Abstract
1. We have measured the effect of the aglycone acetylstrophanthidin (ACS) on twitches, cooling contractures and microscopic tension fluctuations in rabbit ventricular muscle. 2. Both developed twitches and cooling contractures are strengthened by applications of ACS in the range 1-4 microM. This positive inotropy averages 150-160% of control (zero ACS) in both twitches and cooling contractures. Cooling contracture magnitude is assumed to reflect the availability of sarcoplasmic reticulum (SR) Ca2+ for contraction (Bridge, 1986). We infer that ACS increases the availability of SR Ca2+ by enlarging SR Ca2+ stores and this may contribute to the positive inotropy. 3. However, twitches appear to increase at lower concentrations of ACS than those required to increase cooling contractures. This observation suggests that the initial ACS inotropy may be achieved without an increase in SR Ca2+. Furthermore, low doses of ACS produce positive inotropy in the presence of 10.0 mM-caffeine where cooling contractures are abolished. This also suggests that positive inotropy occurs in the absence of SR Ca2+ accumulation. 4. Rest decay of both cooling contractures and twitches is significantly slowed in 4 and 8 microM-ACS. We infer that ACS slows the rate of decline of SR Ca2+ available for contraction by slowing the rate at which Ca2+ is lost from the cell during rest. This suggests that ACS produces a net slowing of Ca2+ efflux during activity which in the absence of altered Ca2+ influx will result in net Ca2+ gain and presumably enlarged SR Ca2+ stores. 