Abstract
1. Sodium channel current density was measured using the loose-patch voltage clamp technique. Innervated rat, mouse and snake muscle had the highest density of Na+ channels in the end-plate region. These high Na+ channel densities were maintained in denervated muscle. 2. Perijunctional membrane had a Na+ current density 5- to 10-fold greater than the density several hundred micrometres from the end-plate. In all muscles this concentration of channels near the end-plate persisted following denervation. 3. At the tendon Na+ current density fell to low values (approximately 1 mA/cm2). The decrease in density began about 300-500 microns from the tendon. This pattern was found in all snake twitch fibres and fast-twitch (EDL) rat and mouse muscle fibres. This reduction in channel density near the tendon was not affected by denervation. 4. Sodium channels in all regions of innervated rat and snake muscle fibres were highly sensitive to tetrodotoxin (TTX). Sodium channels in snake muscle remained sensitive to TTX after denervation. Sodium channels that are relatively resistant to TTX appeared in rat muscle after denervation. TTX-resistant channels were even more concentrated near the end-plate than were TTX-sensitive channels in innervated muscle. At the tendon TTX-resistant Na+ channel density decreased. 5. We conclude that although the nerve presumably directs the localization of Na+ channels during development, the ability to maintain this distribution and to control the distribution of newly appearing channels persists long after the nerve has been removed.
Full text
PDF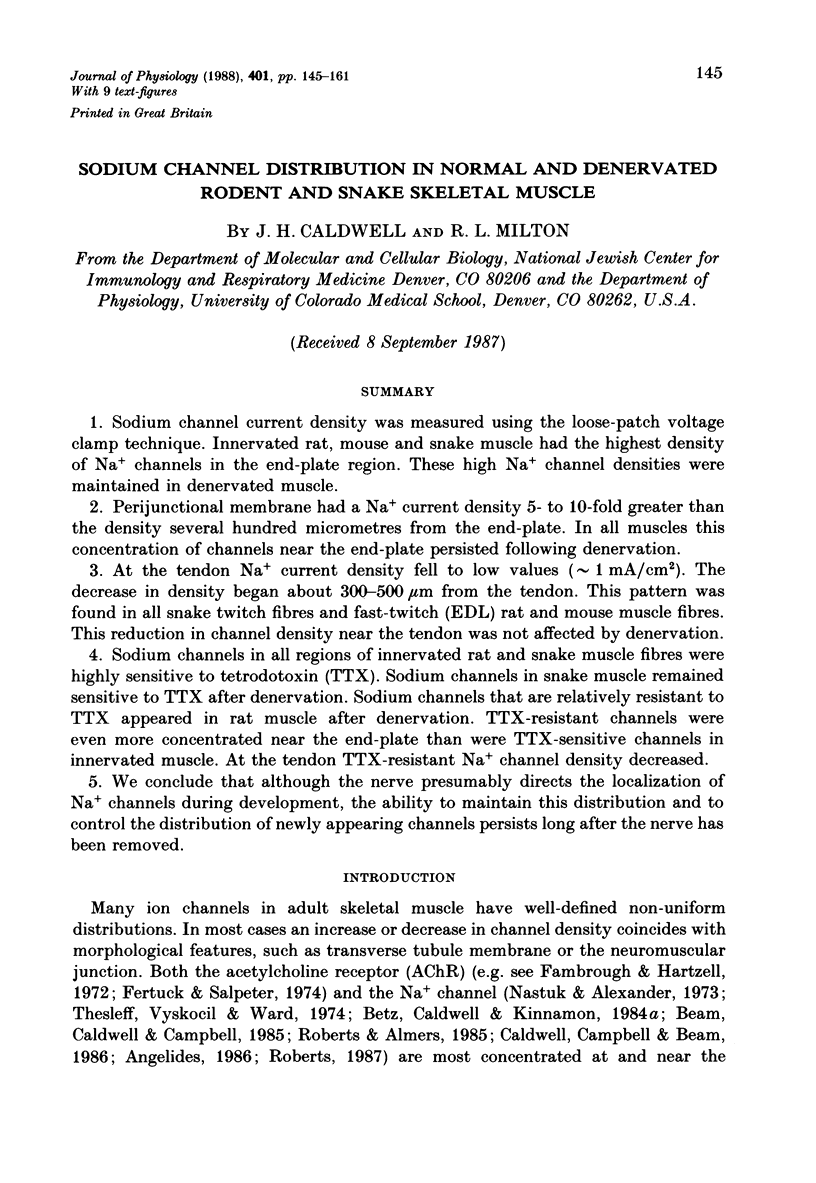
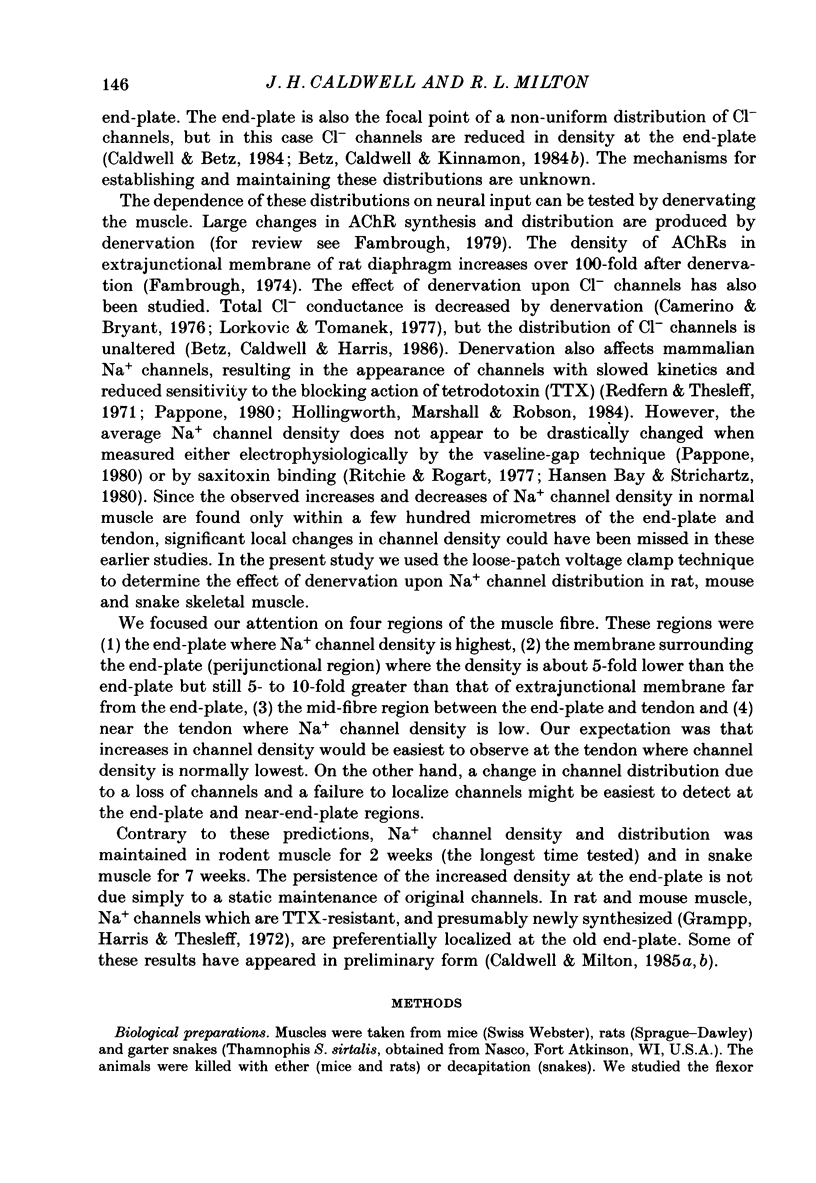
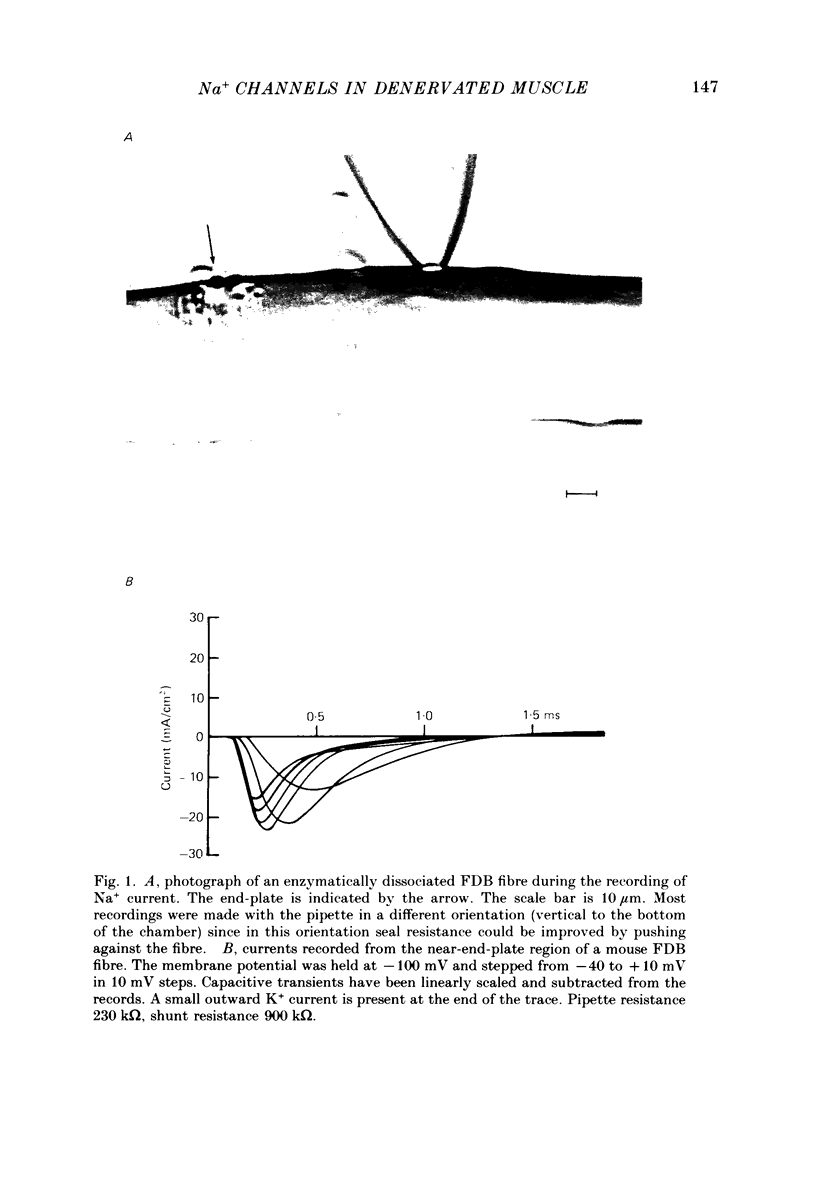
