Abstract
1. Radioactive amino acid, either [3H]leucine or [3H]proline, was injected into neurone R2 in the abdominal ganglion of Aplysia kurodai to investigate the intra-axonal transport of protein in a single axon. 2. Some of the injected amino acid which was not utilized for protein synthesis diffused intra-axonally with a diffusion constant of 6.0 X 10(-6) cm2/s (25 degrees C), which is the value expected from the known diffusion constants of acetylcholine and gamma-aminobutyric acid in the axoplasm (Koike & Nagata, 1979). The true length of the zigzagging axon in the nerve bundle was measured by the diffusion distances. 3. The radioactive proteins newly synthesized in the cell body from either of the injected amino acid were transported axonally in a single axon of R2. 4. Elongation of the axon resulted in a suppression of the fast axonal transport of the proteins and the amount of protein transported. This contrasts with the observation that action potential propagation along the elongated axon never failed. 5. A possible site for the suppression of the axonal transport would be axonal microtubules whose structure is not likely to be resistant to the distortion caused by the elongation.
Full text
PDF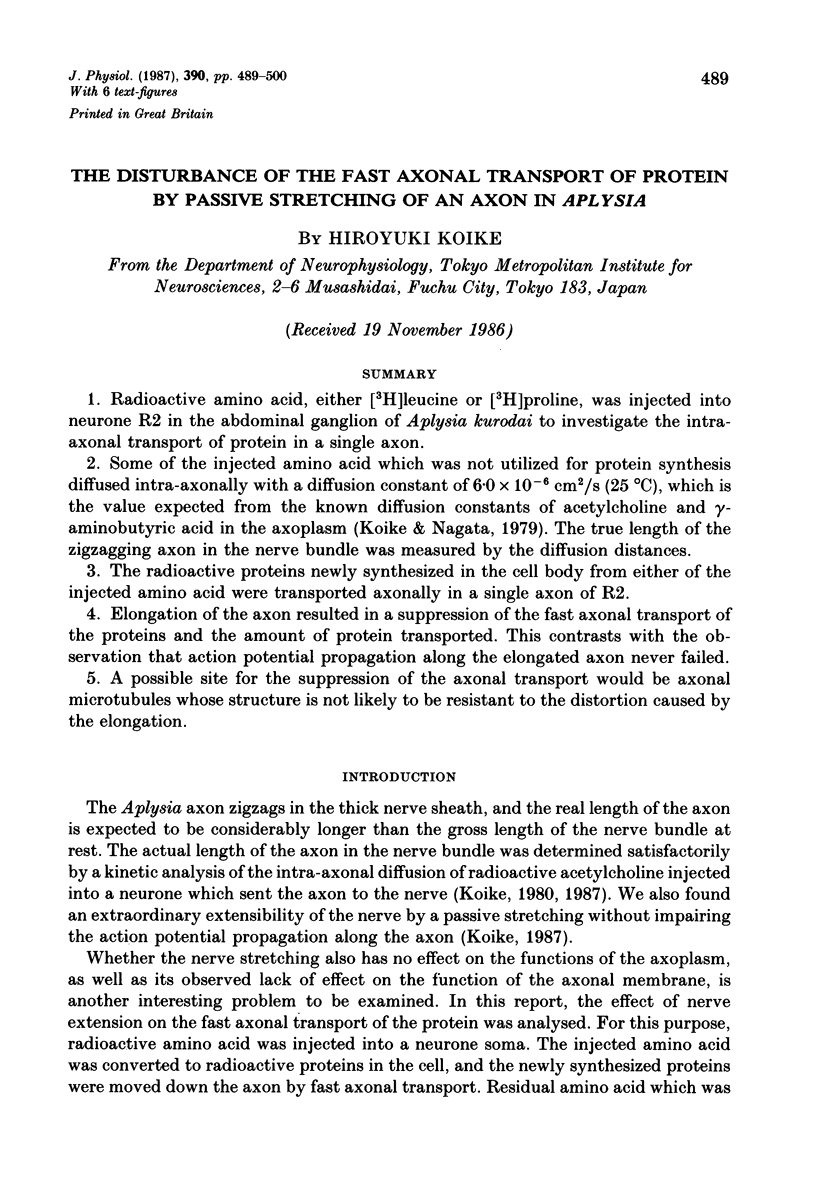
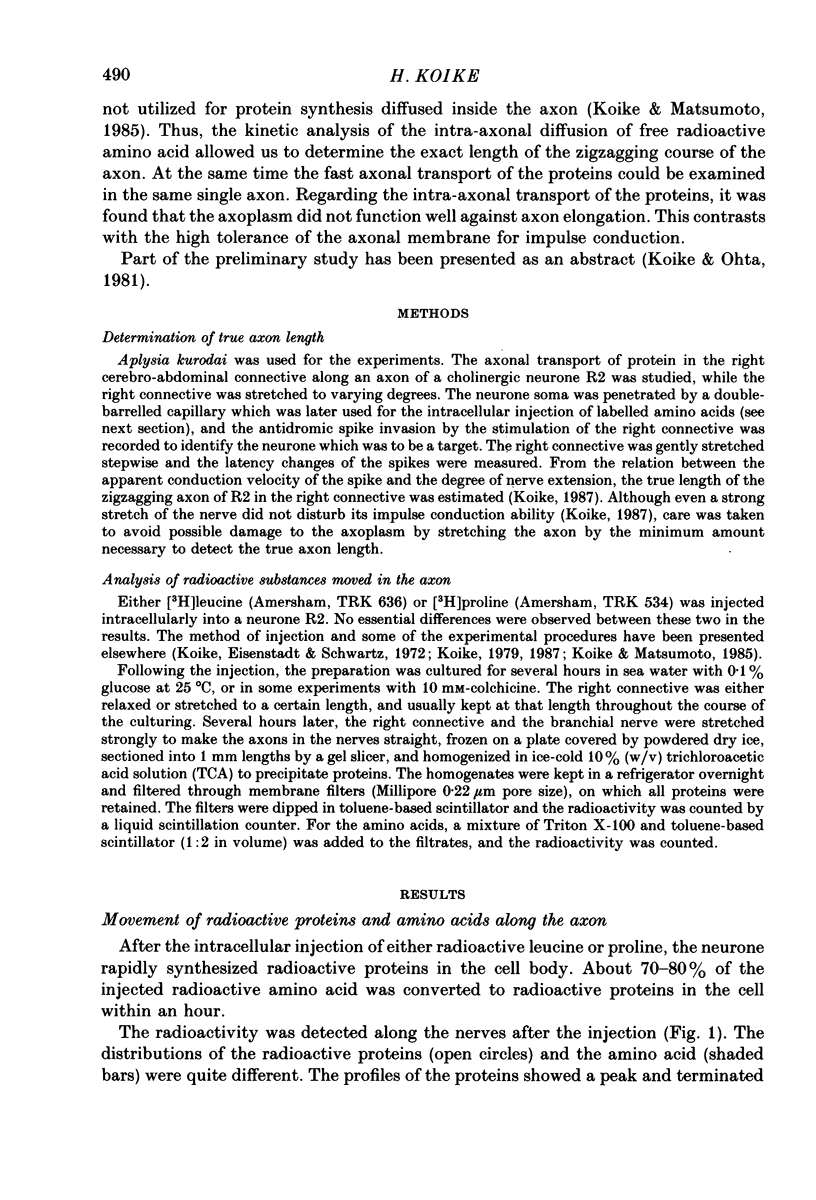
