Abstract
1. Enzymatically isolated, cultured myocytes from hearts of adult guinea-pigs were voltage clamped with a whole-cell patch-clamp technique. The pipette-filling solution for internal dialysis contained 65 mM-citrate and 50 microM-EGTA as Ca2+-chelating agents and 20 mM-Na+. Potassium channel currents were blocked by replacing this ion on both sides of the membrane by Cs+. 2. In the above conditions myocytes develop spontaneous transient inward currents (Iti) at constant negative membrane holding potentials. At a given membrane potential Iti can be recorded with constant amplitude and frequency for periods of up to ca. 40 min. A membrane current with similar properties can be evoked by superfusion of the cell with caffeine-containing (5-10 mM) solution. 3. Depolarization results in a reduction of Iti amplitude and a prolongation of its duration. After a step change of the membrane potential to ca. -10 mV or a less-negative level only one inward current change is observed. Thereafter the membrane current remains inward with regard to the instantaneous current at this membrane potential. Complete relaxation of Iti then is only observed after repolarization to a more-negative membrane potential. 4. The current change caused by sarcoplasmic Ca2+ release is inward in a range of membrane potentials between -90 and +75 mV. A reversal of Iti was never detected. 5. Both the instantaneous current-voltage (I-V) relation and voltage dependence of peak Iti display distinct outward rectification. Both I-V relations can be described by a formalism suggested for a membrane current caused by electrogenic Na+-Ca2+ exchange (INa, Ca) assuming a 3:1 stoichiometry and a single energy barrier in the electric field of the membrane. 