Abstract
1. The length dependence of Ca2+-activated tension within the ascending limb of the length-tension relationship, corresponding to sarcomere lengths below about 2.25 micron, was investigated in skinned fibres from rabbit psoas muscle. At high [Ca2+] a shallow phase and then a steep phase of tension decline were observed as sarcomere length was reduced, while at low [Ca2+] tension decreased monotonically with decreases in sarcomere length. The sarcomere length at which the ascending limb intersected zero tension was greater for lower concentrations of Ca2+. 2. The length tension relationship from maximally activated fibres changed when filament lattice spacing was reduced by osmotic compression. Relationships obtained in the presence of 5% (w/v) dextran T500 more distinctly demonstrated both the shallow and steep portions of the ascending limb than did relationships from untreated fibres. 3. As striation spacing was decreased a progressive decline in the Ca2+ sensitivity of tension development was observed. Tension-pCa relationships from both control and dextran-treated fibres underwent a rightward shift (i.e. to a higher [Ca2+]) by 0.23 pCa units as sarcomere length was reduced between 2.46 and 1.54 microns. 4. Fibre stiffness was studied by applying a 3.3 kHz sinusoidal length change at one end of the fibre and measuring the resultant tension change. At submaximal activation (pCa 5.8), stiffness increased relative to tension as sarcomere length was decreased below approximately 2.4 microns, suggesting that there is an activation-related internal load at low [Ca2+]. At maximal activation, a significant increase in this ratio occurred only at sarcomere lengths less than approximately 1.8 microns, and presumably involved collision of the thick filaments with the Z-lines. 