5. Increasing the concentration of ACS (6-10 microM) results in a decline in developed twitch tension, total tension and an increase in rest tension. Measurement of microscopic tension fluctuations indicates that as developed twitches decline, the root mean square (r.m.s.) of the tension fluctuations increases in a reciprocal manner. This supports the suggestion of others that the decline in developed twitch tension and the appearance of tension fluctuations are causally related. 6. Although ACS (6-10 microM) causes a decline in twitch tension, rapid cooling contractures remain elevated. We suggest that in the presence of Ca2+ oscillations the magnitude of cooling contractures reflects the sum of cytosolic Ca2+ and Ca2+ that is available for release. If microscopic tension fluctuations do represent Ca2+ moving between the SR and cytosol the sum of SR and cytosolic Ca2+ and hence cooling contracture might not decline.(ABSTRACT TRUNCATED AT 400 WORDS)
Full text
PDF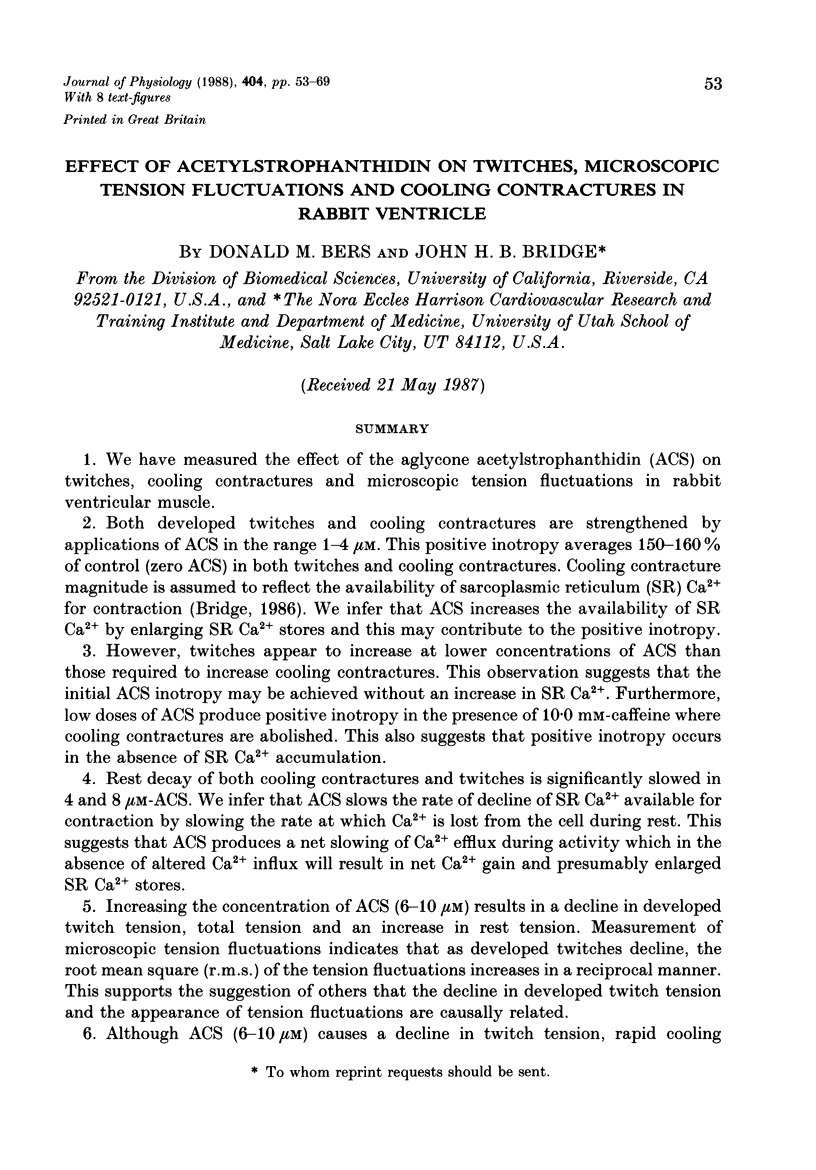
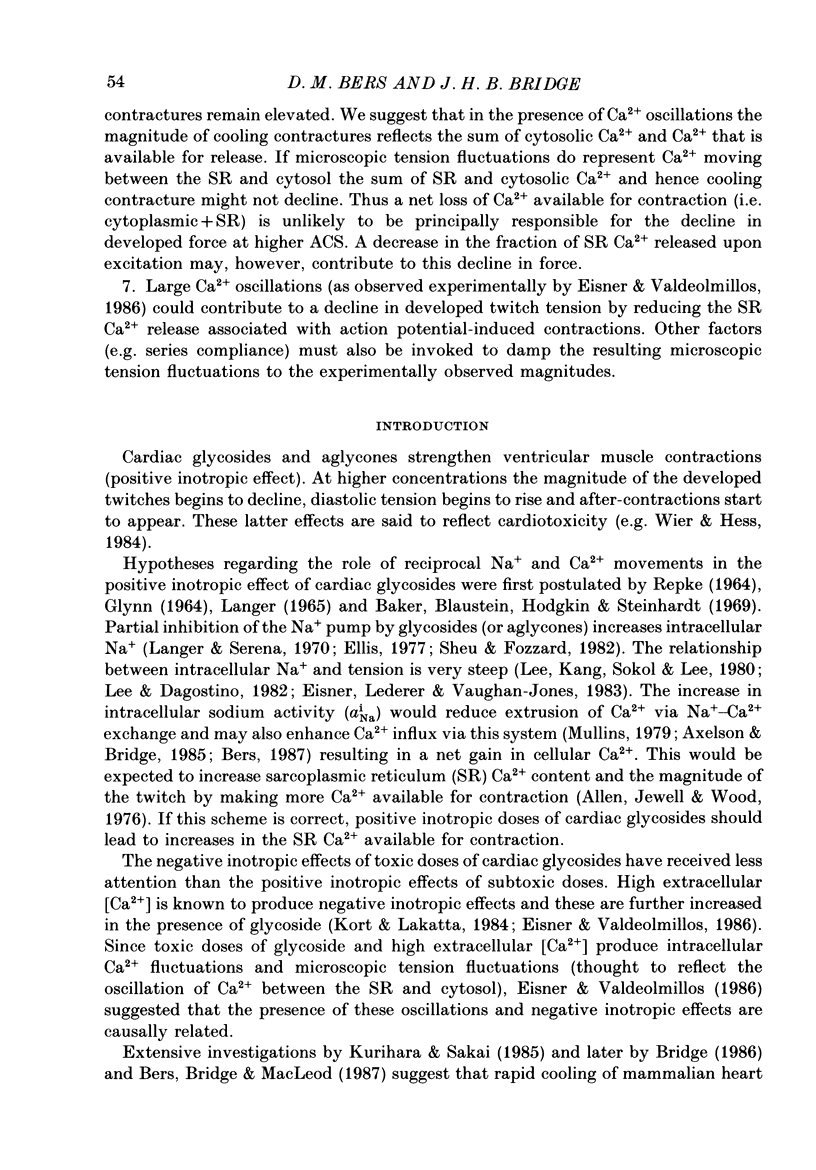
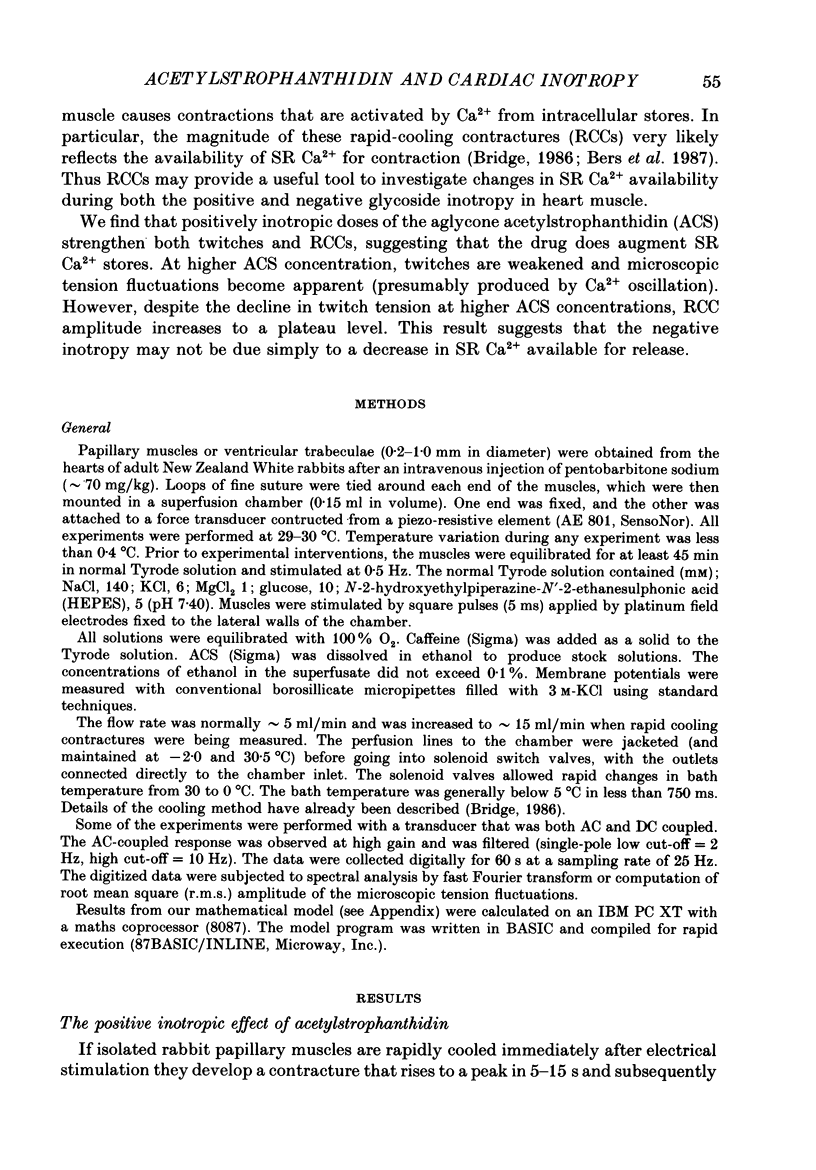
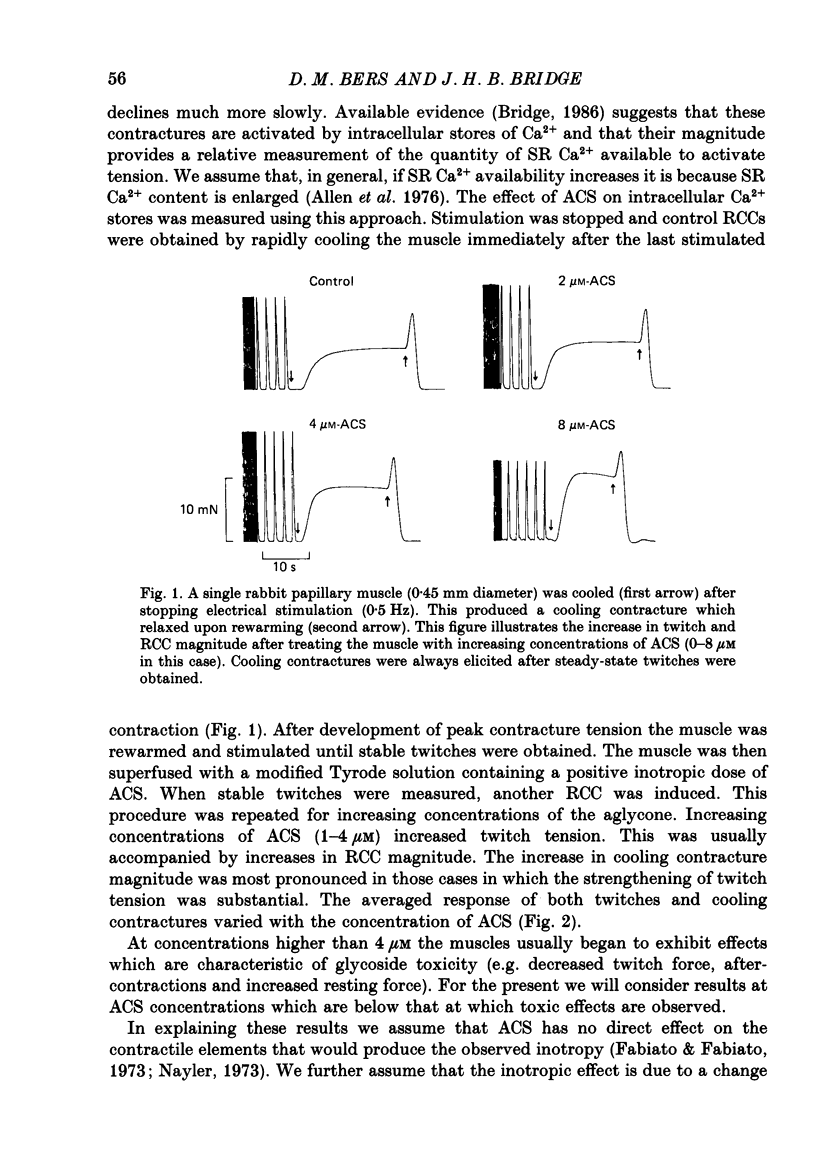
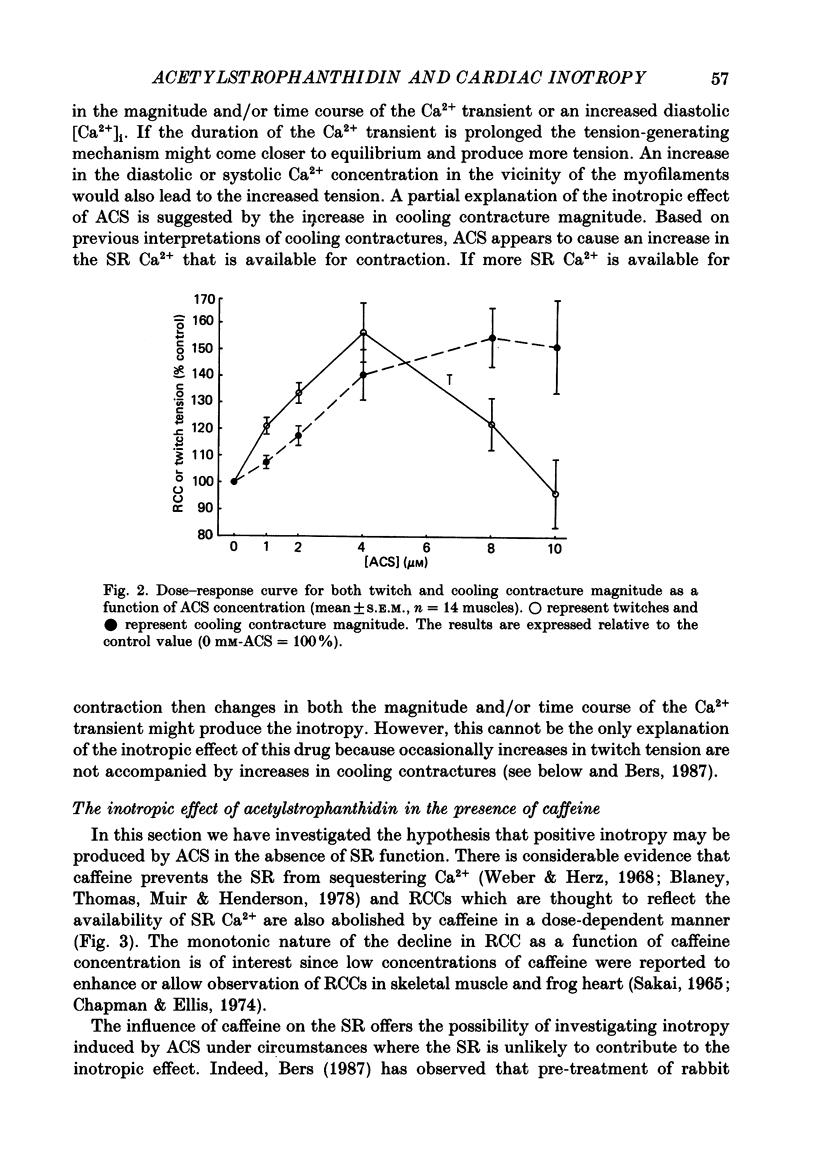
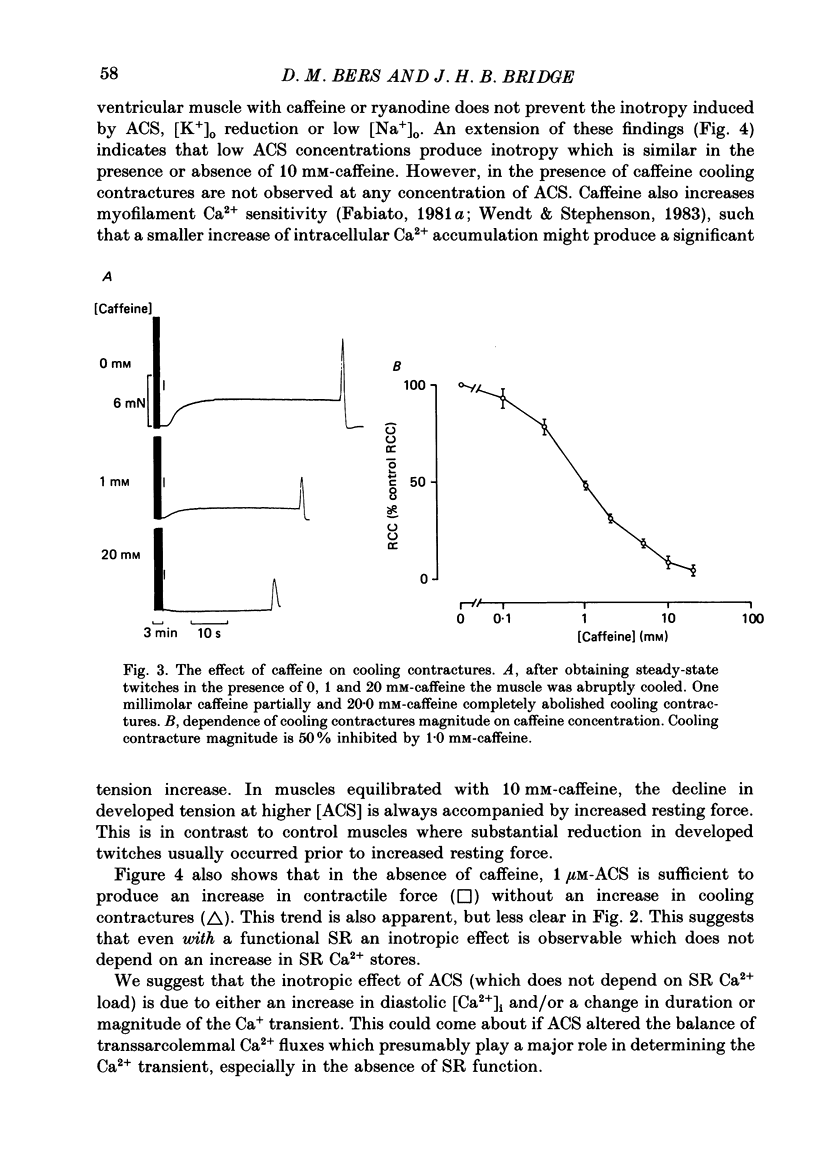
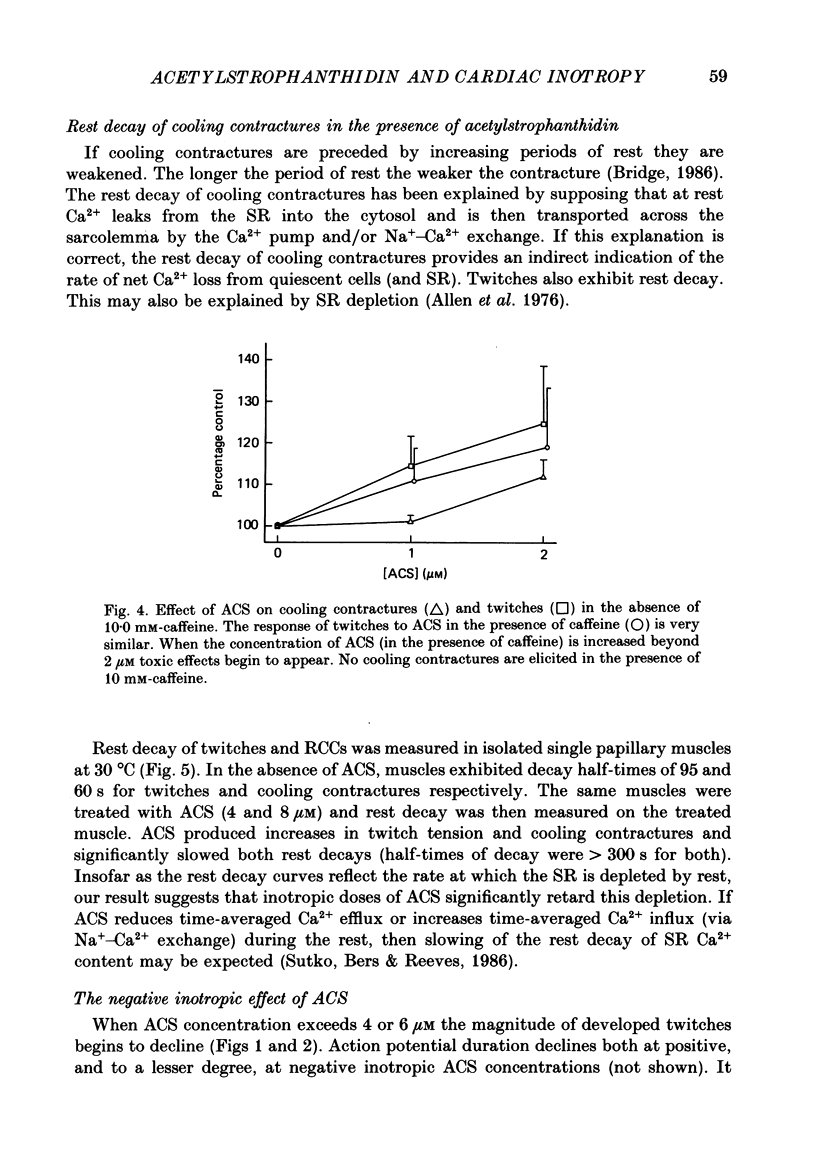
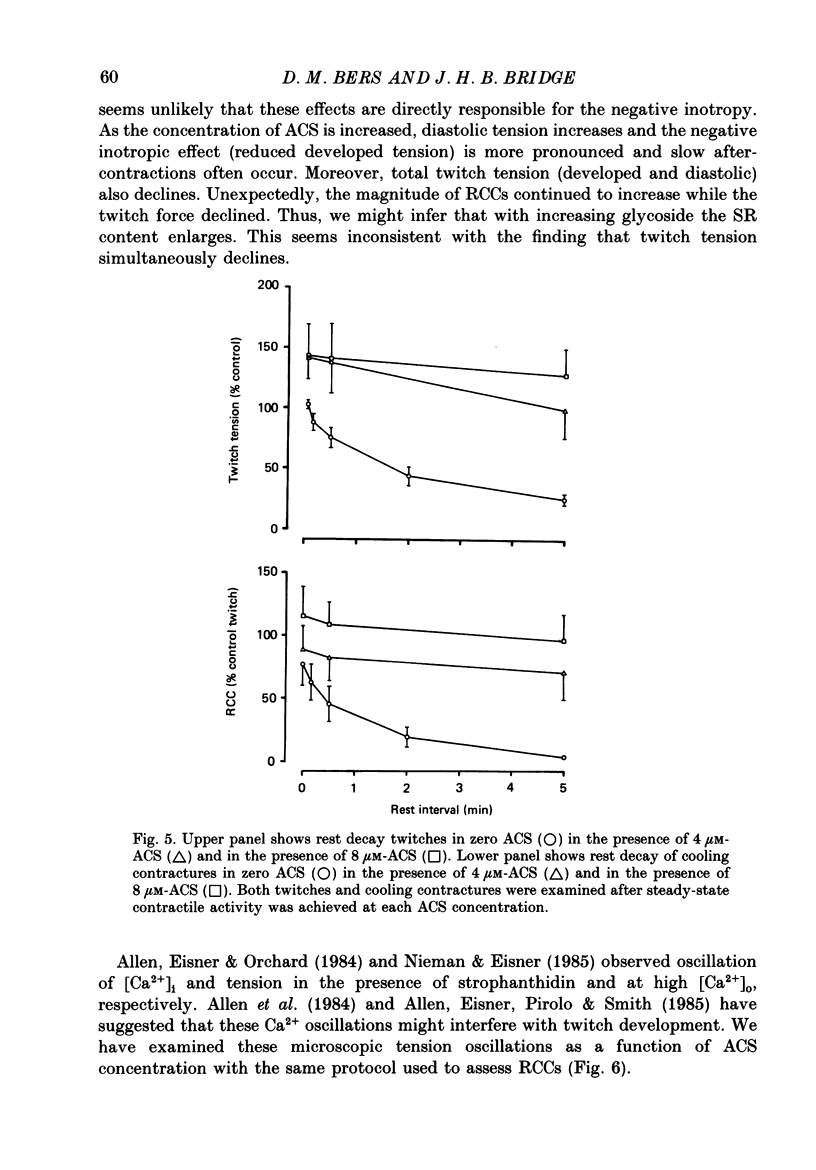
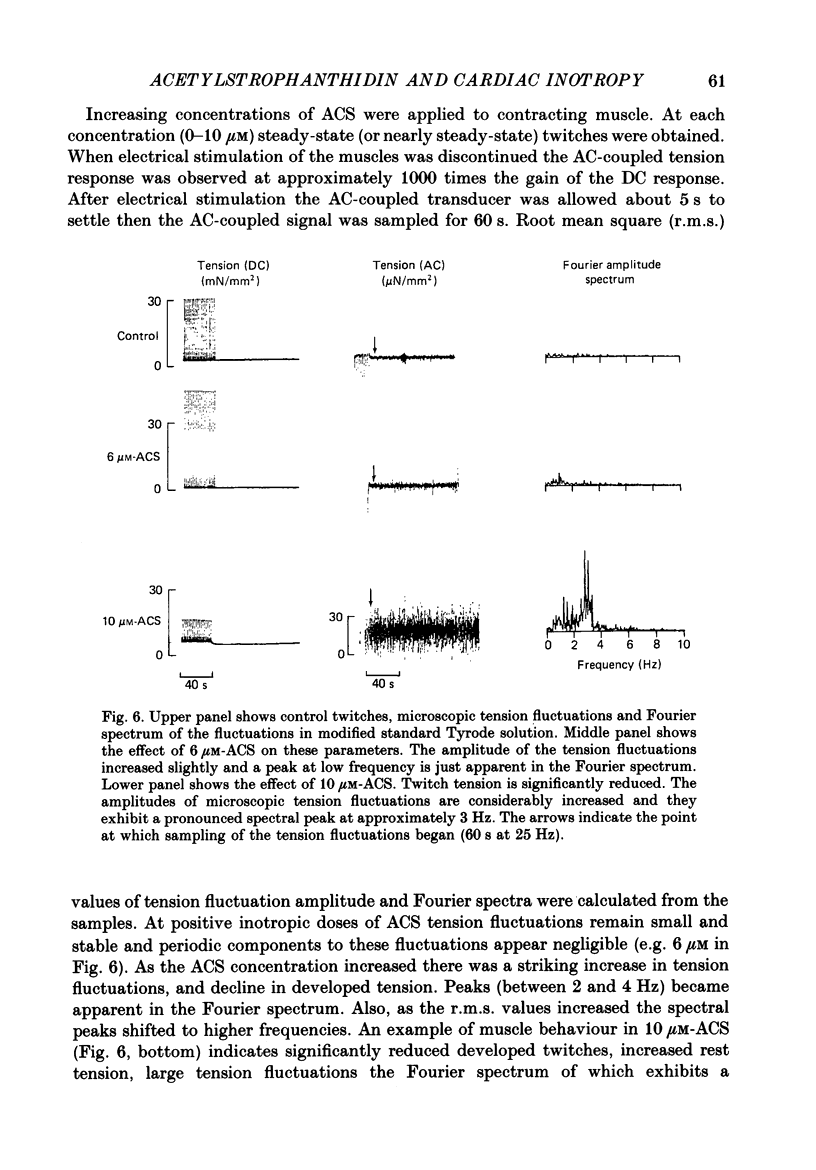
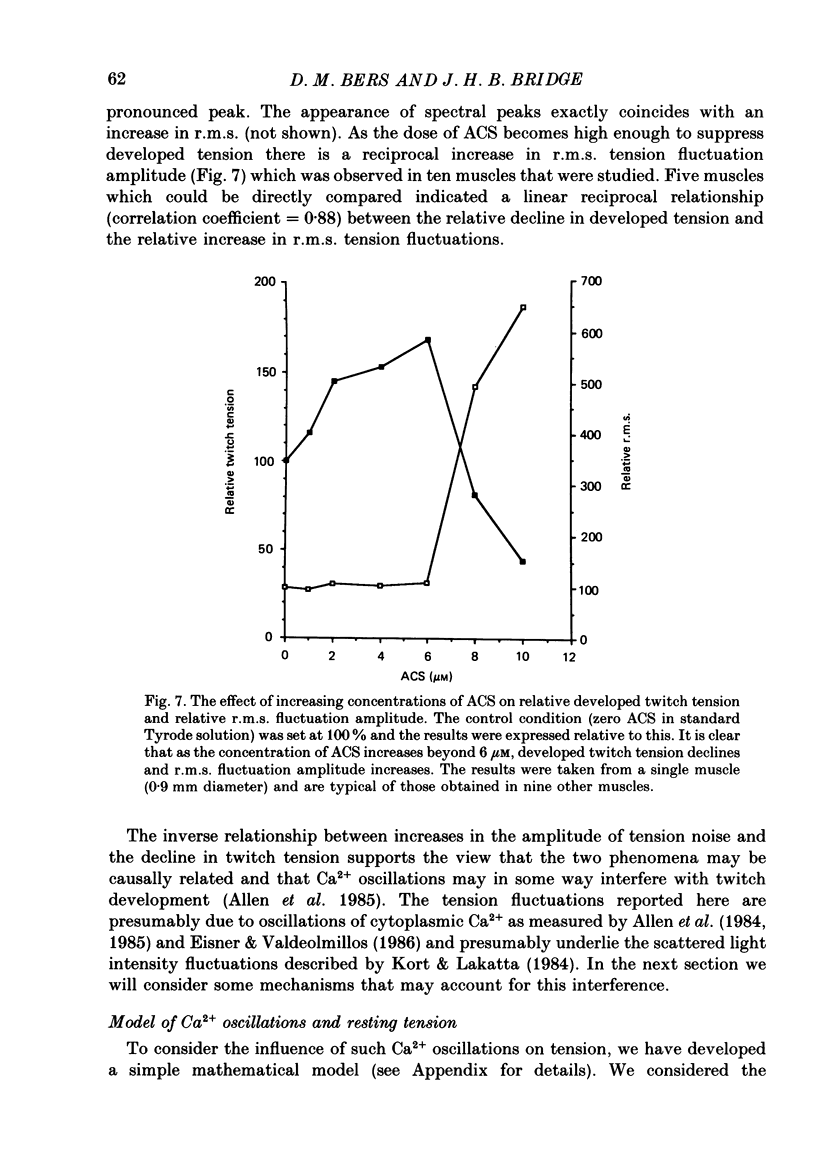
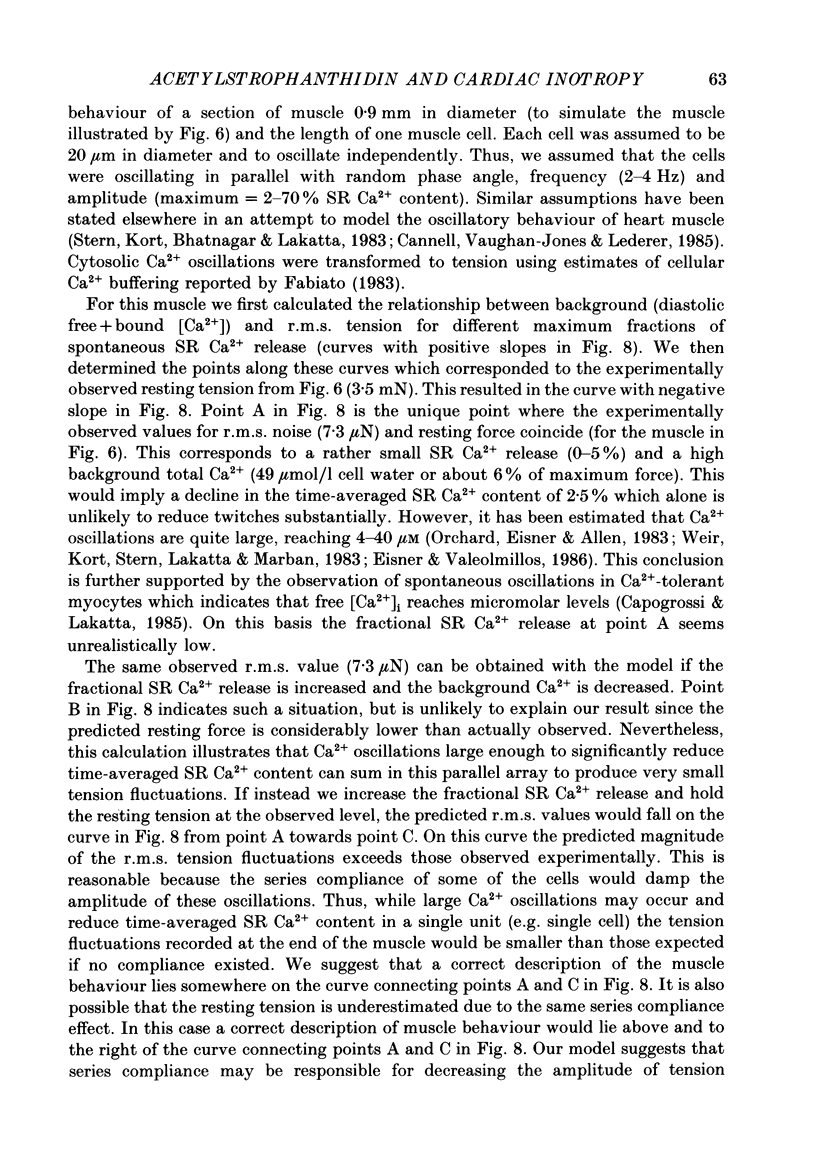
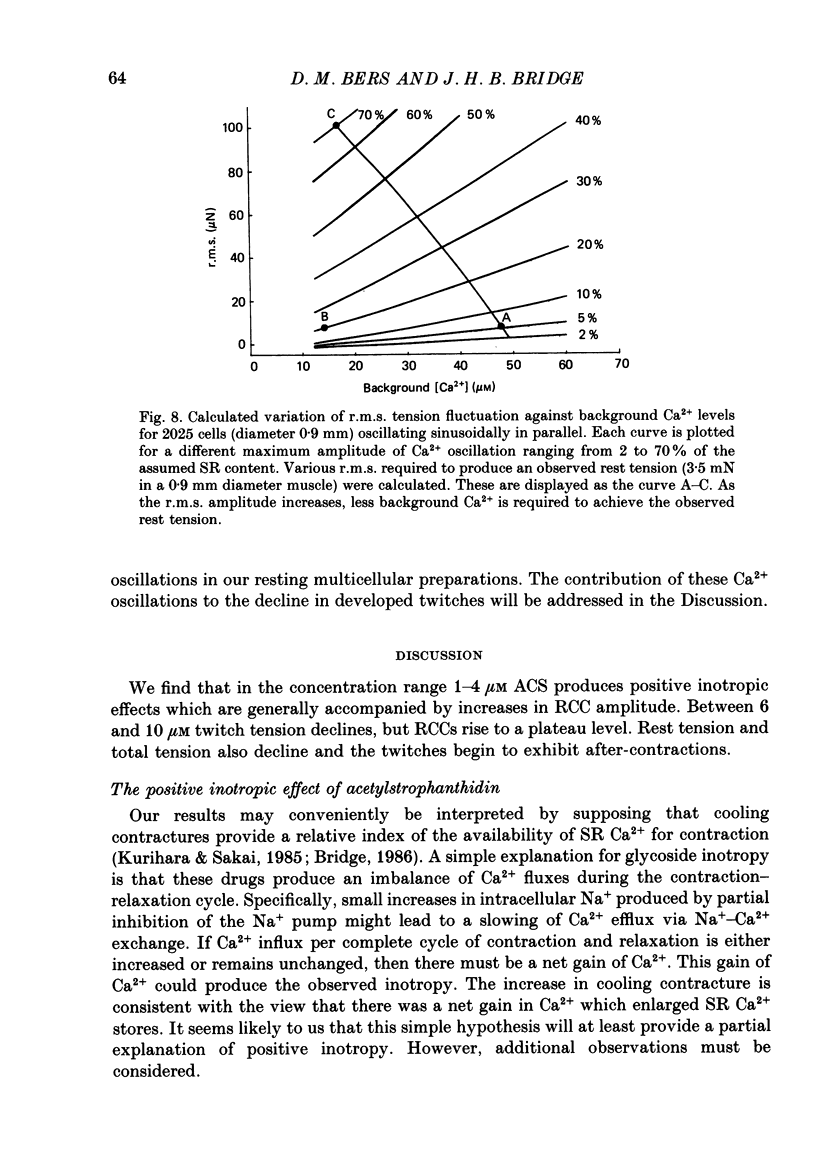
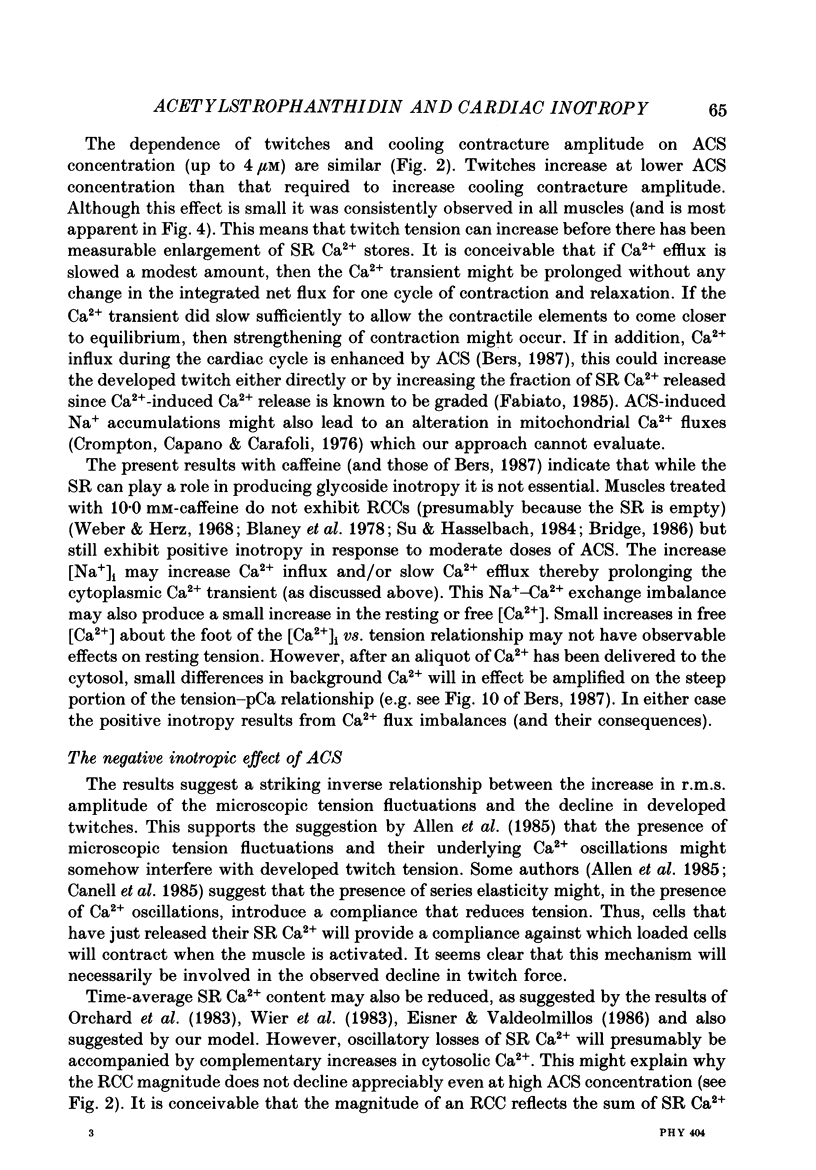
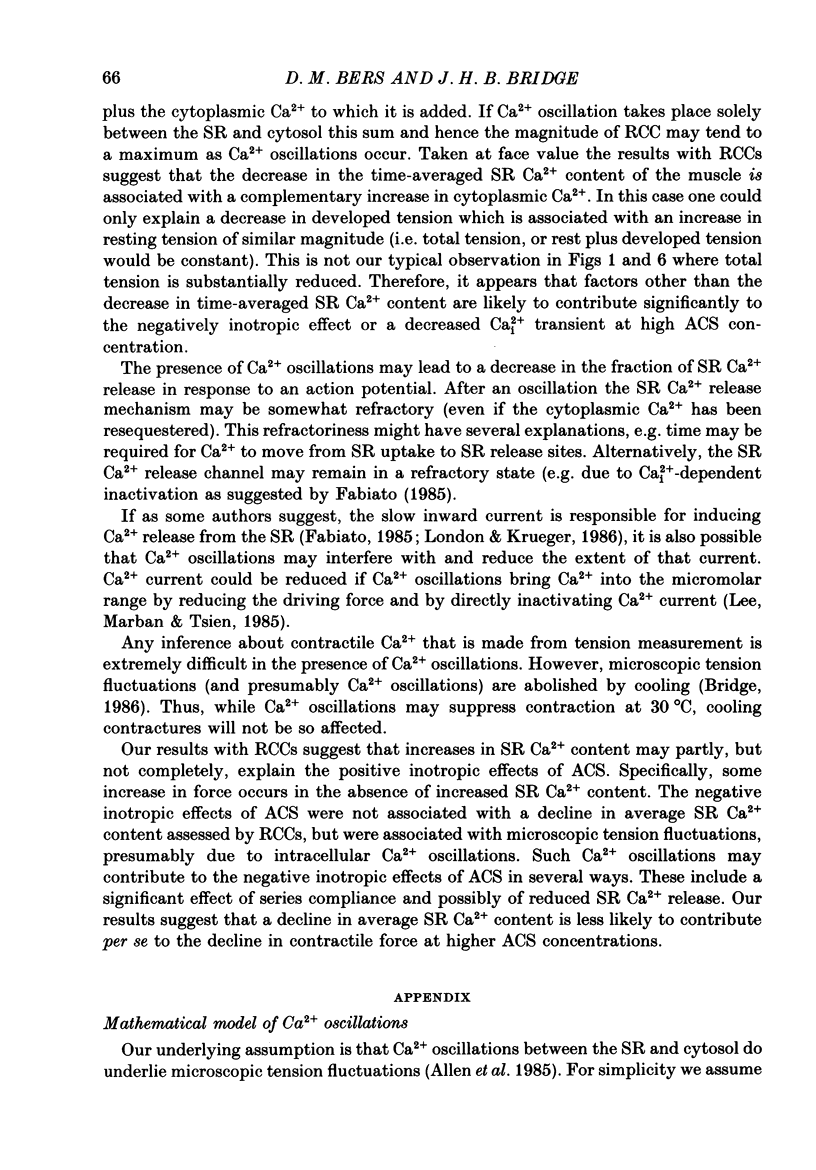
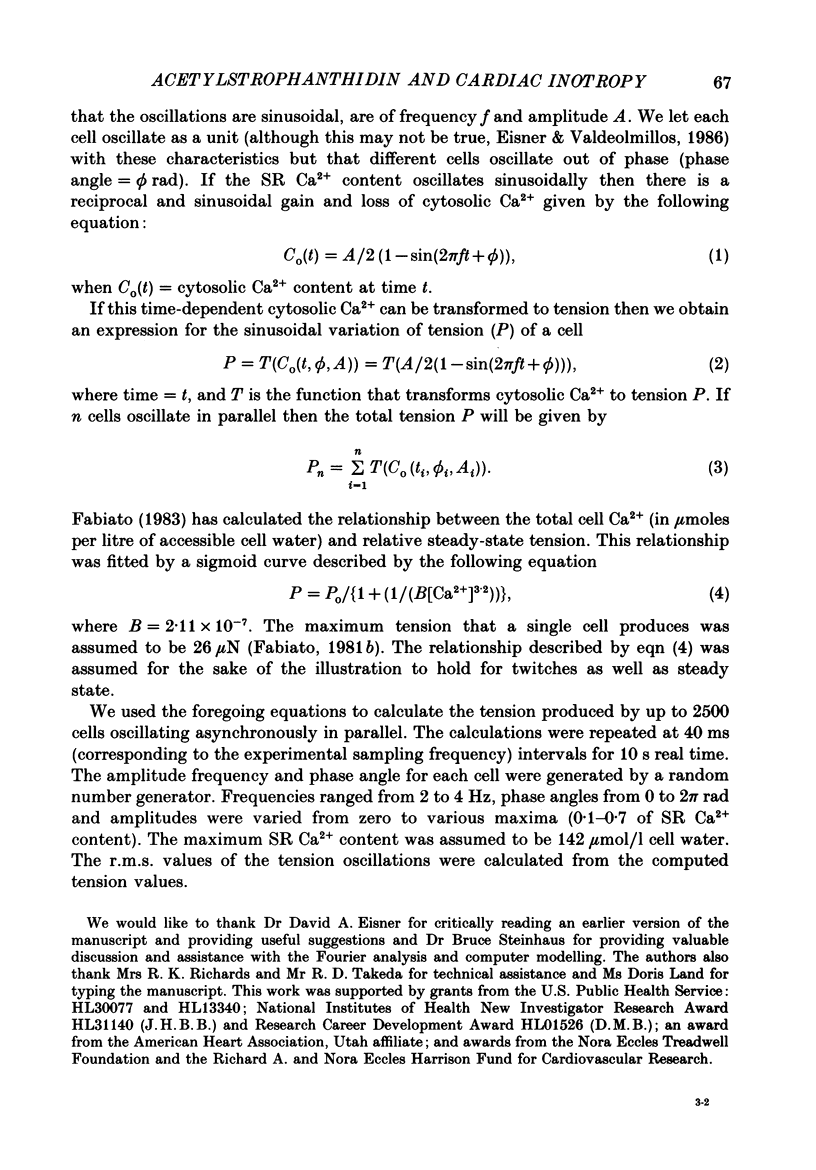
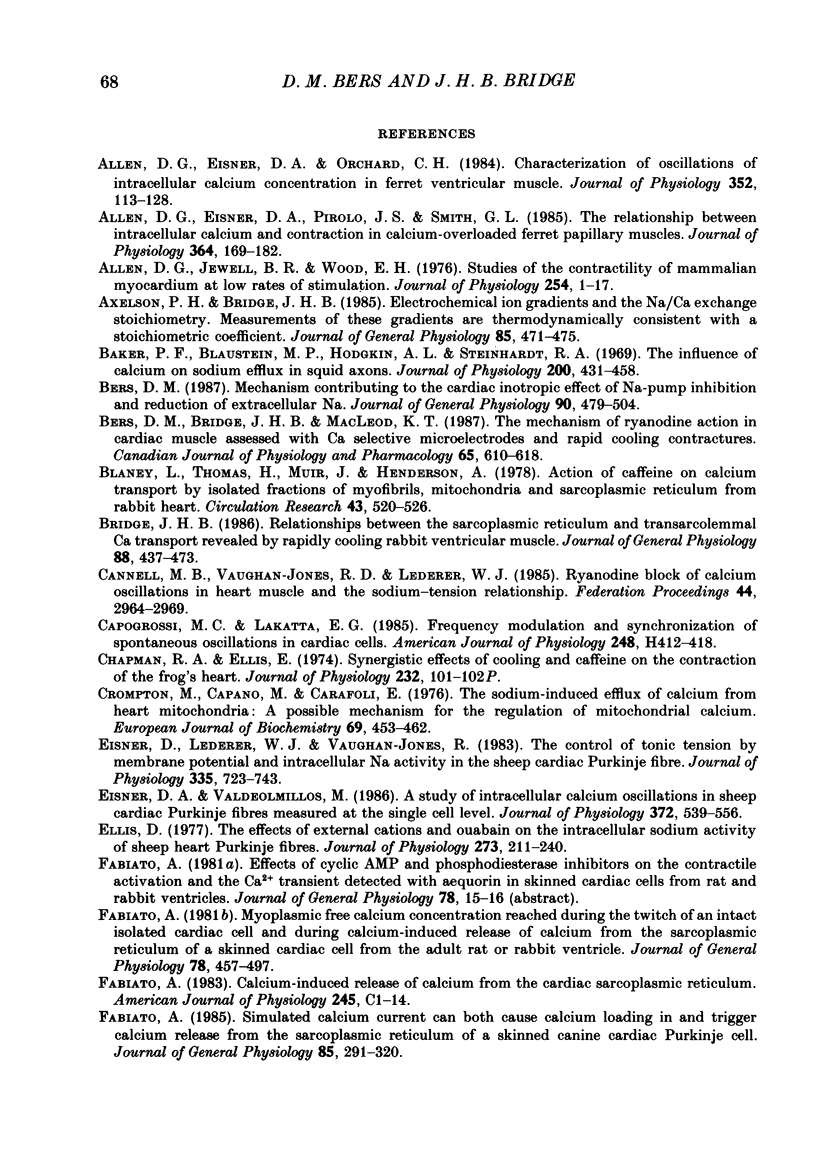
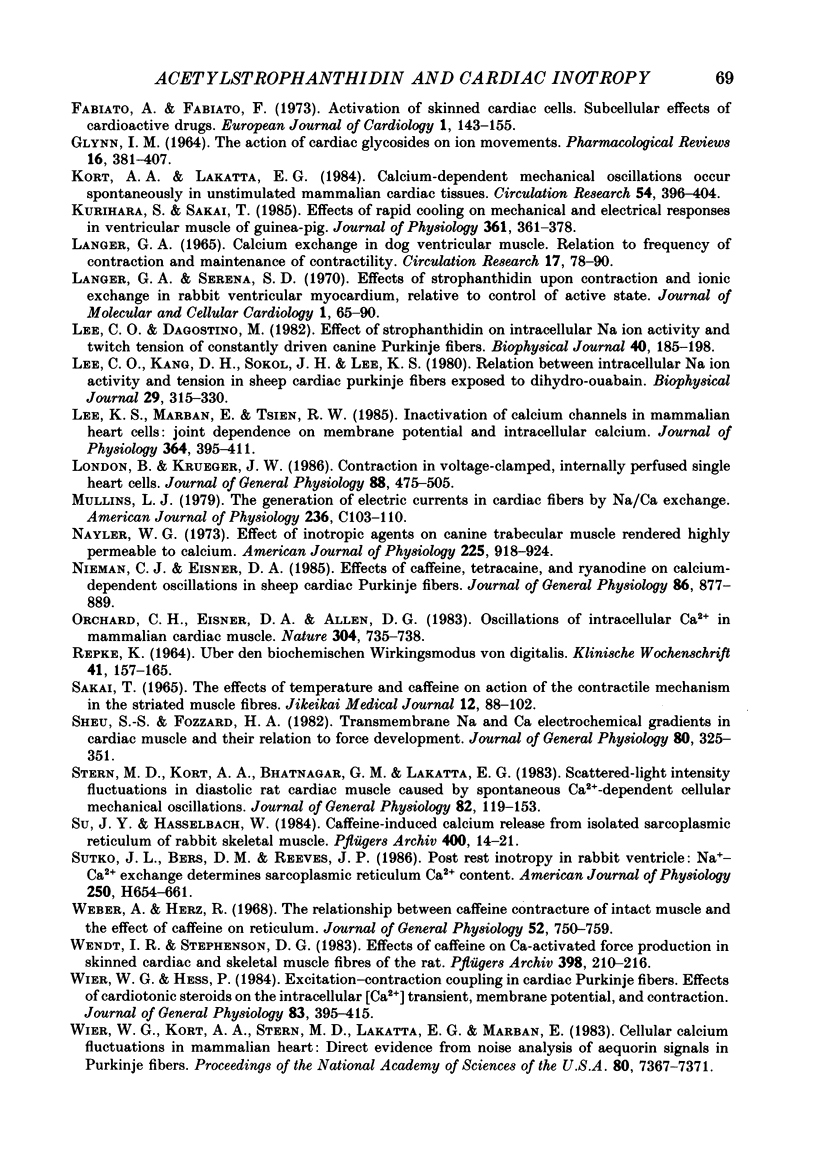
Selected References
These references are in PubMed. This may not be the complete list of references from this article.