6. An increase of the time integral of Iti at the holding potential is observed after depolarizations to positive membrane potentials, where the outward-rectifying current component is prominent. This supports the view that the outward current represents INa, Ca in the 'reverse mode', carrying Ca2+ ions into the cell. 7. After prolonged cell dialysis a run-down of Iti is observed. Since strong depolarizations in this condition still can cause inward currents upon repolarization, run-down is likely to reflect an impairment of sarcoplasmic reticulum function rather than an effect of cell dialysis on the exchanger. 8. We conclude that under the present conditions a membrane current is measured, which to a large extent determines the 'passive' I-V curve of the myocytes. This current is modified by a rise in Ca2+(i) following sarcoplasmic Ca2+ release.(ABSTRACT TRUNCATED AT 400 WORDS)
Full text
PDF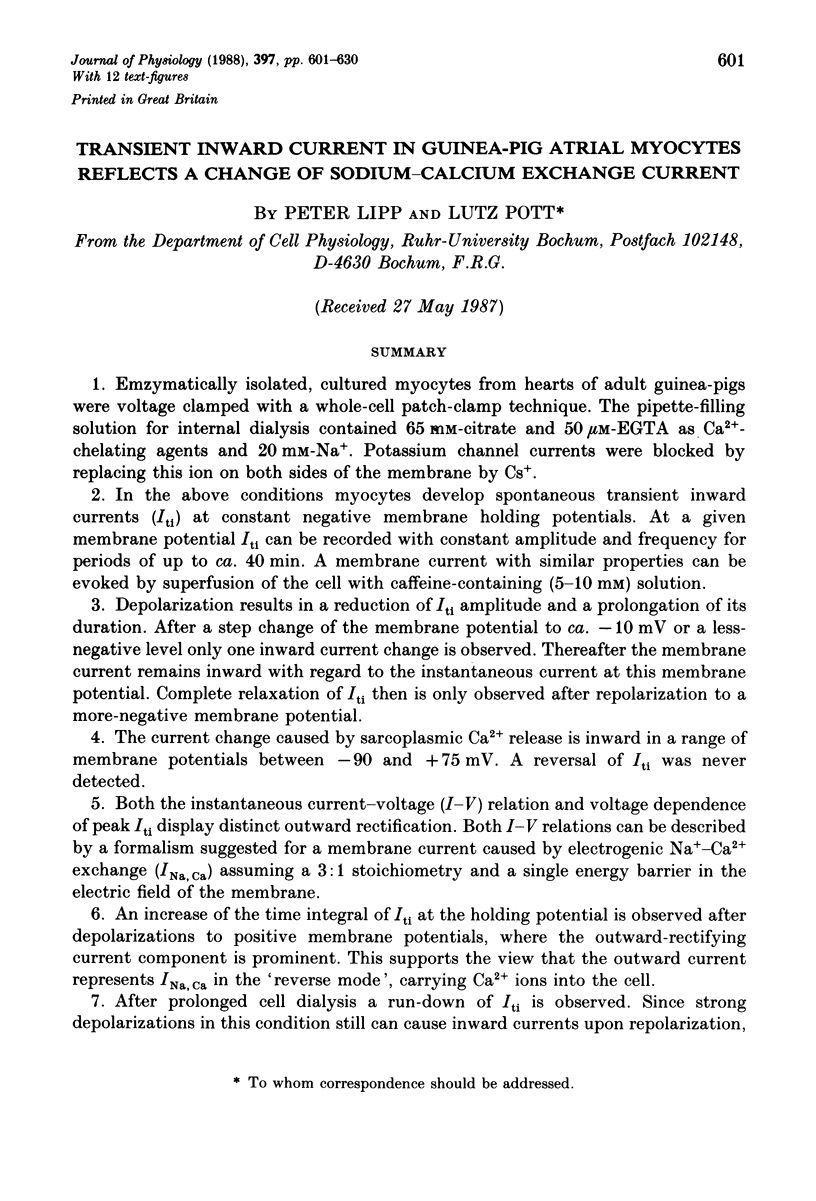
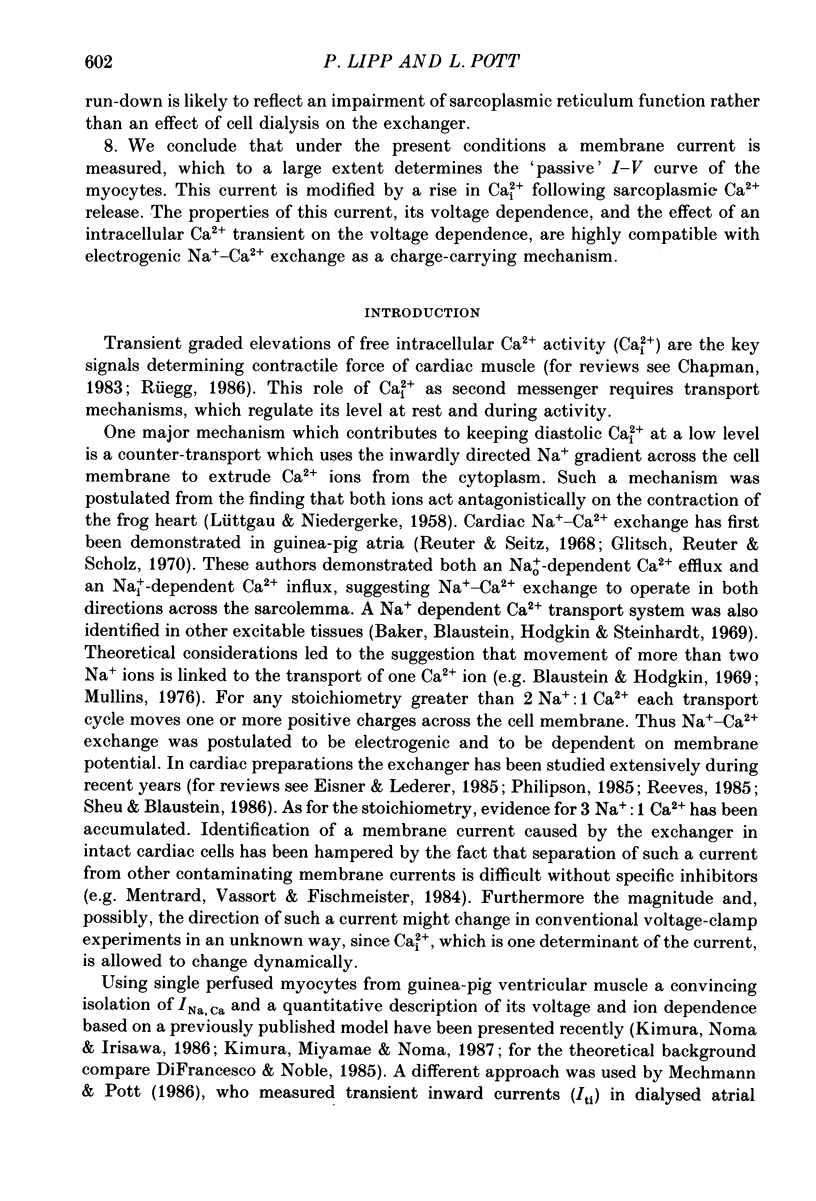
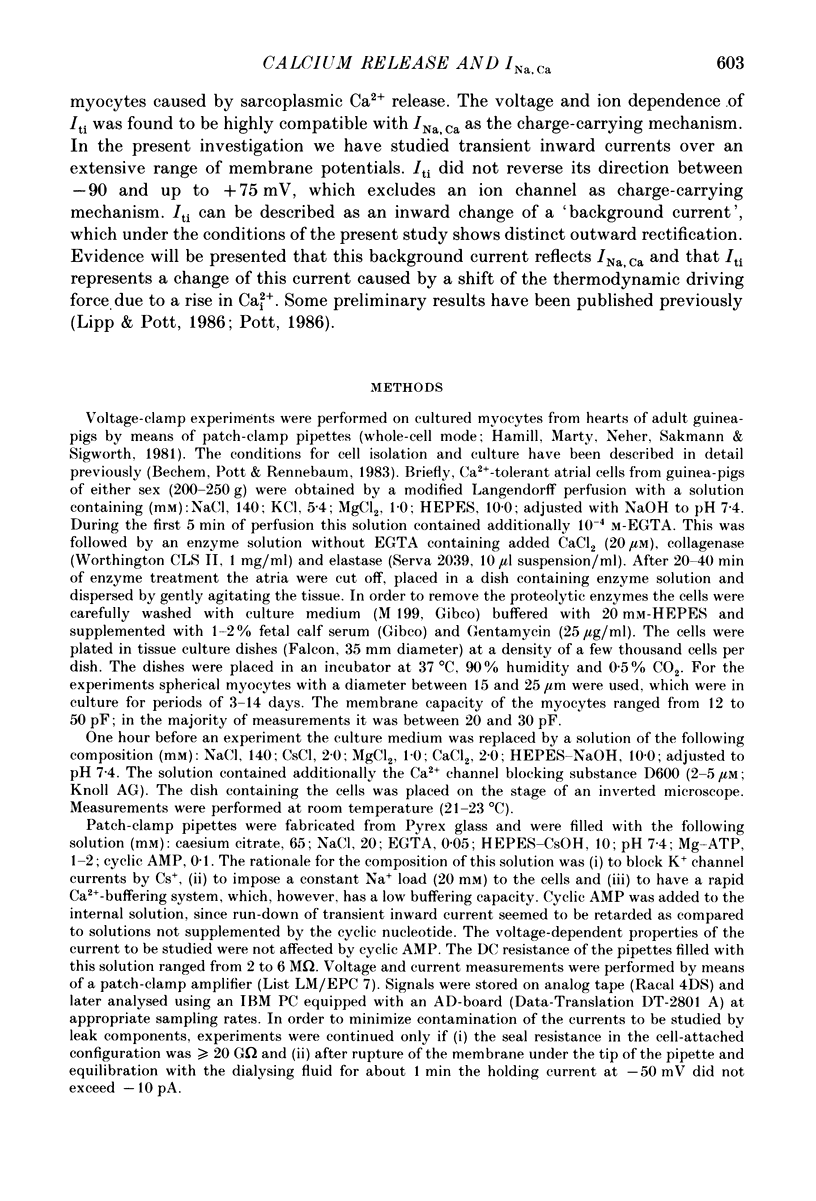
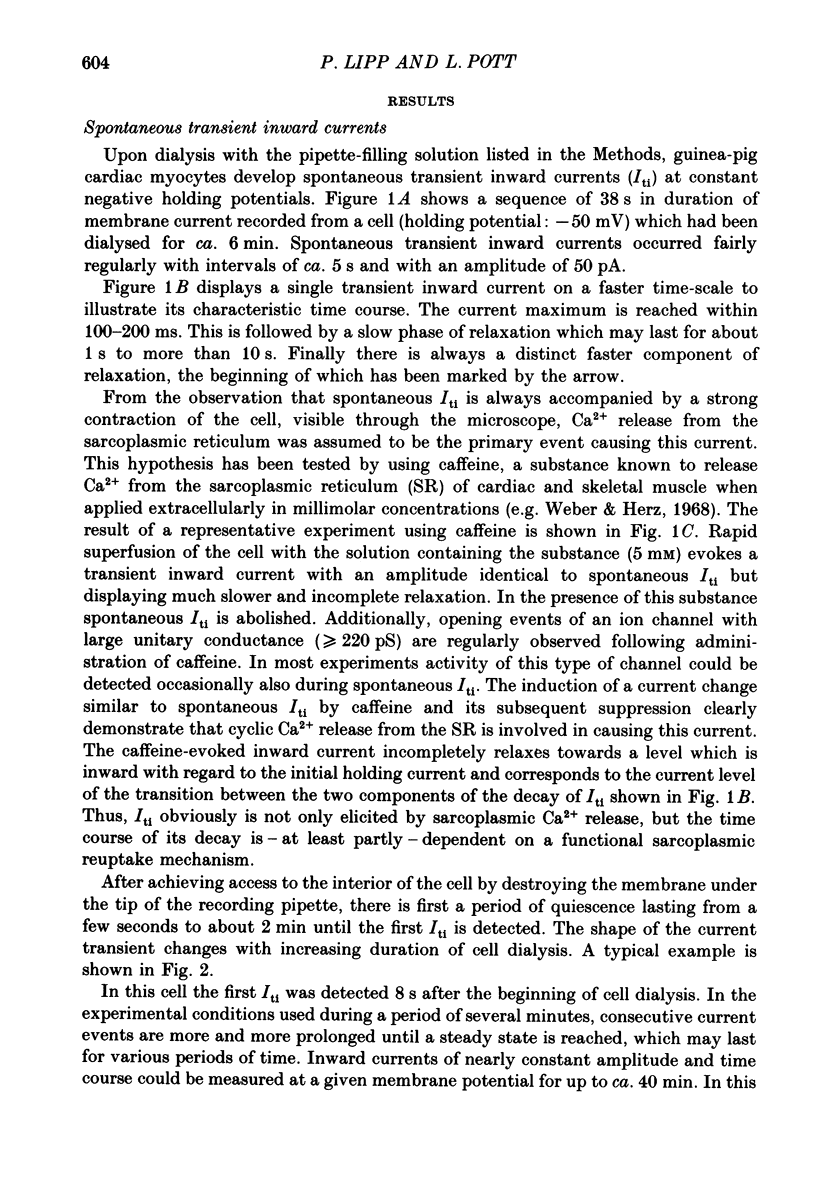
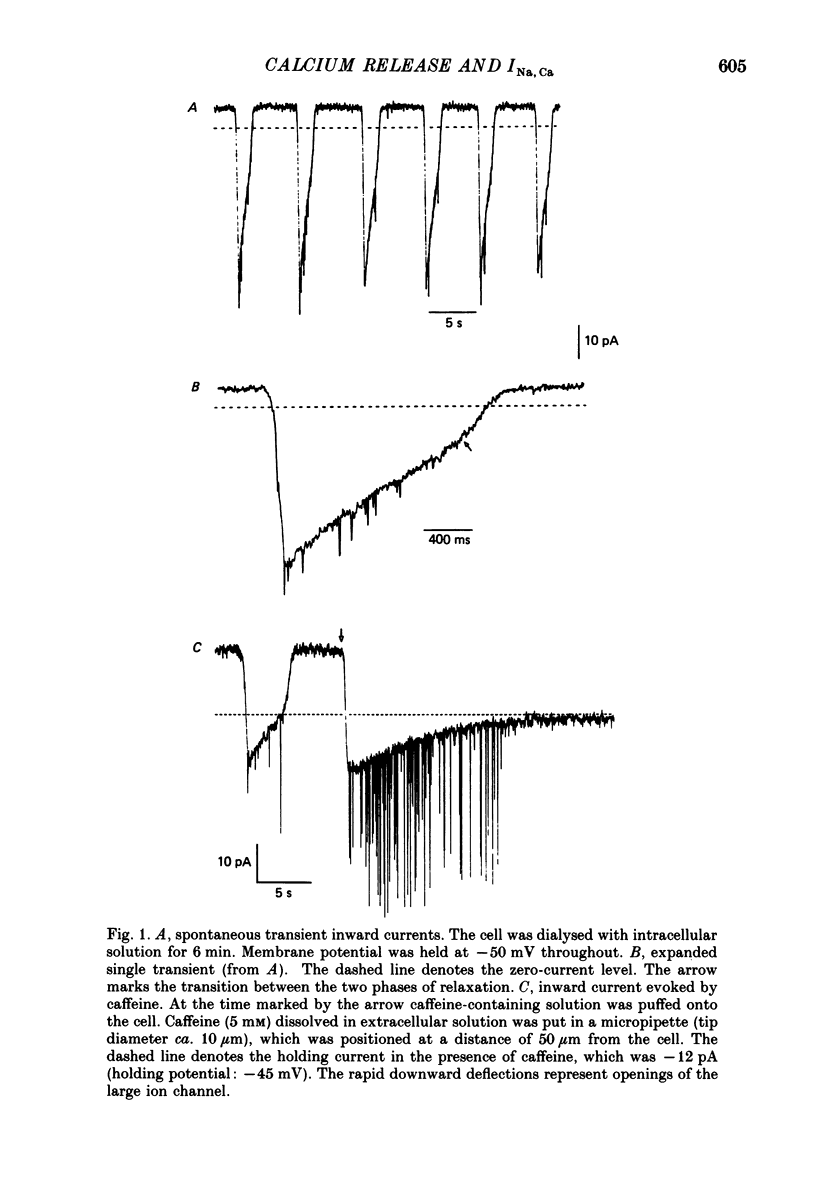
