Abstract
1. Calcium and sodium currents and non-linear capacitive currents were recorded from isolated ventricular cells from neonatal rats, using the whole-cell patch-clamp technique, usually with a holding potential of -100 mV. 2. When recording with internal and external solutions designed to suppress virtually all ionic currents except the calcium current, careful subtraction of all linear capacitive and ionic currents revealed that depolarizations elicited a small transient outward current which preceded the inward calcium current. This outward current was discernible just below the threshold potential for the calcium current and increased with larger depolarizations to a maximum for potentials of about +30 mV and above. 3. Elimination of the calcium current revealed that at each potential the transient outward current was accompanied by a roughly equal transient inward current upon repolarization. The properties of these currents indicate that they are non-linear capacitive currents. Best-fit Boltzmann curves of the 'on' charge (integral of the transient outward current) gave values for qmax, V and k of 3.9 nC/microF, -29.3 mV and 15.5 mV with internal Cs+. The maximum 'on' charge is similar to that found with calcium currents (4.3 nC/microF). Similar values were obtained with internal TEA+. 4. Boltzmann fits of conductance vs. voltage for the calcium channel gave mean values of -15.5 and 13.3 mV for V and k (with internal Cs+); the corresponding values for the sodium channel were -49.9 and 5.4 mV. 5. Pre-pulses (20 ms) to -60 mV inactivated 77% of the peak sodium current, but only inactivated about 10% of the peak calcium current and reduced the maximum 'on' charge (moved at potentials positive to -60 mV) by 19%. 6. With a holding potential of -100 mV, 10 microM-nifedipine blocked 89% of the calcium current, but had little effect on the amount of 'on' charge. The 'off' charge appeared to be slower in the presence of nifedipine. 