Abstract
1. Maintenance heat produced in tetani of frogs' sartorius muscles (Rana japonica) was measured under various values of intracellular pH (pHi) brought about by increasing the CO2 concentration in Ringer solution. The pHi values were measured using 31P nuclear magnetic resonance from the chemical shifts of the inorganic phosphate resonance. The pHi was 7.10 +/- 0.009 (mean +/- S.E. of the mean, n = 10) in the gas mixture of 5% CO2/95% O2 at 4 degrees C and it was reduced to 6.44 +/- 0.001 (n = 23) in 45% CO2. 2. As CO2 was increased, the maximum force was decreased and relaxation was prolonged. This is in accordance with the results of Edman & Mattiazzi (1981) and Curtin (1986). 3. An increase in CO2 induced a reduction of the maintenance heat production, which can be divided into stable and labile heats (Aubert, 1956). The stable heat, which is produced with a steady rate during contraction, was decreased as CO2 was increased. The labile heat, which is produced with an exponentially declining rate, was not significantly altered by increasing CO2 within the range studied. 4. The effect of previous contractile activity on the labile heat production, i.e. the time course of repriming of the labile heat, could be described by an equation with two exponential terms in 5% CO2 in accordance with the result of Peckham & Woledge (1986). The time course of repriming of the labile heat was not affected by increasing CO2 to 20%.
Full text
PDF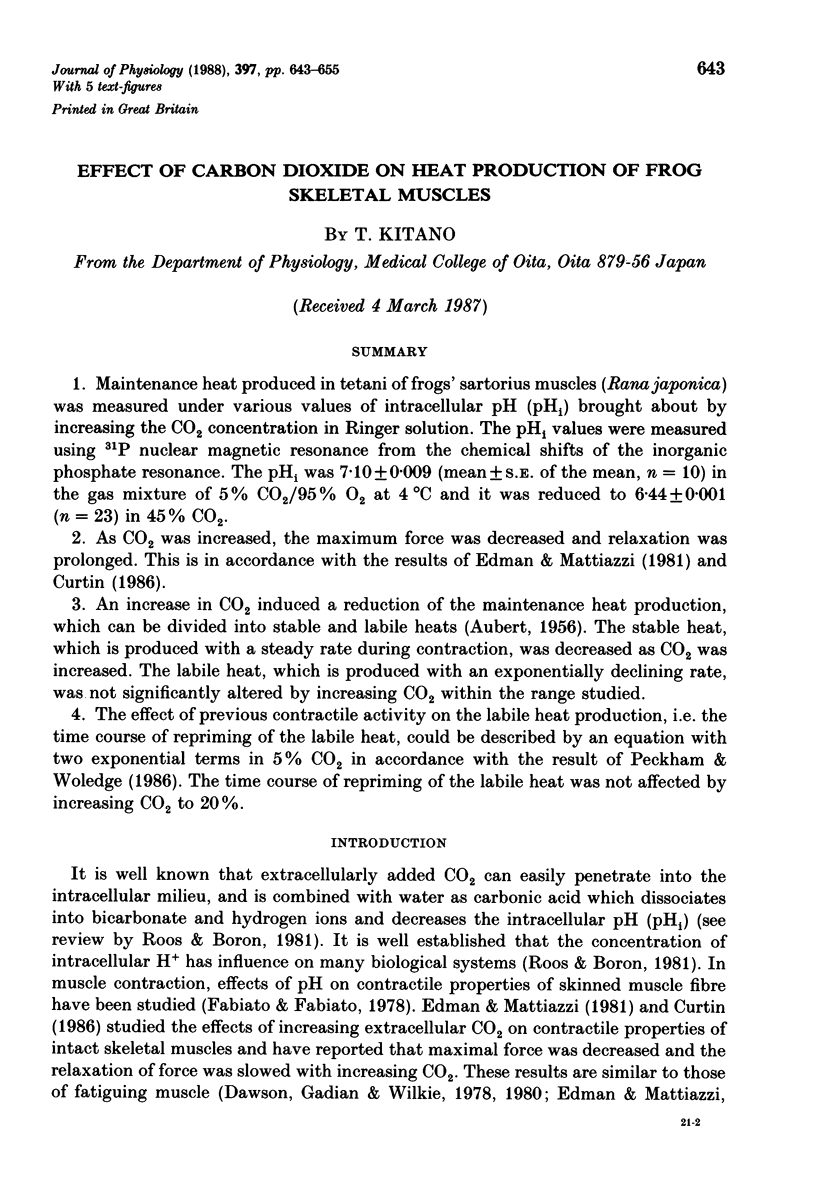
