Abstract
1. We evaluated human visual cortical contrast gain using visual evoked potential (VEP) measurements. The steady-state VEP was elicited by 7.5 Hz contrast modulation of a 6 cycles/deg sinusoidal grating. The stimulus may be regarded as the sum of a steady grating (C) and a counterphase grating of the same spatial frequency (delta C). The counterphase grating is modulated sinusoidally in time. 2. The VEP was measured to combinations of different modulation contrasts (delta C) and different mean levels of grating contrast (C) which produced stimuli with contrast modulation depths (delta C/C) ranging from 0.0625 to 1.0 ('on-off'). 3. The VEP signals were Fourier analysed and the amplitude and phase of the first (7.5 Hz) and second (15 Hz) harmonic frequency components were examined. The monocular VEP to a contrast-modulated grating contains significant first and second harmonic frequency components. 4. The amplitude and phase of the monocular VEP was plotted as a function of delta C for each mean level of contrast explored. The amplitudes of both the first and second harmonic frequency components grow with increasing delta C. However, the slope of each function depends on the mean contrast (C): with higher levels of C, the slope of the function is more shallow. Furthermore, at each level of C the amplitude of the first harmonic frequency saturates at a lower delta C than does the second harmonic frequency component. VEP amplitude is therefore not determined by the absolute