7. These results and consideration of the number of calcium channels and high-affinity binding sites for dihydropyridines (DHP), suggest that a large part of the charge movement may be related to DHP binding sites and involved with gating calcium channels. Comparison with skeletal muscle suggests similarities in the mechanisms involved in excitation-contraction coupling.
Full text
PDF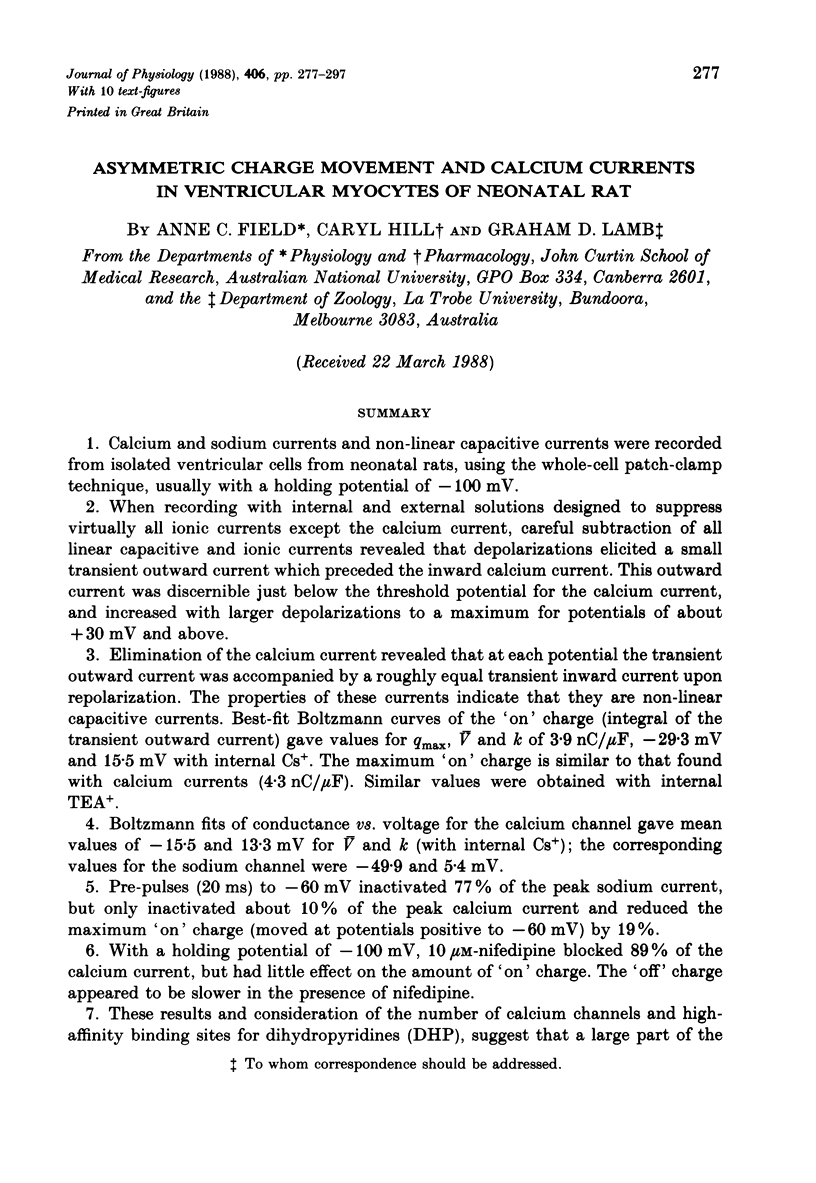