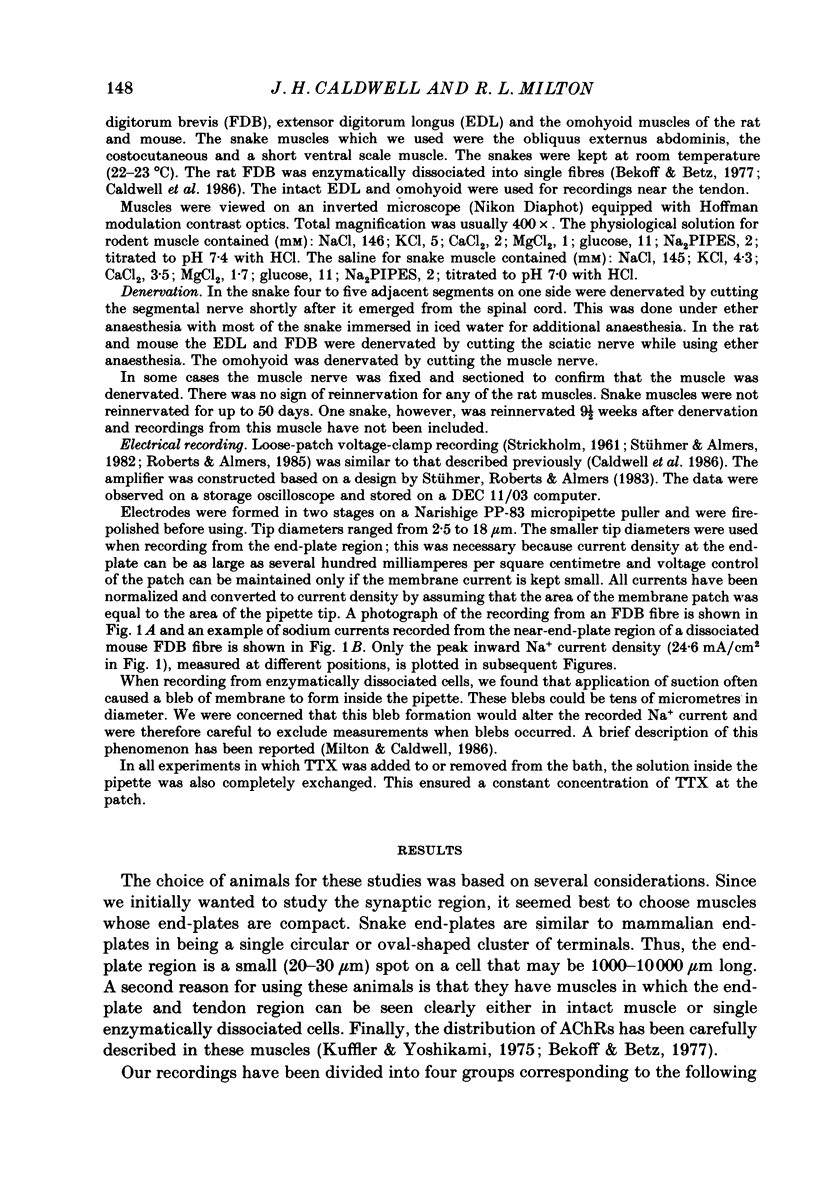
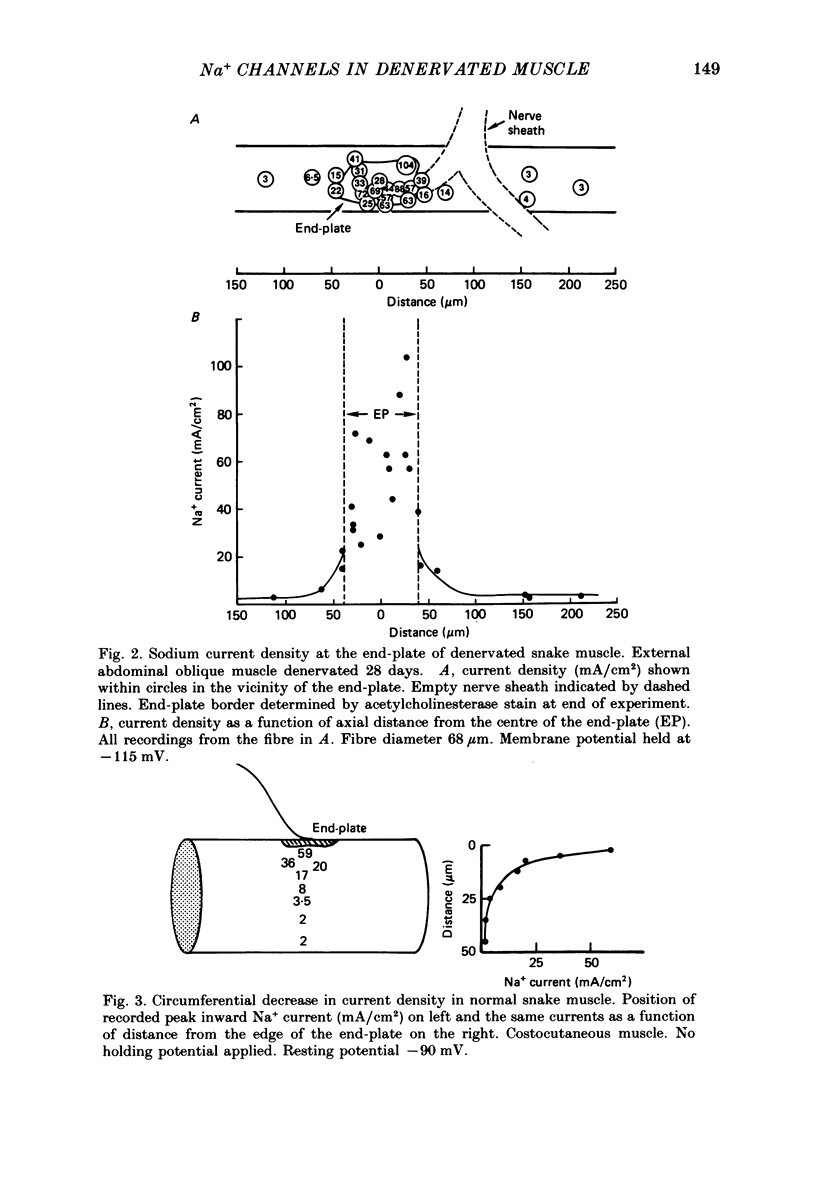
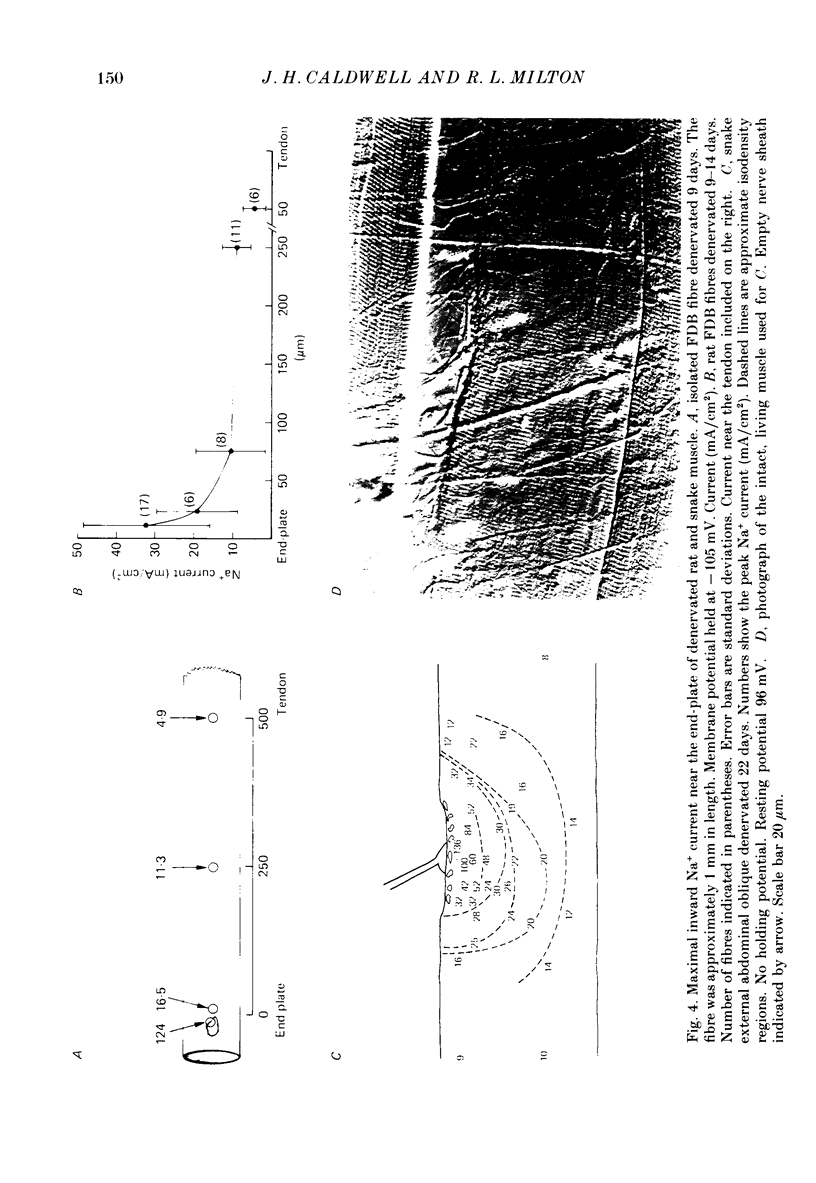
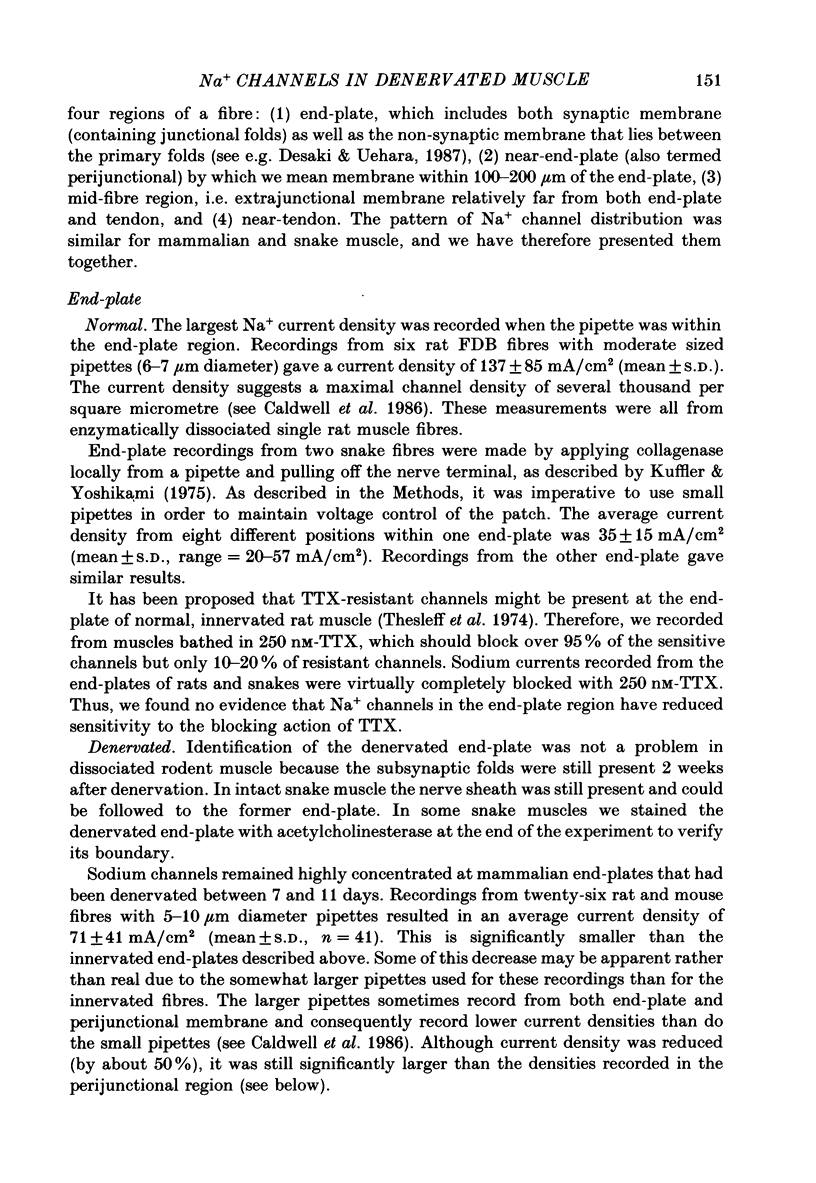
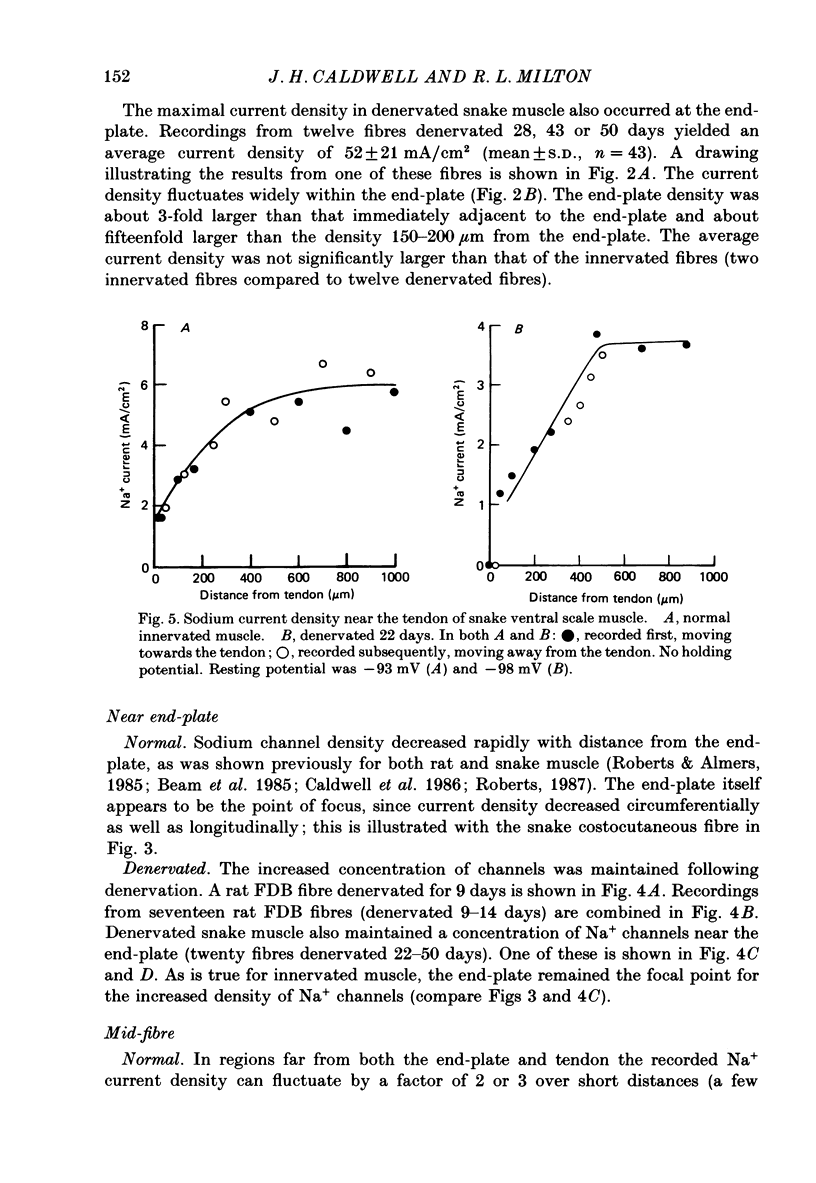
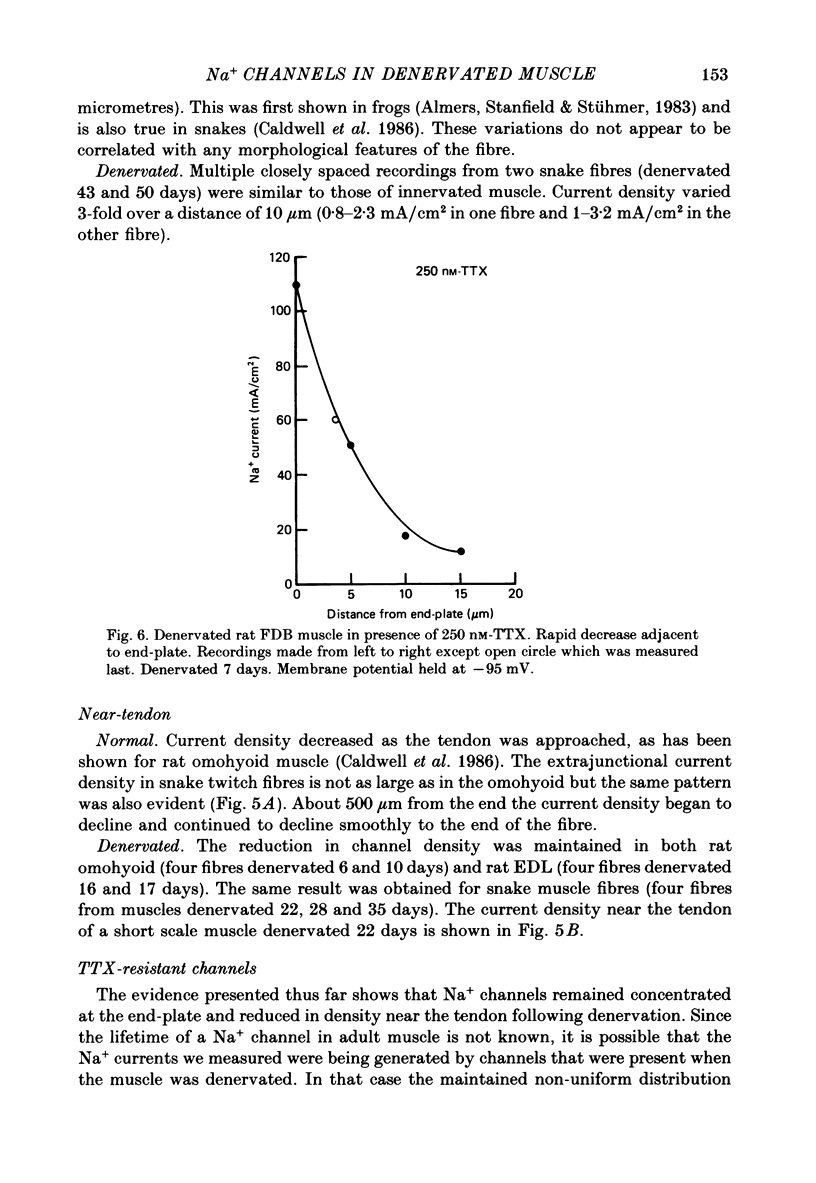
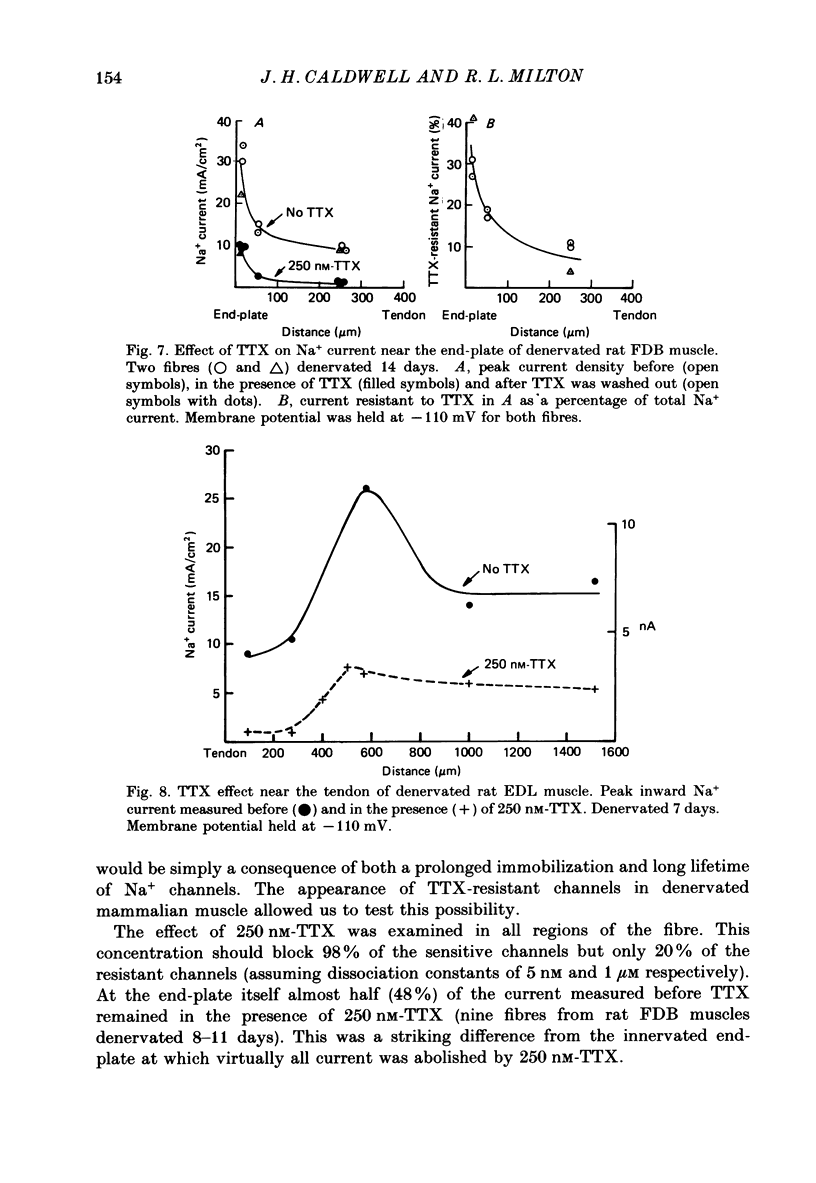
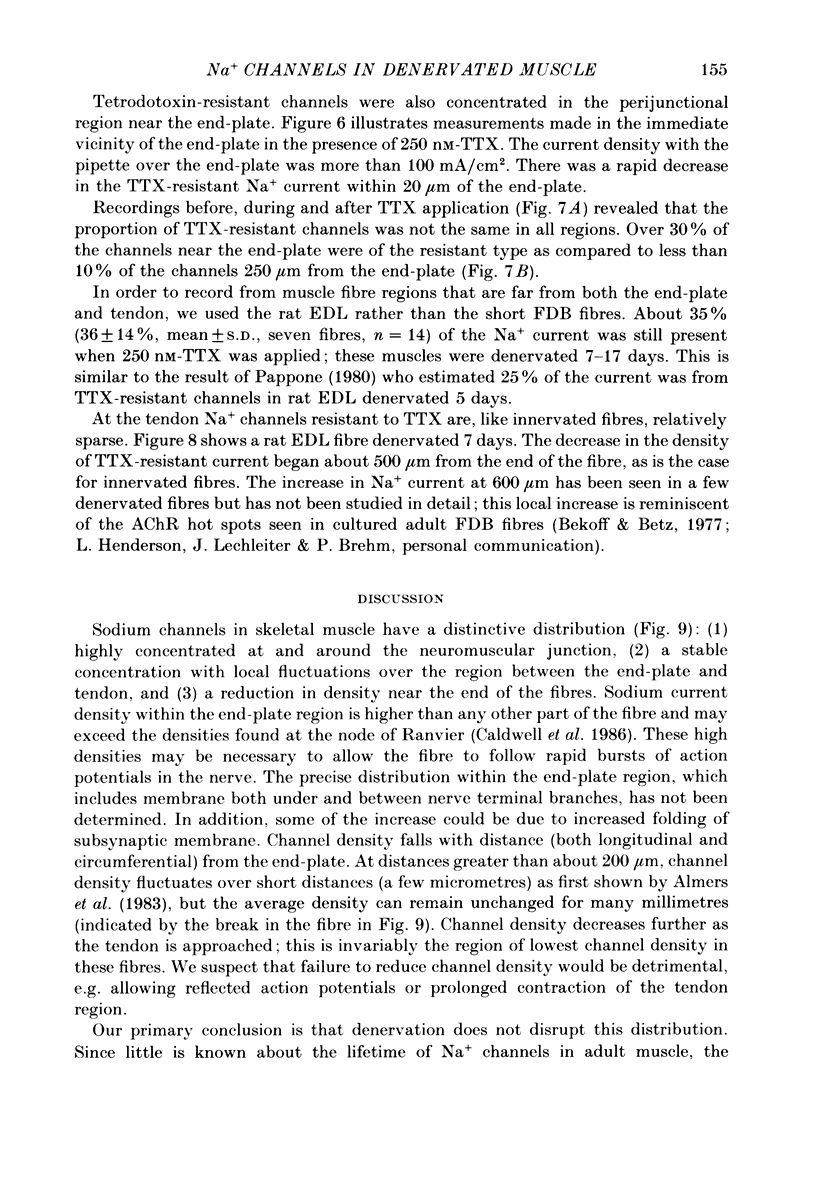
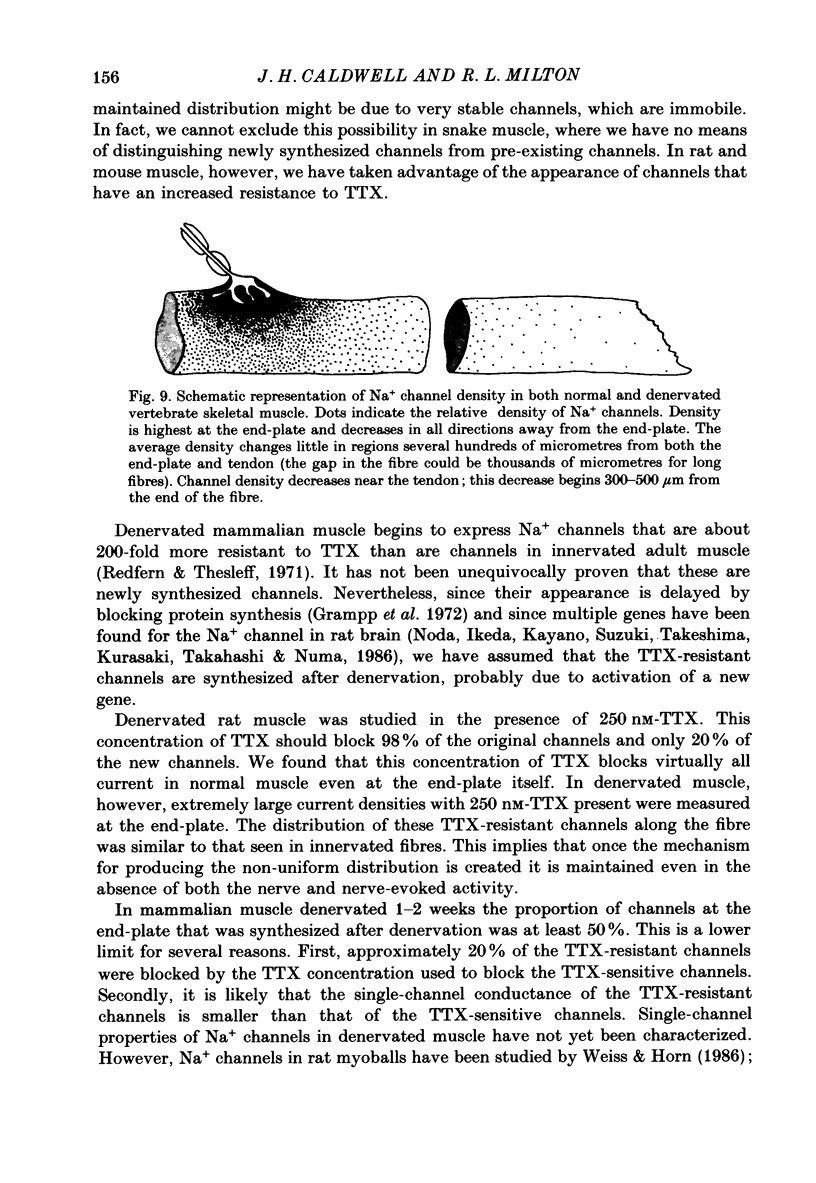
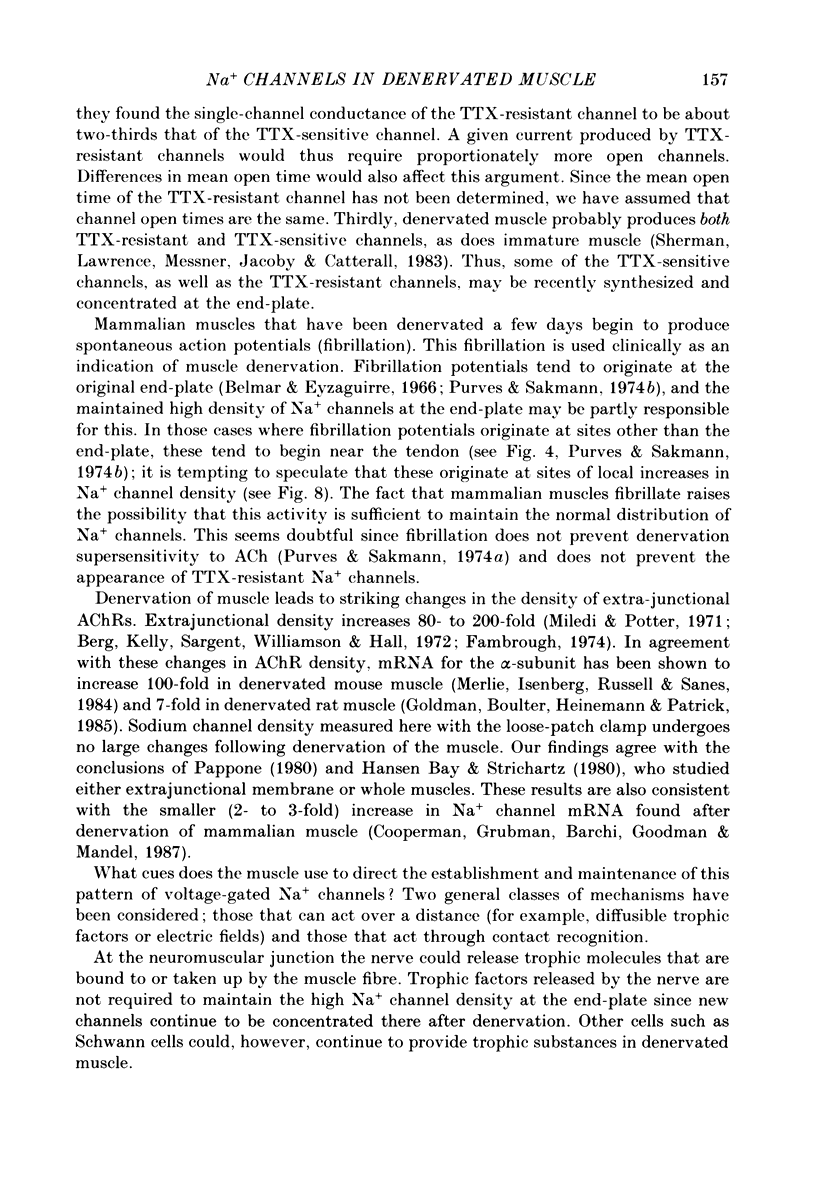
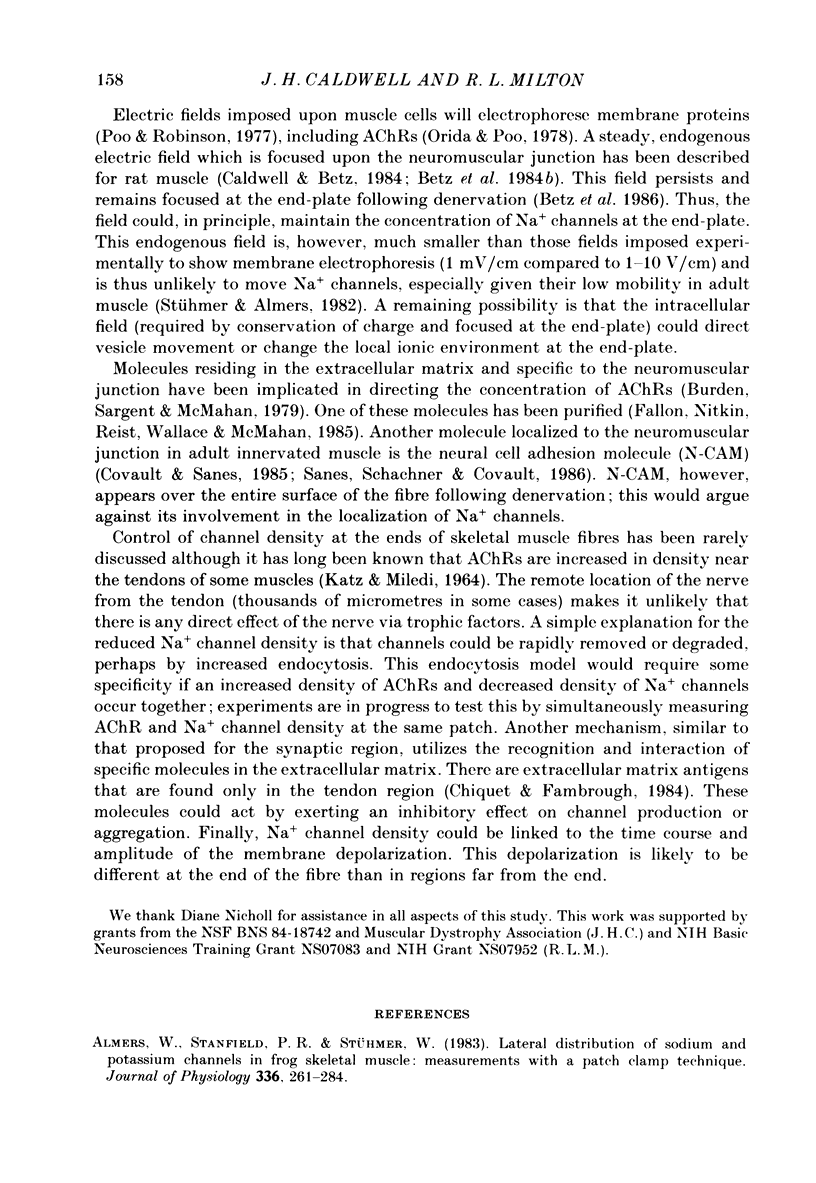
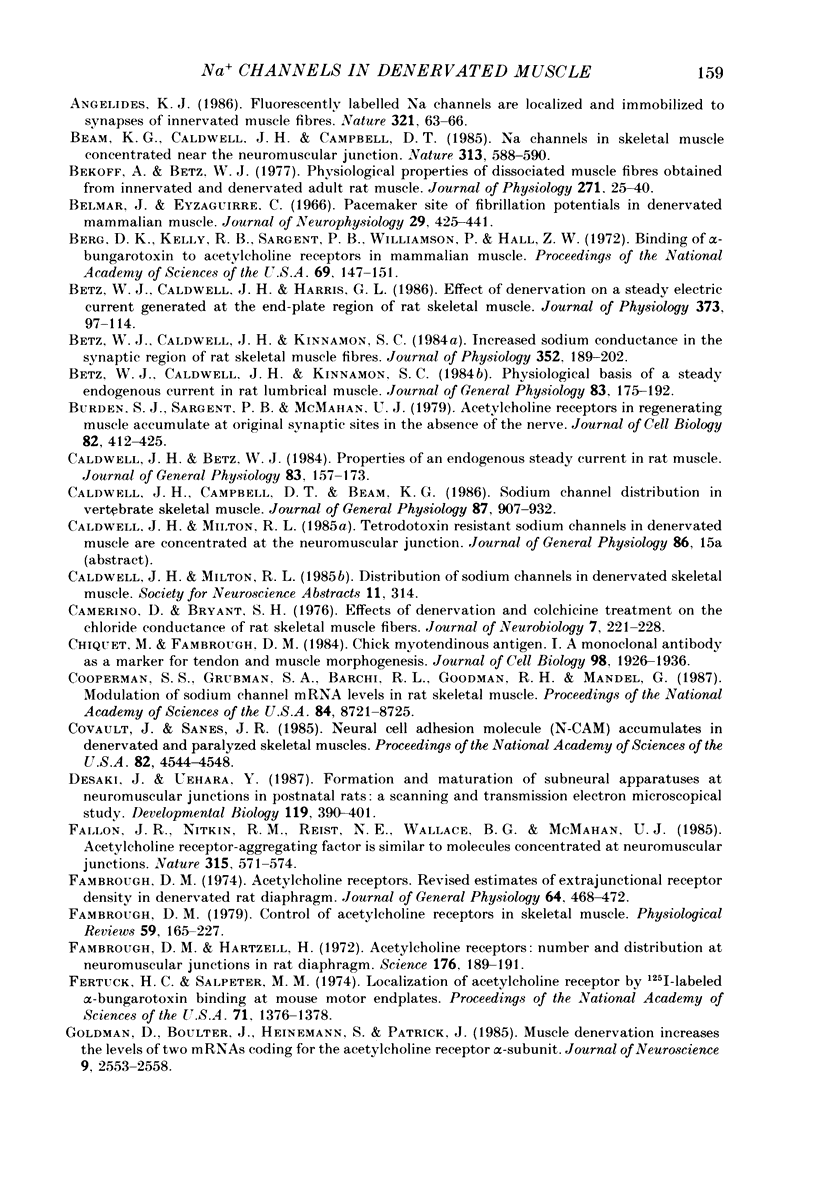
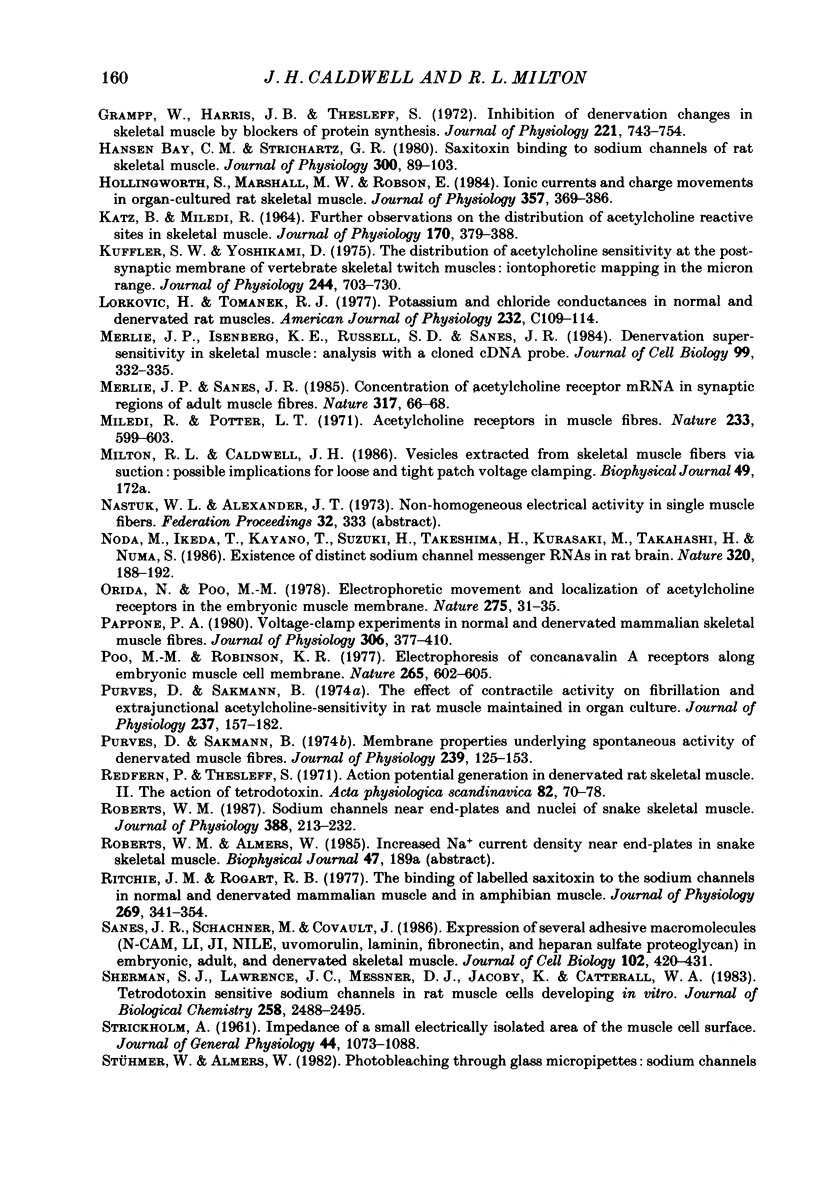
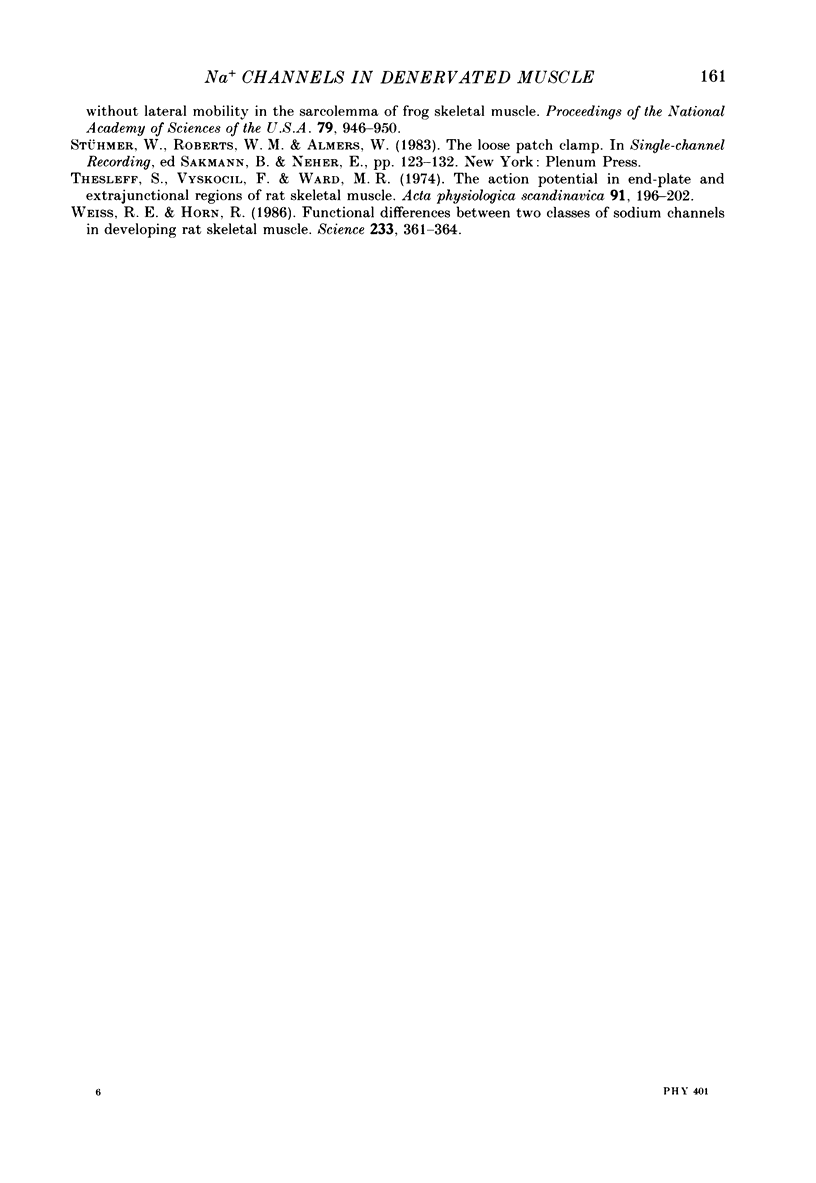
Images in this article
Selected References
These references are in PubMed. This may not be the complete list of references from this article.
- Almers W., Stanfield P. R., Stühmer W. Lateral distribution of sodium and potassium channels in frog skeletal muscle: measurements with a patch-clamp technique. J Physiol. 1983 Mar;336:261–284. doi: 10.1113/jphysiol.1983.sp014580. [DOI] [PMC free article] [PubMed] [Google Scholar]
- Angelides K. J. Fluorescently labelled Na+ channels are localized and immobilized to synapses of innervated muscle fibres. Nature. 1986 May 1;321(6065):63–66. doi: 10.1038/321063a0. [DOI] [PubMed] [Google Scholar]
- Bay C. M., Strichartz G. R. Saxitoxin binding to sodium channels of rat skeletal muscles. J Physiol. 1980 Mar;300:89–103. doi: 10.1113/jphysiol.1980.sp013153. [DOI] [PMC free article] [PubMed] [Google Scholar]
- Beam K. G., Caldwell J. H., Campbell D. T. Na channels in skeletal muscle concentrated near the neuromuscular junction. Nature. 1985 Feb 14;313(6003):588–590. doi: 10.1038/313588a0. [DOI] [PubMed] [Google Scholar]