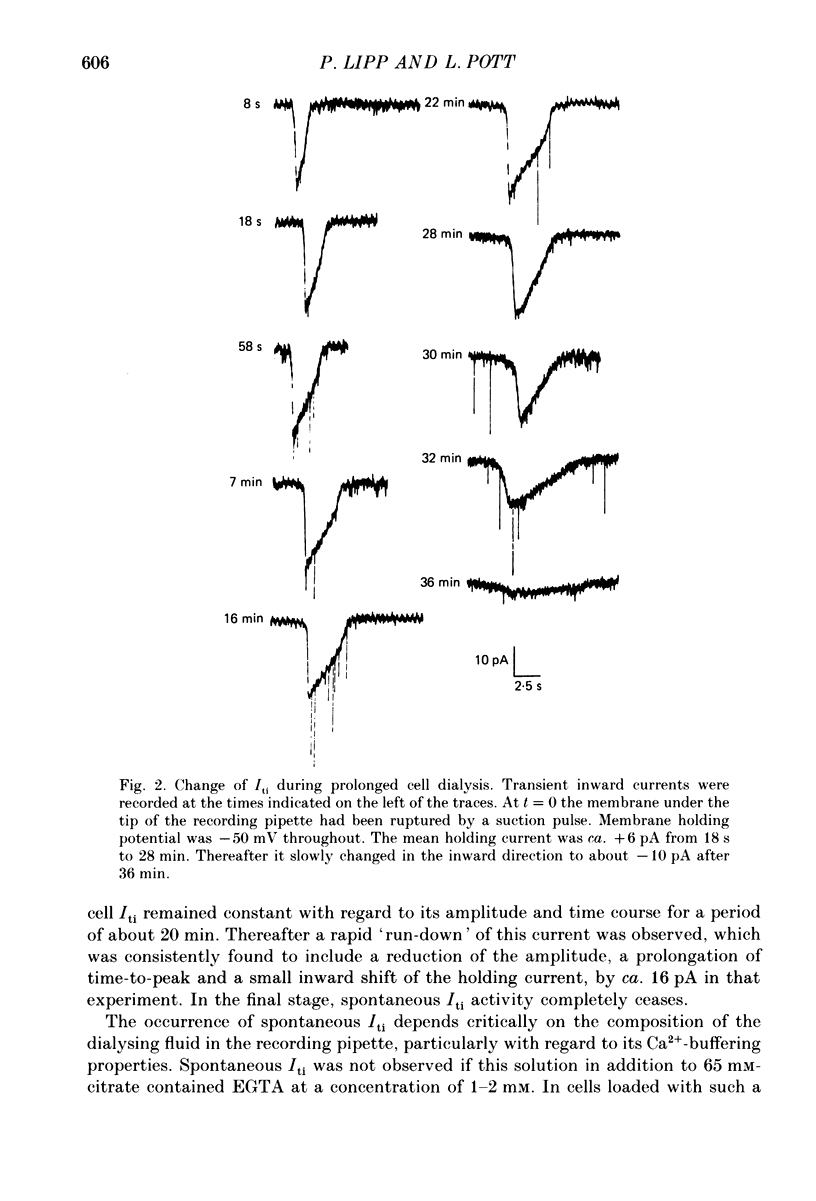
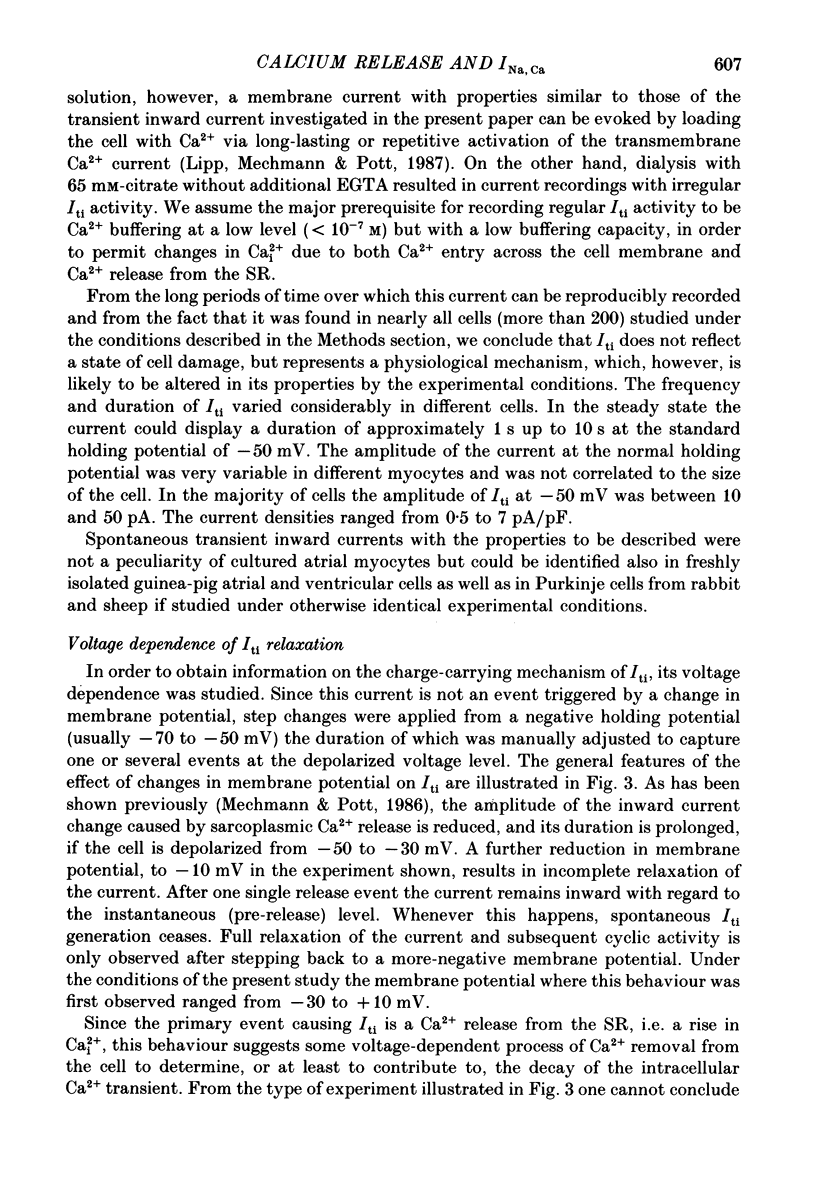
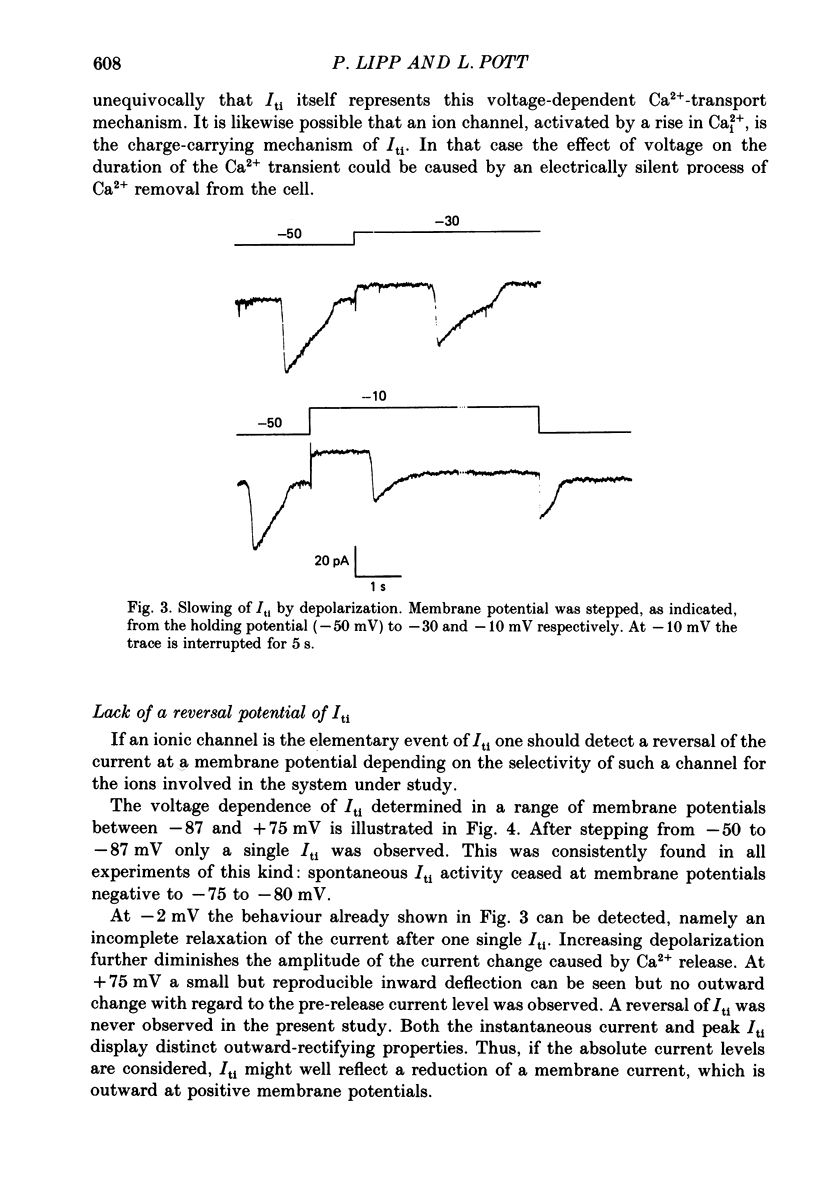
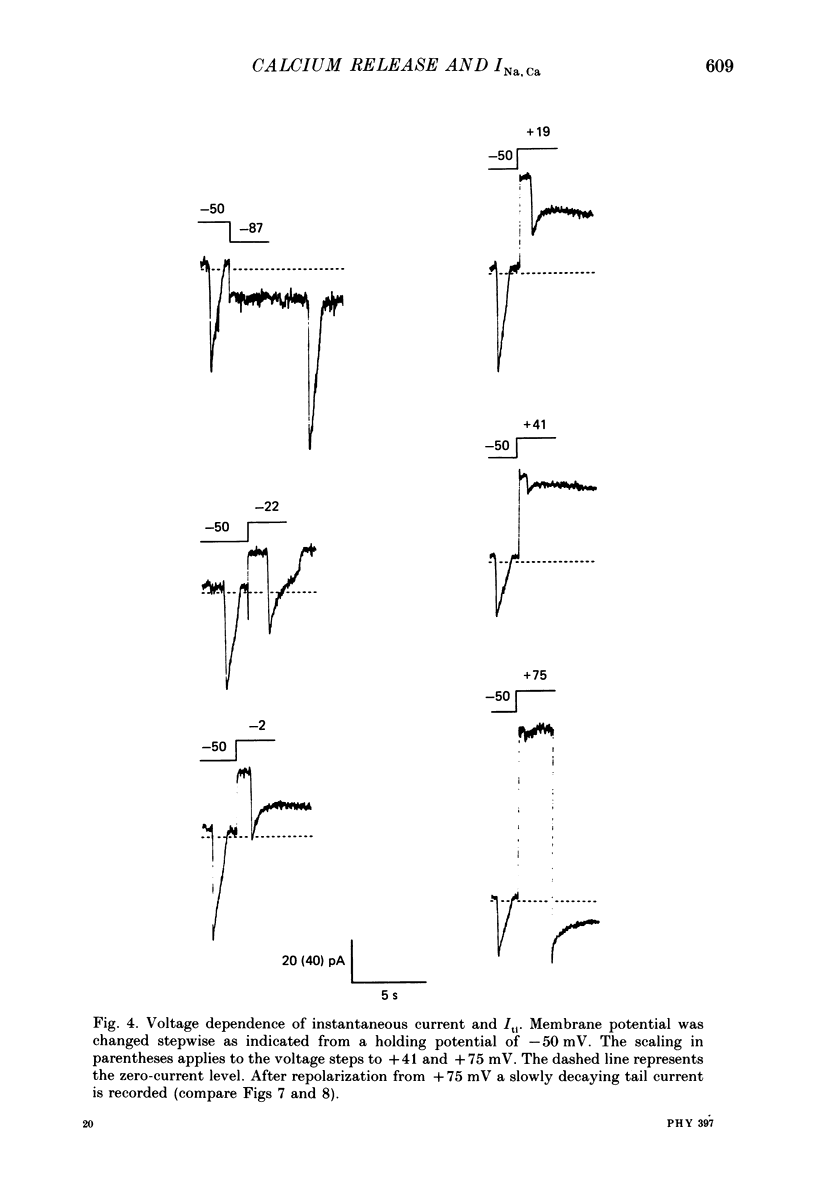
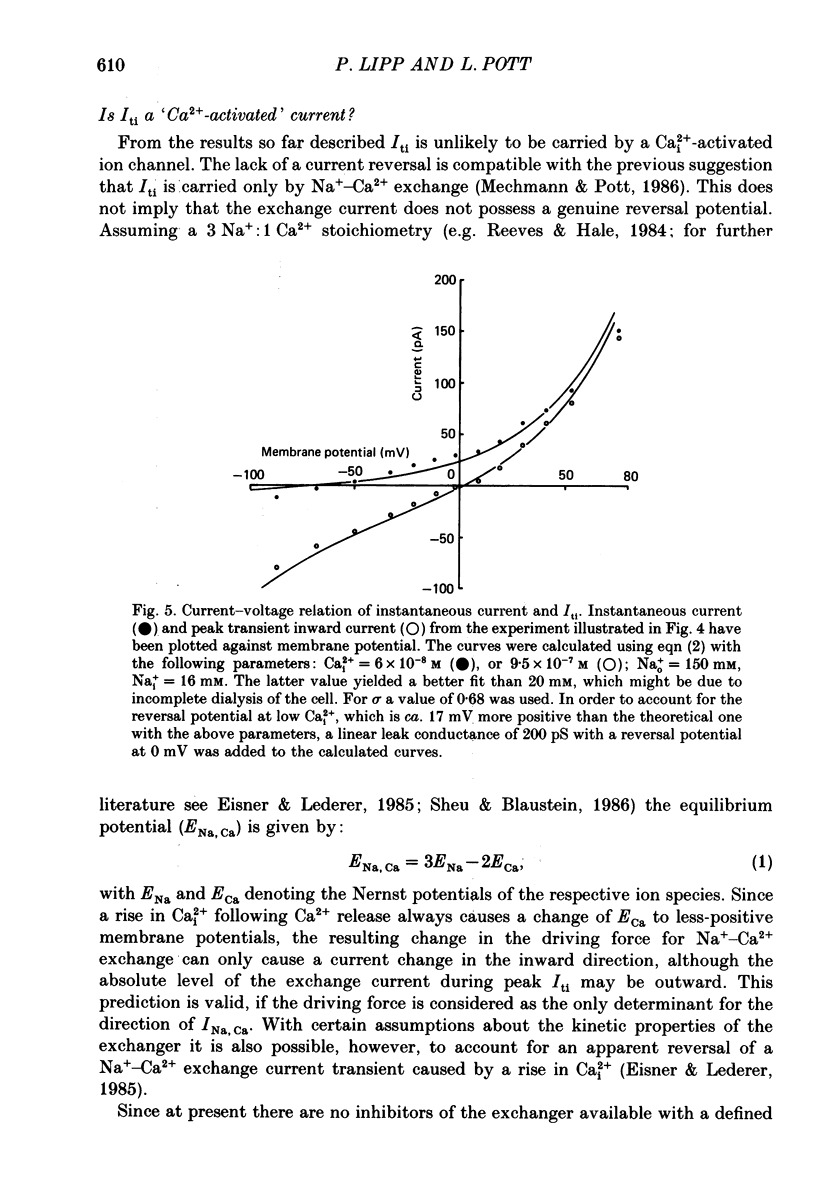
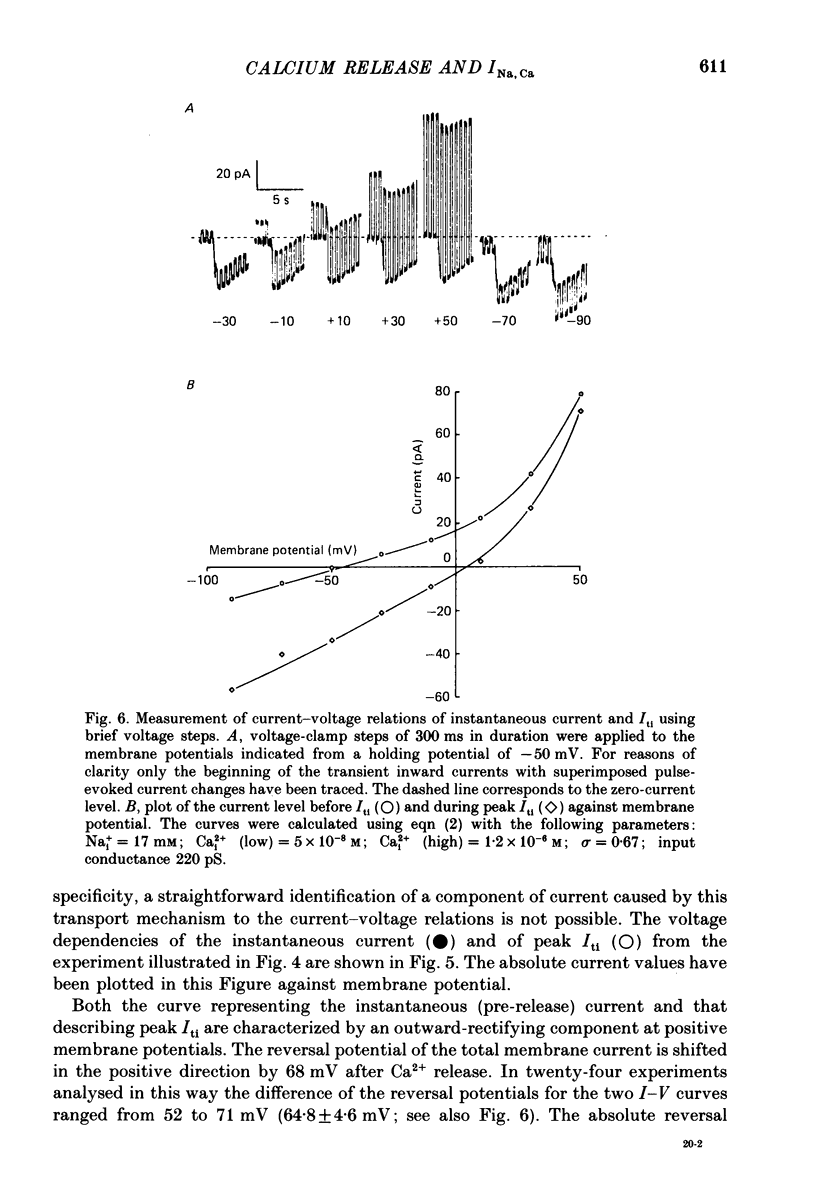
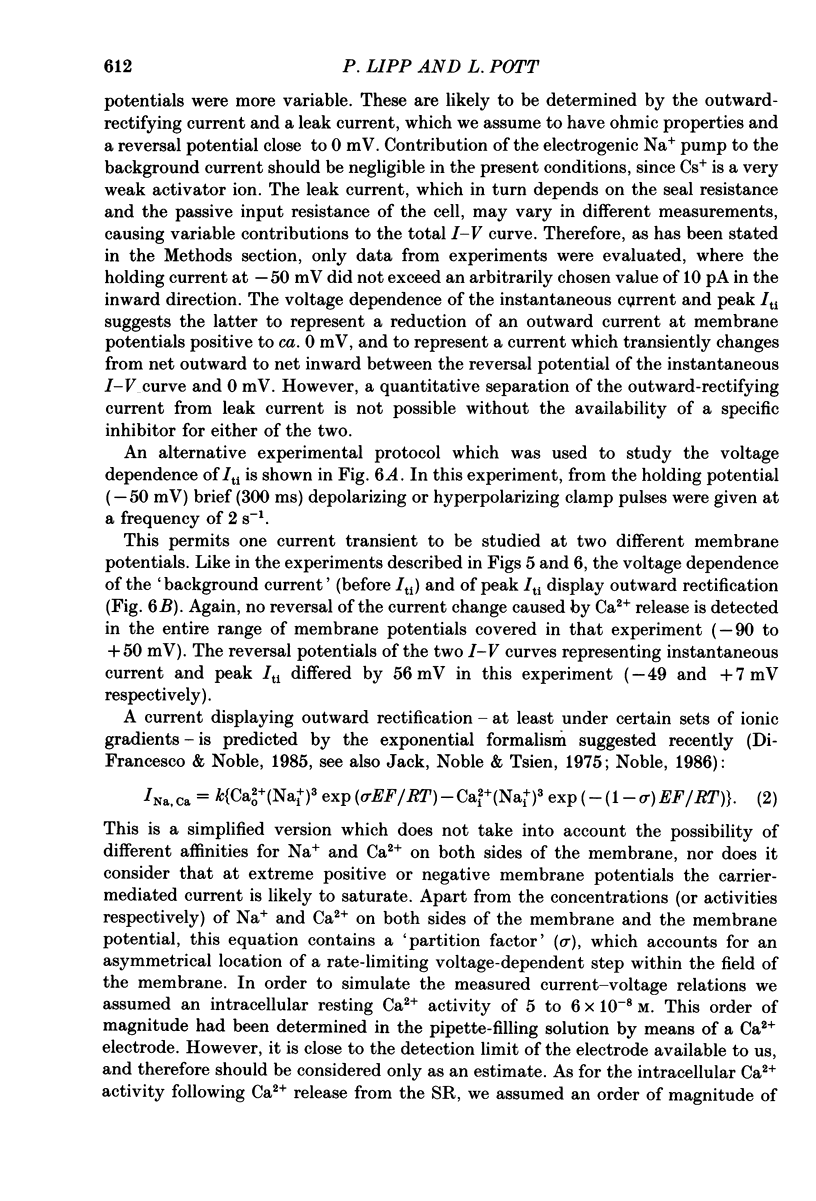
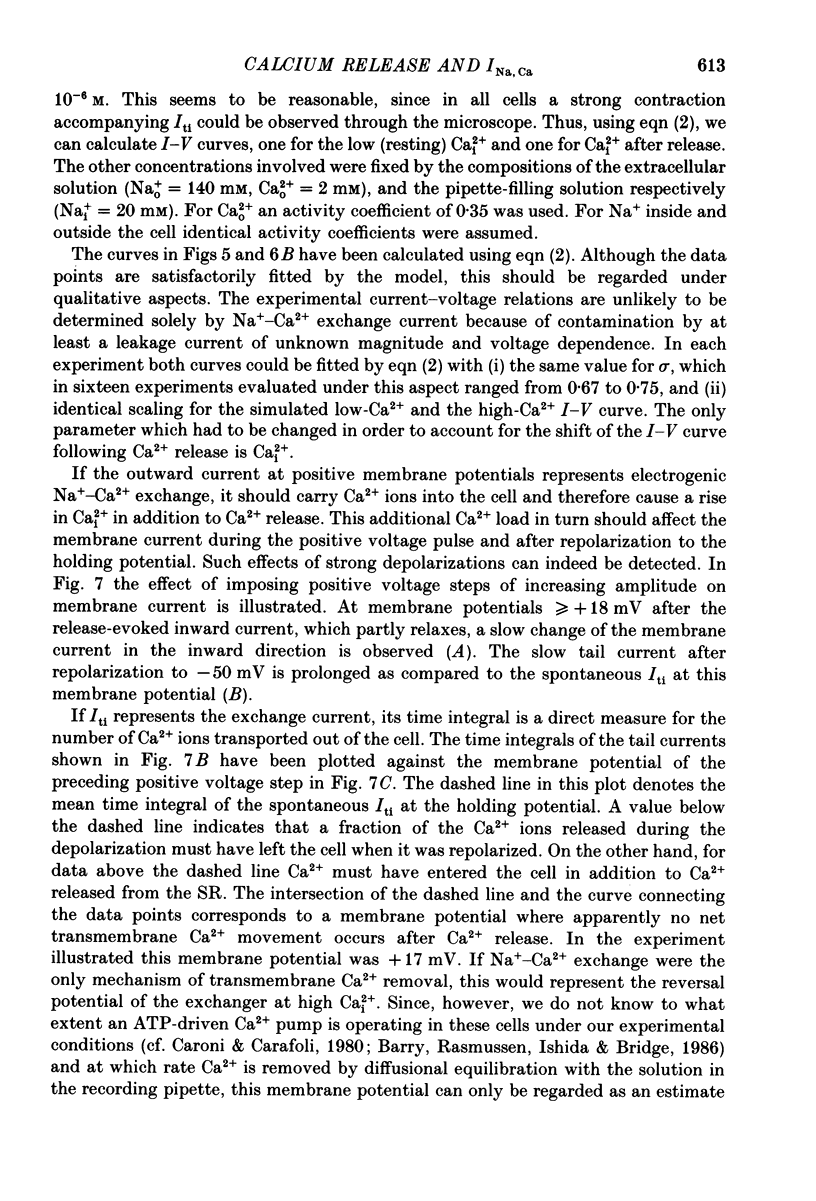
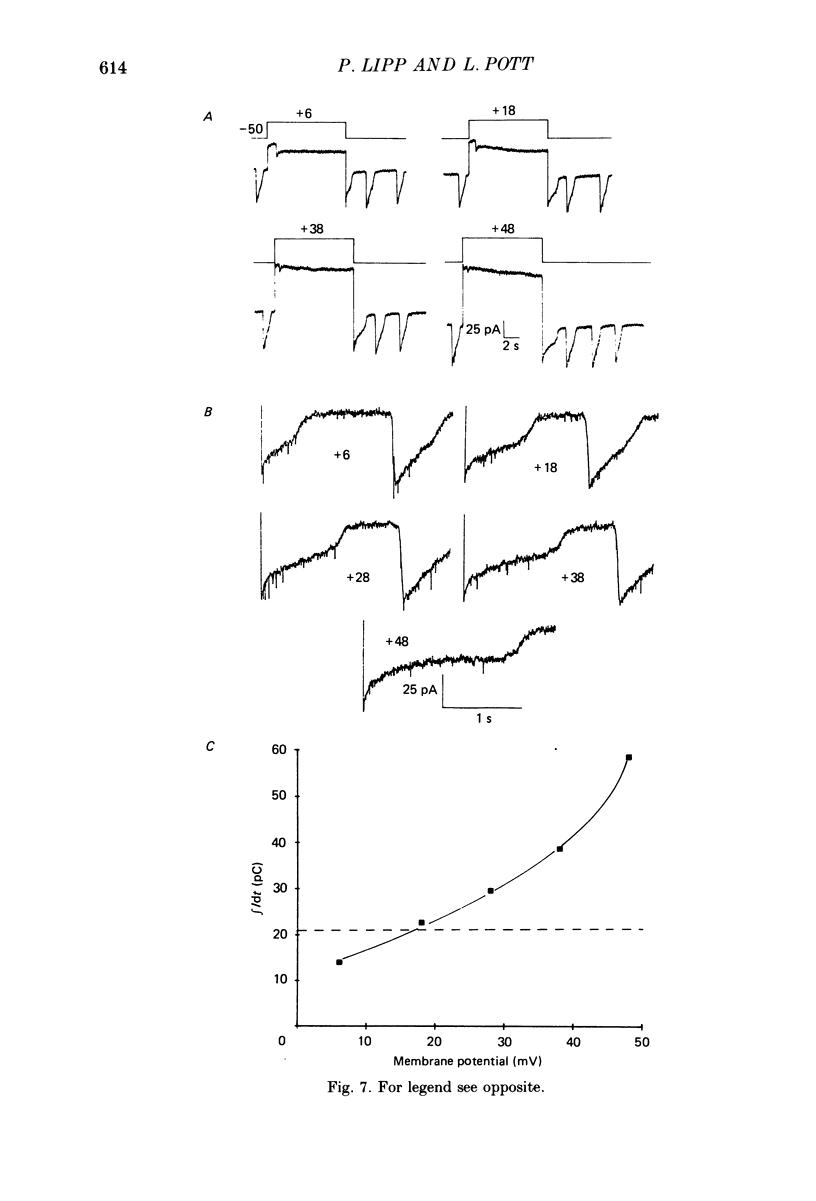
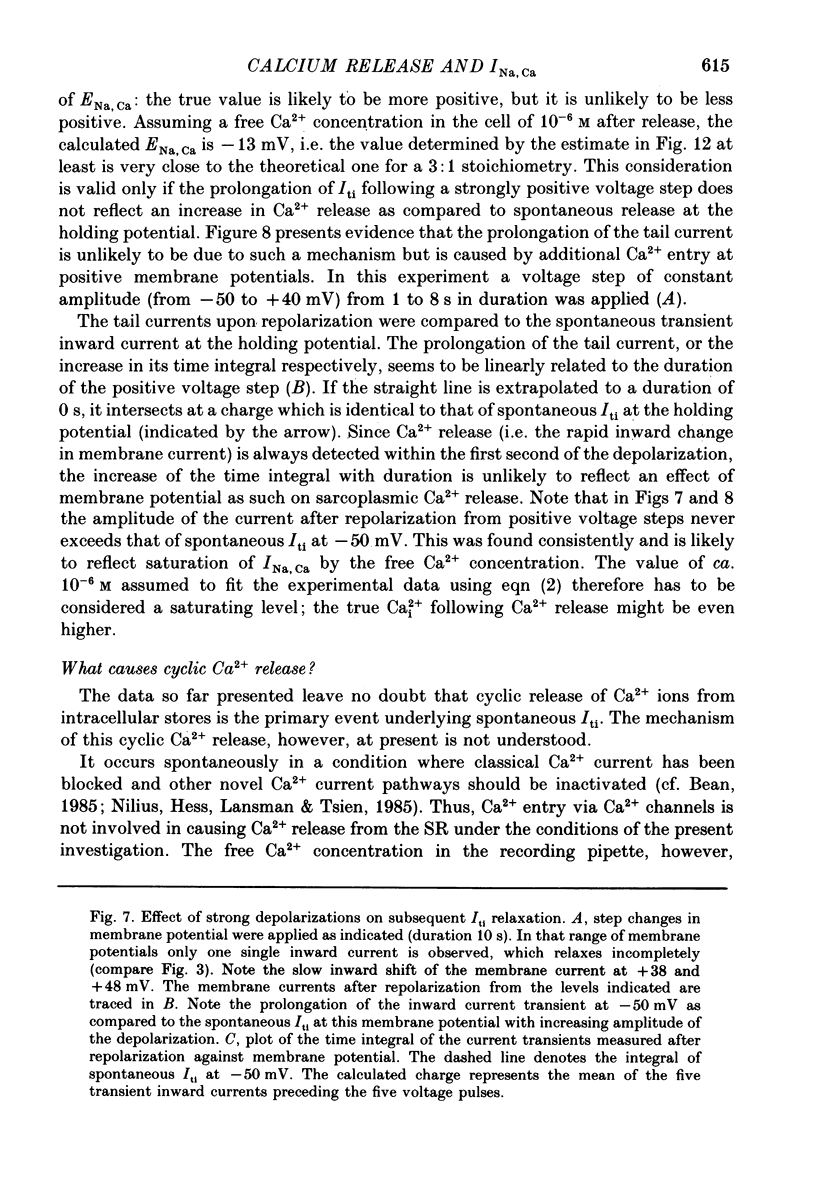
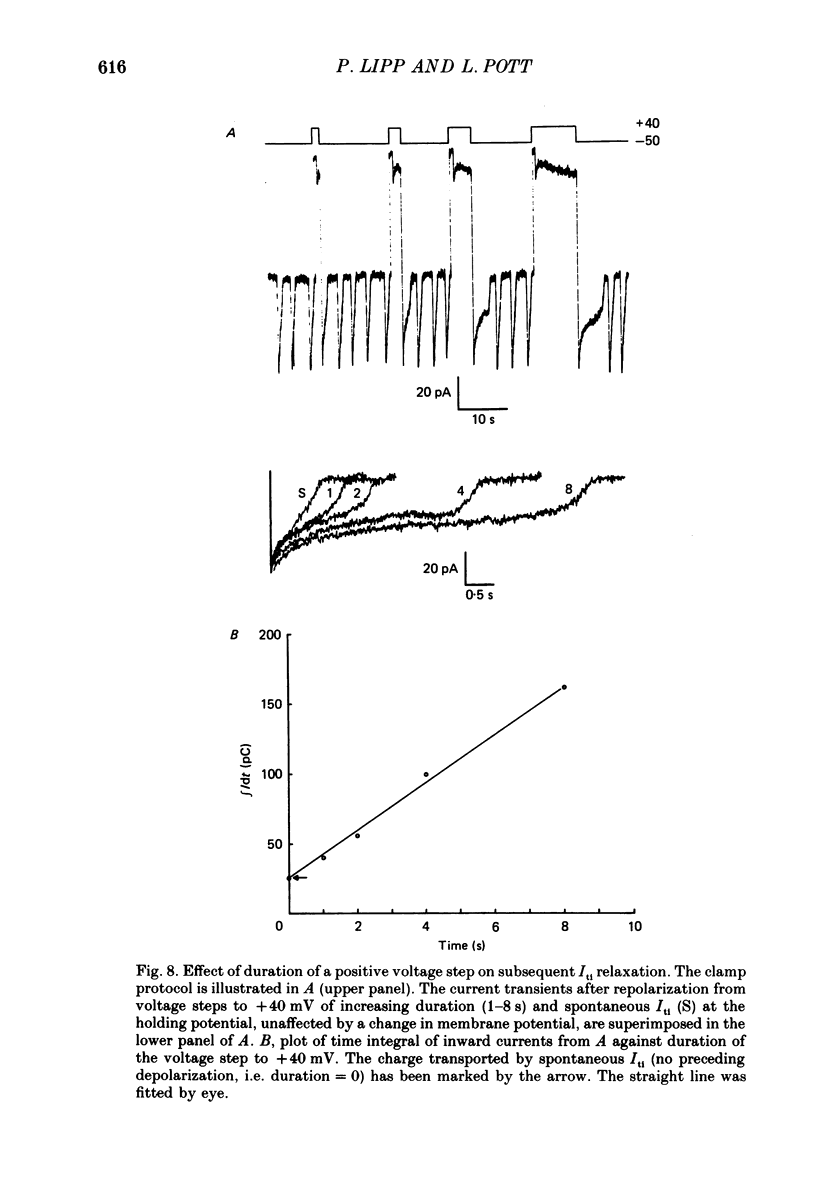
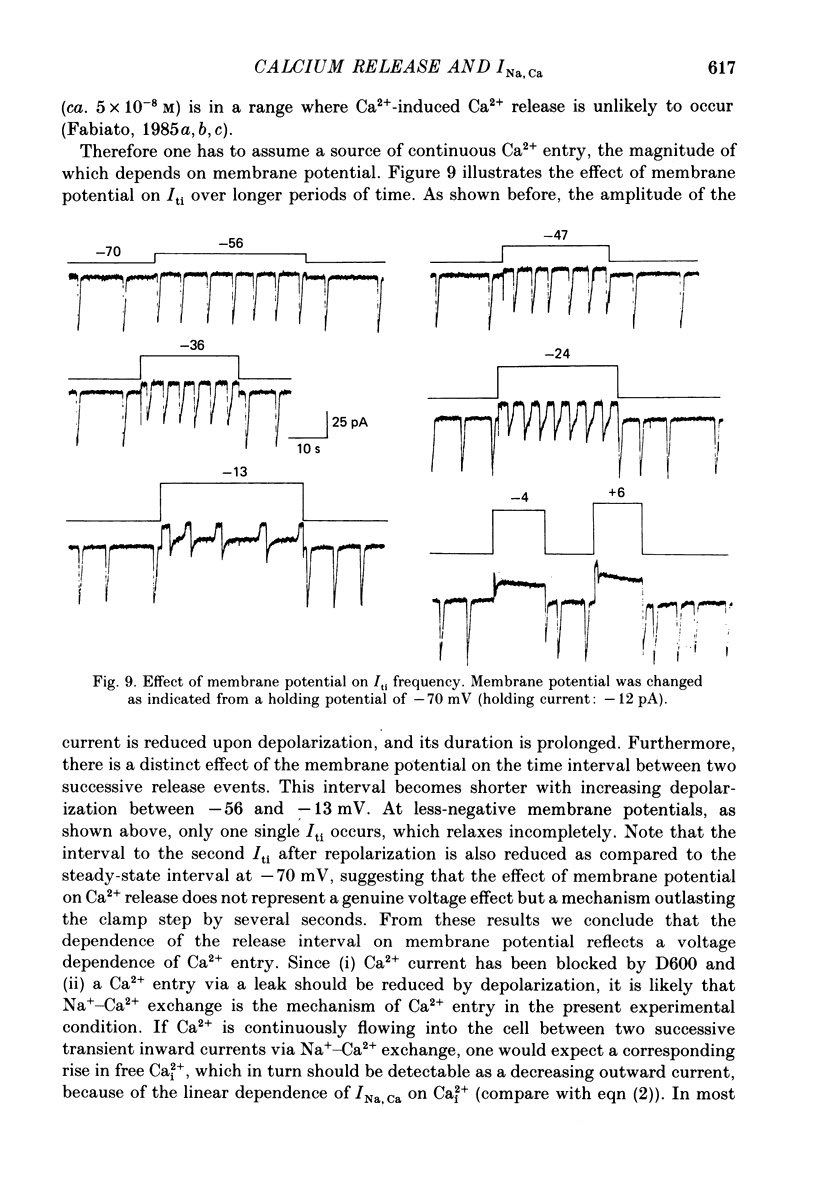
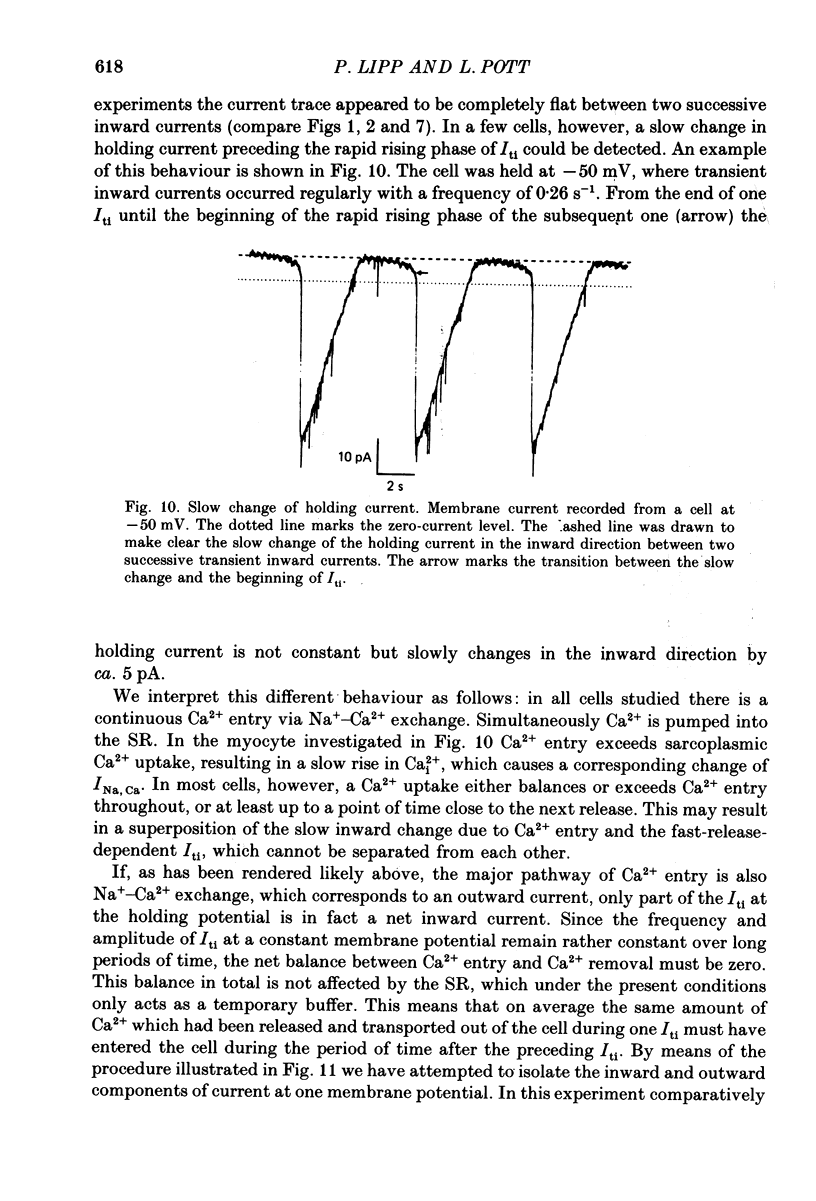
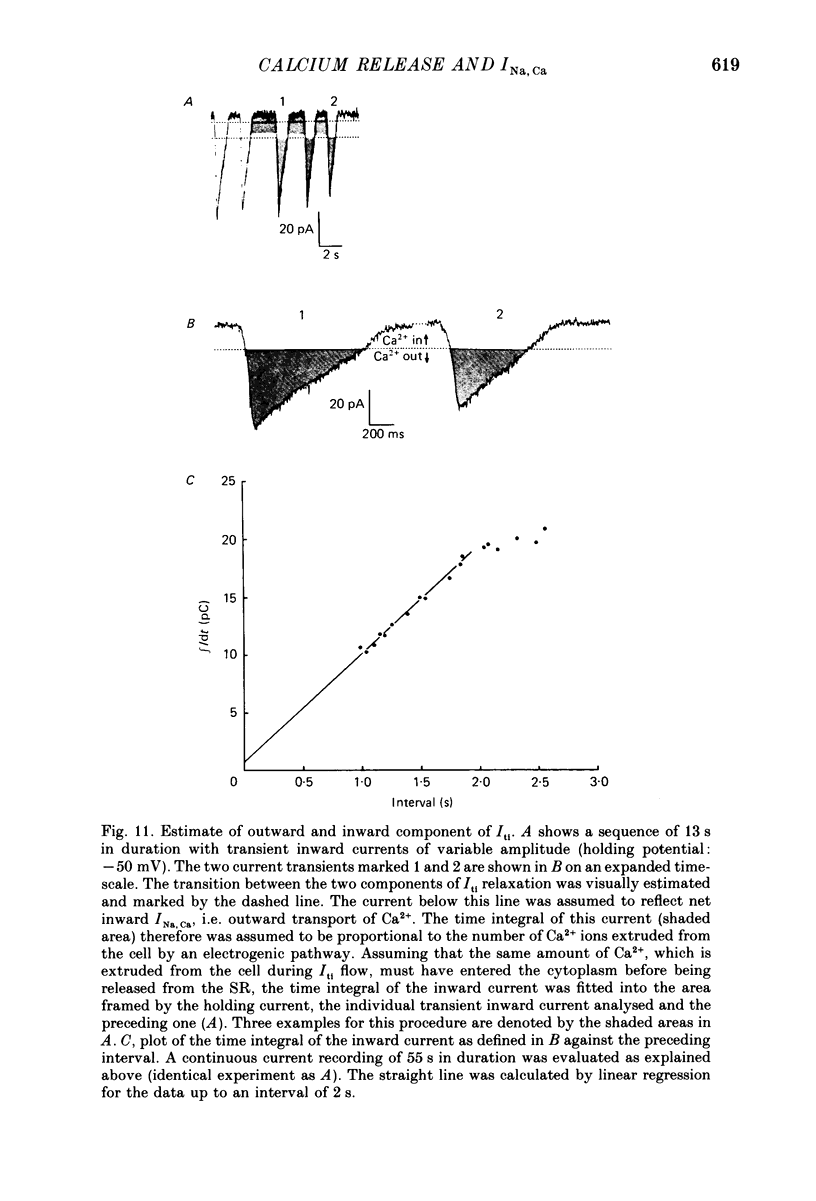
