Abstract
1. Intracellular recordings were obtained from cerebellar nuclear neurones in the isolated brain stem-cerebellar preparation of guinea-pigs in vitro. The electrical properties of the cells were quite similar to those reported in in vitro slice studies. They had an average resting potential of -56.7 +/- 1.8 mV, an input resistance of 23.8 +/- 4.9 M omega, and a time constant of 12.5 +/- 2.7 ms. The action potentials had an average amplitude of 57.3 +/- 5.28 mV (n = 20). 2. In addition to the ionic mechanisms required for the generation of the fast action potential, cerebellar nuclear neurones displayed a low-threshold Ca2+-dependent spike which produced a powerful rebound excitation following anodal break. This type of electroresponsiveness was absent in the slice preparation. 3. The anodal break response was further enhanced by the presence of a non-inactivating Na+ conductance similar to that described in Purkinje cells. 4. Following electrical stimulation of the cerebellar cortex or the underlying white matter, excitatory and inhibitory synaptic potentials (EPSP-IPSP sequences) could be recorded in cerebellar nuclear neurones. The EPSPs were elicited by direct activation of collaterals of mossy or climbing fibre afferents. The IPSPs followed direct or orthodromic Purkinje cell activation. 5. The integrity of the olivo-cerebellar system was tested by the administration of harmaline which produced powerful EPSP-IPSP sequences or pure IPSPs in cerebellar nuclear neurones. These IPSPs were often followed by a rebound firing of the cells. 