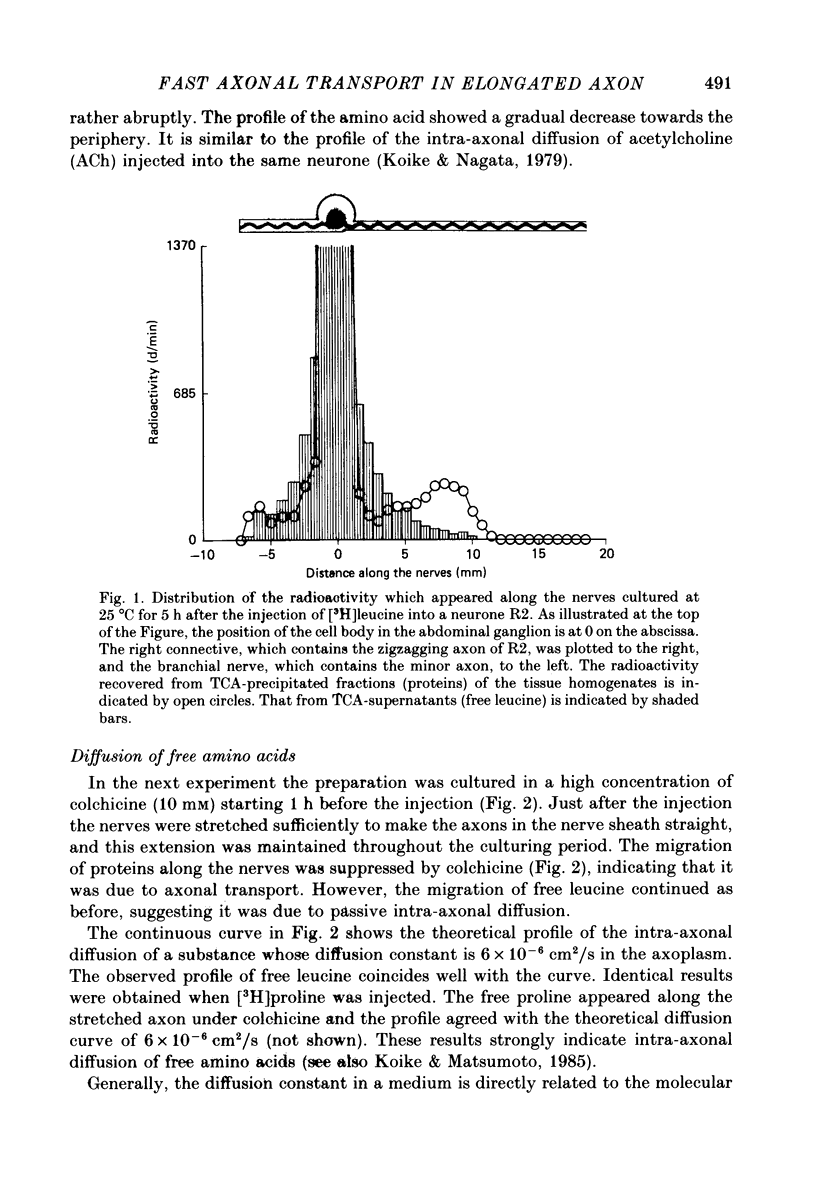
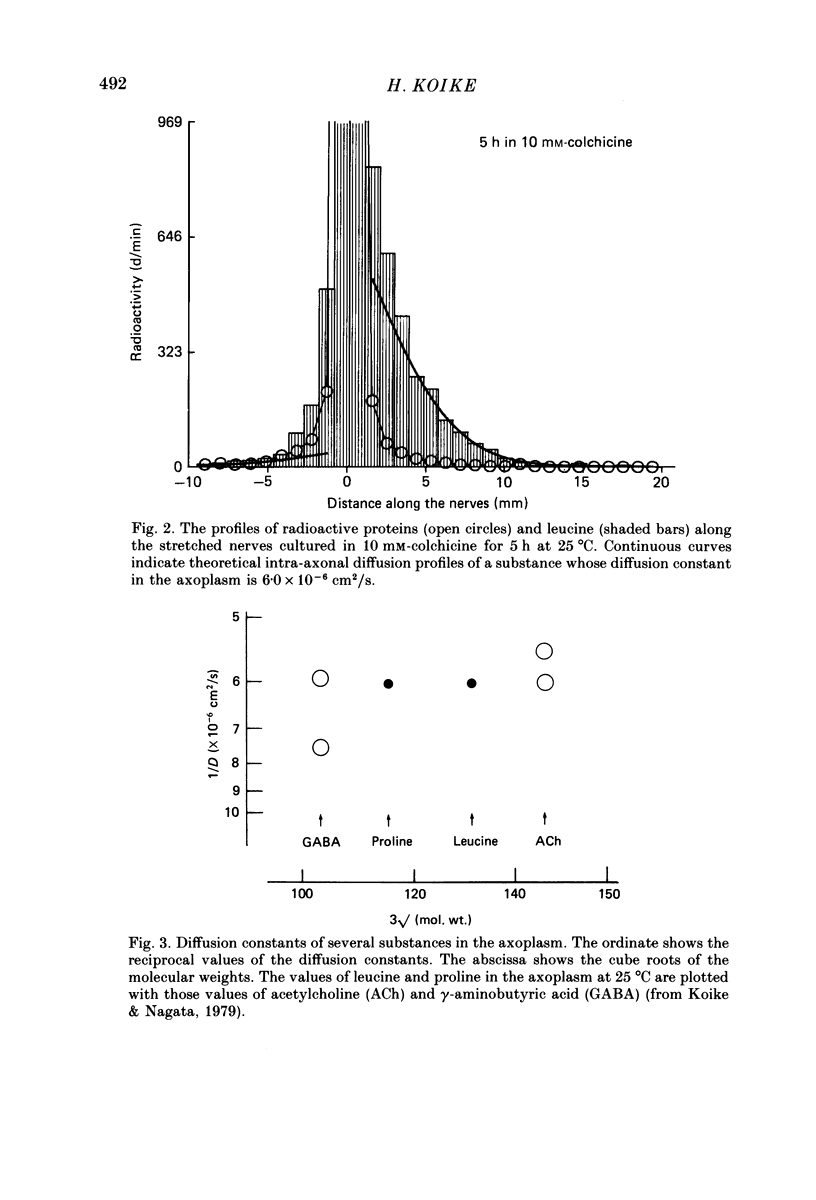
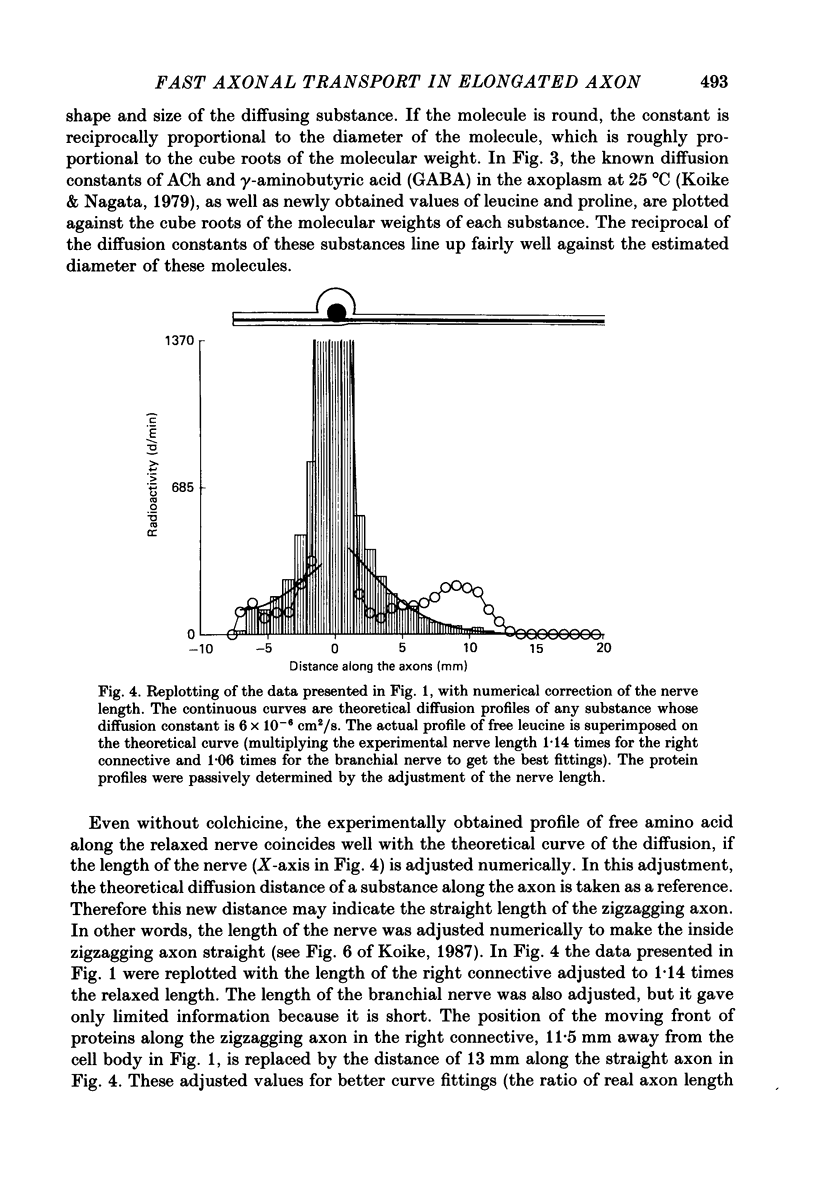
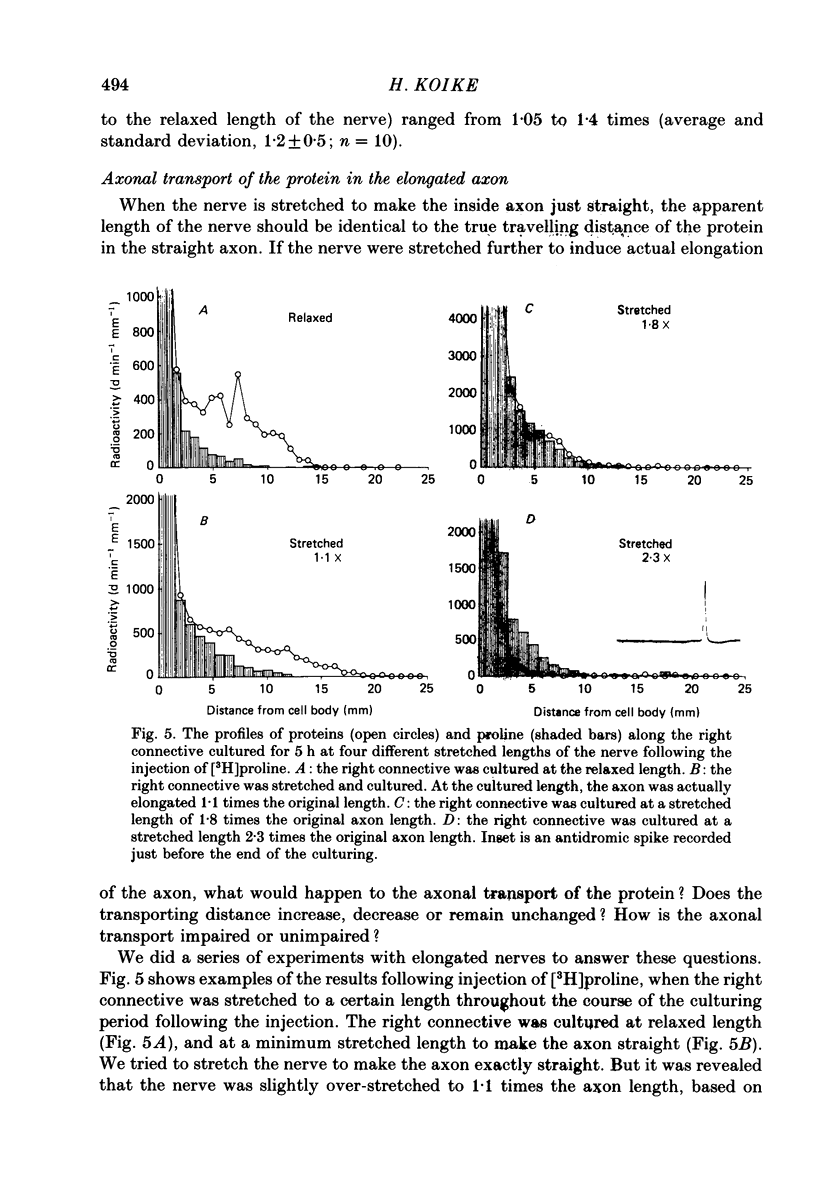
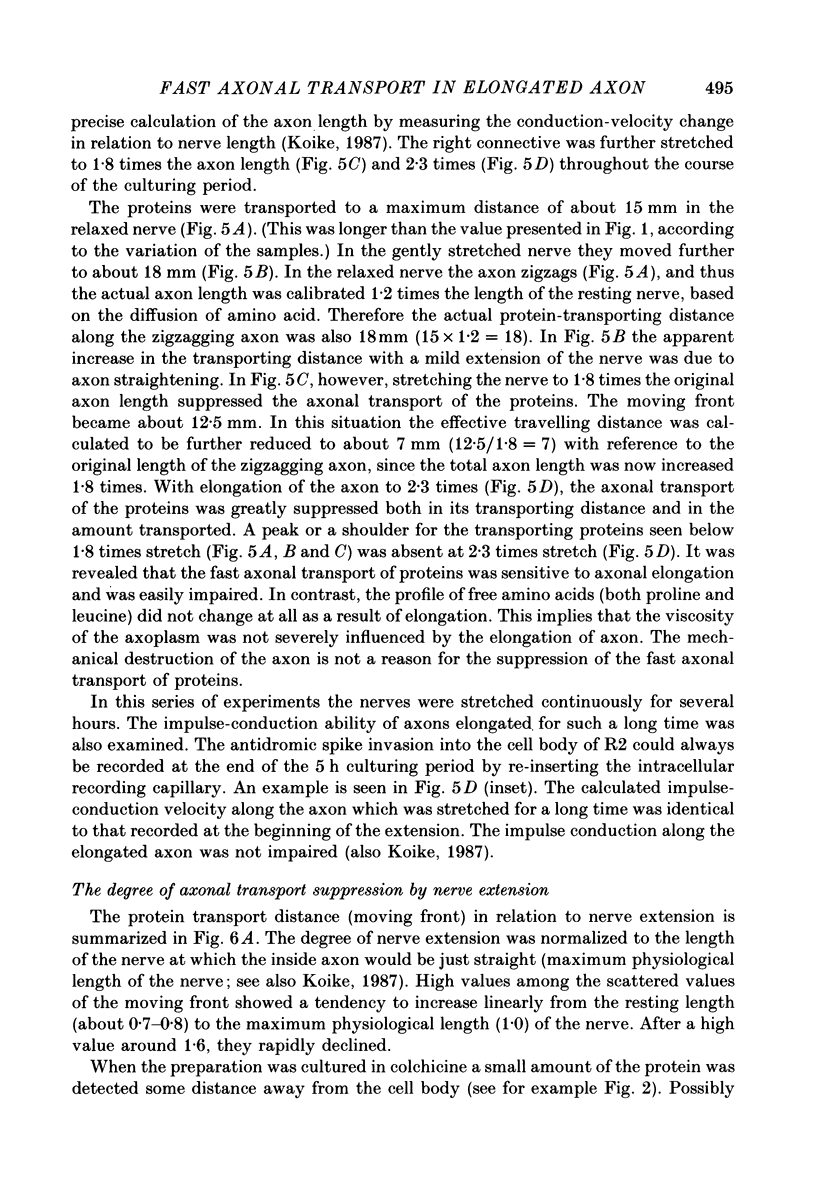
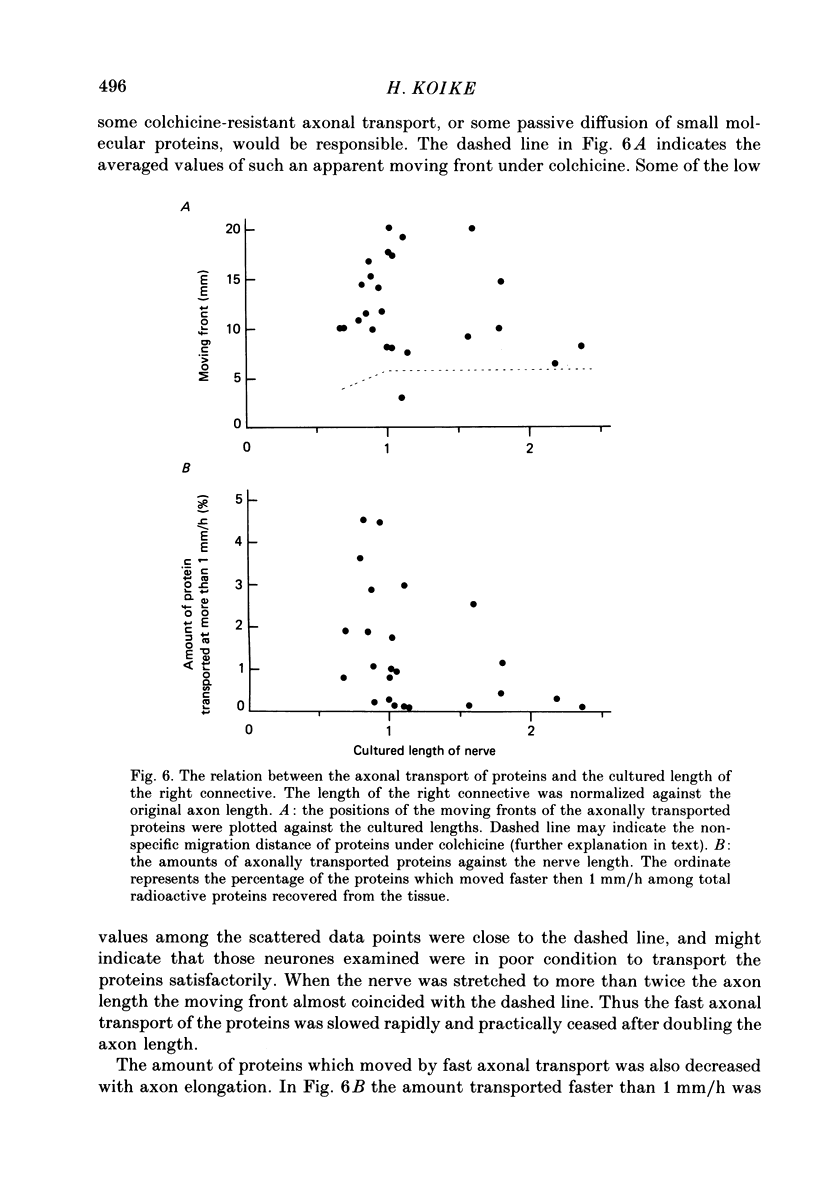
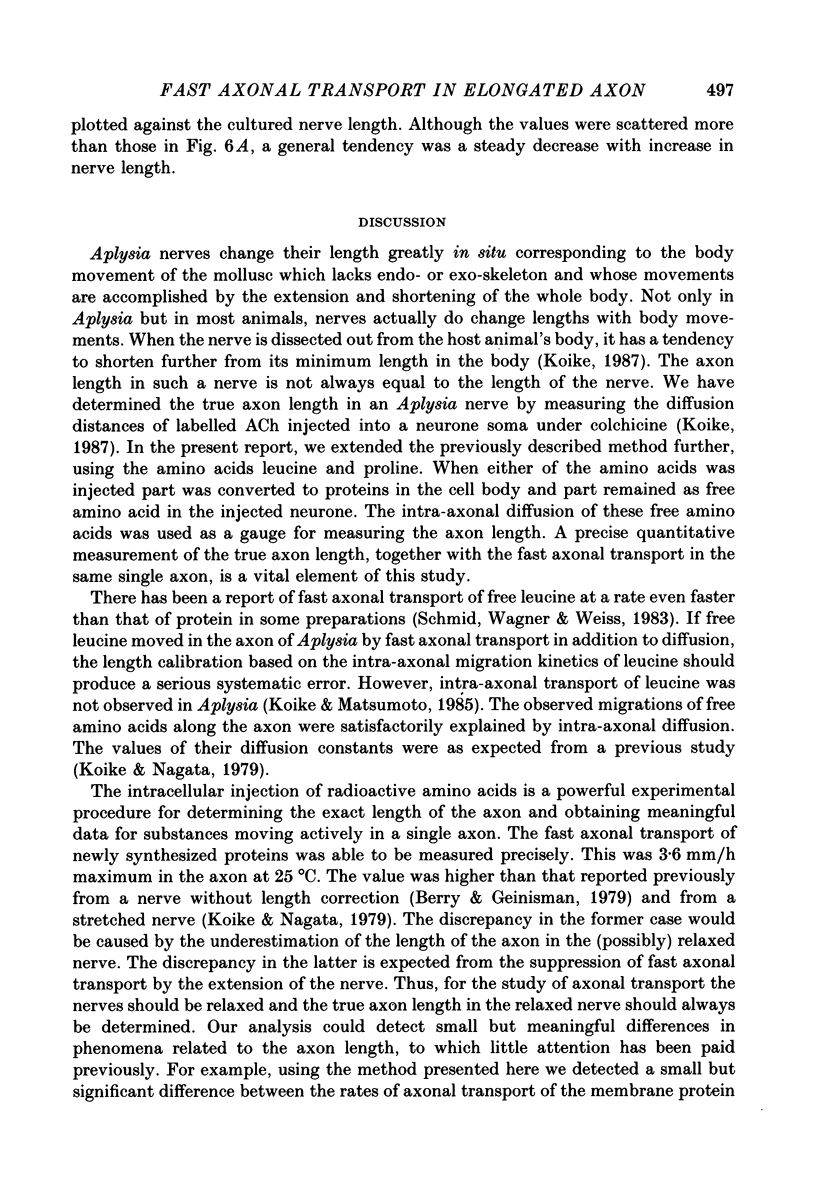