- Bekoff A., Betz W. J. Physiological properties of dissociated muscle fibres obtained from innervated and denervated adult rat muscle. J Physiol. 1977 Sep;271(1):25–40. doi: 10.1113/jphysiol.1977.sp011988. [DOI] [PMC free article] [PubMed] [Google Scholar]
- Belmar J., Eyzaguirre C. Pacemaker site of fibrillation potentials in denervated mammmalian muscle. J Neurophysiol. 1966 May;29(3):425–441. doi: 10.1152/jn.1966.29.3.425. [DOI] [PubMed] [Google Scholar]
- Berg D. K., Kelly R. B., Sargent P. B., Williamson P., Hall Z. W. Binding of -bungarotoxin to acetylcholine receptors in mammalian muscle (snake venom-denervated muscle-neonatal muscle-rat diaphragm-SDS-polyacrylamide gel electrophoresis). Proc Natl Acad Sci U S A. 1972 Jan;69(1):147–151. doi: 10.1073/pnas.69.1.147. [DOI] [PMC free article] [PubMed] [Google Scholar]
- Betz W. J., Caldwell J. H., Harris G. L. Effect of denervation on a steady electric current generated at the end-plate region of rat skeletal muscle. J Physiol. 1986 Apr;373:97–114. doi: 10.1113/jphysiol.1986.sp016037. [DOI] [PMC free article] [PubMed] [Google Scholar]
- Betz W. J., Caldwell J. H., Kinnamon S. C. Increased sodium conductance in the synaptic region of rat skeletal muscle fibres. J Physiol. 1984 Jul;352:189–202. doi: 10.1113/jphysiol.1984.sp015286. [DOI] [PMC free article] [PubMed] [Google Scholar]
- Betz W. J., Caldwell J. H., Kinnamon S. C. Physiological basis of a steady endogenous current in rat lumbrical muscle. J Gen Physiol. 1984 Feb;83(2):175–192. doi: 10.1085/jgp.83.2.175. [DOI] [PMC free article] [PubMed] [Google Scholar]
- Burden S. J., Sargent P. B., McMahan U. J. Acetylcholine receptors in regenerating muscle accumulate at original synaptic sites in the absence of the nerve. J Cell Biol. 1979 Aug;82(2):412–425. doi: 10.1083/jcb.82.2.412. [DOI] [PMC free article] [PubMed] [Google Scholar]