- Allen D. G., Eisner D. A., Orchard C. H. Characterization of oscillations of intracellular calcium concentration in ferret ventricular muscle. J Physiol. 1984 Jul;352:113–128. doi: 10.1113/jphysiol.1984.sp015281. [DOI] [PMC free article] [PubMed] [Google Scholar]
- Allen D. G., Eisner D. A., Pirolo J. S., Smith G. L. The relationship between intracellular calcium and contraction in calcium-overloaded ferret papillary muscles. J Physiol. 1985 Jul;364:169–182. doi: 10.1113/jphysiol.1985.sp015737. [DOI] [PMC free article] [PubMed] [Google Scholar]
- Allen D. G., Jewell B. R., Wood E. H. Studies of the contractility of mammalian myocardium at low rates of stimulation. J Physiol. 1976 Jan;254(1):1–17. doi: 10.1113/jphysiol.1976.sp011217. [DOI] [PMC free article] [PubMed] [Google Scholar]
- Axelsen P. H., Bridge J. H. Electrochemical ion gradients and the Na/Ca exchange stoichiometry. Measurements of these gradients are thermodynamically consistent with a stoichiometric coefficient greater than or equal to 3. J Gen Physiol. 1985 Mar;85(3):471–475. doi: 10.1085/jgp.85.3.471. [DOI] [PMC free article] [PubMed] [Google Scholar]
- Baker P. F., Blaustein M. P., Hodgkin A. L., Steinhardt R. A. The influence of calcium on sodium efflux in squid axons. J Physiol. 1969 Feb;200(2):431–458. doi: 10.1113/jphysiol.1969.sp008702. [DOI] [PMC free article] [PubMed] [Google Scholar]
- Bers D. M., Bridge J. H., MacLeod K. T. The mechanism of ryanodine action in rabbit ventricular muscle evaluated with Ca-selective microelectrodes and rapid cooling contractures. Can J Physiol Pharmacol. 1987 Apr;65(4):610–618. doi: 10.1139/y87-103. [DOI] [PubMed] [Google Scholar]
