Abstract
1. Patch-clamp techniques were used to study the properties of a Na+-activated K+ current (IK(Na) in neurones cultured from embryonic chick brain stem. 2. With whole-cell clamp, a depolarizing voltage command evoked an inward current that was followed by an outward current with two components, the first transient, the second sustained. 3. Tetrodotoxin (TTX, 1 microM) eliminated the inward current and the transient component of the outward current, without affecting the sustained outward current. In addition, the transient outward current was attenuated when all external Na+ was replaced by Li+, suggesting that it was activated specifically by Na+ entry into the cell. 4. The time course of the transient outward current was obtained by subtracting records obtained in Li+ solution from those obtained in Na+ solution. There was significant overlap between the decay of the inward current and the onset of the transient outward current. 5. When just after the peak of the transient outward current, the membrane was stepped to progressively more hyperpolarized levels, the tail currents associated with the current reversed polarity near the calculated K+ equilibrium potential. 6. 4-Aminopyridine (4-AP, 4 mM) abolished the transient outward current and approximately half of the sustained late current. Tetraethylammonium (TEA, 2 mM) had no effect on the transient current, but reduced the sustained current slightly. 7. Inside-out patches, made in LiCl bathing solutions, contained channels that were activated by exposing the cytoplasmic face of the patch to Na+. Channel activity continued as long as Na+ was present. 8. The single-channel currents reversed at the K+ equilibrium potential, and were associated with a main conductance that depended upon K+ concentration (about 50 pS with [K+]o = 15 mM, [K+]i = 5 mM, and 100 pS when [K+]i was increased to 75 mM). 