Abstract
1. Low-voltage-activated Ca2+ channels which produce a transient inward current were studied in neurones freshly isolated from the ventromedial hypothalamic region of the rat. Membrane currents were recorded using a suction-pipette technique which allows for internal perfusion under a single-electrode voltage clamp. A concentration-jump technique was also used for rapid drug application. 2. In most cells superfused with 10 mM-Ca2+, a transient inward Ca2+ current was evoked by a step depolarization to potentials more positive than -65 mV from a holding potential of -100 mV. Such a low-threshold Ca2+ current could easily be separated from a high-threshold, steady type of Ca2+ current by selecting the holding and test potential levels, as well as by resistance to the wash-out during cell dialysis. 3. Activation and inactivation processes of the low-threshold Ca2+ current were highly potential dependent at 20-22 degrees C. For a test potential change from -60 to +20 mV, the time to peak of the current decreased from 45 to 9 ms, and the time constant of the current decay decreased from 90 to 40 ms. The steady-state inactivation occurred at very negative potentials, reaching a 50% level at -93 mV. Recovery from inactivation showed a time constant between 2.63 and 0.94 s for a potential change from -80 to -120 mV. 4. The amplitude of the low-threshold Ca2+ current depended on the external Ca2+ concentration [( Ca2+]o), approaching saturation at 100 mM [Ca2+]o. Ba2+ substituted for Ca2+ reduced the current amplitude by 30-50% while Sr2+ produced no definite changes in the current amplitude. 5. The low-threshold Ca2+ current was blocked by various di- or trivalent cations in the sequence of La3+ greater than Zn2+ greater than Cd2+ greater than Ni2+ greater than Co2+. The