Abstract
1. Mammalian platelets were freshly isolated from human, rabbit, or rat blood. The whole-cell and cell-attached voltage-clamp variations of the patch-clamp technique were employed to study the passive electrical properties and ion channels of unstimulated platelets. 2. The input capacitance of a platelet measured by the phase-sensitive detection method was about 128 fF, the input resistance of a platelet was about 59 G omega and the resting membrane potential was about -50 mV which was directly measured by a whole-cell recording in the current-clamp mode. 3. The predominant ion channel was a voltage-gated K+ channel resembling the delayed rectifier K+ channel of nerve, muscle and T-lymphocyte. There was no indication of any inward current in the platelet membrane. The activation of the K+ current could be fitted by n4 kinetics, and was half-maximal at about -35 mV. 4. The time constant of K+ current inactivation was virtually independent of voltage and varied from cell to cell. Recovery from inactivation was slow and dependent on the size and duration of the preceding conditional voltage step. Steady-state inactivation was half-maximal at about -50 mV and was complete at positive potentials. 5. The predominant single-K+-channel conductance was 9 pS and the estimated number of K+ channels per platelet was about 325, corresponding to a density of 25/micron2 apparent membrane area.
Full text
PDF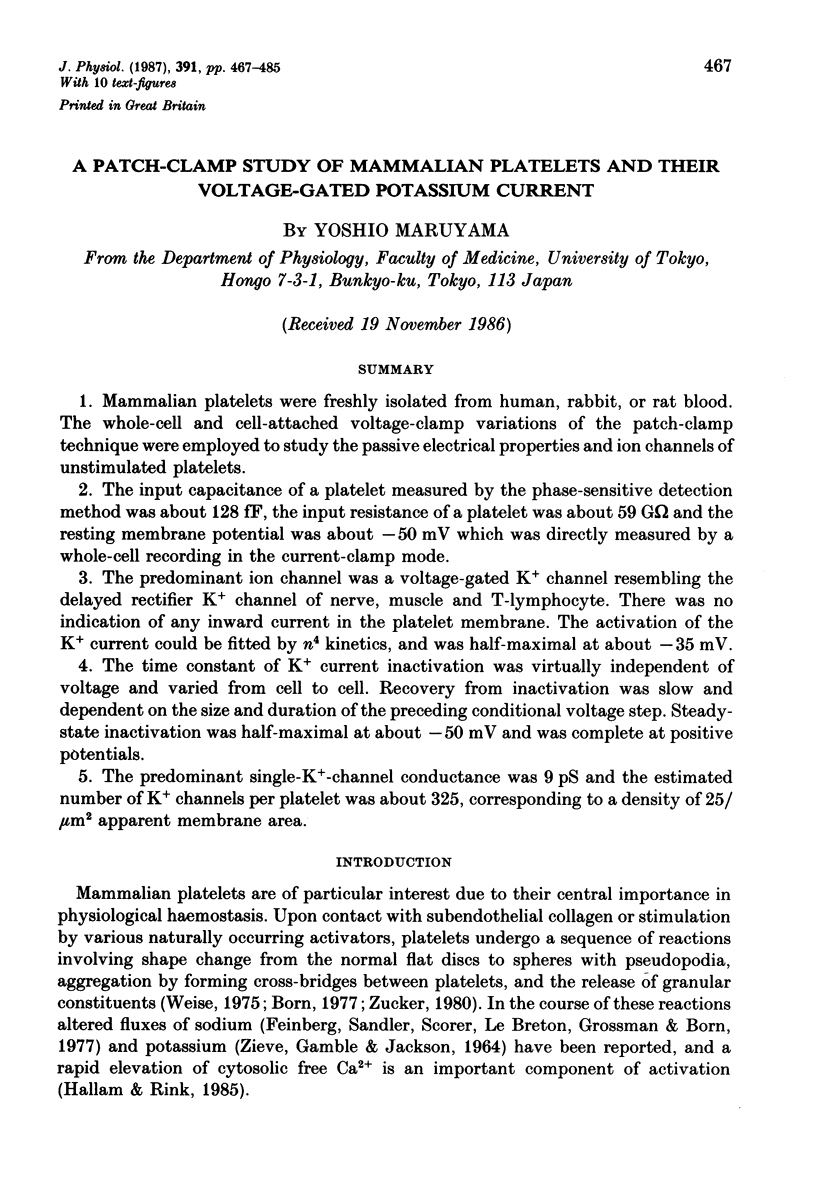
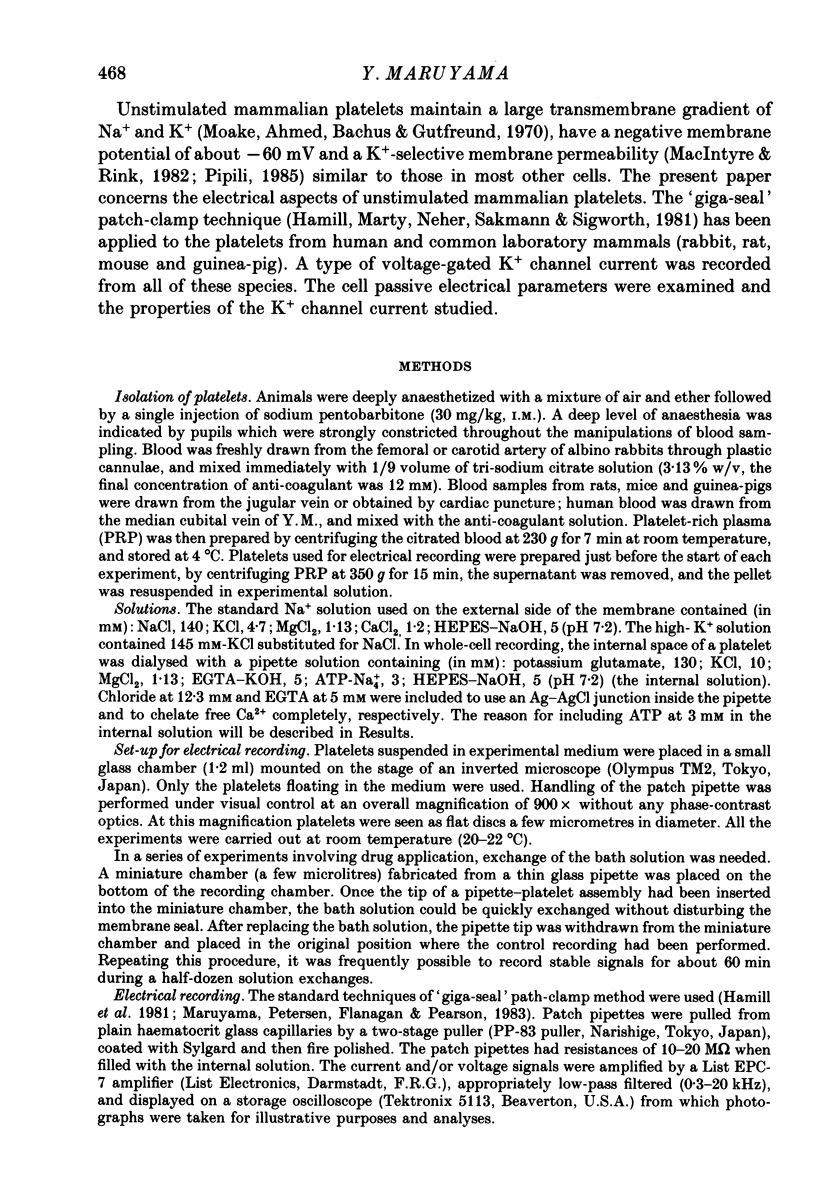
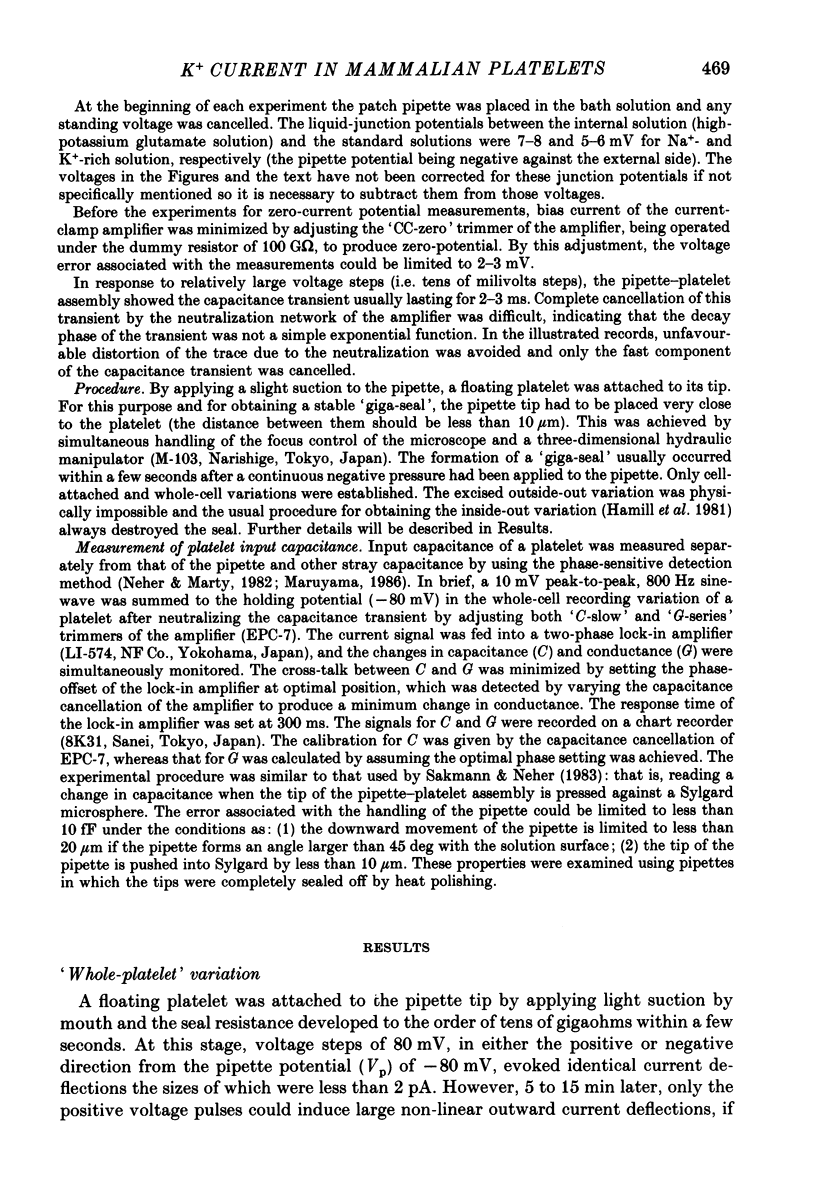
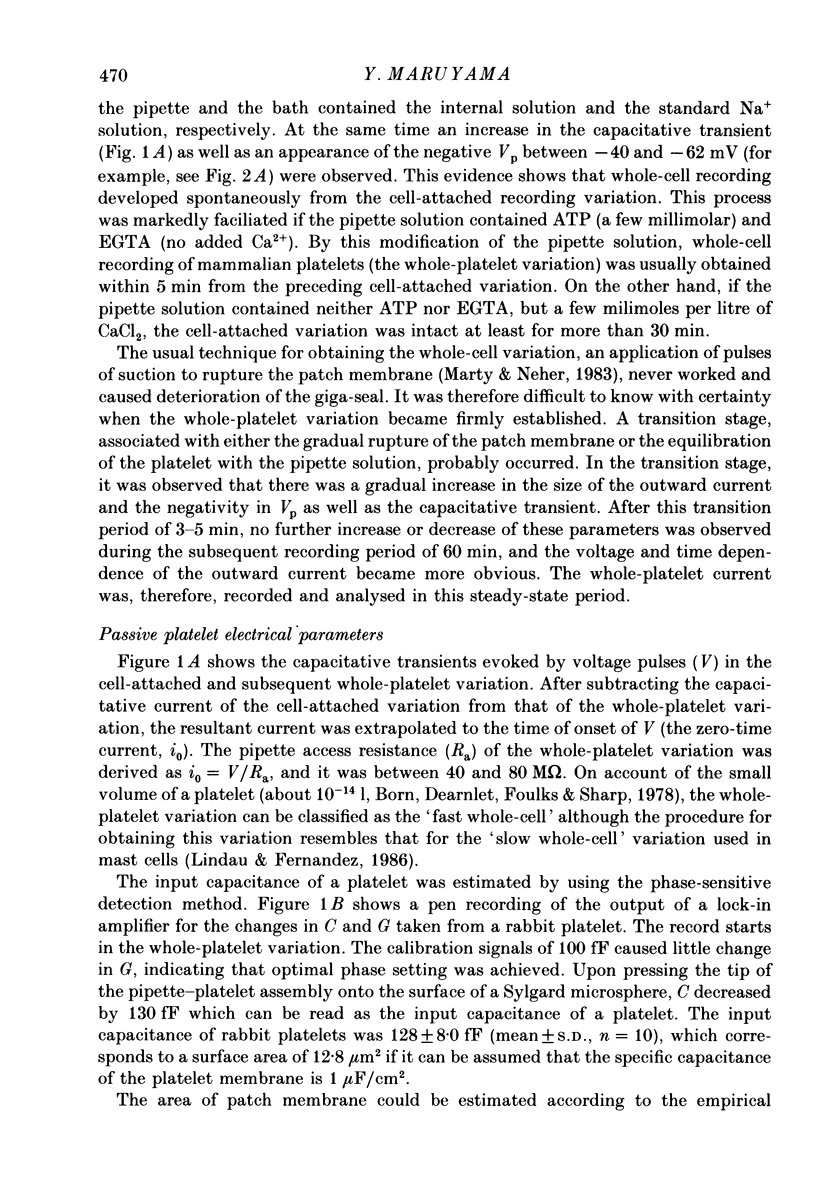
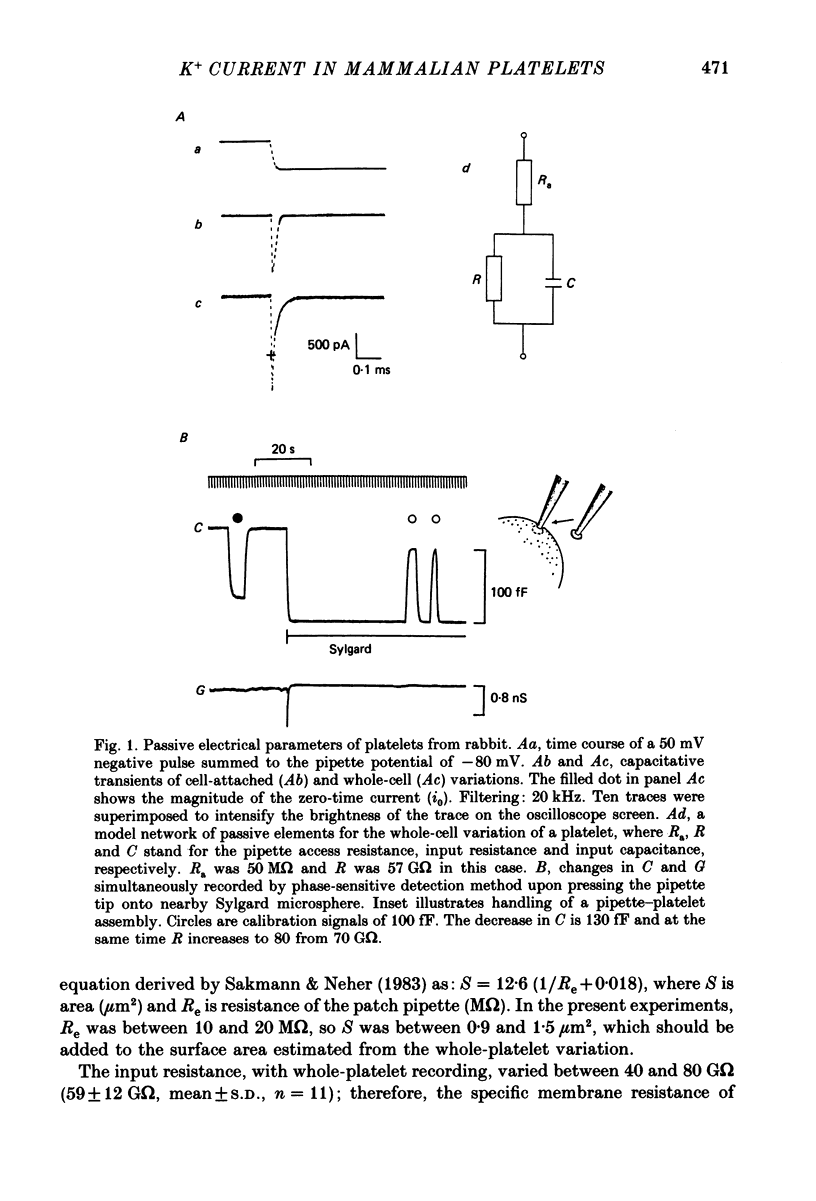
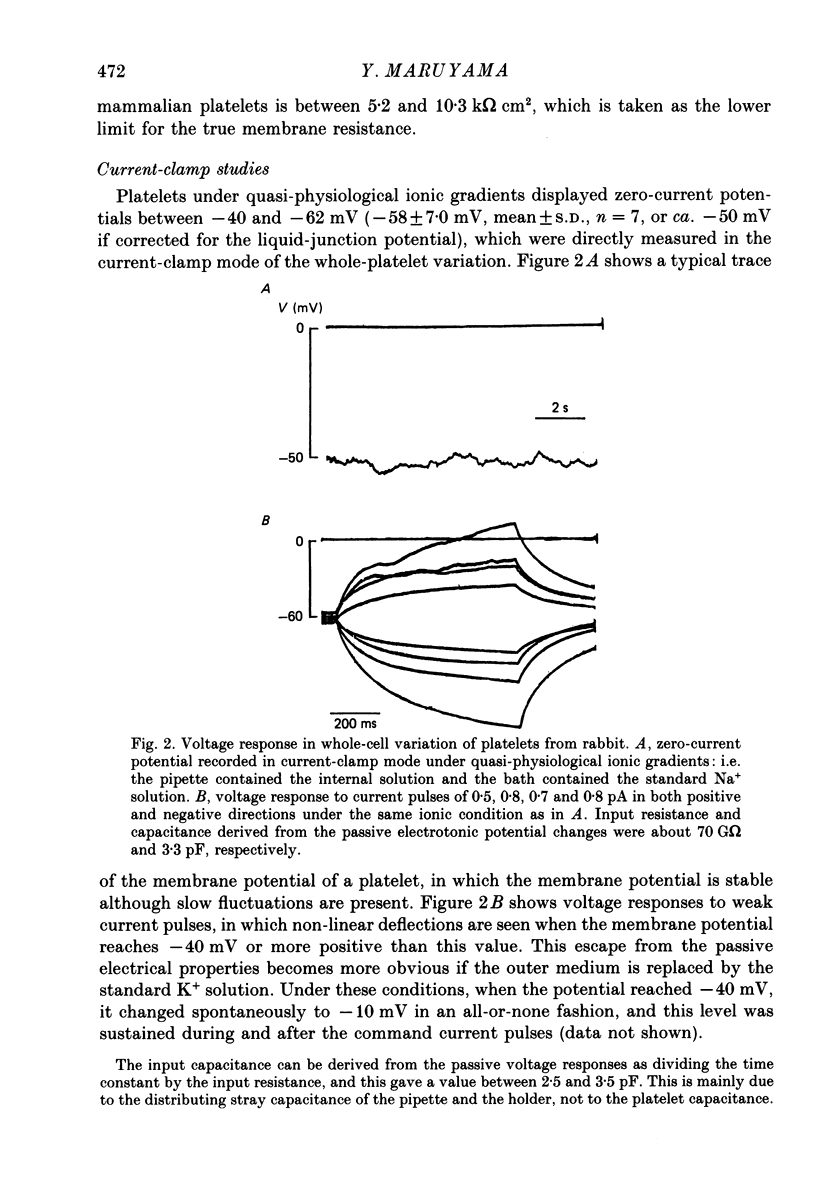