- Bers D. M. Mechanisms contributing to the cardiac inotropic effect of Na pump inhibition and reduction of extracellular Na. J Gen Physiol. 1987 Oct;90(4):479–504. doi: 10.1085/jgp.90.4.479. [DOI] [PMC free article] [PubMed] [Google Scholar]
- Blayney L., Thomas H., Muir J., Henderson A. Action of caffeine on calcium transport by isolated fractions of myofibrils, mitochondria, and sarcoplasmic reticulum from rabbit heart. Circ Res. 1978 Oct;43(4):520–526. doi: 10.1161/01.res.43.4.520. [DOI] [PubMed] [Google Scholar]
- Bridge J. H. Relationships between the sarcoplasmic reticulum and sarcolemmal calcium transport revealed by rapidly cooling rabbit ventricular muscle. J Gen Physiol. 1986 Oct;88(4):437–473. doi: 10.1085/jgp.88.4.437. [DOI] [PMC free article] [PubMed] [Google Scholar]
- Cannell M. B., Vaughan-Jones R. D., Lederer W. J. Ryanodine block of calcium oscillations in heart muscle and the sodium-tension relationship. Fed Proc. 1985 Dec;44(15):2964–2969. [PubMed] [Google Scholar]
- Capogrossi M. C., Lakatta E. G. Frequency modulation and synchronization of spontaneous oscillations in cardiac cells. Am J Physiol. 1985 Mar;248(3 Pt 2):H412–H418. doi: 10.1152/ajpheart.1985.248.3.H412. [DOI] [PubMed] [Google Scholar]
- Chapman R. A., Ellis D. Synergistic effects of cooling and caffeine on the contraction of the frog's heart. J Physiol. 1973 Jul;232(2):101P–102P. [PubMed] [Google Scholar]
- Eisner D. A., Lederer W. J., Vaughan-Jones R. D. The control of tonic tension by membrane potential and intracellular sodium activity in the sheep cardiac Purkinje fibre. J Physiol. 1983 Feb;335:723–743. doi: 10.1113/jphysiol.1983.sp014560. [DOI] [PMC free article] [PubMed] [Google Scholar]