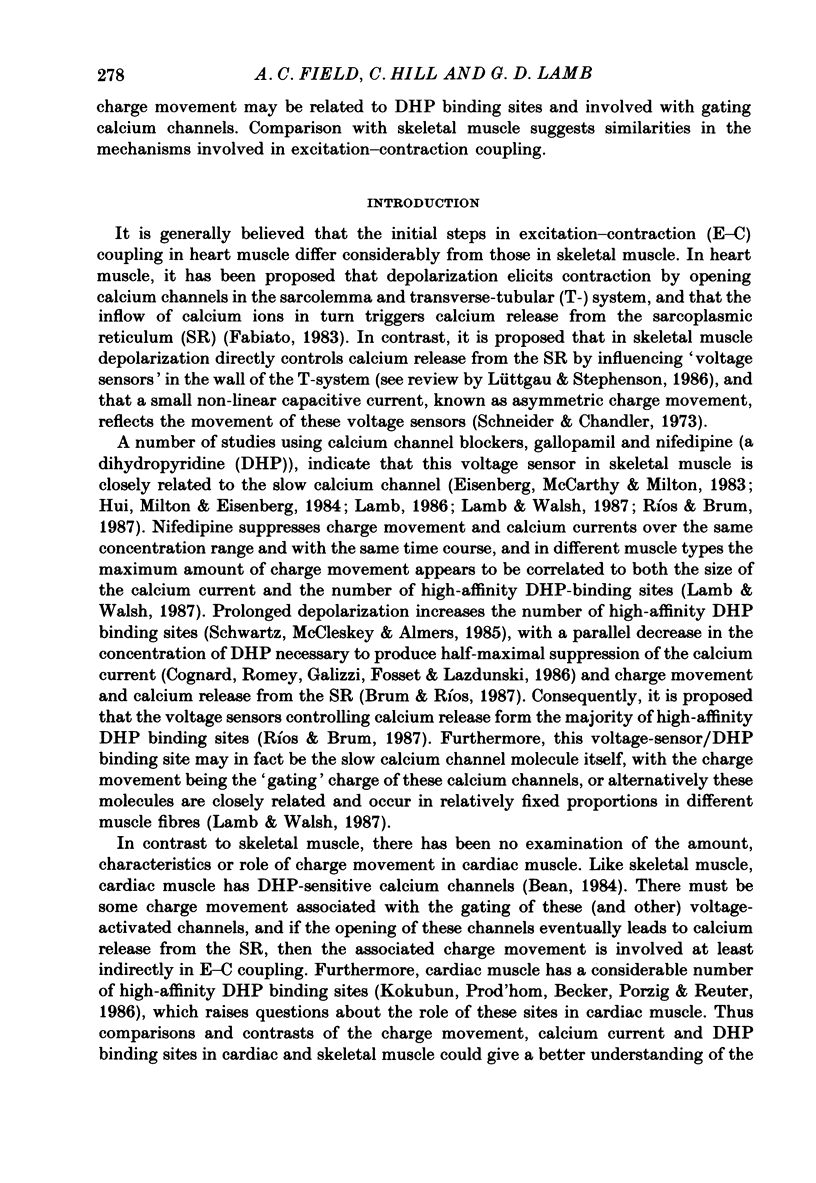
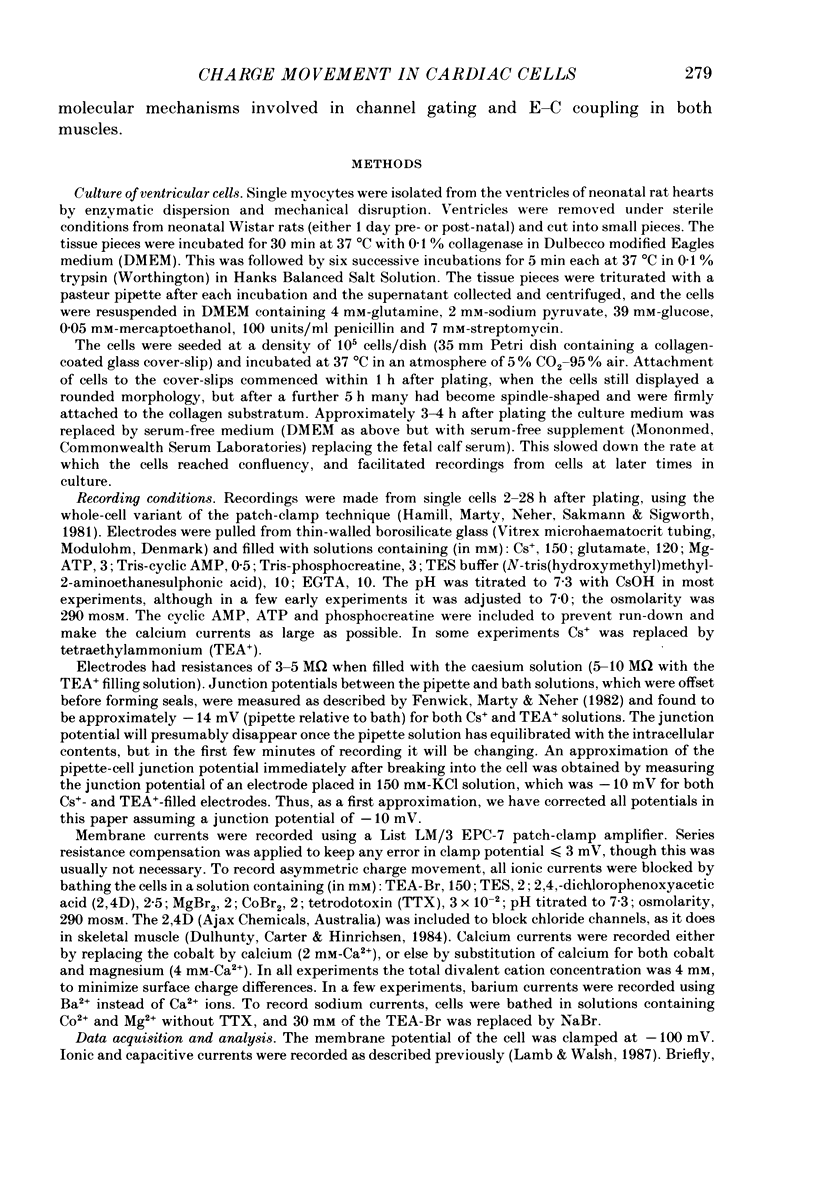
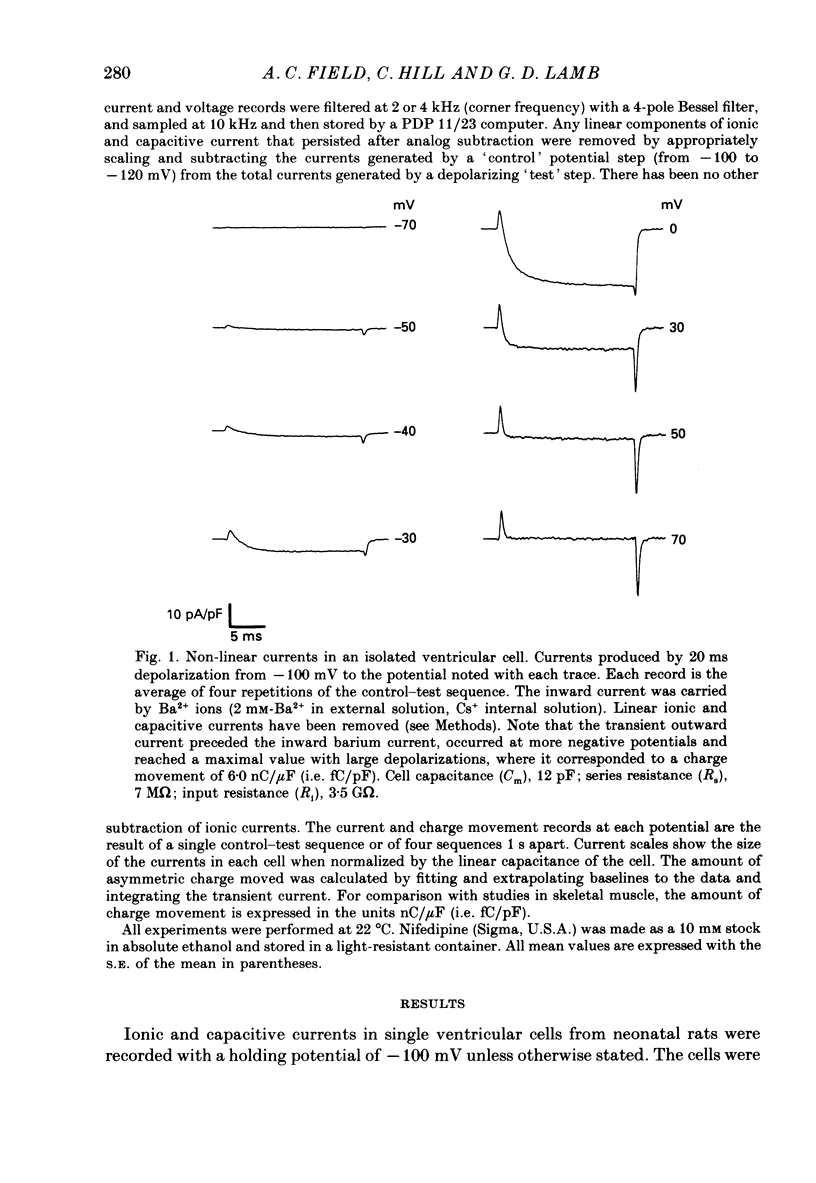
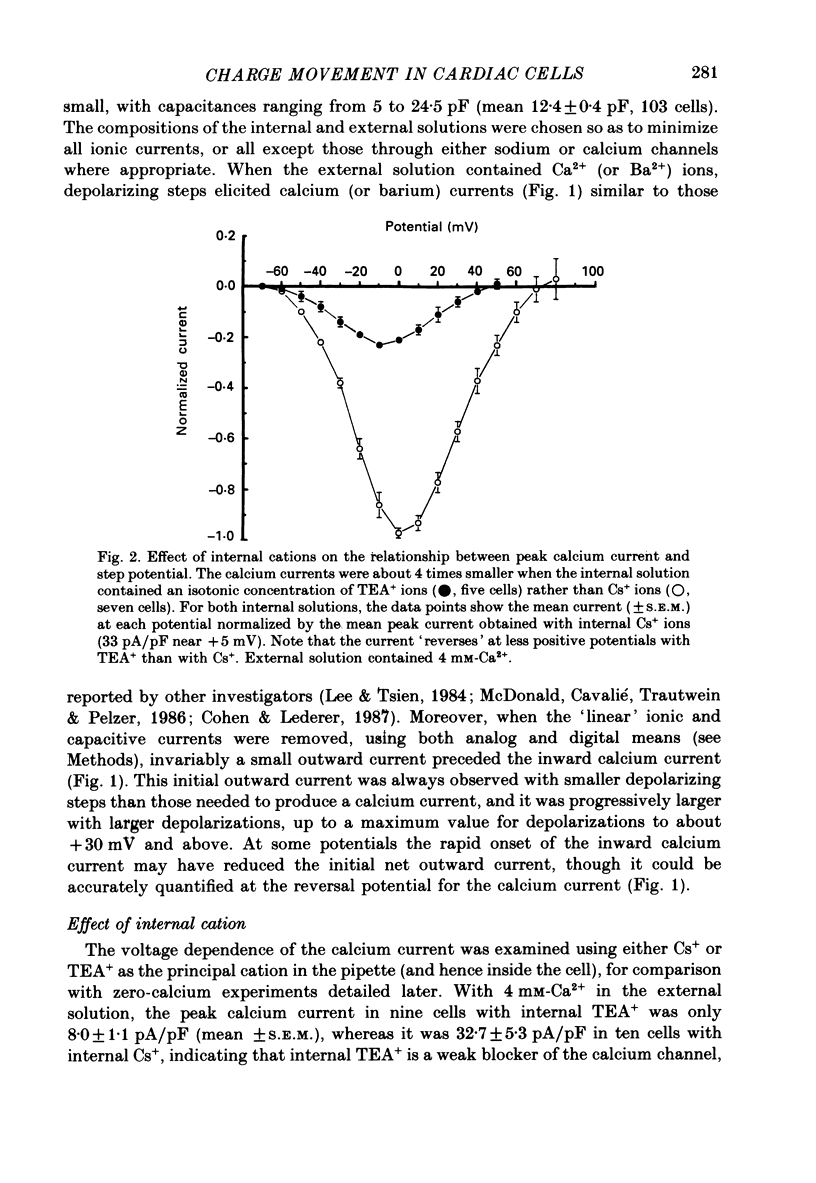
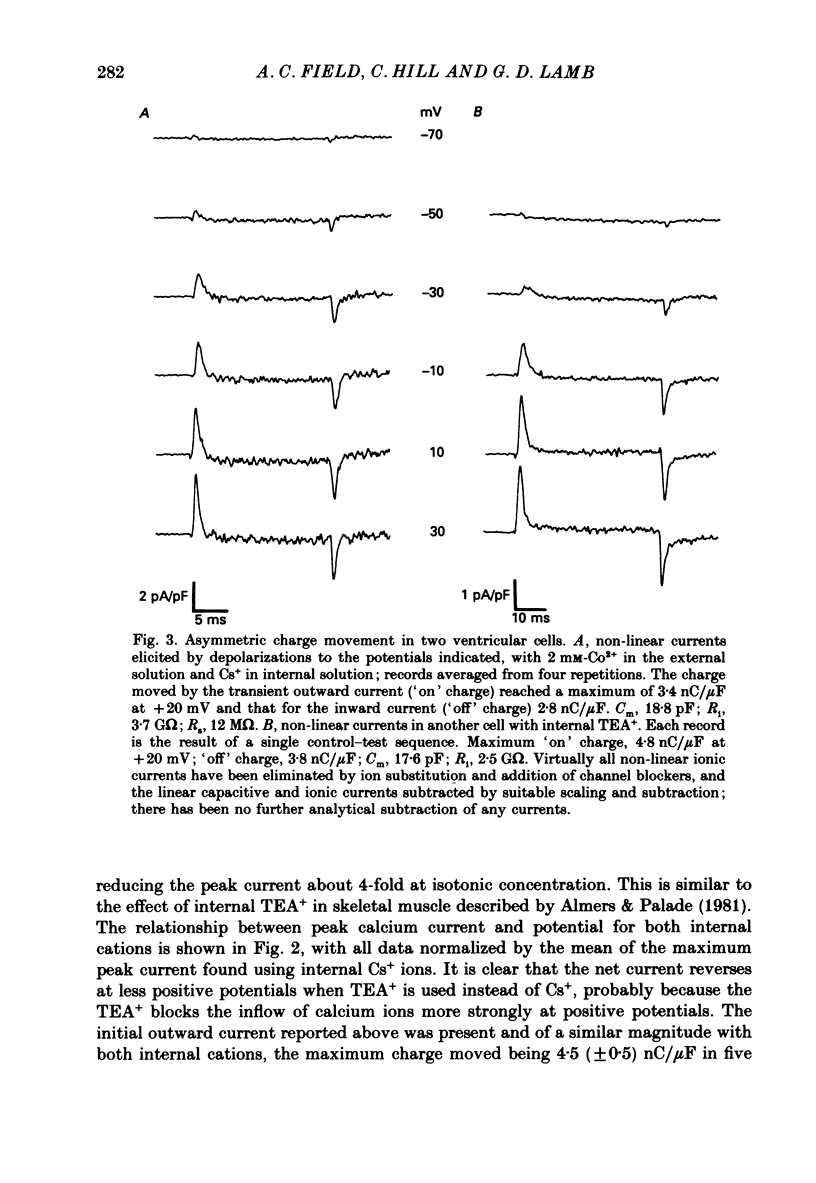
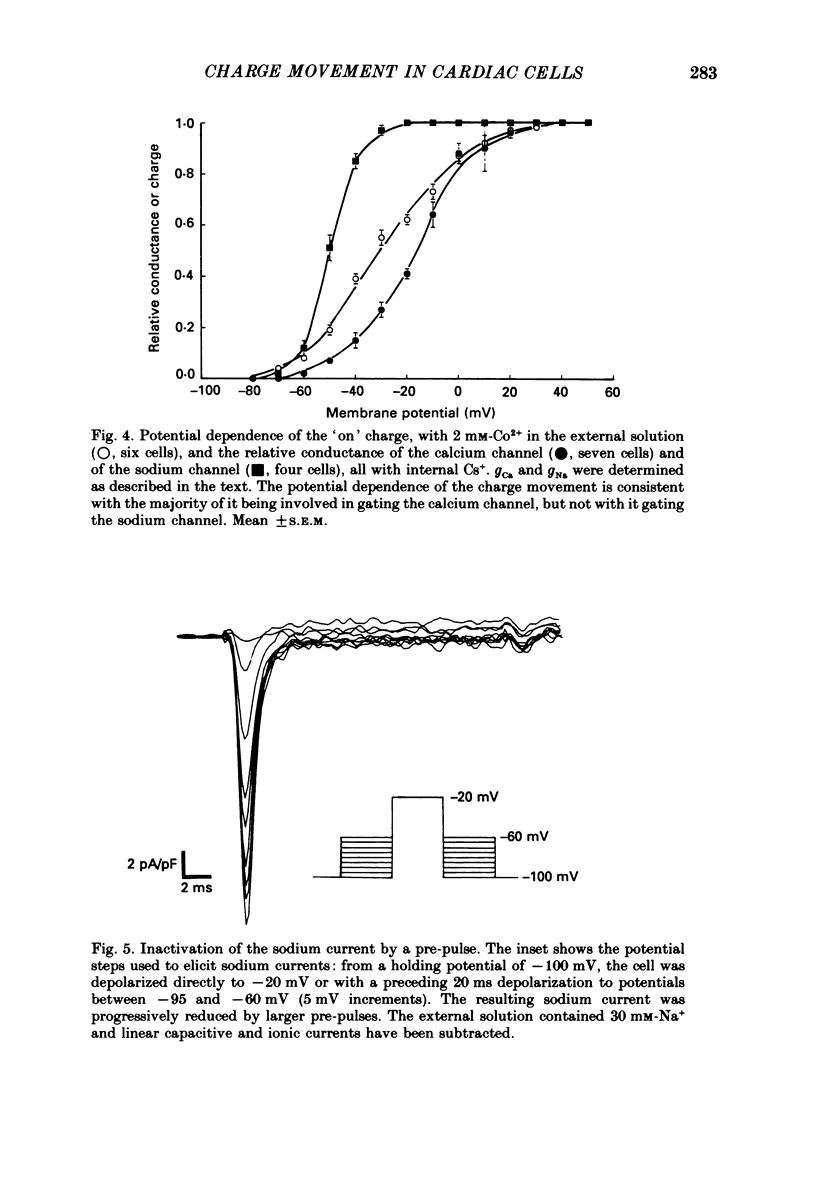
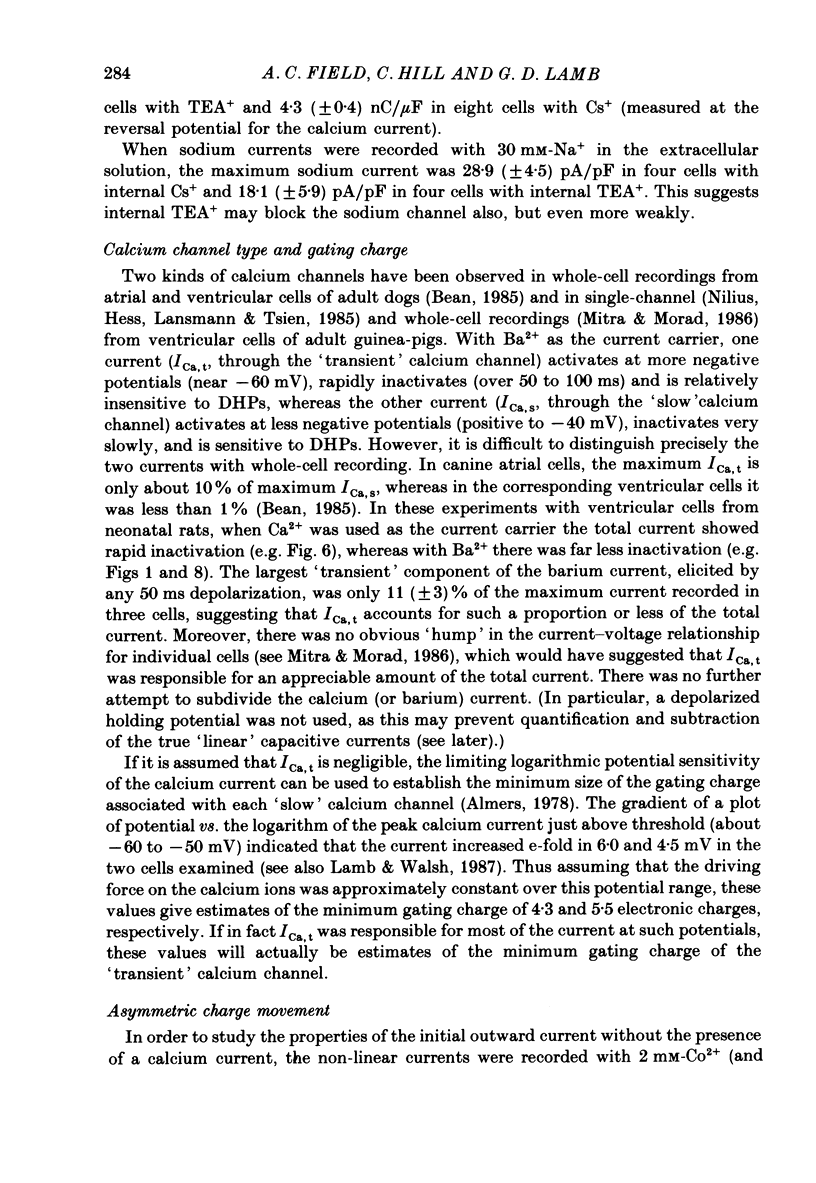
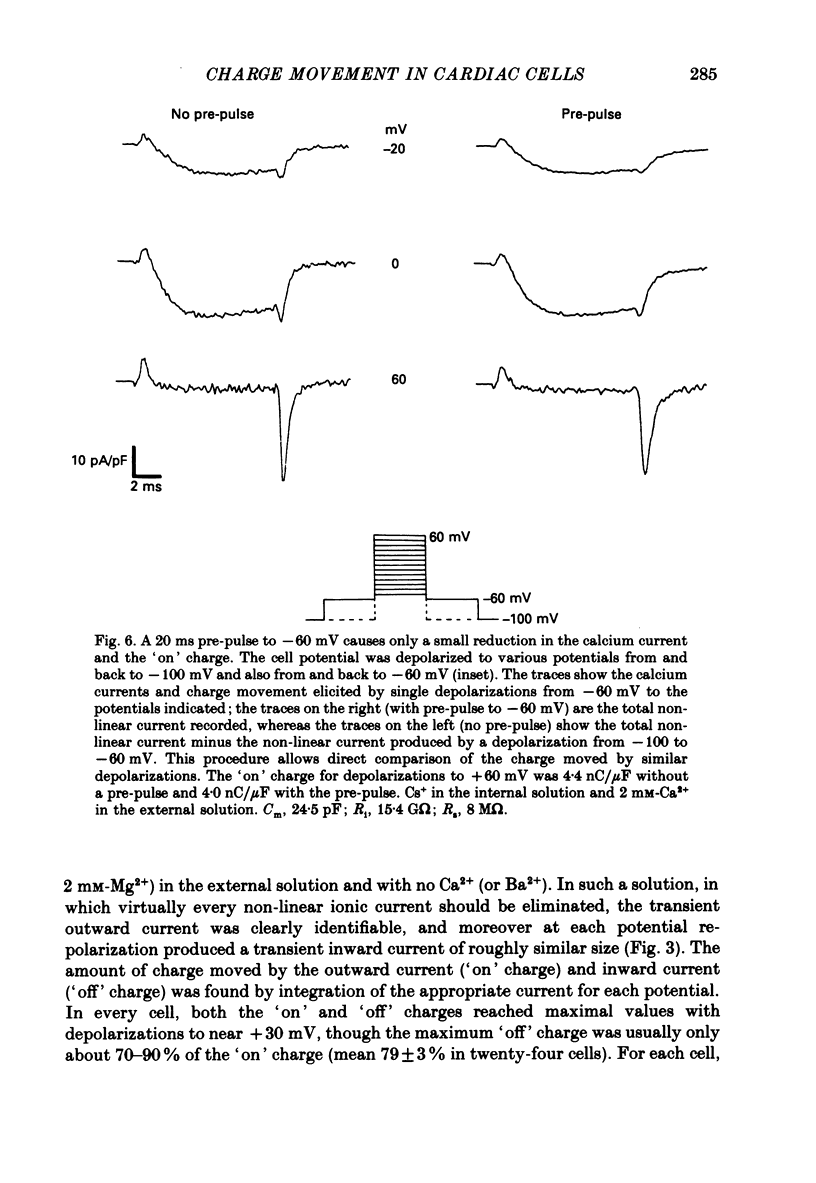
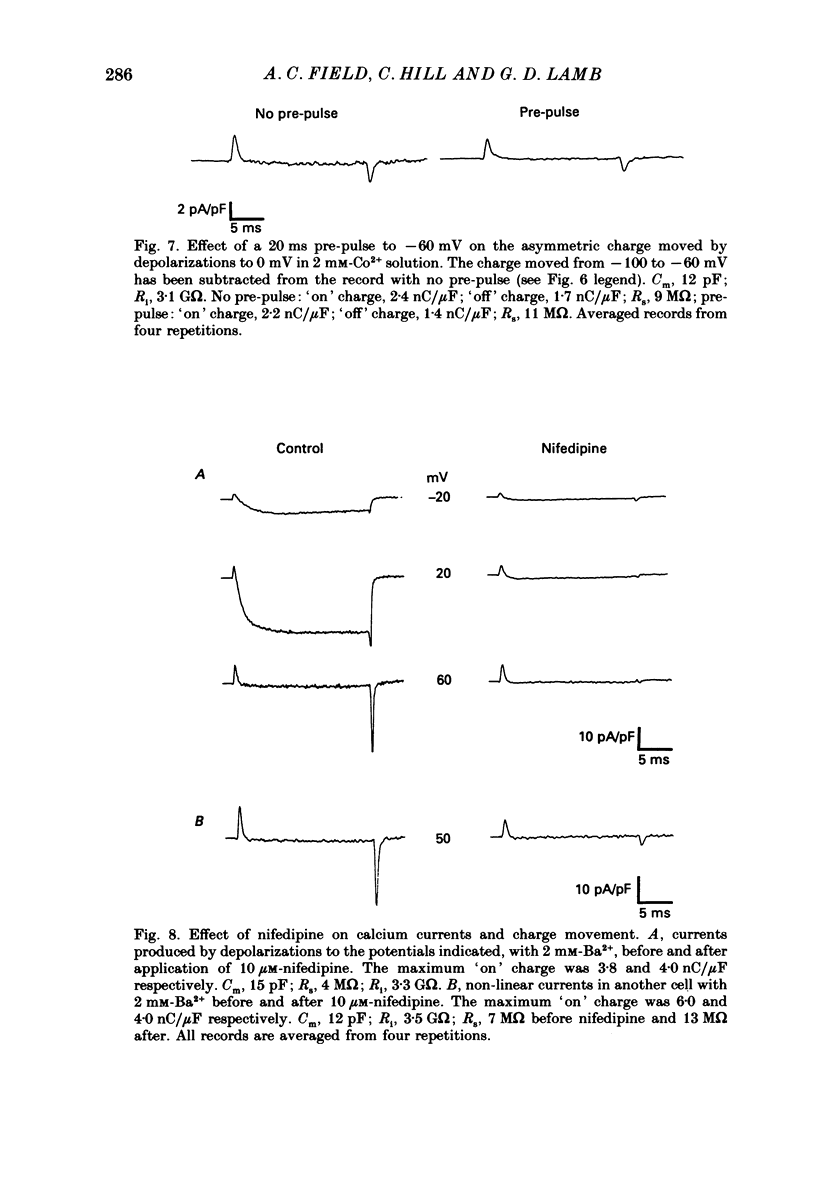
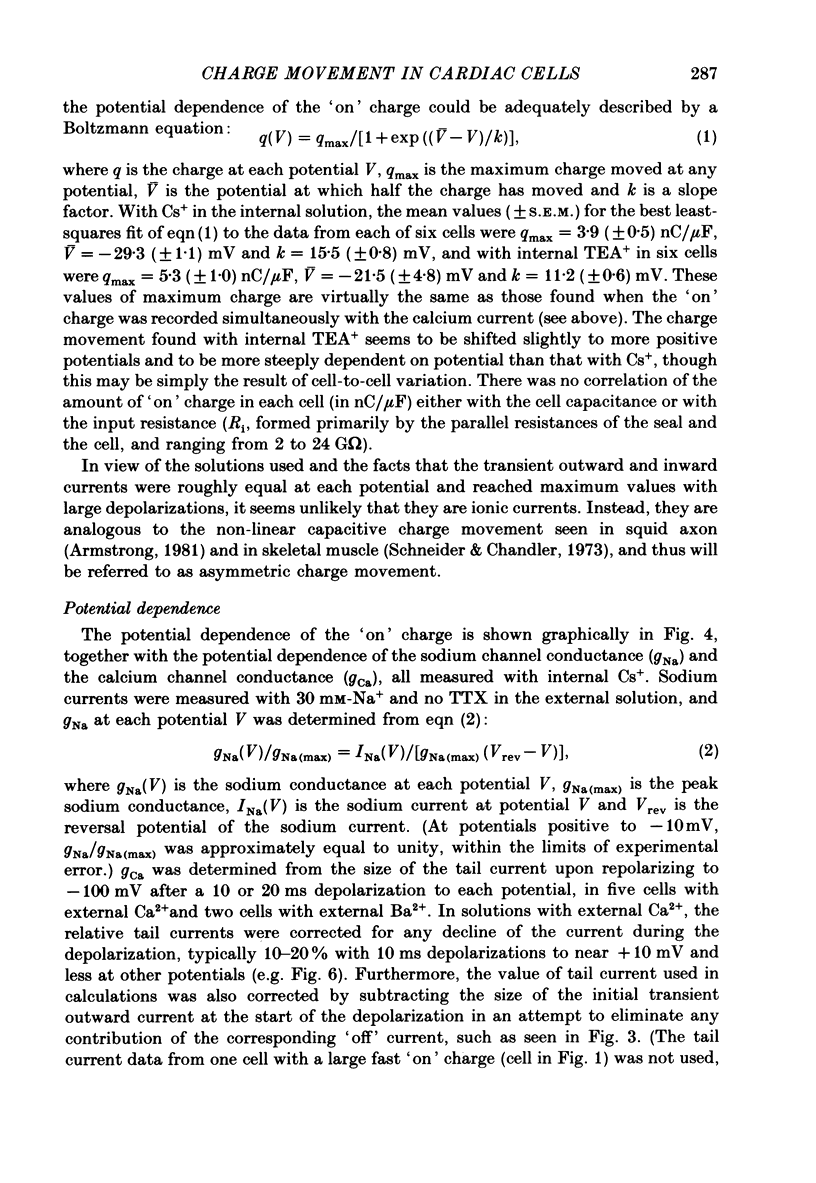
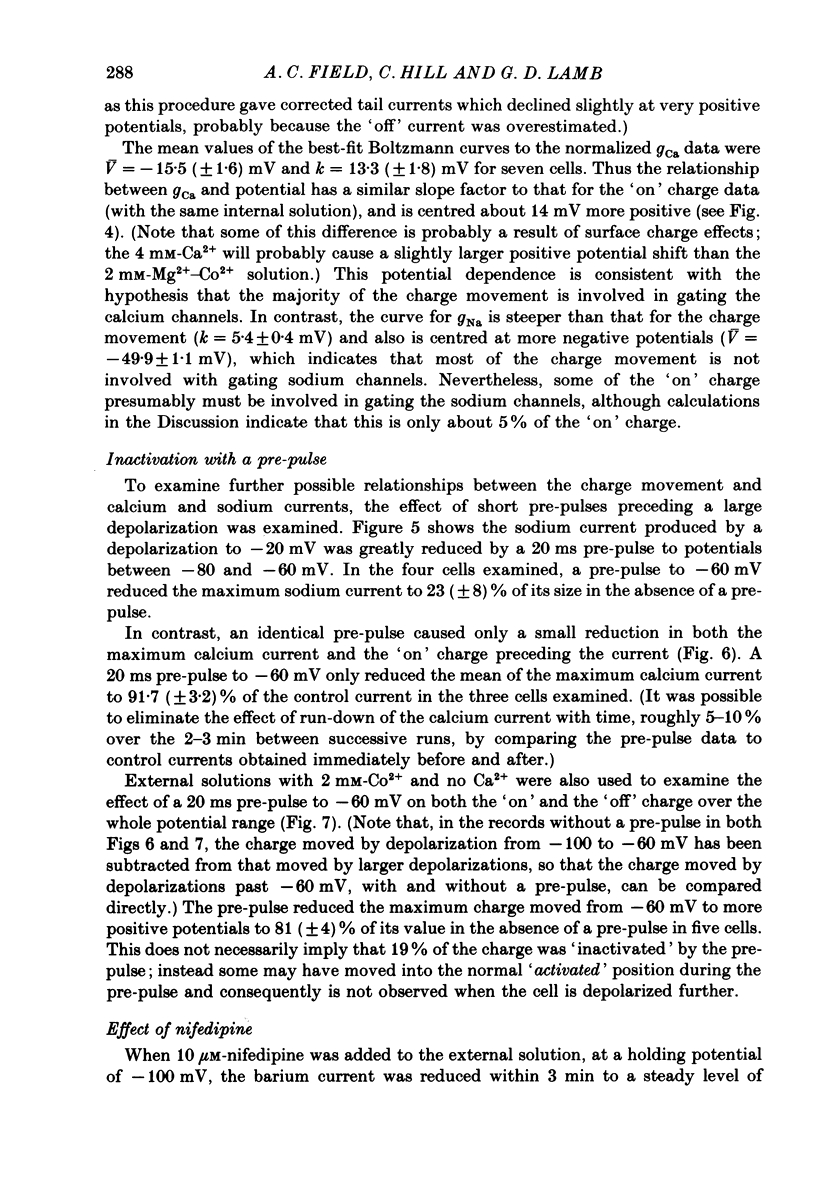