9. The open probability of the channels increased with increasing cytoplasmic Na+ concentration. At [Na+]i = 150 mM (the maximum concentration tested), channels were open almost continuously. Open probability was considerably less at 50 mM, and still measureable at 20 mM. 10. The magnitude of IK(Na) and its overlap with the inward Na+ current indicate that these channels contribute significantly to the repolarizing phase of the action potential. In addition, the relation between channel activity and Na+ concentration suggests that the channels may make a measurable contribution to membrane conductance at resting intracellular Na+ concentrations.
Full text
PDF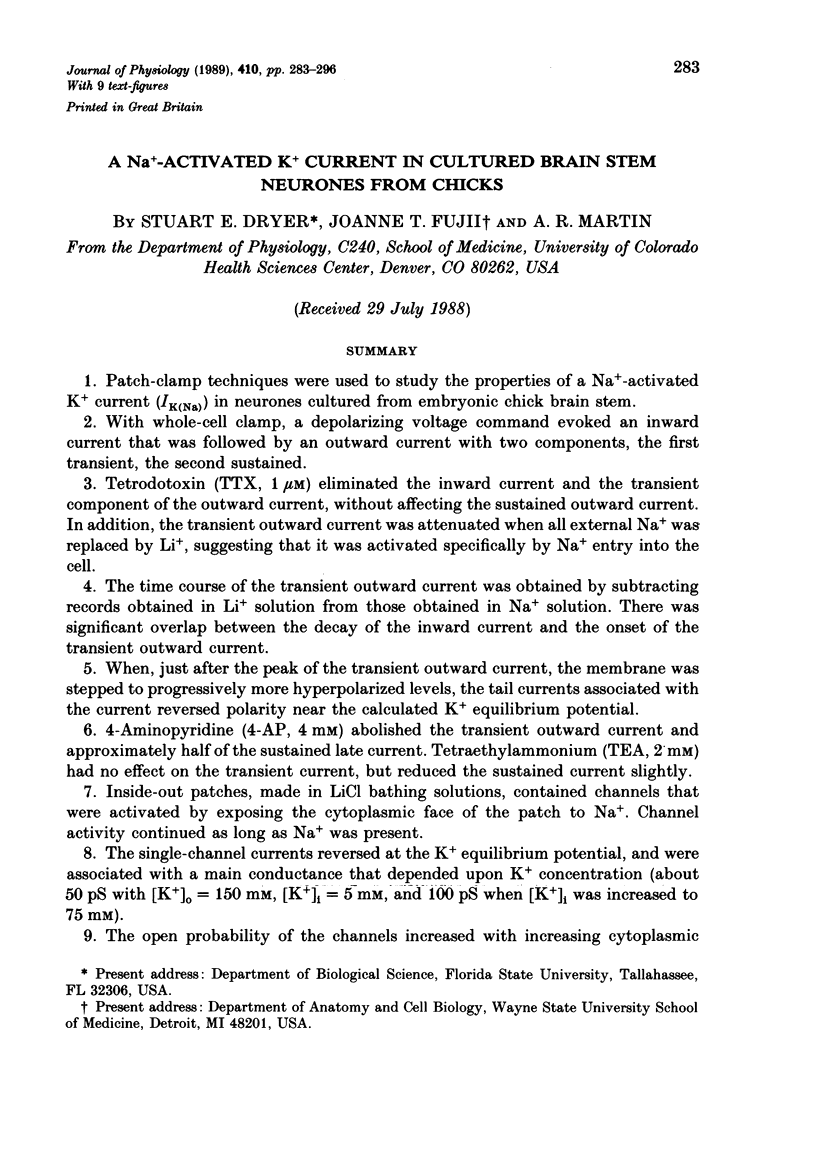
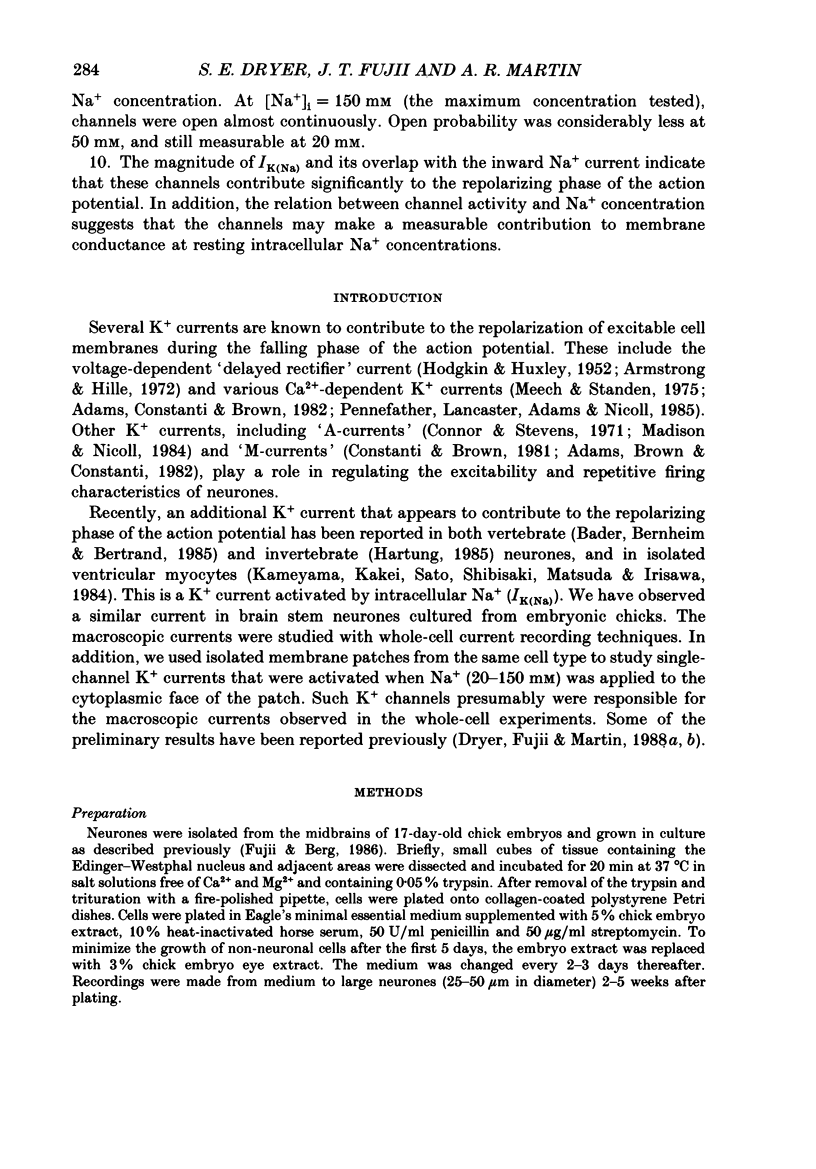
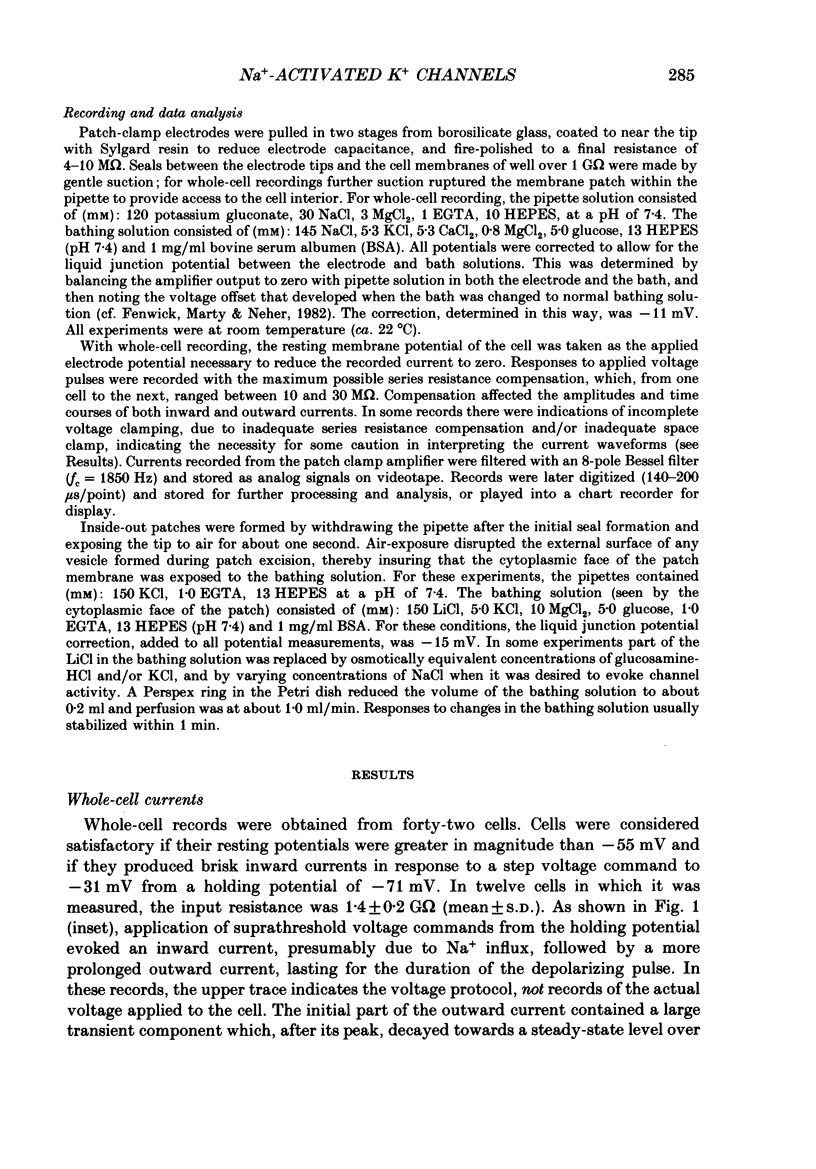
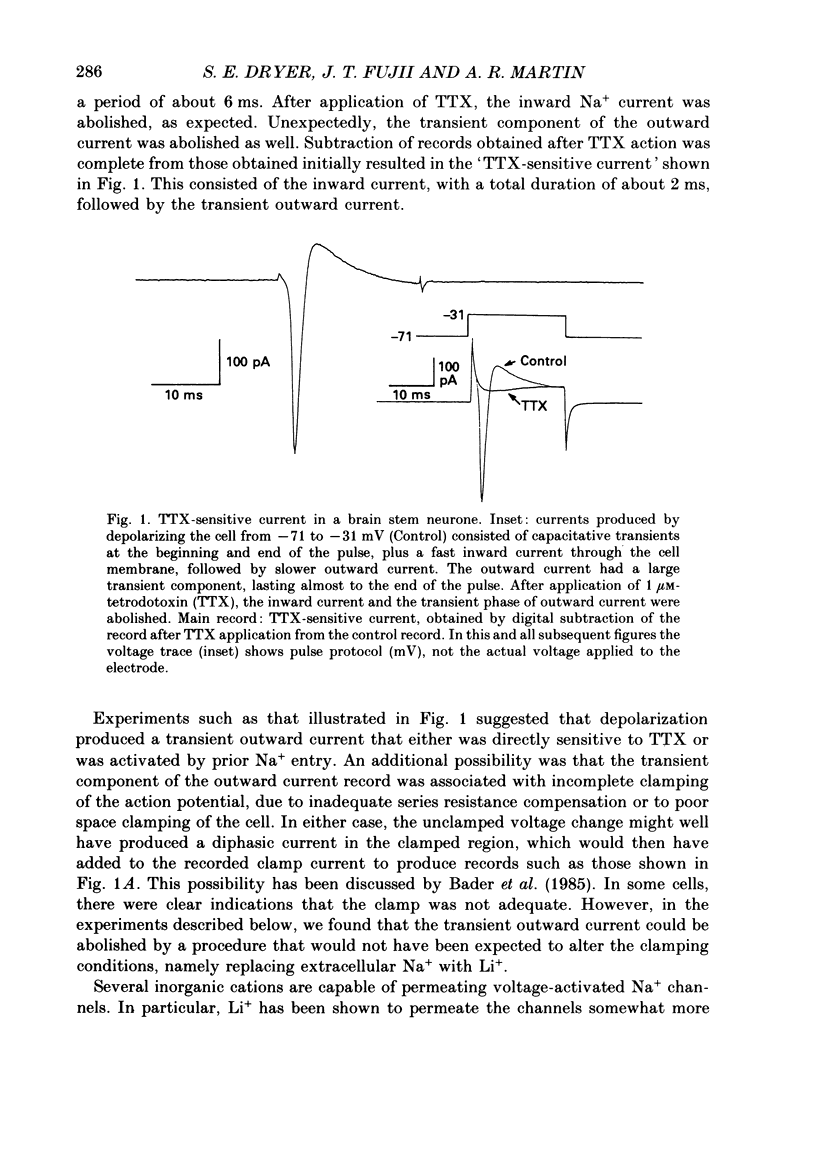
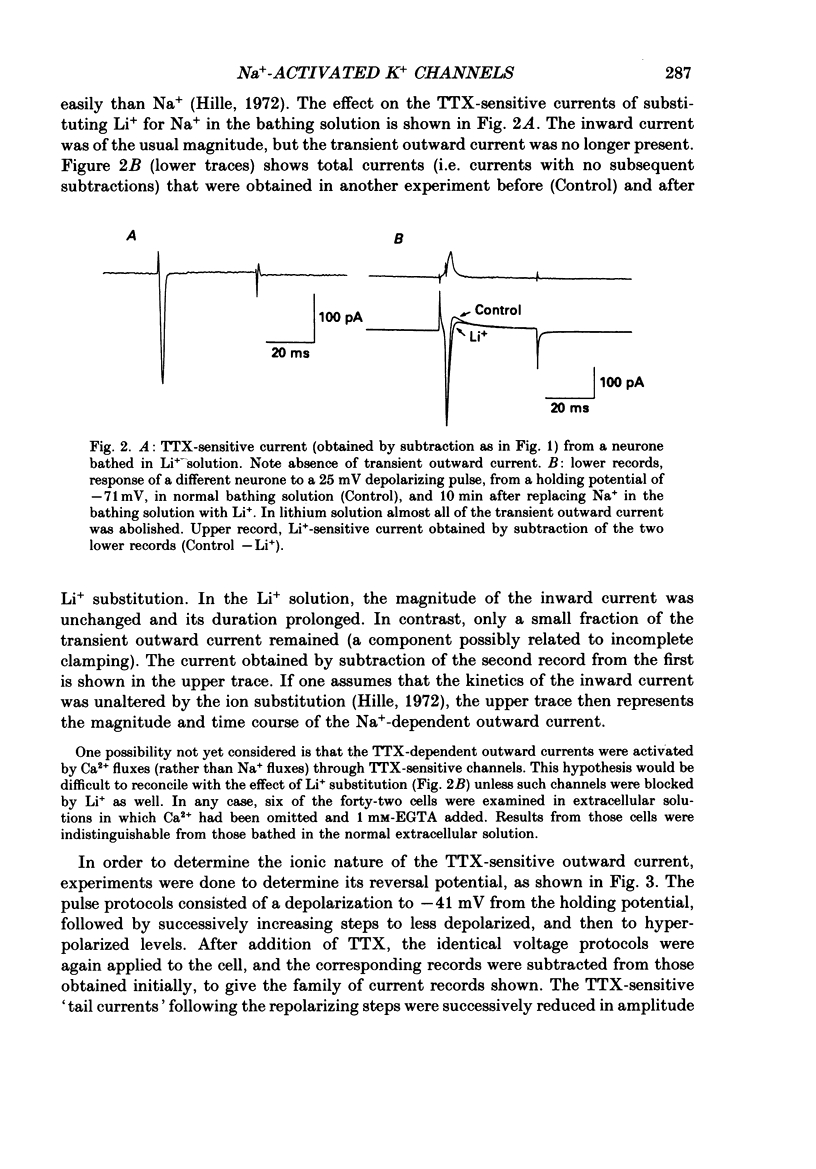
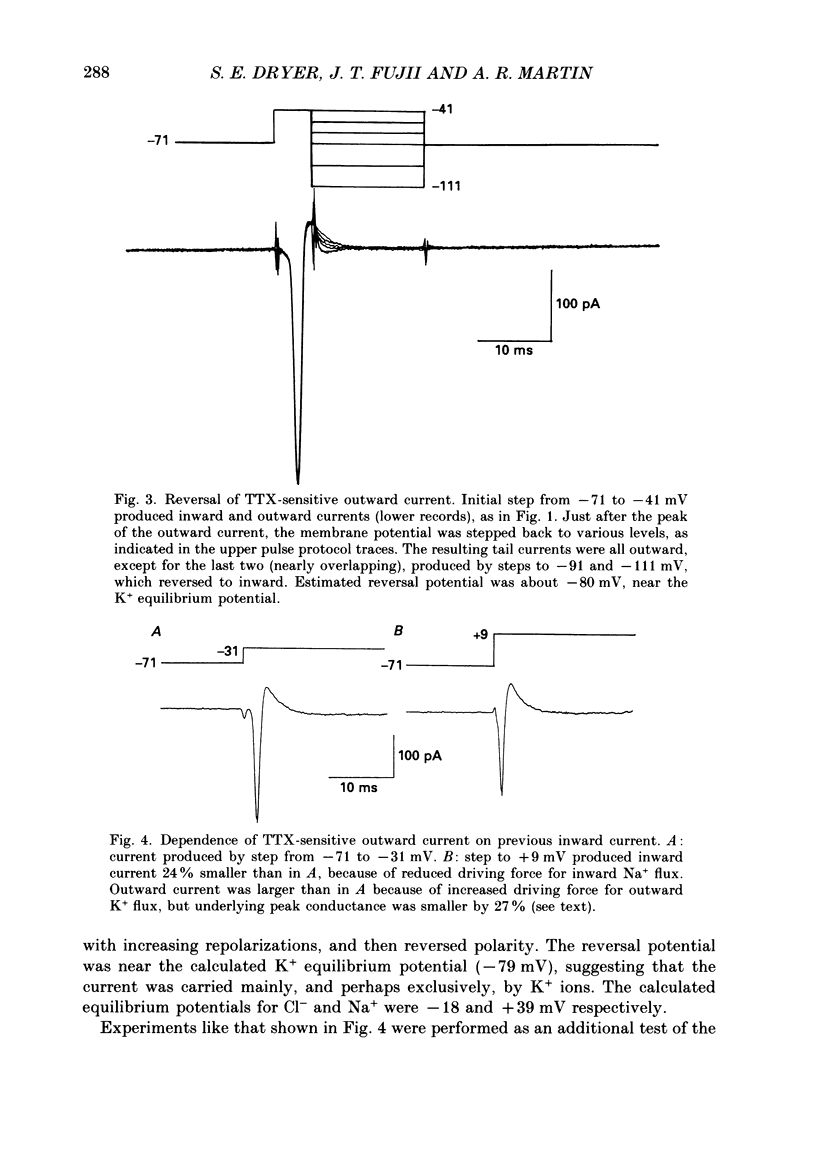