- Eisner D. A., Valdeolmillos M. A study of intracellular calcium oscillations in sheep cardiac Purkinje fibres measured at the single cell level. J Physiol. 1986 Mar;372:539–556. doi: 10.1113/jphysiol.1986.sp016024. [DOI] [PMC free article] [PubMed] [Google Scholar]
- Ellis D. The effects of external cations and ouabain on the intracellular sodium activity of sheep heart Purkinje fibres. J Physiol. 1977 Dec;273(1):211–240. doi: 10.1113/jphysiol.1977.sp012090. [DOI] [PMC free article] [PubMed] [Google Scholar]
- Fabiato A. Calcium-induced release of calcium from the cardiac sarcoplasmic reticulum. Am J Physiol. 1983 Jul;245(1):C1–14. doi: 10.1152/ajpcell.1983.245.1.C1. [DOI] [PubMed] [Google Scholar]
- Fabiato A., Fabiato F. Activation of skinned cardiac cells. Subcellular effects of cardioactive drugs. Eur J Cardiol. 1973 Dec;1(2):143–155. [PubMed] [Google Scholar]
- Fabiato A. Myoplasmic free calcium concentration reached during the twitch of an intact isolated cardiac cell and during calcium-induced release of calcium from the sarcoplasmic reticulum of a skinned cardiac cell from the adult rat or rabbit ventricle. J Gen Physiol. 1981 Nov;78(5):457–497. doi: 10.1085/jgp.78.5.457. [DOI] [PMC free article] [PubMed] [Google Scholar]
- Fabiato A. Simulated calcium current can both cause calcium loading in and trigger calcium release from the sarcoplasmic reticulum of a skinned canine cardiac Purkinje cell. J Gen Physiol. 1985 Feb;85(2):291–320. doi: 10.1085/jgp.85.2.291. [DOI] [PMC free article] [PubMed] [Google Scholar]
- GLYNN I. M. THE ACTION OF CARDIAC GLYCOSIDES ON ION MOVEMENTS. Pharmacol Rev. 1964 Dec;16:381–407. [PubMed] [Google Scholar]
- Kort A. A., Lakatta E. G. Calcium-dependent mechanical oscillations occur spontaneously in unstimulated mammalian cardiac tissues. Circ Res. 1984 Apr;54(4):396–404. doi: 10.1161/01.res.54.4.396. [DOI] [PubMed] [Google Scholar]