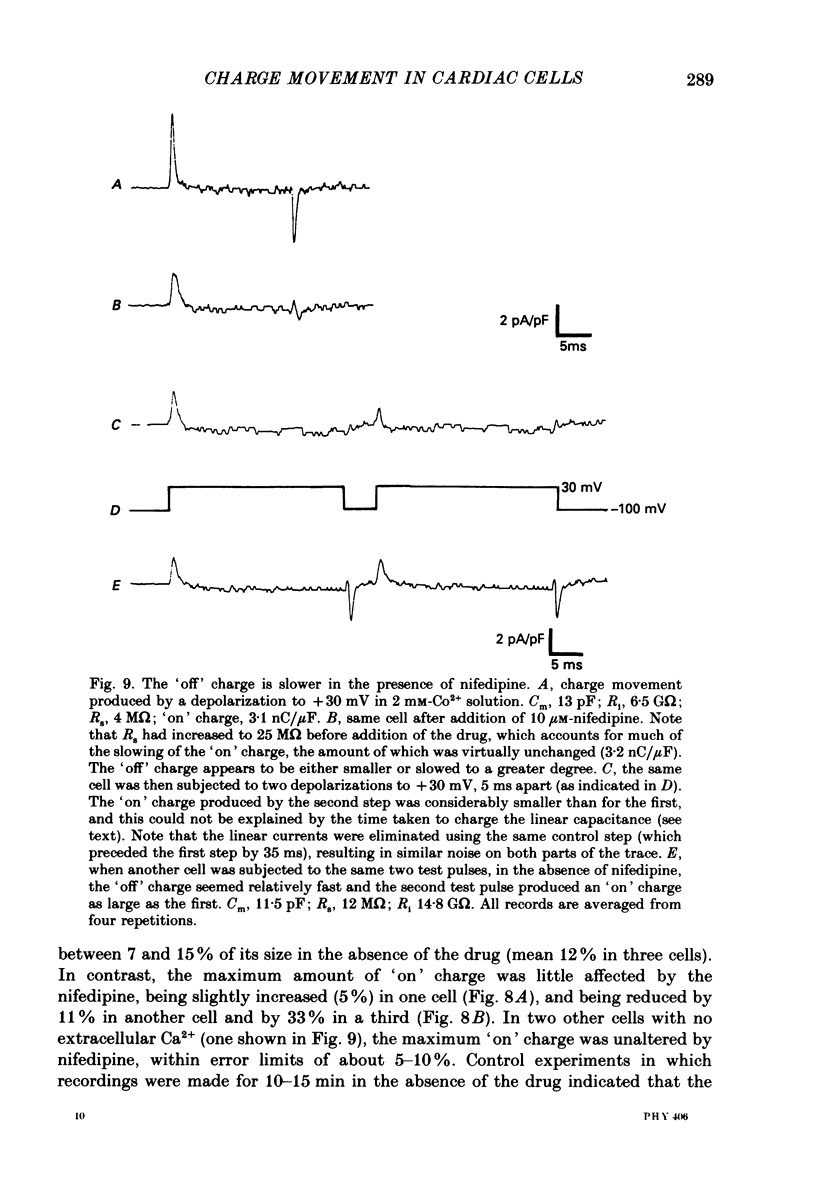
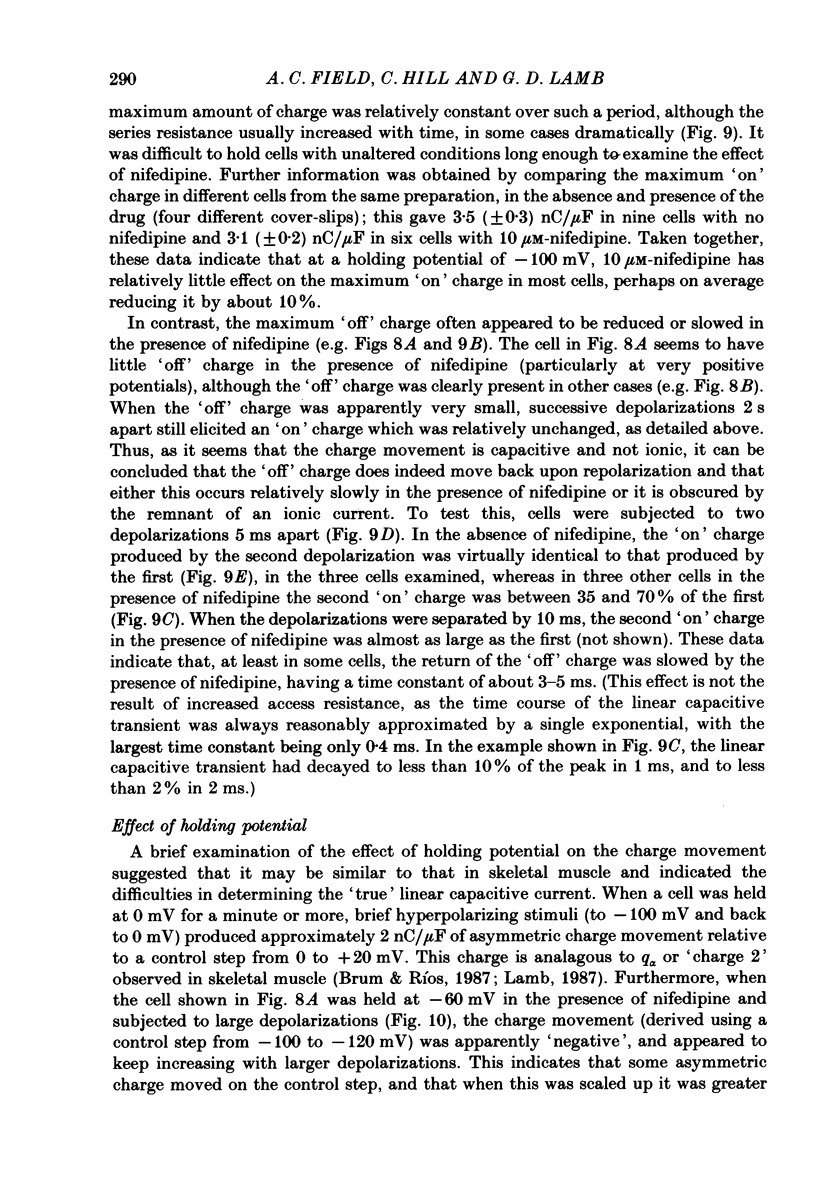
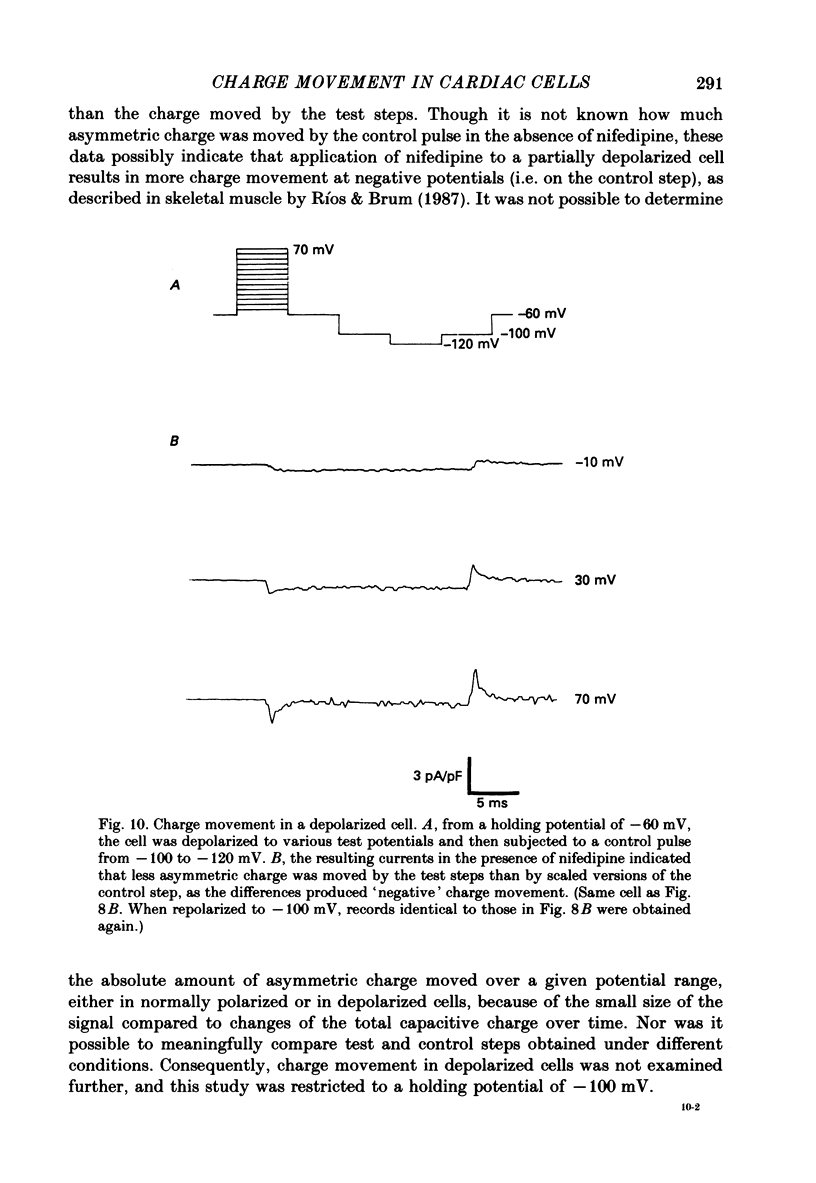
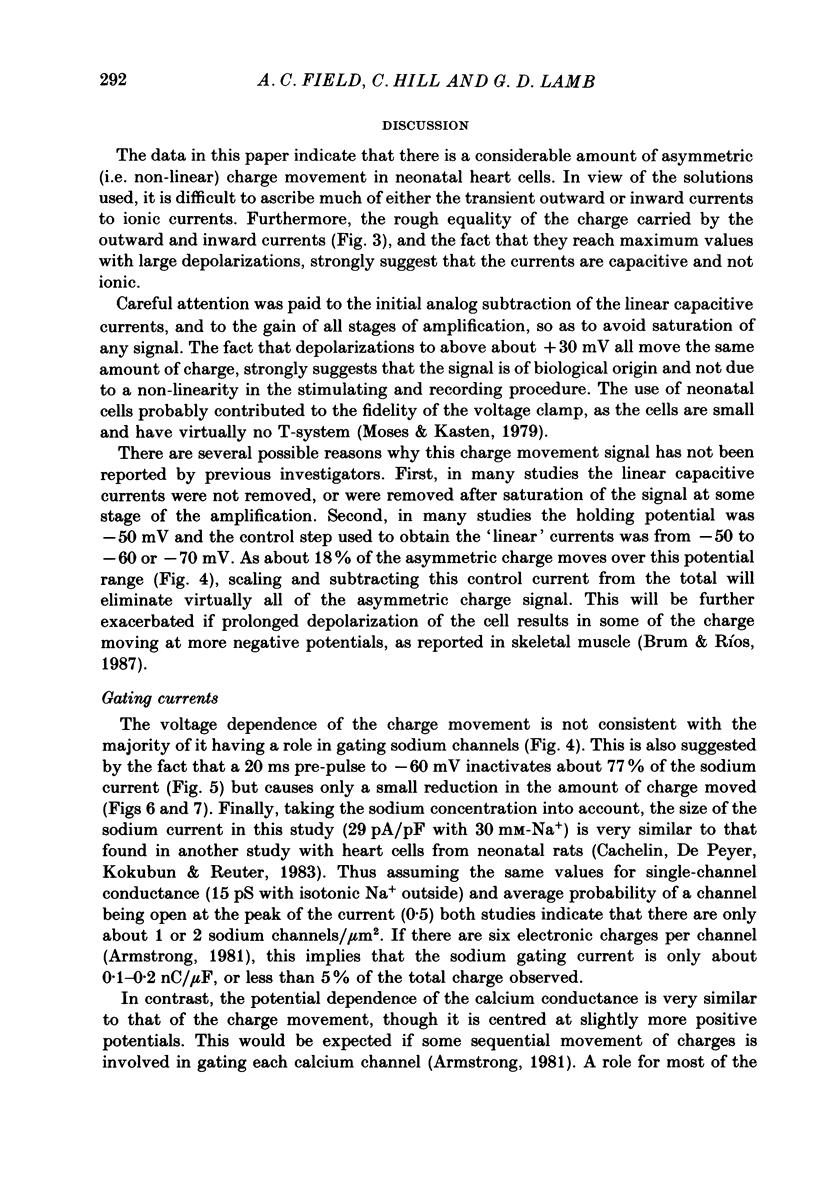
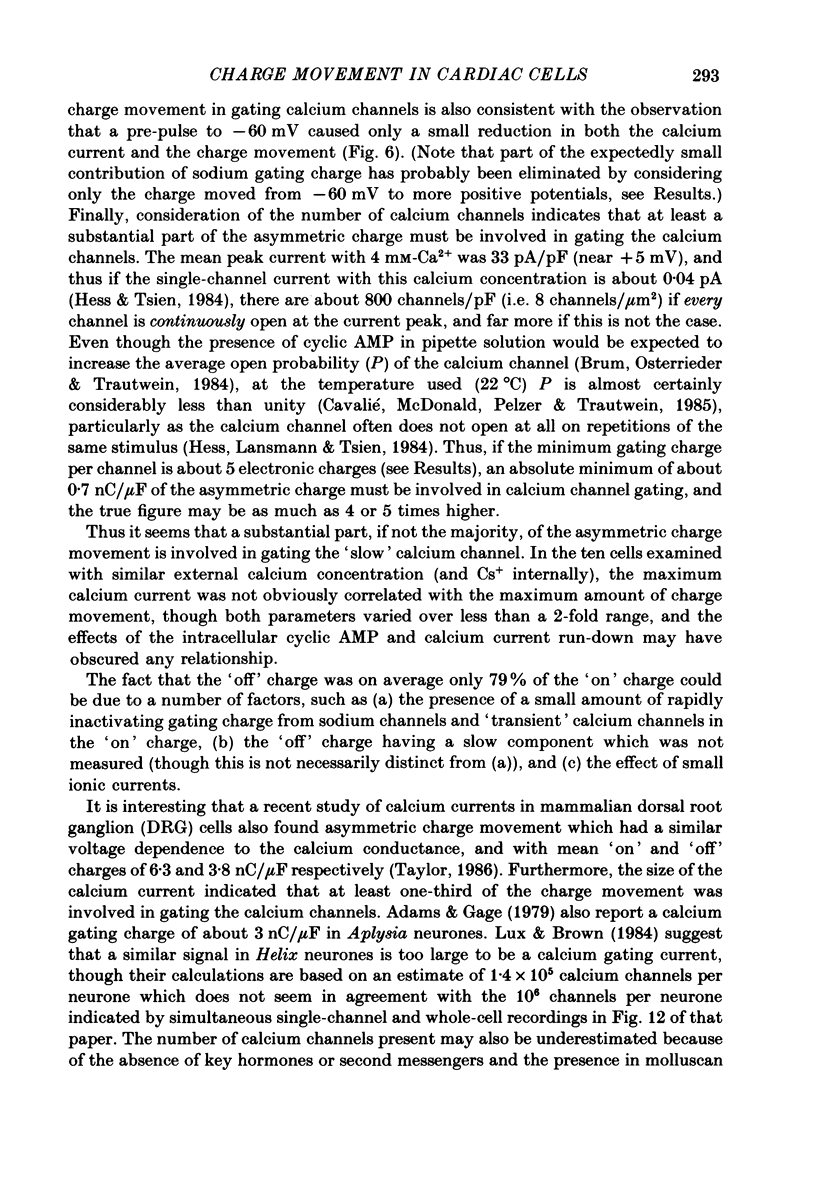
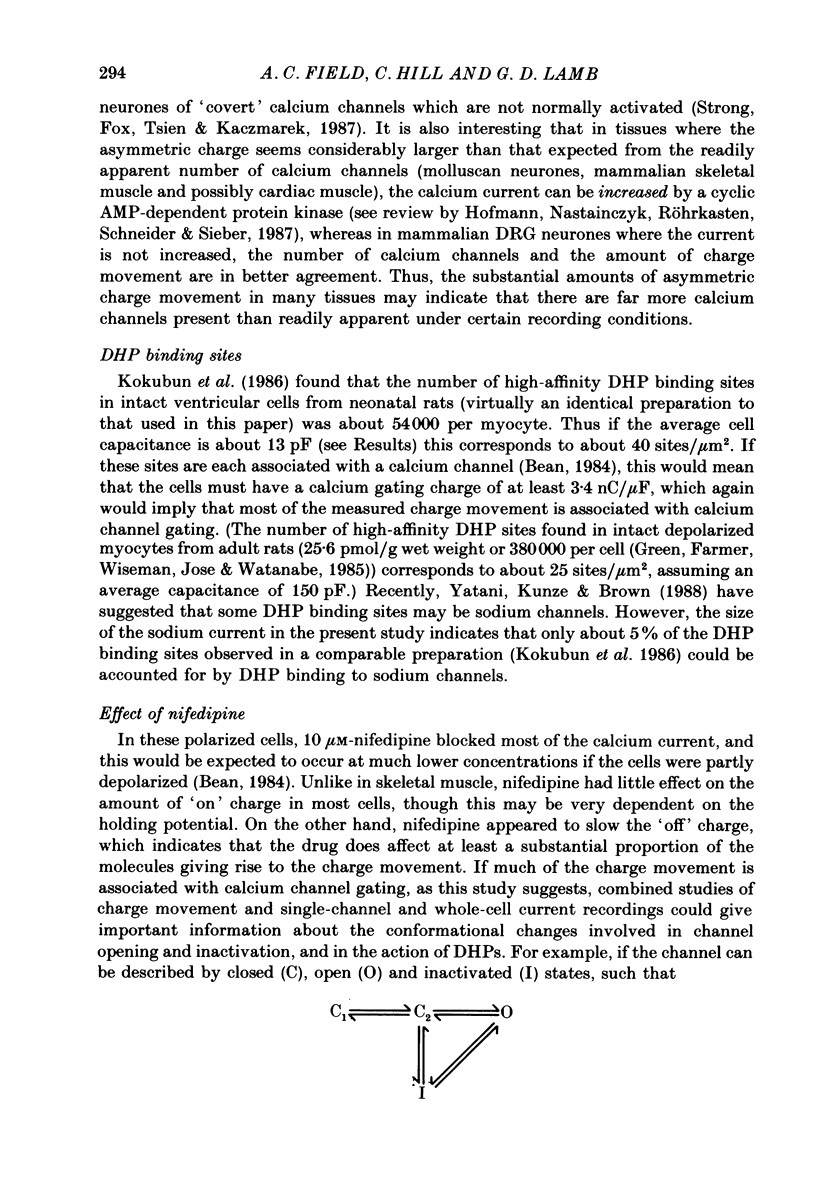
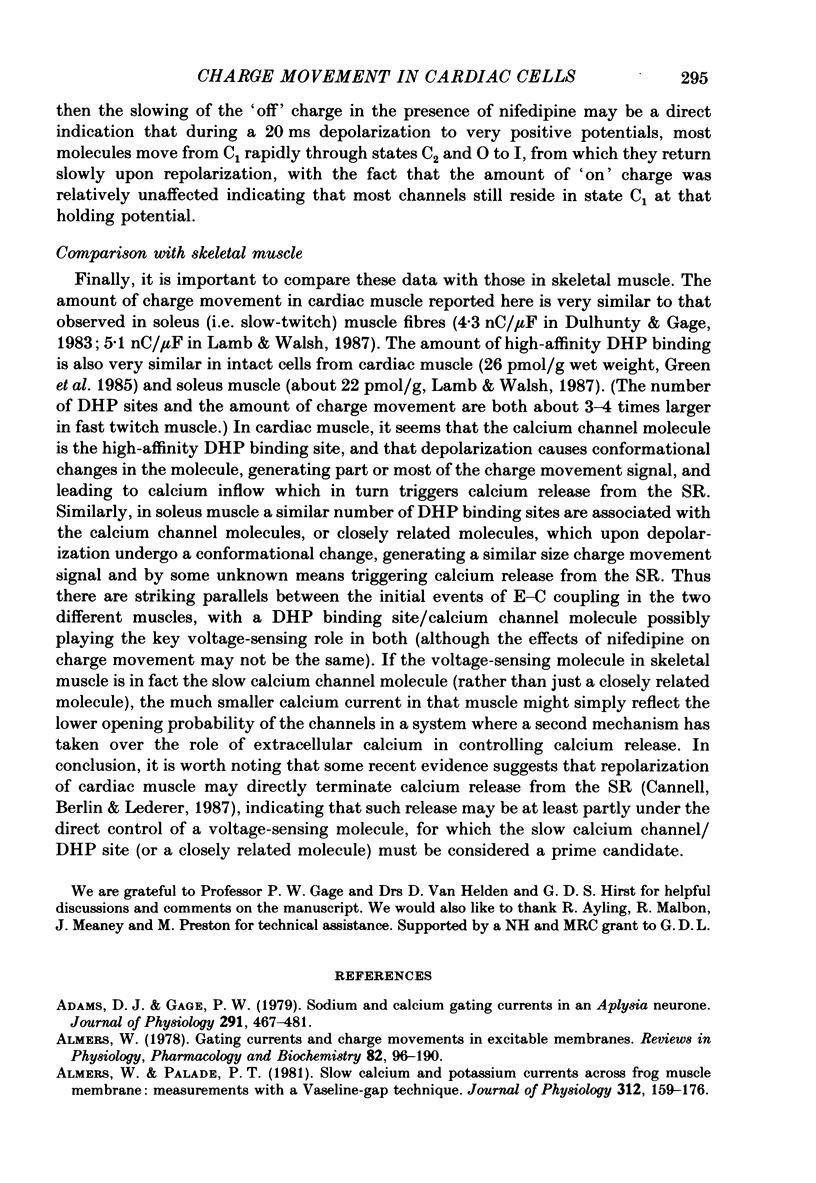
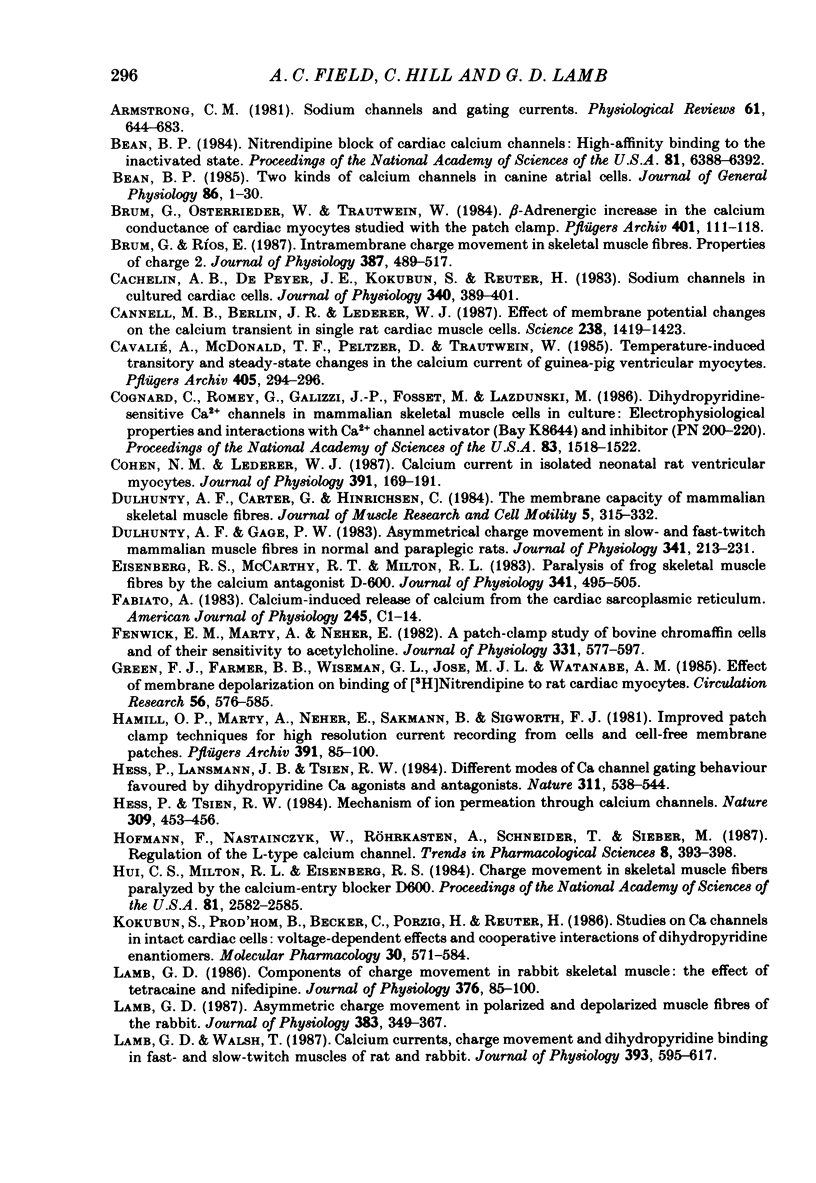
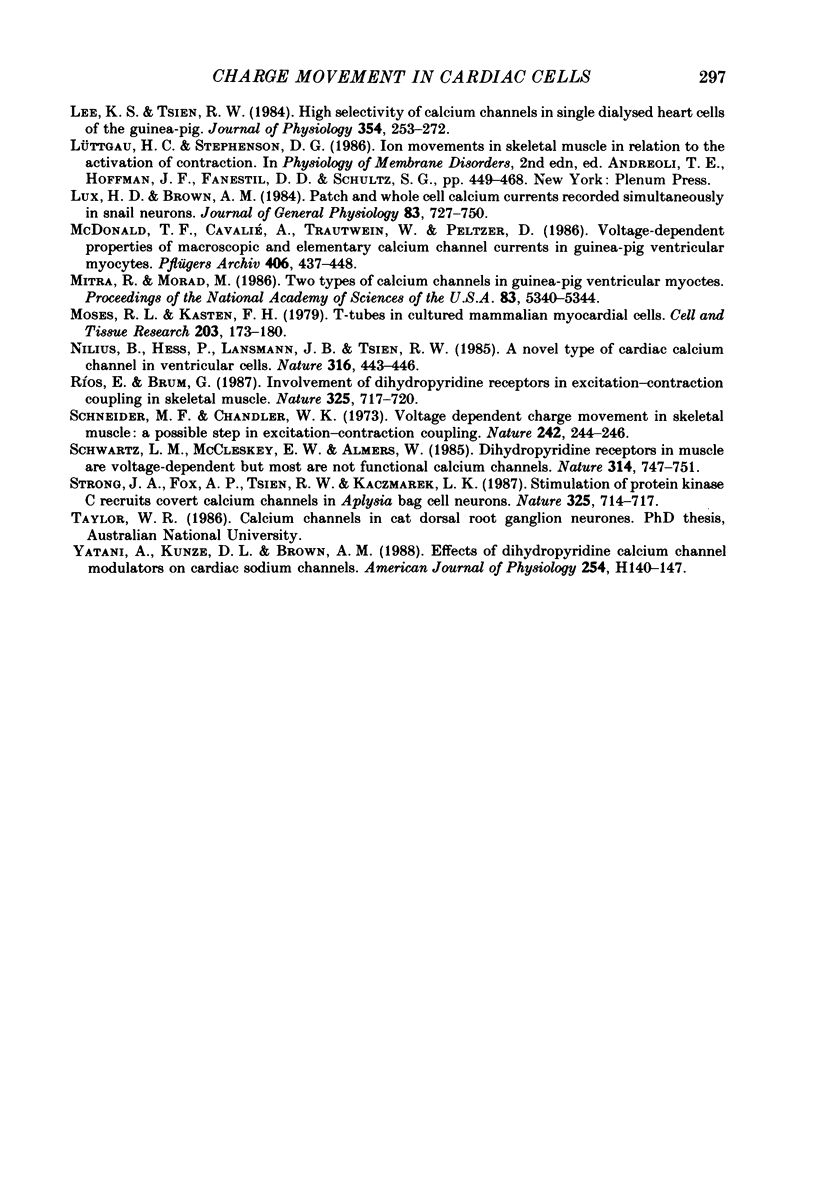
Selected References
These references are in PubMed. This may not be the complete list of references from this article.
