Abstract
1. Suspended Swiss 3T3 fibroblasts were voltage clamped using the whole-cell technique. 2. Passage from the cell-attached to the whole-cell mode was accompanied by only a minor decrease in input resistance. Direct measurement of resting potential gave values between O and -15 mV. 3. In order to account for the effects of leak on the membrane potential measurements, I-V curves were obtained immediately before and after patch rupture by applying voltage ramps. After subtraction of the cell-attached current from the whole-cell current, the true membrane potential was estimated as the zero-current potential in the I-V curve. An average value of -8.2 +/- 0.9 mV in 8 mM-Ca2+ was obtained in this way. 4. In 2 mM-Ca2+, step depolarizations 100 ms long from holding potentials (Vh) more negative than -60 mV caused a transient inward current to appear. From Vh greater than -60 mV only a linear leakage component was apparent. 5. In 2 mM-Ca2+ depolarizations to potentials greater than +40 mV (from Vh = -100 mV) generated transient, outwardly directed currents. 6. Increasing extracellular Ca2+ up to 32 mM shifted the peak current vs. voltage curve and the reversal potential (Erev) towards more positive potentials, and caused an increase of the peak current. 7. The steady-state inactivation curve was the same for both inward and outward currents, indicating that they flow through the same channels. The currents are completely inactivated at V = -60 mV. 8. Recovery of the fully inactivated current upon hyperpolarization had an exponential time course with tau = 0.22 s at V = -80 mV and tau = 0.18 s at V = -100 mV. 9. In the absence of Ca2+ (but with Mg2+ present) the inward current disappeared but a large, inactivating outward current appeared when V greater than 0 mV. The current was strongly reduced by Cd2+ (1 mM) or Co2+ (10 mM). 