- Kurihara S., Sakai T. Effects of rapid cooling on mechanical and electrical responses in ventricular muscle of guinea-pig. J Physiol. 1985 Apr;361:361–378. doi: 10.1113/jphysiol.1985.sp015650. [DOI] [PMC free article] [PubMed] [Google Scholar]
- LANGER G. A. CALCIUM EXCHANGE IN DOG VENTRICULAR MUSCLE: RELATION TO FREQUENCY OF CONTRACTION AND MAINTENANCE OF CONTRACTILITY. Circ Res. 1965 Jul;17:78–89. doi: 10.1161/01.res.17.1.78. [DOI] [PubMed] [Google Scholar]
- Langer G. A., Serena S. D. Effects of strophanthidin upon contraction and ionic exchange in rabbit ventricular myocardium: relation to control of active state. J Mol Cell Cardiol. 1970 Mar;1(1):65–90. doi: 10.1016/0022-2828(70)90029-5. [DOI] [PubMed] [Google Scholar]
- Lee C. O., Dagostino M. Effect of strophanthidin on intracellular Na ion activity and twitch tension of constantly driven canine cardiac Purkinje fibers. Biophys J. 1982 Dec;40(3):185–198. doi: 10.1016/S0006-3495(82)84474-3. [DOI] [PMC free article] [PubMed] [Google Scholar]
- Lee C. O., Kang D. H., Sokol J. H., Lee K. S. Relation between intracellular Na ion activity and tension of sheep cardiac Purkinje fibers exposed to dihydro-ouabain. Biophys J. 1980 Feb;29(2):315–330. doi: 10.1016/S0006-3495(80)85135-6. [DOI] [PMC free article] [PubMed] [Google Scholar]
- Lee K. S., Marban E., Tsien R. W. Inactivation of calcium channels in mammalian heart cells: joint dependence on membrane potential and intracellular calcium. J Physiol. 1985 Jul;364:395–411. doi: 10.1113/jphysiol.1985.sp015752. [DOI] [PMC free article] [PubMed] [Google Scholar]