- Adams D. J., Gage P. W. Sodium and calcium gating currents in an Aplysia neurone. J Physiol. 1979 Jun;291:467–481. doi: 10.1113/jphysiol.1979.sp012826. [DOI] [PMC free article] [PubMed] [Google Scholar]
- Almers W. Gating currents and charge movements in excitable membranes. Rev Physiol Biochem Pharmacol. 1978;82:96–190. doi: 10.1007/BFb0030498. [DOI] [PubMed] [Google Scholar]
- Almers W., Palade P. T. Slow calcium and potassium currents across frog muscle membrane: measurements with a vaseline-gap technique. J Physiol. 1981 Mar;312:159–176. doi: 10.1113/jphysiol.1981.sp013622. [DOI] [PMC free article] [PubMed] [Google Scholar]
- Armstrong C. M. Sodium channels and gating currents. Physiol Rev. 1981 Jul;61(3):644–683. doi: 10.1152/physrev.1981.61.3.644. [DOI] [PubMed] [Google Scholar]
- Bean B. P. Nitrendipine block of cardiac calcium channels: high-affinity binding to the inactivated state. Proc Natl Acad Sci U S A. 1984 Oct;81(20):6388–6392. doi: 10.1073/pnas.81.20.6388. [DOI] [PMC free article] [PubMed] [Google Scholar]
- Bean B. P. Two kinds of calcium channels in canine atrial cells. Differences in kinetics, selectivity, and pharmacology. J Gen Physiol. 1985 Jul;86(1):1–30. doi: 10.1085/jgp.86.1.1. [DOI] [PMC free article] [PubMed] [Google Scholar]
- Brum G., Osterrieder W., Trautwein W. Beta-adrenergic increase in the calcium conductance of cardiac myocytes studied with the patch clamp. Pflugers Arch. 1984 Jun;401(2):111–118. doi: 10.1007/BF00583870. [DOI] [PubMed] [Google Scholar]
- Brum G., Rios E. Intramembrane charge movement in frog skeletal muscle fibres. Properties of charge 2. J Physiol. 1987 Jun;387:489–517. doi: 10.1113/jphysiol.1987.sp016586. [DOI] [PMC free article] [PubMed] [Google Scholar]
- Cachelin A. B., De Peyer J. E., Kokubun S., Reuter H. Sodium channels in cultured cardiac cells. J Physiol. 1983 Jul;340:389–401. doi: 10.1113/jphysiol.1983.sp014768. [DOI] [PMC free article] [PubMed] [Google Scholar]
- Cannell M. B., Berlin J. R., Lederer W. J. Effect of membrane potential changes on the calcium transient in single rat cardiac muscle cells. Science. 1987 Dec 4;238(4832):1419–1423. doi: 10.1126/science.2446391. [DOI] [PubMed] [Google Scholar]
- Cavalié A., McDonald T. F., Pelzer D., Trautwein W. Temperature-induced transitory and steady-state changes in the calcium current of guinea pig ventricular myocytes. Pflugers Arch. 1985 Oct;405(3):294–296. doi: 10.1007/BF00582574. [DOI] [PubMed] [Google Scholar]
- Cognard C., Romey G., Galizzi J. P., Fosset M., Lazdunski M. Dihydropyridine-sensitive Ca2+ channels in mammalian skeletal muscle cells in culture: electrophysiological properties and interactions with Ca2+ channel activator (Bay K8644) and inhibitor (PN 200-110). Proc Natl Acad Sci U S A. 1986 Mar;83(5):1518–1522. doi: 10.1073/pnas.83.5.1518. [DOI] [PMC free article] [PubMed] [Google Scholar]
- Cohen N. M., Lederer W. J. Calcium current in isolated neonatal rat ventricular myocytes. J Physiol. 1987 Oct;391:169–191. doi: 10.1113/jphysiol.1987.sp016732. [DOI] [PMC free article] [PubMed] [Google Scholar]
- Dulhunty A. F., Gage P. W. Asymmetrical charge movement in slow- and fast-twitch mammalian muscle fibres in normal and paraplegic rats. J Physiol. 1983 Aug;341:213–231. doi: 10.1113/jphysiol.1983.sp014802. [DOI] [PMC free article] [PubMed] [Google Scholar]
- Dulhunty A., Carter G., Hinrichsen C. The membrane capacity of mammalian skeletal muscle fibres. J Muscle Res Cell Motil. 1984 Jun;5(3):315–332. doi: 10.1007/BF00713110. [DOI] [PubMed] [Google Scholar]
- Eisenberg R. S., McCarthy R. T., Milton R. L. Paralysis of frog skeletal muscle fibres by the calcium antagonist D-600. J Physiol. 1983 Aug;341:495–505. doi: 10.1113/jphysiol.1983.sp014819. [DOI] [PMC free article] [PubMed] [Google Scholar]
- Fabiato A. Calcium-induced release of calcium from the cardiac sarcoplasmic reticulum. Am J Physiol. 1983 Jul;245(1):C1–14. doi: 10.1152/ajpcell.1983.245.1.C1. [DOI] [PubMed] [Google Scholar]
- Fenwick E. M., Marty A., Neher E. A patch-clamp study of bovine chromaffin cells and of their sensitivity to acetylcholine. J Physiol. 1982 Oct;331:577–597. doi: 10.1113/jphysiol.1982.sp014393. [DOI] [PMC free article] [PubMed] [Google Scholar]
- Green F. J., Farmer B. B., Wiseman G. L., Jose M. J., Watanabe A. M. Effect of membrane depolarization on binding of [3H]nitrendipine to rat cardiac myocytes. Circ Res. 1985 Apr;56(4):576–585. doi: 10.1161/01.res.56.4.576. [DOI] [PubMed] [Google Scholar]
- Hamill O. P., Marty A., Neher E., Sakmann B., Sigworth F. J. Improved patch-clamp techniques for high-resolution current recording from cells and cell-free membrane patches. Pflugers Arch. 1981 Aug;391(2):85–100. doi: 10.1007/BF00656997. [DOI] [PubMed] [Google Scholar]
- Hess P., Lansman J. B., Tsien R. W. Different modes of Ca channel gating behaviour favoured by dihydropyridine Ca agonists and antagonists. Nature. 1984 Oct 11;311(5986):538–544. doi: 10.1038/311538a0. [DOI] [PubMed] [Google Scholar]
- Hess P., Tsien R. W. Mechanism of ion permeation through calcium channels. 1984 May 31-Jun 6Nature. 309(5967):453–456. doi: 10.1038/309453a0. [DOI] [PubMed] [Google Scholar]
- Hui C. S., Milton R. L., Eisenberg R. S. Charge movement in skeletal muscle fibers paralyzed by the calcium-entry blocker D600. Proc Natl Acad Sci U S A. 1984 Apr;81(8):2582–2585. doi: 10.1073/pnas.81.8.2582. [DOI] [PMC free article] [PubMed] [Google Scholar]
- Kokubun S., Prod'hom B., Becker C., Porzig H., Reuter H. Studies on Ca channels in intact cardiac cells: voltage-dependent effects and cooperative interactions of dihydropyridine enantiomers. Mol Pharmacol. 1986 Dec;30(6):571–584. [PubMed] [Google Scholar]
- Lamb G. D. Asymmetric charge movement in polarized and depolarized muscle fibres of the rabbit. J Physiol. 1987 Feb;383:349–367. doi: 10.1113/jphysiol.1987.sp016413. [DOI] [PMC free article] [PubMed] [Google Scholar]
- Lamb G. D. Components of charge movement in rabbit skeletal muscle: the effect of tetracaine and nifedipine. J Physiol. 1986 Jul;376:85–100. doi: 10.1113/jphysiol.1986.sp016143. [DOI] [PMC free article] [PubMed] [Google Scholar]
- Lamb G. D., Walsh T. Calcium currents, charge movement and dihydropyridine binding in fast- and slow-twitch muscles of rat and rabbit. J Physiol. 1987 Dec;393:595–617. doi: 10.1113/jphysiol.1987.sp016843. [DOI] [PMC free article] [PubMed] [Google Scholar]
- Lee K. S., Tsien R. W. High selectivity of calcium channels in single dialysed heart cells of the guinea-pig. J Physiol. 1984 Sep;354:253–272. doi: 10.1113/jphysiol.1984.sp015374. [DOI] [PMC free article] [PubMed] [Google Scholar]
- Lux H. D., Brown A. M. Patch and whole cell calcium currents recorded simultaneously in snail neurons. J Gen Physiol. 1984 May;83(5):727–750. doi: 10.1085/jgp.83.5.727. [DOI] [PMC free article] [PubMed] [Google Scholar]
- McDonald T. F., Cavalié A., Trautwein W., Pelzer D. Voltage-dependent properties of macroscopic and elementary calcium channel currents in guinea pig ventricular myocytes. Pflugers Arch. 1986 May;406(5):437–448. doi: 10.1007/BF00583365. [DOI] [PubMed] [Google Scholar]
- Mitra R., Morad M. Two types of calcium channels in guinea pig ventricular myocytes. Proc Natl Acad Sci U S A. 1986 Jul;83(14):5340–5344. doi: 10.1073/pnas.83.14.5340. [DOI] [PMC free article] [PubMed] [Google Scholar]
- Moses R. L., Kasten F. H. T-tubes in cultured mammalian myocardial cells. Cell Tissue Res. 1979;203(2):173–180. doi: 10.1007/BF00237231. [DOI] [PubMed] [Google Scholar]
- Nilius B., Hess P., Lansman J. B., Tsien R. W. A novel type of cardiac calcium channel in ventricular cells. Nature. 1985 Aug 1;316(6027):443–446. doi: 10.1038/316443a0. [DOI] [PubMed] [Google Scholar]
- Rios E., Brum G. Involvement of dihydropyridine receptors in excitation-contraction coupling in skeletal muscle. Nature. 1987 Feb 19;325(6106):717–720. doi: 10.1038/325717a0. [DOI] [PubMed] [Google Scholar]
- Schneider M. F., Chandler W. K. Voltage dependent charge movement of skeletal muscle: a possible step in excitation-contraction coupling. Nature. 1973 Mar 23;242(5395):244–246. doi: 10.1038/242244a0. [DOI] [PubMed] [Google Scholar]
- Schwartz L. M., McCleskey E. W., Almers W. Dihydropyridine receptors in muscle are voltage-dependent but most are not functional calcium channels. 1985 Apr 25-May 1Nature. 314(6013):747–751. doi: 10.1038/314747a0. [DOI] [PubMed] [Google Scholar]
- Strong J. A., Fox A. P., Tsien R. W., Kaczmarek L. K. Stimulation of protein kinase C recruits covert calcium channels in Aplysia bag cell neurons. Nature. 1987 Feb 19;325(6106):714–717. doi: 10.1038/325714a0. [DOI] [PubMed] [Google Scholar]
- Yatani A., Kunze D. L., Brown A. M. Effects of dihydropyridine calcium channel modulators on cardiac sodium channels. Am J Physiol. 1988 Jan;254(1 Pt 2):H140–H147. doi: 10.1152/ajpheart.1988.254.1.H140. [DOI] [PubMed] [Google Scholar]