- Caldwell J. H., Betz W. J. Properties of an endogenous steady current in rat muscle. J Gen Physiol. 1984 Feb;83(2):157–173. doi: 10.1085/jgp.83.2.157. [DOI] [PMC free article] [PubMed] [Google Scholar]
- Caldwell J. H., Campbell D. T., Beam K. G. Na channel distribution in vertebrate skeletal muscle. J Gen Physiol. 1986 Jun;87(6):907–932. doi: 10.1085/jgp.87.6.907. [DOI] [PMC free article] [PubMed] [Google Scholar]
- Camerino D., Bryant S. H. Effects of denervation and colchicine treatment on the chloride conductance of rat skeletal muscle fibers. J Neurobiol. 1976 May;7(3):221–228. doi: 10.1002/neu.480070305. [DOI] [PubMed] [Google Scholar]
- Chiquet M., Fambrough D. M. Chick myotendinous antigen. I. A monoclonal antibody as a marker for tendon and muscle morphogenesis. J Cell Biol. 1984 Jun;98(6):1926–1936. doi: 10.1083/jcb.98.6.1926. [DOI] [PMC free article] [PubMed] [Google Scholar]
- Cooperman S. S., Grubman S. A., Barchi R. L., Goodman R. H., Mandel G. Modulation of sodium-channel mRNA levels in rat skeletal muscle. Proc Natl Acad Sci U S A. 1987 Dec;84(23):8721–8725. doi: 10.1073/pnas.84.23.8721. [DOI] [PMC free article] [PubMed] [Google Scholar]
- Covault J., Sanes J. R. Neural cell adhesion molecule (N-CAM) accumulates in denervated and paralyzed skeletal muscles. Proc Natl Acad Sci U S A. 1985 Jul;82(13):4544–4548. doi: 10.1073/pnas.82.13.4544. [DOI] [PMC free article] [PubMed] [Google Scholar]
- Desaki J., Uehara Y. Formation and maturation of subneural apparatuses at neuromuscular junctions in postnatal rats: a scanning and transmission electron microscopical study. Dev Biol. 1987 Feb;119(2):390–401. doi: 10.1016/0012-1606(87)90044-3. [DOI] [PubMed] [Google Scholar]