5. Length-dependent changes in the Ca2+ sensitivity of tension development do not appear to be the result of shortening-induced dissociation of Ca2+ from troponin-C, the Ca2+ binding subunit of troponin. Fibres activated in the absence of Ca2+, by the partial removal of whole troponin complexes, produced length-tension relationships similar to those observed in the same fibres before troponin removal at a submaximal [Ca2+] yielding similar active tensions.
Full text
PDF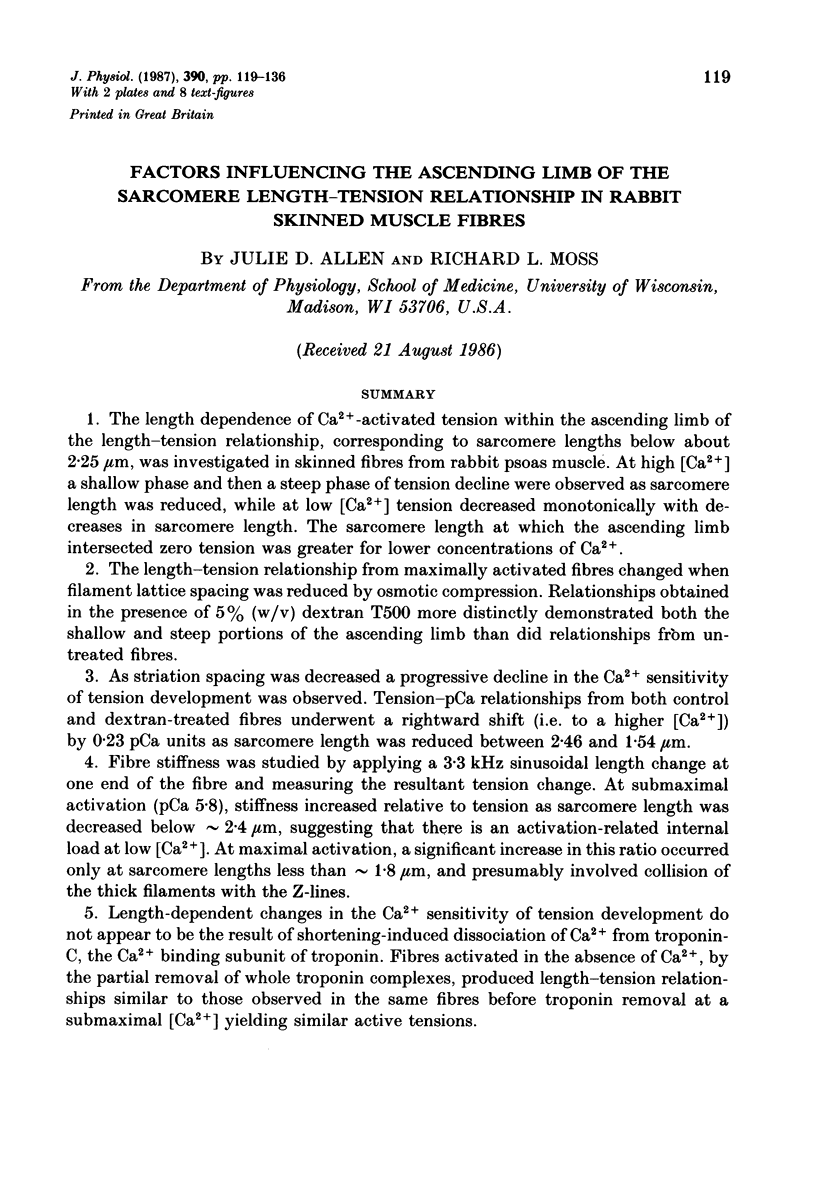
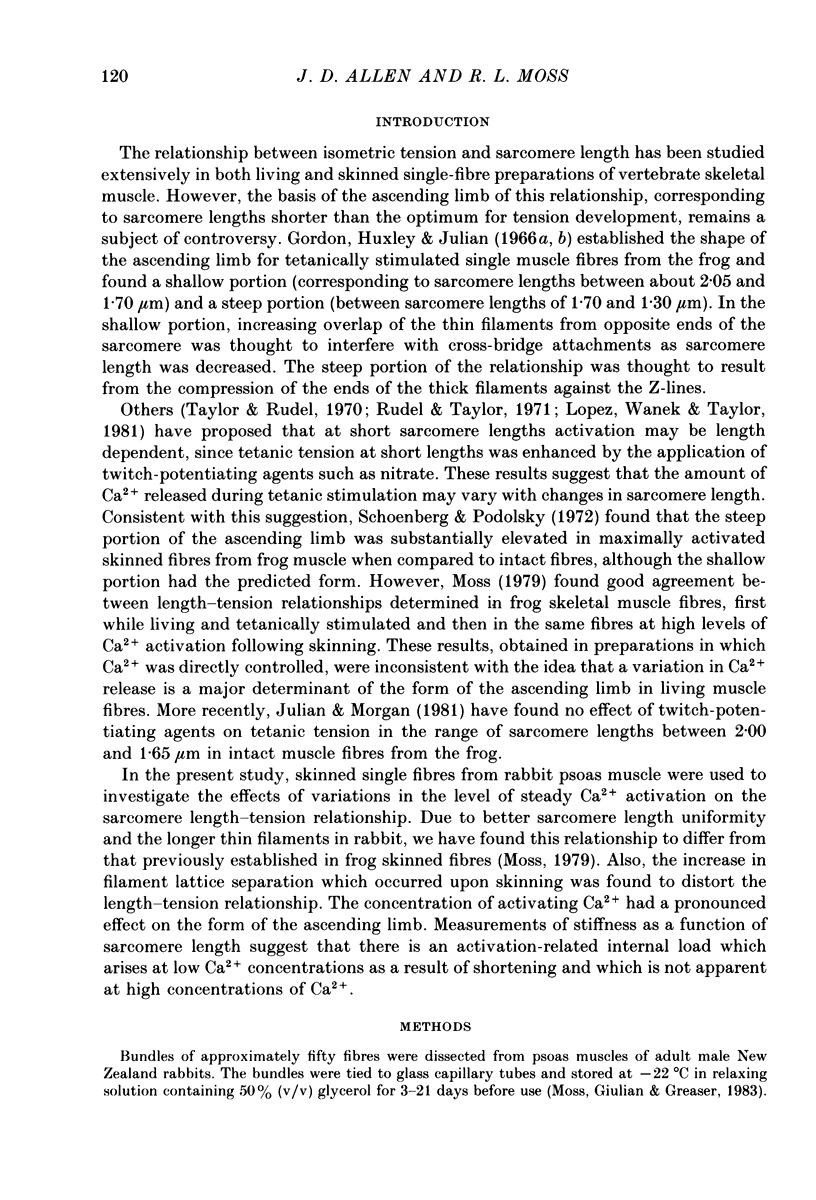
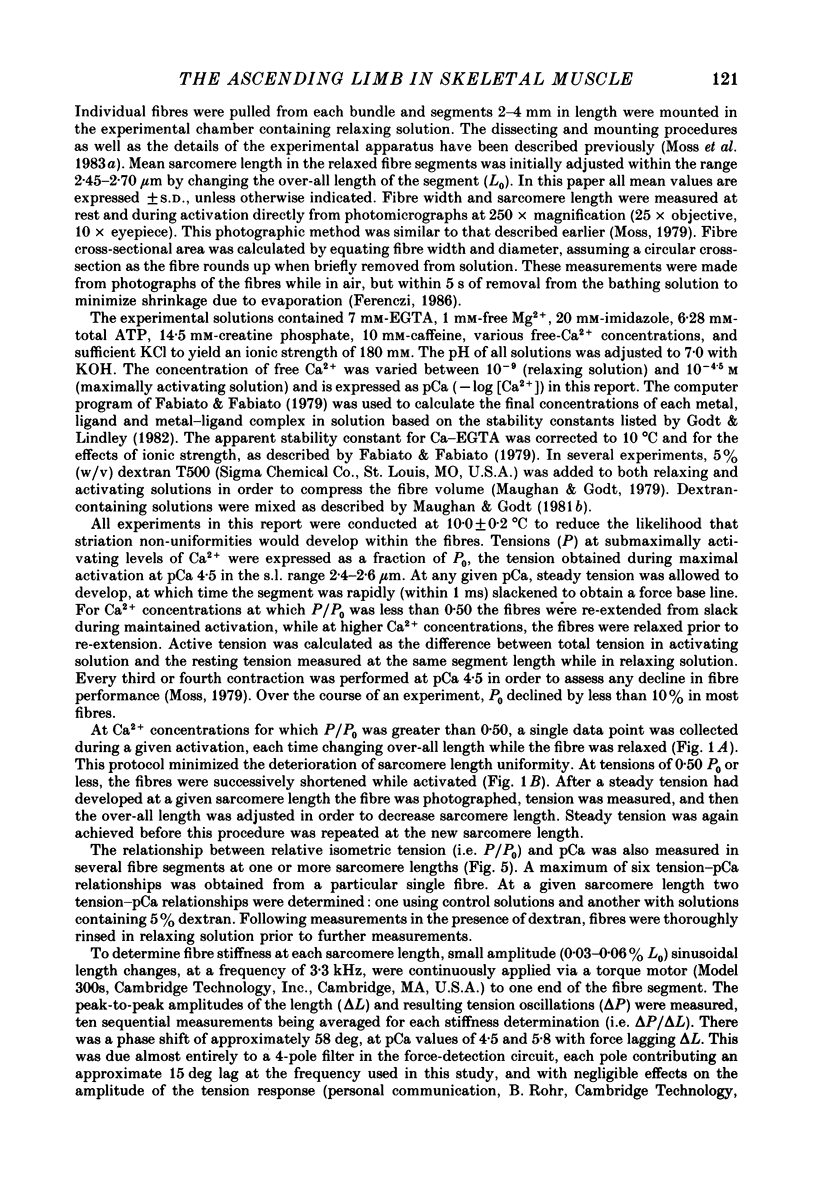
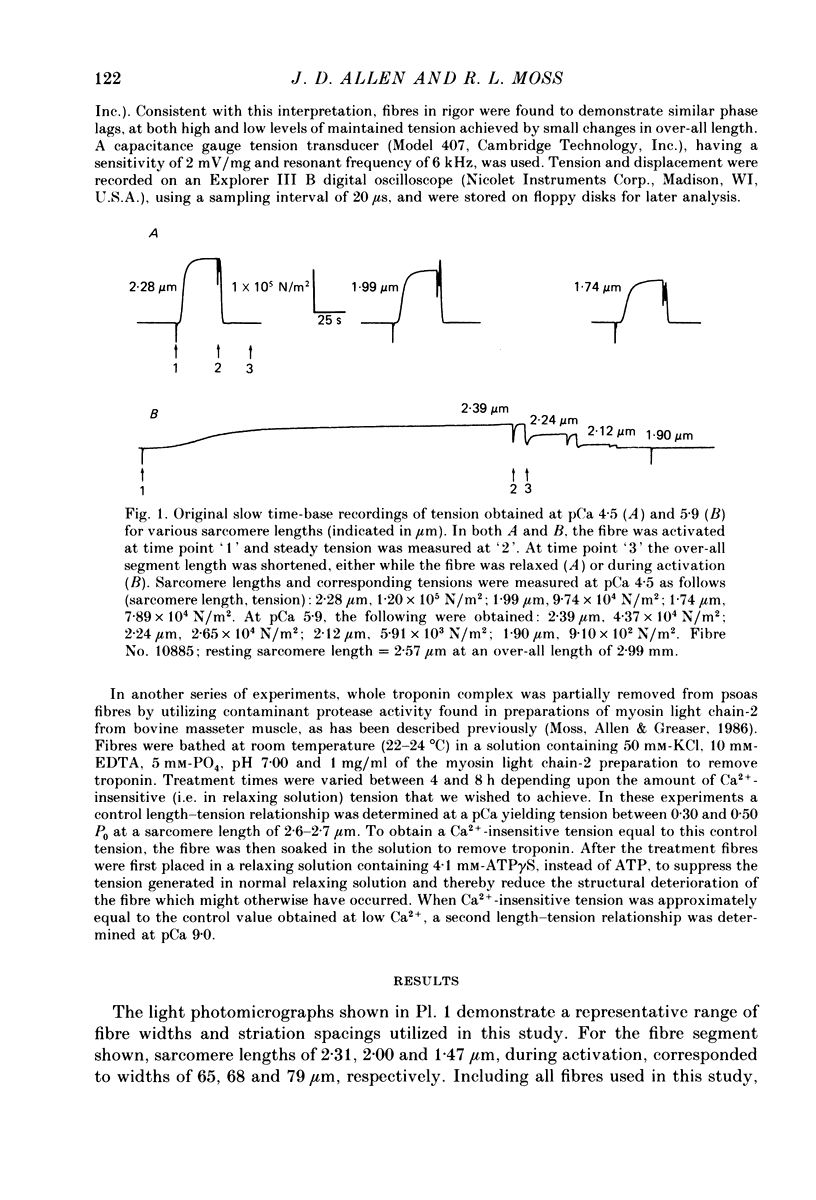
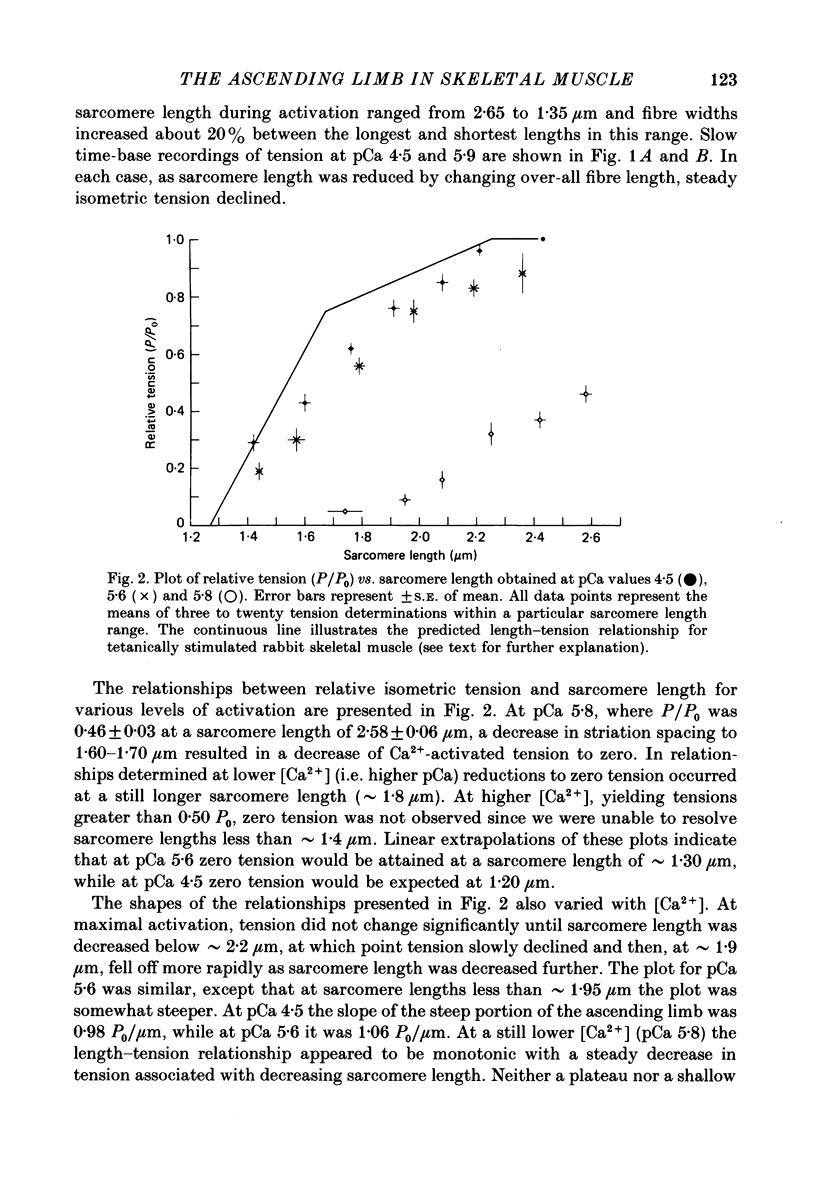
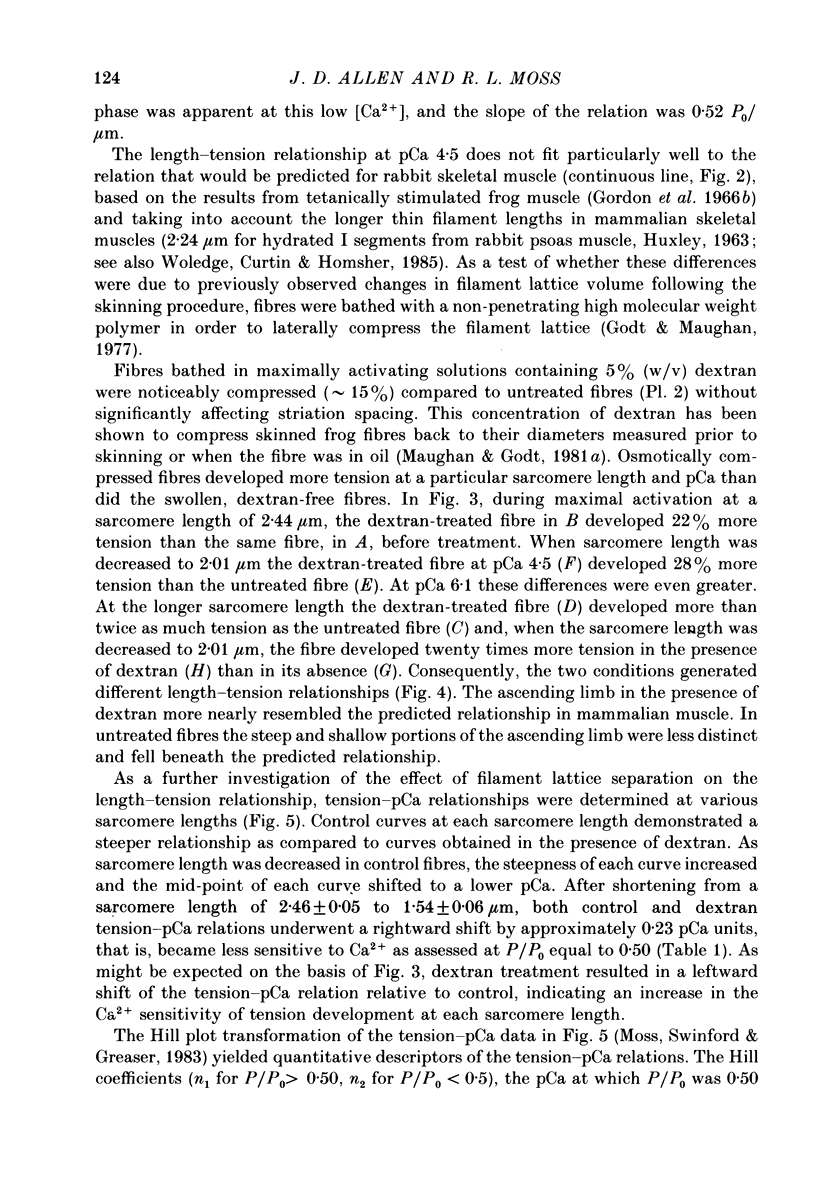
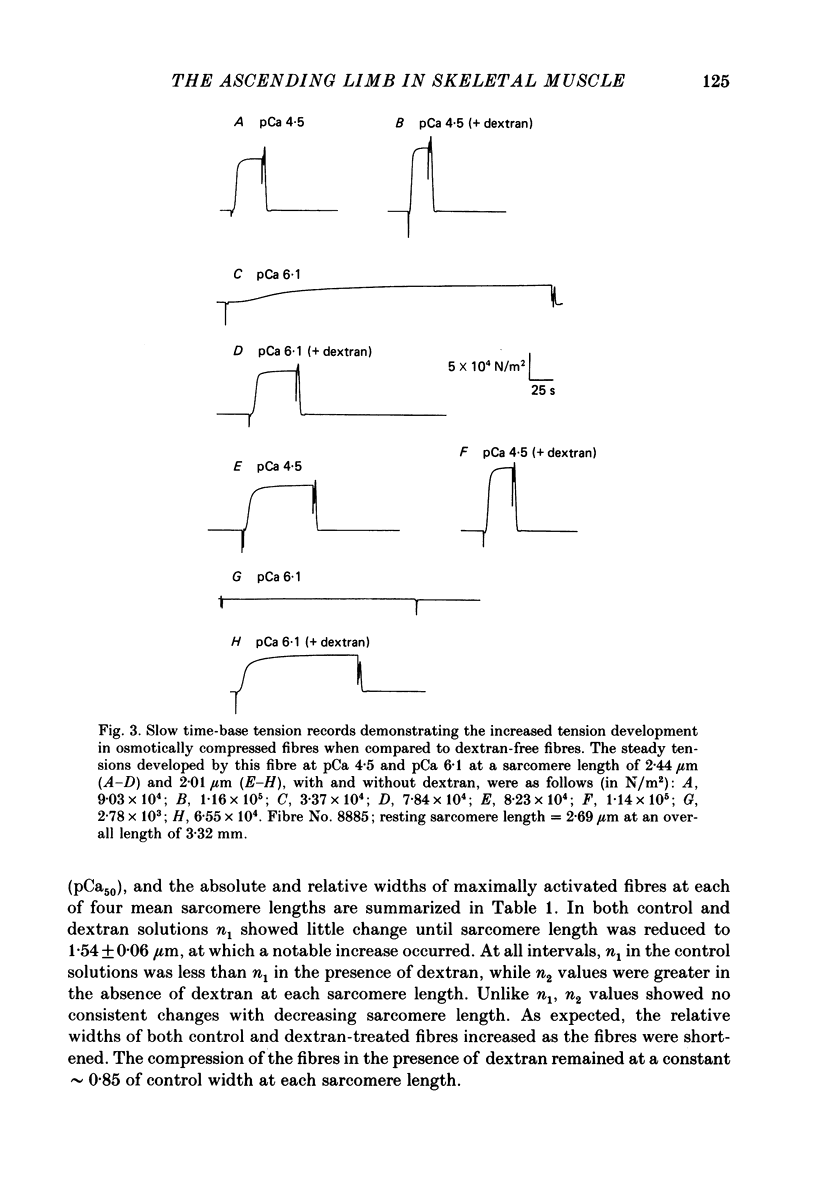
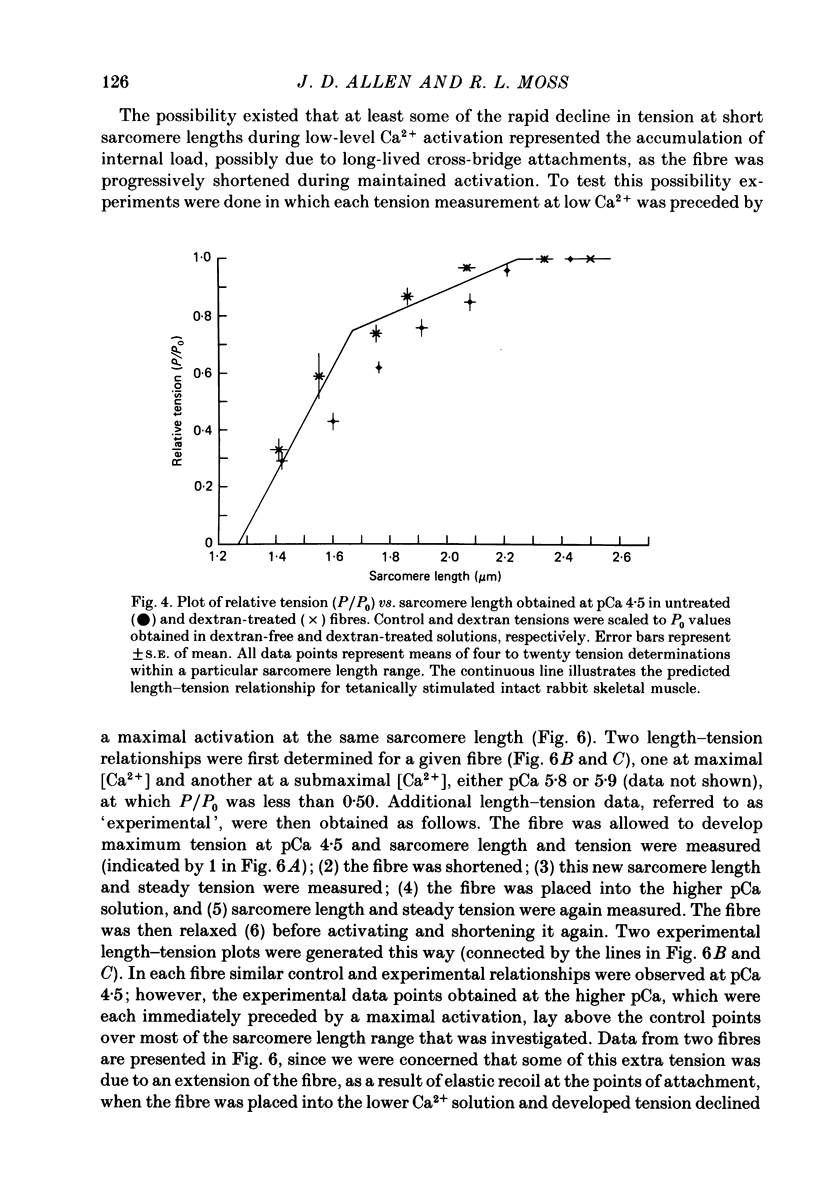
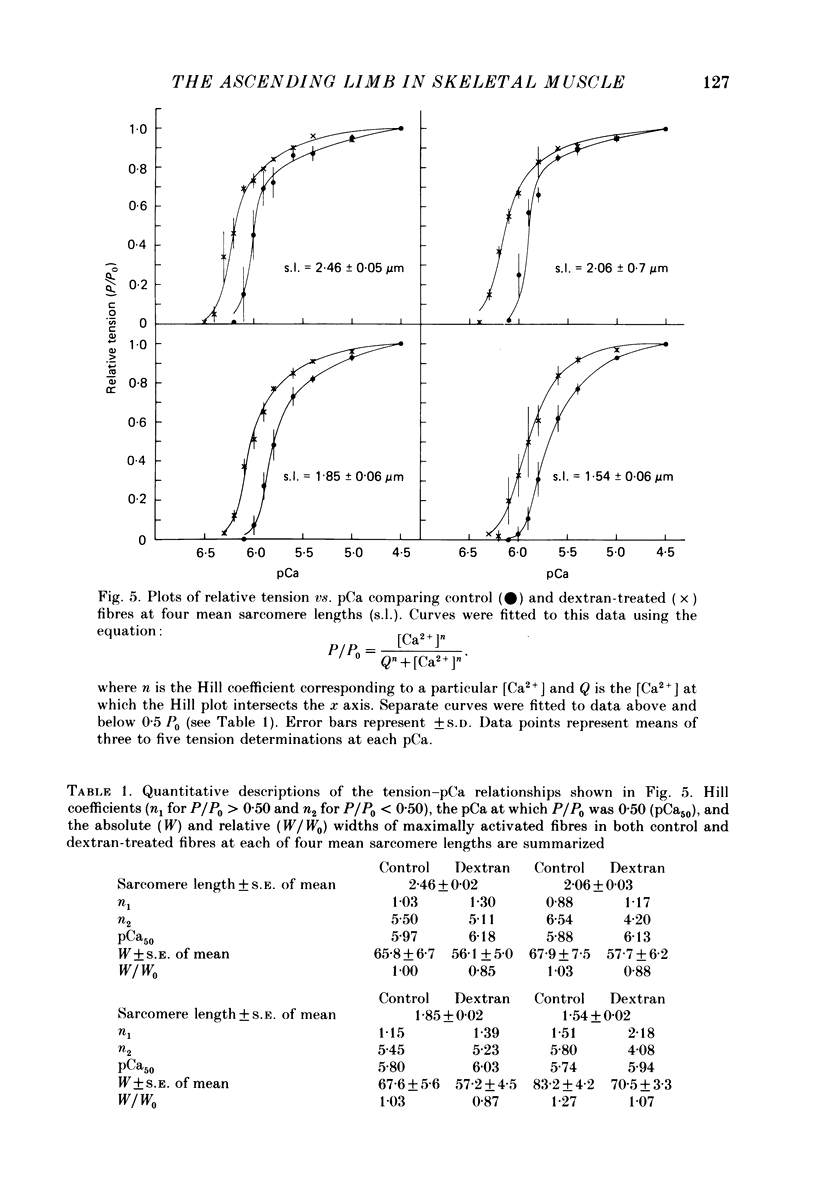
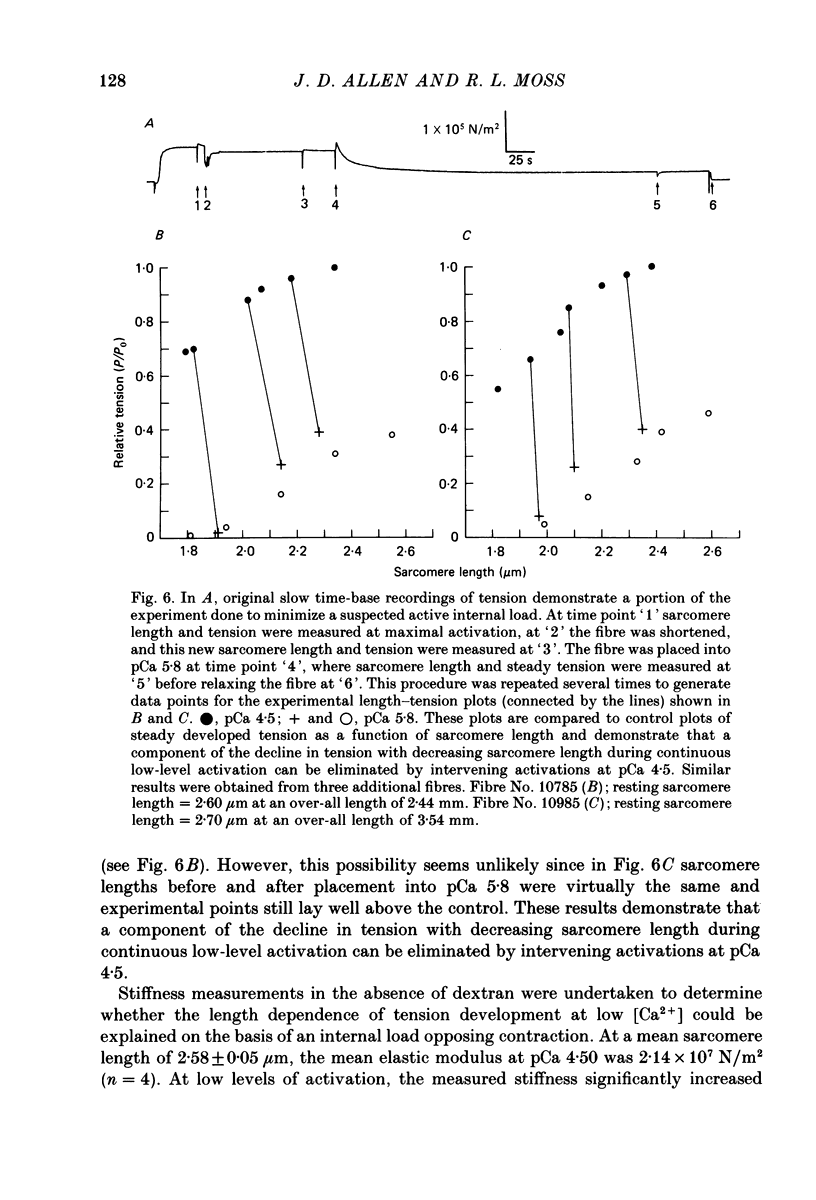
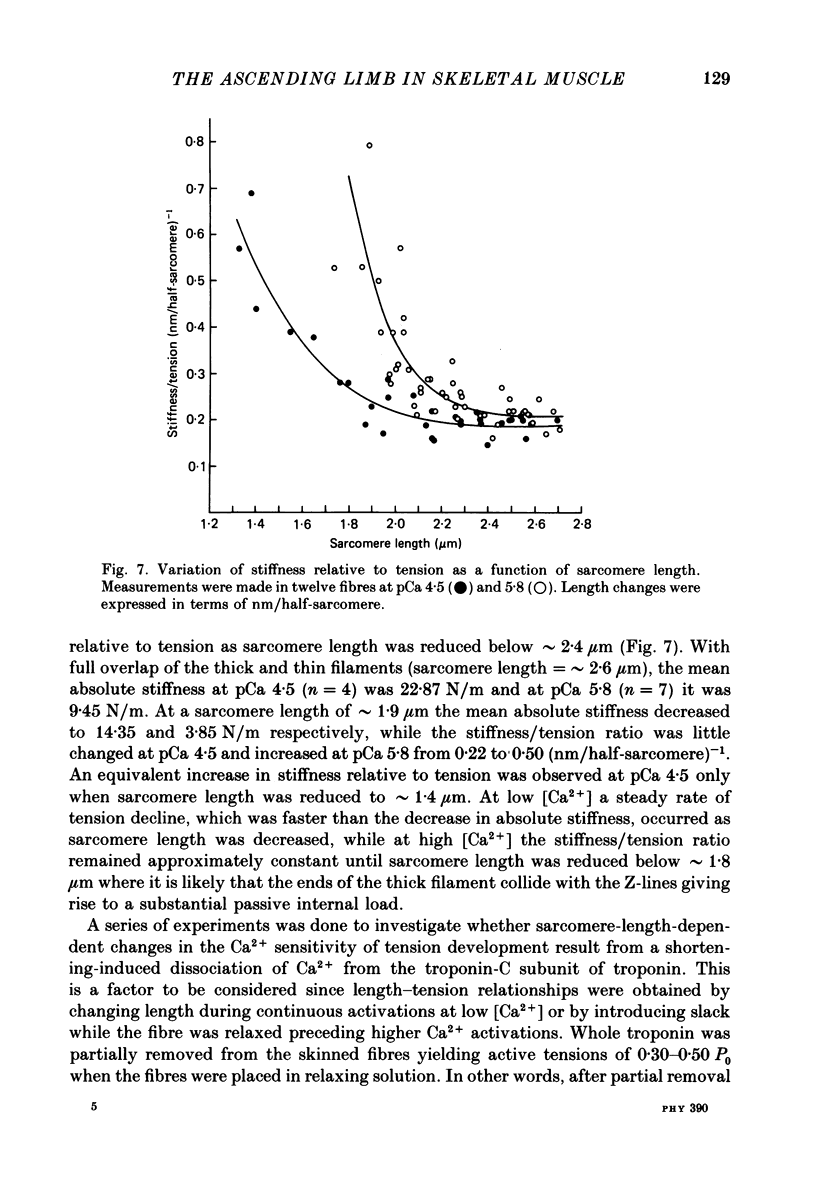