corresponding apparent dissociation constants (KD) were 7 x 10(-7), 1 x 10(-4), 3 x 10(-4), 6 x 10(-4) and 3 x 10(-3) M. 6. Various organic Ca2+ antagonists were effective in blocking the low-threshold Ca2+ current in the following sequence: flunarizine greater than nicardipine greater than nifedipine greater than nimodipine greater than D600 (methoxyverapamil) greater than diltiazem. The corresponding KDs were 7 x 10(-7), 3.5 x 10(-6), 5 x 10(-6), 7 x 10(-6), 5 x 10(-5) and 7 x 10(-5) M. These Ca2+ antagonists induced a use-dependent decrease in the current amplitude.(ABSTRACT TRUNCATED AT 400 WORDS)
Full text
PDF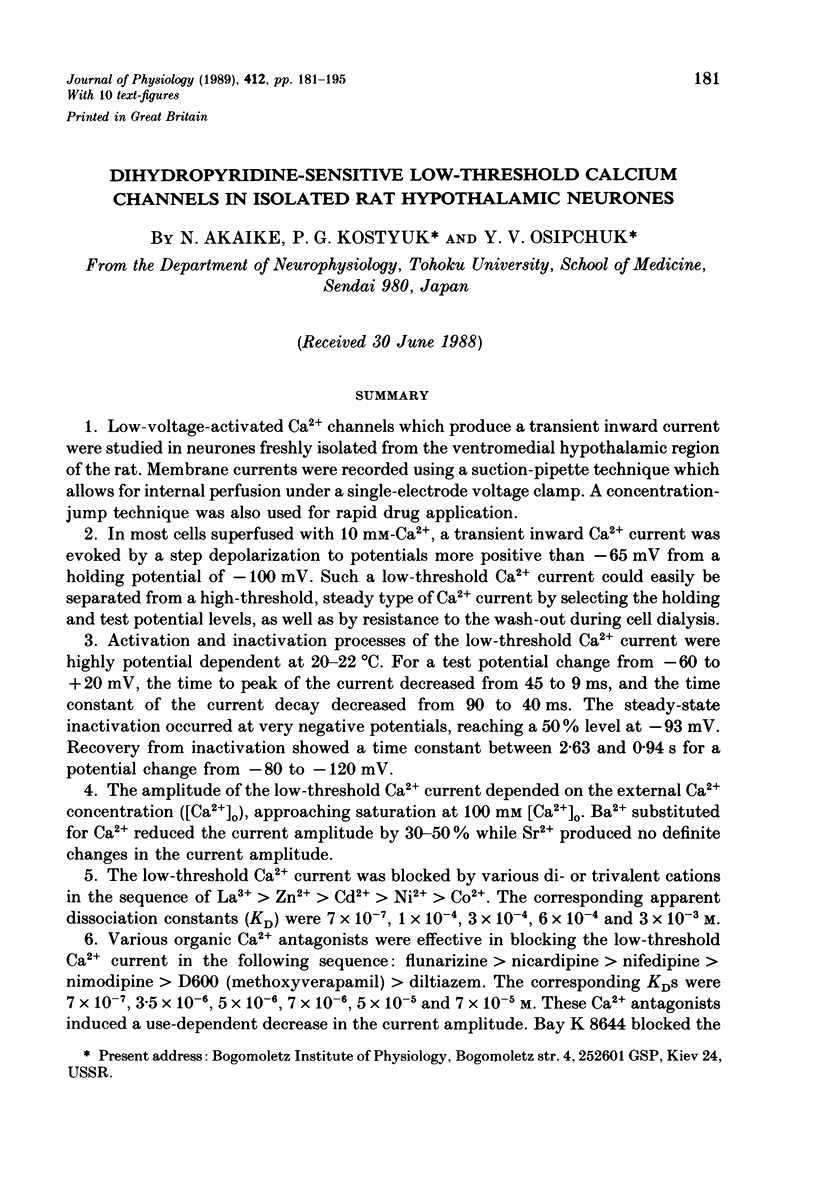
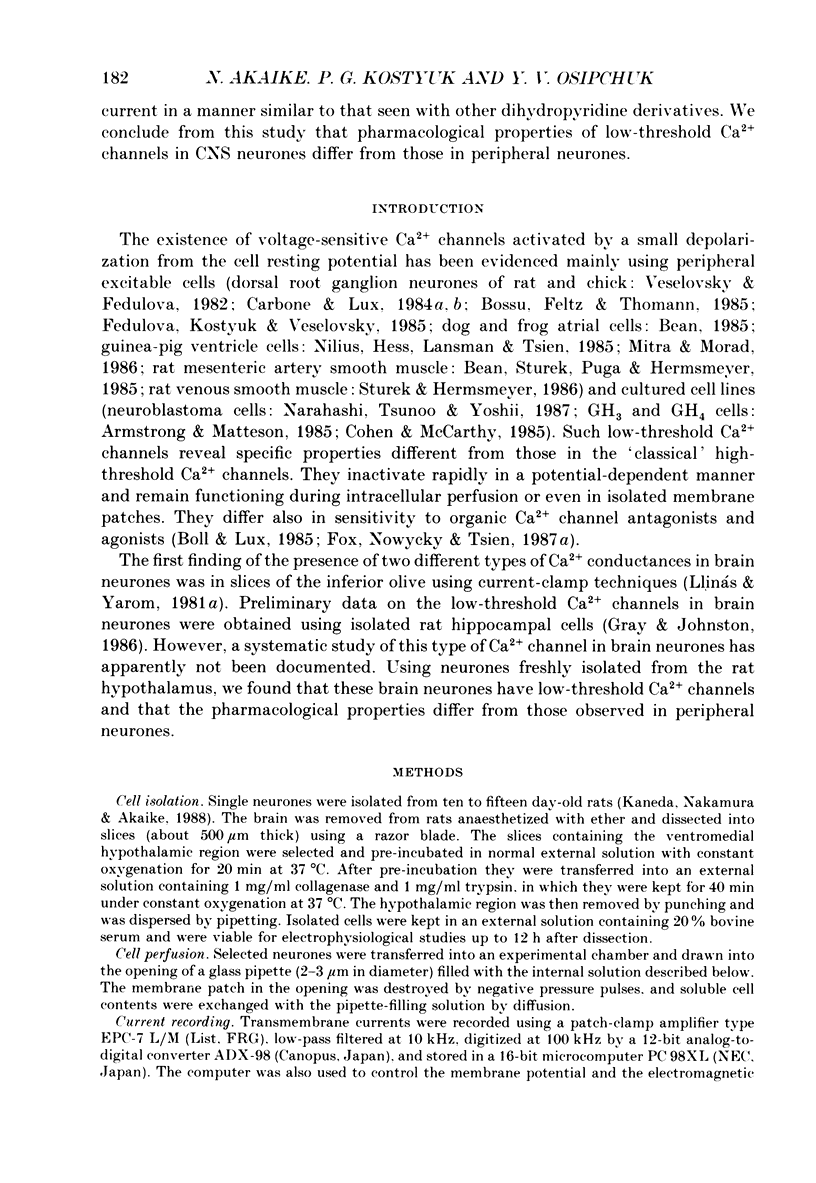
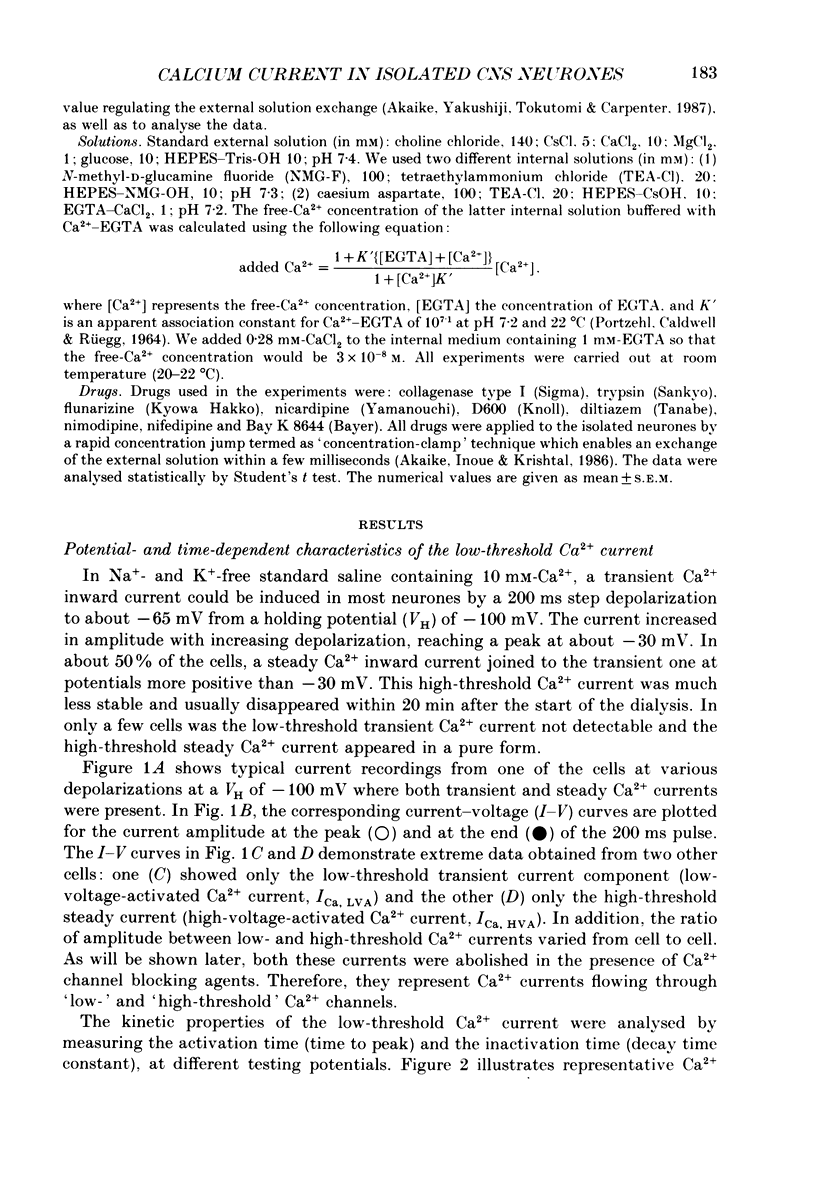
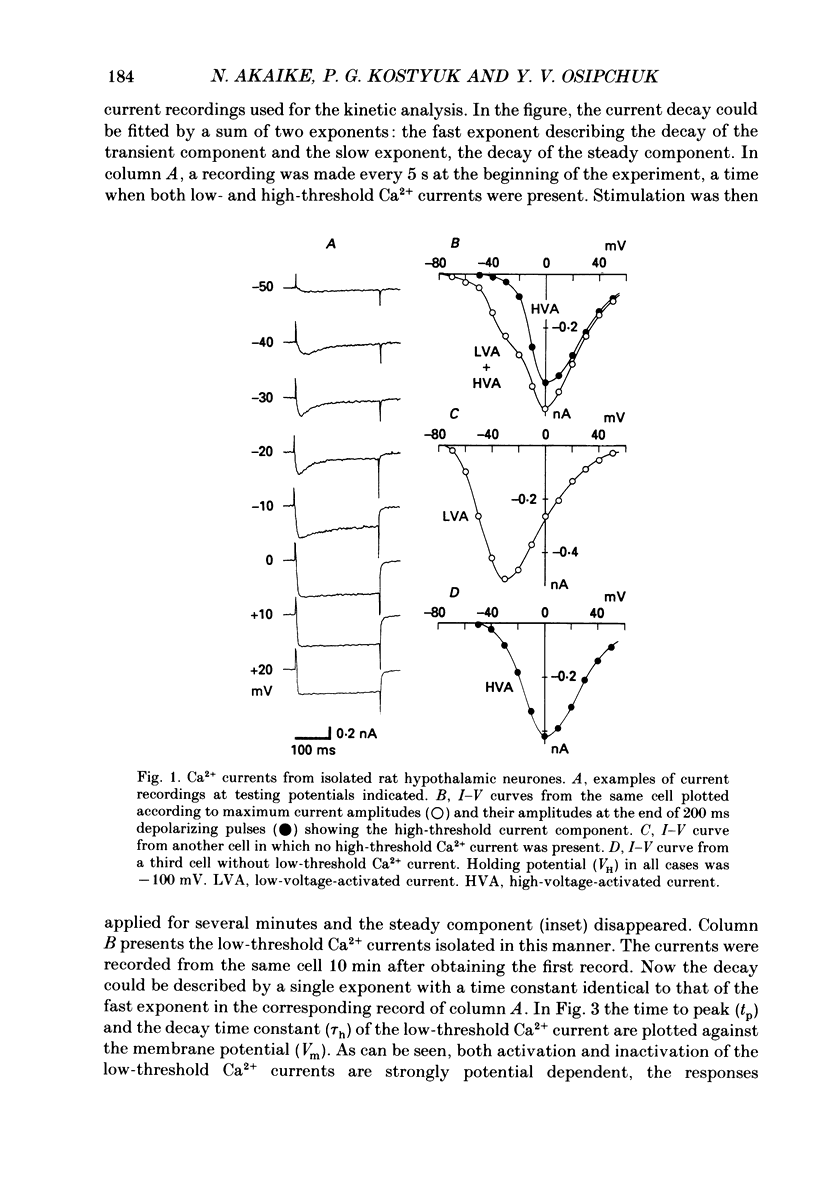
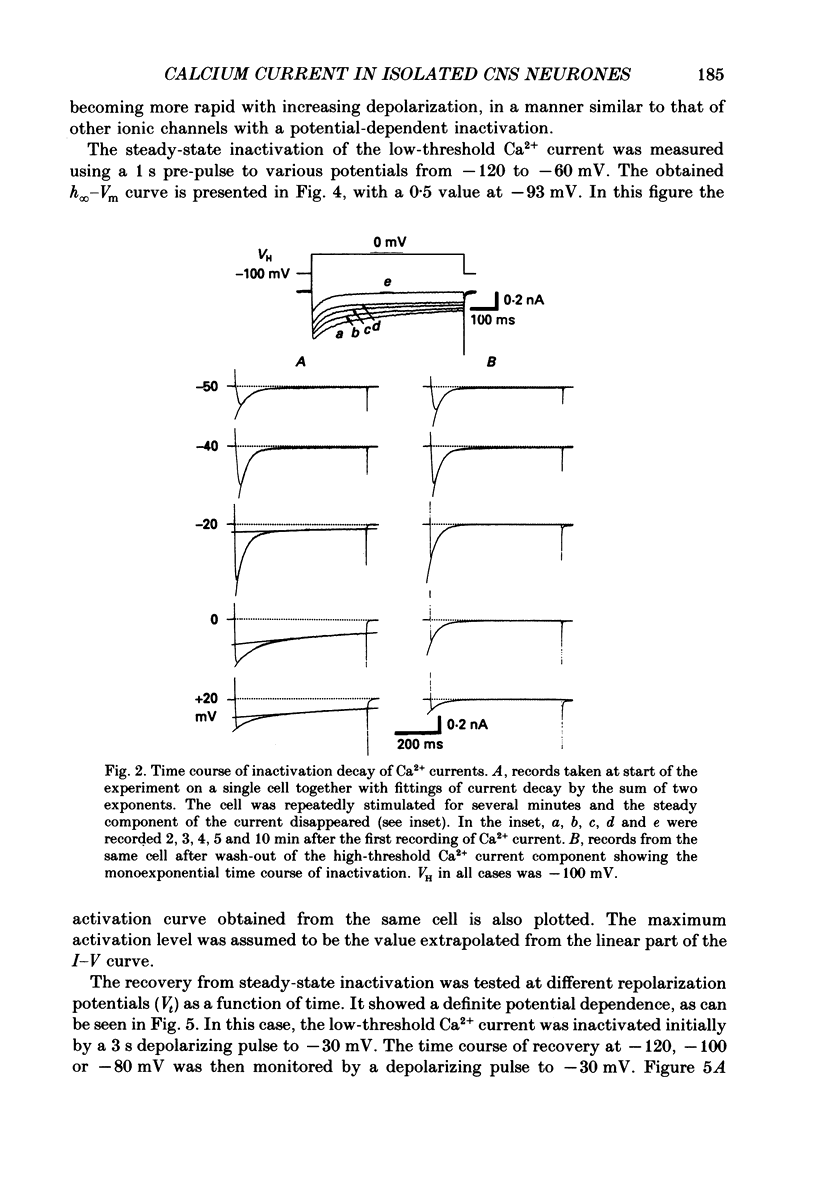
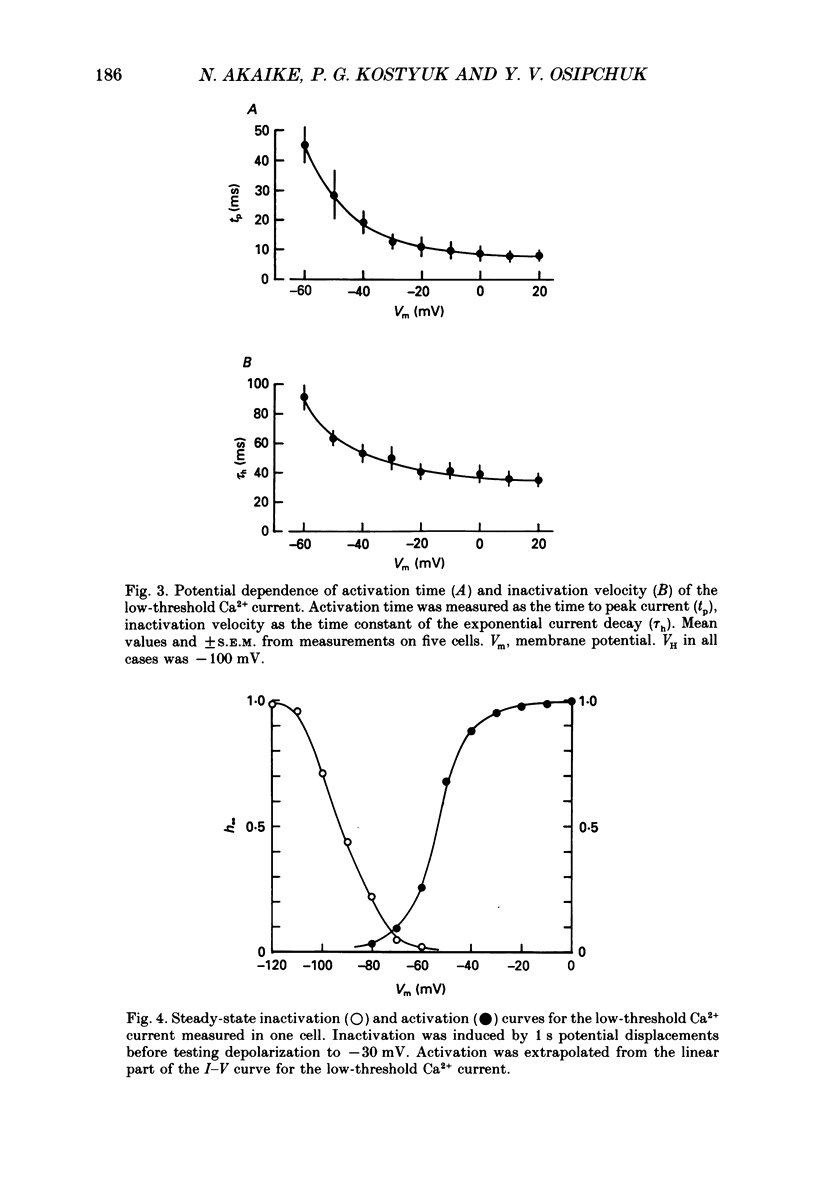
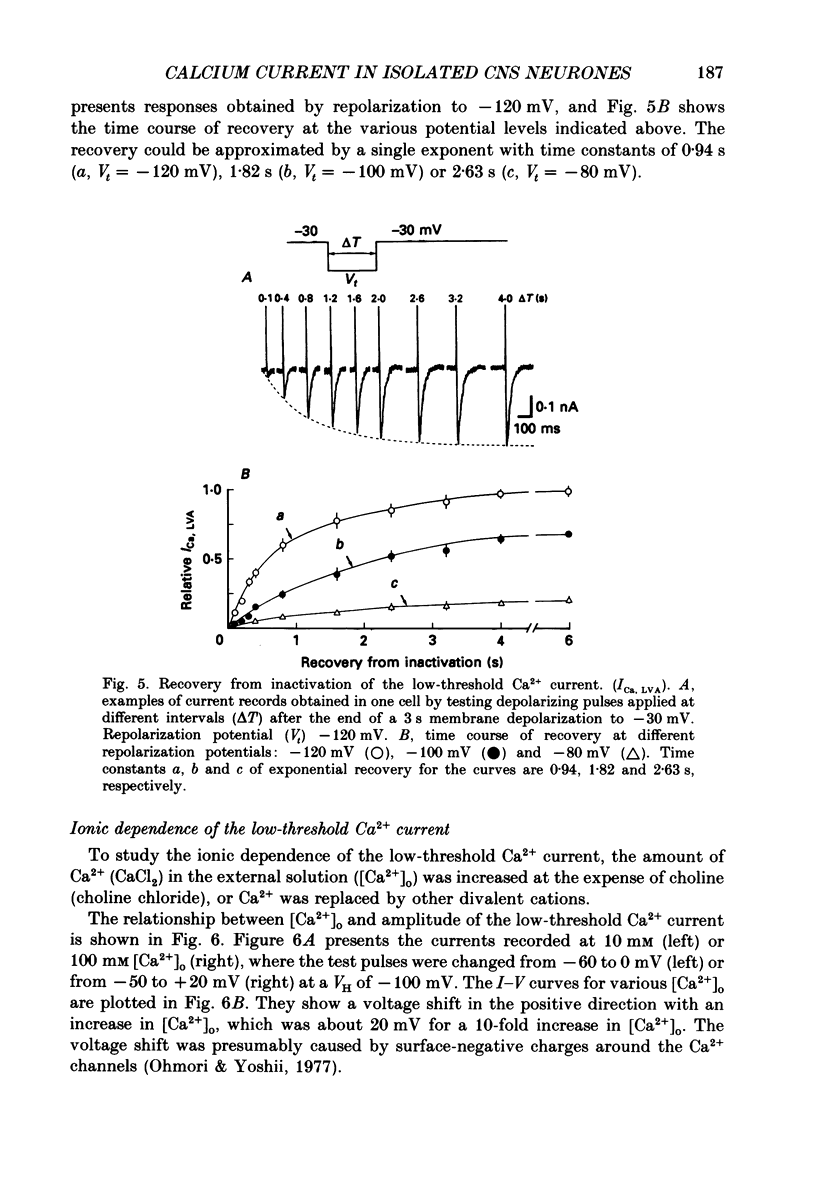