- London B., Krueger J. W. Contraction in voltage-clamped, internally perfused single heart cells. J Gen Physiol. 1986 Oct;88(4):475–505. doi: 10.1085/jgp.88.4.475. [DOI] [PMC free article] [PubMed] [Google Scholar]
- Mullins L. J. The generation of electric currents in cardiac fibers by Na/Ca exchange. Am J Physiol. 1979 Mar;236(3):C103–C110. doi: 10.1152/ajpcell.1979.236.3.C103. [DOI] [PubMed] [Google Scholar]
- Nayler W. G. Effect of inotropic agents on canine trabecular muscle rendered highly permeable to calcium. Am J Physiol. 1973 Oct;225(4):918–924. doi: 10.1152/ajplegacy.1973.225.4.918. [DOI] [PubMed] [Google Scholar]
- Nieman C. J., Eisner D. A. Effects of caffeine, tetracaine, and ryanodine on calcium-dependent oscillations in sheep cardiac Purkinje fibers. J Gen Physiol. 1985 Dec;86(6):877–889. doi: 10.1085/jgp.86.6.877. [DOI] [PMC free article] [PubMed] [Google Scholar]
- Orchard C. H., Eisner D. A., Allen D. G. Oscillations of intracellular Ca2+ in mammalian cardiac muscle. Nature. 1983 Aug 25;304(5928):735–738. doi: 10.1038/304735a0. [DOI] [PubMed] [Google Scholar]
- REPKE K. UBER DEN BIOCHEMISCHEN WIRKUNGSMODUS VON DIGITALIS. Klin Wochenschr. 1964 Feb 15;42:157–165. doi: 10.1007/BF01482616. [DOI] [PubMed] [Google Scholar]
- Sheu S. S., Fozzard H. A. Transmembrane Na+ and Ca2+ electrochemical gradients in cardiac muscle and their relationship to force development. J Gen Physiol. 1982 Sep;80(3):325–351. doi: 10.1085/jgp.80.3.325. [DOI] [PMC free article] [PubMed] [Google Scholar]
- Stern M. D., Kort A. A., Bhatnagar G. M., Lakatta E. G. Scattered-light intensity fluctuations in diastolic rat cardiac muscle caused by spontaneous Ca++-dependent cellular mechanical oscillations. J Gen Physiol. 1983 Jul;82(1):119–153. doi: 10.1085/jgp.82.1.119. [DOI] [PMC free article] [PubMed] [Google Scholar]