6. These results indicate that the olivo-cerebellar pathway, in addition to its activation of the cerebellar cortex, exerts a powerful and complex set of synaptic events on cerebellar nuclear cells. As such it is a true afferent system, having a distinct role in cerebellar physiology.
Full text
PDF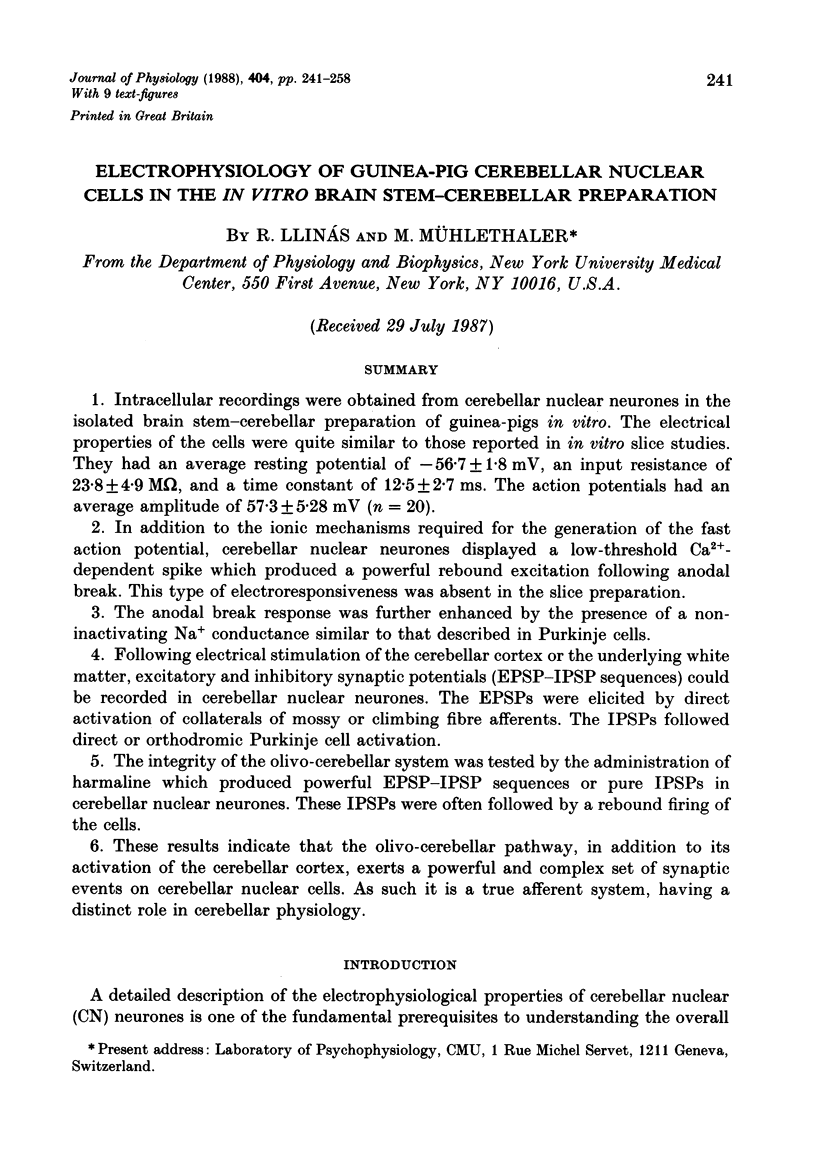
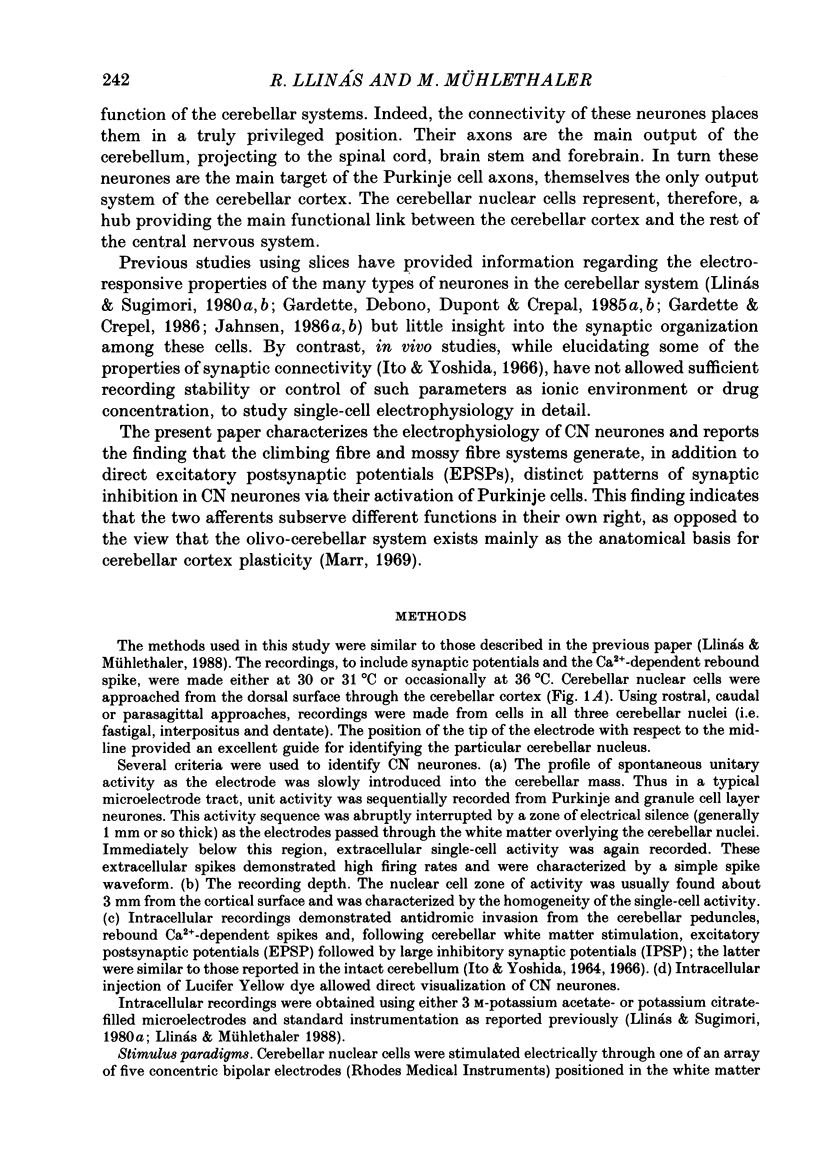