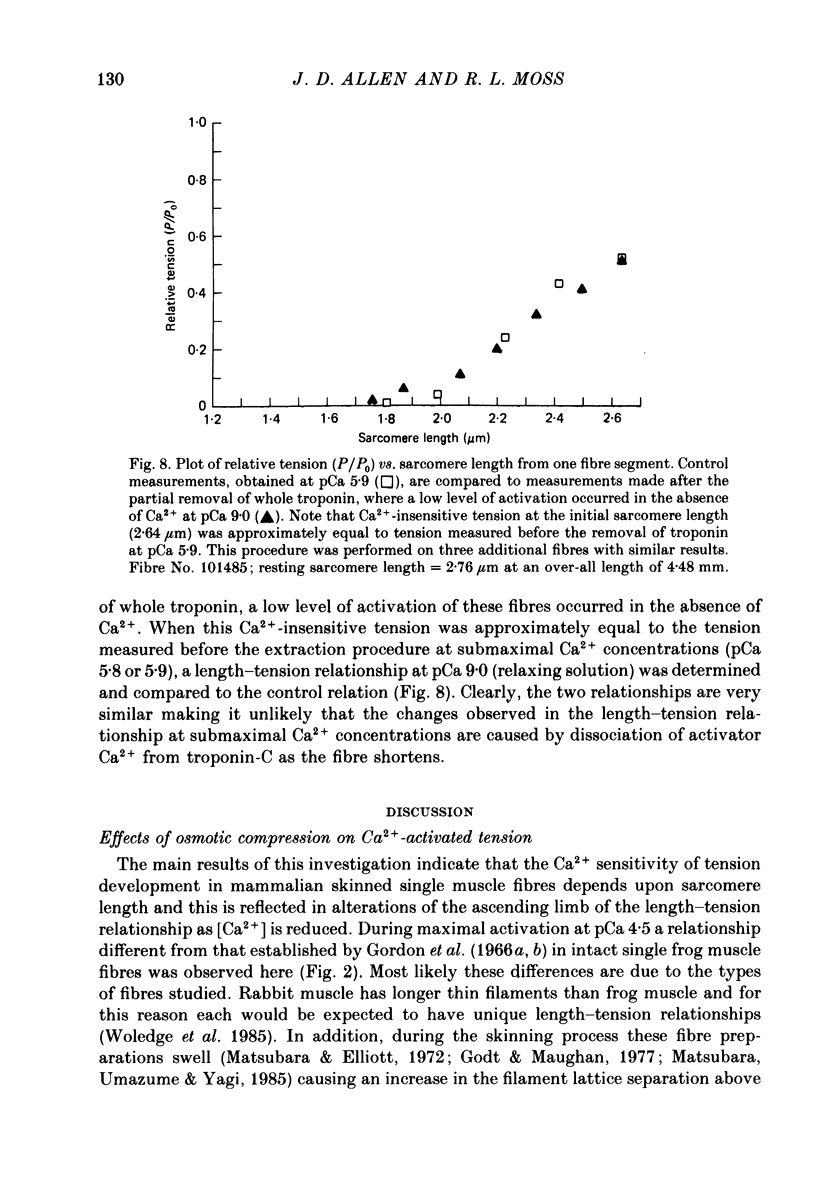
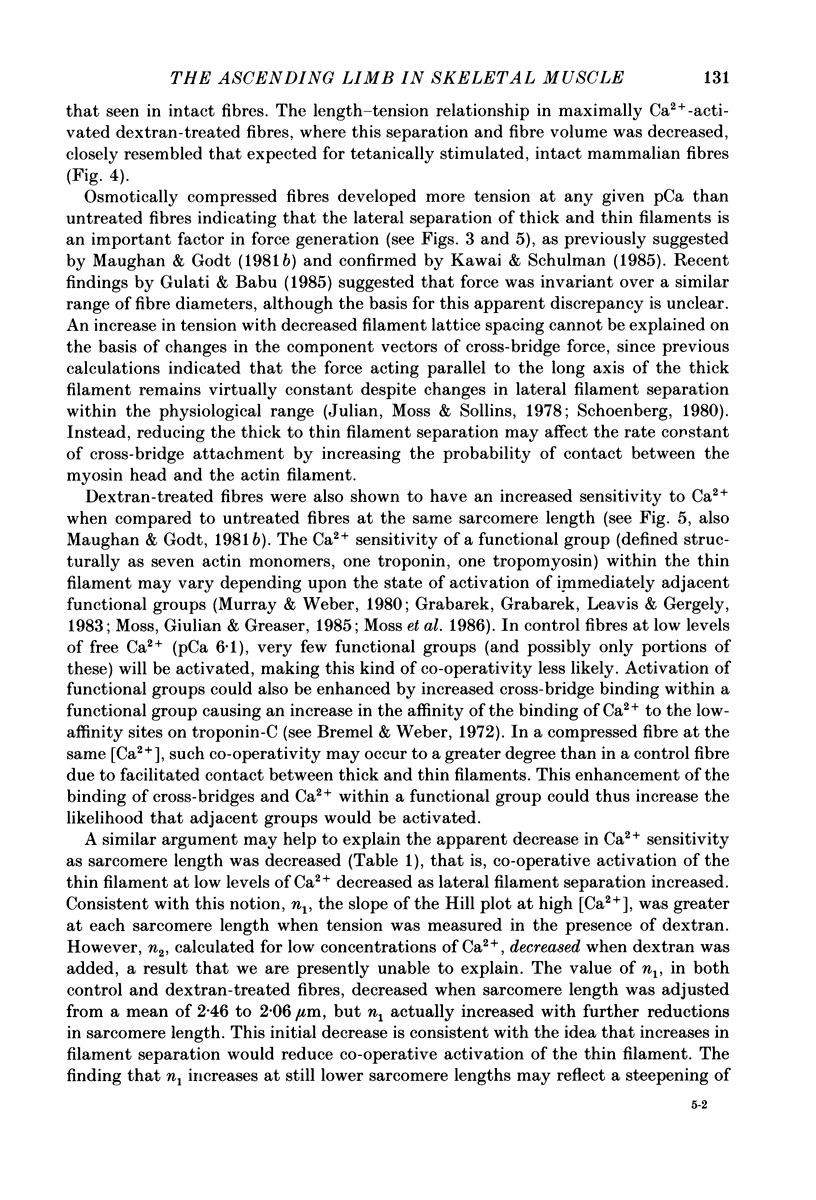
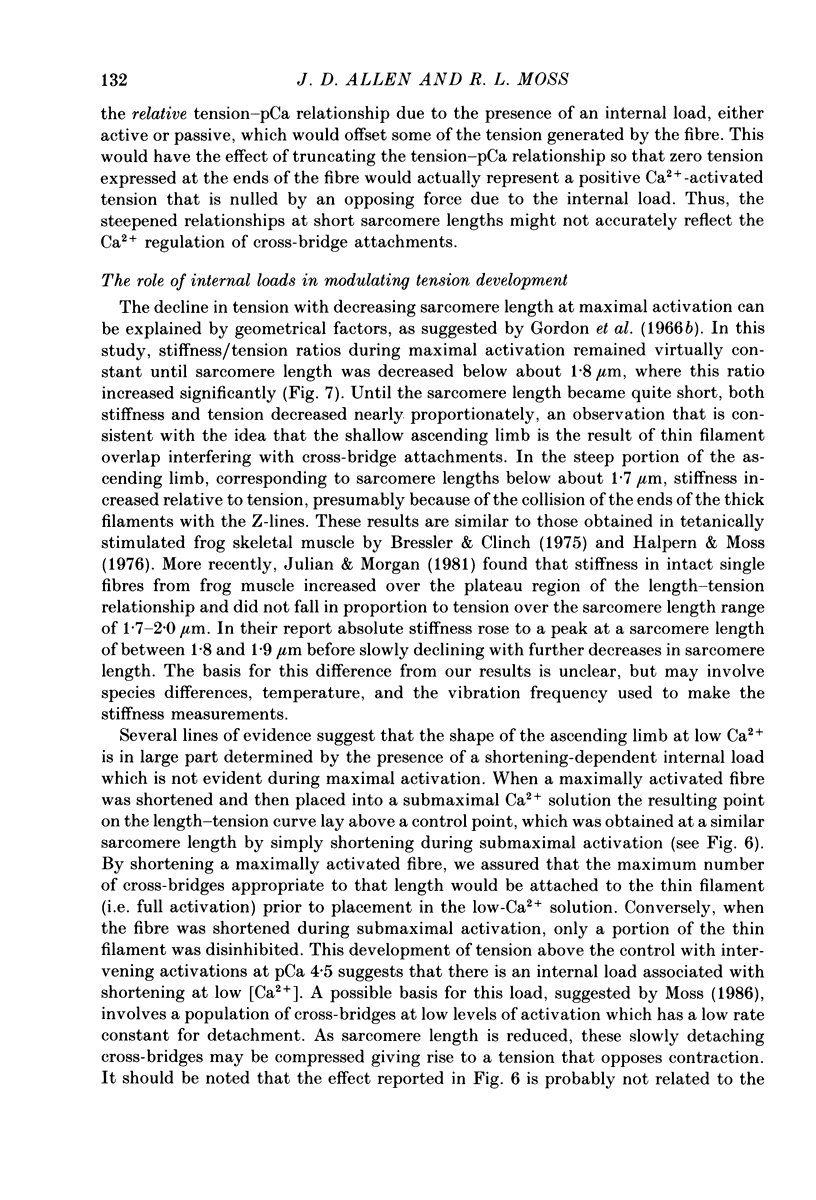
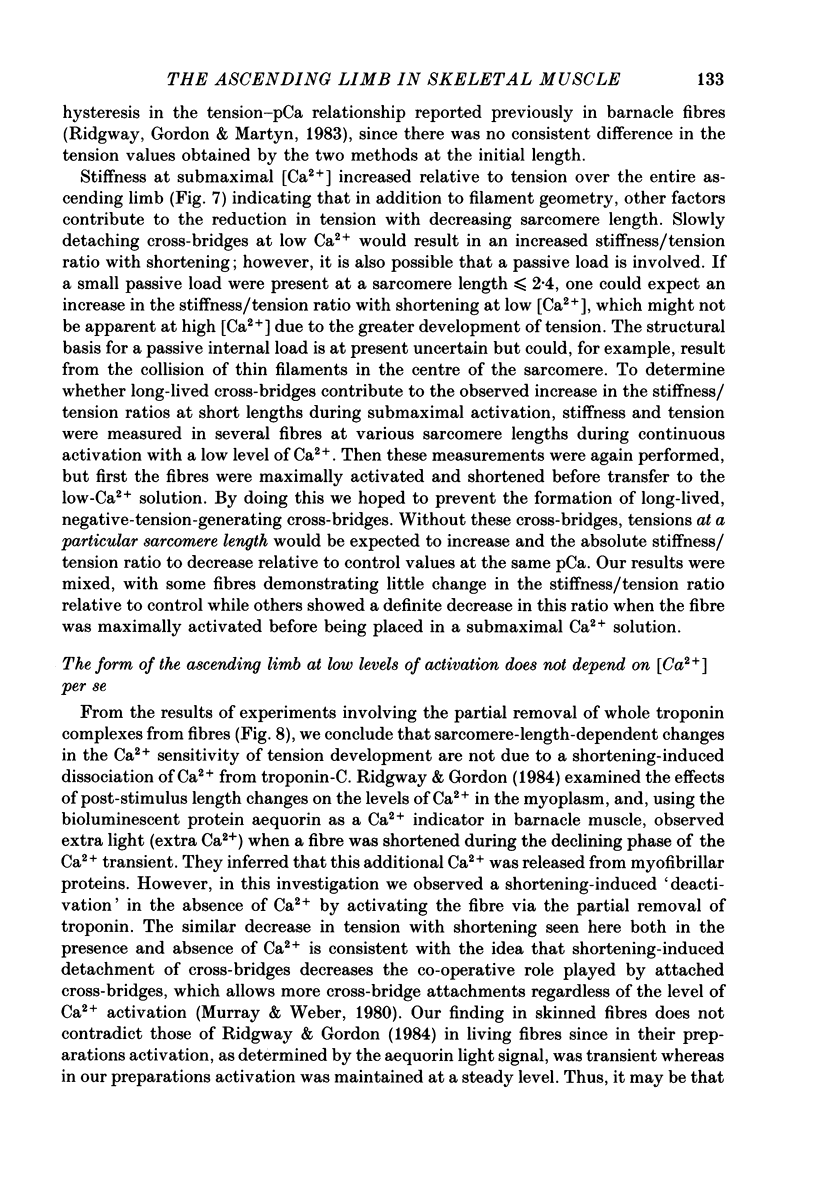
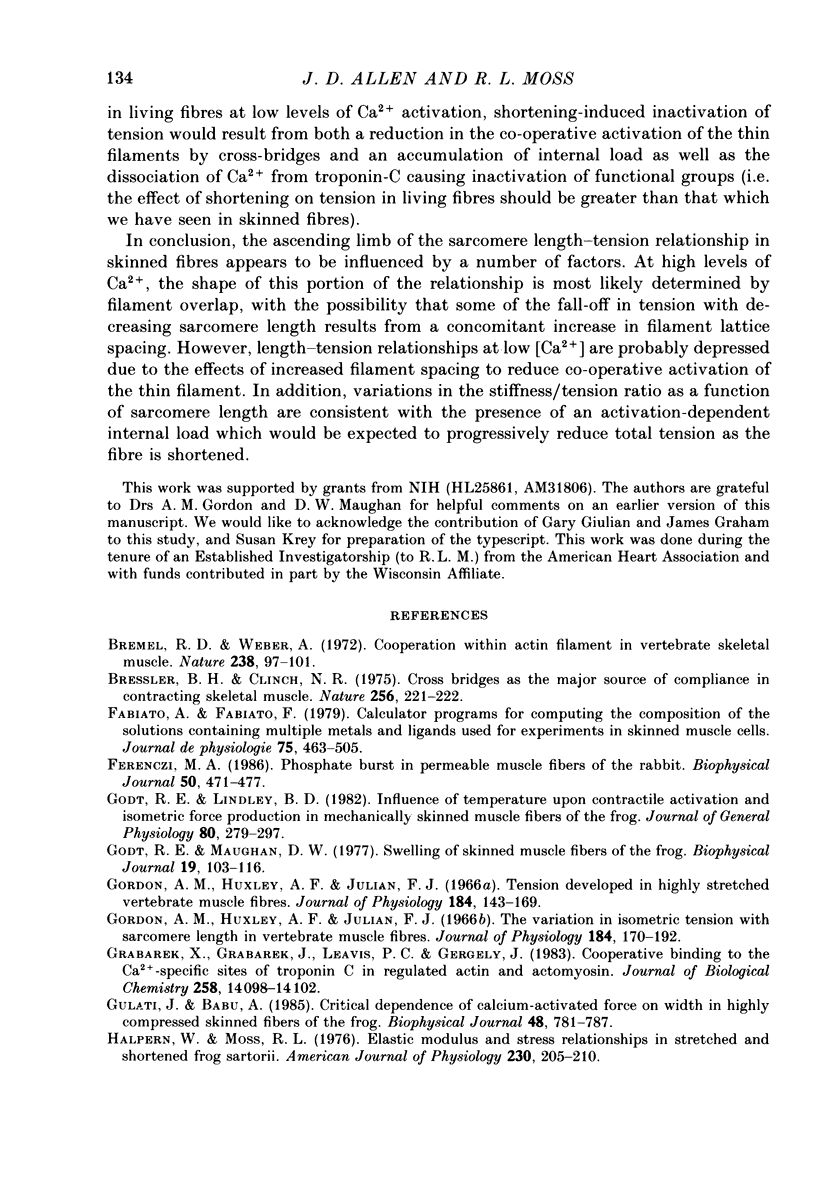
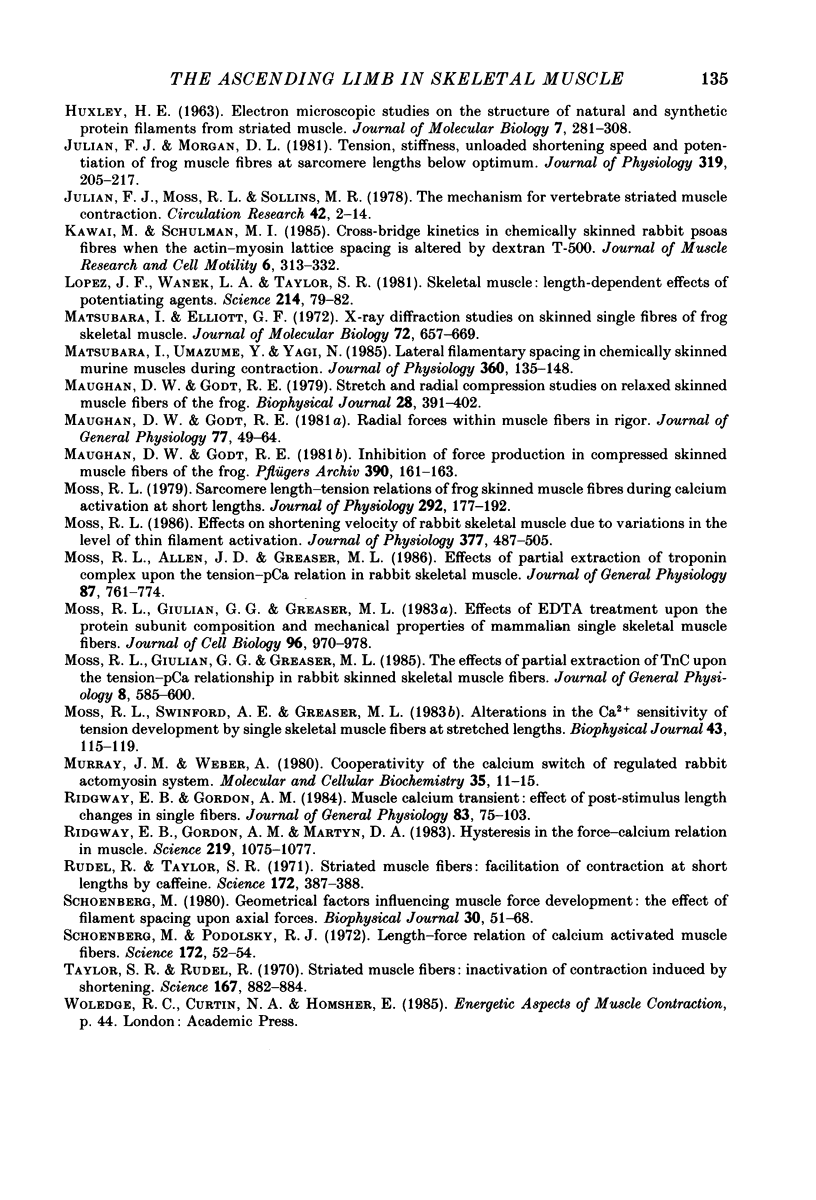
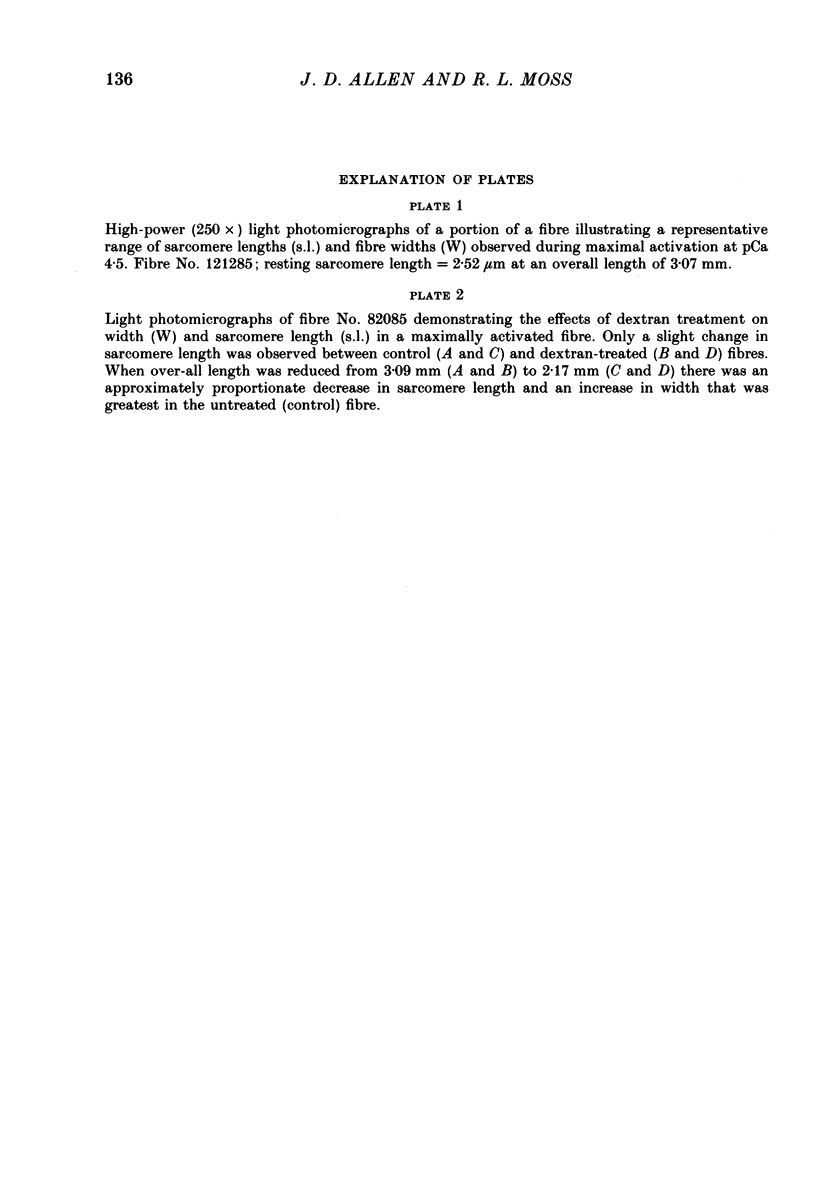
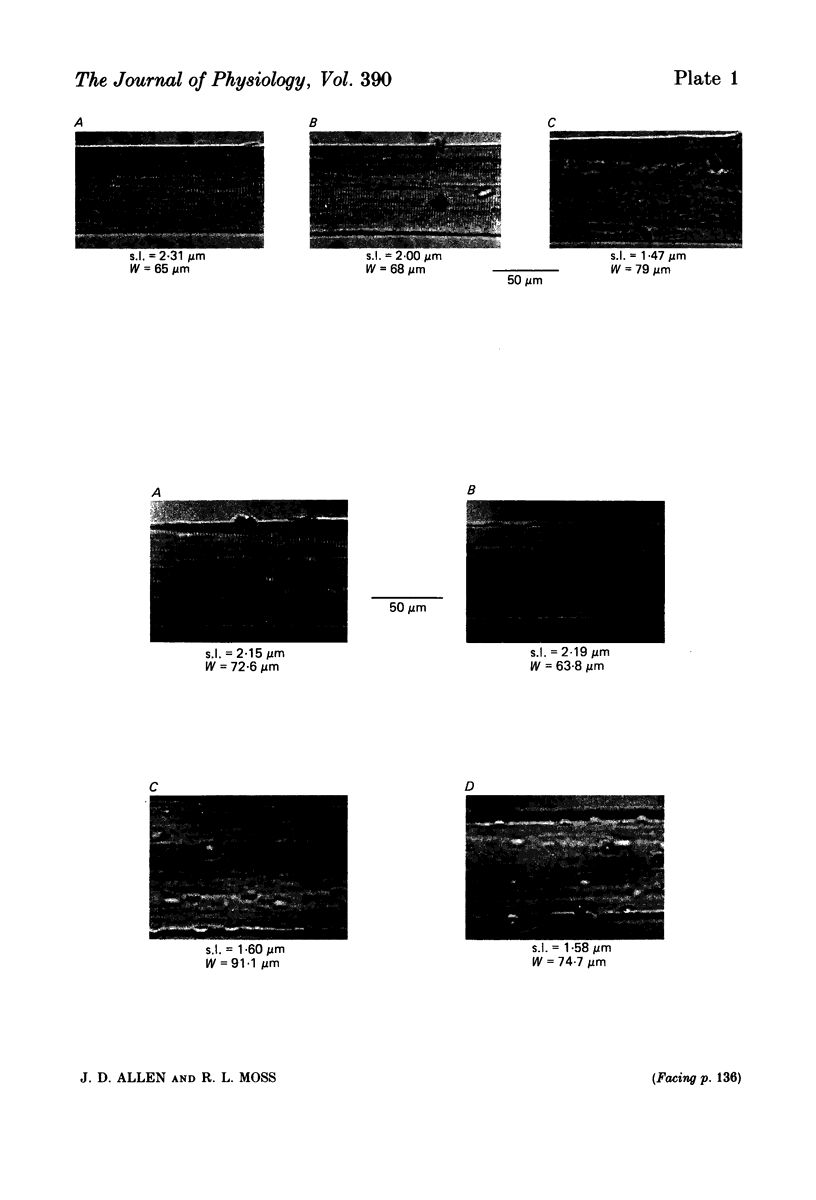
Images in this article
Selected References
These references are in PubMed. This may not be the complete list of references from this article.