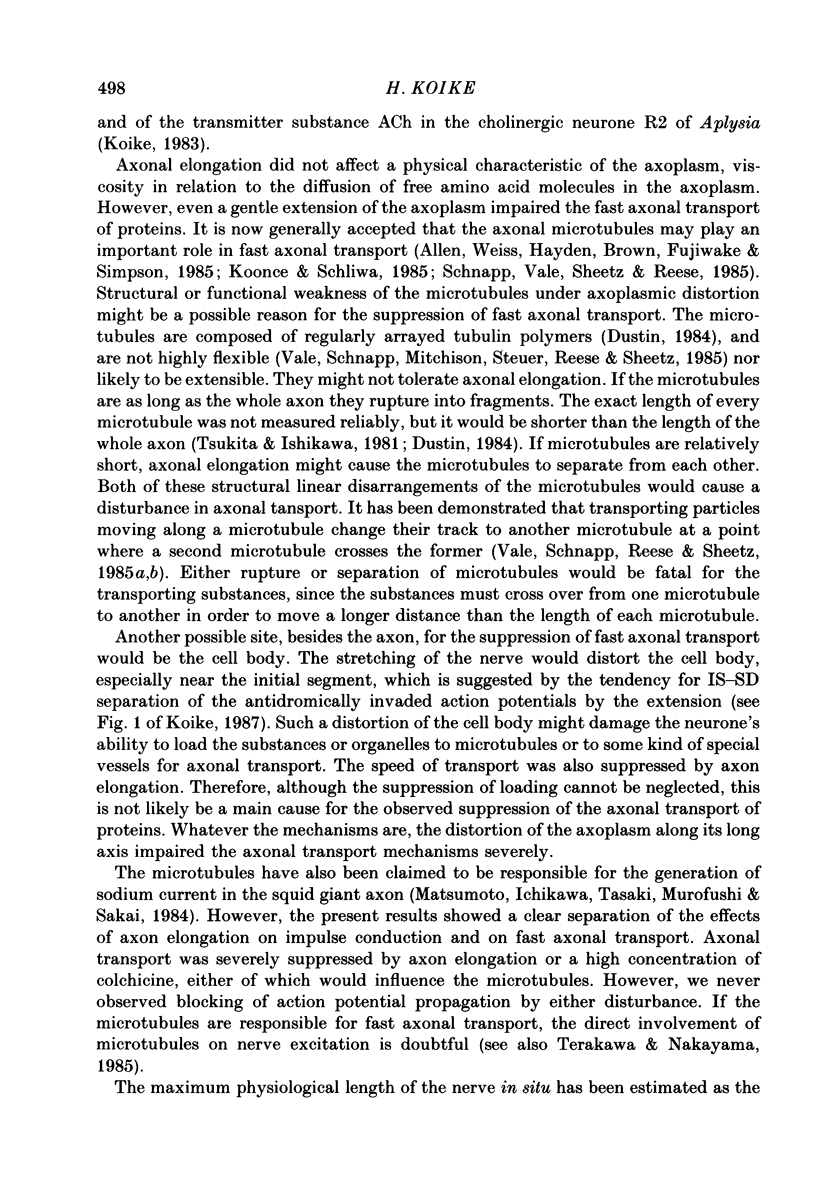
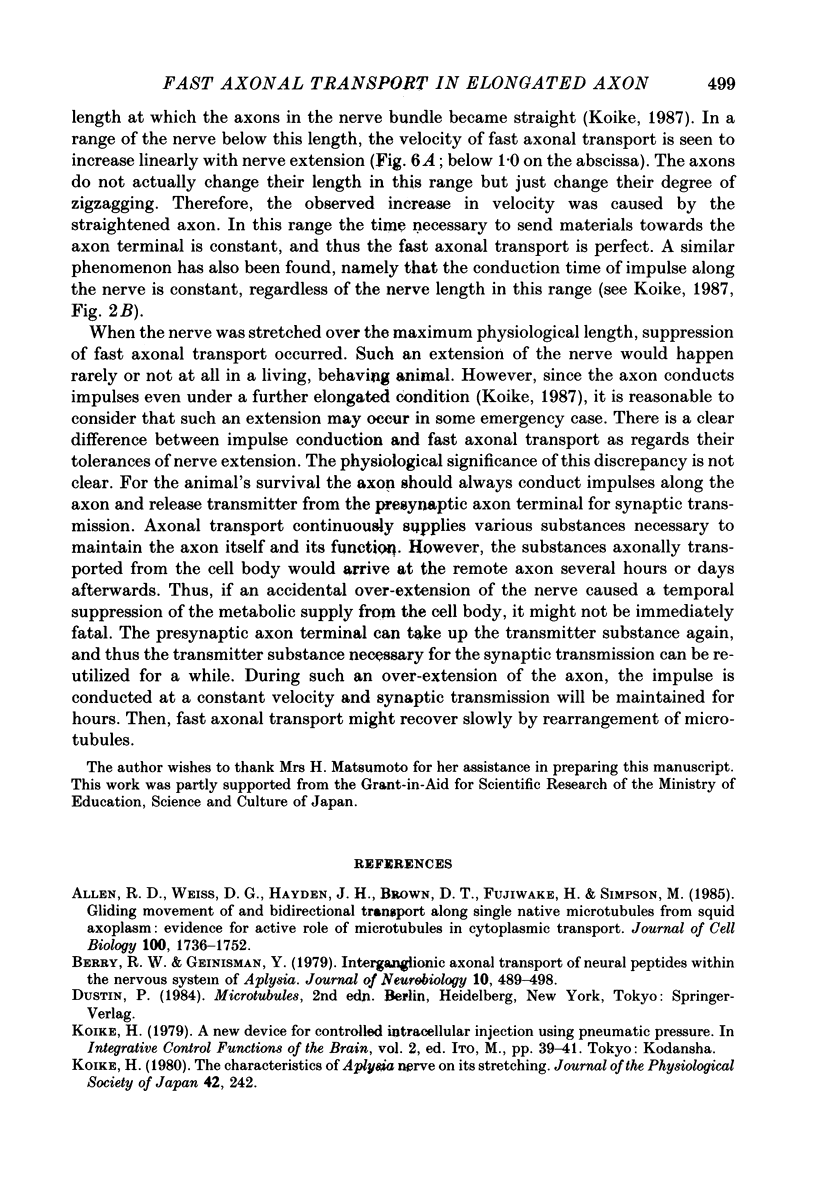
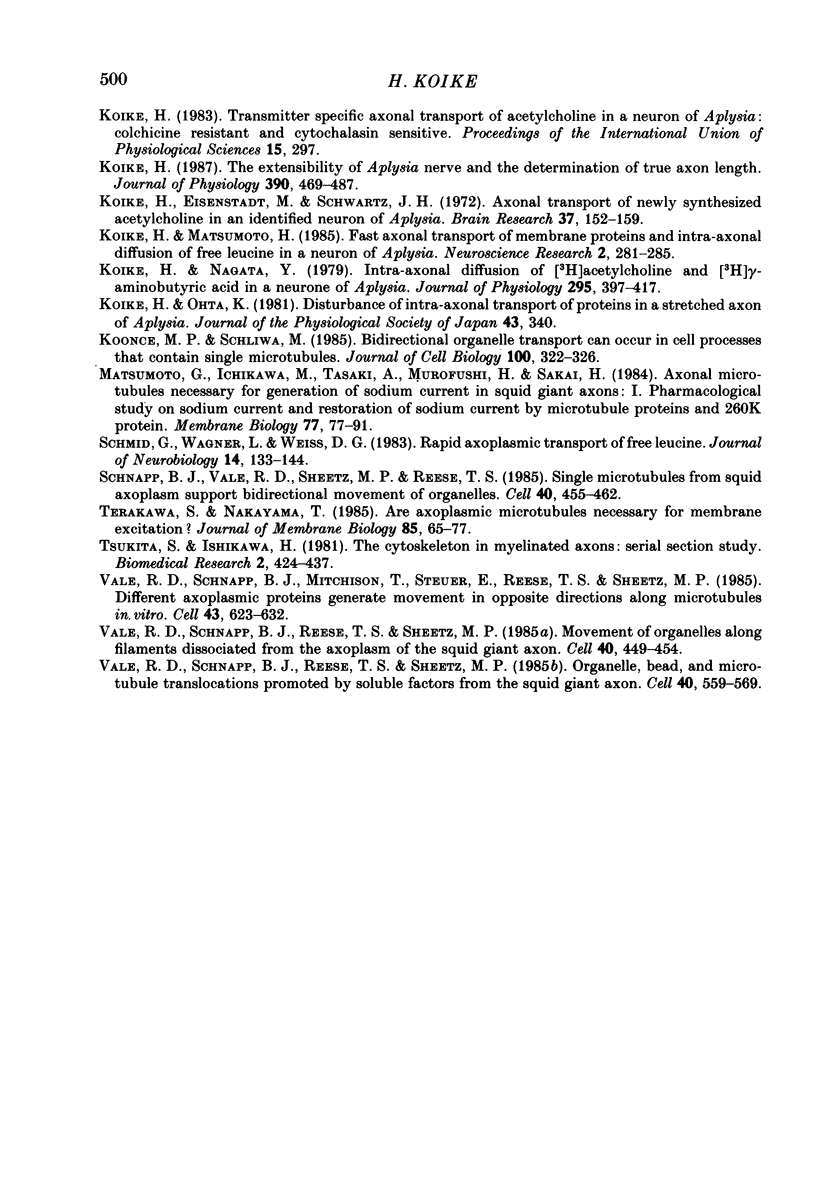
Selected References
These references are in PubMed. This may not be the complete list of references from this article.