10. Complete removal of divalent cations from the external solution caused the channel to become highly permeable to monovalent cations. 11. Nitrendipine (10 microM) and verapamil (5 microM) were unable to block the current. 12. On the whole the present results indicate that voltage-dependent Ca2+ channels are present in these cells. Their sensitivity to divalent cations, to organic blockers and to potential is similar to that of the low-voltage-activated, or 'T' type, Ca2+ channels described in other cells.
Full text
PDF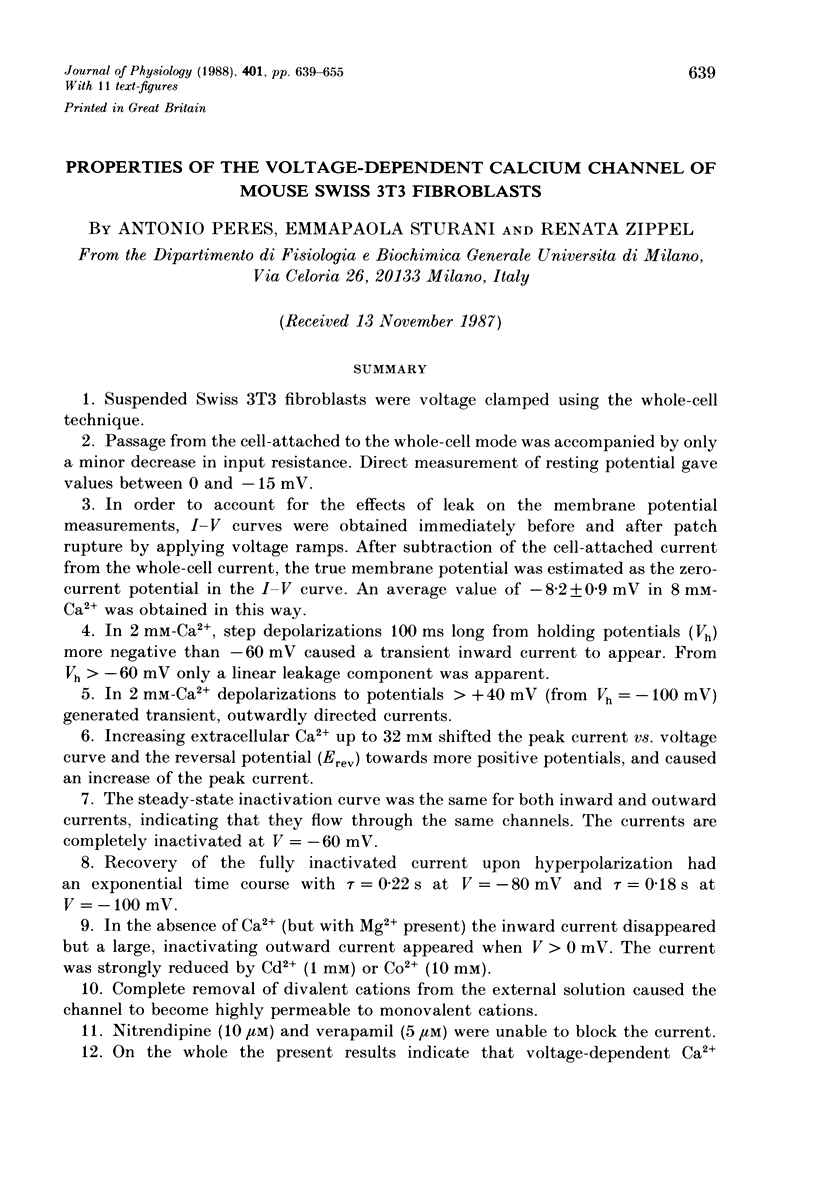
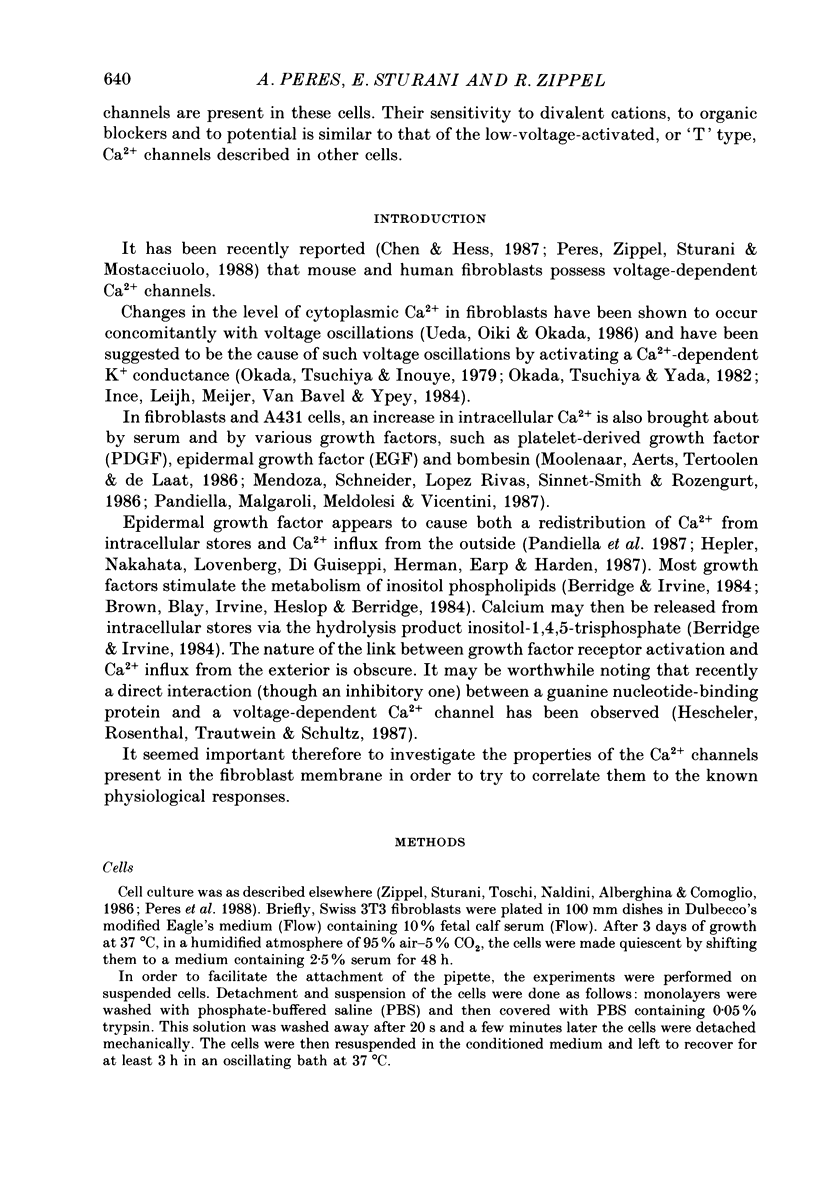
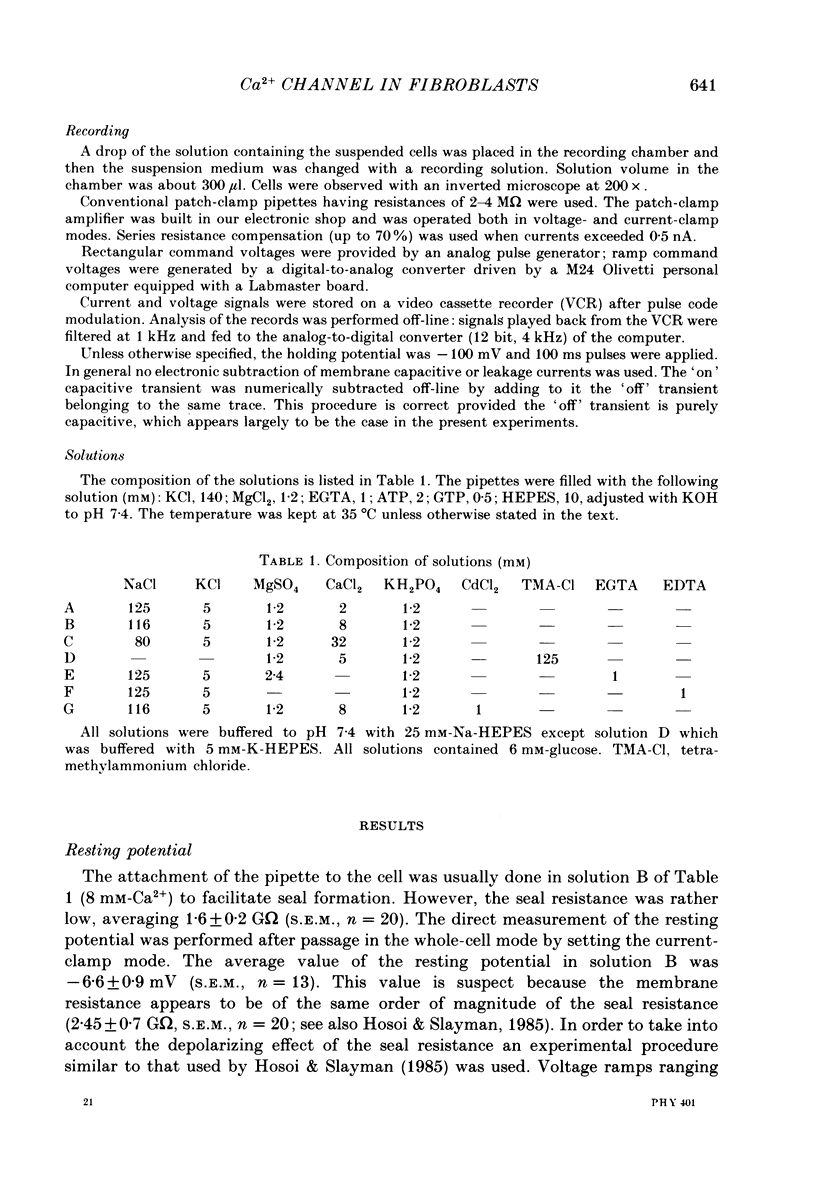
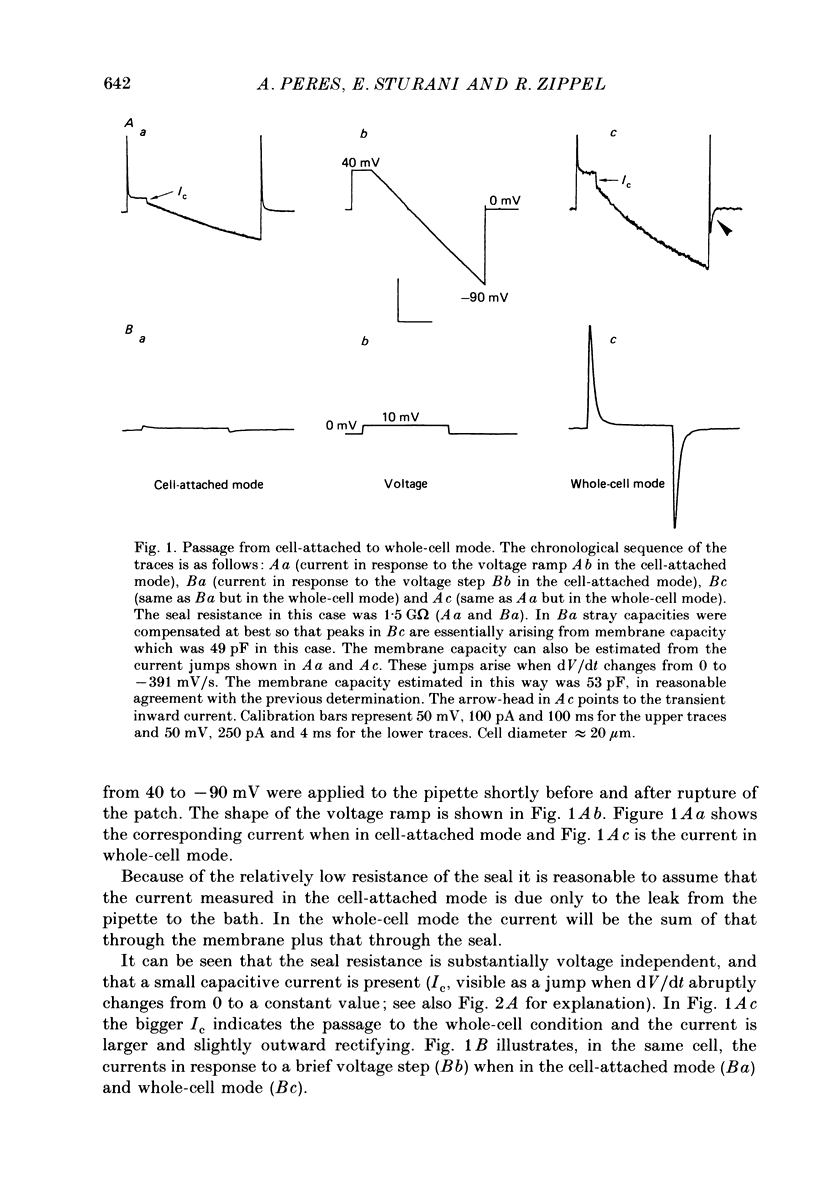
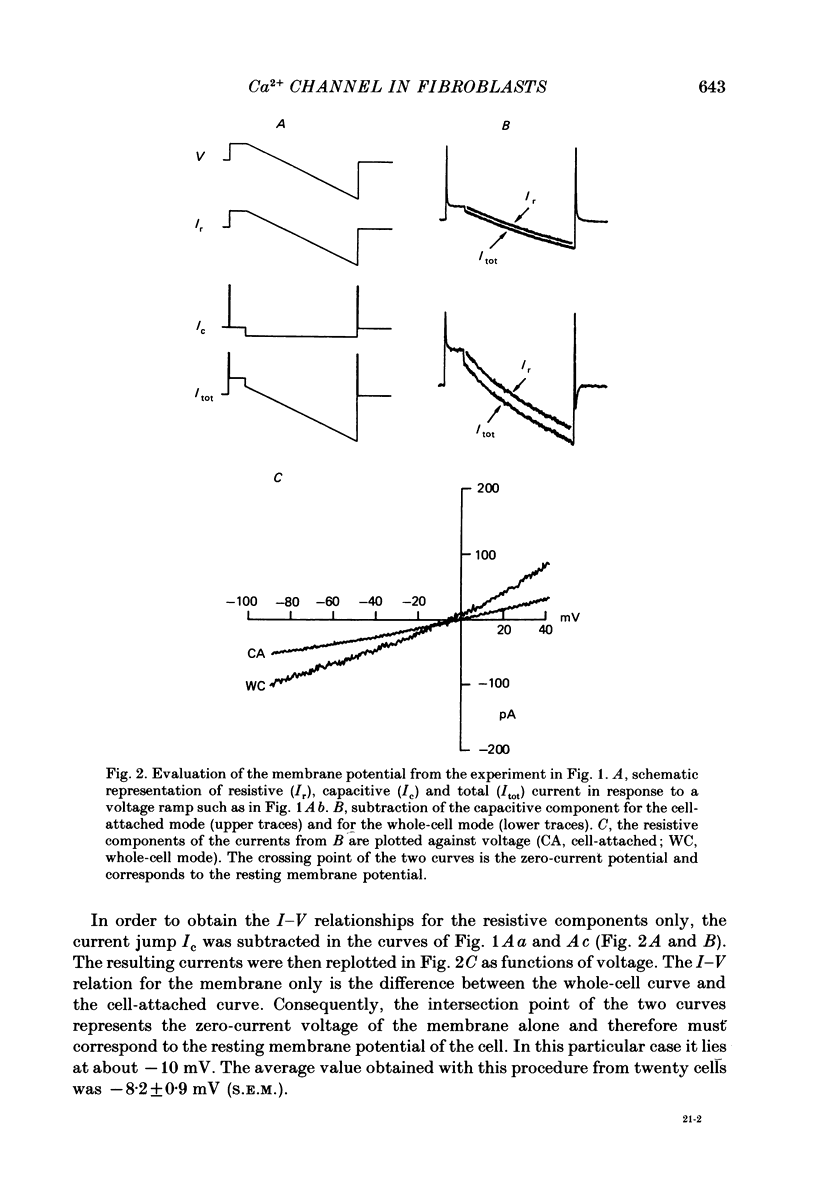
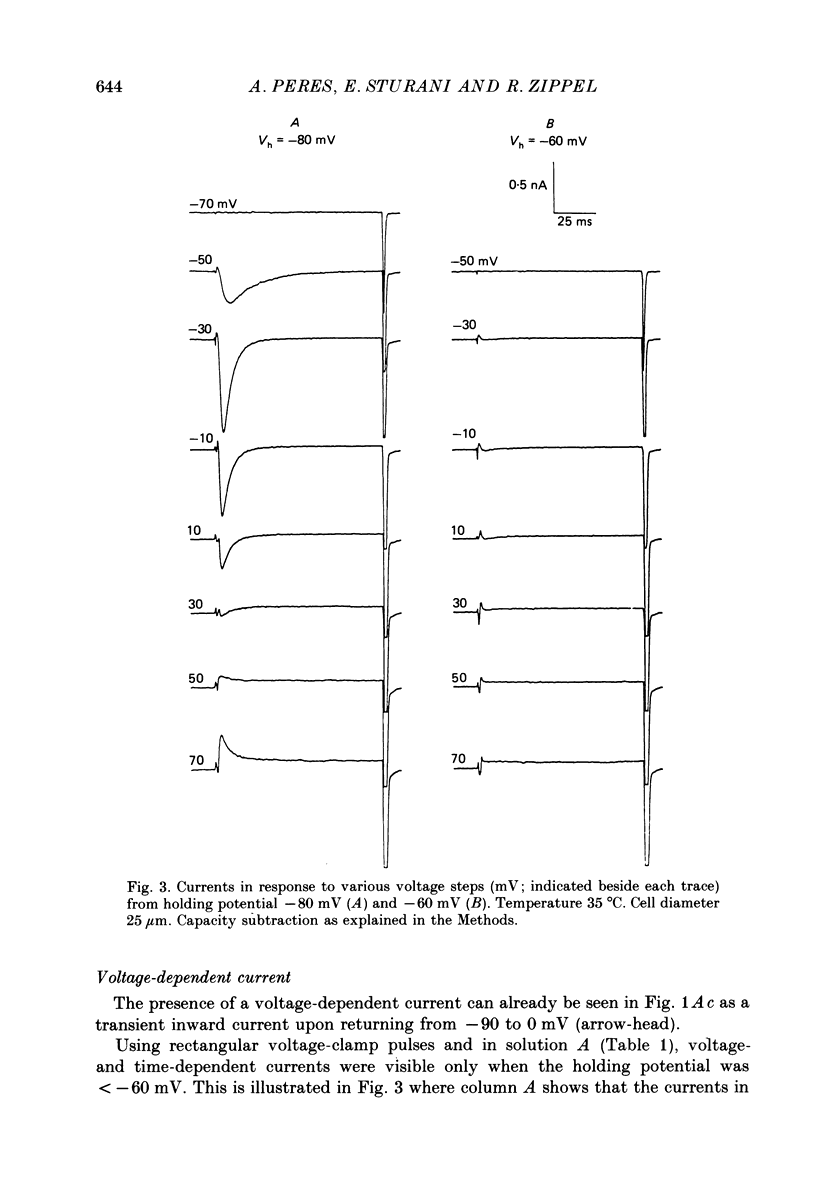
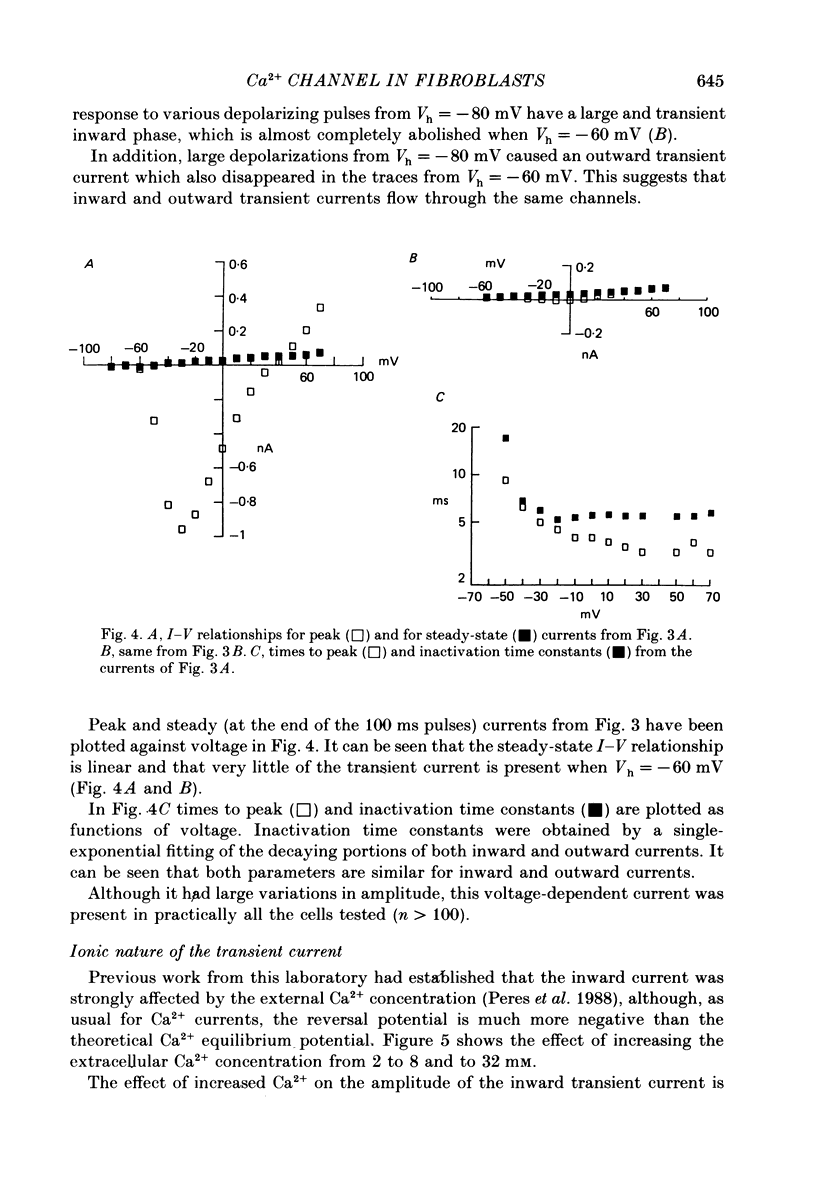
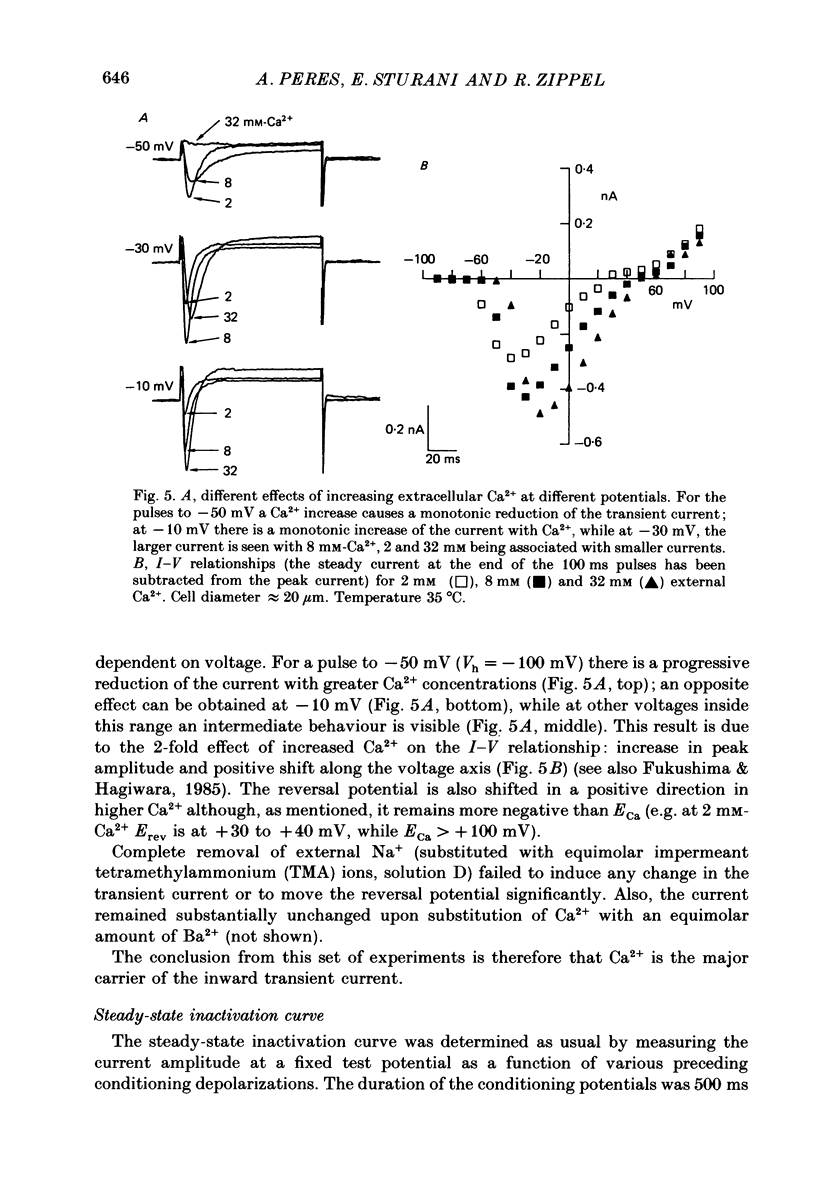
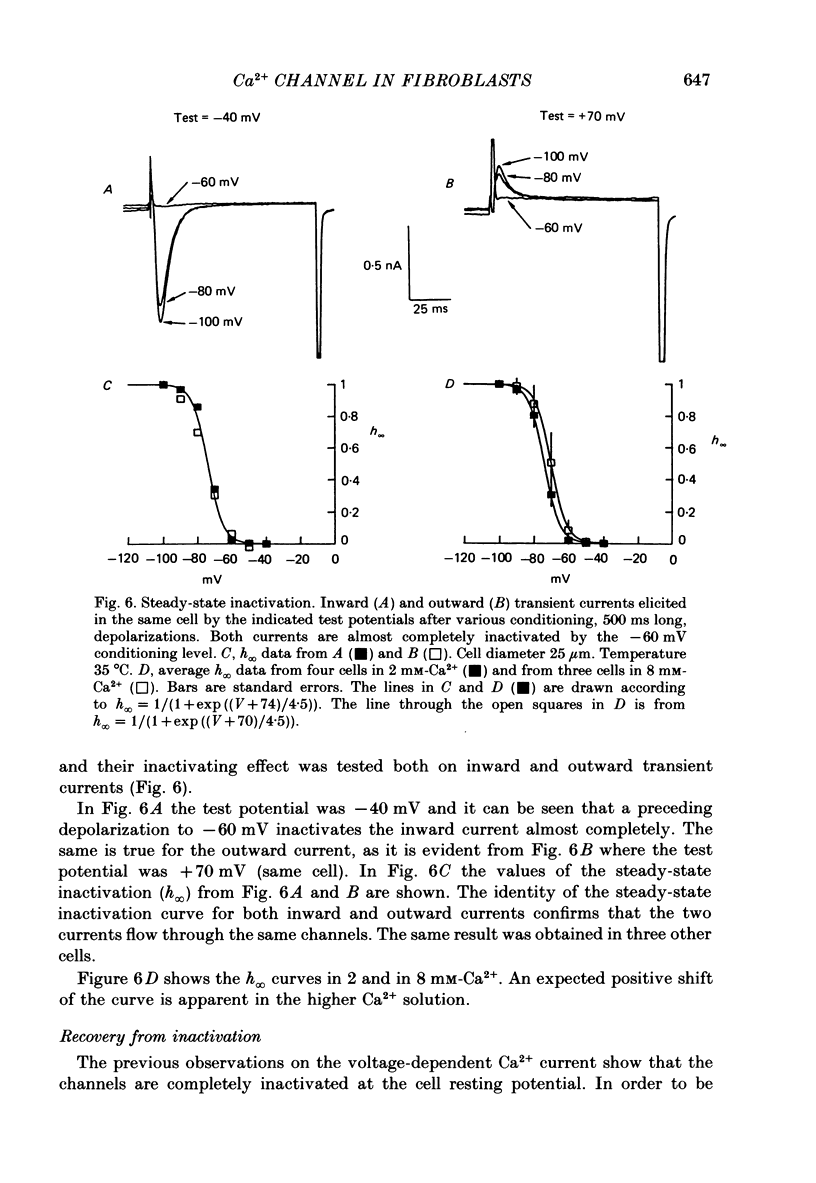