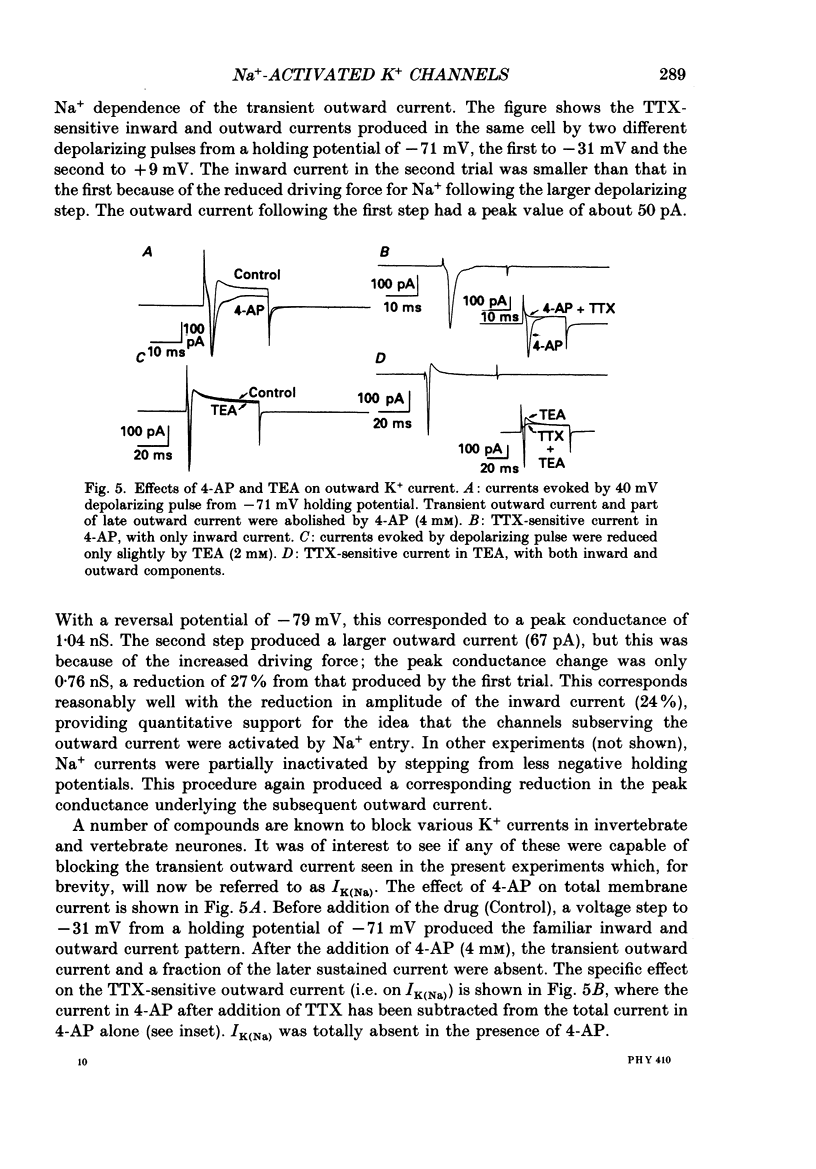
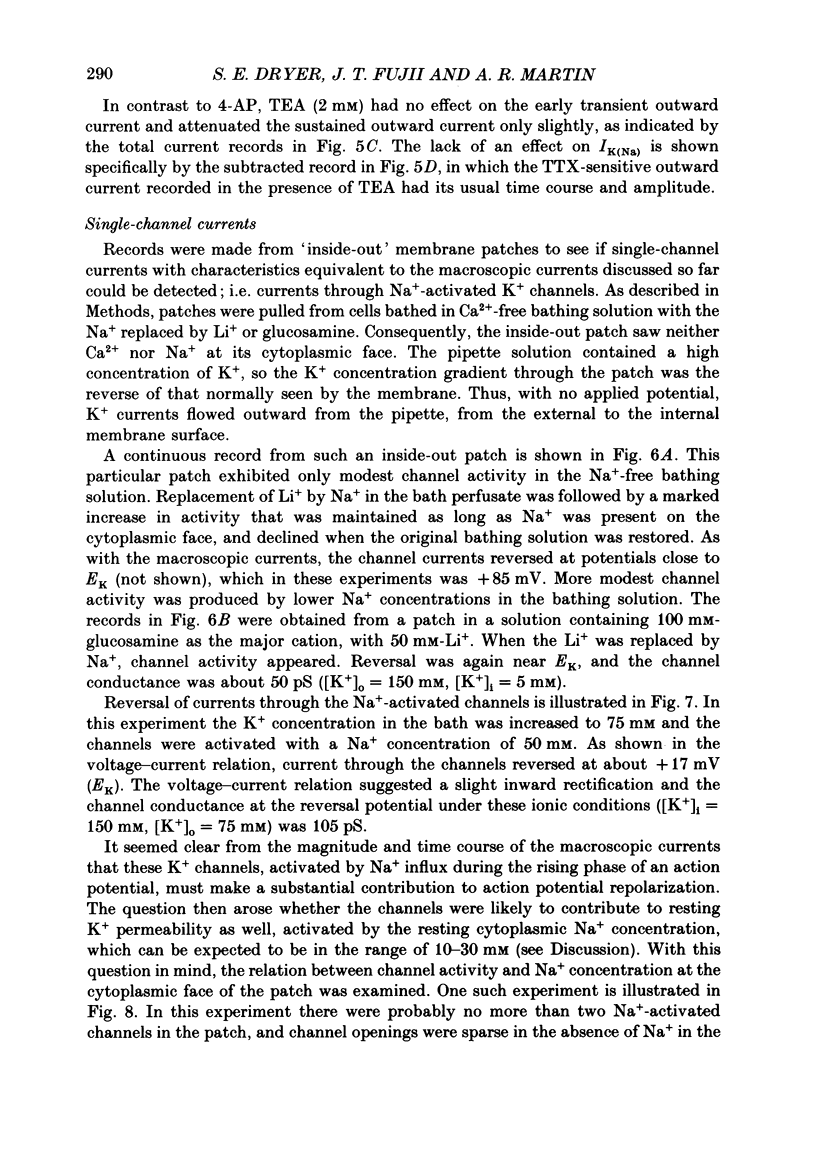
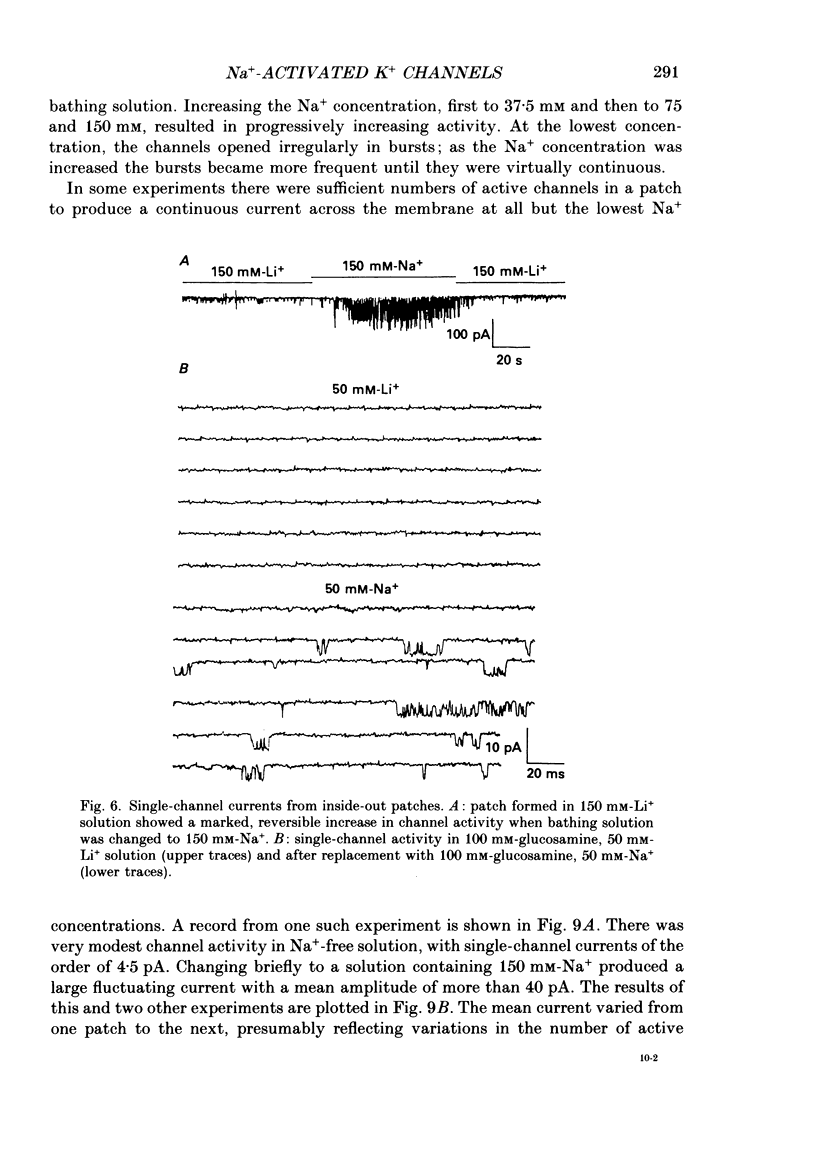
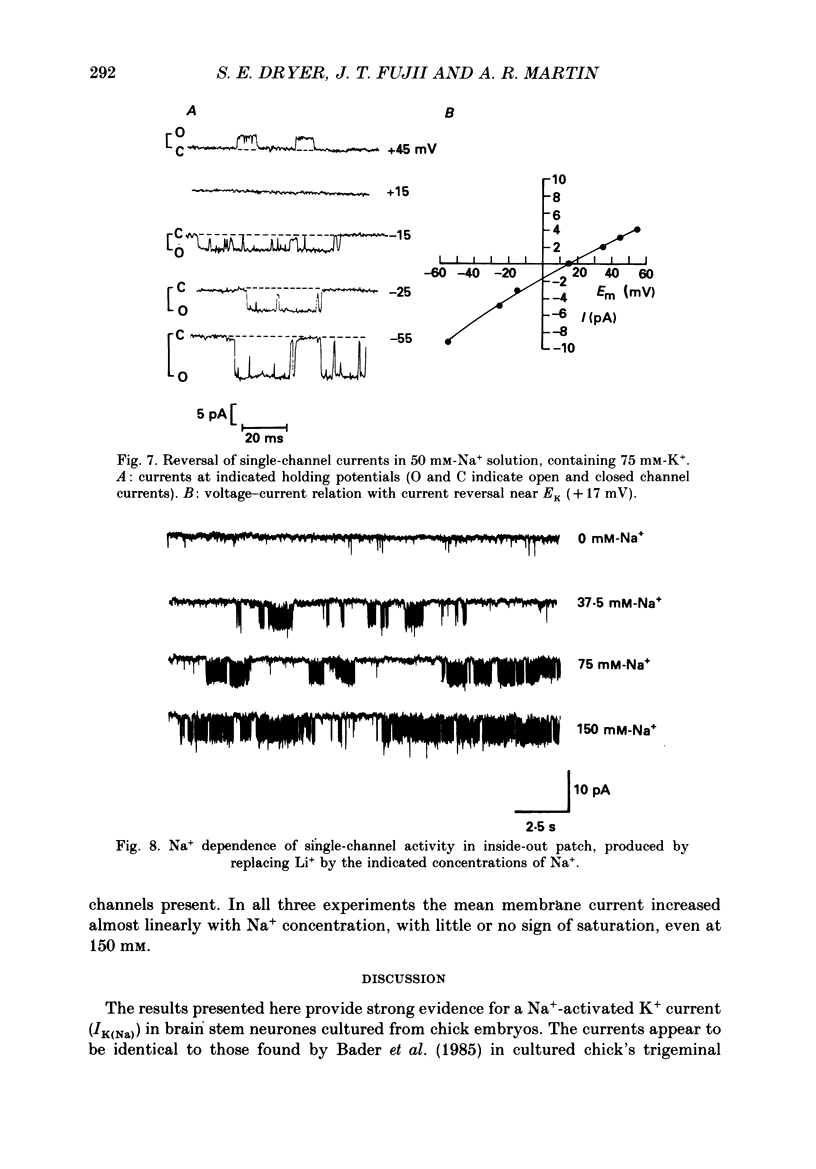
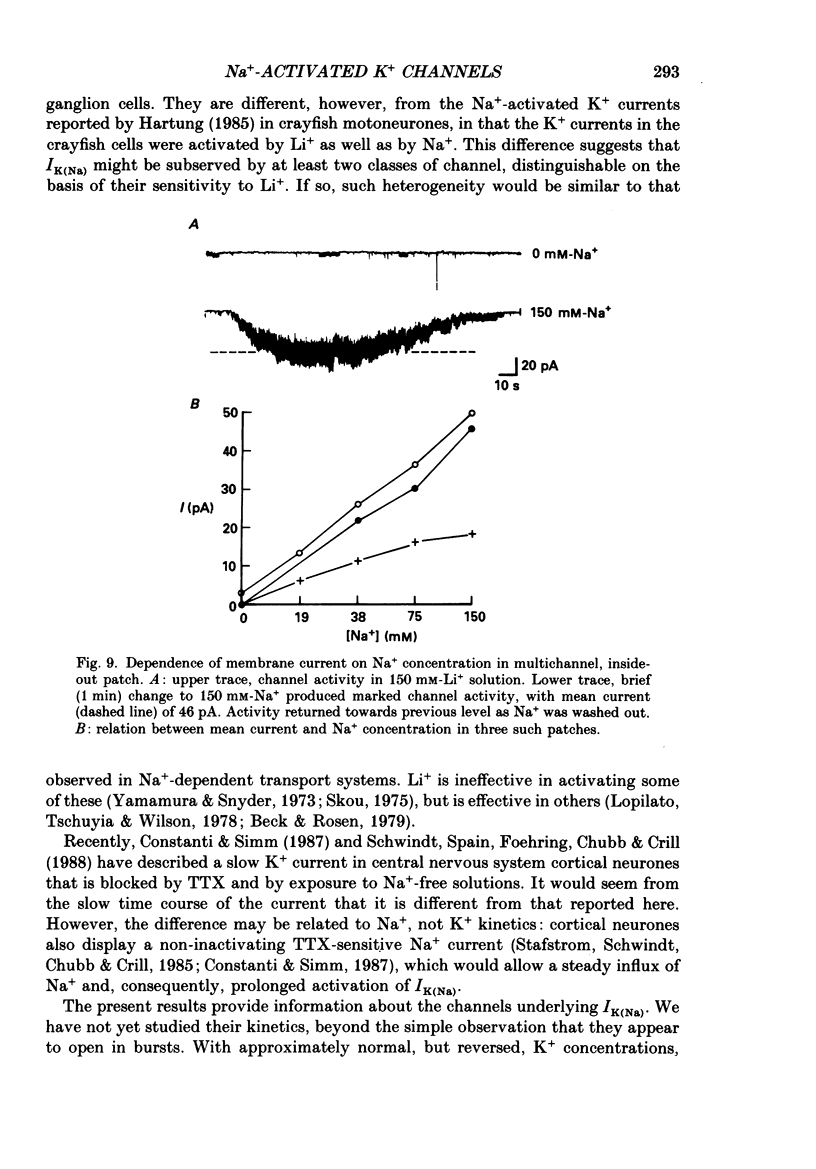
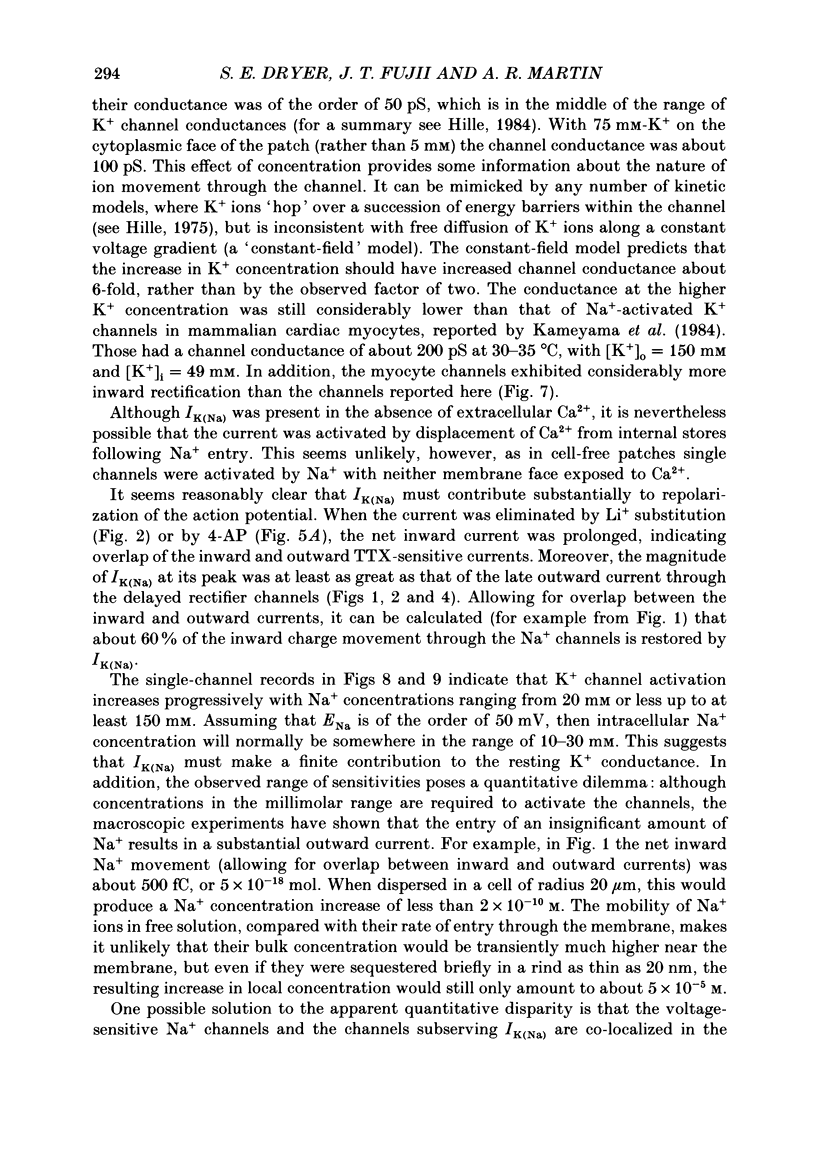
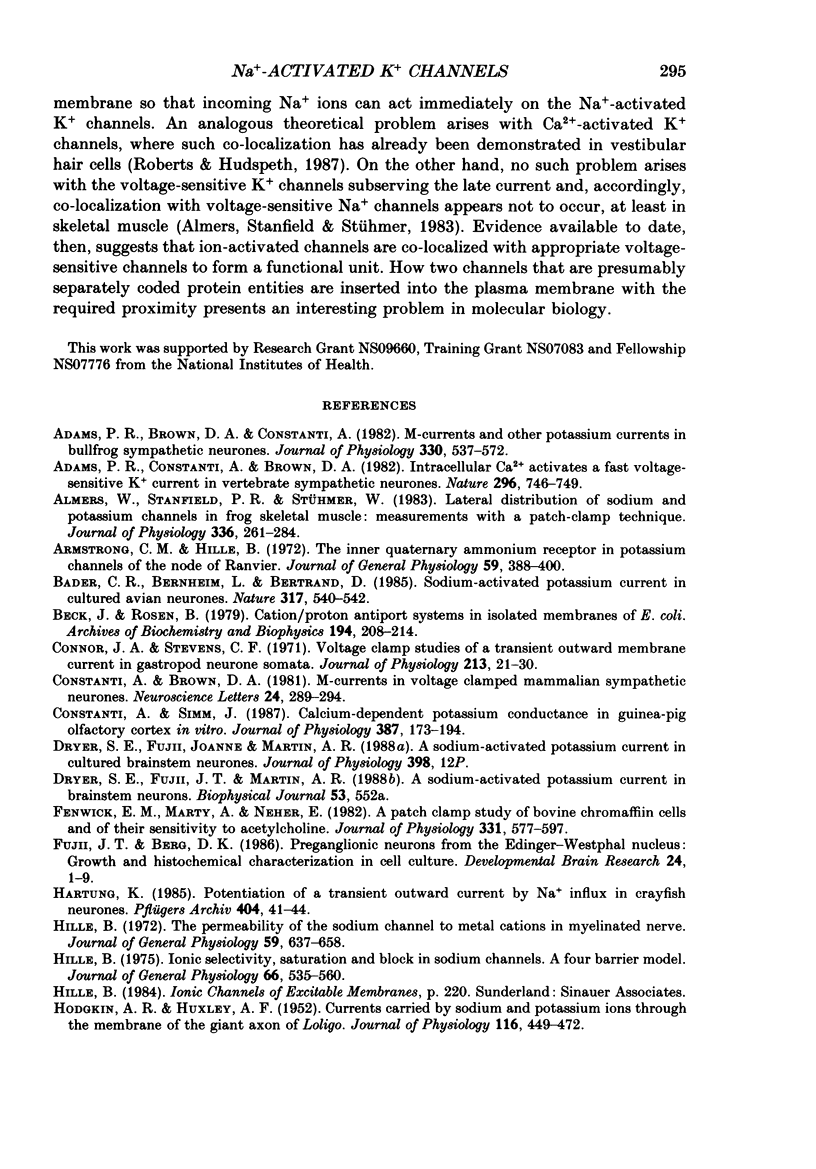
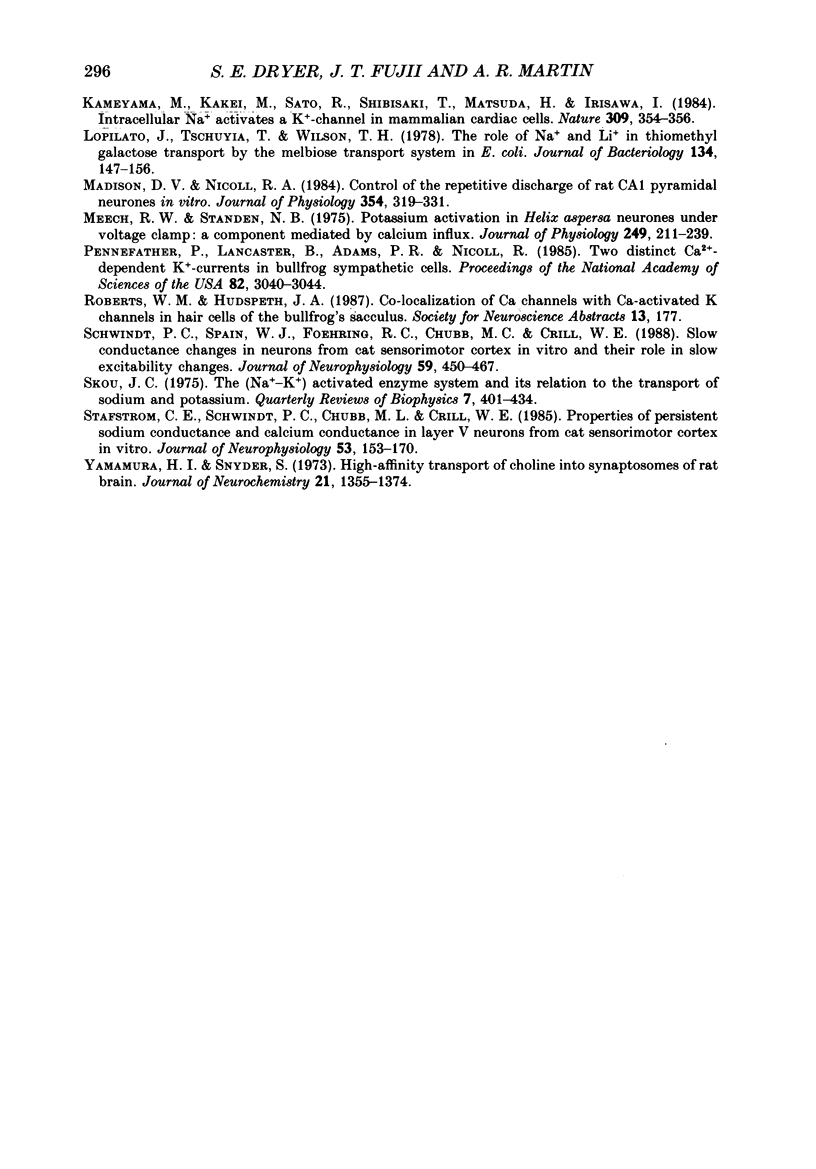
Selected References
These references are in PubMed. This may not be the complete list of references from this article.