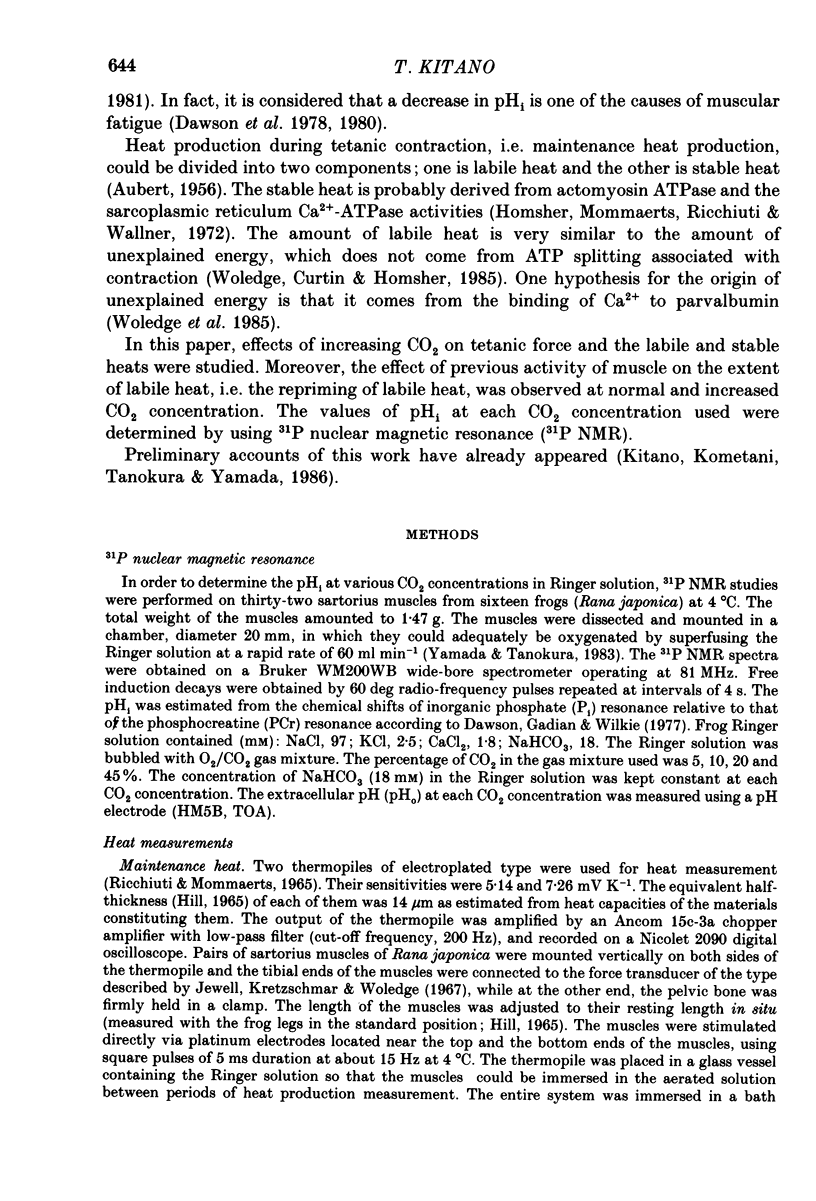
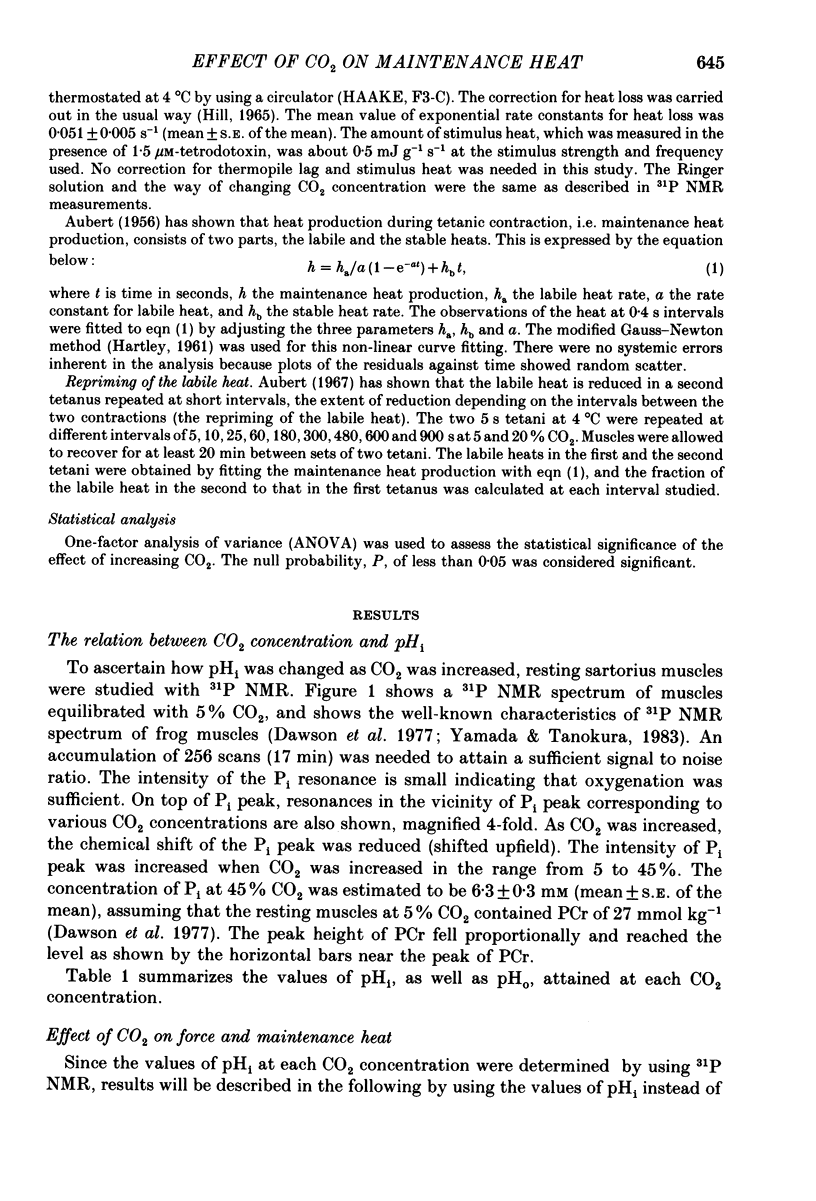

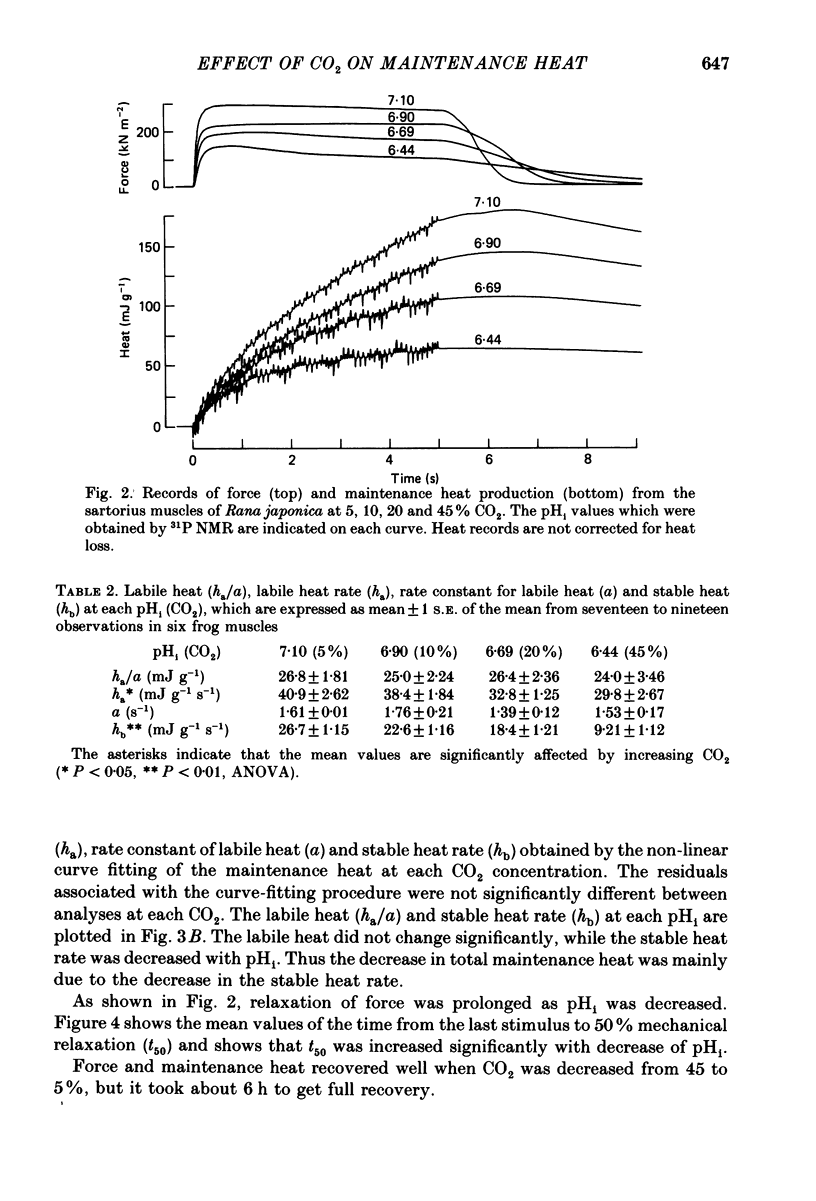
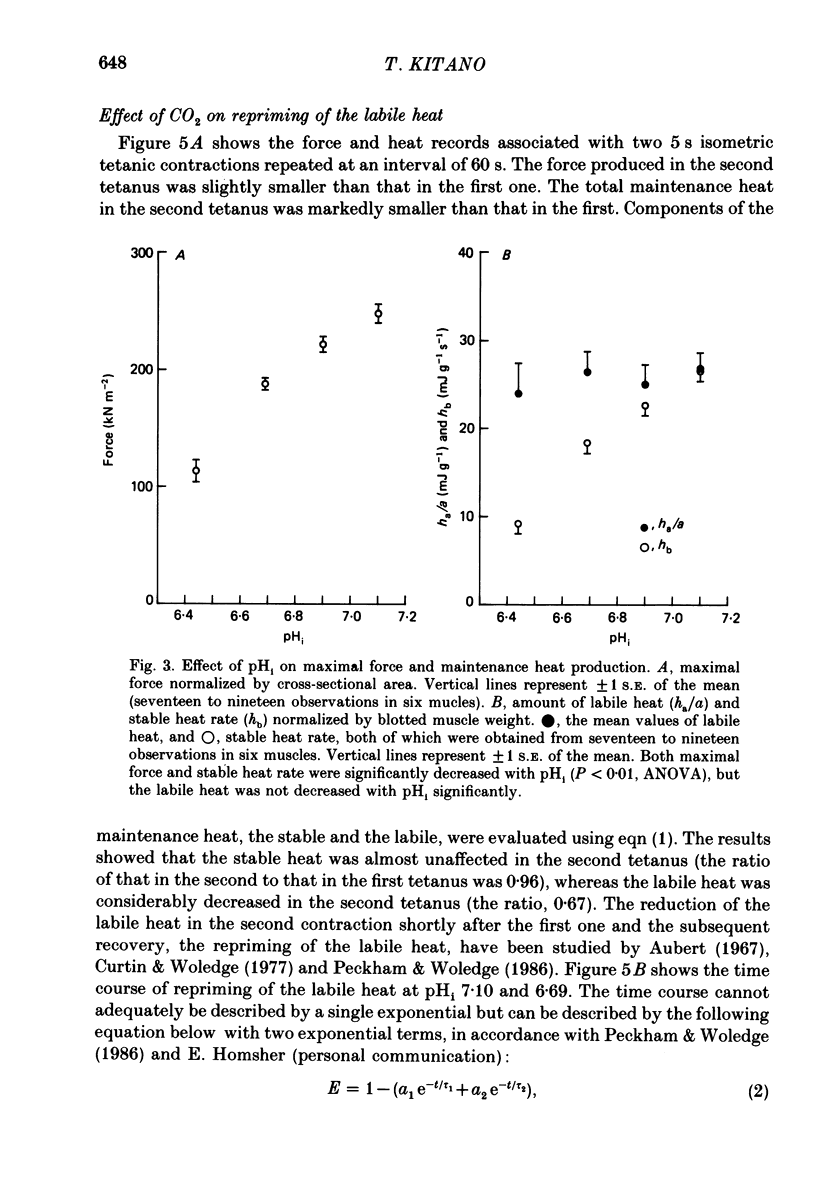
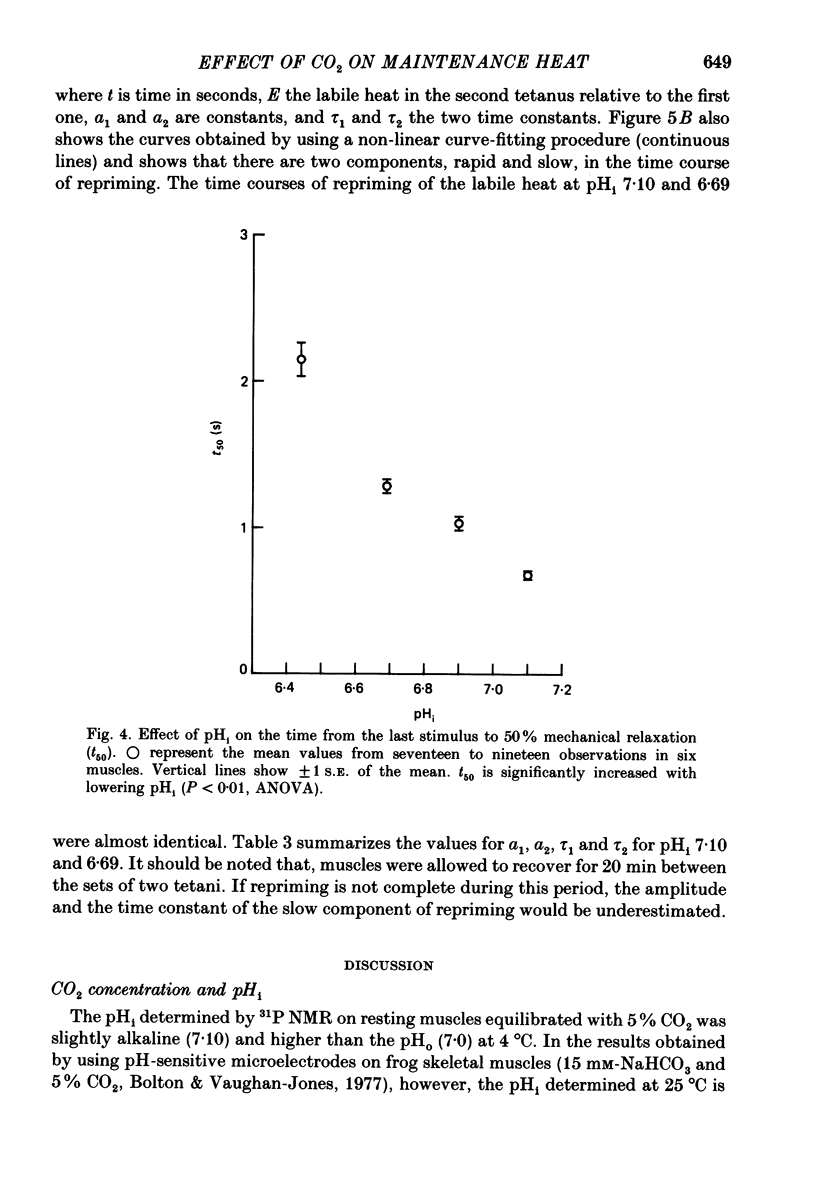
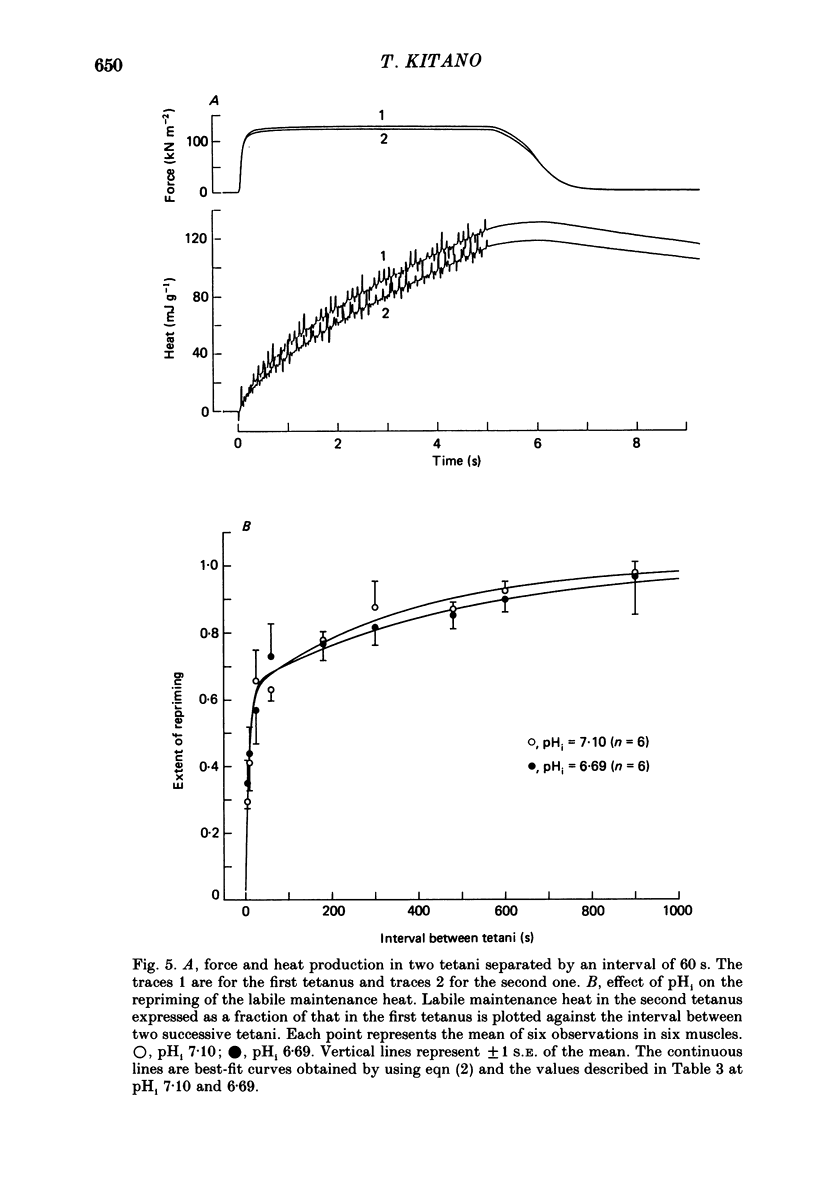
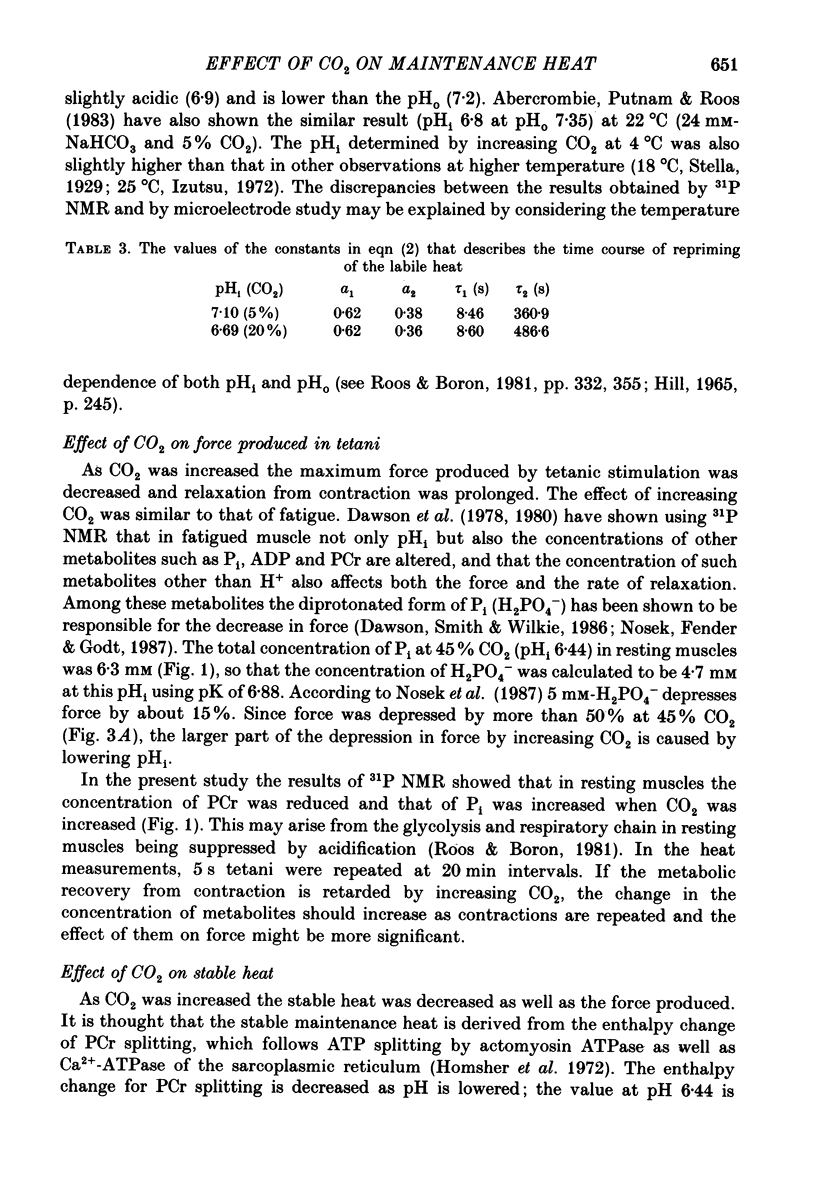