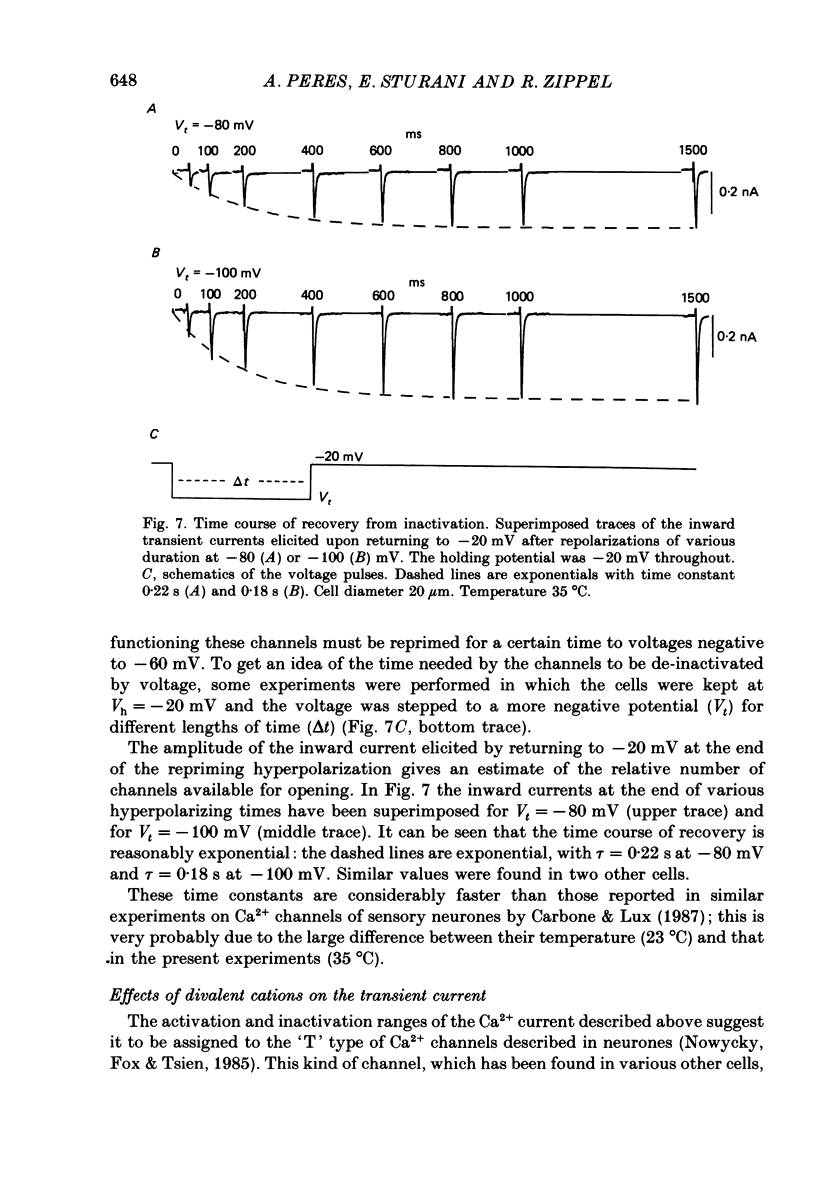
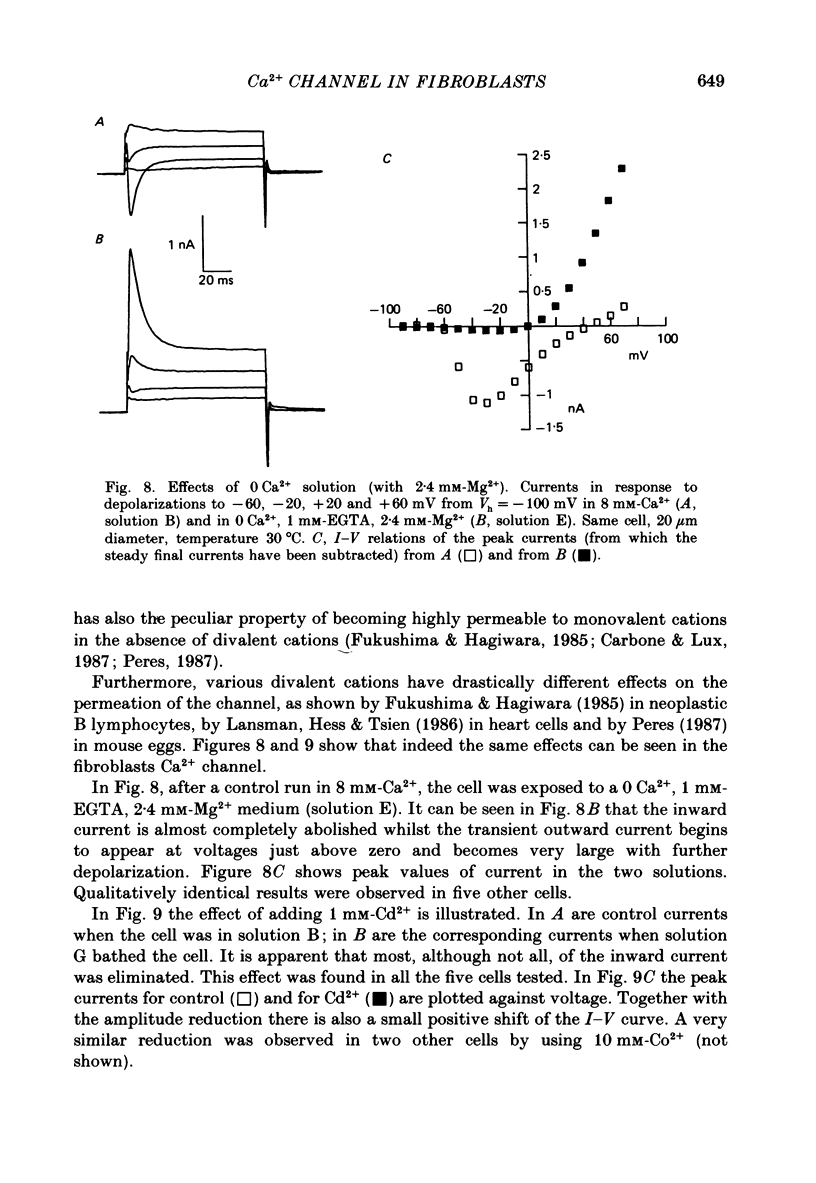
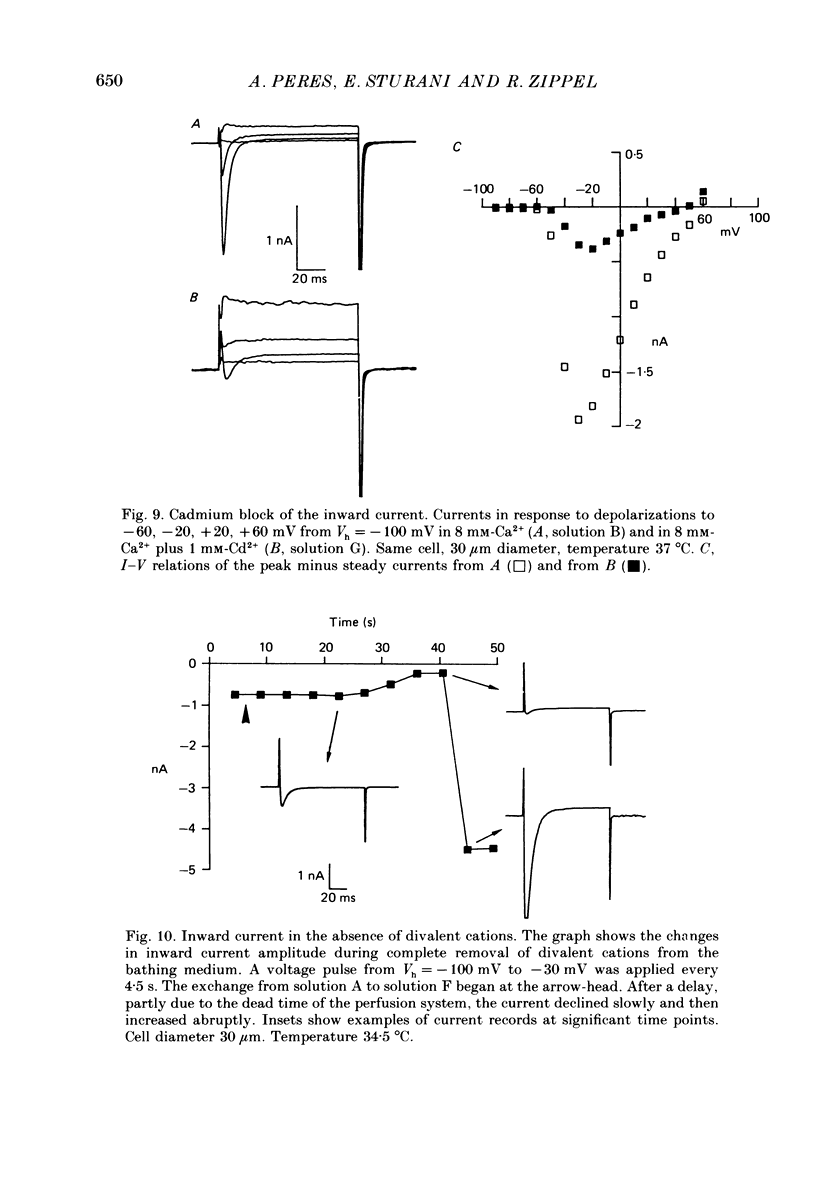
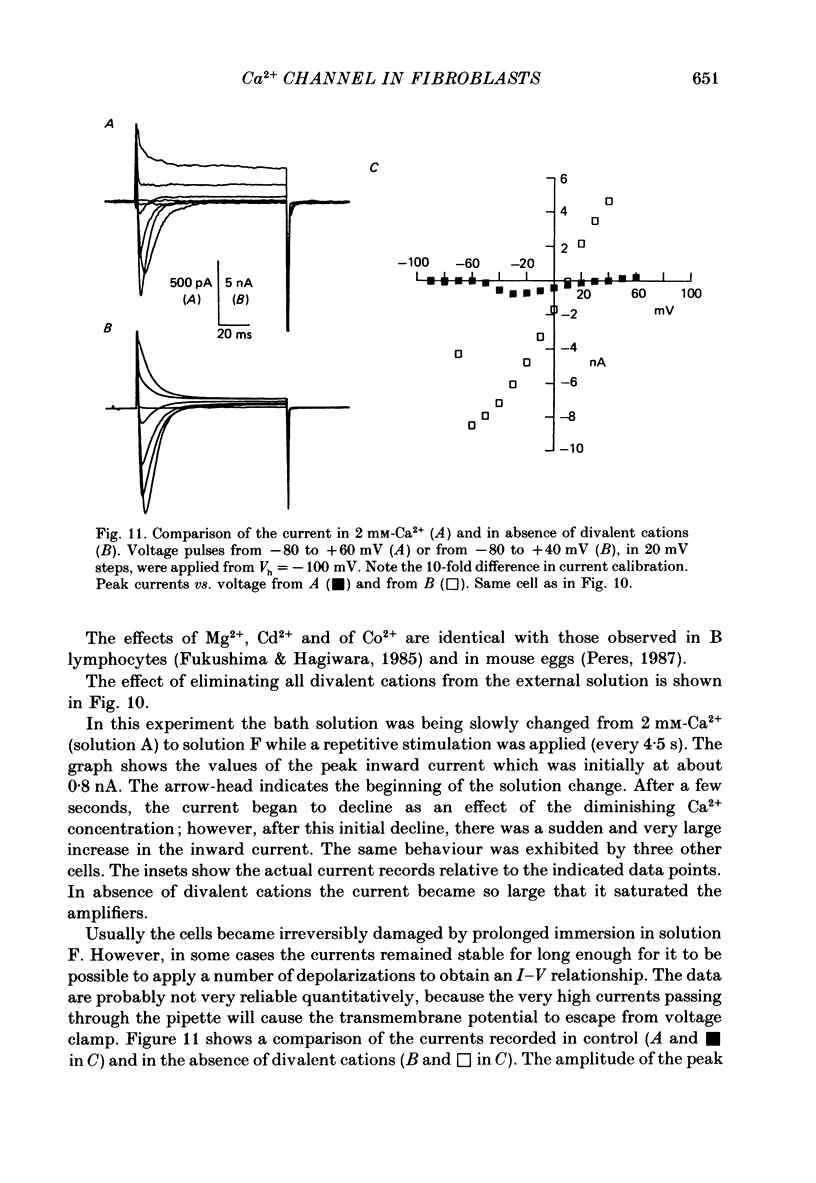
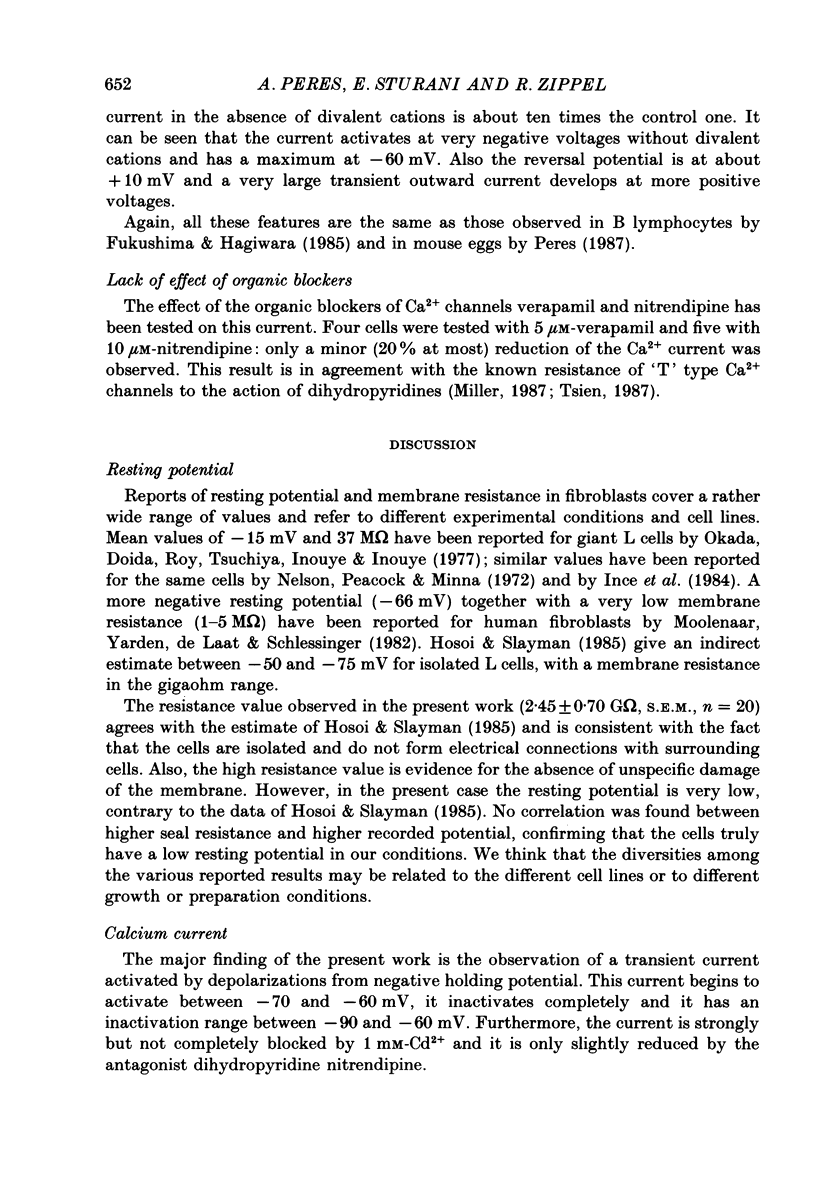
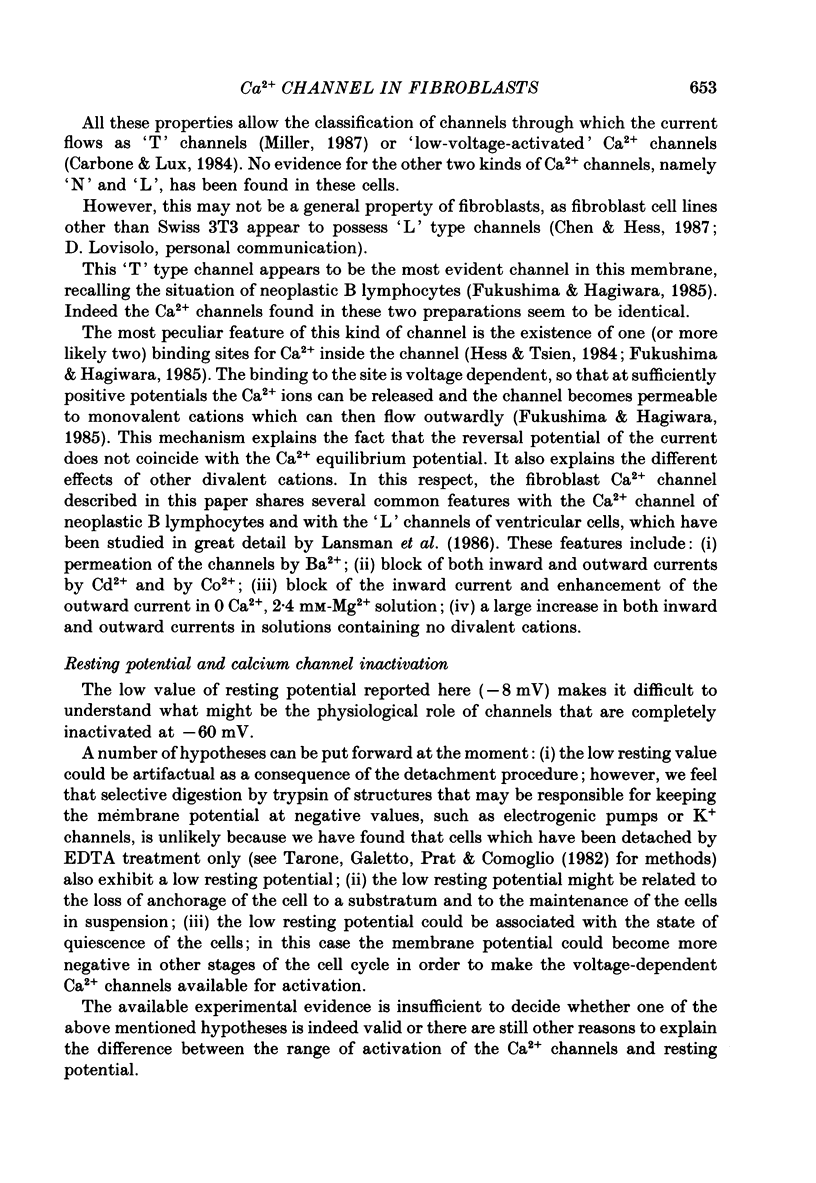
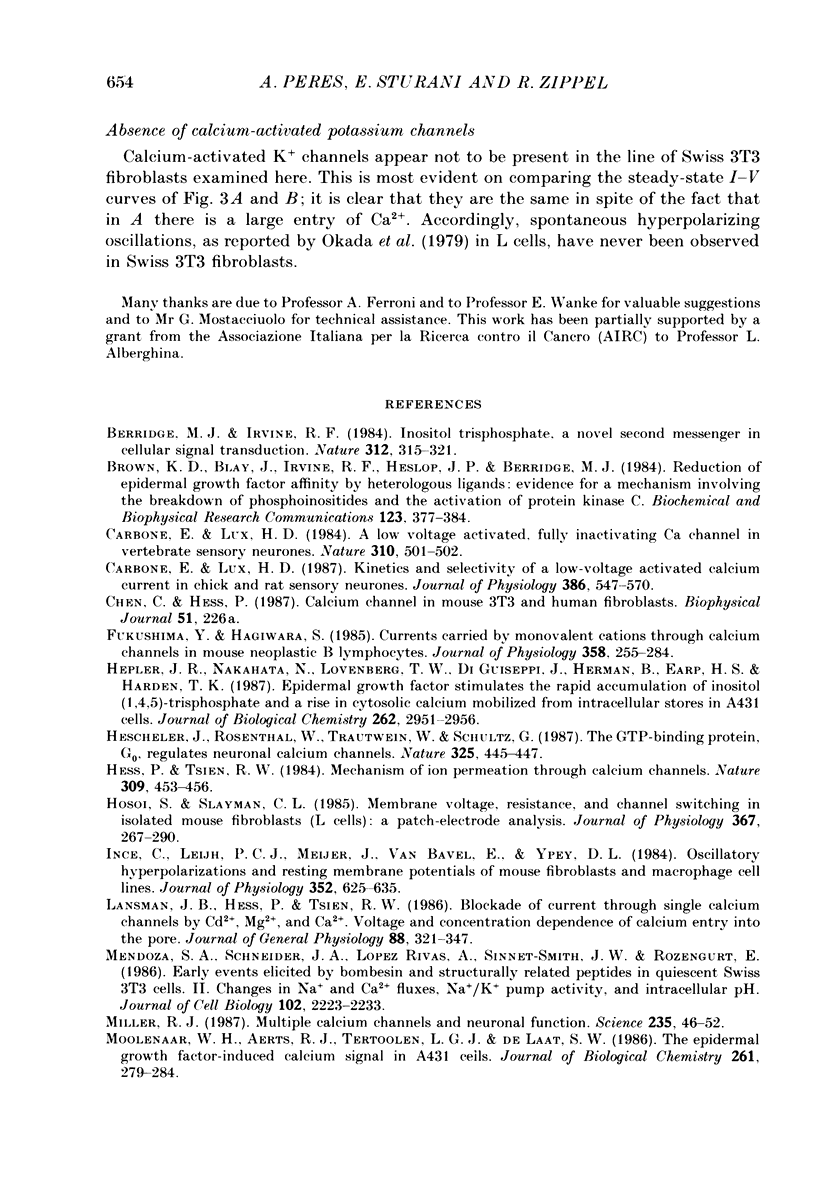
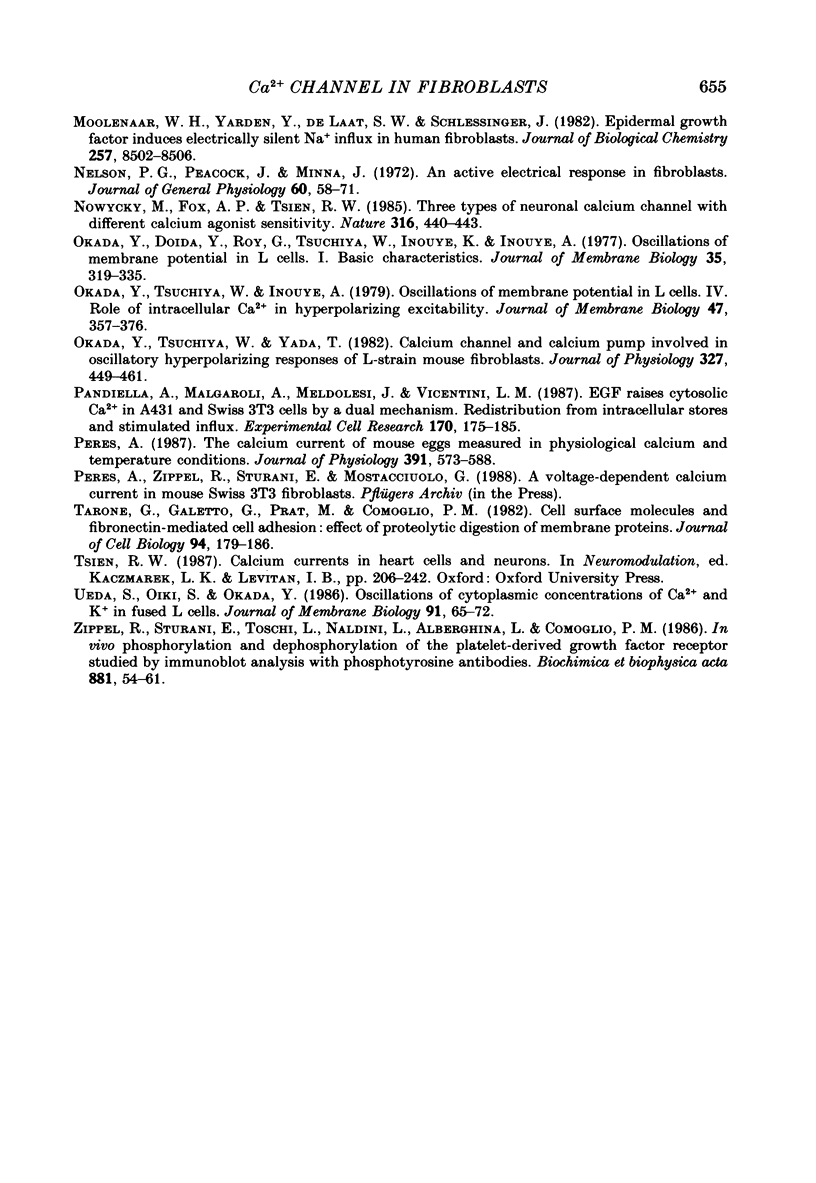
Selected References
These references are in PubMed. This may not be the complete list of references from this article.