- Su J. Y., Hasselbach W. Caffeine-induced calcium release from isolated sarcoplasmic reticulum of rabbit skeletal muscle. Pflugers Arch. 1984 Jan;400(1):14–21. doi: 10.1007/BF00670530. [DOI] [PubMed] [Google Scholar]
- Sutko J. L., Bers D. M., Reeves J. P. Postrest inotropy in rabbit ventricle: Na+-Ca2+ exchange determines sarcoplasmic reticulum Ca2+ content. Am J Physiol. 1986 Apr;250(4 Pt 2):H654–H661. doi: 10.1152/ajpheart.1986.250.4.H654. [DOI] [PubMed] [Google Scholar]
- Weber A., Herz R. The relationship between caffeine contracture of intact muscle and the effect of caffeine on reticulum. J Gen Physiol. 1968 Nov;52(5):750–759. doi: 10.1085/jgp.52.5.750. [DOI] [PMC free article] [PubMed] [Google Scholar]
- Wendt I. R., Stephenson D. G. Effects of caffeine on Ca-activated force production in skinned cardiac and skeletal muscle fibres of the rat. Pflugers Arch. 1983 Aug;398(3):210–216. doi: 10.1007/BF00657153. [DOI] [PubMed] [Google Scholar]
- Wier W. G., Hess P. Excitation-contraction coupling in cardiac Purkinje fibers. Effects of cardiotonic steroids on the intracellular [Ca2+] transient, membrane potential, and contraction. J Gen Physiol. 1984 Mar;83(3):395–415. doi: 10.1085/jgp.83.3.395. [DOI] [PMC free article] [PubMed] [Google Scholar]
- Wier W. G., Kort A. A., Stern M. D., Lakatta E. G., Marban E. Cellular calcium fluctuations in mammalian heart: direct evidence from noise analysis of aequorin signals in Purkinje fibers. Proc Natl Acad Sci U S A. 1983 Dec;80(23):7367–7371. doi: 10.1073/pnas.80.23.7367. [DOI] [PMC free article] [PubMed] [Google Scholar]