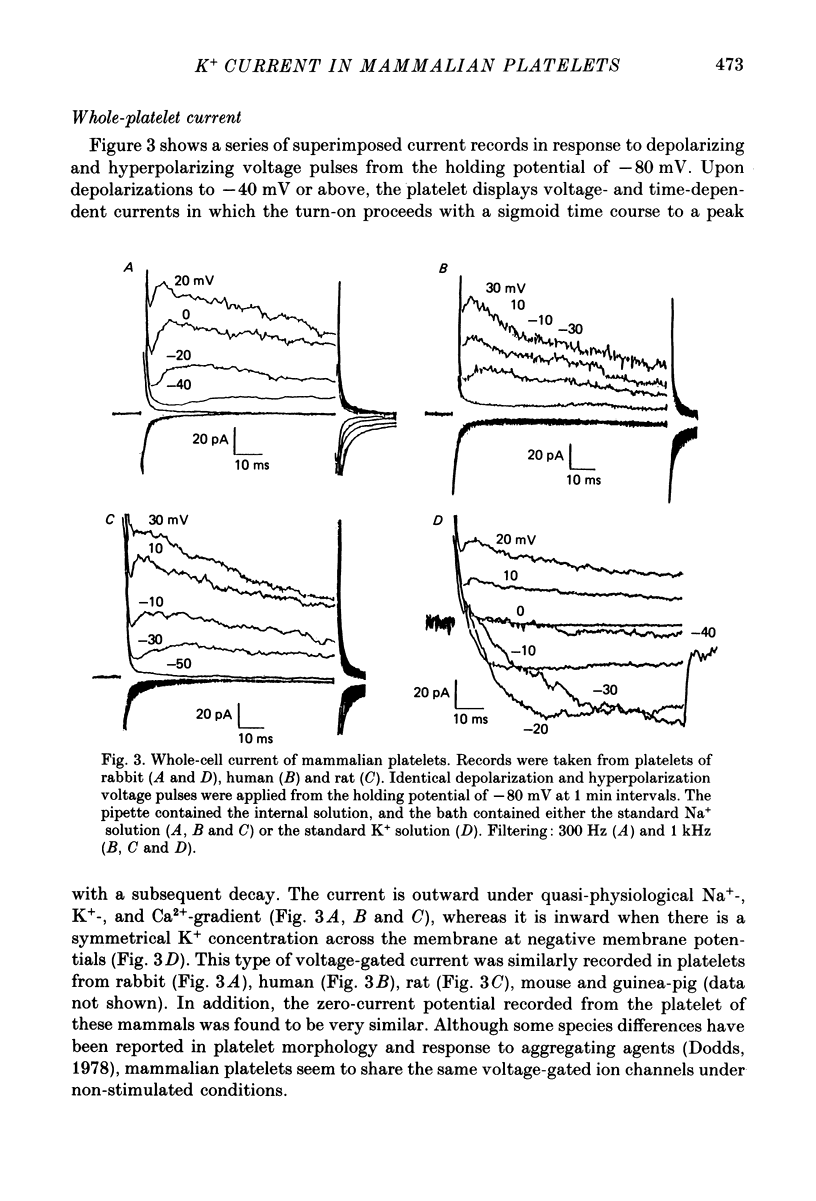
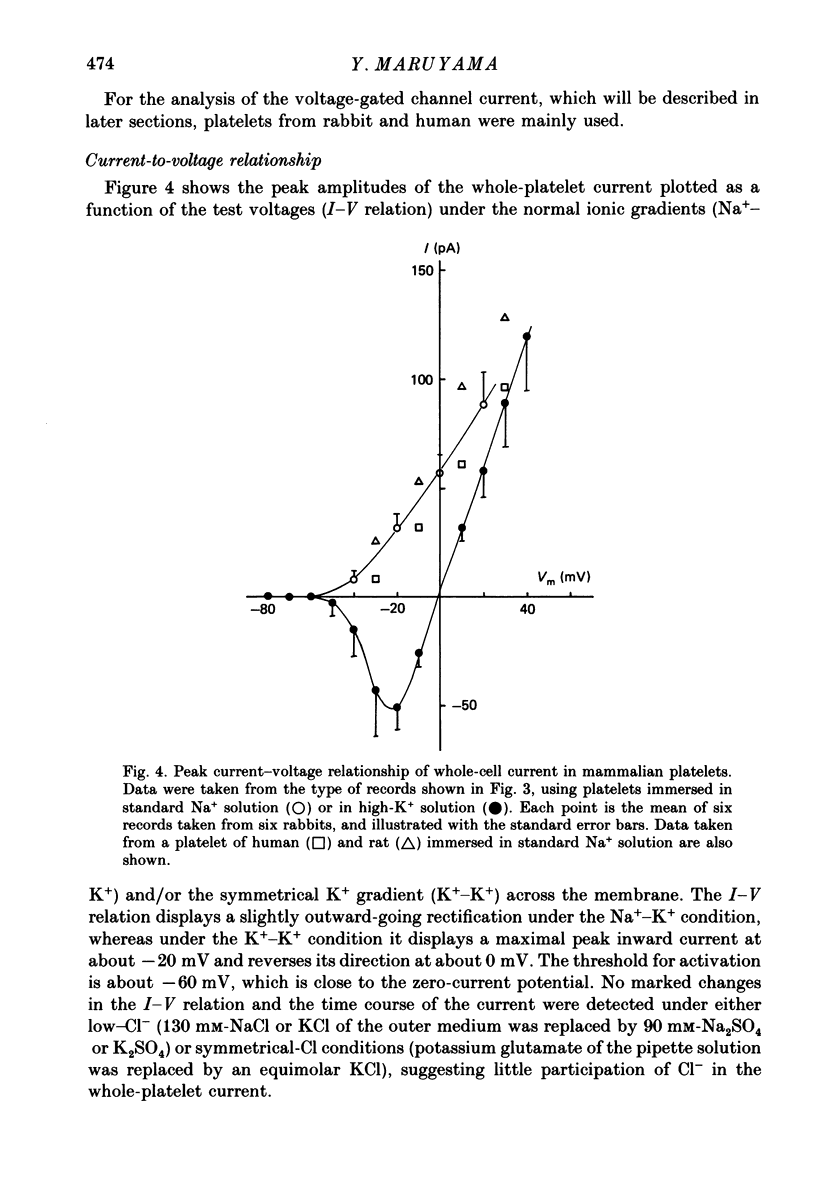
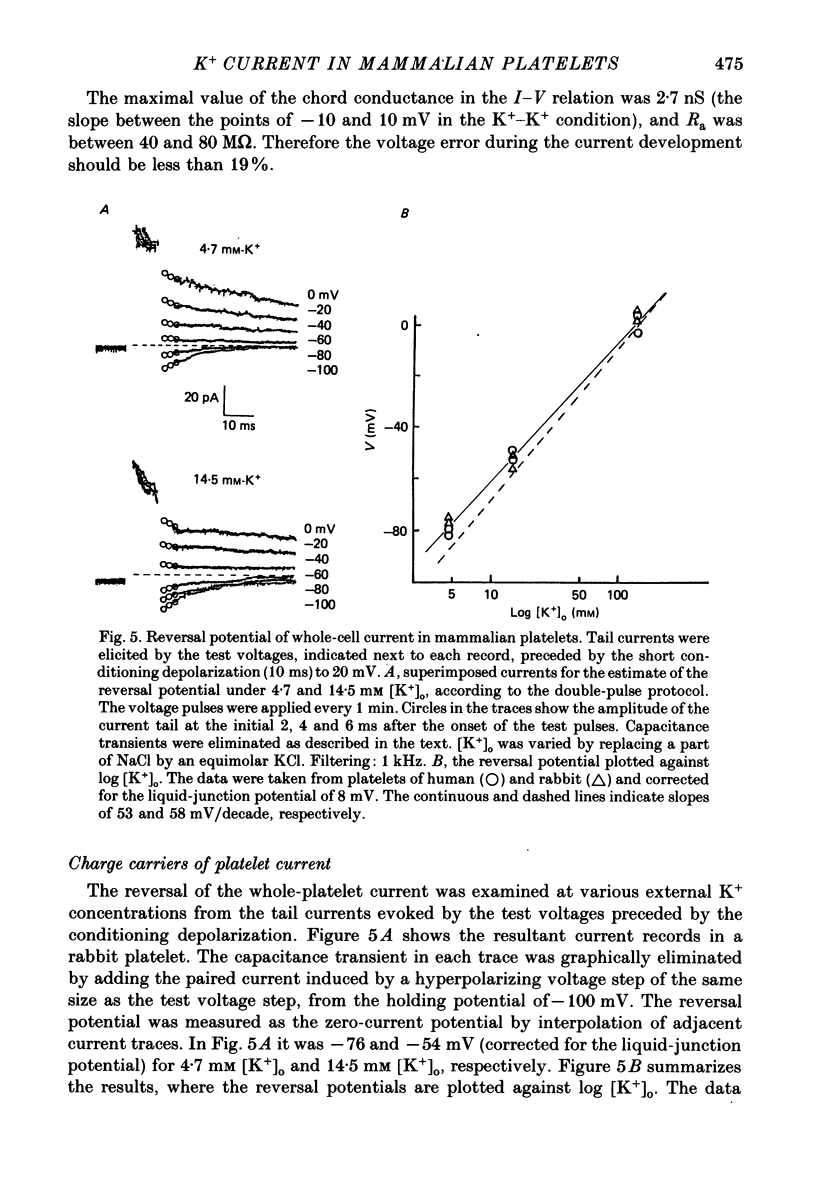
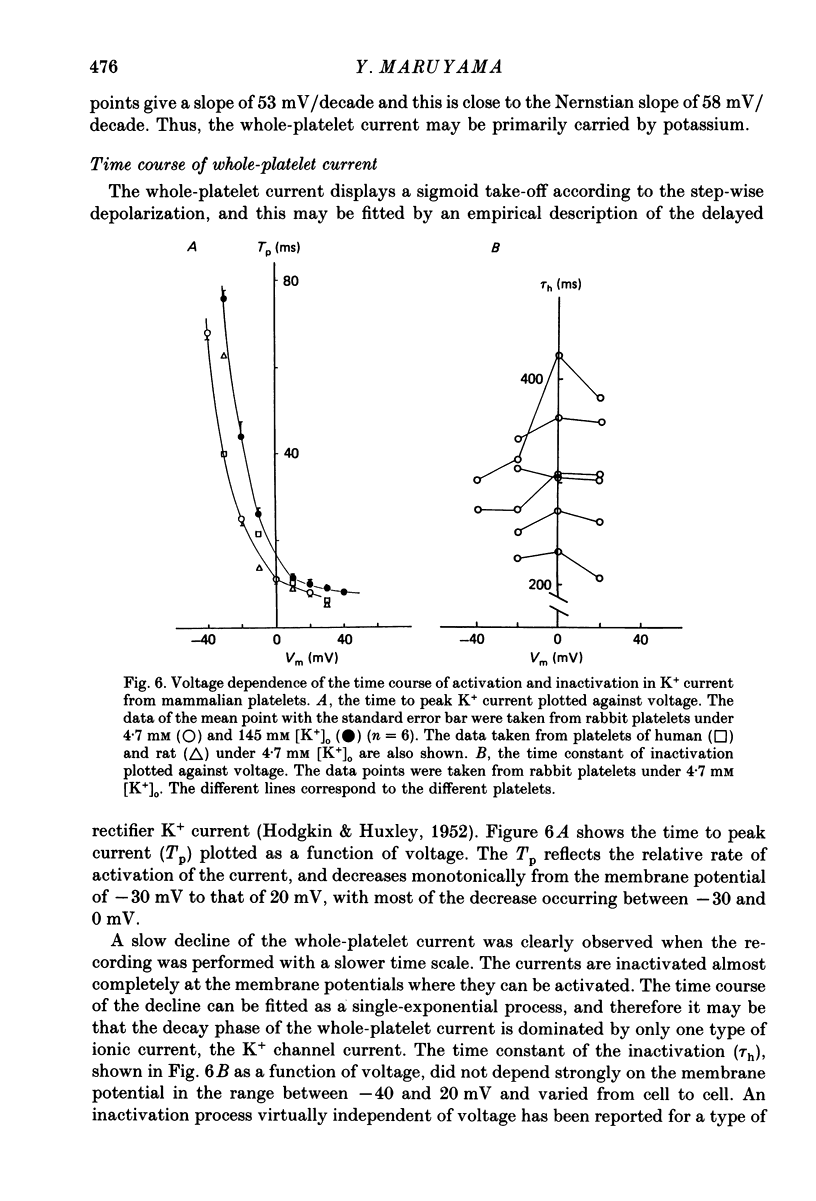
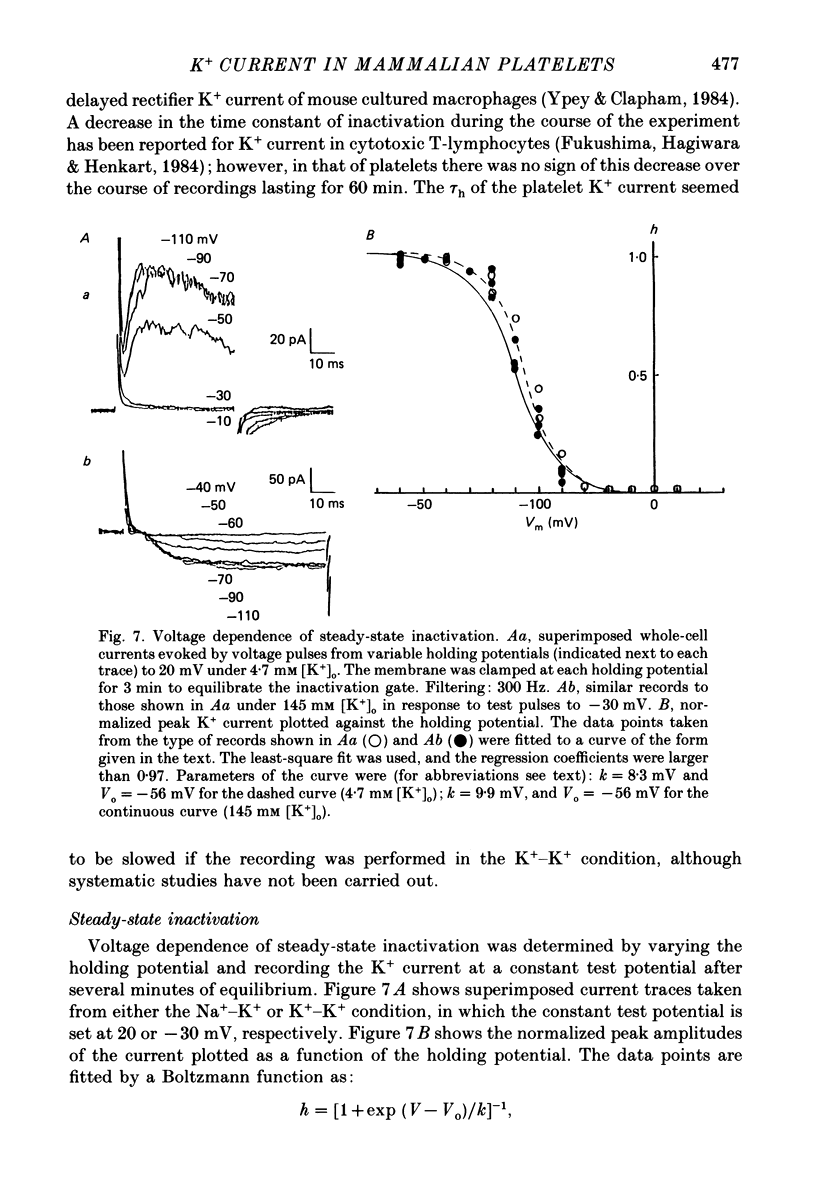
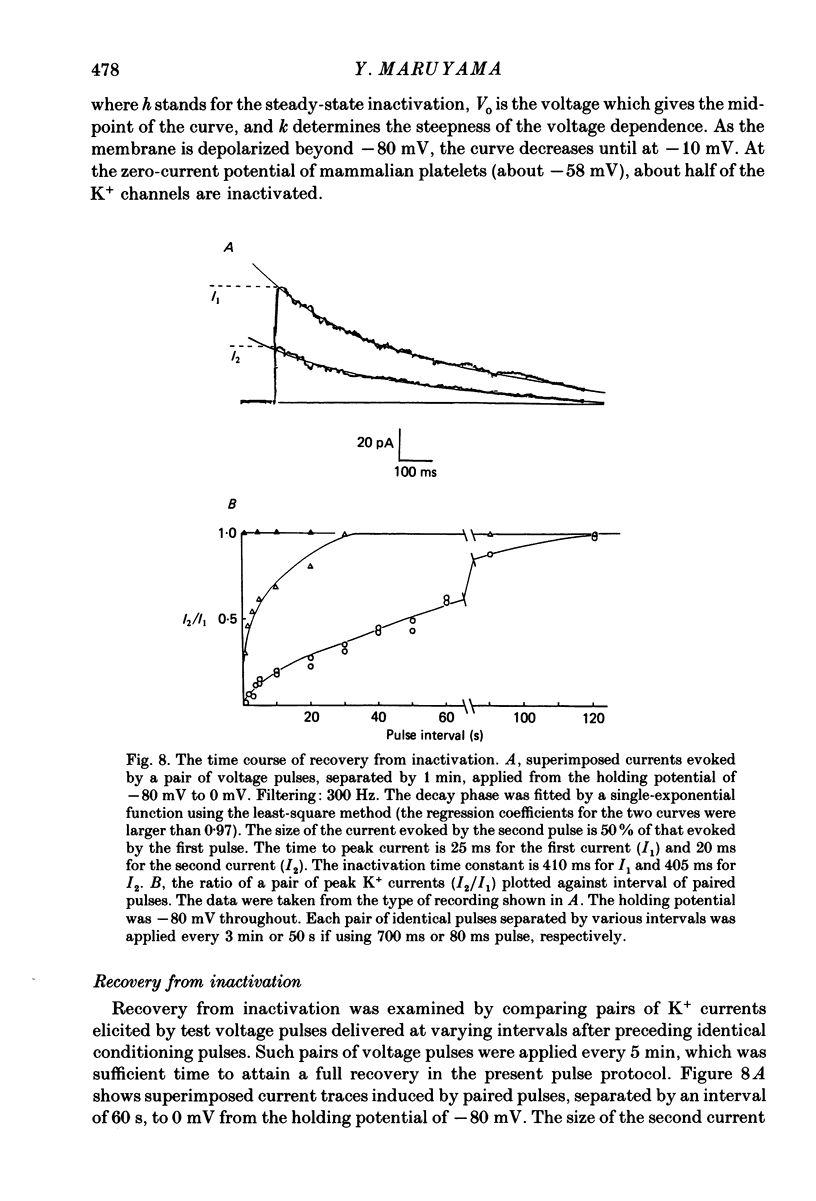
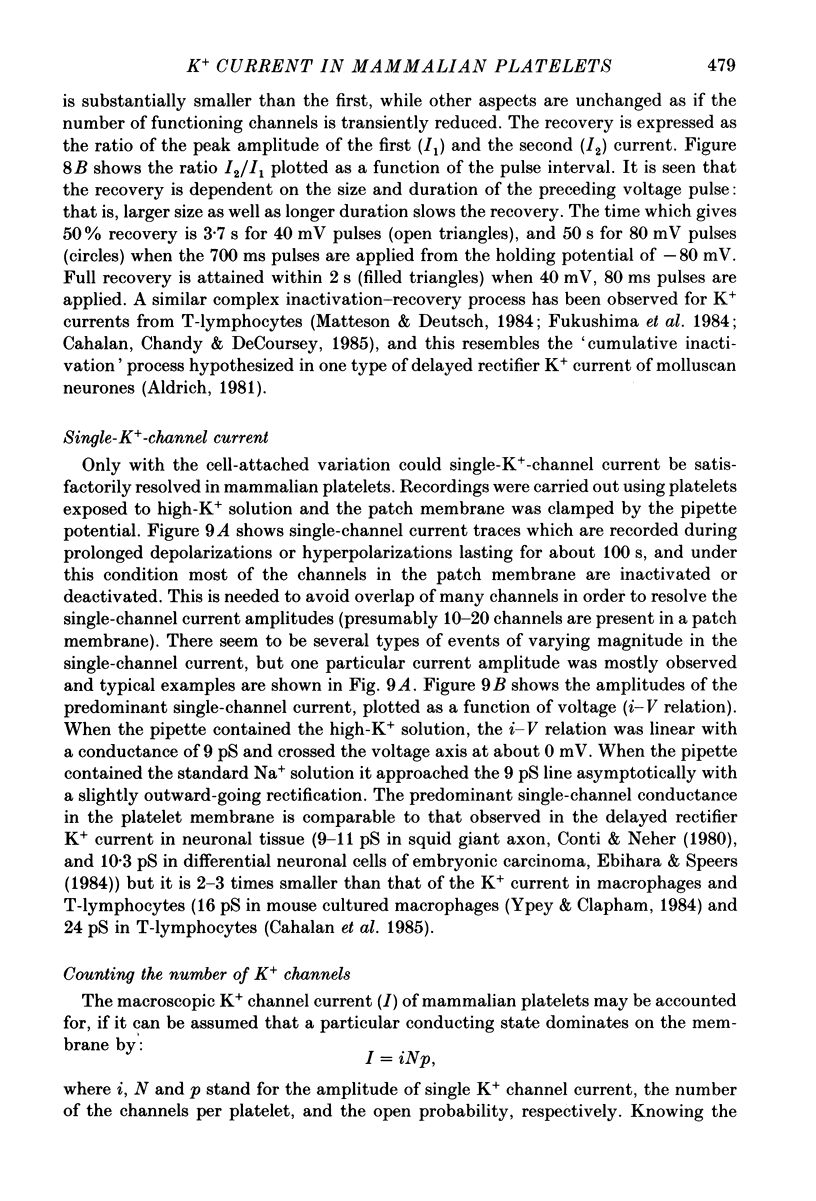
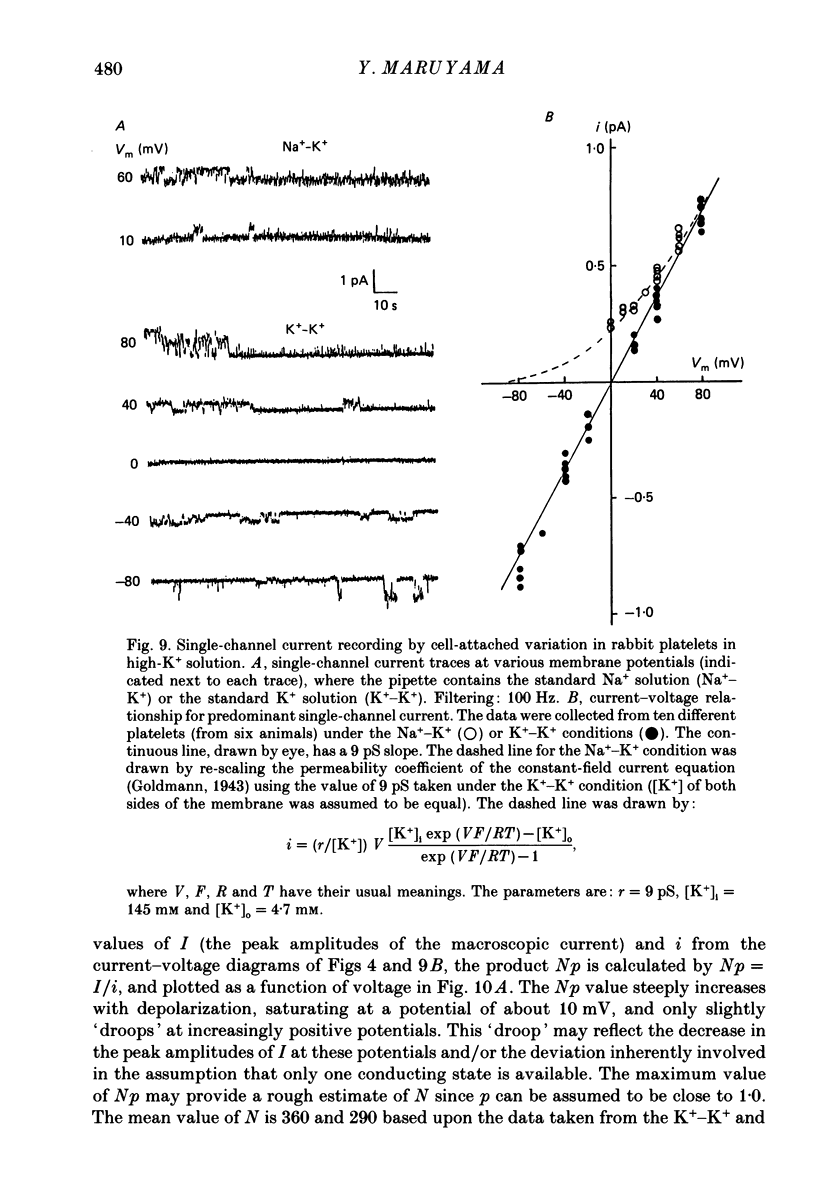