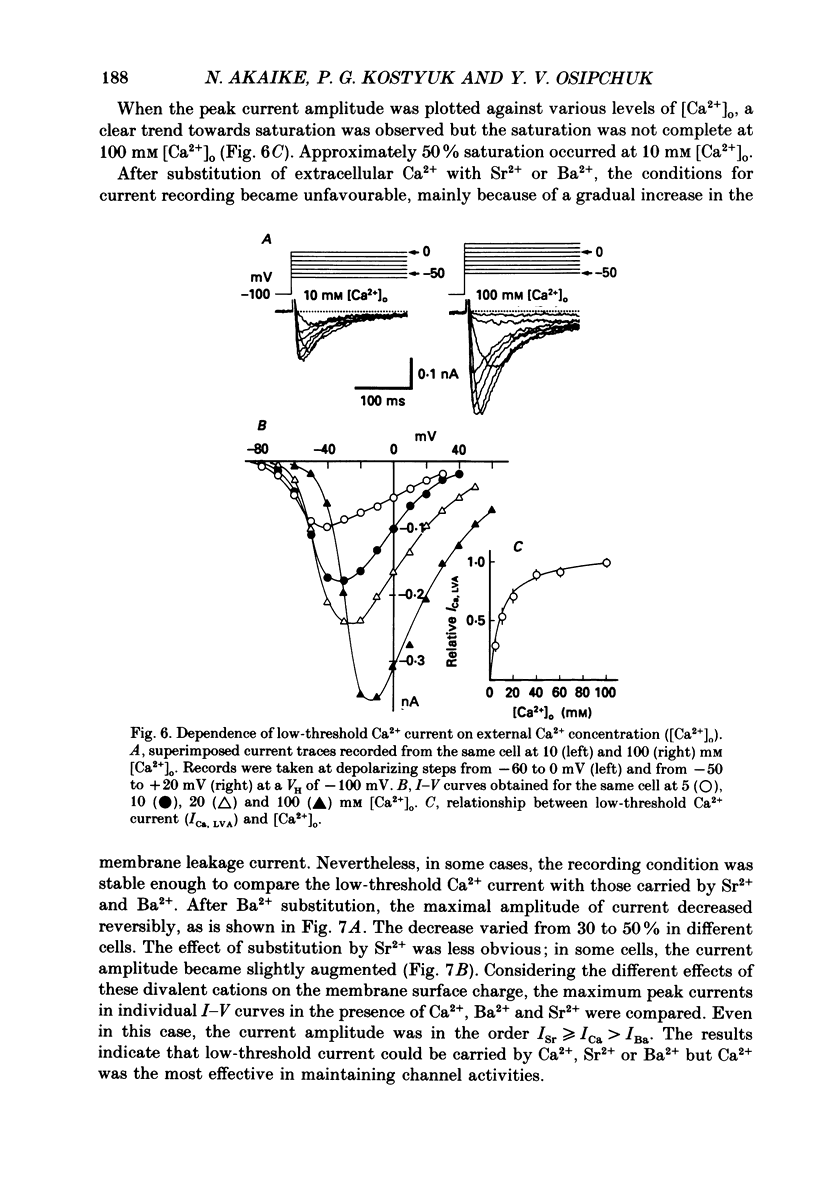
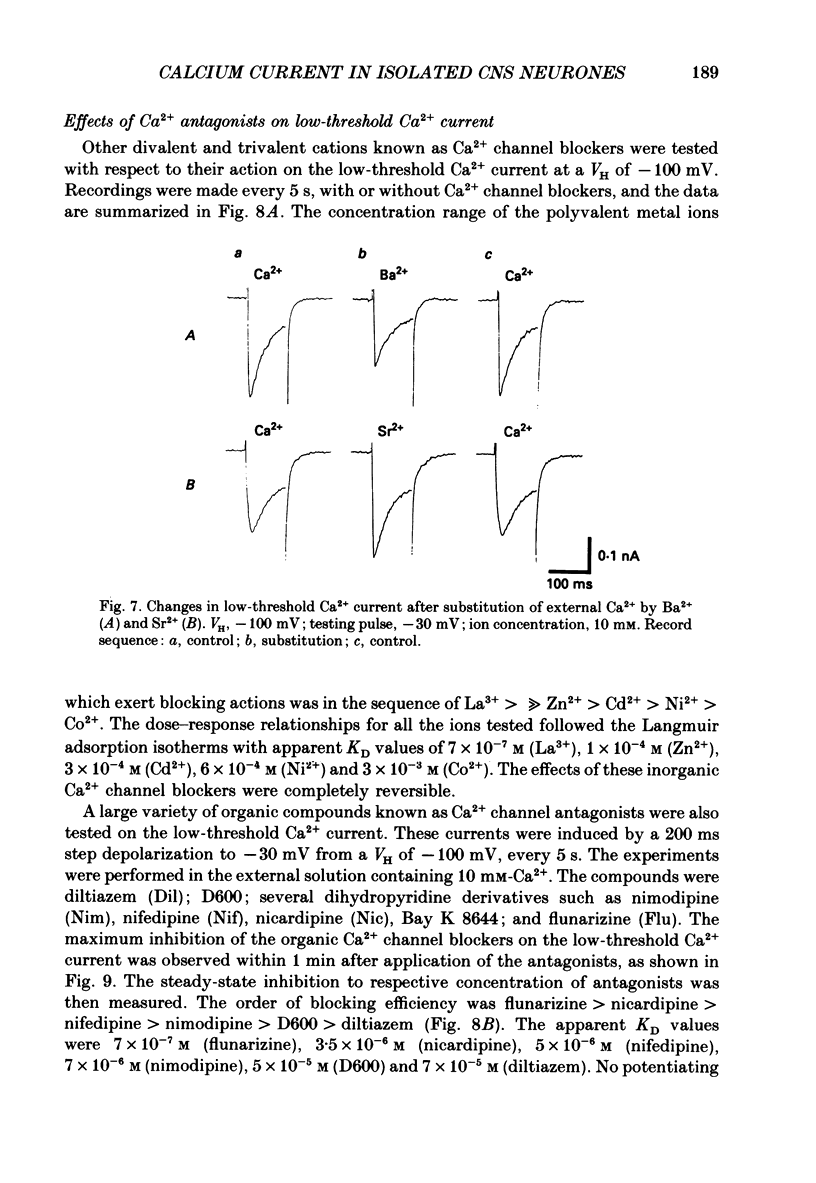
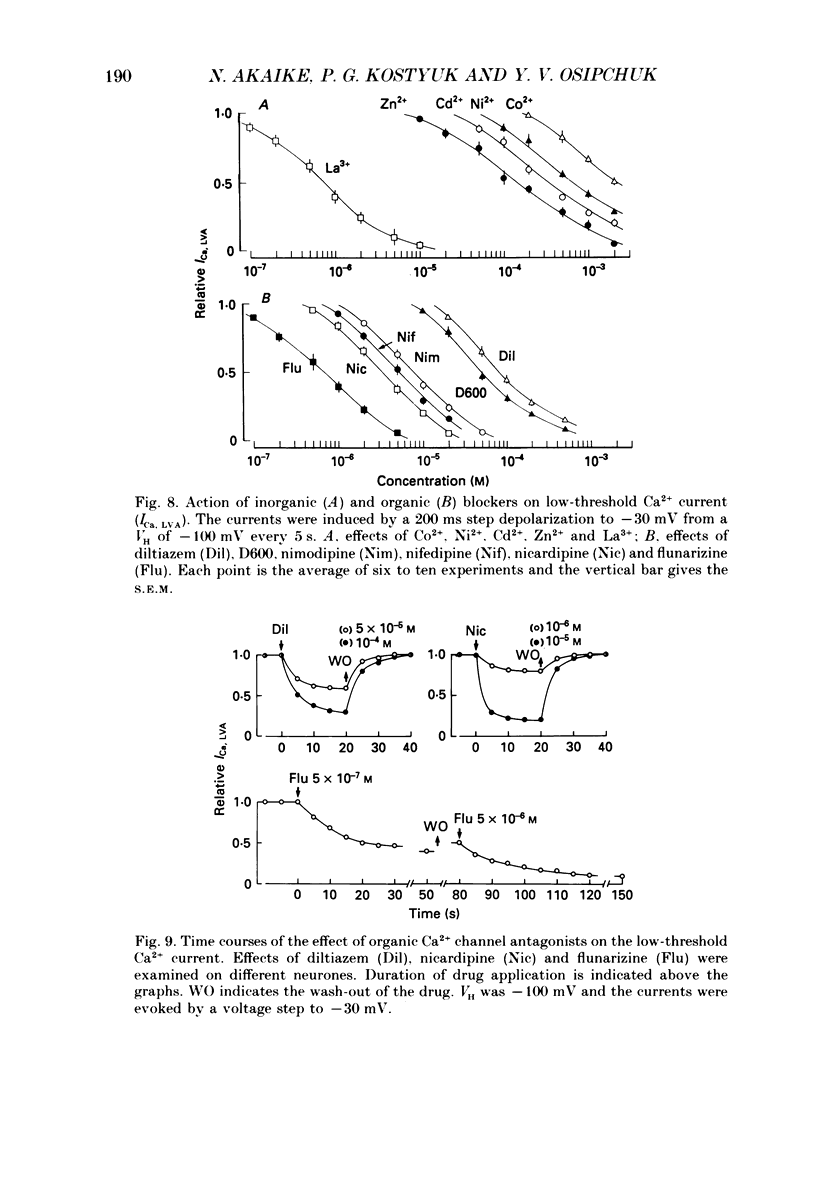
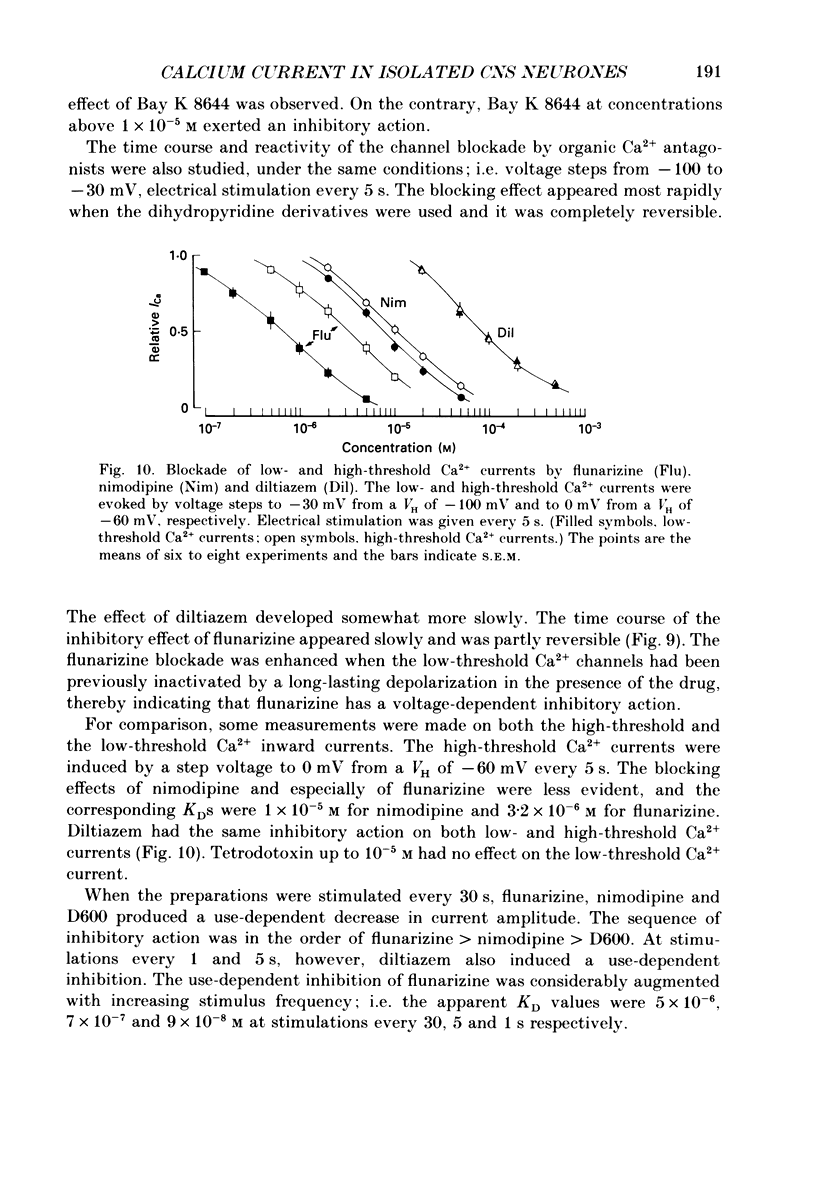
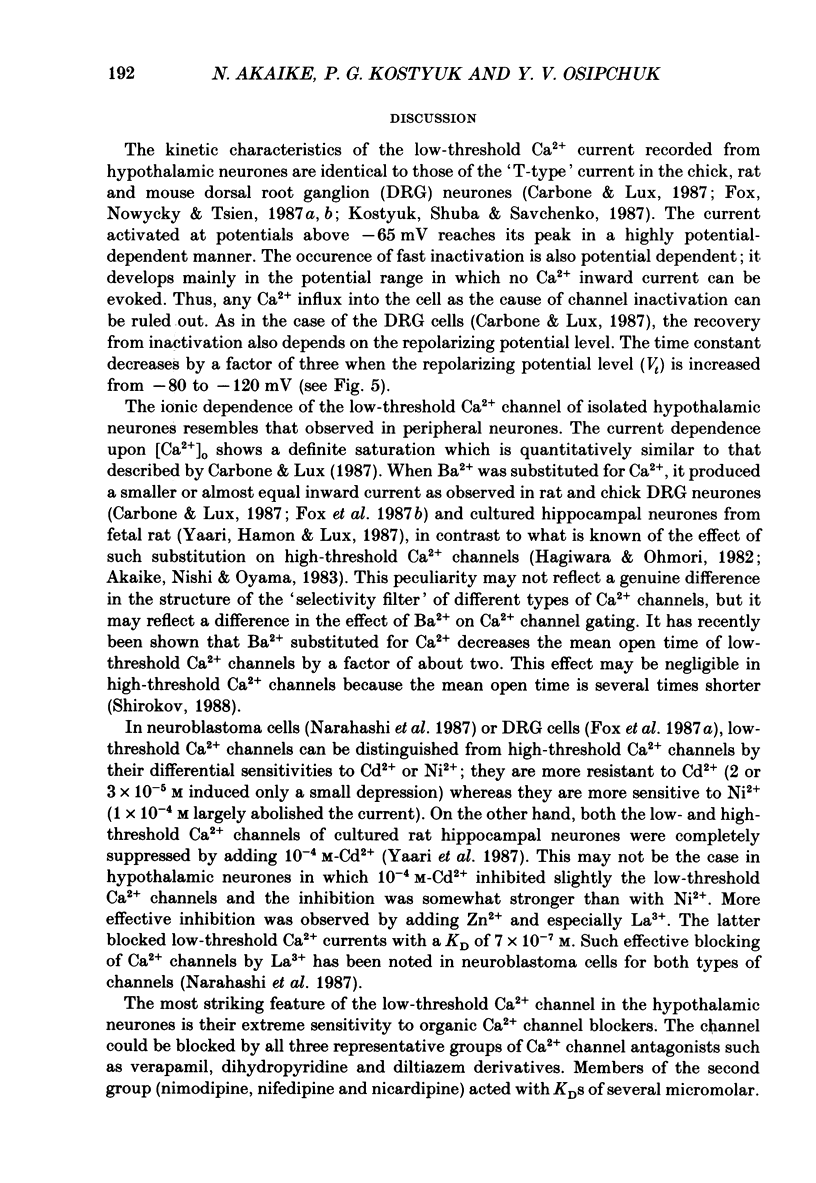
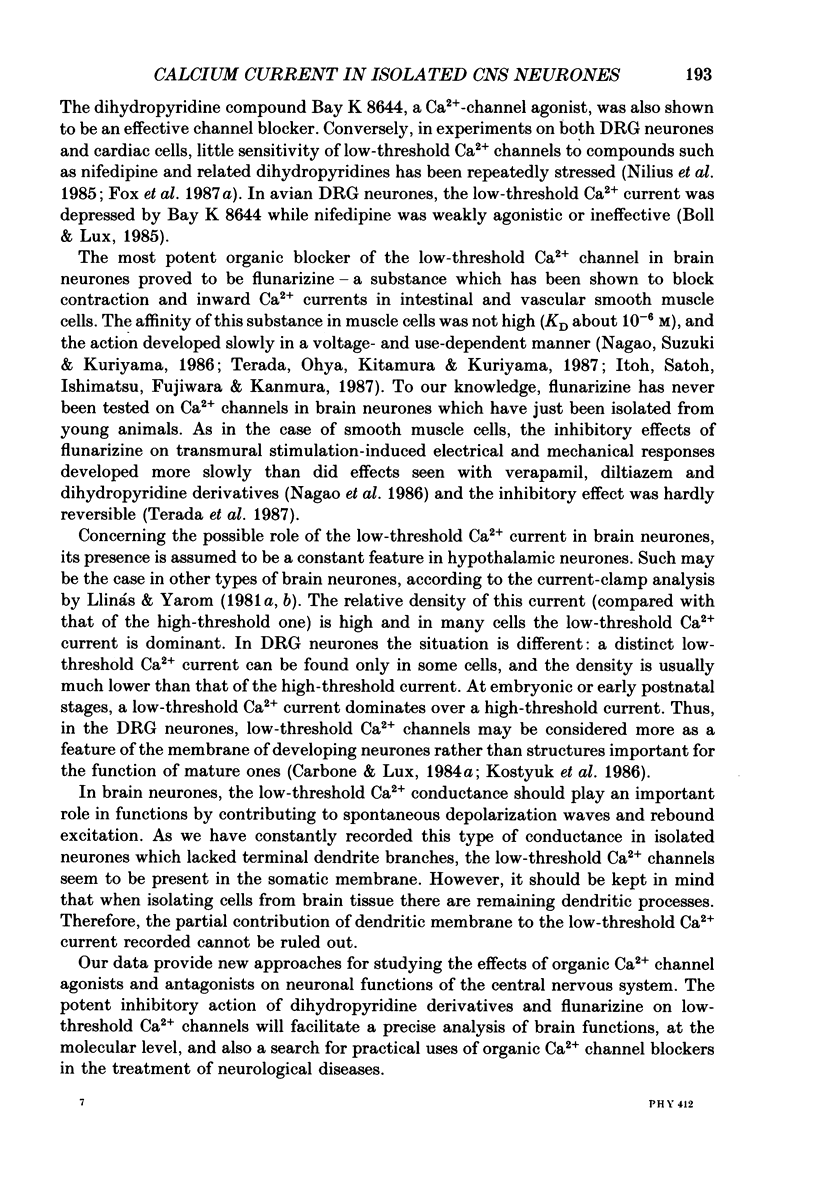
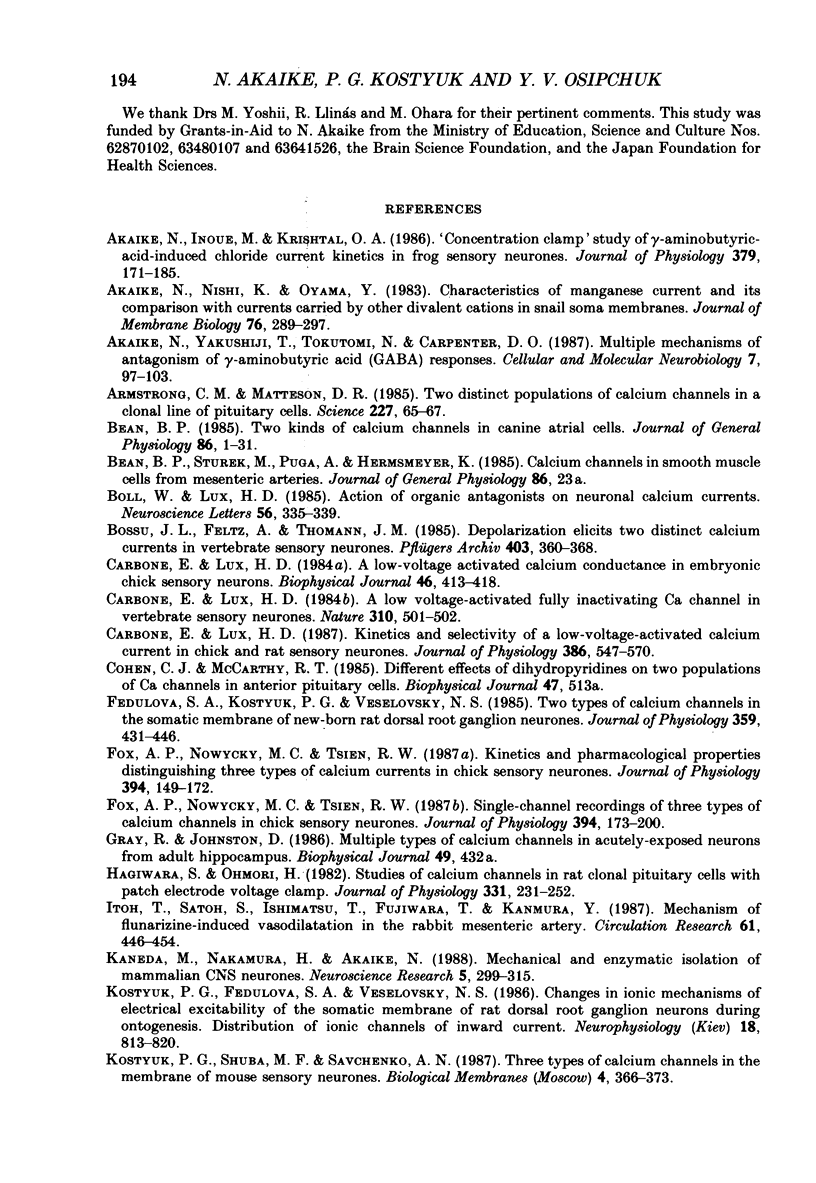
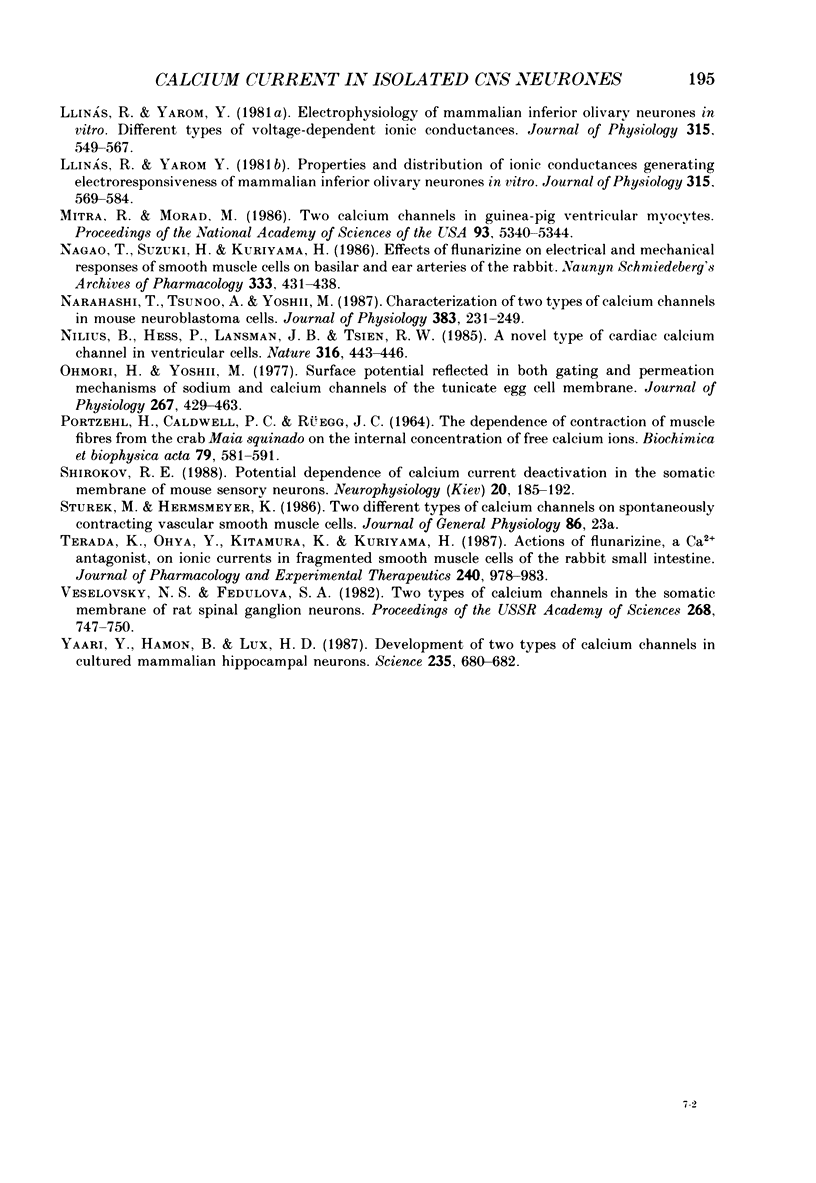
Selected References
These references are in PubMed. This may not be the complete list of references from this article.