contrast change (delta C) alone. The VEP phase of the first harmonic frequency shows less dependence on either modulation or on mean contrast; the phase of the second harmonic frequency component is strongly dependent on mean contrast (C) but not on delta C. 5. When the second harmonic amplitude component of the VEP response (R) is expressed as Ractual/Rmax, where Rmax is the response to C = delta C (i.e. 'on-off'), all second harmonic VEP functions can be well fitted with a power function. This is not the case for the function of the first harmonic amplitude data. 6. A dichoptic VEP was obtained by presenting the steady and counterphase gratings to opposite eyes. The dichoptic VEP, in distinction to the monocular VEP, contains only a second harmonic frequency component. The amplitude of the second harmonic frequency component grows with increasing delta C, similar to the function seen for the monocular VEP.(ABSTRACT TRUNCATED AT 400 WORDS)
Full text
PDF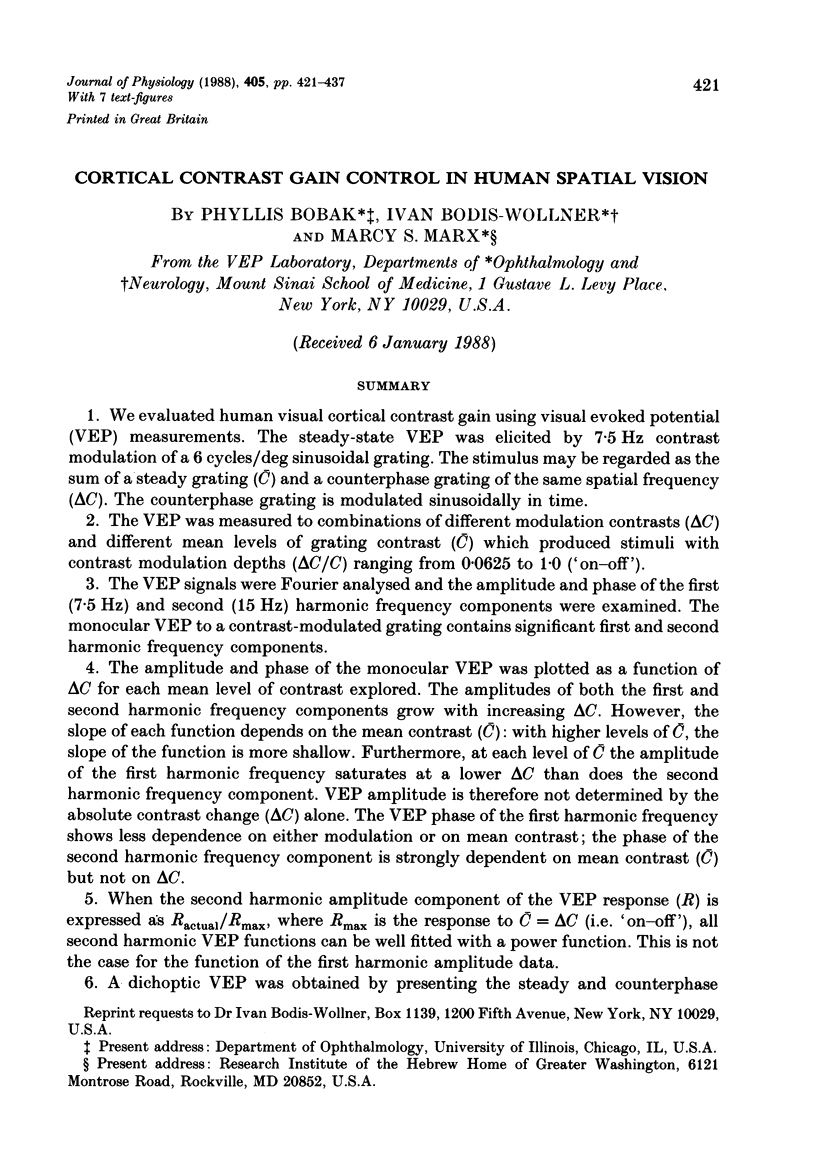
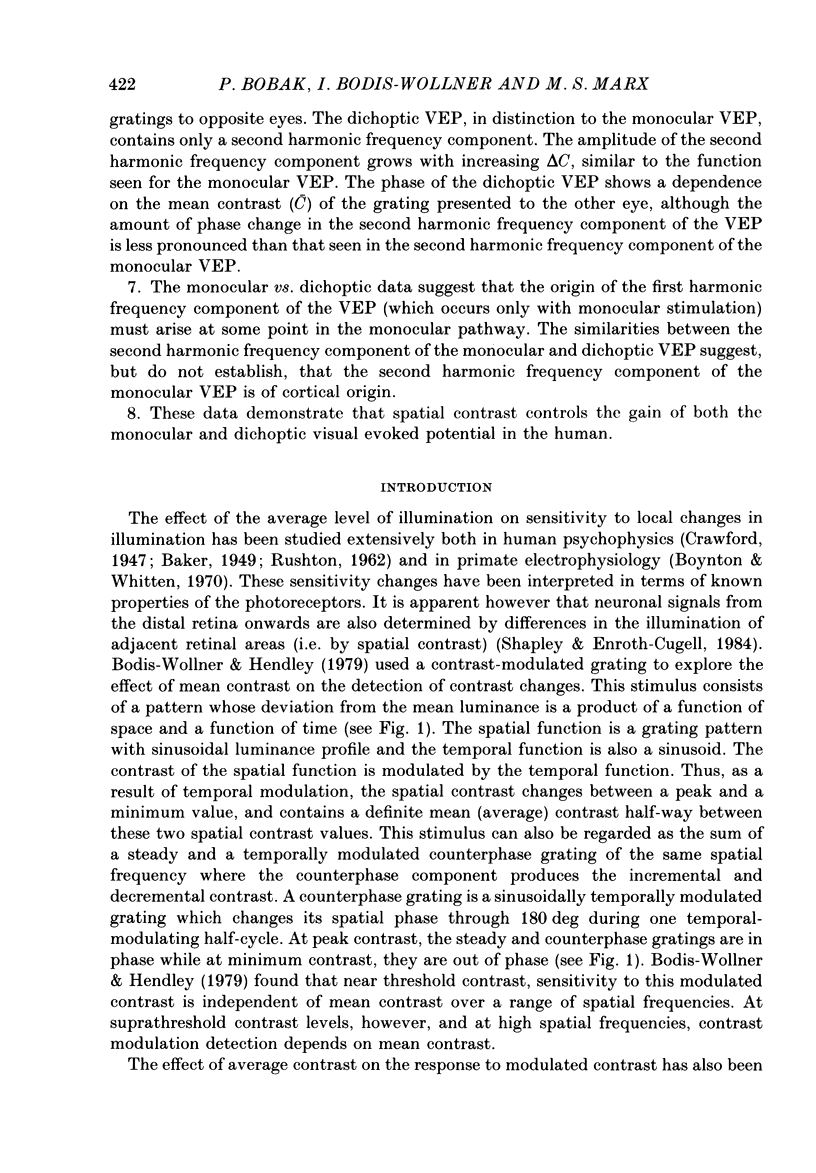
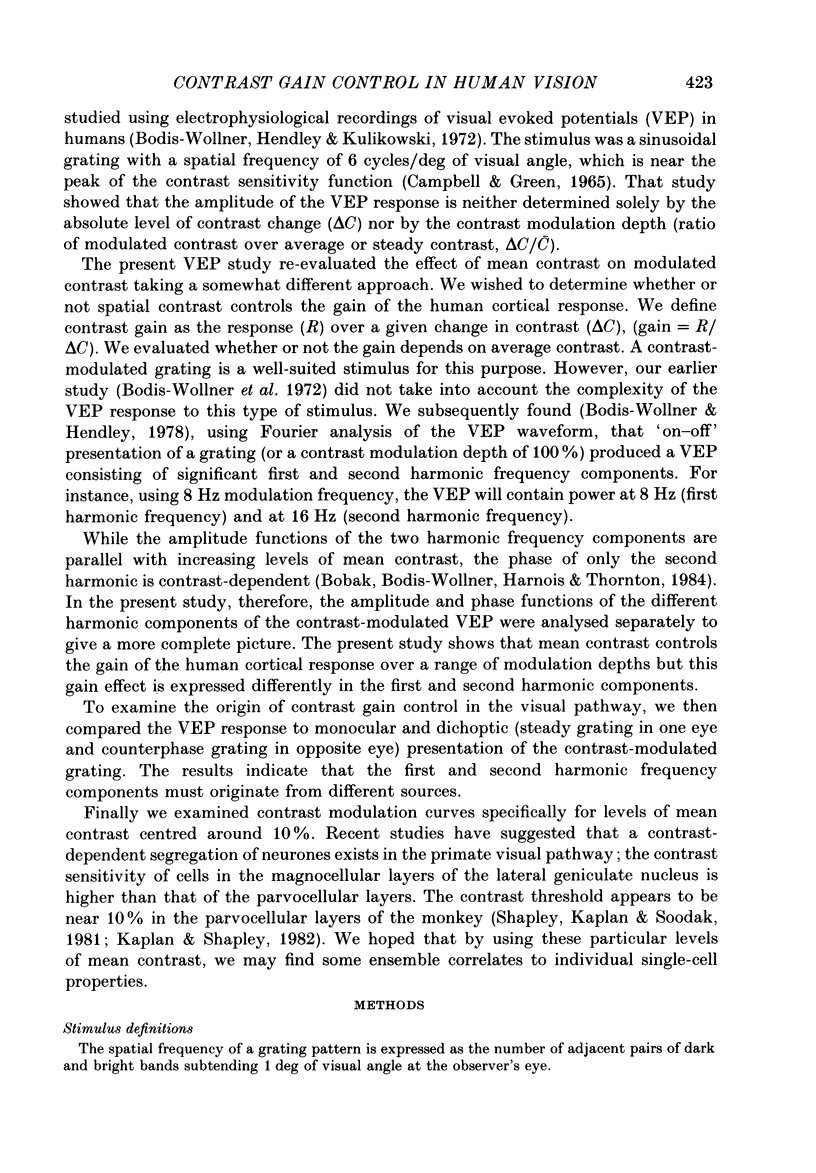
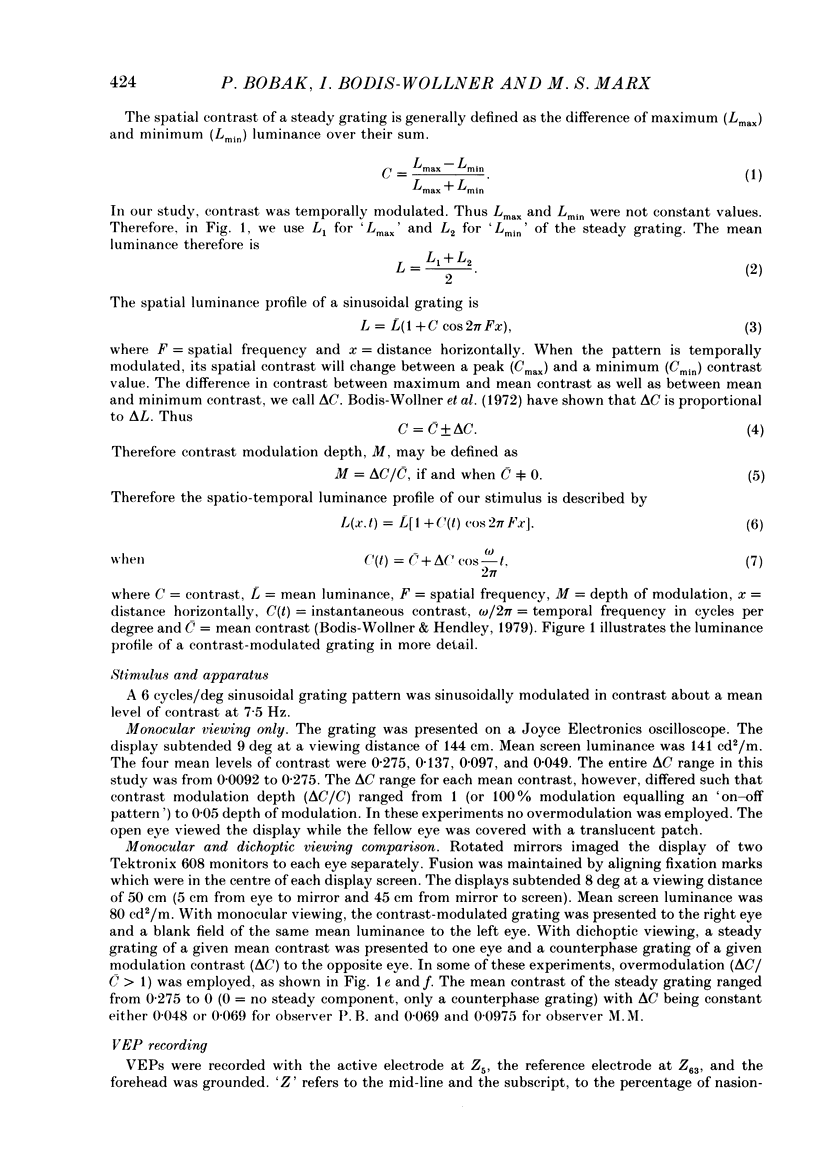
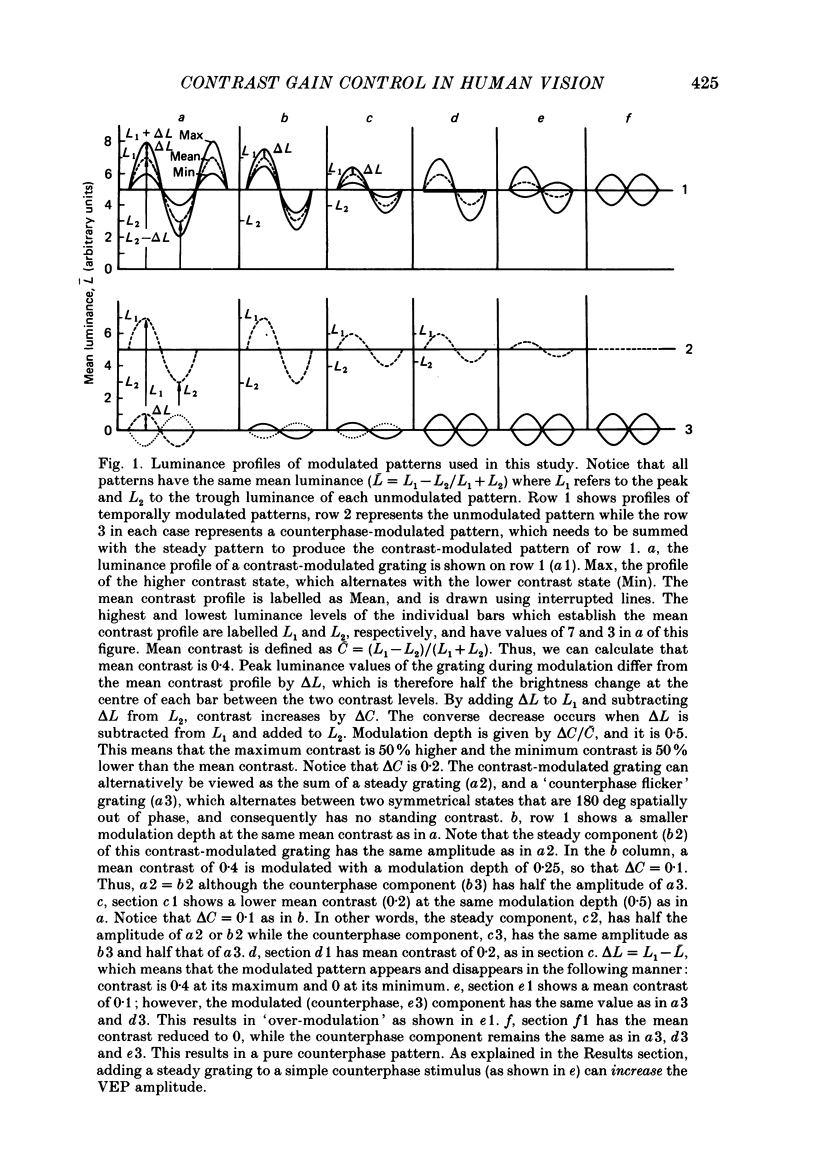
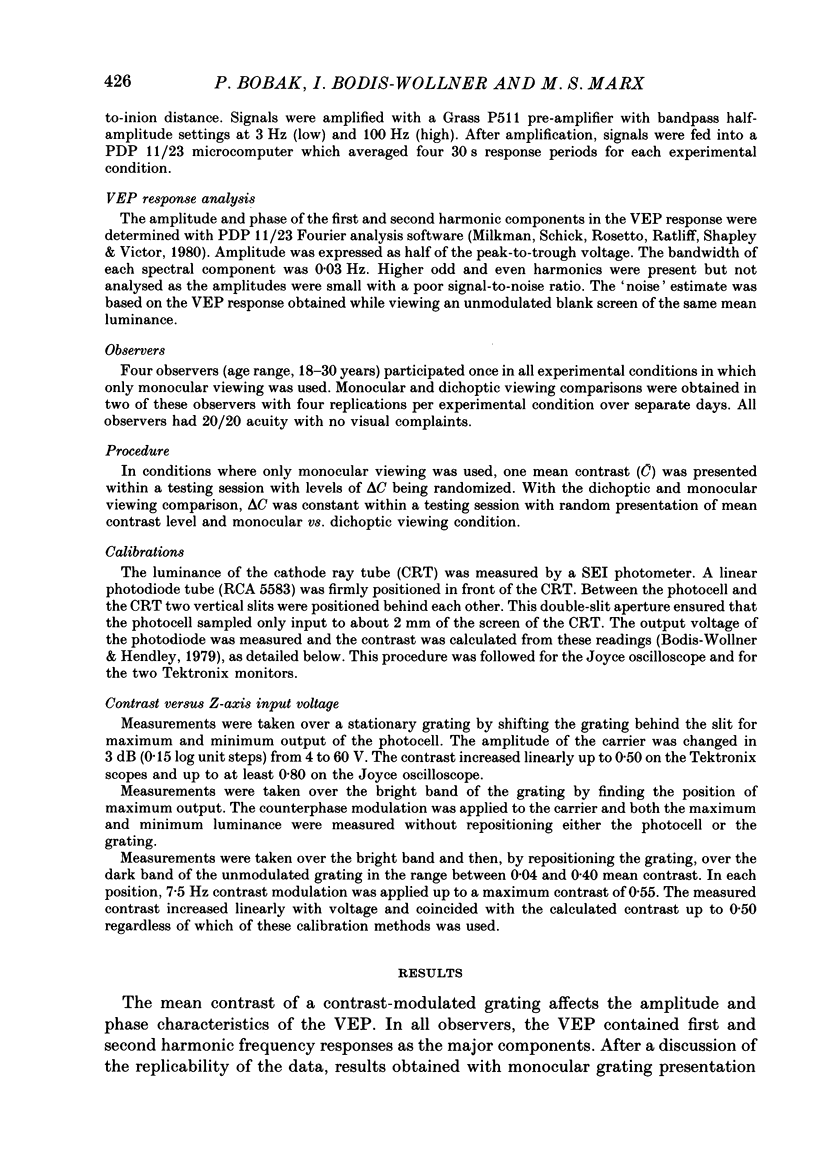
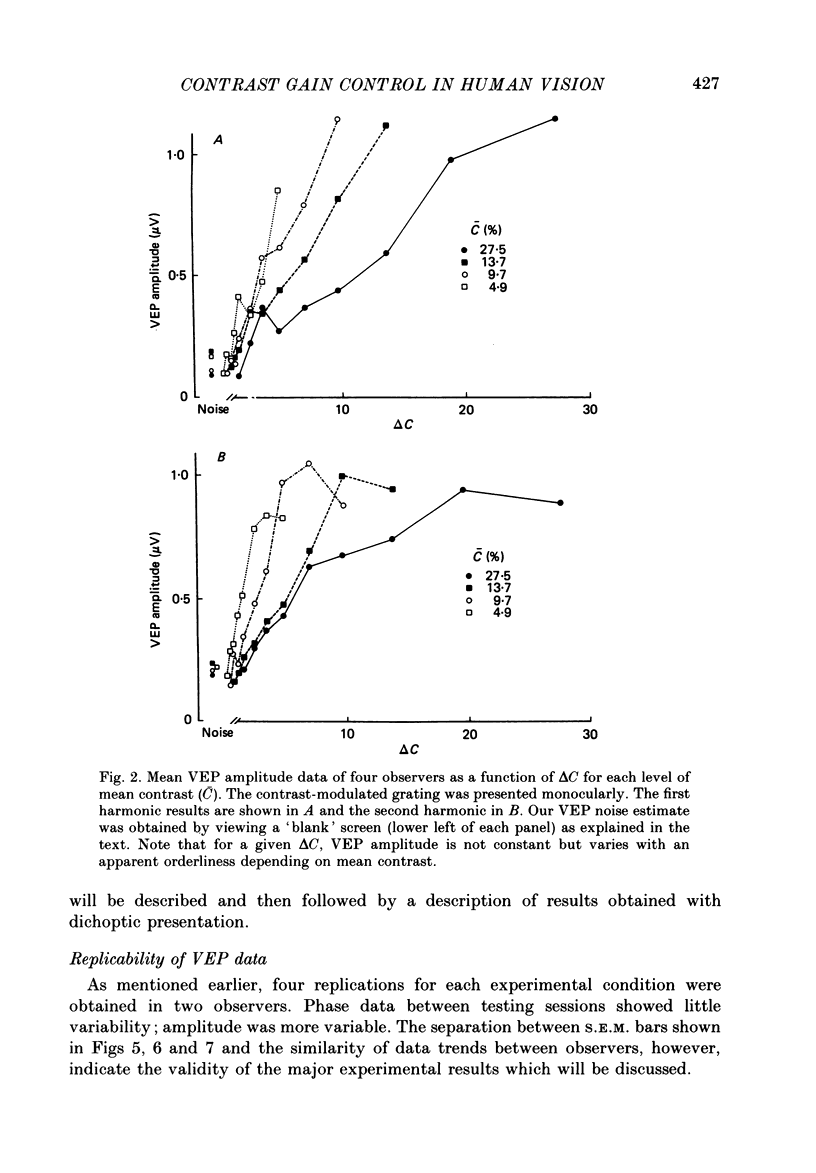
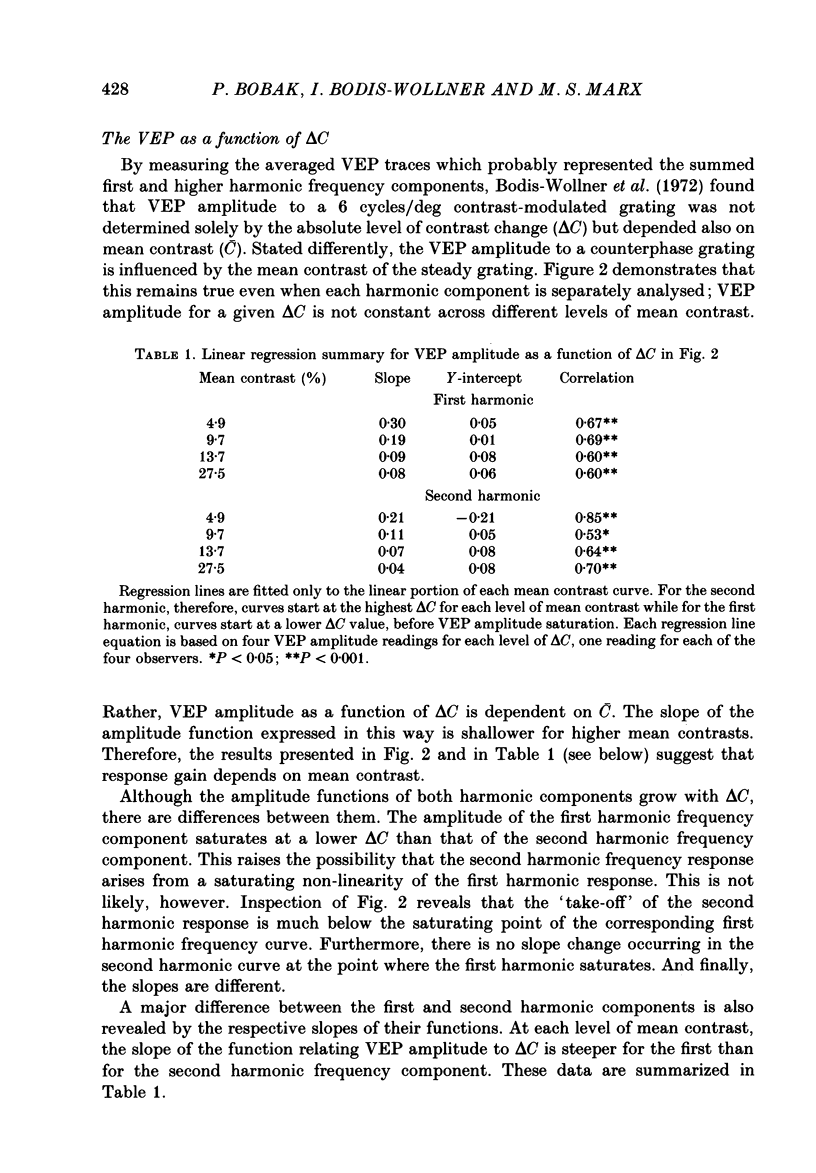
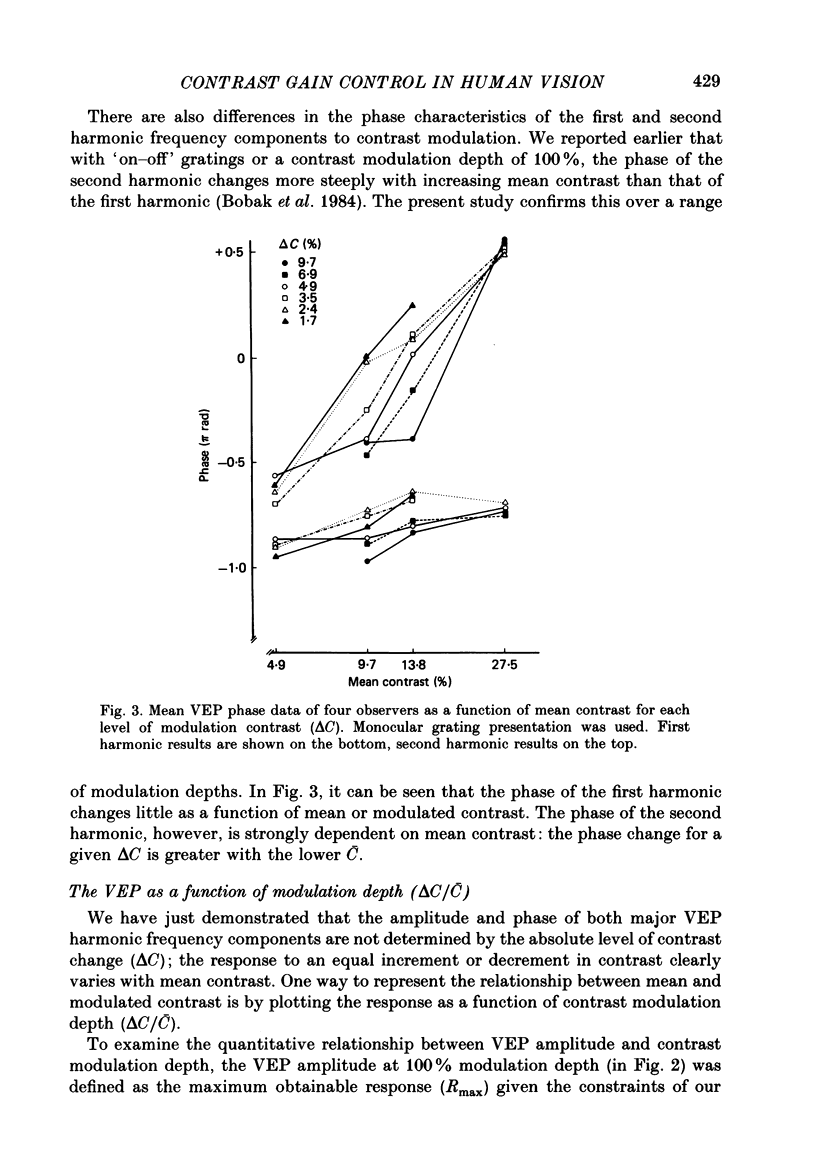
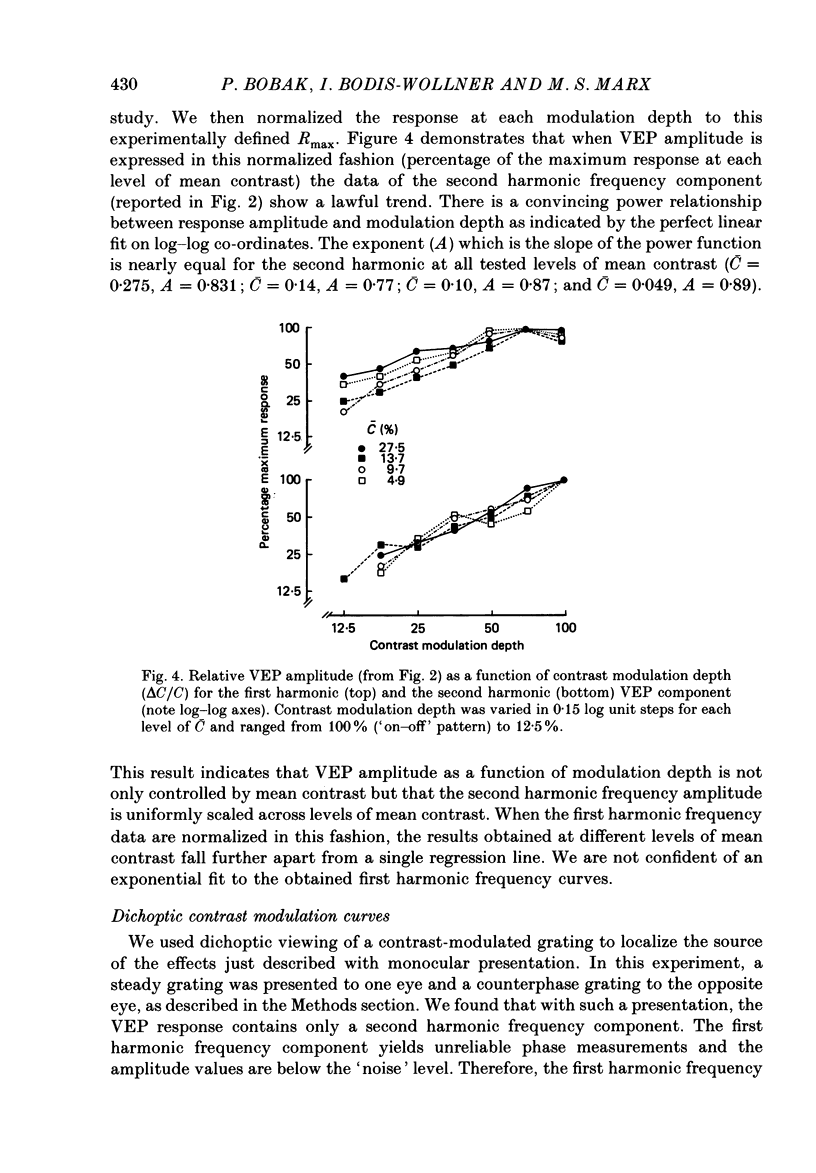
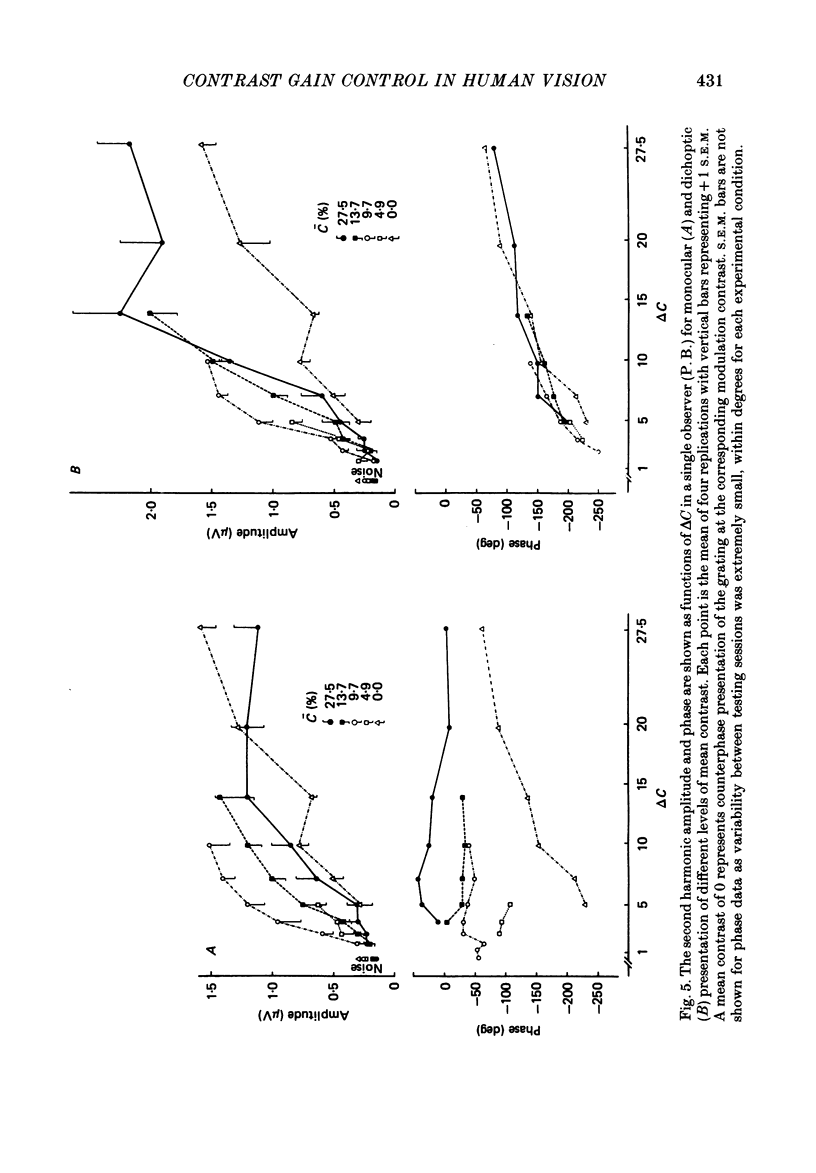
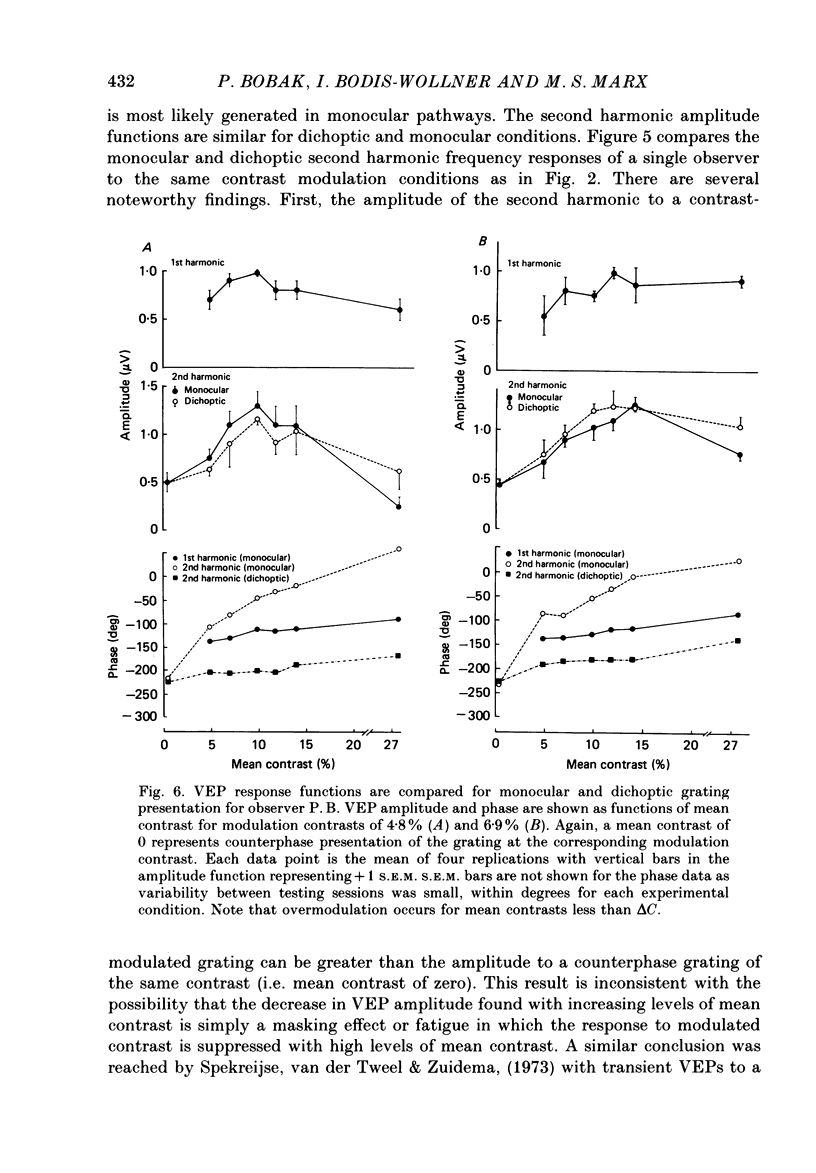
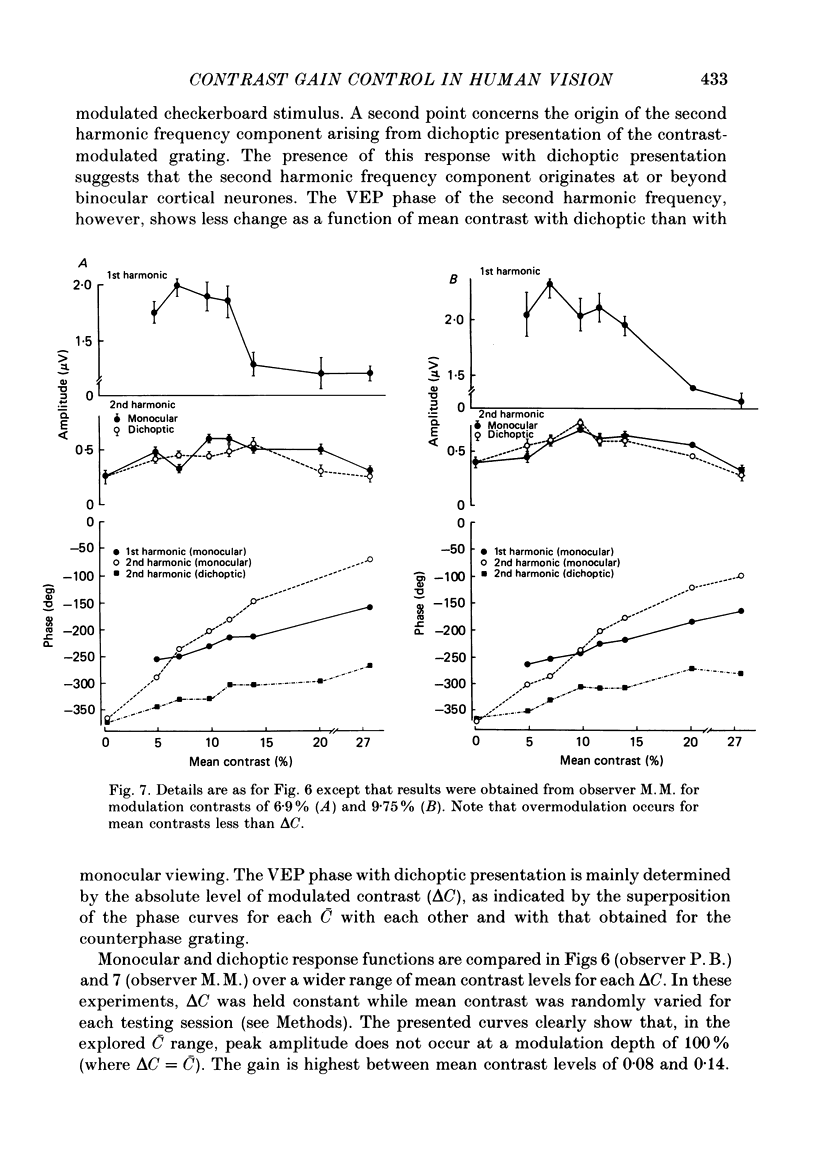