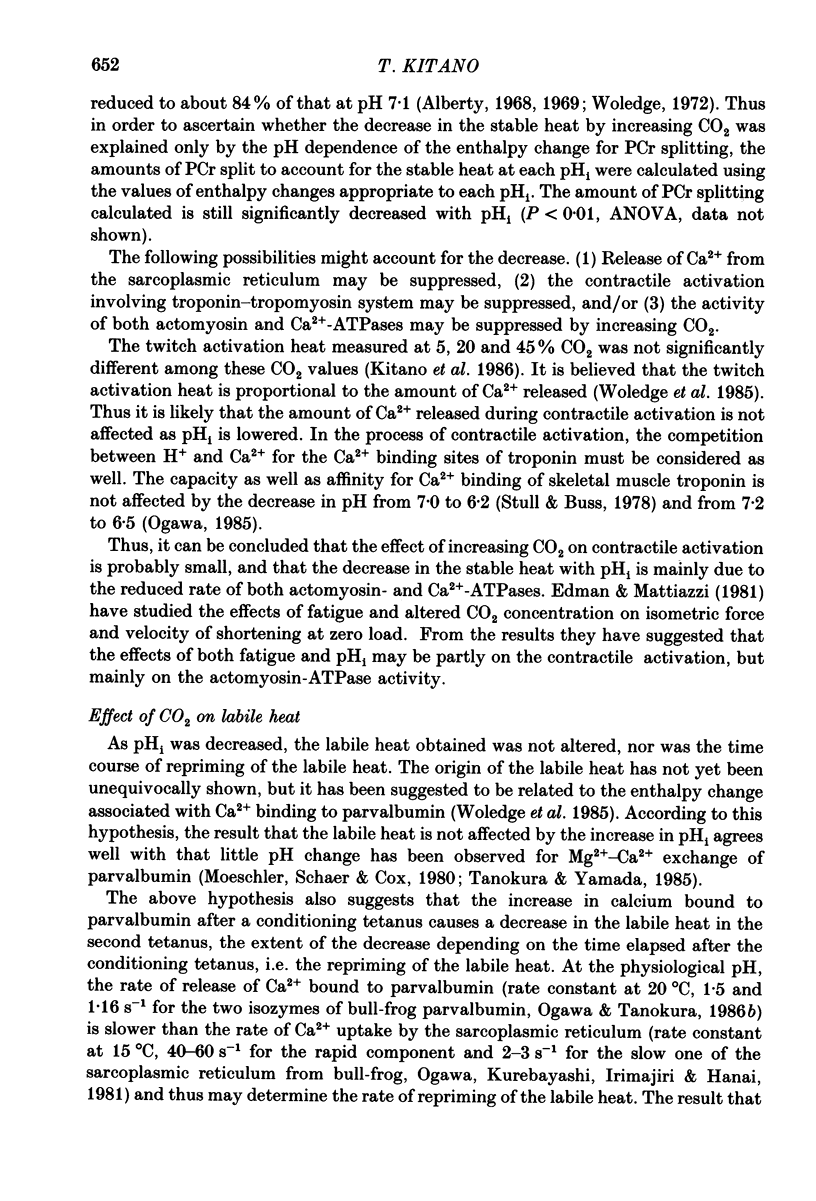
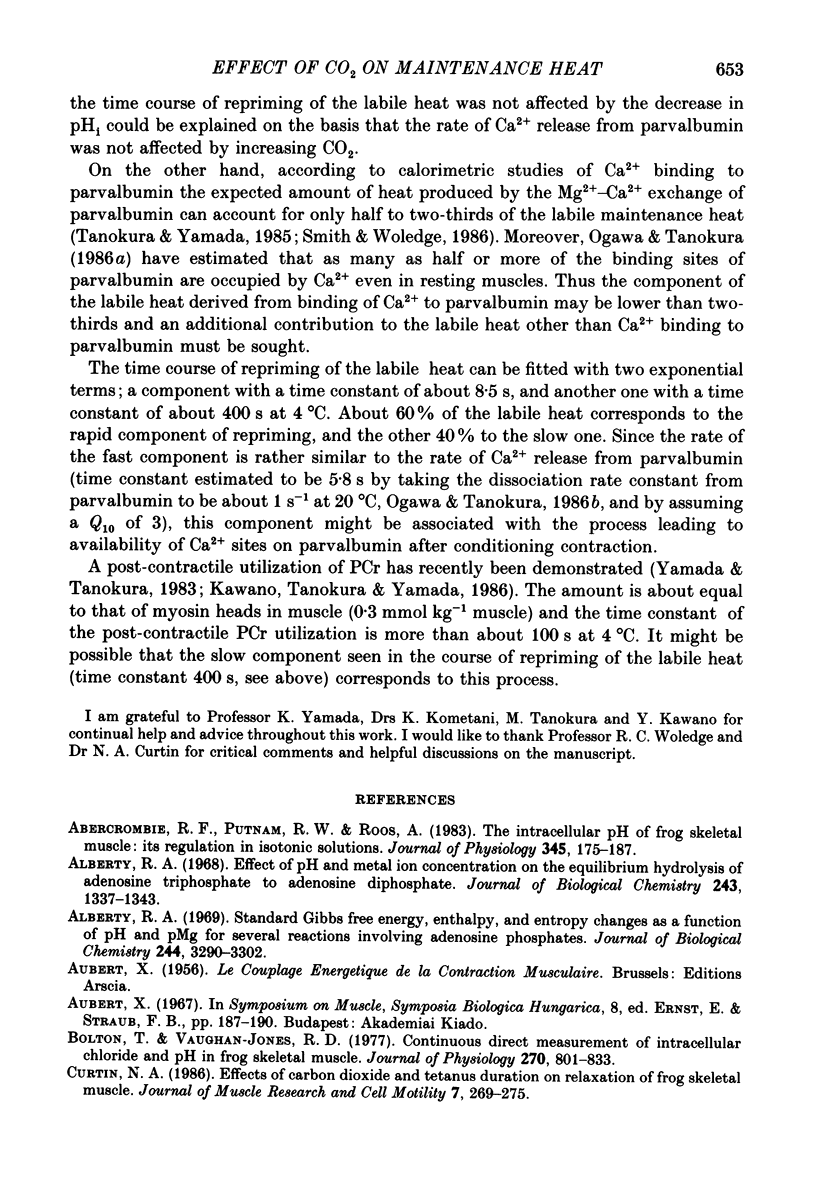
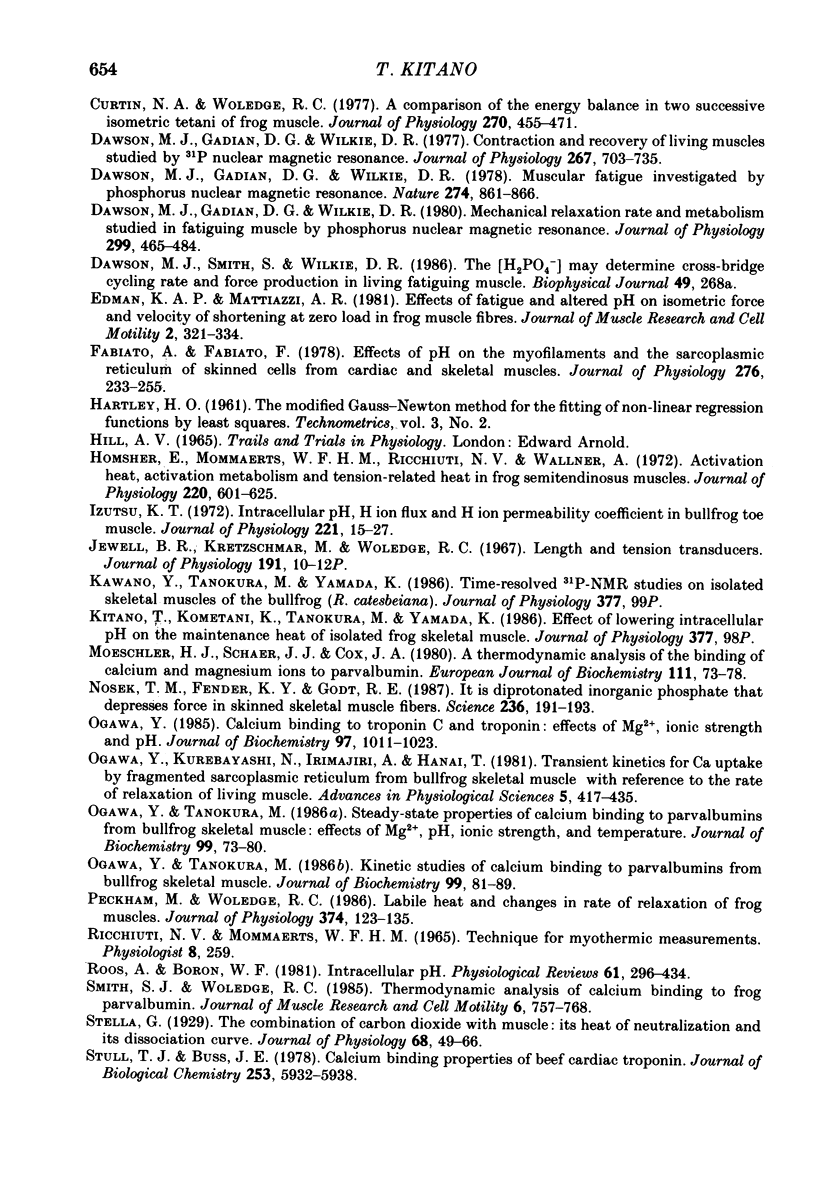
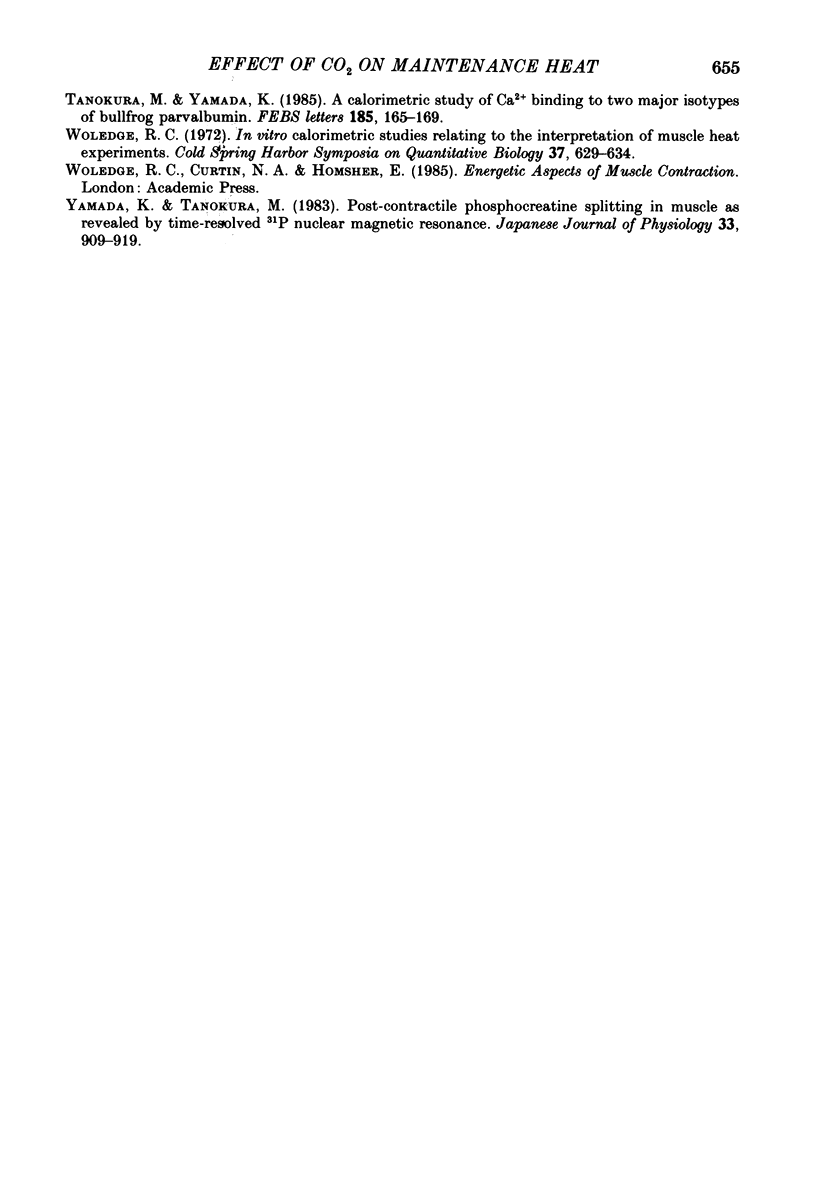
Selected References
These references are in PubMed. This may not be the complete list of references from this article.