- Fallon J. R., Nitkin R. M., Reist N. E., Wallace B. G., McMahan U. J. Acetylcholine receptor-aggregating factor is similar to molecules concentrated at neuromuscular junctions. Nature. 1985 Jun 13;315(6020):571–574. doi: 10.1038/315571a0. [DOI] [PubMed] [Google Scholar]
- Fambrough D. M. Acetylcholine receptors. Revised estimates of extrajunctional receptor density in denervated rat diaphragm. J Gen Physiol. 1974 Oct;64(4):468–472. doi: 10.1085/jgp.64.4.468. [DOI] [PMC free article] [PubMed] [Google Scholar]
- Fambrough D. M. Control of acetylcholine receptors in skeletal muscle. Physiol Rev. 1979 Jan;59(1):165–227. doi: 10.1152/physrev.1979.59.1.165. [DOI] [PubMed] [Google Scholar]
- Fambrough D. M., Hartzell H. C. Acetylcholine receptors: number and distribution at neuromuscular junctions in rat diaphragm. Science. 1972 Apr 14;176(4031):189–191. doi: 10.1126/science.176.4031.189. [DOI] [PubMed] [Google Scholar]
- Fertuck H. C., Salpeter M. M. Localization of acetylcholine receptor by 125I-labeled alpha-bungarotoxin binding at mouse motor endplates. Proc Natl Acad Sci U S A. 1974 Apr;71(4):1376–1378. doi: 10.1073/pnas.71.4.1376. [DOI] [PMC free article] [PubMed] [Google Scholar]
- Goldman D., Boulter J., Heinemann S., Patrick J. Muscle denervation increases the levels of two mRNAs coding for the acetylcholine receptor alpha-subunit. J Neurosci. 1985 Sep;5(9):2553–2558. doi: 10.1523/JNEUROSCI.05-09-02553.1985. [DOI] [PMC free article] [PubMed] [Google Scholar]
- Grampp W., Harris J. B., Thesleff S. Inhibition of denervation changes in skeletal muscle by blockers of protein synthesis. J Physiol. 1972 Mar;221(3):743–754. doi: 10.1113/jphysiol.1972.sp009780. [DOI] [PMC free article] [PubMed] [Google Scholar]