- Allen R. D., Weiss D. G., Hayden J. H., Brown D. T., Fujiwake H., Simpson M. Gliding movement of and bidirectional transport along single native microtubules from squid axoplasm: evidence for an active role of microtubules in cytoplasmic transport. J Cell Biol. 1985 May;100(5):1736–1752. doi: 10.1083/jcb.100.5.1736. [DOI] [PMC free article] [PubMed] [Google Scholar]
- Berry R. W., Geinisman Y. Interganglionic axonal transport of neural peptides within the nervous system of Aplysia. J Neurobiol. 1979 Sep;10(5):489–498. doi: 10.1002/neu.480100506. [DOI] [PMC free article] [PubMed] [Google Scholar]
- Koike H., Eisenstadt M., Schwartz J. H. Axonal transport of newly synthesized acetylcholine in an identified neuron of Aplysia. Brain Res. 1972 Feb 11;37(1):152–159. doi: 10.1016/0006-8993(72)90359-9. [DOI] [PubMed] [Google Scholar]
- Koike H., Matsumoto H. Fast axonal transport of membrane protein and intra-axonal diffusion of free leucine in a neuron of Aplysia. Neurosci Res. 1985 Apr;2(4):281–285. doi: 10.1016/0168-0102(85)90006-9. [DOI] [PubMed] [Google Scholar]
- Koike H., Nagata Y. Intra-axonal diffusion of [3H]acetylcholine and [3H]gamma-aminobutyric acid in a neurone of Aplysia. J Physiol. 1979 Oct;295:397–417. doi: 10.1113/jphysiol.1979.sp012976. [DOI] [PMC free article] [PubMed] [Google Scholar]
- Koike H. The extensibility of Aplysia nerve and the determination of true axon length. J Physiol. 1987 Sep;390:469–487. doi: 10.1113/jphysiol.1987.sp016712. [DOI] [PMC free article] [PubMed] [Google Scholar]
- Koonce M. P., Schliwa M. Bidirectional organelle transport can occur in cell processes that contain single microtubules. J Cell Biol. 1985 Jan;100(1):322–326. doi: 10.1083/jcb.100.1.322. [DOI] [PMC free article] [PubMed] [Google Scholar]
- Matsumoto G., Ichikawa M., Tasaki A., Murofushi H., Sakai H. Axonal microtubules necessary for generation of sodium current in squid giant axons: I. Pharmacological study on sodium current and restoration of sodium current by microtubule proteins and 260K protein. J Membr Biol. 1984;77(2):77–91. doi: 10.1007/BF01925858. [DOI] [PubMed] [Google Scholar]
- Schmid G., Wagner L., Weiss D. G. Rapid axoplasmic transport of free leucine. J Neurobiol. 1983 Mar;14(2):133–144. doi: 10.1002/neu.480140205. [DOI] [PubMed] [Google Scholar]
- Schnapp B. J., Vale R. D., Sheetz M. P., Reese T. S. Single microtubules from squid axoplasm support bidirectional movement of organelles. Cell. 1985 Feb;40(2):455–462. doi: 10.1016/0092-8674(85)90160-6. [DOI] [PubMed] [Google Scholar]
- Terakawa S., Nakayama T. Are axoplasmic microtubules necessary for membrane excitation? J Membr Biol. 1985;85(1):65–77. doi: 10.1007/BF01872006. [DOI] [PubMed] [Google Scholar]
- Vale R. D., Schnapp B. J., Mitchison T., Steuer E., Reese T. S., Sheetz M. P. Different axoplasmic proteins generate movement in opposite directions along microtubules in vitro. Cell. 1985 Dec;43(3 Pt 2):623–632. doi: 10.1016/0092-8674(85)90234-x. [DOI] [PubMed] [Google Scholar]
- Vale R. D., Schnapp B. J., Reese T. S., Sheetz M. P. Movement of organelles along filaments dissociated from the axoplasm of the squid giant axon. Cell. 1985 Feb;40(2):449–454. doi: 10.1016/0092-8674(85)90159-x. [DOI] [PubMed] [Google Scholar]
- Vale R. D., Schnapp B. J., Reese T. S., Sheetz M. P. Organelle, bead, and microtubule translocations promoted by soluble factors from the squid giant axon. Cell. 1985 Mar;40(3):559–569. doi: 10.1016/0092-8674(85)90204-1. [DOI] [PubMed] [Google Scholar]