- Abercrombie R. F., Putnam R. W., Roos A. The intracellular pH of frog skeletal muscle: its regulation in isotonic solutions. J Physiol. 1983 Dec;345:175–187. doi: 10.1113/jphysiol.1983.sp014973. [DOI] [PMC free article] [PubMed] [Google Scholar]
- Alberty R. A. Effect of pH and metal ion concentration on the equilibrium hydrolysis of adenosine triphosphate to adenosine diphosphate. J Biol Chem. 1968 Apr 10;243(7):1337–1343. [PubMed] [Google Scholar]
- Alberty R. A. Standard Gibbs free energy, enthalpy, and entropy changes as a function of pH and pMg for several reactions involving adenosine phosphates. J Biol Chem. 1969 Jun 25;244(12):3290–3302. [PubMed] [Google Scholar]
- Bolton T. B., Vaughan-Jones R. D. Continuous direct measurement of intracellular chloride and pH in frog skeletal muscle. J Physiol. 1977 Sep;270(3):801–833. doi: 10.1113/jphysiol.1977.sp011983. [DOI] [PMC free article] [PubMed] [Google Scholar]
- Curtin N. A. Effects of carbon dioxide and tetanus duration on relaxation of frog skeletal muscle. J Muscle Res Cell Motil. 1986 Jun;7(3):269–275. doi: 10.1007/BF01753560. [DOI] [PubMed] [Google Scholar]
- Curtin N. A., Woledge R. C. A comparison of the energy balance in two successive isometric tetani of frog muscle. J Physiol. 1977 Sep;270(2):455–471. doi: 10.1113/jphysiol.1977.sp011962. [DOI] [PMC free article] [PubMed] [Google Scholar]
- Dawson M. J., Gadian D. G., Wilkie D. R. Contraction and recovery of living muscles studies by 31P nuclear magnetic resonance. J Physiol. 1977 Jun;267(3):703–735. doi: 10.1113/jphysiol.1977.sp011835. [DOI] [PMC free article] [PubMed] [Google Scholar]
- Dawson M. J., Gadian D. G., Wilkie D. R. Mechanical relaxation rate and metabolism studied in fatiguing muscle by phosphorus nuclear magnetic resonance. J Physiol. 1980 Feb;299:465–484. doi: 10.1113/jphysiol.1980.sp013137. [DOI] [PMC free article] [PubMed] [Google Scholar]
- Dawson M. J., Gadian D. G., Wilkie D. R. Muscular fatigue investigated by phosphorus nuclear magnetic resonance. Nature. 1978 Aug 31;274(5674):861–866. doi: 10.1038/274861a0. [DOI] [PubMed] [Google Scholar]
- Edman K. A., Mattiazzi A. R. Effects of fatigue and altered pH on isometric force and velocity of shortening at zero load in frog muscle fibres. J Muscle Res Cell Motil. 1981 Sep;2(3):321–334. doi: 10.1007/BF00713270. [DOI] [PubMed] [Google Scholar]
- Fabiato A., Fabiato F. Effects of pH on the myofilaments and the sarcoplasmic reticulum of skinned cells from cardiace and skeletal muscles. J Physiol. 1978 Mar;276:233–255. doi: 10.1113/jphysiol.1978.sp012231. [DOI] [PMC free article] [PubMed] [Google Scholar]
- Homsher E., Mommaerts W. F., Ricchiuti N. V., Wallner A. Activation heat, activation metabolism and tension-related heat in frog semitendinosus muscles. J Physiol. 1972 Feb;220(3):601–625. doi: 10.1113/jphysiol.1972.sp009725. [DOI] [PMC free article] [PubMed] [Google Scholar]
- Izutsu K. T. Intracellular pH, H ion flux and H ion permeability coefficient in bullfrog toe muscle. J Physiol. 1972 Feb;221(1):15–27. doi: 10.1113/jphysiol.1972.sp009735. [DOI] [PMC free article] [PubMed] [Google Scholar]
- Jewell B. R., Kretzschmar M., Woledge R. C. Length and tension transducers. J Physiol. 1967 Jul;191(1):10P–12P. [PubMed] [Google Scholar]
- Moeschler H. J., Schaer J. J., Cox J. A. A thermodynamic analysis of the binding of calcium and magnesium ions to parvalbumin. Eur J Biochem. 1980 Oct;111(1):73–78. doi: 10.1111/j.1432-1033.1980.tb06076.x. [DOI] [PubMed] [Google Scholar]
- Nosek T. M., Fender K. Y., Godt R. E. It is diprotonated inorganic phosphate that depresses force in skinned skeletal muscle fibers. Science. 1987 Apr 10;236(4798):191–193. doi: 10.1126/science.3563496. [DOI] [PubMed] [Google Scholar]
- Ogawa Y. Calcium binding to troponin C and troponin: effects of Mg2+, ionic strength and pH. J Biochem. 1985 Apr;97(4):1011–1023. doi: 10.1093/oxfordjournals.jbchem.a135143. [DOI] [PubMed] [Google Scholar]
- Ogawa Y., Tanokura M. Kinetic studies of calcium binding to parvalbumins from bullfrog skeletal muscle. J Biochem. 1986 Jan;99(1):81–89. doi: 10.1093/oxfordjournals.jbchem.a135482. [DOI] [PubMed] [Google Scholar]
- Ogawa Y., Tanokura M. Steady-state properties of calcium binding to parvalbumins from bullfrog skeletal muscle: effects of Mg2+, pH, ionic strength, and temperature. J Biochem. 1986 Jan;99(1):73–80. doi: 10.1093/oxfordjournals.jbchem.a135481. [DOI] [PubMed] [Google Scholar]
- Peckham M., Woledge R. C. Labile heat and changes in rate of relaxation of frog muscles. J Physiol. 1986 May;374:123–135. doi: 10.1113/jphysiol.1986.sp016070. [DOI] [PMC free article] [PubMed] [Google Scholar]
- Roos A., Boron W. F. Intracellular pH. Physiol Rev. 1981 Apr;61(2):296–434. doi: 10.1152/physrev.1981.61.2.296. [DOI] [PubMed] [Google Scholar]
- Smith S. J., Woledge R. C. Thermodynamic analysis of calcium binding to frog parvalbumin. J Muscle Res Cell Motil. 1985 Dec;6(6):757–768. doi: 10.1007/BF00712240. [DOI] [PubMed] [Google Scholar]
- Stella G. The combination of carbon dioxide with muscle: its heat of neutralization and its dissociation curve. J Physiol. 1929 Aug 28;68(1):49–66. doi: 10.1113/jphysiol.1929.sp002595. [DOI] [PMC free article] [PubMed] [Google Scholar]
- Stull J. T., Buss J. E. Calcium binding properties of beef cardiac troponin. J Biol Chem. 1978 Sep 10;253(17):5932–5938. [PubMed] [Google Scholar]
- Tanokura M., Yamada K. A calorimetric study of Ca2+ binding to two major isotypes of bullfrog parvalbumin. FEBS Lett. 1985 Jun 3;185(1):165–169. doi: 10.1016/0014-5793(85)80763-8. [DOI] [PubMed] [Google Scholar]
- Yamada K., Tanokura M. Post-contractile phosphocreatine splitting in muscle as revealed by time-resolved 31P nuclear magnetic resonance. Jpn J Physiol. 1983;33(6):909–919. doi: 10.2170/jjphysiol.33.909. [DOI] [PubMed] [Google Scholar]