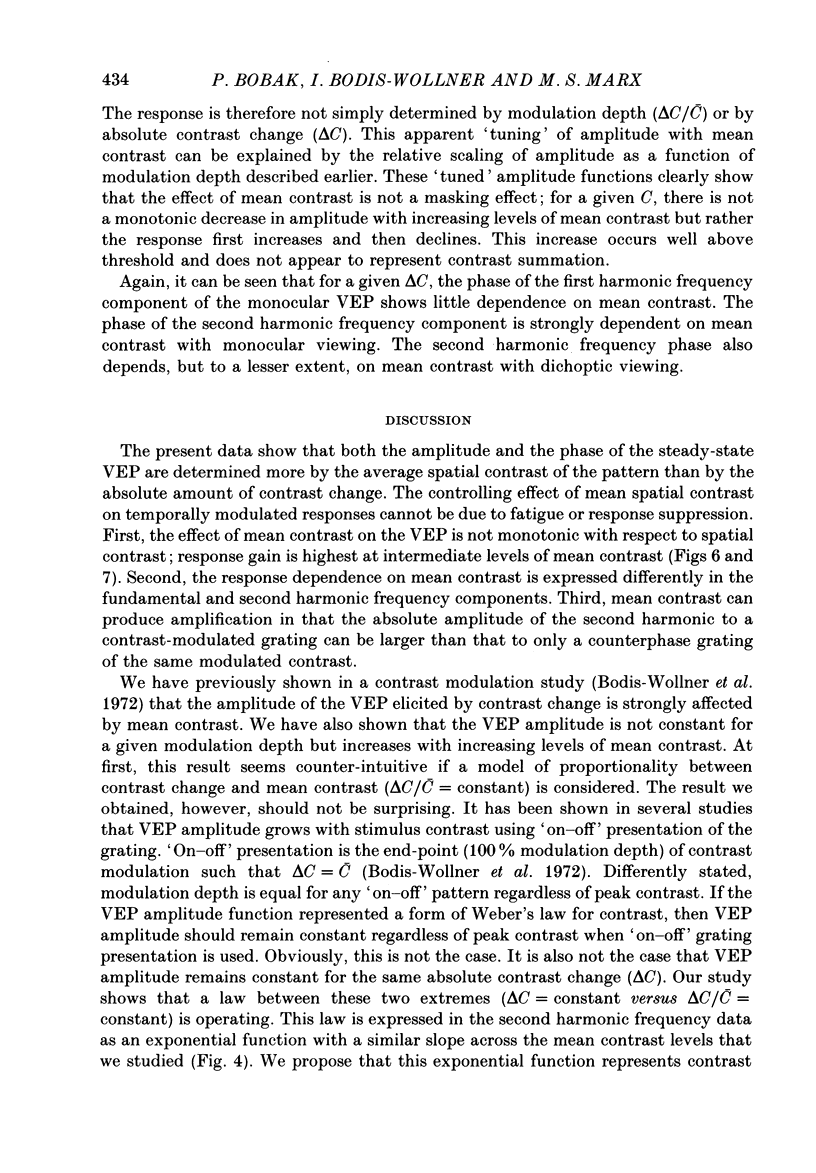
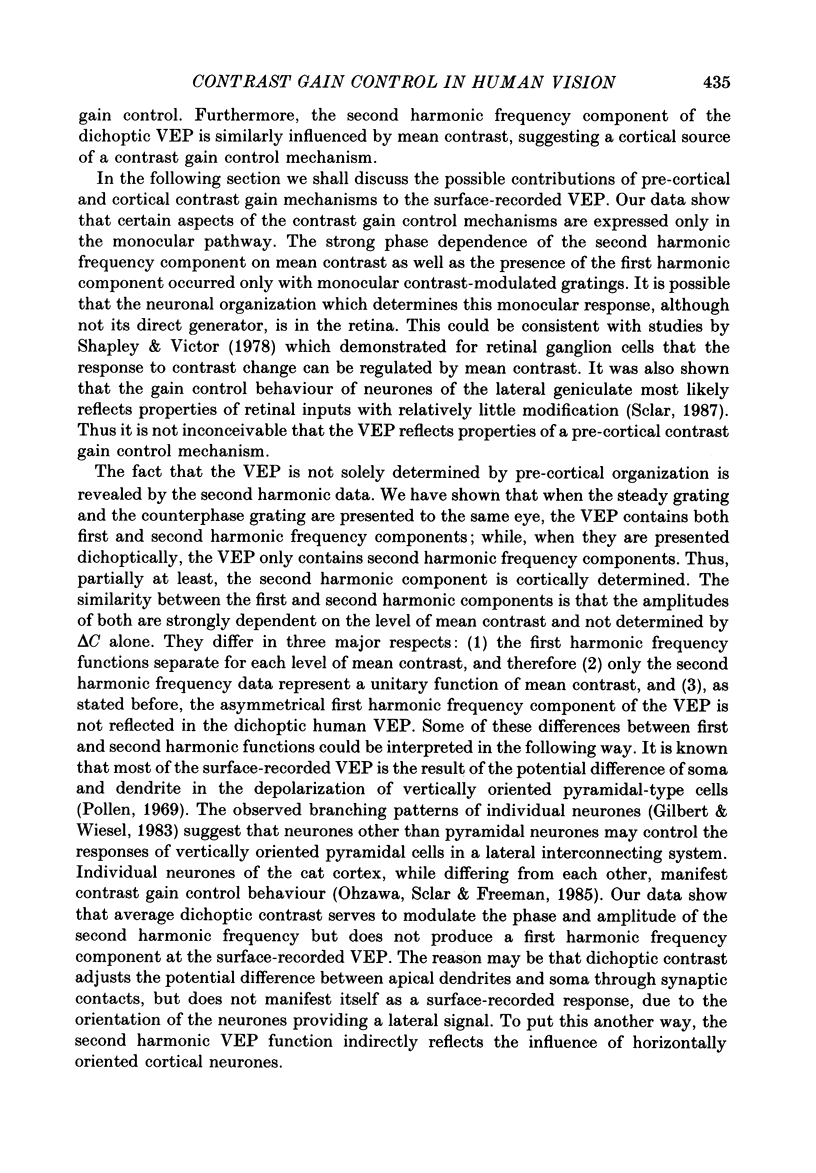
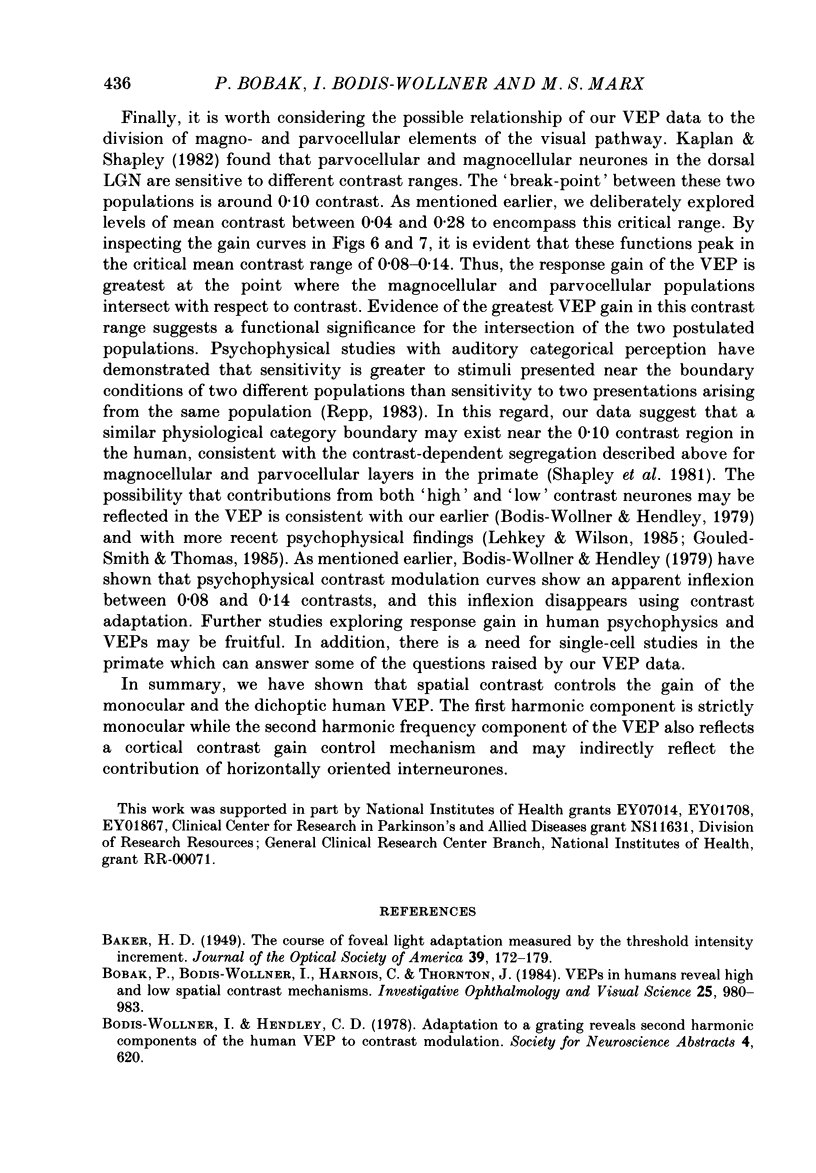
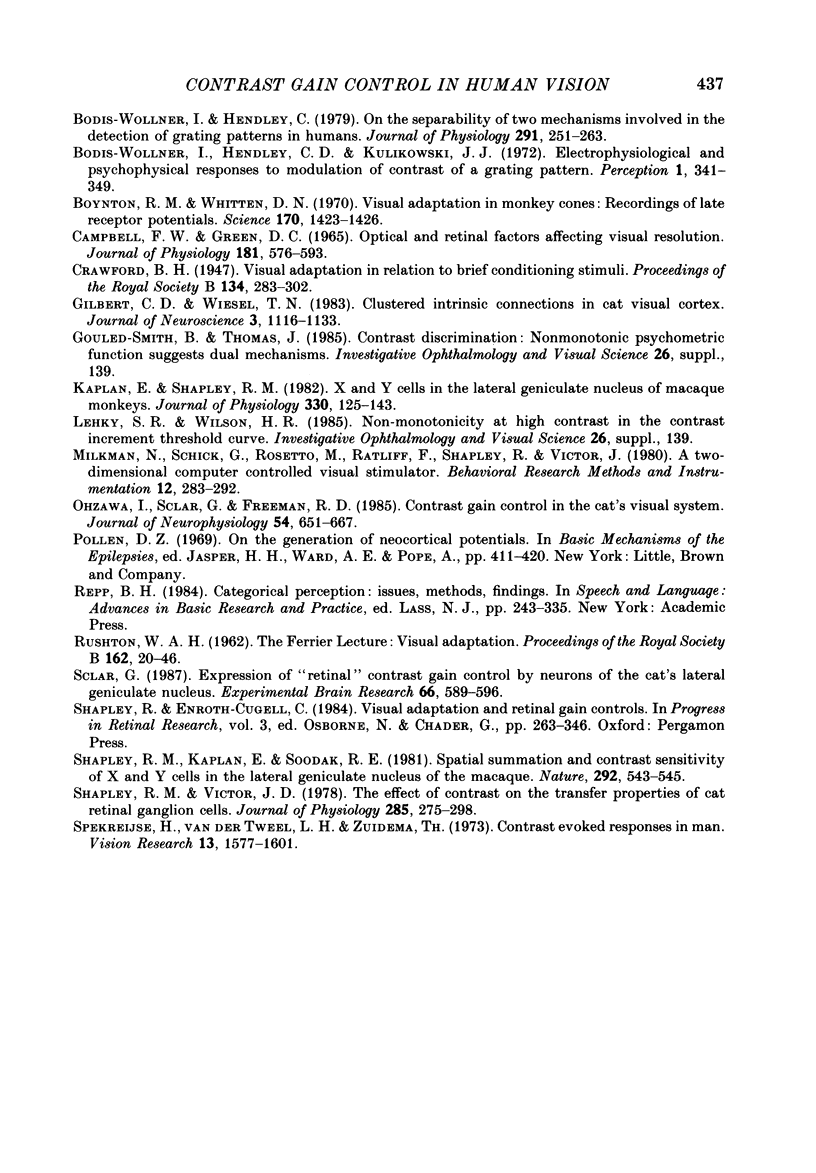
Selected References
These references are in PubMed. This may not be the complete list of references from this article.
- Bobak P., Bodis-Wollner I., Harnois C., Thornton J. VEPs in humans reveal high and low spatial contrast mechanisms. Invest Ophthalmol Vis Sci. 1984 Aug;25(8):980–983. [PubMed] [Google Scholar]
- Bodis-Wollner I., Hendley C. D., Kulikowski J. J. Electrophysiological and psychophysical responses to modulation of contrast of a grating pattern. Perception. 1972;1(3):341–349. doi: 10.1068/p010341. [DOI] [PubMed] [Google Scholar]