- Akaike N., Inoue M., Krishtal O. A. 'Concentration-clamp' study of gamma-aminobutyric-acid-induced chloride current kinetics in frog sensory neurones. J Physiol. 1986 Oct;379:171–185. doi: 10.1113/jphysiol.1986.sp016246. [DOI] [PMC free article] [PubMed] [Google Scholar]
- Akaike N., Yakushiji T., Tokutomi N., Carpenter D. O. Multiple mechanisms of antagonism of gamma-aminobutyric acid (GABA) responses. Cell Mol Neurobiol. 1987 Mar;7(1):97–103. doi: 10.1007/BF00734993. [DOI] [PubMed] [Google Scholar]
- Armstrong C. M., Matteson D. R. Two distinct populations of calcium channels in a clonal line of pituitary cells. Science. 1985 Jan 4;227(4682):65–67. doi: 10.1126/science.2578071. [DOI] [PubMed] [Google Scholar]
- Bean B. P. Two kinds of calcium channels in canine atrial cells. Differences in kinetics, selectivity, and pharmacology. J Gen Physiol. 1985 Jul;86(1):1–30. doi: 10.1085/jgp.86.1.1. [DOI] [PMC free article] [PubMed] [Google Scholar]
- Boll W., Lux H. D. Action of organic antagonists on neuronal calcium currents. Neurosci Lett. 1985 May 23;56(3):335–339. doi: 10.1016/0304-3940(85)90265-4. [DOI] [PubMed] [Google Scholar]
- Bossu J. L., Feltz A., Thomann J. M. Depolarization elicits two distinct calcium currents in vertebrate sensory neurones. Pflugers Arch. 1985 Apr;403(4):360–368. doi: 10.1007/BF00589247. [DOI] [PubMed] [Google Scholar]
- Carbone E., Lux H. D. A low voltage-activated calcium conductance in embryonic chick sensory neurons. Biophys J. 1984 Sep;46(3):413–418. doi: 10.1016/S0006-3495(84)84037-0. [DOI] [PMC free article] [PubMed] [Google Scholar]
- Carbone E., Lux H. D. A low voltage-activated, fully inactivating Ca channel in vertebrate sensory neurones. Nature. 1984 Aug 9;310(5977):501–502. doi: 10.1038/310501a0. [DOI] [PubMed] [Google Scholar]
- Carbone E., Lux H. D. Kinetics and selectivity of a low-voltage-activated calcium current in chick and rat sensory neurones. J Physiol. 1987 May;386:547–570. doi: 10.1113/jphysiol.1987.sp016551. [DOI] [PMC free article] [PubMed] [Google Scholar]
- Fedulova S. A., Kostyuk P. G., Veselovsky N. S. Two types of calcium channels in the somatic membrane of new-born rat dorsal root ganglion neurones. J Physiol. 1985 Feb;359:431–446. doi: 10.1113/jphysiol.1985.sp015594. [DOI] [PMC free article] [PubMed] [Google Scholar]
- Fox A. P., Nowycky M. C., Tsien R. W. Kinetic and pharmacological properties distinguishing three types of calcium currents in chick sensory neurones. J Physiol. 1987 Dec;394:149–172. doi: 10.1113/jphysiol.1987.sp016864. [DOI] [PMC free article] [PubMed] [Google Scholar]
- Fox A. P., Nowycky M. C., Tsien R. W. Single-channel recordings of three types of calcium channels in chick sensory neurones. J Physiol. 1987 Dec;394:173–200. doi: 10.1113/jphysiol.1987.sp016865. [DOI] [PMC free article] [PubMed] [Google Scholar]
- Hagiwara S., Ohmori H. Studies of calcium channels in rat clonal pituitary cells with patch electrode voltage clamp. J Physiol. 1982 Oct;331:231–252. doi: 10.1113/jphysiol.1982.sp014371. [DOI] [PMC free article] [PubMed] [Google Scholar]
- Itoh T., Satoh S., Ishimatsu T., Fujiwara T., Kanmura Y. Mechanisms of flunarizine-induced vasodilation in the rabbit mesenteric artery. Circ Res. 1987 Sep;61(3):446–454. doi: 10.1161/01.res.61.3.446. [DOI] [PubMed] [Google Scholar]
- Kaneda M., Nakamura H., Akaike N. Mechanical and enzymatic isolation of mammalian CNS neurons. Neurosci Res. 1988 Apr;5(4):299–315. doi: 10.1016/0168-0102(88)90032-6. [DOI] [PubMed] [Google Scholar]
- Llinás R., Yarom Y. Electrophysiology of mammalian inferior olivary neurones in vitro. Different types of voltage-dependent ionic conductances. J Physiol. 1981 Jun;315:549–567. doi: 10.1113/jphysiol.1981.sp013763. [DOI] [PMC free article] [PubMed] [Google Scholar]
- Llinás R., Yarom Y. Properties and distribution of ionic conductances generating electroresponsiveness of mammalian inferior olivary neurones in vitro. J Physiol. 1981 Jun;315:569–584. doi: 10.1113/jphysiol.1981.sp013764. [DOI] [PMC free article] [PubMed] [Google Scholar]
- Mitra R., Morad M. Two types of calcium channels in guinea pig ventricular myocytes. Proc Natl Acad Sci U S A. 1986 Jul;83(14):5340–5344. doi: 10.1073/pnas.83.14.5340. [DOI] [PMC free article] [PubMed] [Google Scholar]
- Nagao T., Suzuki H., Kuriyama H. Effects of flunarizine on electrical and mechanical responses of smooth muscle cells in basilar and ear arteries of the rabbit. Naunyn Schmiedebergs Arch Pharmacol. 1986 Aug;333(4):431–438. doi: 10.1007/BF00500020. [DOI] [PubMed] [Google Scholar]
- Narahashi T., Tsunoo A., Yoshii M. Characterization of two types of calcium channels in mouse neuroblastoma cells. J Physiol. 1987 Feb;383:231–249. doi: 10.1113/jphysiol.1987.sp016406. [DOI] [PMC free article] [PubMed] [Google Scholar]
- Nilius B., Hess P., Lansman J. B., Tsien R. W. A novel type of cardiac calcium channel in ventricular cells. Nature. 1985 Aug 1;316(6027):443–446. doi: 10.1038/316443a0. [DOI] [PubMed] [Google Scholar]
- Ohmori H., Yoshii M. Surface potential reflected in both gating and permeation mechanisms of sodium and calcium channels of the tunicate egg cell membrane. J Physiol. 1977 May;267(2):429–463. doi: 10.1113/jphysiol.1977.sp011821. [DOI] [PMC free article] [PubMed] [Google Scholar]
- PORTZEHL H., CALDWELL P. C., RUEEGG J. C. THE DEPENDENCE OF CONTRACTION AND RELAXATION OF MUSCLE FIBRES FROM THE CRAB MAIA SQUINADO ON THE INTERNAL CONCENTRATION OF FREE CALCIUM IONS. Biochim Biophys Acta. 1964 May 25;79:581–591. doi: 10.1016/0926-6577(64)90224-4. [DOI] [PubMed] [Google Scholar]
- Shirokov R. E. Potentsialozavisimost' deaktivatsii kal'tsievykh tokov cherez somaticheskuiu membranu sensornykh neironov myshi. Neirofiziologiia. 1988;20(2):185–193. [PubMed] [Google Scholar]
- Terada K., Ohya Y., Kitamura K., Kuriyama H. Actions of flunarizine, a Ca++ antagonist, on ionic currents in fragmented smooth muscle cells of the rabbit small intestine. J Pharmacol Exp Ther. 1987 Mar;240(3):978–983. [PubMed] [Google Scholar]
- Veselovskii N. S., Fedulova S. A. Dva tipa kal'tsievykh kanalov v somaticheskoi membrane neironov spinal'nykh gangliev krys. Dokl Akad Nauk SSSR. 1983;268(3):747–750. [PubMed] [Google Scholar]
- Yaari Y., Hamon B., Lux H. D. Development of two types of calcium channels in cultured mammalian hippocampal neurons. Science. 1987 Feb 6;235(4789):680–682. doi: 10.1126/science.2433765. [DOI] [PubMed] [Google Scholar]