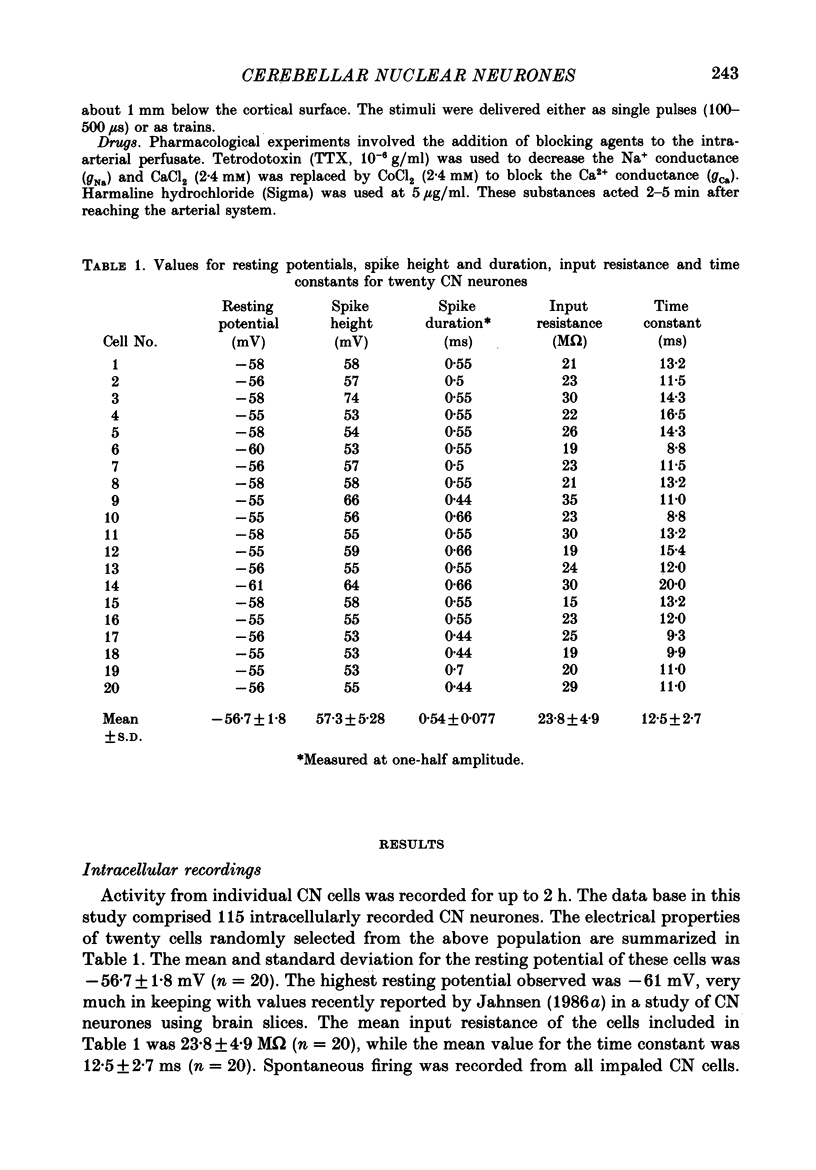
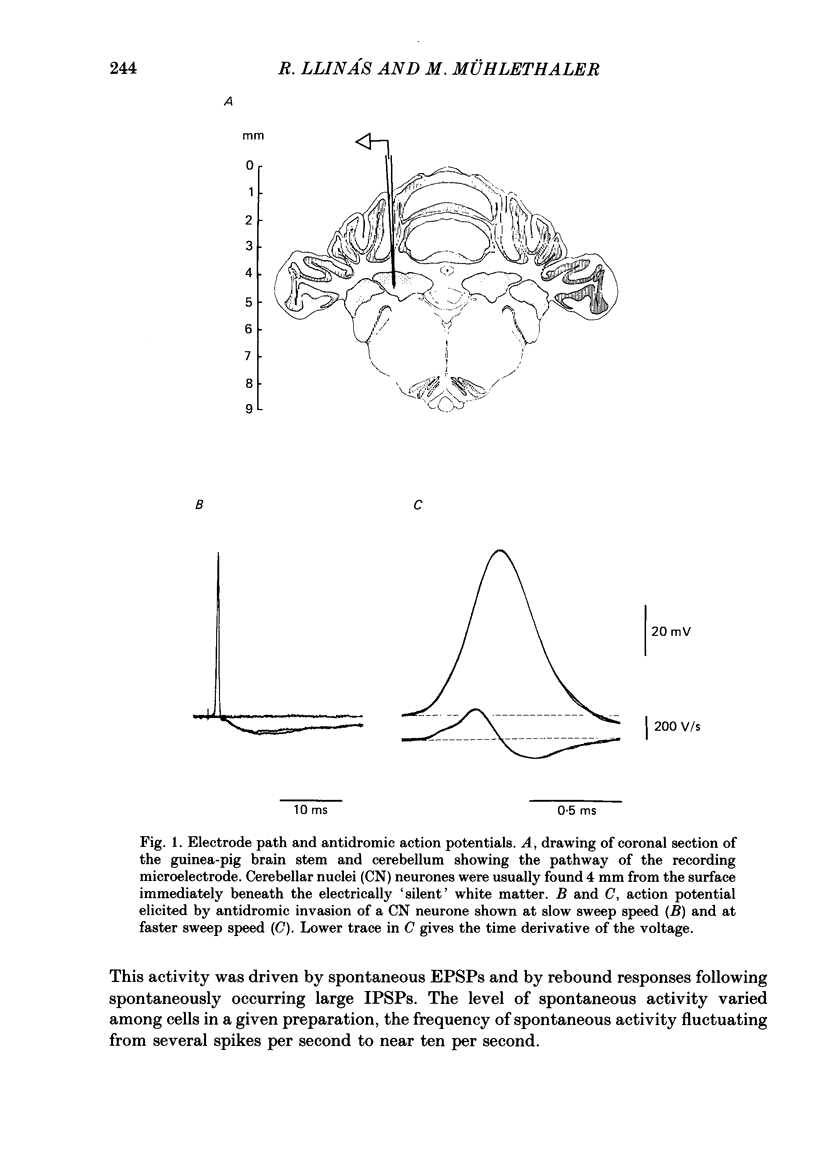
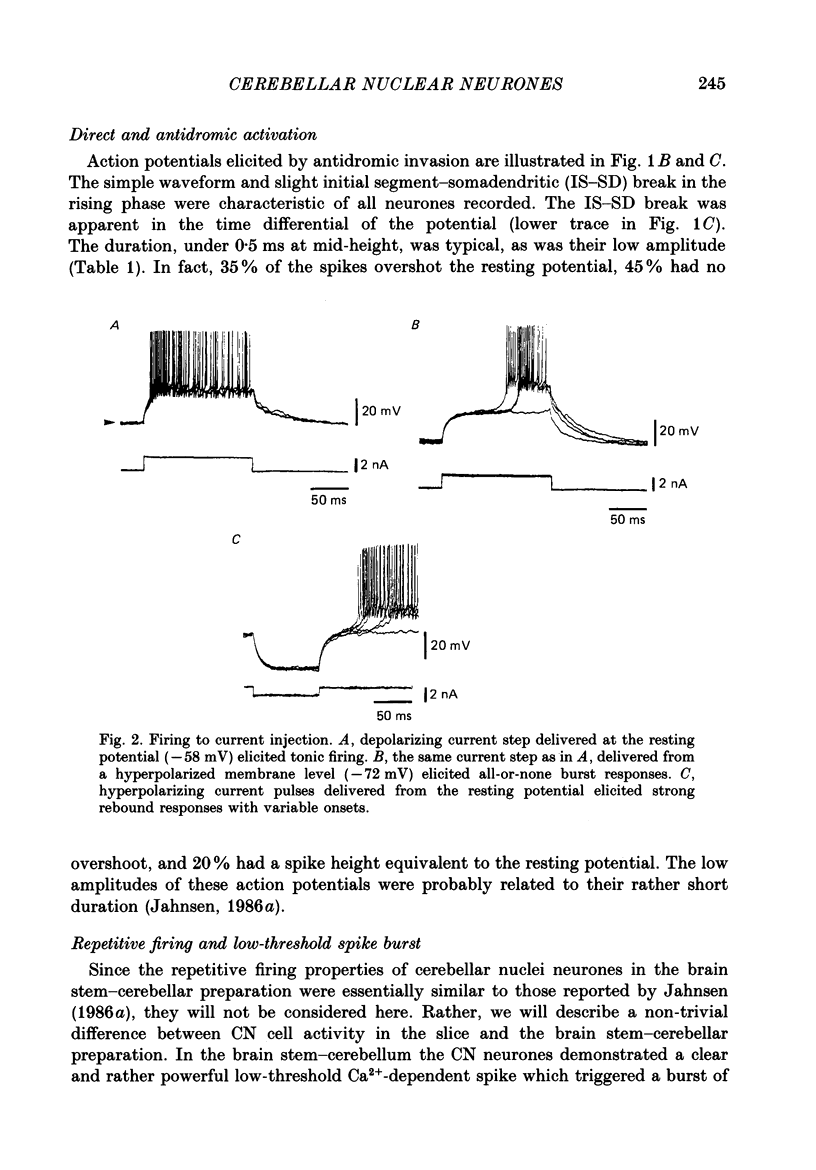
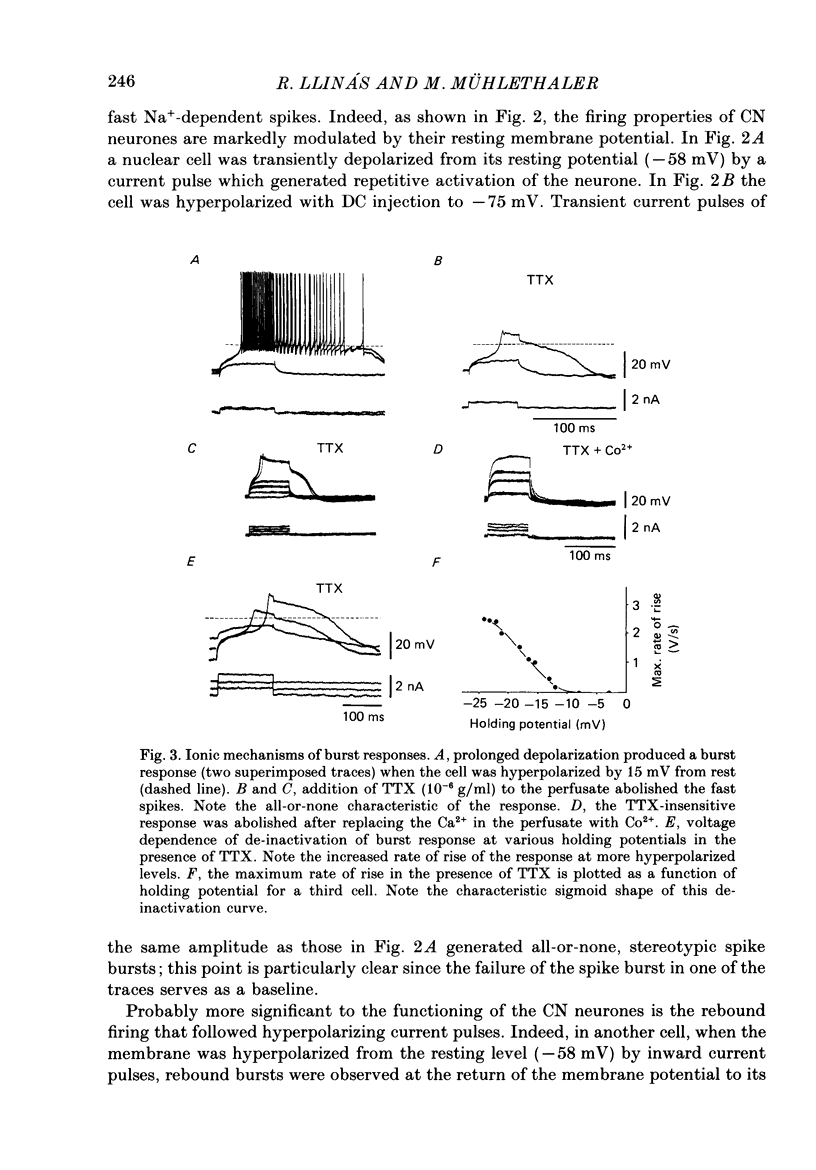
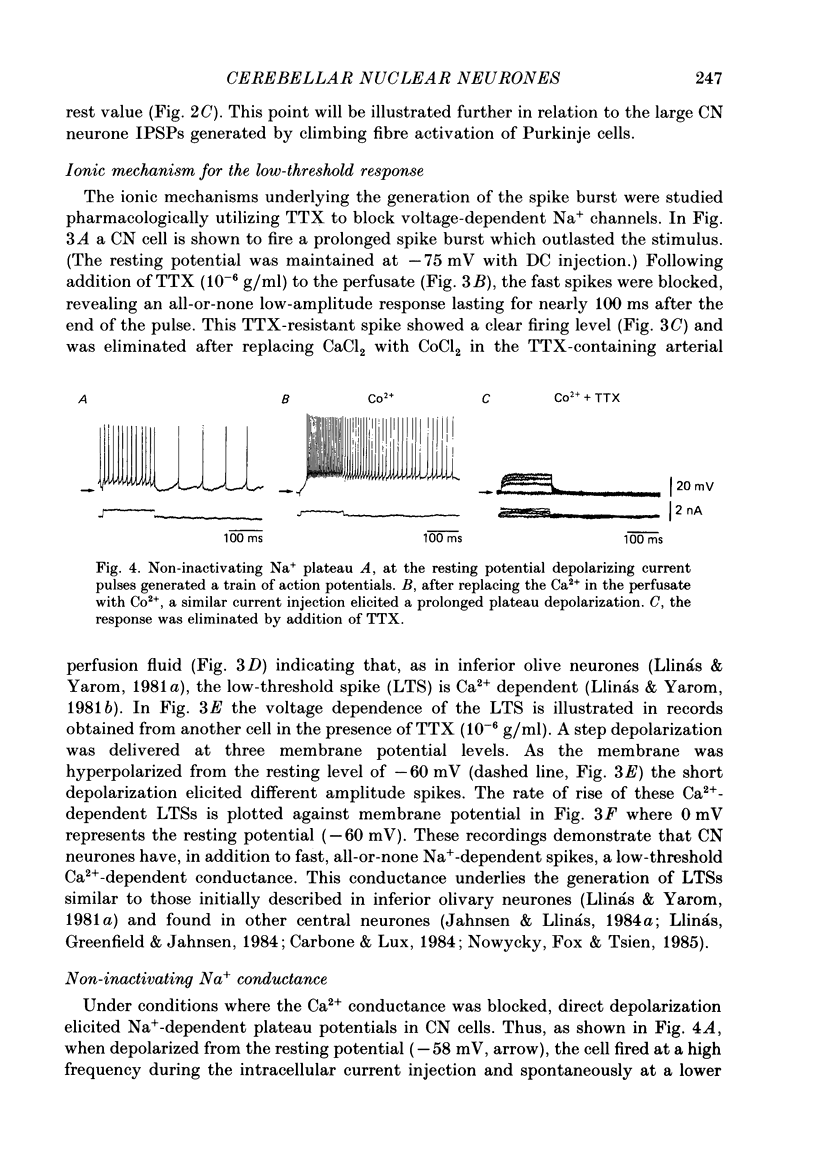
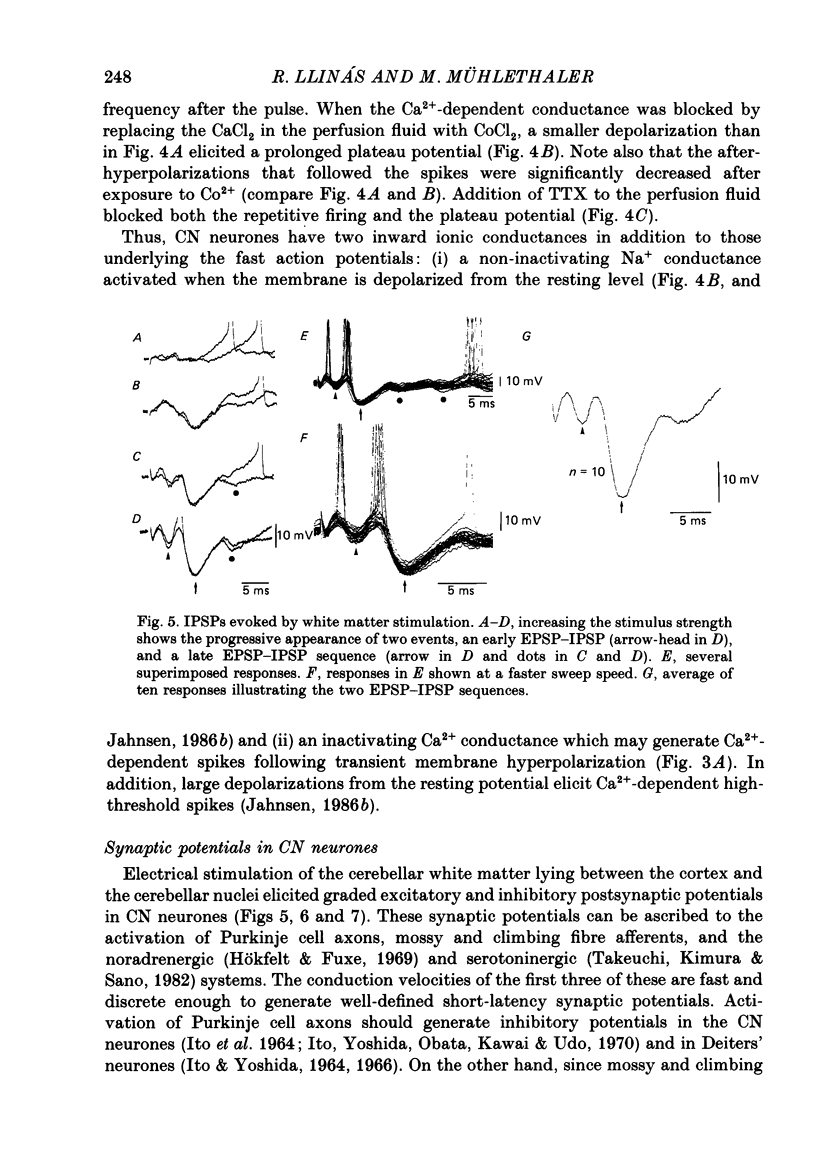
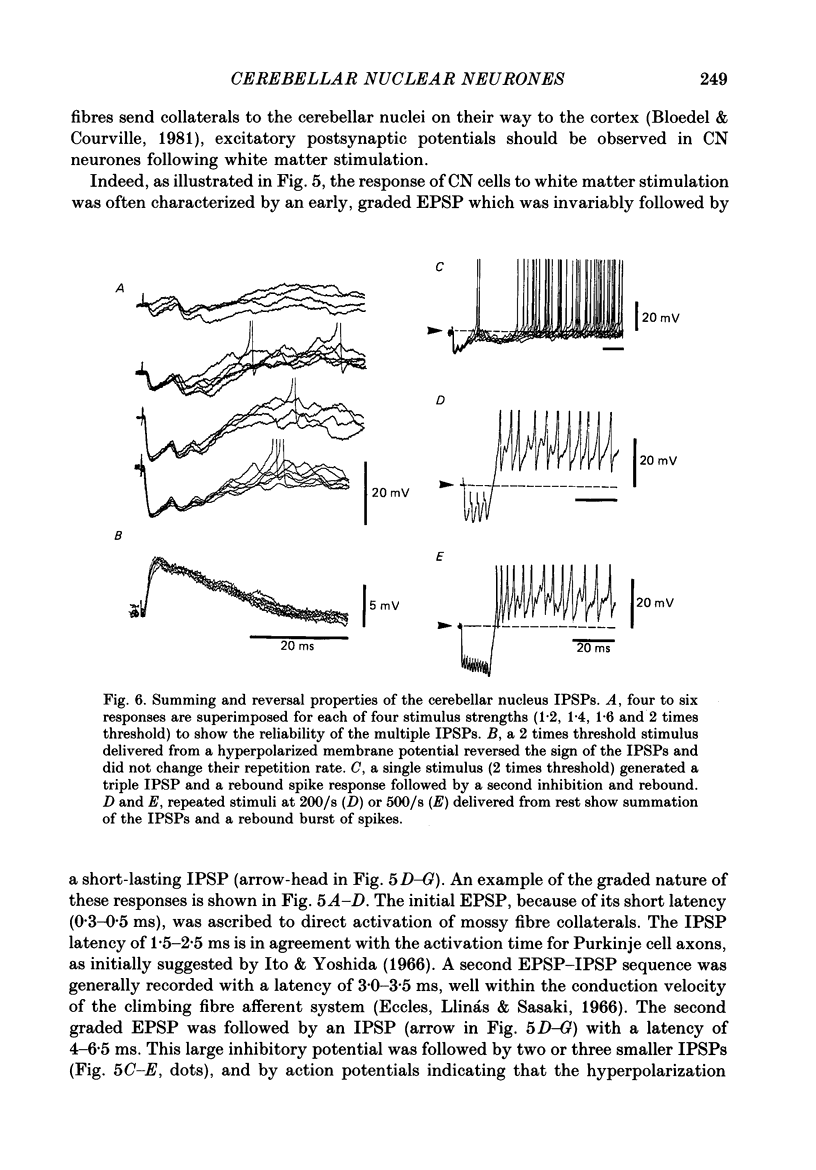
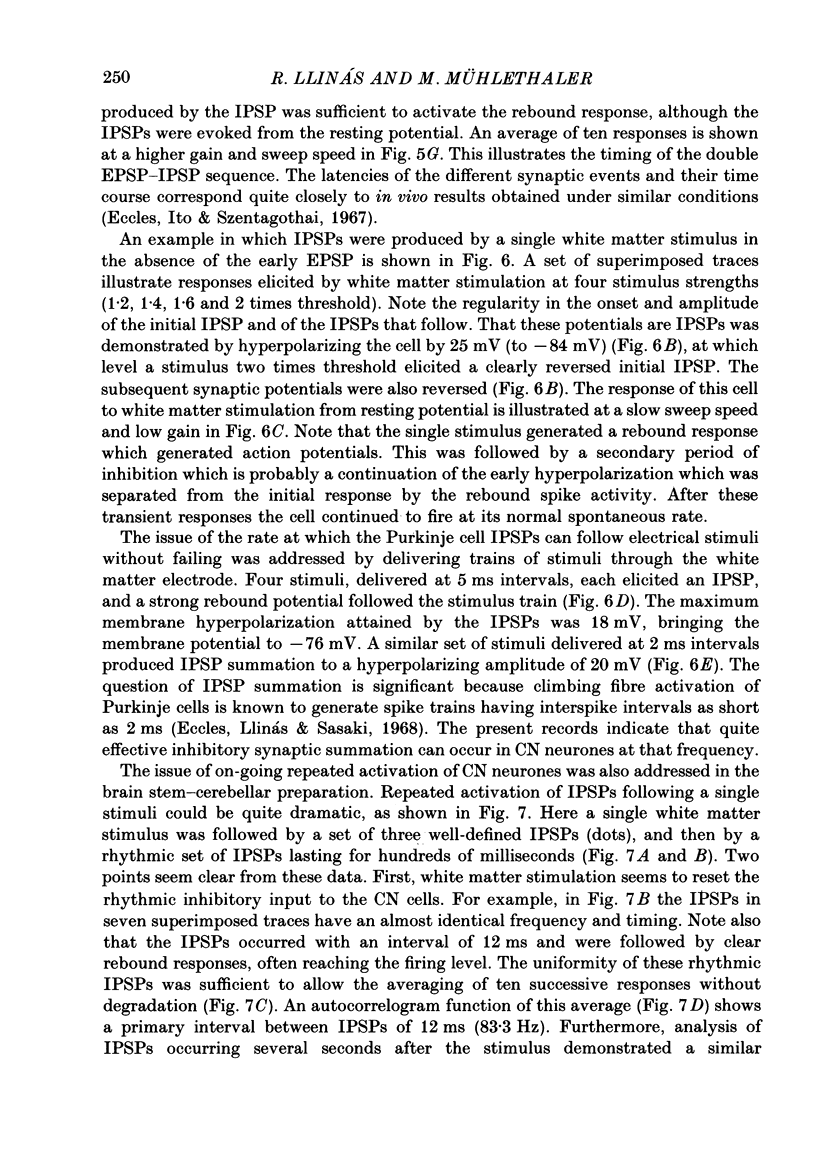
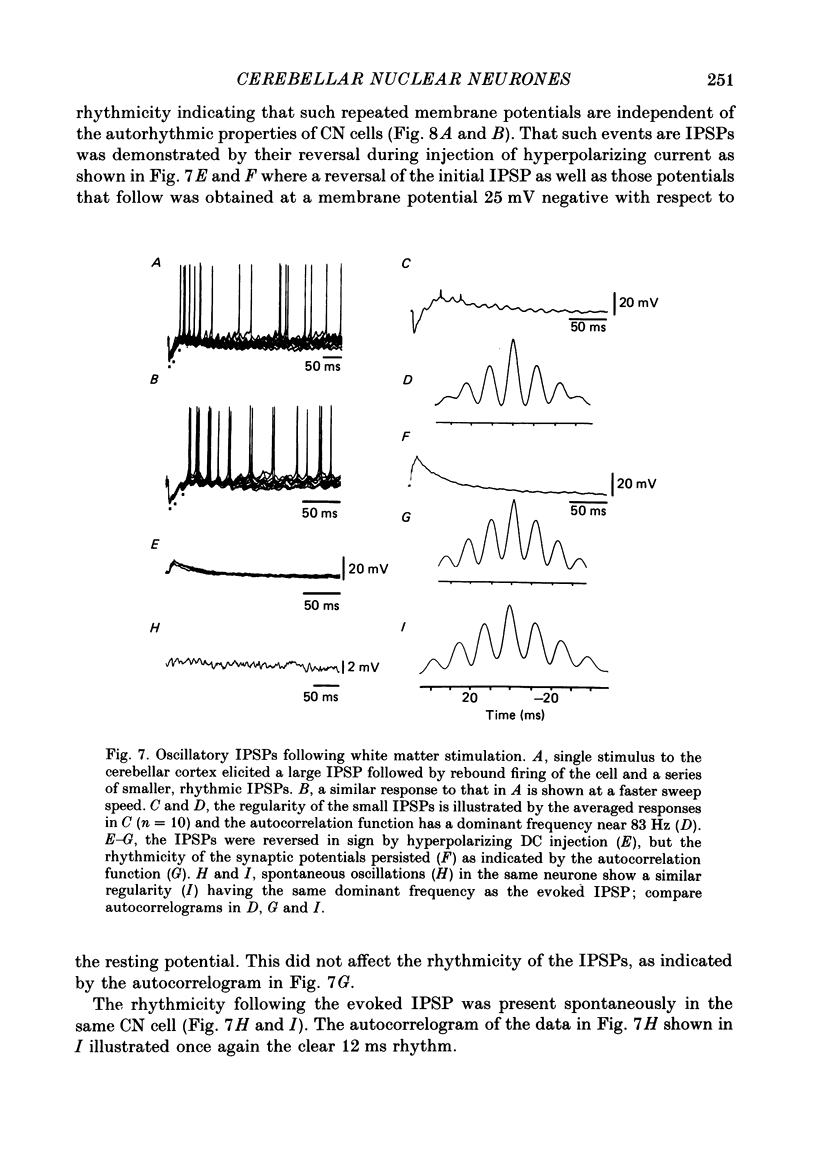