- Bodis-Wollner I., Hendley C. D. On the separability of two mechanisms involved in the detection of grating patterns in humans. J Physiol. 1979 Jun;291:251–263. doi: 10.1113/jphysiol.1979.sp012810. [DOI] [PMC free article] [PubMed] [Google Scholar]
- Boynton R. M., Whitten D. N. Visual adaptation in monkey cones: recordings of late receptor potentials. Science. 1970 Dec 25;170(3965):1423–1426. doi: 10.1126/science.170.3965.1423. [DOI] [PubMed] [Google Scholar]
- Campbell F. W., Green D. G. Optical and retinal factors affecting visual resolution. J Physiol. 1965 Dec;181(3):576–593. doi: 10.1113/jphysiol.1965.sp007784. [DOI] [PMC free article] [PubMed] [Google Scholar]
- Gilbert C. D., Wiesel T. N. Clustered intrinsic connections in cat visual cortex. J Neurosci. 1983 May;3(5):1116–1133. doi: 10.1523/JNEUROSCI.03-05-01116.1983. [DOI] [PMC free article] [PubMed] [Google Scholar]
- Kaplan E., Shapley R. M. X and Y cells in the lateral geniculate nucleus of macaque monkeys. J Physiol. 1982 Sep;330:125–143. doi: 10.1113/jphysiol.1982.sp014333. [DOI] [PMC free article] [PubMed] [Google Scholar]
- Ohzawa I., Sclar G., Freeman R. D. Contrast gain control in the cat's visual system. J Neurophysiol. 1985 Sep;54(3):651–667. doi: 10.1152/jn.1985.54.3.651. [DOI] [PubMed] [Google Scholar]
- RUSHTON W. A. VISUAL ADAPTATION. Proc R Soc Lond B Biol Sci. 1965 Mar 16;162:20–46. doi: 10.1098/rspb.1965.0024. [DOI] [PubMed] [Google Scholar]
- Sclar G. Expression of "retinal" contrast gain control by neurons of the cat's lateral geniculate nucleus. Exp Brain Res. 1987;66(3):589–596. doi: 10.1007/BF00270692. [DOI] [PubMed] [Google Scholar]
- Shapley R. M., Victor J. D. The effect of contrast on the transfer properties of cat retinal ganglion cells. J Physiol. 1978 Dec;285:275–298. doi: 10.1113/jphysiol.1978.sp012571. [DOI] [PMC free article] [PubMed] [Google Scholar]
- Shapley R., Kaplan E., Soodak R. Spatial summation and contrast sensitivity of X and Y cells in the lateral geniculate nucleus of the macaque. Nature. 1981 Aug 6;292(5823):543–545. doi: 10.1038/292543a0. [DOI] [PubMed] [Google Scholar]
- Spekreijse H., van der Twell L. H., Zuidema T. Contrast evoked responses in man. Vision Res. 1973 Aug;13(8):1577–1601. doi: 10.1016/0042-6989(73)90016-3. [DOI] [PubMed] [Google Scholar]