normalized tetanic force of single frog muscle fibres. J Physiol. 1989 Mar;410:157–170. doi: 10.1113/jphysiol.1989.sp017526. [DOI] [PMC free article] [PubMed] [Google Scholar]
- Fabiato A., Fabiato F. Calculator programs for computing the composition of the solutions containing multiple metals and ligands used for experiments in skinned muscle cells. J Physiol (Paris) 1979;75(5):463–505. [PubMed] [Google Scholar]
- Godt R. E., Lindley B. D. Influence of temperature upon contractile activation and isometric force production in mechanically skinned muscle fibers of the frog. J Gen Physiol. 1982 Aug;80(2):279–297. doi: 10.1085/jgp.80.2.279. [DOI] [PMC free article] [PubMed] [Google Scholar]
- Godt R. E., Maughan D. W. Swelling of skinned muscle fibers of the frog. Experimental observations. Biophys J. 1977 Aug;19(2):103–116. doi: 10.1016/S0006-3495(77)85573-2. [DOI] [PMC free article] [PubMed] [Google Scholar]
- Goldman Y. E., Simmons R. M. The stiffness of frog skinned muscle fibres at altered lateral filament spacing. J Physiol. 1986 Sep;378:175–194. doi: 10.1113/jphysiol.1986.sp016213. [DOI] [PMC free article] [PubMed] [Google Scholar]
- Hibberd M. G., Dantzig J. A., Trentham D. R., Goldman Y. E. Phosphate release and force generation in skeletal muscle fibers. Science. 1985 Jun 14;228(4705):1317–1319. doi: 10.1126/science.3159090. [DOI] [PubMed] [Google Scholar]
- Kentish J. C. The effects of inorganic phosphate and creatine phosphate on force production in skinned muscles from rat ventricle. J Physiol. 1986 Jan;370:585–604. doi: 10.1113/jphysiol.1986.sp015952. [DOI] [PMC free article] [PubMed] [Google Scholar]
- Levy R. M., Umazume Y., Kushmerick M. J. Ca2+ dependence of tension and ADP production in segments of chemically skinned muscle fibers. Biochim Biophys Acta. 1976 May 14;430(2):352–365. doi: 10.1016/0005-2728(76)90091-8. [DOI] [PubMed] [Google Scholar]
- Matsubara I., Elliott G. F. X-ray diffraction studies on skinned single fibres of frog skeletal muscle. J Mol Biol. 1972 Dec 30;72(3):657–669. doi: 10.1016/0022-2836(72)90183-0. [DOI] [PubMed] [Google Scholar]
- Moisescu D. G., Thieleczek R. Calcium and strontium concentration changes within skinned muscle preparations following a change in the external bathing solution. J Physiol. 1978 Feb;275:241–262. doi: 10.1113/jphysiol.1978.sp012188. [DOI] [PMC free article] [PubMed] [Google Scholar]
- Moss R. L. Sarcomere length-tension relations of frog skinned muscle fibres during calcium activation at short lengths. J Physiol. 1979 Jul;292:177–192. doi: 10.1113/jphysiol.1979.sp012845. [DOI] [PMC free article] [PubMed] [Google Scholar]
- Nosek T. M., Fender K. Y., Godt R. E. It is diprotonated inorganic phosphate that depresses force in skinned skeletal muscle fibers. Science. 1987 Apr 10;236(4798):191–193. doi: 10.1126/science.3563496. [DOI] [PubMed] [Google Scholar]
- Rüegg J. C., Schädler M., Steiger G. J., Müller G. Effects of inorganic phosphate on the contractile mechanism. Pflugers Arch. 1971;325(4):359–364. doi: 10.1007/BF00592176. [DOI] [PubMed] [Google Scholar]
- Stienen G. J., Güth K., Rüegg J. C. Force and force transients in skeletal muscle fibres of the frog skinned by freeze-drying. Pflugers Arch. 1983 Jun 1;397(4):272–276. doi: 10.1007/BF00580260. [DOI] [PubMed] [Google Scholar]
- Webb M. R., Hibberd M. G., Goldman Y. E., Trentham D. R. Oxygen exchange between Pi in the medium and water during ATP hydrolysis mediated by skinned fibers from rabbit skeletal muscle. Evidence for Pi binding to a force-generating state. J Biol Chem. 1986 Nov 25;261(33):15557–15564. [PubMed] [Google Scholar]
- Winegrad S., Weisberg A. Isozyme specific modification of myosin ATPase by cAMP in rat heart. Circ Res. 1987 Mar;60(3):384–392. doi: 10.1161/01.res.60.3.384. [DOI] [PubMed] [Google Scholar]
- Woledge R. C., Reilly P. J. Molar enthalpy change for hydrolysis of phosphorylcreatine under conditions in muscle cells. Biophys J. 1988 Jul;54(1):97–104. doi: 10.1016/S0006-3495(88)82934-5. [DOI] [PMC free article] [PubMed] [Google Scholar]
- Yoshizaki K., Seo Y., Nishikawa H., Morimoto T. Application of pulsed-gradient 31P NMR on frog muscle to measure the diffusion rates of phosphorus compounds in cells. Biophys J. 1982 May;38(2):209–211. doi: 10.1016/S0006-3495(82)84549-9. [DOI] [PMC free article] [PubMed] [Google Scholar]