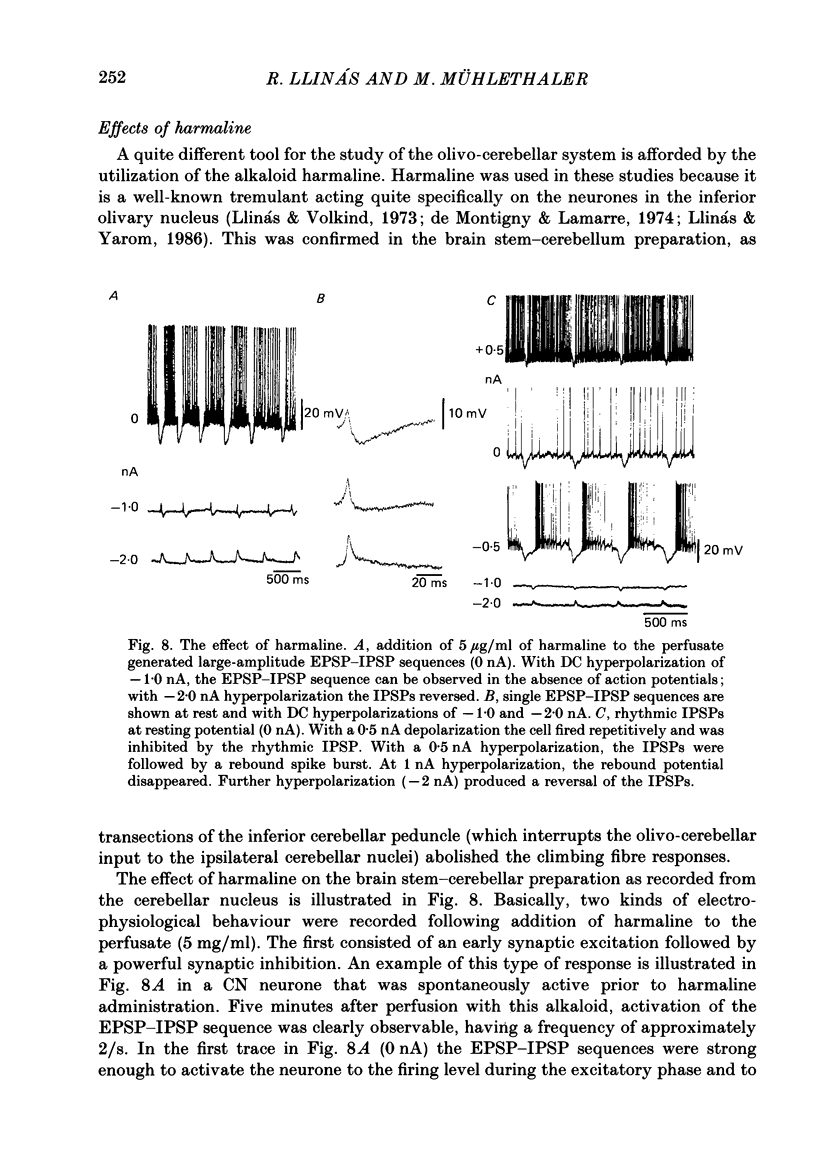
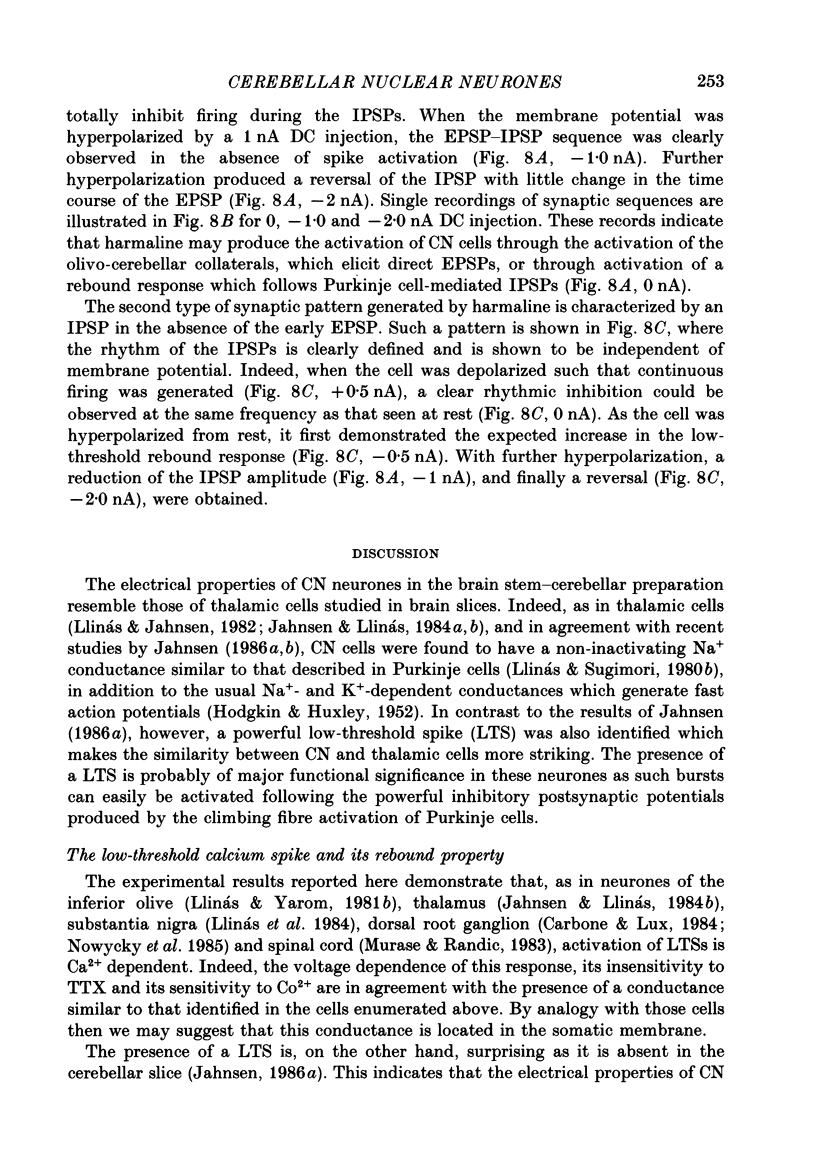
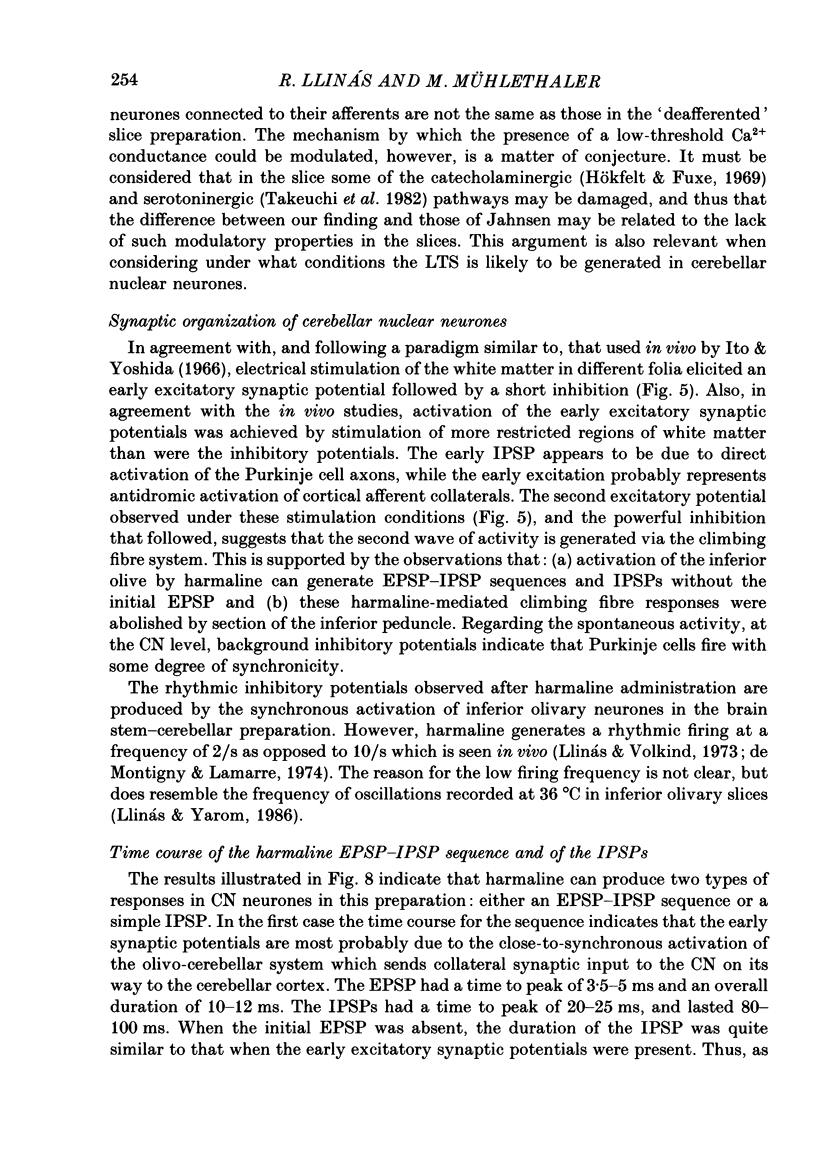
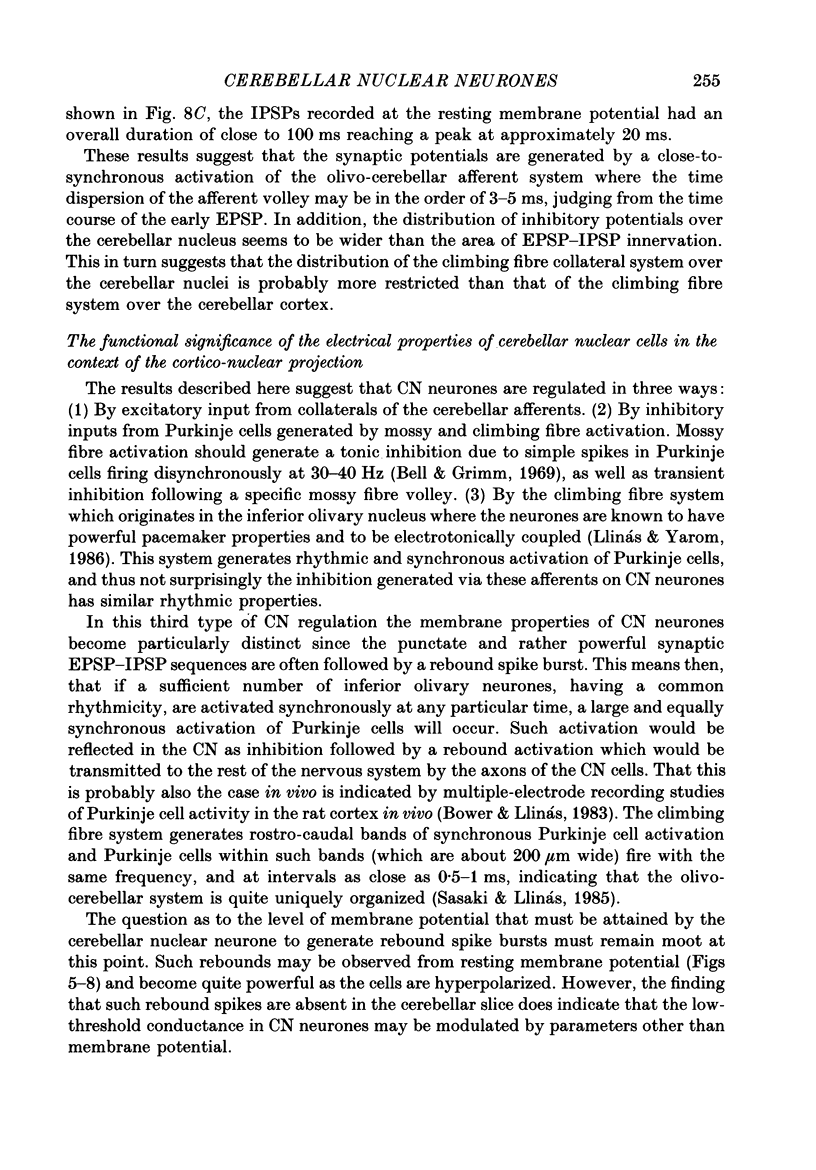
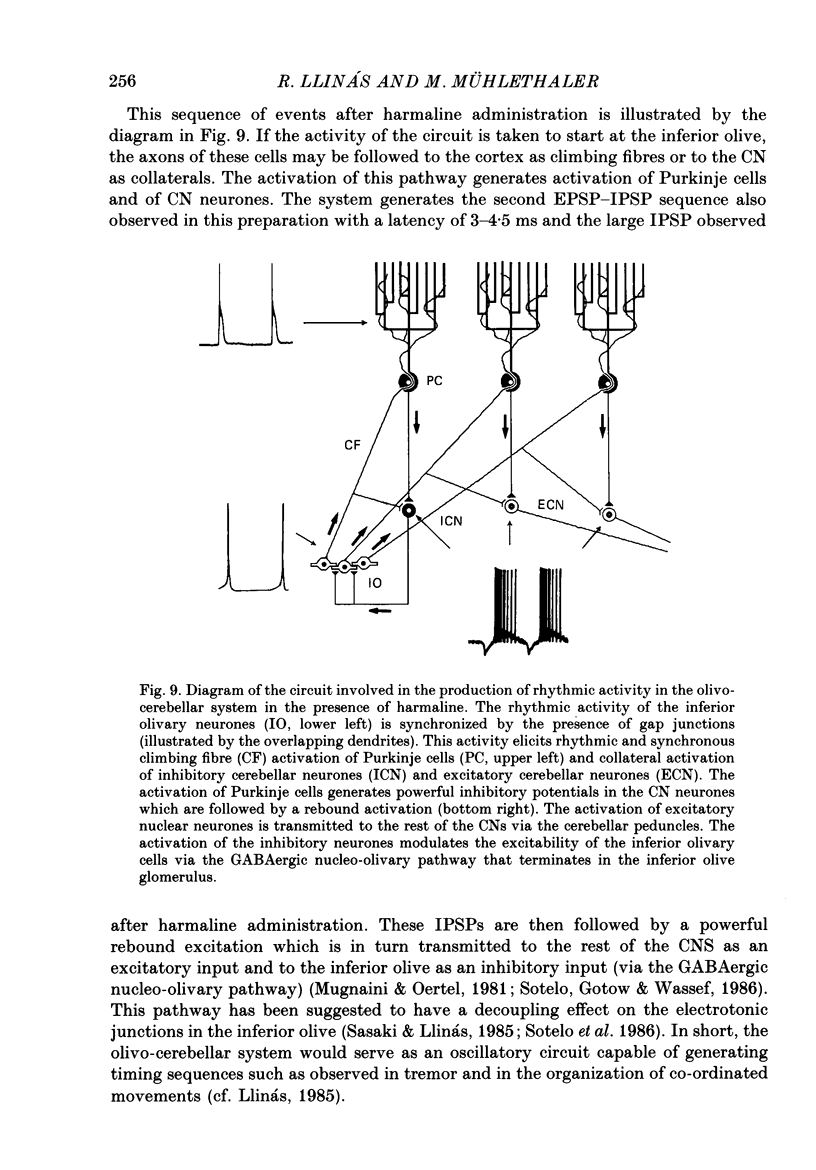
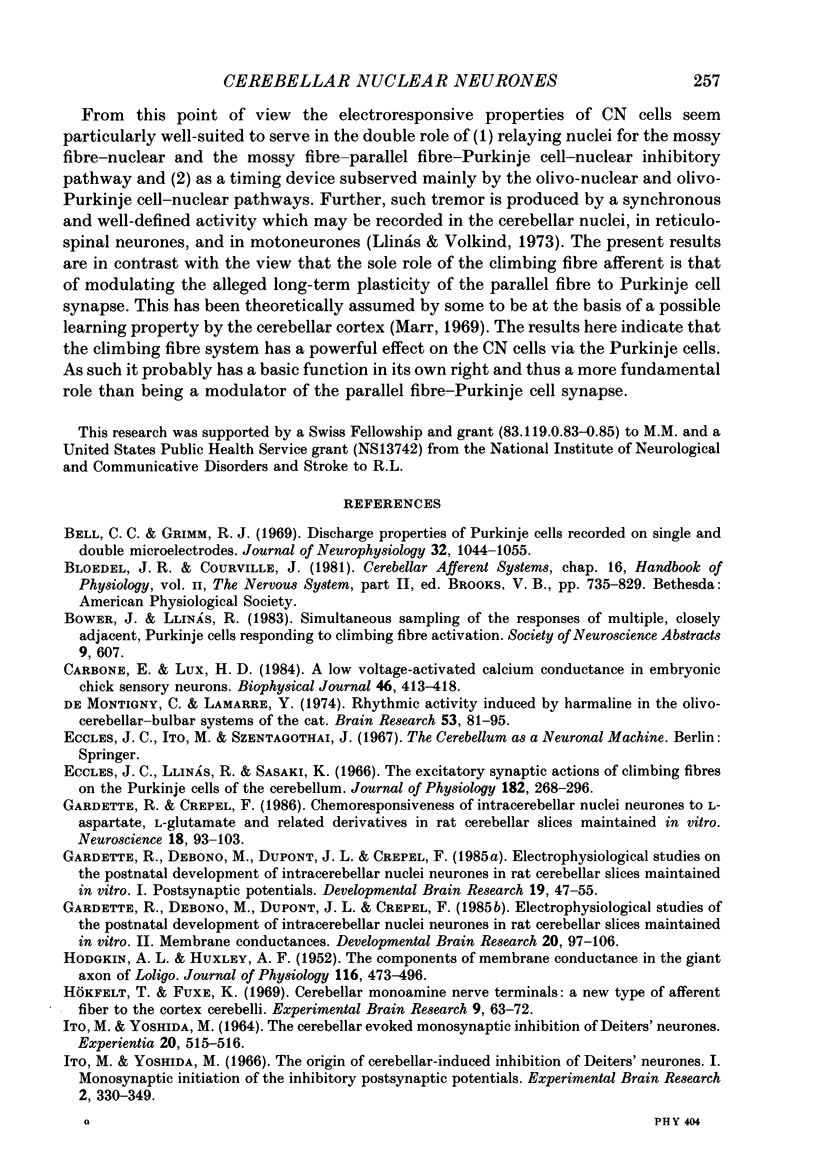
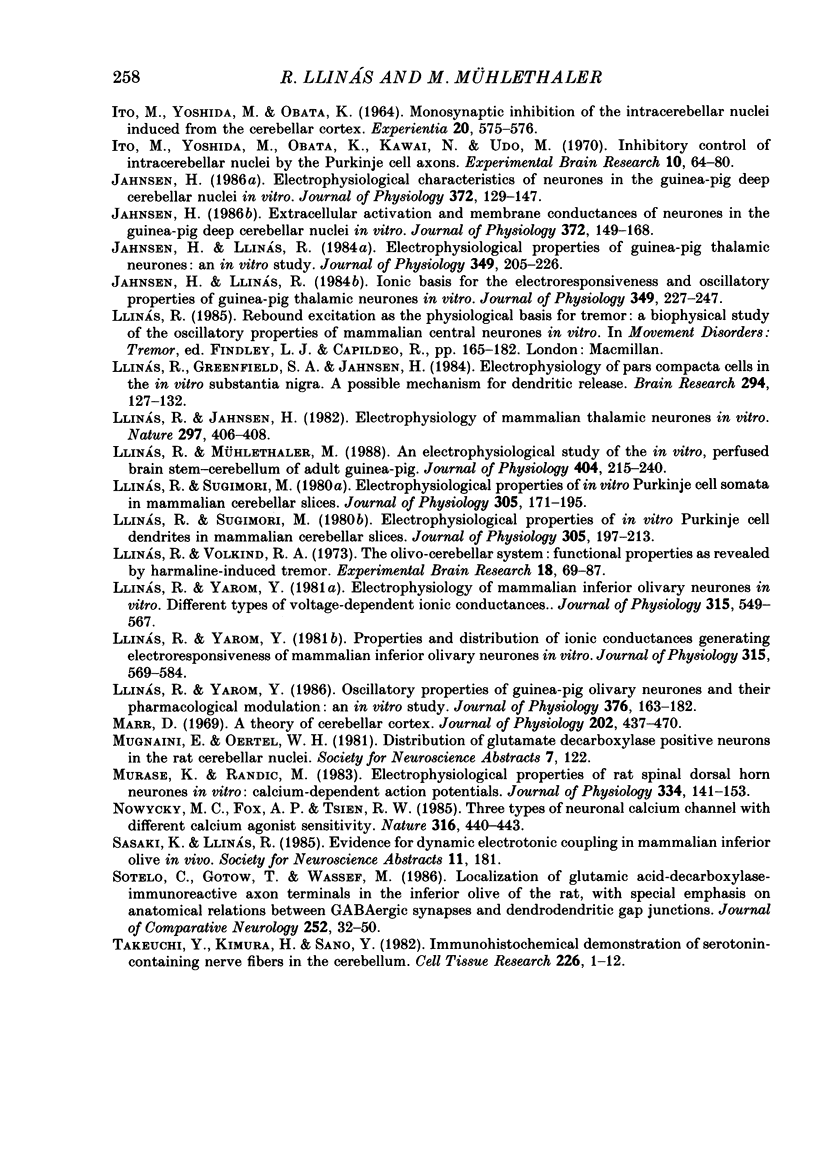
Selected References
These references are in PubMed. This may not be the complete list of references from this article.