- Bremel R. D., Weber A. Cooperation within actin filament in vertebrate skeletal muscle. Nat New Biol. 1972 Jul 26;238(82):97–101. doi: 10.1038/newbio238097a0. [DOI] [PubMed] [Google Scholar]
- Bressler B. H., Clinch N. F. Cross bridges as the major source of compliance in contracting skeletal muscle. Nature. 1975 Jul 17;256(5514):221–222. doi: 10.1038/256221a0. [DOI] [PubMed] [Google Scholar]
- Fabiato A., Fabiato F. Calculator programs for computing the composition of the solutions containing multiple metals and ligands used for experiments in skinned muscle cells. J Physiol (Paris) 1979;75(5):463–505. [PubMed] [Google Scholar]
- Ferenczi M. A. Phosphate burst in permeable muscle fibers of the rabbit. Biophys J. 1986 Sep;50(3):471–477. doi: 10.1016/S0006-3495(86)83484-1. [DOI] [PMC free article] [PubMed] [Google Scholar]
- Godt R. E., Lindley B. D. Influence of temperature upon contractile activation and isometric force production in mechanically skinned muscle fibers of the frog. J Gen Physiol. 1982 Aug;80(2):279–297. doi: 10.1085/jgp.80.2.279. [DOI] [PMC free article] [PubMed] [Google Scholar]
- Godt R. E., Maughan D. W. Swelling of skinned muscle fibers of the frog. Experimental observations. Biophys J. 1977 Aug;19(2):103–116. doi: 10.1016/S0006-3495(77)85573-2. [DOI] [PMC free article] [PubMed] [Google Scholar]
- Gordon A. M., Huxley A. F., Julian F. J. Tension development in highly stretched vertebrate muscle fibres. J Physiol. 1966 May;184(1):143–169. doi: 10.1113/jphysiol.1966.sp007908. [DOI] [PMC free article] [PubMed] [Google Scholar]
- Gordon A. M., Huxley A. F., Julian F. J. The variation in isometric tension with sarcomere length in vertebrate muscle fibres. J Physiol. 1966 May;184(1):170–192. doi: 10.1113/jphysiol.1966.sp007909. [DOI] [PMC free article] [PubMed] [Google Scholar]
- Grabarek Z., Grabarek J., Leavis P. C., Gergely J. Cooperative binding to the Ca2+-specific sites of troponin C in regulated actin and actomyosin. J Biol Chem. 1983 Dec 10;258(23):14098–14102. [PubMed] [Google Scholar]
- Gulati J., Babu A. Critical dependence of calcium-activated force on width in highly compressed skinned fibers of the frog. Biophys J. 1985 Nov;48(5):781–787. doi: 10.1016/S0006-3495(85)83836-4. [DOI] [PMC free article] [PubMed] [Google Scholar]
- Halpern W., Moss R. L. Elastic modulus and stress relationships in stretched and shortened frog sartorii. Am J Physiol. 1976 Jan;230(1):205–210. doi: 10.1152/ajplegacy.1976.230.1.205. [DOI] [PubMed] [Google Scholar]
- Julian F. J., Morgan D. L. Tension, stiffness, unloaded shortening speed and potentiation of frog muscle fibres at sarcomere lengths below optimum. J Physiol. 1981;319:205–217. doi: 10.1113/jphysiol.1981.sp013902. [DOI] [PMC free article] [PubMed] [Google Scholar]
- Julian F. J., Moss R. L., Sollins M. R. The mechanism for vertebrate striated muscle contraction. Circ Res. 1978 Jan;42(1):2–14. doi: 10.1161/01.res.42.1.2. [DOI] [PubMed] [Google Scholar]
- Kawai M., Schulman M. I. Crossbridge kinetics in chemically skinned rabbit psoas fibres when the actin-myosin lattice spacing is altered by dextran T-500. J Muscle Res Cell Motil. 1985 Jun;6(3):313–332. doi: 10.1007/BF00713172. [DOI] [PubMed] [Google Scholar]
- Lopez J. R., Wanek L. A., Taylor S. R. Skeletal muscle: length-dependent effects of potentiating agents. Science. 1981 Oct 2;214(4516):79–82. doi: 10.1126/science.6974399. [DOI] [PubMed] [Google Scholar]
- Matsubara I., Elliott G. F. X-ray diffraction studies on skinned single fibres of frog skeletal muscle. J Mol Biol. 1972 Dec 30;72(3):657–669. doi: 10.1016/0022-2836(72)90183-0. [DOI] [PubMed] [Google Scholar]
- Matsubara I., Umazume Y., Yagi N. Lateral filamentary spacing in chemically skinned murine muscles during contraction. J Physiol. 1985 Mar;360:135–148. doi: 10.1113/jphysiol.1985.sp015608. [DOI] [PMC free article] [PubMed] [Google Scholar]
- Maughan D. W., Godt R. E. Inhibition of force production in compressed skinned muscle fibers of the frog. Pflugers Arch. 1981 May;390(2):161–163. doi: 10.1007/BF00590200. [DOI] [PubMed] [Google Scholar]
- Maughan D. W., Godt R. E. Radial forces within muscle fibers in rigor. J Gen Physiol. 1981 Jan;77(1):49–64. doi: 10.1085/jgp.77.1.49. [DOI] [PMC free article] [PubMed] [Google Scholar]
- Maughan D. W., Godt R. E. Stretch and radial compression studies on relaxed skinned muscle fibers of the frog. Biophys J. 1979 Dec;28(3):391–402. doi: 10.1016/S0006-3495(79)85188-7. [DOI] [PMC free article] [PubMed] [Google Scholar]
- Moss R. L., Allen J. D., Greaser M. L. Effects of partial extraction of troponin complex upon the tension-pCa relation in rabbit skeletal muscle. Further evidence that tension development involves cooperative effects within the thin filament. J Gen Physiol. 1986 May;87(5):761–774. doi: 10.1085/jgp.87.5.761. [DOI] [PMC free article] [PubMed] [Google Scholar]
- Moss R. L. Effects on shortening velocity of rabbit skeletal muscle due to variations in the level of thin-filament activation. J Physiol. 1986 Aug;377:487–505. doi: 10.1113/jphysiol.1986.sp016199. [DOI] [PMC free article] [PubMed] [Google Scholar]
- Moss R. L., Giulian G. G., Greaser M. L. Effects of EDTA treatment upon the protein subunit composition and mechanical properties of mammalian single skeletal muscle fibers. J Cell Biol. 1983 Apr;96(4):970–978. doi: 10.1083/jcb.96.4.970. [DOI] [PMC free article] [PubMed] [Google Scholar]
- Moss R. L., Giulian G. G., Greaser M. L. The effects of partial extraction of TnC upon the tension-pCa relationship in rabbit skinned skeletal muscle fibers. J Gen Physiol. 1985 Oct;86(4):585–600. doi: 10.1085/jgp.86.4.585. [DOI] [PMC free article] [PubMed] [Google Scholar]
- Moss R. L. Sarcomere length-tension relations of frog skinned muscle fibres during calcium activation at short lengths. J Physiol. 1979 Jul;292:177–192. doi: 10.1113/jphysiol.1979.sp012845. [DOI] [PMC free article] [PubMed] [Google Scholar]
- Moss R. L., Swinford A. E., Greaser M. L. Alterations in the Ca2+ sensitivity of tension development by single skeletal muscle fibers at stretched lengths. Biophys J. 1983 Jul;43(1):115–119. doi: 10.1016/S0006-3495(83)84329-X. [DOI] [PMC free article] [PubMed] [Google Scholar]
- Murray J. M., Weber A. Cooperativity of the calcium switch of regulated rabbit actomyosin system. Mol Cell Biochem. 1981 Feb 26;35(1):11–15. doi: 10.1007/BF02358183. [DOI] [PubMed] [Google Scholar]
- Ridgway E. B., Gordon A. M., Martyn D. A. Hysteresis in the force-calcium relation in muscle. Science. 1983 Mar 4;219(4588):1075–1077. doi: 10.1126/science.6823567. [DOI] [PubMed] [Google Scholar]
- Ridgway E. B., Gordon A. M. Muscle calcium transient. Effect of post-stimulus length changes in single fibers. J Gen Physiol. 1984 Jan;83(1):75–103. doi: 10.1085/jgp.83.1.75. [DOI] [PMC free article] [PubMed] [Google Scholar]
- Rüdel R., Taylor S. R. Striated muscle fibers: facilitation of contraction at short lengths by caffeine. Science. 1971 Apr 23;172(3981):387–389. doi: 10.1126/science.172.3981.387. [DOI] [PubMed] [Google Scholar]
- Schoenberg M. Geometrical factors influencing muscle force development. I. The effect of filament spacing upon axial forces. Biophys J. 1980 Apr;30(1):51–67. doi: 10.1016/S0006-3495(80)85076-4. [DOI] [PMC free article] [PubMed] [Google Scholar]
- Schoenberg M., Podolsky R. J. Length-force relation of calcium activated muscle fibers. Science. 1972 Apr 7;176(4030):52–54. doi: 10.1126/science.176.4030.52. [DOI] [PubMed] [Google Scholar]
- Taylor S. R., Rüdel R. Striated muscle fibers: inactivation of contraction induced by shortening. Science. 1970 Feb 6;167(3919):882–884. doi: 10.1126/science.167.3919.882. [DOI] [PubMed] [Google Scholar]