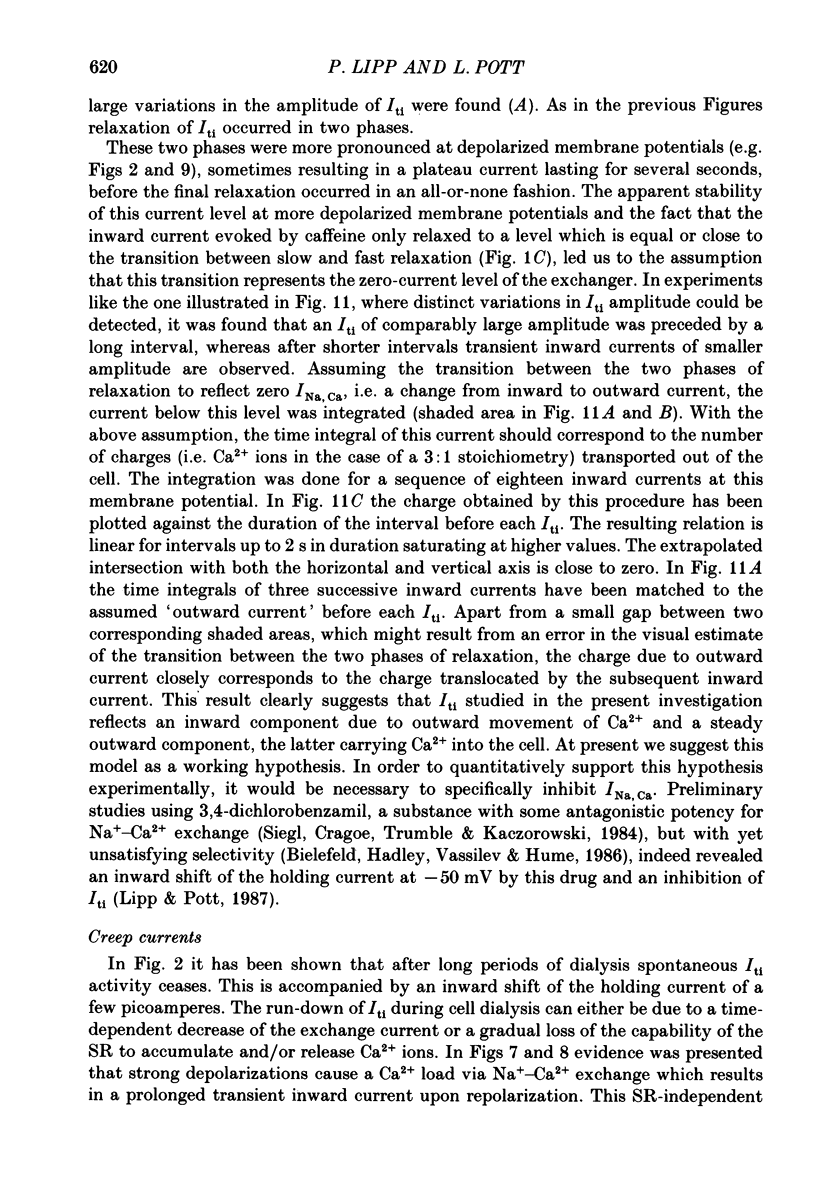
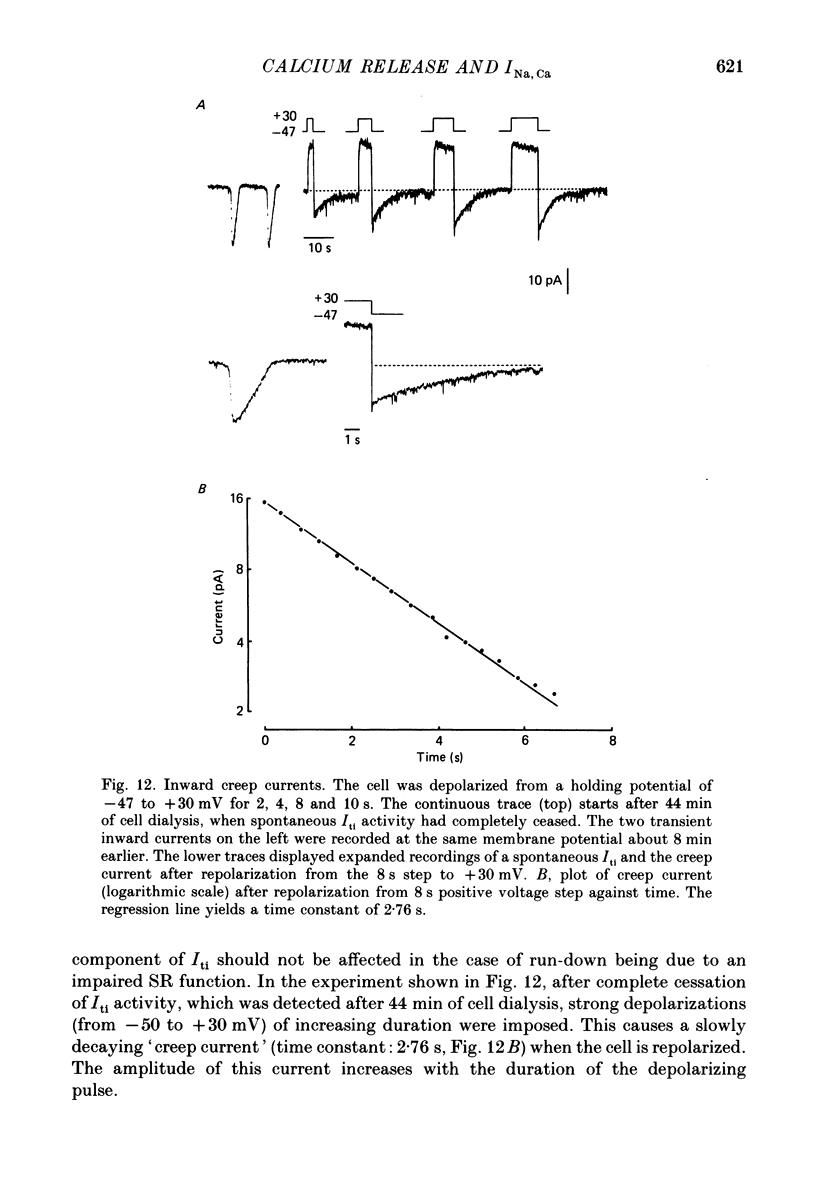
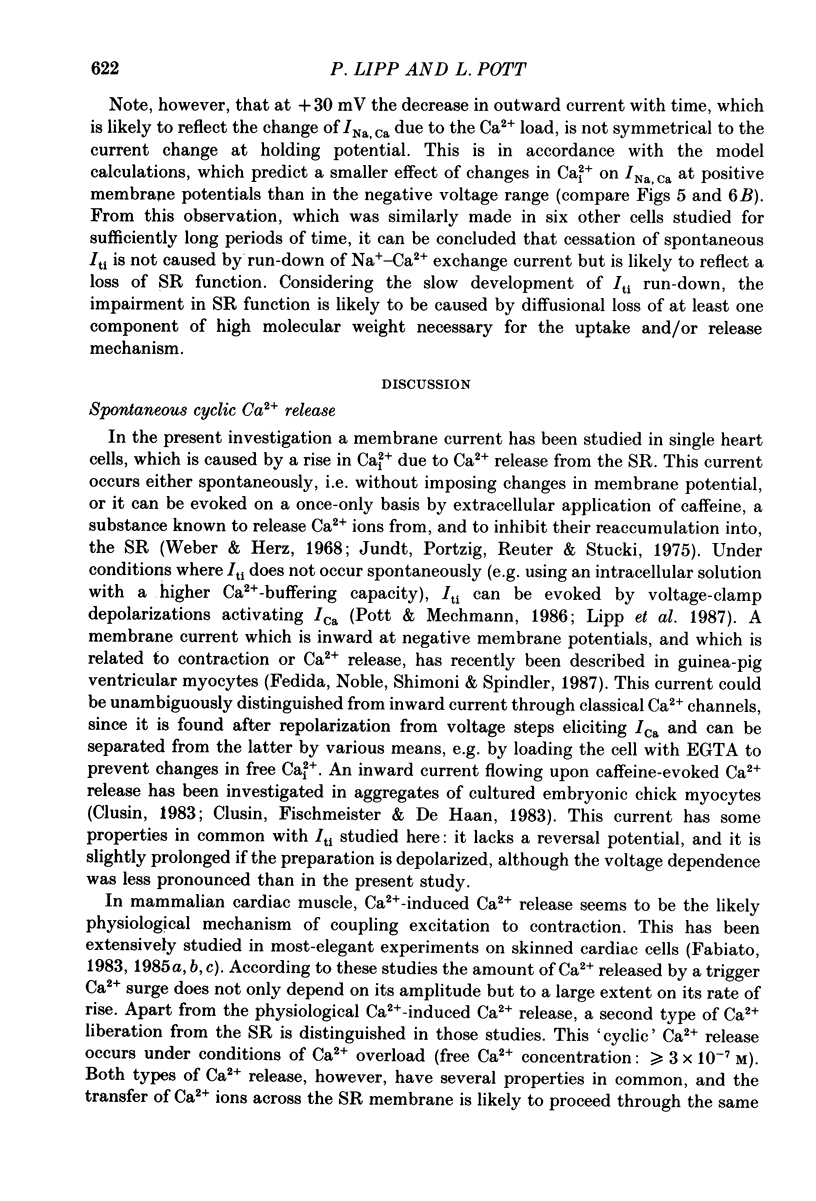
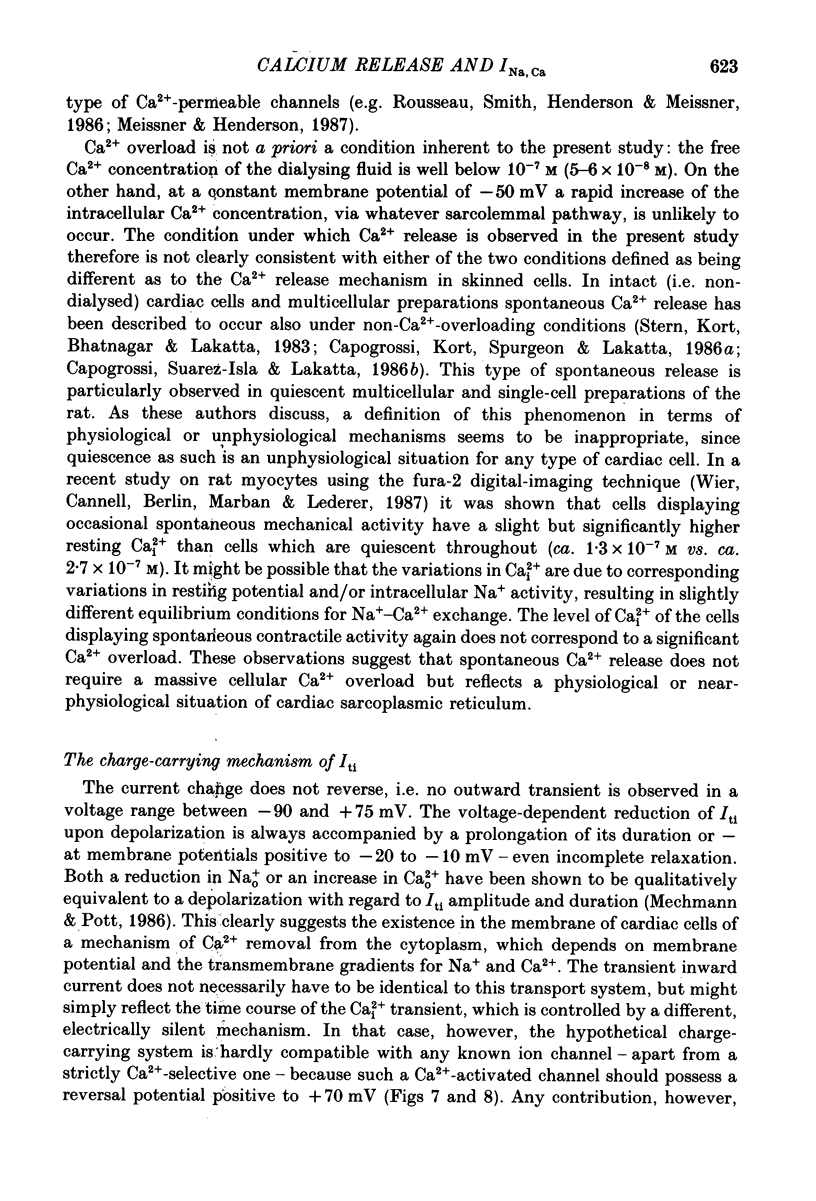
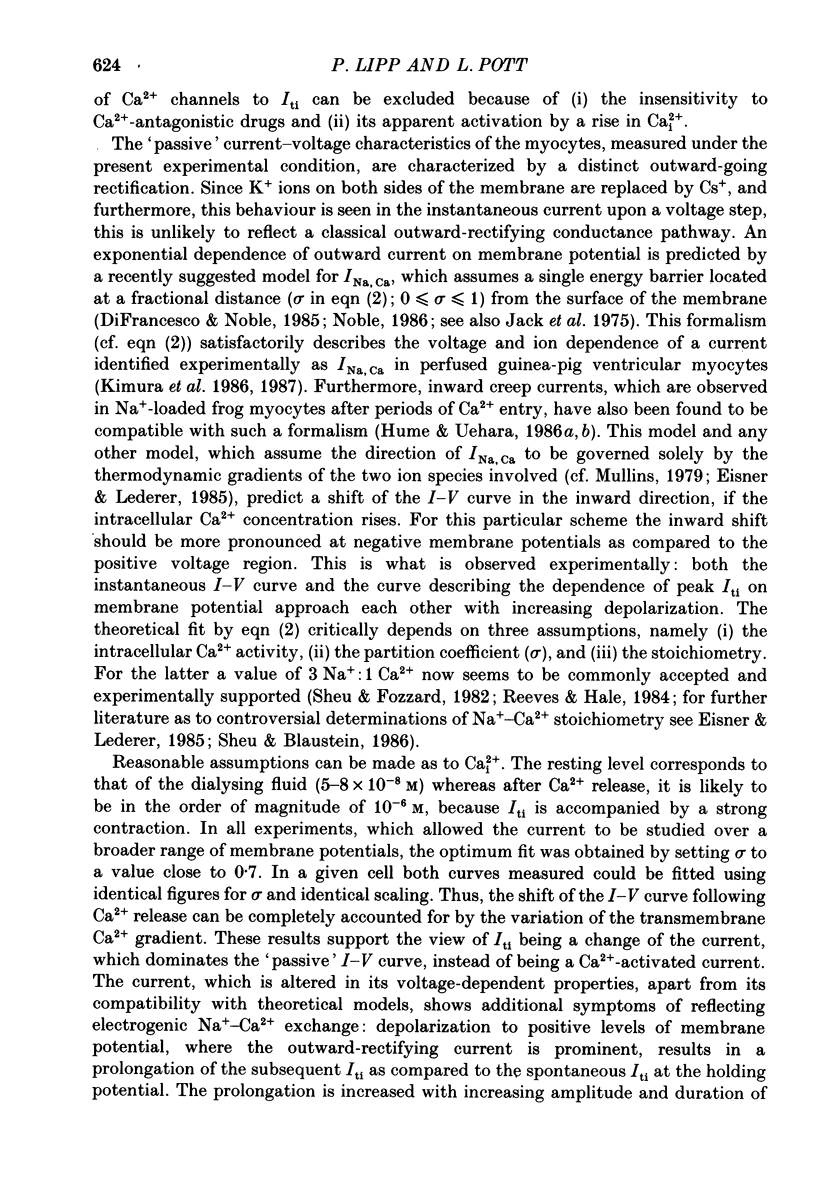
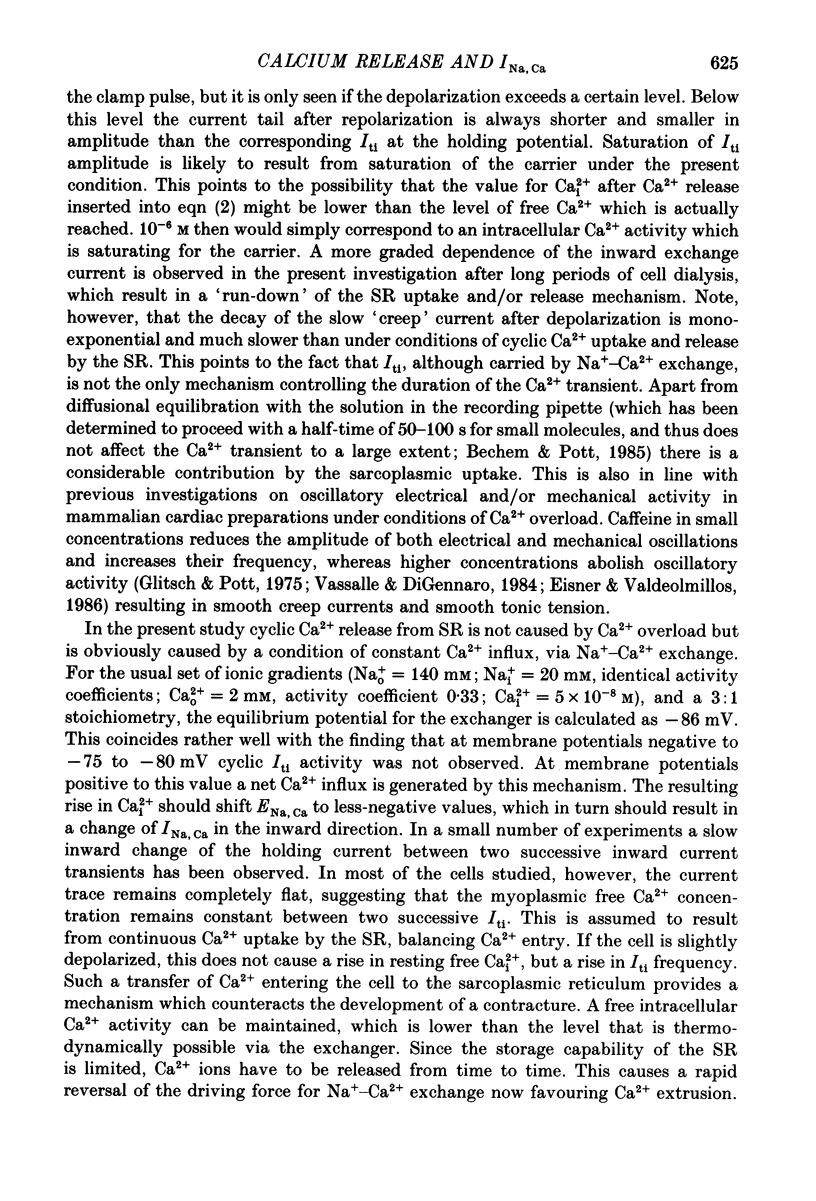
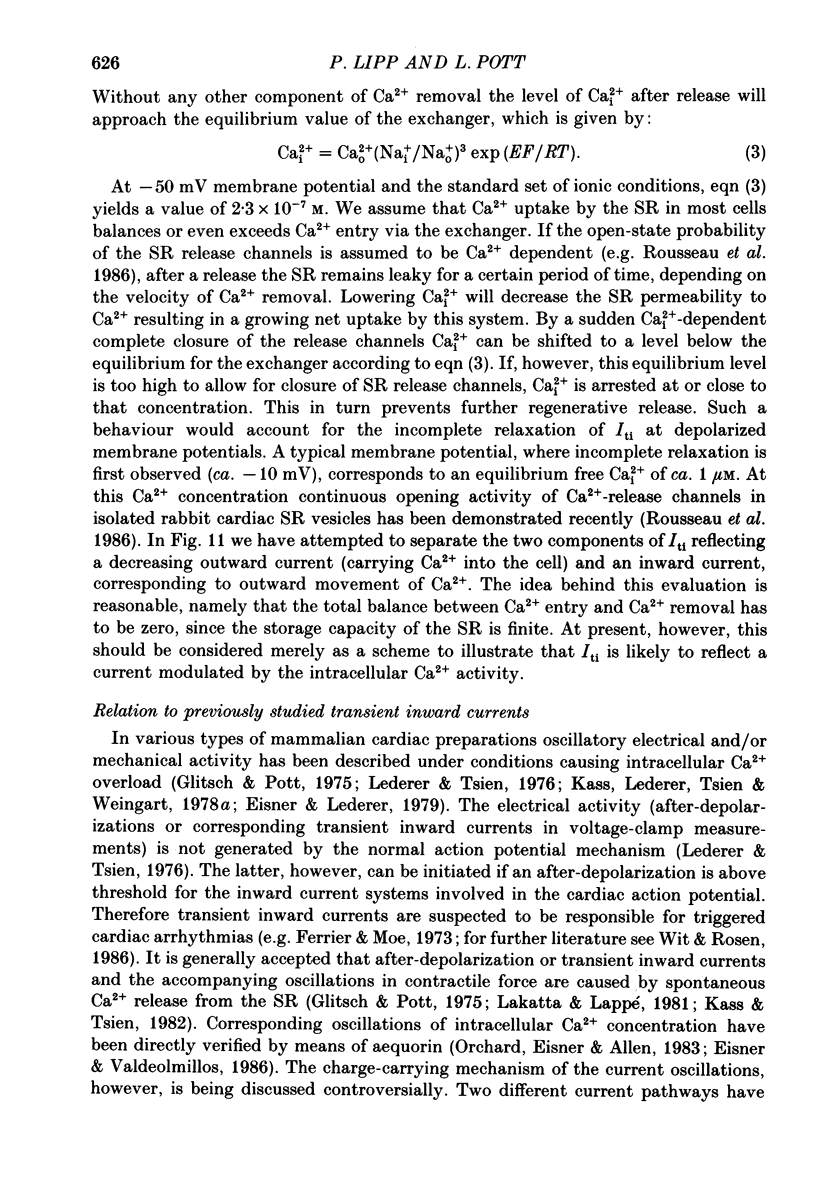
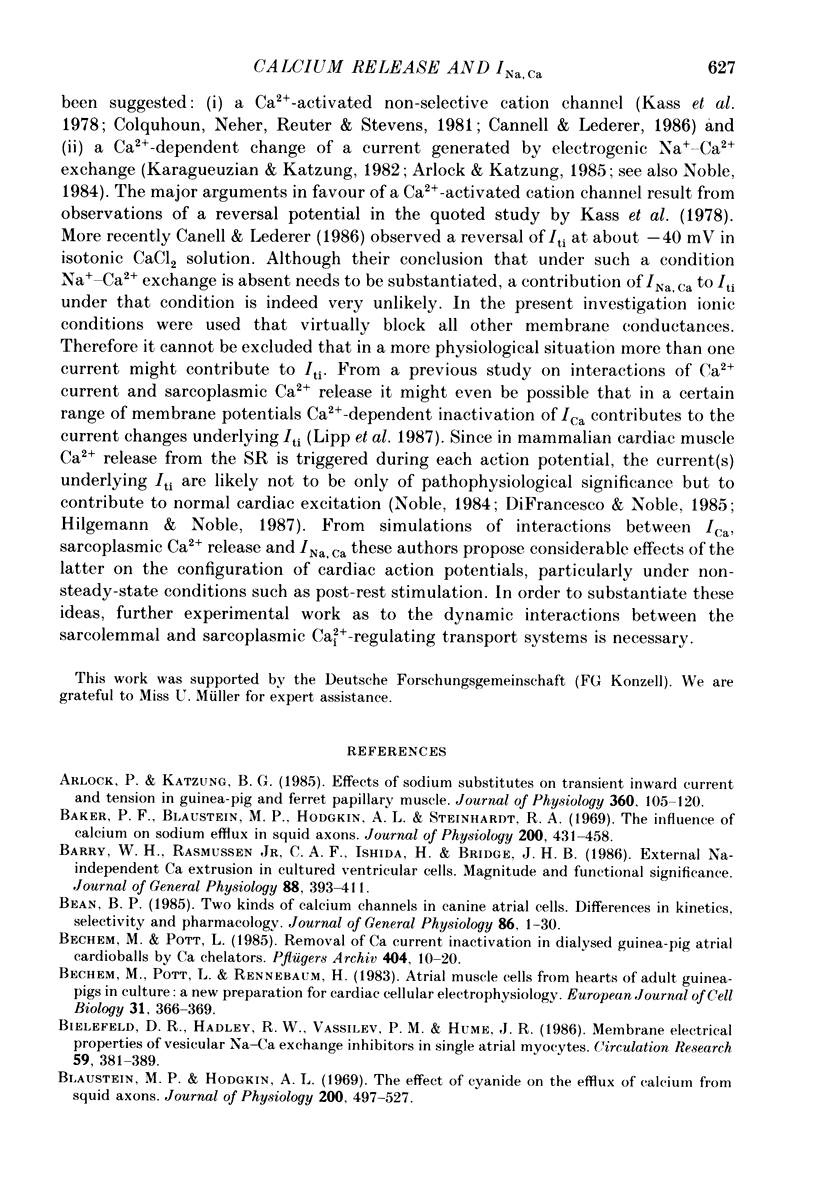
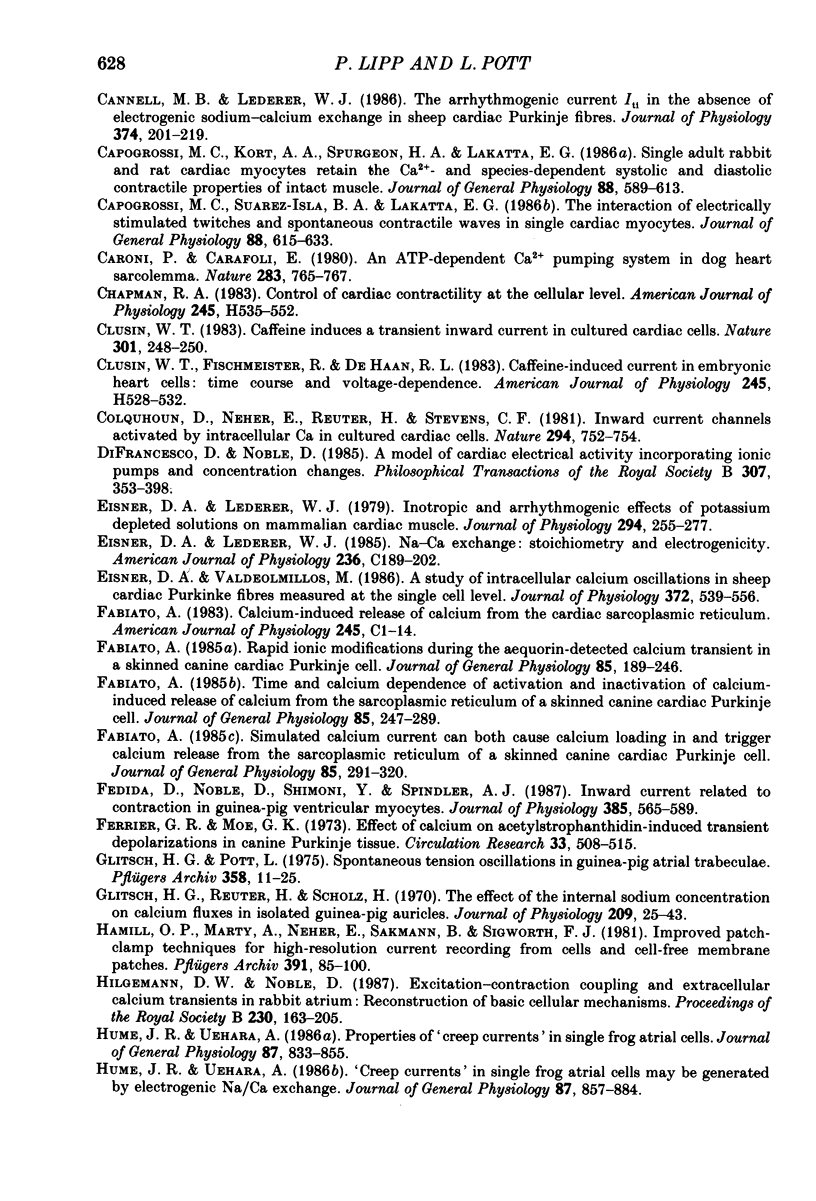
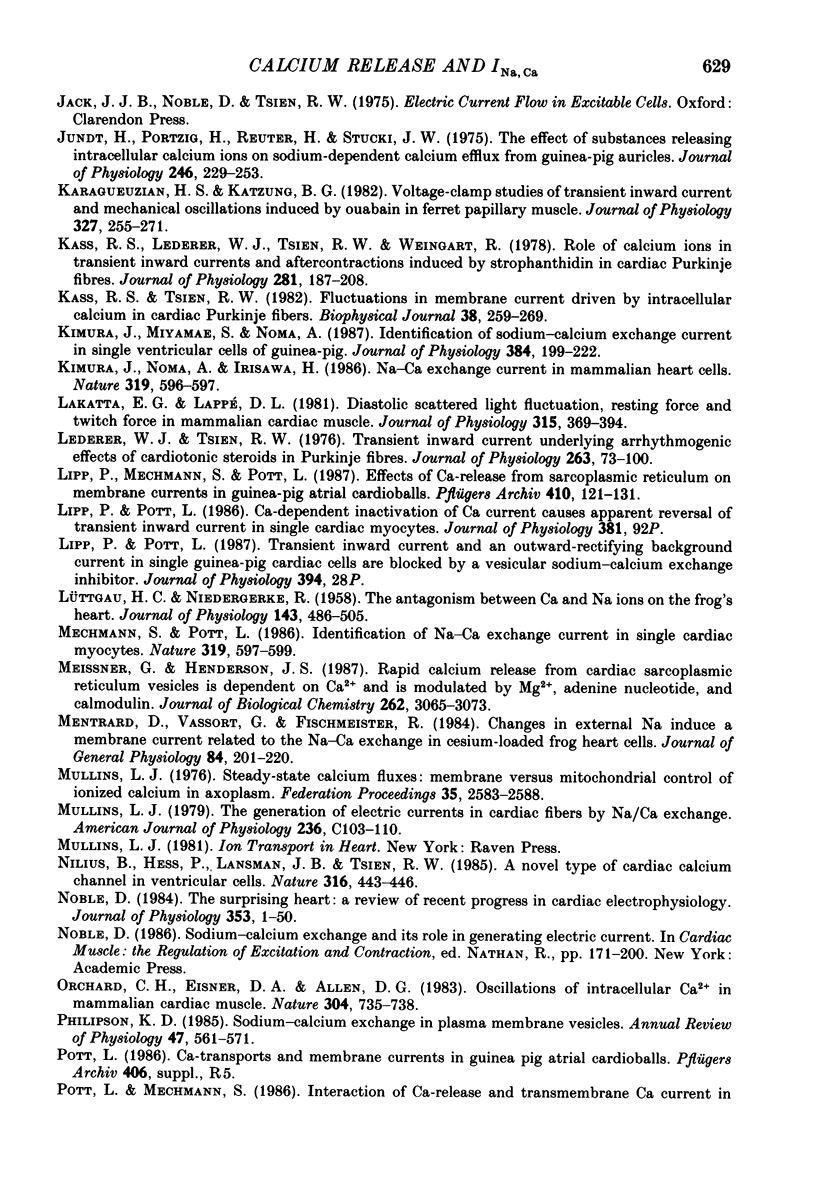
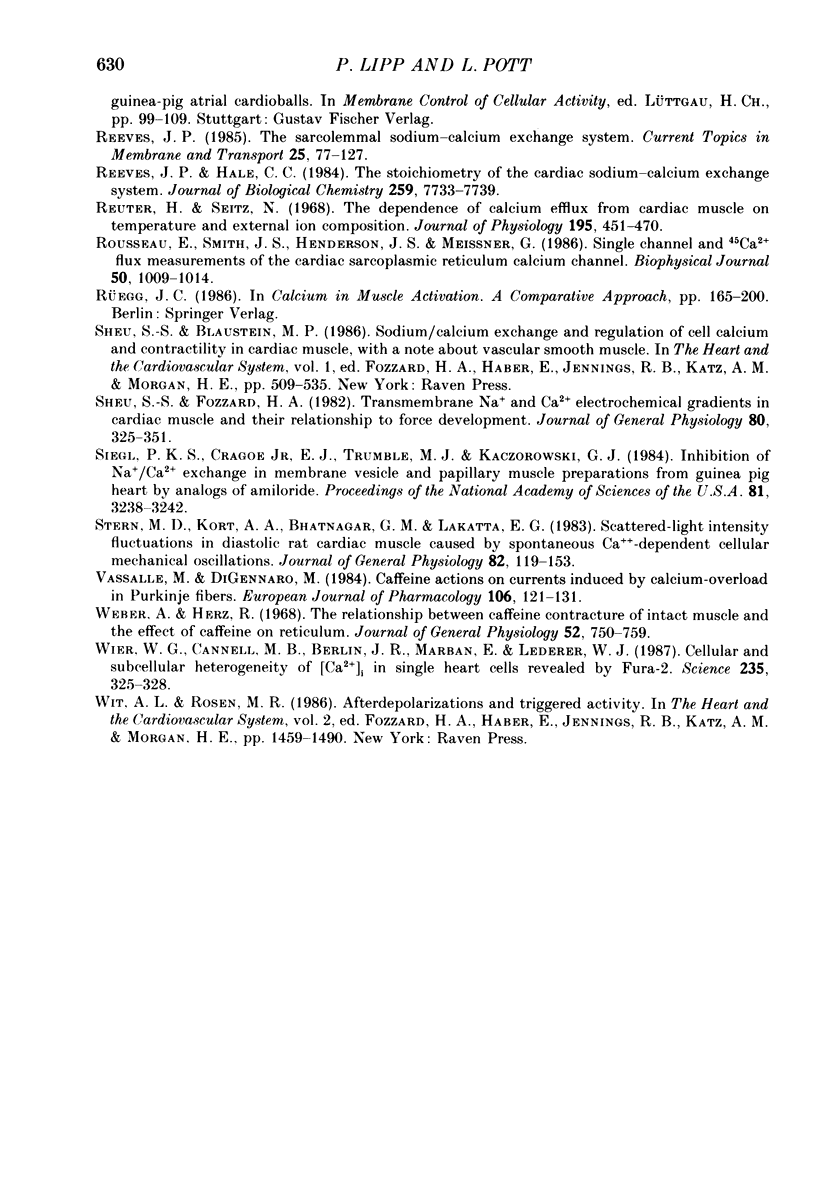
Images in this article
Selected References
These references are in PubMed. This may not be the complete list of references from this article.