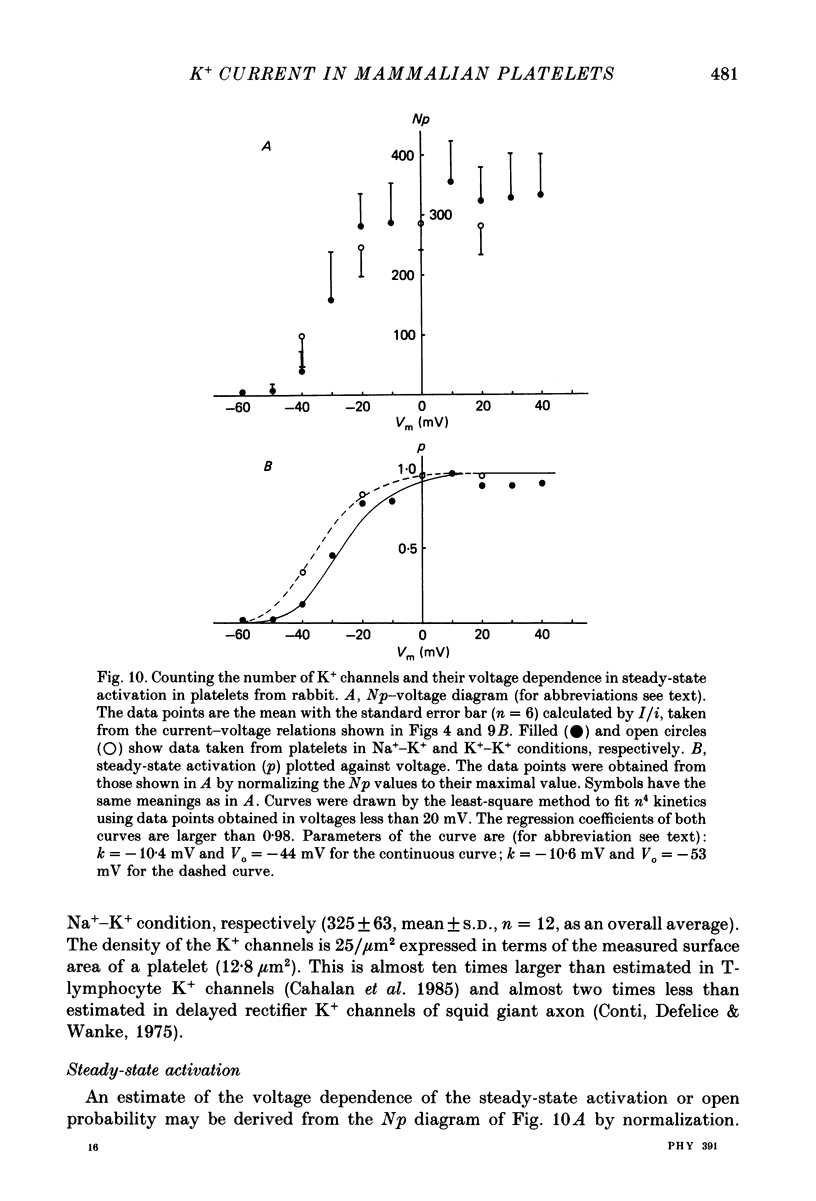
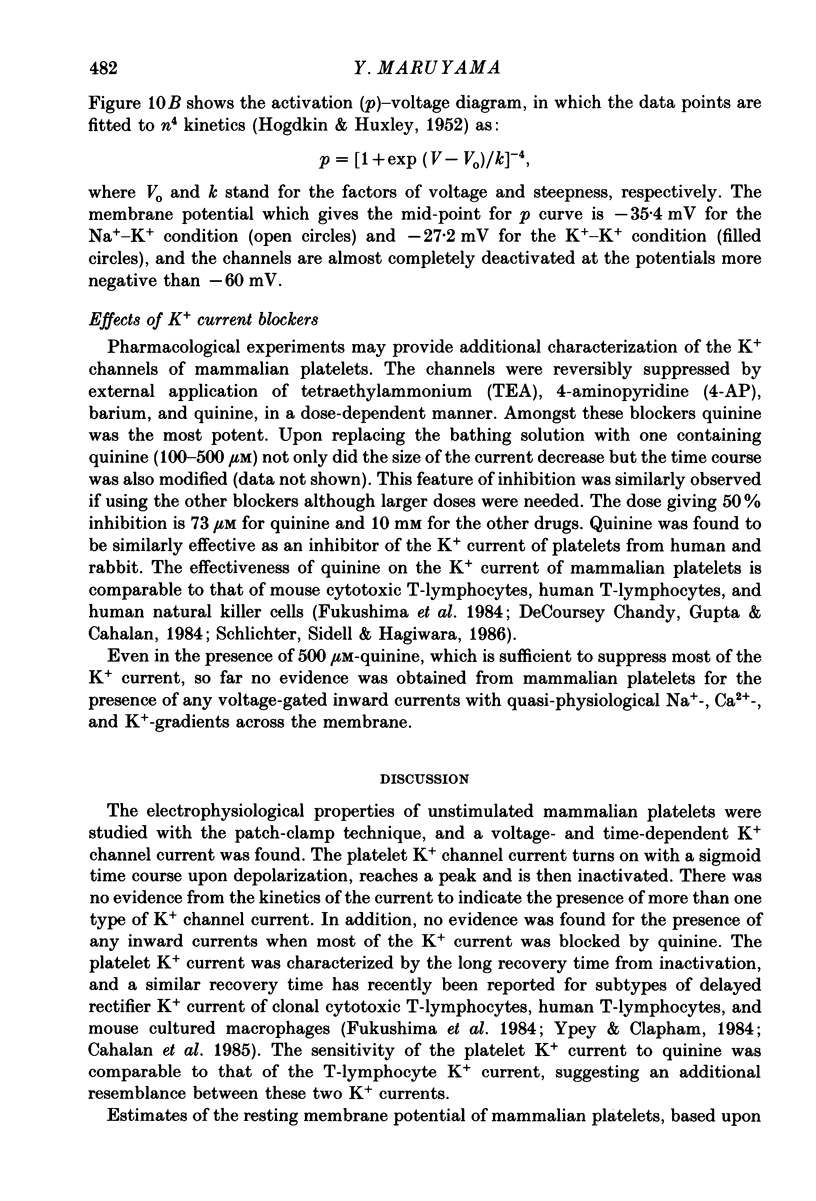
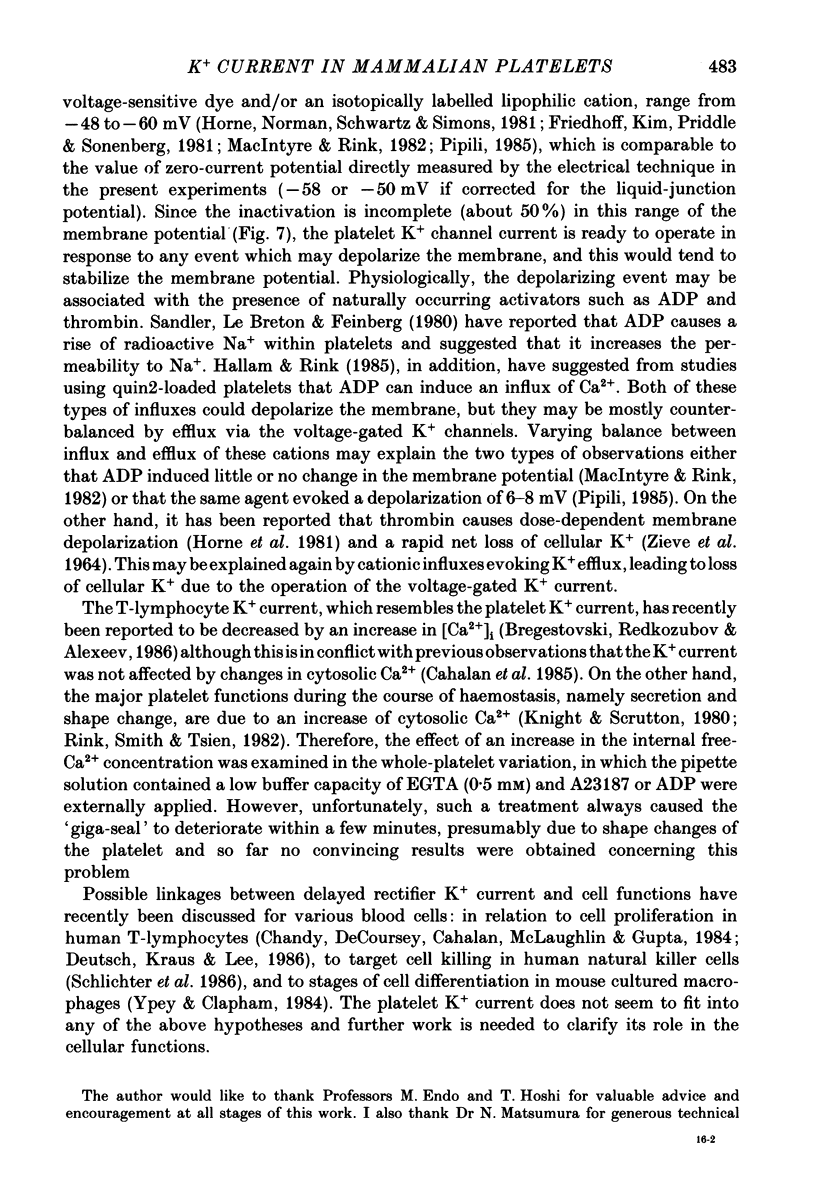
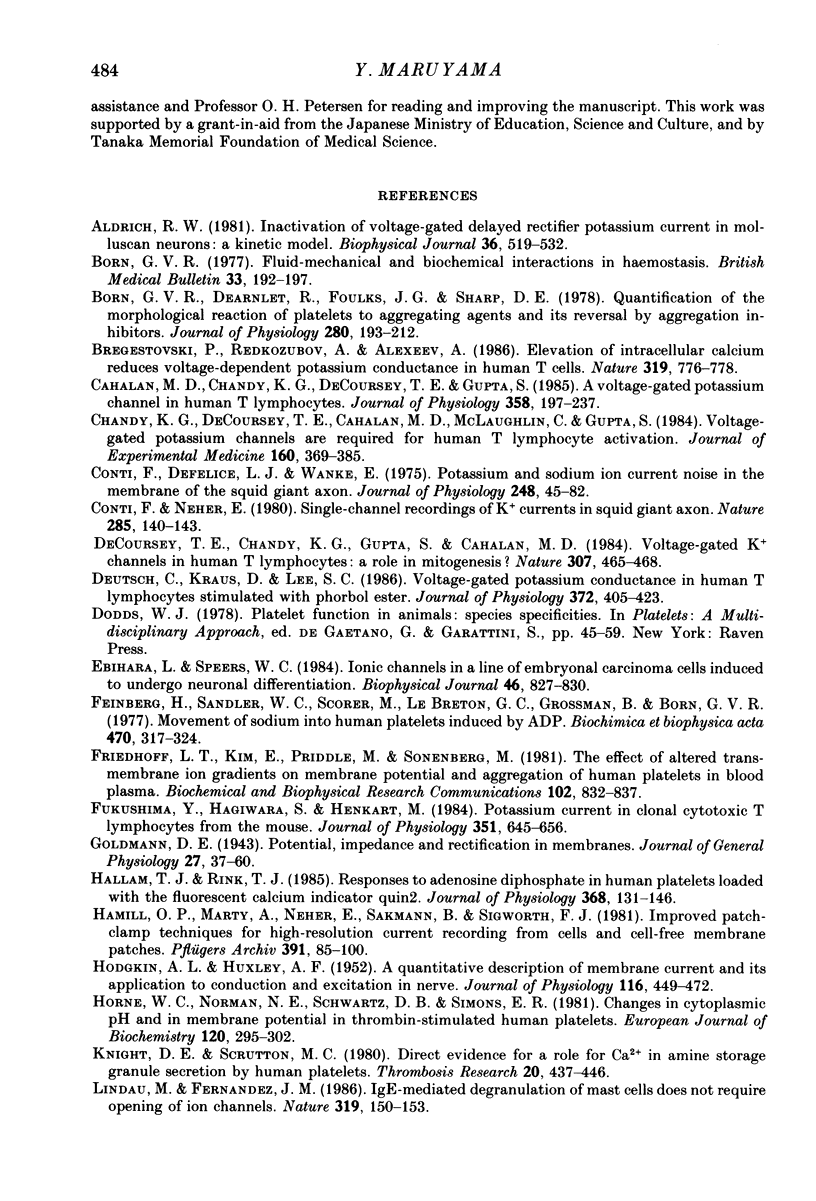
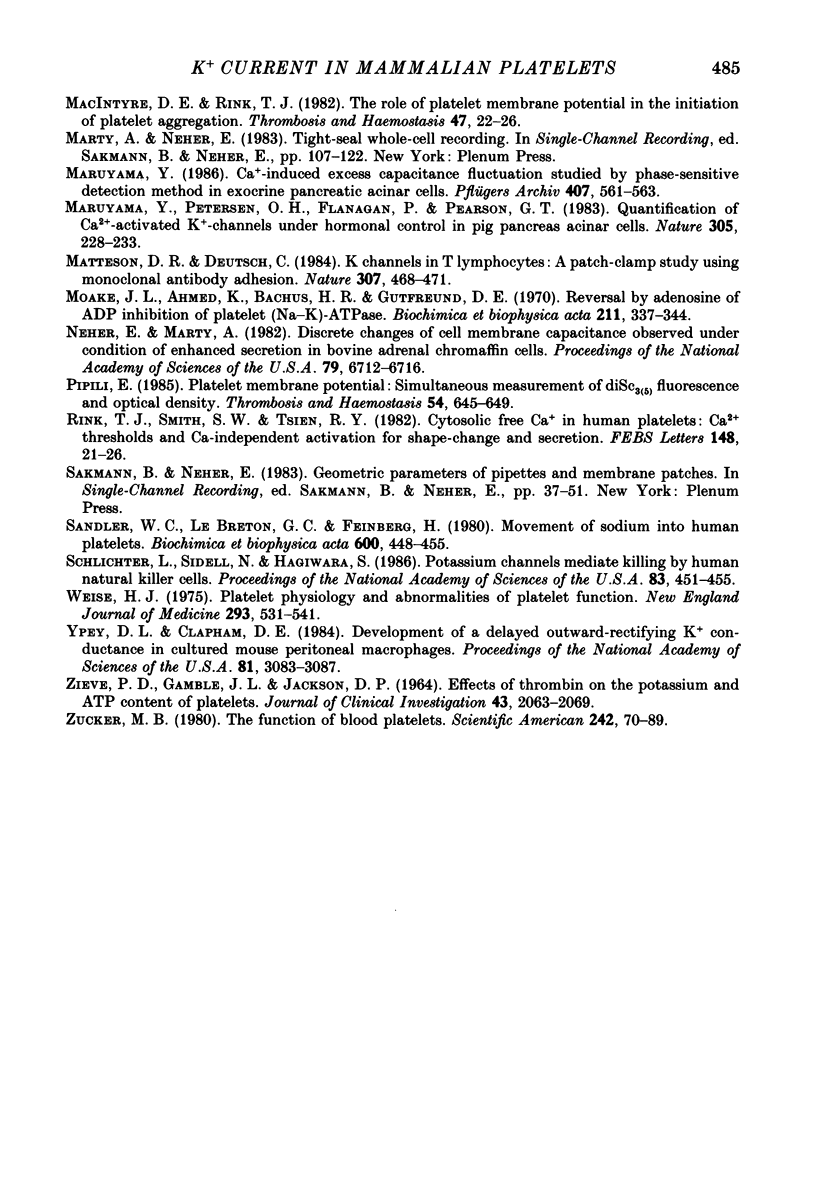
Selected References
These references are in PubMed. This may not be the complete list of references from this article.