- Berridge M. J., Irvine R. F. Inositol trisphosphate, a novel second messenger in cellular signal transduction. Nature. 1984 Nov 22;312(5992):315–321. doi: 10.1038/312315a0. [DOI] [PubMed] [Google Scholar]
- Brown K. D., Blay J., Irvine R. F., Heslop J. P., Berridge M. J. Reduction of epidermal growth factor receptor affinity by heterologous ligands: evidence for a mechanism involving the breakdown of phosphoinositides and the activation of protein kinase C. Biochem Biophys Res Commun. 1984 Aug 30;123(1):377–384. doi: 10.1016/0006-291x(84)90424-8. [DOI] [PubMed] [Google Scholar]
- Carbone E., Lux H. D. A low voltage-activated, fully inactivating Ca channel in vertebrate sensory neurones. Nature. 1984 Aug 9;310(5977):501–502. doi: 10.1038/310501a0. [DOI] [PubMed] [Google Scholar]
- Carbone E., Lux H. D. Kinetics and selectivity of a low-voltage-activated calcium current in chick and rat sensory neurones. J Physiol. 1987 May;386:547–570. doi: 10.1113/jphysiol.1987.sp016551. [DOI] [PMC free article] [PubMed] [Google Scholar]
- Fukushima Y., Hagiwara S. Currents carried by monovalent cations through calcium channels in mouse neoplastic B lymphocytes. J Physiol. 1985 Jan;358:255–284. doi: 10.1113/jphysiol.1985.sp015550. [DOI] [PMC free article] [PubMed] [Google Scholar]
- Hepler J. R., Nakahata N., Lovenberg T. W., DiGuiseppi J., Herman B., Earp H. S., Harden T. K. Epidermal growth factor stimulates the rapid accumulation of inositol (1,4,5)-trisphosphate and a rise in cytosolic calcium mobilized from intracellular stores in A431 cells. J Biol Chem. 1987 Mar 5;262(7):2951–2956. [PubMed] [Google Scholar]
- Hescheler J., Rosenthal W., Trautwein W., Schultz G. The GTP-binding protein, Go, regulates neuronal calcium channels. 1987 Jan 29-Feb 4Nature. 325(6103):445–447. doi: 10.1038/325445a0. [DOI] [PubMed] [Google Scholar]
- Hess P., Tsien R. W. Mechanism of ion permeation through calcium channels. 1984 May 31-Jun 6Nature. 309(5967):453–456. doi: 10.1038/309453a0. [DOI] [PubMed] [Google Scholar]
- Hosoi S., Slayman C. L. Membrane voltage, resistance, and channel switching in isolated mouse fibroblasts (L cells): a patch-electrode analysis. J Physiol. 1985 Oct;367:267–290. doi: 10.1113/jphysiol.1985.sp015824. [DOI] [PMC free article] [PubMed] [Google Scholar]
- Ince C., Leijh P. C., Meijer J., Van Bavel E., Ypey D. L. Oscillatory hyperpolarizations and resting membrane potentials of mouse fibroblast and macrophage cell lines. J Physiol. 1984 Jul;352:625–635. doi: 10.1113/jphysiol.1984.sp015313. [DOI] [PMC free article] [PubMed] [Google Scholar]
- Lansman J. B., Hess P., Tsien R. W. Blockade of current through single calcium channels by Cd2+, Mg2+, and Ca2+. Voltage and concentration dependence of calcium entry into the pore. J Gen Physiol. 1986 Sep;88(3):321–347. doi: 10.1085/jgp.88.3.321. [DOI] [PMC free article] [PubMed] [Google Scholar]
- Mendoza S. A., Schneider J. A., Lopez-Rivas A., Sinnett-Smith J. W., Rozengurt E. Early events elicited by bombesin and structurally related peptides in quiescent Swiss 3T3 cells. II. Changes in Na+ and Ca2+ fluxes, Na+/K+ pump activity, and intracellular pH. J Cell Biol. 1986 Jun;102(6):2223–2233. doi: 10.1083/jcb.102.6.2223. [DOI] [PMC free article] [PubMed] [Google Scholar]
- Miller R. J. Multiple calcium channels and neuronal function. Science. 1987 Jan 2;235(4784):46–52. doi: 10.1126/science.2432656. [DOI] [PubMed] [Google Scholar]
- Moolenaar W. H., Aerts R. J., Tertoolen L. G., de Laat S. W. The epidermal growth factor-induced calcium signal in A431 cells. J Biol Chem. 1986 Jan 5;261(1):279–284. [PubMed] [Google Scholar]
- Moolenaar W. H., Yarden Y., de Laat S. W., Schlessinger J. Epidermal growth factor induces electrically silent Na+ influx in human fibroblasts. J Biol Chem. 1982 Jul 25;257(14):8502–8506. [PubMed] [Google Scholar]
- Nelson P. G., Peacock J., Minna J. An active electrical response in fibroblasts. J Gen Physiol. 1972 Jul;60(1):58–71. doi: 10.1085/jgp.60.1.58. [DOI] [PMC free article] [PubMed] [Google Scholar]
- Nowycky M. C., Fox A. P., Tsien R. W. Three types of neuronal calcium channel with different calcium agonist sensitivity. Nature. 1985 Aug 1;316(6027):440–443. doi: 10.1038/316440a0. [DOI] [PubMed] [Google Scholar]
- Okada Y., Doida Y., Roy G., Tsuchiya W., Inouye K., Inouye A. Oscillations of membrane potential in L cells. I. Basic characteristics. J Membr Biol. 1977 Aug 4;35(4):319–335. doi: 10.1007/BF01869957. [DOI] [PubMed] [Google Scholar]
- Okada Y., Tsuchiya W., Inouye A. Oscillations of membrane potential in L cells. IV. Role of intracellular Ca2+ in hyperpolarizing excitability. J Membr Biol. 1979 Jun 7;47(4):357–376. doi: 10.1007/BF01869744. [DOI] [PubMed] [Google Scholar]
- Okada Y., Tsuchiya W., Yada T. Calcium channel and calcium pump involved in oscillatory hyperpolarizing responses of L-strain mouse fibroblasts. J Physiol. 1982 Jun;327:449–461. doi: 10.1113/jphysiol.1982.sp014242. [DOI] [PMC free article] [PubMed] [Google Scholar]
- Pandiella A., Malgaroli A., Meldolesi J., Vicentini L. M. EGF raises cytosolic Ca2+ in A431 and Swiss 3T3 cells by a dual mechanism. Redistribution from intracellular stores and stimulated influx. Exp Cell Res. 1987 May;170(1):175–185. doi: 10.1016/0014-4827(87)90127-3. [DOI] [PubMed] [Google Scholar]
- Peres A. The calcium current of mouse egg measured in physiological calcium and temperature conditions. J Physiol. 1987 Oct;391:573–588. doi: 10.1113/jphysiol.1987.sp016757. [DOI] [PMC free article] [PubMed] [Google Scholar]
- Tarone G., Galetto G., Prat M., Comoglio P. M. Cell surface molecules and fibronectin-mediated cell adhesion: effect of proteolytic digestion of membrane proteins. J Cell Biol. 1982 Jul;94(1):179–186. doi: 10.1083/jcb.94.1.179. [DOI] [PMC free article] [PubMed] [Google Scholar]
- Ueda S., Oiki S., Okada Y. Oscillations of cytoplasmic concentrations of Ca2+ and K+ in fused L cells. J Membr Biol. 1986;91(1):65–72. doi: 10.1007/BF01870215. [DOI] [PubMed] [Google Scholar]
- Zippel R., Sturani E., Toschi L., Naldini L., Alberghina L., Comoglio P. M. In vivo phosphorylation and dephosphorylation of the platelet-derived growth factor receptor studied by immunoblot analysis with phosphotyrosine antibodies. Biochim Biophys Acta. 1986 Mar 19;881(1):54–61. doi: 10.1016/0304-4165(86)90096-6. [DOI] [PubMed] [Google Scholar]