- Adams P. R., Brown D. A., Constanti A. M-currents and other potassium currents in bullfrog sympathetic neurones. J Physiol. 1982 Sep;330:537–572. doi: 10.1113/jphysiol.1982.sp014357. [DOI] [PMC free article] [PubMed] [Google Scholar]
- Adams P. R., Constanti A., Brown D. A., Clark R. B. Intracellular Ca2+ activates a fast voltage-sensitive K+ current in vertebrate sympathetic neurones. Nature. 1982 Apr 22;296(5859):746–749. doi: 10.1038/296746a0. [DOI] [PubMed] [Google Scholar]
- Almers W., Stanfield P. R., Stühmer W. Lateral distribution of sodium and potassium channels in frog skeletal muscle: measurements with a patch-clamp technique. J Physiol. 1983 Mar;336:261–284. doi: 10.1113/jphysiol.1983.sp014580. [DOI] [PMC free article] [PubMed] [Google Scholar]
- Armstrong C. M., Hille B. The inner quaternary ammonium ion receptor in potassium channels of the node of Ranvier. J Gen Physiol. 1972 Apr;59(4):388–400. doi: 10.1085/jgp.59.4.388. [DOI] [PMC free article] [PubMed] [Google Scholar]
- Bader C. R., Bernheim L., Bertrand D. Sodium-activated potassium current in cultured avian neurones. Nature. 1985 Oct 10;317(6037):540–542. doi: 10.1038/317540a0. [DOI] [PubMed] [Google Scholar]
- Beck J. C., Rosen B. P. Cation/proton antiport systems in escherichia coli: properties of the sodium/proton antiporter. Arch Biochem Biophys. 1979 Apr 15;194(1):208–214. doi: 10.1016/0003-9861(79)90611-8. [DOI] [PubMed] [Google Scholar]
- Connor J. A., Stevens C. F. Voltage clamp studies of a transient outward membrane current in gastropod neural somata. J Physiol. 1971 Feb;213(1):21–30. doi: 10.1113/jphysiol.1971.sp009365. [DOI] [PMC free article] [PubMed] [Google Scholar]
- Constanti A., Brown D. A. M-Currents in voltage-clamped mammalian sympathetic neurones. Neurosci Lett. 1981 Jul 17;24(3):289–294. doi: 10.1016/0304-3940(81)90173-7. [DOI] [PubMed] [Google Scholar]
- Constanti A., Sim J. A. Calcium-dependent potassium conductance in guinea-pig olfactory cortex neurones in vitro. J Physiol. 1987 Jun;387:173–194. doi: 10.1113/jphysiol.1987.sp016569. [DOI] [PMC free article] [PubMed] [Google Scholar]
- Fenwick E. M., Marty A., Neher E. A patch-clamp study of bovine chromaffin cells and of their sensitivity to acetylcholine. J Physiol. 1982 Oct;331:577–597. doi: 10.1113/jphysiol.1982.sp014393. [DOI] [PMC free article] [PubMed] [Google Scholar]
- Fujii J. T., Berg D. K. Preganglionic neurons from the Edinger-Westphal nucleus: growth and histochemical characterization in cell culture. Brain Res. 1986 Jan;389(1-2):1–9. doi: 10.1016/0165-3806(86)90167-7. [DOI] [PubMed] [Google Scholar]
- HODGKIN A. L., HUXLEY A. F. Currents carried by sodium and potassium ions through the membrane of the giant axon of Loligo. J Physiol. 1952 Apr;116(4):449–472. doi: 10.1113/jphysiol.1952.sp004717. [DOI] [PMC free article] [PubMed] [Google Scholar]
- Hartung K. Potentiation of a transient outward current by Na+ influx in crayfish neurones. Pflugers Arch. 1985 May;404(1):41–44. doi: 10.1007/BF00581488. [DOI] [PubMed] [Google Scholar]
- Hille B. Ionic selectivity, saturation, and block in sodium channels. A four-barrier model. J Gen Physiol. 1975 Nov;66(5):535–560. doi: 10.1085/jgp.66.5.535. [DOI] [PMC free article] [PubMed] [Google Scholar]
- Hille B. The permeability of the sodium channel to metal cations in myelinated nerve. J Gen Physiol. 1972 Jun;59(6):637–658. doi: 10.1085/jgp.59.6.637. [DOI] [PMC free article] [PubMed] [Google Scholar]
- Kameyama M., Kakei M., Sato R., Shibasaki T., Matsuda H., Irisawa H. Intracellular Na+ activates a K+ channel in mammalian cardiac cells. Nature. 1984 May 24;309(5966):354–356. doi: 10.1038/309354a0. [DOI] [PubMed] [Google Scholar]
- Lopilato J., Tsuchiya T., Wilson T. H. Role of Na+ and Li+ in thiomethylgalactoside transport by the melibiose transport system of Escherichia coli. J Bacteriol. 1978 Apr;134(1):147–156. doi: 10.1128/jb.134.1.147-156.1978. [DOI] [PMC free article] [PubMed] [Google Scholar]
- Madison D. V., Nicoll R. A. Control of the repetitive discharge of rat CA 1 pyramidal neurones in vitro. J Physiol. 1984 Sep;354:319–331. doi: 10.1113/jphysiol.1984.sp015378. [DOI] [PMC free article] [PubMed] [Google Scholar]
- Meech R. W., Standen N. B. Potassium activation in Helix aspersa neurones under voltage clamp: a component mediated by calcium influx. J Physiol. 1975 Jul;249(2):211–239. doi: 10.1113/jphysiol.1975.sp011012. [DOI] [PMC free article] [PubMed] [Google Scholar]
- Pennefather P., Lancaster B., Adams P. R., Nicoll R. A. Two distinct Ca-dependent K currents in bullfrog sympathetic ganglion cells. Proc Natl Acad Sci U S A. 1985 May;82(9):3040–3044. doi: 10.1073/pnas.82.9.3040. [DOI] [PMC free article] [PubMed] [Google Scholar]
- Schwindt P. C., Spain W. J., Foehring R. C., Chubb M. C., Crill W. E. Slow conductances in neurons from cat sensorimotor cortex in vitro and their role in slow excitability changes. J Neurophysiol. 1988 Feb;59(2):450–467. doi: 10.1152/jn.1988.59.2.450. [DOI] [PubMed] [Google Scholar]
- Skou J. C. The (Na++K+) activated enzyme system and its relationship to transport of sodium and potassium. Q Rev Biophys. 1974 Jul;7(3):401–434. doi: 10.1017/s0033583500001475. [DOI] [PubMed] [Google Scholar]
- Stafstrom C. E., Schwindt P. C., Chubb M. C., Crill W. E. Properties of persistent sodium conductance and calcium conductance of layer V neurons from cat sensorimotor cortex in vitro. J Neurophysiol. 1985 Jan;53(1):153–170. doi: 10.1152/jn.1985.53.1.153. [DOI] [PubMed] [Google Scholar]
- Yamamura H. I., Snyder S. H. High affinity transport of choline into synaptosomes of rat brain. J Neurochem. 1973 Dec;21(6):1355–1374. doi: 10.1111/j.1471-4159.1973.tb06022.x. [DOI] [PubMed] [Google Scholar]