- Arlock P., Katzung B. G. Effects of sodium substitutes on transient inward current and tension in guinea-pig and ferret papillary muscle. J Physiol. 1985 Mar;360:105–120. doi: 10.1113/jphysiol.1985.sp015606. [DOI] [PMC free article] [PubMed] [Google Scholar]
- Baker P. F., Blaustein M. P., Hodgkin A. L., Steinhardt R. A. The influence of calcium on sodium efflux in squid axons. J Physiol. 1969 Feb;200(2):431–458. doi: 10.1113/jphysiol.1969.sp008702. [DOI] [PMC free article] [PubMed] [Google Scholar]
- Barry W. H., Rasmussen C. A., Jr, Ishida H., Bridge J. H. External Na-independent Ca extrusion in cultured ventricular cells. Magnitude and functional significance. J Gen Physiol. 1986 Sep;88(3):393–411. doi: 10.1085/jgp.88.3.393. [DOI] [PMC free article] [PubMed] [Google Scholar]
- Bean B. P. Two kinds of calcium channels in canine atrial cells. Differences in kinetics, selectivity, and pharmacology. J Gen Physiol. 1985 Jul;86(1):1–30. doi: 10.1085/jgp.86.1.1. [DOI] [PMC free article] [PubMed] [Google Scholar]
- Bechem M., Pott L. Removal of Ca current inactivation in dialysed guinea-pig atrial cardioballs by Ca chelators. Pflugers Arch. 1985 May;404(1):10–20. doi: 10.1007/BF00581485. [DOI] [PubMed] [Google Scholar]
- Bechem M., Pott L., Rennebaum H. Atrial muscle cells from hearts of adult guinea-pigs in culture: a new preparation for cardiac cellular electrophysiology. Eur J Cell Biol. 1983 Sep;31(2):366–369. [PubMed] [Google Scholar]
- Bielefeld D. R., Hadley R. W., Vassilev P. M., Hume J. R. Membrane electrical properties of vesicular Na-Ca exchange inhibitors in single atrial myocytes. Circ Res. 1986 Oct;59(4):381–389. doi: 10.1161/01.res.59.4.381. [DOI] [PubMed] [Google Scholar]
- Blaustein M. P., Hodgkin A. L. The effect of cyanide on the efflux of calcium from squid axons. J Physiol. 1969 Feb;200(2):497–527. doi: 10.1113/jphysiol.1969.sp008704. [DOI] [PMC free article] [PubMed] [Google Scholar]
- Cannell M. B., Lederer W. J. The arrhythmogenic current ITI in the absence of electrogenic sodium-calcium exchange in sheep cardiac Purkinje fibres. J Physiol. 1986 May;374:201–219. doi: 10.1113/jphysiol.1986.sp016075. [DOI] [PMC free article] [PubMed] [Google Scholar]
- Capogrossi M. C., Kort A. A., Spurgeon H. A., Lakatta E. G. Single adult rabbit and rat cardiac myocytes retain the Ca2+- and species-dependent systolic and diastolic contractile properties of intact muscle. J Gen Physiol. 1986 Nov;88(5):589–613. doi: 10.1085/jgp.88.5.589. [DOI] [PMC free article] [PubMed] [Google Scholar]
- Capogrossi M. C., Suarez-Isla B. A., Lakatta E. G. The interaction of electrically stimulated twitches and spontaneous contractile waves in single cardiac myocytes. J Gen Physiol. 1986 Nov;88(5):615–633. doi: 10.1085/jgp.88.5.615. [DOI] [PMC free article] [PubMed] [Google Scholar]
- Caroni P., Carafoli E. An ATP-dependent Ca2+-pumping system in dog heart sarcolemma. Nature. 1980 Feb 21;283(5749):765–767. doi: 10.1038/283765a0. [DOI] [PubMed] [Google Scholar]
- Chapman R. A. Control of cardiac contractility at the cellular level. Am J Physiol. 1983 Oct;245(4):H535–H552. doi: 10.1152/ajpheart.1983.245.4.H535. [DOI] [PubMed] [Google Scholar]
- Clusin W. T. Caffeine induces a transient inward current in cultured cardiac cells. Nature. 1983 Jan 20;301(5897):248–250. doi: 10.1038/301248a0. [DOI] [PubMed] [Google Scholar]
- Clusin W. T., Fischmeister R., DeHaan R. L. Caffeine-induced current in embryonic heart cells: time course and voltage dependence. Am J Physiol. 1983 Sep;245(3):H528–H532. doi: 10.1152/ajpheart.1983.245.3.H528. [DOI] [PubMed] [Google Scholar]
- Colquhoun D., Neher E., Reuter H., Stevens C. F. Inward current channels activated by intracellular Ca in cultured cardiac cells. Nature. 1981 Dec 24;294(5843):752–754. doi: 10.1038/294752a0. [DOI] [PubMed] [Google Scholar]
- DiFrancesco D., Noble D. A model of cardiac electrical activity incorporating ionic pumps and concentration changes. Philos Trans R Soc Lond B Biol Sci. 1985 Jan 10;307(1133):353–398. doi: 10.1098/rstb.1985.0001. [DOI] [PubMed] [Google Scholar]
- Eisner D. A., Lederer W. J. Inotropic and arrhythmogenic effects of potassium-depleted solutions on mammalian cardiac muscle. J Physiol. 1979 Sep;294:255–277. doi: 10.1113/jphysiol.1979.sp012929. [DOI] [PMC free article] [PubMed] [Google Scholar]
- Eisner D. A., Lederer W. J. Na-Ca exchange: stoichiometry and electrogenicity. Am J Physiol. 1985 Mar;248(3 Pt 1):C189–C202. doi: 10.1152/ajpcell.1985.248.3.C189. [DOI] [PubMed] [Google Scholar]
- Eisner D. A., Valdeolmillos M. A study of intracellular calcium oscillations in sheep cardiac Purkinje fibres measured at the single cell level. J Physiol. 1986 Mar;372:539–556. doi: 10.1113/jphysiol.1986.sp016024. [DOI] [PMC free article] [PubMed] [Google Scholar]
- Fabiato A. Calcium-induced release of calcium from the cardiac sarcoplasmic reticulum. Am J Physiol. 1983 Jul;245(1):C1–14. doi: 10.1152/ajpcell.1983.245.1.C1. [DOI] [PubMed] [Google Scholar]
- Fabiato A. Rapid ionic modifications during the aequorin-detected calcium transient in a skinned canine cardiac Purkinje cell. J Gen Physiol. 1985 Feb;85(2):189–246. doi: 10.1085/jgp.85.2.189. [DOI] [PMC free article] [PubMed] [Google Scholar]
- Fabiato A. Simulated calcium current can both cause calcium loading in and trigger calcium release from the sarcoplasmic reticulum of a skinned canine cardiac Purkinje cell. J Gen Physiol. 1985 Feb;85(2):291–320. doi: 10.1085/jgp.85.2.291. [DOI] [PMC free article] [PubMed] [Google Scholar]
- Fabiato A. Time and calcium dependence of activation and inactivation of calcium-induced release of calcium from the sarcoplasmic reticulum of a skinned canine cardiac Purkinje cell. J Gen Physiol. 1985 Feb;85(2):247–289. doi: 10.1085/jgp.85.2.247. [DOI] [PMC free article] [PubMed] [Google Scholar]
- Fedida D., Noble D., Shimoni Y., Spindler A. J. Inward current related to contraction in guinea-pig ventricular myocytes. J Physiol. 1987 Apr;385:565–589. doi: 10.1113/jphysiol.1987.sp016508. [DOI] [PMC free article] [PubMed] [Google Scholar]
- Ferrier G. R., Moe G. K. Effect of calcium on acetylstrophanthidin-induced transient depolarizations in canine Purkinje tissue. Circ Res. 1973 Nov;33(5):508–515. doi: 10.1161/01.res.33.5.508. [DOI] [PubMed] [Google Scholar]
- Glitsch H. G., Pott L. Spontaneous tension oscillations in guinea-pig atrial trabeculae. Pflugers Arch. 1975 Jul 9;358(1):11–25. doi: 10.1007/BF00584566. [DOI] [PubMed] [Google Scholar]
- Glitsch H. G., Reuter H., Scholz H. The effect of the internal sodium concentration on calcium fluxes in isolated guinea-pig auricles. J Physiol. 1970 Jul;209(1):25–43. doi: 10.1113/jphysiol.1970.sp009153. [DOI] [PMC free article] [PubMed] [Google Scholar]
- Hamill O. P., Marty A., Neher E., Sakmann B., Sigworth F. J. Improved patch-clamp techniques for high-resolution current recording from cells and cell-free membrane patches. Pflugers Arch. 1981 Aug;391(2):85–100. doi: 10.1007/BF00656997. [DOI] [PubMed] [Google Scholar]
- Hilgemann D. W., Noble D. Excitation-contraction coupling and extracellular calcium transients in rabbit atrium: reconstruction of basic cellular mechanisms. Proc R Soc Lond B Biol Sci. 1987 Mar 23;230(1259):163–205. doi: 10.1098/rspb.1987.0015. [DOI] [PubMed] [Google Scholar]
- Hume J. R., Uehara A. "Creep currents" in single frog atrial cells may be generated by electrogenic Na/Ca exchange. J Gen Physiol. 1986 Jun;87(6):857–884. doi: 10.1085/jgp.87.6.857. [DOI] [PMC free article] [PubMed] [Google Scholar]
- Hume J. R., Uehara A. Properties of "creep currents" in single frog atrial cells. J Gen Physiol. 1986 Jun;87(6):833–855. doi: 10.1085/jgp.87.6.833. [DOI] [PMC free article] [PubMed] [Google Scholar]
- Jundt H., Porzig H., Reuter H., Stucki J. W. The effect of substances releasing intracellular calcium ions on sodium-dependent calcium efflux from guinea-pig auricles. J Physiol. 1975 Mar;246(1):229–253. doi: 10.1113/jphysiol.1975.sp010888. [DOI] [PMC free article] [PubMed] [Google Scholar]
- Karagueuzian H. S., Katzung B. G. Voltage-clamp studies of transient inward current and mechanical oscillations induced by ouabain in ferret papillary muscle. J Physiol. 1982 Jun;327:255–271. doi: 10.1113/jphysiol.1982.sp014230. [DOI] [PMC free article] [PubMed] [Google Scholar]
- Kass R. S., Lederer W. J., Tsien R. W., Weingart R. Role of calcium ions in transient inward currents and aftercontractions induced by strophanthidin in cardiac Purkinje fibres. J Physiol. 1978 Aug;281:187–208. doi: 10.1113/jphysiol.1978.sp012416. [DOI] [PMC free article] [PubMed] [Google Scholar]
- Kass R. S., Tsien R. W. Fluctuations in membrane current driven by intracellular calcium in cardiac Purkinje fibers. Biophys J. 1982 Jun;38(3):259–269. doi: 10.1016/S0006-3495(82)84557-8. [DOI] [PMC free article] [PubMed] [Google Scholar]
- Kimura J., Miyamae S., Noma A. Identification of sodium-calcium exchange current in single ventricular cells of guinea-pig. J Physiol. 1987 Mar;384:199–222. doi: 10.1113/jphysiol.1987.sp016450. [DOI] [PMC free article] [PubMed] [Google Scholar]
- Kimura J., Noma A., Irisawa H. Na-Ca exchange current in mammalian heart cells. Nature. 1986 Feb 13;319(6054):596–597. doi: 10.1038/319596a0. [DOI] [PubMed] [Google Scholar]
- LUTTGAU H. C., NIEDERGERKE R. The antagonism between Ca and Na ions on the frog's heart. J Physiol. 1958 Oct 31;143(3):486–505. doi: 10.1113/jphysiol.1958.sp006073. [DOI] [PMC free article] [PubMed] [Google Scholar]
- Lakatta E. G., Lappé D. L. Diastolic scattered light fluctuation, resting force and twitch force in mammalian cardiac muscle. J Physiol. 1981 Jun;315:369–394. doi: 10.1113/jphysiol.1981.sp013753. [DOI] [PMC free article] [PubMed] [Google Scholar]
- Lederer W. J., Tsien R. W. Transient inward current underlying arrhythmogenic effects of cardiotonic steroids in Purkinje fibres. J Physiol. 1976 Dec;263(2):73–100. doi: 10.1113/jphysiol.1976.sp011622. [DOI] [PMC free article] [PubMed] [Google Scholar]
- Lipp P., Mechmann S., Pott L. Effects of calcium release from sarcoplasmic reticulum on membrane currents in guinea pig atrial cardioballs. Pflugers Arch. 1987 Sep;410(1-2):121–131. doi: 10.1007/BF00581904. [DOI] [PubMed] [Google Scholar]
- Mechmann S., Pott L. Identification of Na-Ca exchange current in single cardiac myocytes. Nature. 1986 Feb 13;319(6054):597–599. doi: 10.1038/319597a0. [DOI] [PubMed] [Google Scholar]
- Meissner G., Henderson J. S. Rapid calcium release from cardiac sarcoplasmic reticulum vesicles is dependent on Ca2+ and is modulated by Mg2+, adenine nucleotide, and calmodulin. J Biol Chem. 1987 Mar 5;262(7):3065–3073. [PubMed] [Google Scholar]
- Mentrard D., Vassort G., Fischmeister R. Changes in external Na induce a membrane current related to the Na-Ca exchange in cesium-loaded frog heart cells. J Gen Physiol. 1984 Aug;84(2):201–220. doi: 10.1085/jgp.84.2.201. [DOI] [PMC free article] [PubMed] [Google Scholar]
- Mullins L. J. Steady-state calcium fluxes: membrane versus mitochondrial control of ionized calcium in axoplasm. Fed Proc. 1976 Dec;35(14):2583–2588. [PubMed] [Google Scholar]
- Mullins L. J. The generation of electric currents in cardiac fibers by Na/Ca exchange. Am J Physiol. 1979 Mar;236(3):C103–C110. doi: 10.1152/ajpcell.1979.236.3.C103. [DOI] [PubMed] [Google Scholar]
- Nilius B., Hess P., Lansman J. B., Tsien R. W. A novel type of cardiac calcium channel in ventricular cells. Nature. 1985 Aug 1;316(6027):443–446. doi: 10.1038/316443a0. [DOI] [PubMed] [Google Scholar]
- Noble D. The surprising heart: a review of recent progress in cardiac electrophysiology. J Physiol. 1984 Aug;353:1–50. doi: 10.1113/jphysiol.1984.sp015320. [DOI] [PMC free article] [PubMed] [Google Scholar]
- Orchard C. H., Eisner D. A., Allen D. G. Oscillations of intracellular Ca2+ in mammalian cardiac muscle. Nature. 1983 Aug 25;304(5928):735–738. doi: 10.1038/304735a0. [DOI] [PubMed] [Google Scholar]
- Philipson K. D. Sodium-calcium exchange in plasma membrane vesicles. Annu Rev Physiol. 1985;47:561–571. doi: 10.1146/annurev.ph.47.030185.003021. [DOI] [PubMed] [Google Scholar]
- Reeves J. P., Hale C. C. The stoichiometry of the cardiac sodium-calcium exchange system. J Biol Chem. 1984 Jun 25;259(12):7733–7739. [PubMed] [Google Scholar]
- Reuter H., Seitz N. The dependence of calcium efflux from cardiac muscle on temperature and external ion composition. J Physiol. 1968 Mar;195(2):451–470. doi: 10.1113/jphysiol.1968.sp008467. [DOI] [PMC free article] [PubMed] [Google Scholar]
- Rousseau E., Smith J. S., Henderson J. S., Meissner G. Single channel and 45Ca2+ flux measurements of the cardiac sarcoplasmic reticulum calcium channel. Biophys J. 1986 Nov;50(5):1009–1014. doi: 10.1016/S0006-3495(86)83543-3. [DOI] [PMC free article] [PubMed] [Google Scholar]
- Sheu S. S., Fozzard H. A. Transmembrane Na+ and Ca2+ electrochemical gradients in cardiac muscle and their relationship to force development. J Gen Physiol. 1982 Sep;80(3):325–351. doi: 10.1085/jgp.80.3.325. [DOI] [PMC free article] [PubMed] [Google Scholar]
- Siegl P. K., Cragoe E. J., Jr, Trumble M. J., Kaczorowski G. J. Inhibition of Na+/Ca2+ exchange in membrane vesicle and papillary muscle preparations from guinea pig heart by analogs of amiloride. Proc Natl Acad Sci U S A. 1984 May;81(10):3238–3242. doi: 10.1073/pnas.81.10.3238. [DOI] [PMC free article] [PubMed] [Google Scholar]
- Stern M. D., Kort A. A., Bhatnagar G. M., Lakatta E. G. Scattered-light intensity fluctuations in diastolic rat cardiac muscle caused by spontaneous Ca++-dependent cellular mechanical oscillations. J Gen Physiol. 1983 Jul;82(1):119–153. doi: 10.1085/jgp.82.1.119. [DOI] [PMC free article] [PubMed] [Google Scholar]
- Vassalle M., Di Gennaro M. Caffeine actions on currents induced by calcium-overload in Purkinje fibers. Eur J Pharmacol. 1984 Oct 30;106(1):121–131. doi: 10.1016/0014-2999(84)90685-x. [DOI] [PubMed] [Google Scholar]
- Weber A., Herz R. The relationship between caffeine contracture of intact muscle and the effect of caffeine on reticulum. J Gen Physiol. 1968 Nov;52(5):750–759. doi: 10.1085/jgp.52.5.750. [DOI] [PMC free article] [PubMed] [Google Scholar]
- Wier W. G., Cannell M. B., Berlin J. R., Marban E., Lederer W. J. Cellular and subcellular heterogeneity of [Ca2+]i in single heart cells revealed by fura-2. Science. 1987 Jan 16;235(4786):325–328. doi: 10.1126/science.3798114. [DOI] [PubMed] [Google Scholar]