- Bell C. C., Grimm R. J. Discharge properties of Purkinje cells recorded on single and double microelectrodes. J Neurophysiol. 1969 Nov;32(6):1044–1055. doi: 10.1152/jn.1969.32.6.1044. [DOI] [PubMed] [Google Scholar]
- Carbone E., Lux H. D. A low voltage-activated calcium conductance in embryonic chick sensory neurons. Biophys J. 1984 Sep;46(3):413–418. doi: 10.1016/S0006-3495(84)84037-0. [DOI] [PMC free article] [PubMed] [Google Scholar]
- Eccles J. C., Llinás R., Sasaki K. The excitatory synaptic action of climbing fibres on the Purkinje cells of the cerebellum. J Physiol. 1966 Jan;182(2):268–296. doi: 10.1113/jphysiol.1966.sp007824. [DOI] [PMC free article] [PubMed] [Google Scholar]
- Gardette R., Crepel F. Chemoresponsiveness of intracellular nuclei neurones to L-aspartate, L-glutamate and related derivatives in rat cerebellar slices maintained in vitro. Neuroscience. 1986 May;18(1):93–103. doi: 10.1016/0306-4522(86)90181-8. [DOI] [PubMed] [Google Scholar]
- HODGKIN A. L., HUXLEY A. F. The components of membrane conductance in the giant axon of Loligo. J Physiol. 1952 Apr;116(4):473–496. doi: 10.1113/jphysiol.1952.sp004718. [DOI] [PMC free article] [PubMed] [Google Scholar]
- Hökfelt T., Fuxe K. Cerebellar monoamine nerve terminals, a new type of afferent fibers to the cortex cerebelli. Exp Brain Res. 1969 Aug 19;9(1):63–72. doi: 10.1007/BF00235452. [DOI] [PubMed] [Google Scholar]
- Ito M., Yoshida M., Obata K., Kawai N., Udo M. Inhibitory control of intracerebellar nuclei by the purkinje cell axons. Exp Brain Res. 1970;10(1):64–80. doi: 10.1007/BF00340519. [DOI] [PubMed] [Google Scholar]
- Ito M., Yoshida M., Obata K. Monosynaptic inhibition of the intracerebellar nuclei induced rom the cerebellar cortex. Experientia. 1964 Oct 15;20(10):575–576. doi: 10.1007/BF02150304. [DOI] [PubMed] [Google Scholar]
- Ito M., Yoshida M. The cerebellar-evoked monosynaptic inhibition of Deiters' neurones. Experientia. 1964 Sep 15;20(9):515–516. doi: 10.1007/BF02154085. [DOI] [PubMed] [Google Scholar]
- Ito M., Yoshida M. The origin of cerebral-induced inhibition of Deiters neurones. I. Monosynaptic initiation of the inhibitory postsynaptic potentials. Exp Brain Res. 1966;2(4):330–349. doi: 10.1007/BF00234779. [DOI] [PubMed] [Google Scholar]
- Jahnsen H. Electrophysiological characteristics of neurones in the guinea-pig deep cerebellar nuclei in vitro. J Physiol. 1986 Mar;372:129–147. doi: 10.1113/jphysiol.1986.sp016001. [DOI] [PMC free article] [PubMed] [Google Scholar]
- Jahnsen H. Extracellular activation and membrane conductances of neurones in the guinea-pig deep cerebellar nuclei in vitro. J Physiol. 1986 Mar;372:149–168. doi: 10.1113/jphysiol.1986.sp016002. [DOI] [PMC free article] [PubMed] [Google Scholar]
- Jahnsen H., Llinás R. Electrophysiological properties of guinea-pig thalamic neurones: an in vitro study. J Physiol. 1984 Apr;349:205–226. doi: 10.1113/jphysiol.1984.sp015153. [DOI] [PMC free article] [PubMed] [Google Scholar]
- Jahnsen H., Llinás R. Ionic basis for the electro-responsiveness and oscillatory properties of guinea-pig thalamic neurones in vitro. J Physiol. 1984 Apr;349:227–247. doi: 10.1113/jphysiol.1984.sp015154. [DOI] [PMC free article] [PubMed] [Google Scholar]
- Llinás R., Greenfield S. A., Jahnsen H. Electrophysiology of pars compacta cells in the in vitro substantia nigra--a possible mechanism for dendritic release. Brain Res. 1984 Feb 27;294(1):127–132. doi: 10.1016/0006-8993(84)91316-7. [DOI] [PubMed] [Google Scholar]
- Llinás R., Jahnsen H. Electrophysiology of mammalian thalamic neurones in vitro. Nature. 1982 Jun 3;297(5865):406–408. doi: 10.1038/297406a0. [DOI] [PubMed] [Google Scholar]
- Llinás R., Mühlethaler M. An electrophysiological study of the in vitro, perfused brain stem-cerebellum of adult guinea-pig. J Physiol. 1988 Oct;404:215–240. doi: 10.1113/jphysiol.1988.sp017287. [DOI] [PMC free article] [PubMed] [Google Scholar]
- Llinás R., Sugimori M. Electrophysiological properties of in vitro Purkinje cell dendrites in mammalian cerebellar slices. J Physiol. 1980 Aug;305:197–213. doi: 10.1113/jphysiol.1980.sp013358. [DOI] [PMC free article] [PubMed] [Google Scholar]
- Llinás R., Sugimori M. Electrophysiological properties of in vitro Purkinje cell somata in mammalian cerebellar slices. J Physiol. 1980 Aug;305:171–195. doi: 10.1113/jphysiol.1980.sp013357. [DOI] [PMC free article] [PubMed] [Google Scholar]
- Llinás R., Volkind R. A. The olivo-cerebellar system: functional properties as revealed by harmaline-induced tremor. Exp Brain Res. 1973 Aug 31;18(1):69–87. doi: 10.1007/BF00236557. [DOI] [PubMed] [Google Scholar]
- Llinás R., Yarom Y. Electrophysiology of mammalian inferior olivary neurones in vitro. Different types of voltage-dependent ionic conductances. J Physiol. 1981 Jun;315:549–567. doi: 10.1113/jphysiol.1981.sp013763. [DOI] [PMC free article] [PubMed] [Google Scholar]
- Llinás R., Yarom Y. Oscillatory properties of guinea-pig inferior olivary neurones and their pharmacological modulation: an in vitro study. J Physiol. 1986 Jul;376:163–182. doi: 10.1113/jphysiol.1986.sp016147. [DOI] [PMC free article] [PubMed] [Google Scholar]
- Llinás R., Yarom Y. Properties and distribution of ionic conductances generating electroresponsiveness of mammalian inferior olivary neurones in vitro. J Physiol. 1981 Jun;315:569–584. doi: 10.1113/jphysiol.1981.sp013764. [DOI] [PMC free article] [PubMed] [Google Scholar]
- Marr D. A theory of cerebellar cortex. J Physiol. 1969 Jun;202(2):437–470. doi: 10.1113/jphysiol.1969.sp008820. [DOI] [PMC free article] [PubMed] [Google Scholar]
- Murase K., Randić M. Electrophysiological properties of rat spinal dorsal horn neurones in vitro: calcium-dependent action potentials. J Physiol. 1983 Jan;334:141–153. doi: 10.1113/jphysiol.1983.sp014485. [DOI] [PMC free article] [PubMed] [Google Scholar]
- Nowycky M. C., Fox A. P., Tsien R. W. Three types of neuronal calcium channel with different calcium agonist sensitivity. Nature. 1985 Aug 1;316(6027):440–443. doi: 10.1038/316440a0. [DOI] [PubMed] [Google Scholar]
- Sotelo C., Gotow T., Wassef M. Localization of glutamic-acid-decarboxylase-immunoreactive axon terminals in the inferior olive of the rat, with special emphasis on anatomical relations between GABAergic synapses and dendrodendritic gap junctions. J Comp Neurol. 1986 Oct 1;252(1):32–50. doi: 10.1002/cne.902520103. [DOI] [PubMed] [Google Scholar]
- Takeuchi Y., Kimura H., Sano Y. Immunohistochemical demonstration of serotonin-containing nerve fibers in the cerebellum. Cell Tissue Res. 1982;226(1):1–12. doi: 10.1007/BF00217077. [DOI] [PubMed] [Google Scholar]
- de Montigny C., Lamarre Y. Rhythmic activity induced by harmaline in the olivo-cerebello-bulbar system of the cat. Brain Res. 1973 Apr 13;53(1):81–95. doi: 10.1016/0006-8993(73)90768-3. [DOI] [PubMed] [Google Scholar]