- Hollingworth S., Marshall M. W., Robson E. Ionic currents and charge movements in organ-cultured rat skeletal muscle. J Physiol. 1984 Dec;357:369–386. doi: 10.1113/jphysiol.1984.sp015505. [DOI] [PMC free article] [PubMed] [Google Scholar]
- KATZ B., MILEDI R. FURTHER OBSERVATIONS ON THE DISTRIBUTION OF ACTYLCHOLINE-REACTIVE SITES IN SKELETAL MUSCLE. J Physiol. 1964 Mar;170:379–388. doi: 10.1113/jphysiol.1964.sp007338. [DOI] [PMC free article] [PubMed] [Google Scholar]
- Kuffler S. W., Yoshikami D. The distribution of acetylcholine sensitivity at the post-synaptic membrane of vertebrate skeletal twitch muscles: iontophoretic mapping in the micron range. J Physiol. 1975 Jan;244(3):703–730. doi: 10.1113/jphysiol.1975.sp010821. [DOI] [PMC free article] [PubMed] [Google Scholar]
- Lorković H., Tomanek R. J. Potassium and chloride conductances in normal and denervated rat muscles. Am J Physiol. 1977 Mar;232(3):C109–C114. doi: 10.1152/ajpcell.1977.232.3.C109. [DOI] [PubMed] [Google Scholar]
- Merlie J. P., Isenberg K. E., Russell S. D., Sanes J. R. Denervation supersensitivity in skeletal muscle: analysis with a cloned cDNA probe. J Cell Biol. 1984 Jul;99(1 Pt 1):332–335. doi: 10.1083/jcb.99.1.332. [DOI] [PMC free article] [PubMed] [Google Scholar]
- Merlie J. P., Sanes J. R. Concentration of acetylcholine receptor mRNA in synaptic regions of adult muscle fibres. Nature. 1985 Sep 5;317(6032):66–68. doi: 10.1038/317066a0. [DOI] [PubMed] [Google Scholar]
- Noda M., Ikeda T., Kayano T., Suzuki H., Takeshima H., Kurasaki M., Takahashi H., Numa S. Existence of distinct sodium channel messenger RNAs in rat brain. Nature. 1986 Mar 13;320(6058):188–192. doi: 10.1038/320188a0. [DOI] [PubMed] [Google Scholar]
- Orida N., Poo M. M. Electrophoretic movement and localisation of acetylcholine receptors in the embryonic muscle cell membrane. Nature. 1978 Sep 7;275(5675):31–35. doi: 10.1038/275031a0. [DOI] [PubMed] [Google Scholar]
- Pappone P. A. Voltage-clamp experiments in normal and denervated mammalian skeletal muscle fibres. J Physiol. 1980 Sep;306:377–410. doi: 10.1113/jphysiol.1980.sp013403. [DOI] [PMC free article] [PubMed] [Google Scholar]
- Poo M., Robinson K. R. Electrophoresis of concanavalin A receptors along embryonic muscle cell membrane. Nature. 1977 Feb 17;265(5595):602–605. doi: 10.1038/265602a0. [DOI] [PubMed] [Google Scholar]
- Purves D., Sakmann B. Membrane properties underlying spontaneous activity of denervated muscle fibres. J Physiol. 1974 May;239(1):125–153. doi: 10.1113/jphysiol.1974.sp010559. [DOI] [PMC free article] [PubMed] [Google Scholar]
- Purves D., Sakmann B. The effect of contractile activity on fibrillation and extrajunctional acetylcholine-sensitivity in rat muscle maintained in organ culture. J Physiol. 1974 Feb;237(1):157–182. doi: 10.1113/jphysiol.1974.sp010475. [DOI] [PMC free article] [PubMed] [Google Scholar]
- Redfern P., Thesleff S. Action potential generation in denervated rat skeletal muscle. II. The action of tetrodotoxin. Acta Physiol Scand. 1971 May;82(1):70–78. doi: 10.1111/j.1748-1716.1971.tb04943.x. [DOI] [PubMed] [Google Scholar]
- Ritchie J. M., Rogart R. B. The binding of labelled saxitoxin to the sodium channels in normal and denervated mammalian muscle, and in amphibian muscle. J Physiol. 1977 Jul;269(2):341–354. doi: 10.1113/jphysiol.1977.sp011905. [DOI] [PMC free article] [PubMed] [Google Scholar]
- Roberts W. M. Sodium channels near end-plates and nuclei of snake skeletal muscle. J Physiol. 1987 Jul;388:213–232. doi: 10.1113/jphysiol.1987.sp016611. [DOI] [PMC free article] [PubMed] [Google Scholar]
- Sanes J. R., Schachner M., Covault J. Expression of several adhesive macromolecules (N-CAM, L1, J1, NILE, uvomorulin, laminin, fibronectin, and a heparan sulfate proteoglycan) in embryonic, adult, and denervated adult skeletal muscle. J Cell Biol. 1986 Feb;102(2):420–431. doi: 10.1083/jcb.102.2.420. [DOI] [PMC free article] [PubMed] [Google Scholar]
- Sherman S. J., Lawrence J. C., Messner D. J., Jacoby K., Catterall W. A. Tetrodotoxin-sensitive sodium channels in rat muscle cells developing in vitro. J Biol Chem. 1983 Feb 25;258(4):2488–2495. [PubMed] [Google Scholar]
- Strickholm A. Impedance of a Small Electrically Isolated Area of the Muscle Cell Surface. J Gen Physiol. 1961 Jul 1;44(6):1073–1088. doi: 10.1085/jgp.44.6.1073. [DOI] [PMC free article] [PubMed] [Google Scholar]
- Stühmer W., Almers W. Photobleaching through glass micropipettes: sodium channels without lateral mobility in the sarcolemma of frog skeletal muscle. Proc Natl Acad Sci U S A. 1982 Feb;79(3):946–950. doi: 10.1073/pnas.79.3.946. [DOI] [PMC free article] [PubMed] [Google Scholar]
- Thesleff S., Vyskocil F., Ward M. R. The action potential in end-plate and extrajunctional regions of rat skeletal muscle. Acta Physiol Scand. 1974 Jun;91(2):196–202. doi: 10.1111/j.1748-1716.1974.tb05676.x. [DOI] [PubMed] [Google Scholar]
- Weiss R. E., Horn R. Functional differences between two classes of sodium channels in developing rat skeletal muscle. Science. 1986 Jul 18;233(4761):361–364. doi: 10.1126/science.2425432. [DOI] [PubMed] [Google Scholar]