- Aldrich R. W. Inactivation of voltage-gated delayed potassium current in molluscan neurons. A kinetic model. Biophys J. 1981 Dec;36(3):519–532. doi: 10.1016/S0006-3495(81)84750-9. [DOI] [PMC free article] [PubMed] [Google Scholar]
- Born G. V., Dearnley R., Foulks J. G., Sharp D. E. Quantification of the morphological reaction of platelets to aggregating agents and of its reversal by aggregation inhibitors. J Physiol. 1978 Jul;280:193–212. doi: 10.1113/jphysiol.1978.sp012380. [DOI] [PMC free article] [PubMed] [Google Scholar]
- Bregestovski P., Redkozubov A., Alexeev A. Elevation of intracellular calcium reduces voltage-dependent potassium conductance in human T cells. 1986 Feb 27-Mar 5Nature. 319(6056):776–778. doi: 10.1038/319776a0. [DOI] [PubMed] [Google Scholar]
- Cahalan M. D., Chandy K. G., DeCoursey T. E., Gupta S. A voltage-gated potassium channel in human T lymphocytes. J Physiol. 1985 Jan;358:197–237. doi: 10.1113/jphysiol.1985.sp015548. [DOI] [PMC free article] [PubMed] [Google Scholar]
- Chandy K. G., DeCoursey T. E., Cahalan M. D., McLaughlin C., Gupta S. Voltage-gated potassium channels are required for human T lymphocyte activation. J Exp Med. 1984 Aug 1;160(2):369–385. doi: 10.1084/jem.160.2.369. [DOI] [PMC free article] [PubMed] [Google Scholar]
- Conti F., De Felice L. J., Wanke E. Potassium and sodium ion current noise in the membrane of the squid giant axon. J Physiol. 1975 Jun;248(1):45–82. doi: 10.1113/jphysiol.1975.sp010962. [DOI] [PMC free article] [PubMed] [Google Scholar]
- Conti F., Neher E. Single channel recordings of K+ currents in squid axons. Nature. 1980 May 15;285(5761):140–143. doi: 10.1038/285140a0. [DOI] [PubMed] [Google Scholar]
- DeCoursey T. E., Chandy K. G., Gupta S., Cahalan M. D. Voltage-gated K+ channels in human T lymphocytes: a role in mitogenesis? Nature. 1984 Feb 2;307(5950):465–468. doi: 10.1038/307465a0. [DOI] [PubMed] [Google Scholar]
- Deutsch C., Krause D., Lee S. C. Voltage-gated potassium conductance in human T lymphocytes stimulated with phorbol ester. J Physiol. 1986 Mar;372:405–423. doi: 10.1113/jphysiol.1986.sp016016. [DOI] [PMC free article] [PubMed] [Google Scholar]
- Ebihara L., Speers W. C. Ionic channels in a line of embryonal carcinoma cells induced to undergo neuronal differentiation. Biophys J. 1984 Dec;46(6):827–830. doi: 10.1016/S0006-3495(84)84081-3. [DOI] [PMC free article] [PubMed] [Google Scholar]
- Feinberg H., Sandler W. C., Scorer M., Le Breton G. C., Grossman B., Born G. V. Movement of sodium into human platelets induced by ADP. Biochim Biophys Acta. 1977 Oct 17;470(2):317–324. doi: 10.1016/0005-2736(77)90109-2. [DOI] [PubMed] [Google Scholar]
- Friedhoff L. T., Kim E., Priddle M., Sonenberg M. The effect of altered transmembrane ion gradients on membrane potential and aggregation of human platelets in blood plasma. Biochem Biophys Res Commun. 1981 Oct 15;102(3):832–837. doi: 10.1016/0006-291x(81)91613-2. [DOI] [PubMed] [Google Scholar]
- Fukushima Y., Hagiwara S., Henkart M. Potassium current in clonal cytotoxic T lymphocytes from the mouse. J Physiol. 1984 Jun;351:645–656. doi: 10.1113/jphysiol.1984.sp015268. [DOI] [PMC free article] [PubMed] [Google Scholar]
- HODGKIN A. L., HUXLEY A. F. Currents carried by sodium and potassium ions through the membrane of the giant axon of Loligo. J Physiol. 1952 Apr;116(4):449–472. doi: 10.1113/jphysiol.1952.sp004717. [DOI] [PMC free article] [PubMed] [Google Scholar]
- Hallam T. J., Rink T. J. Responses to adenosine diphosphate in human platelets loaded with the fluorescent calcium indicator quin2. J Physiol. 1985 Nov;368:131–146. doi: 10.1113/jphysiol.1985.sp015850. [DOI] [PMC free article] [PubMed] [Google Scholar]
- Hamill O. P., Marty A., Neher E., Sakmann B., Sigworth F. J. Improved patch-clamp techniques for high-resolution current recording from cells and cell-free membrane patches. Pflugers Arch. 1981 Aug;391(2):85–100. doi: 10.1007/BF00656997. [DOI] [PubMed] [Google Scholar]
- Horne W. C., Norman N. E., Schwartz D. B., Simons E. R. Changes in cytoplasmic pH and in membrane potential in thrombin-stimulated human platelets. Eur J Biochem. 1981 Nov;120(2):295–302. doi: 10.1111/j.1432-1033.1981.tb05703.x. [DOI] [PubMed] [Google Scholar]
- Knight D. E., Scrutton M. C. Direct evidence for a role for Ca2+ in amine storage granule secretion by human platelets. Thromb Res. 1980 Nov 15;20(4):437–446. doi: 10.1016/0049-3848(80)90282-0. [DOI] [PubMed] [Google Scholar]
- Lindau M., Fernandez J. M. IgE-mediated degranulation of mast cells does not require opening of ion channels. Nature. 1986 Jan 9;319(6049):150–153. doi: 10.1038/319150a0. [DOI] [PubMed] [Google Scholar]
- MacIntyre D. E., Rink T. J. The role of platelet membrane potential in the initiation of platelet aggregation. Thromb Haemost. 1982 Feb 26;47(1):22–26. [PubMed] [Google Scholar]
- Maruyama Y. Ca2+-induced excess capacitance fluctuation studied by phase-sensitive detection method in exocrine pancreatic acinar cells. Pflugers Arch. 1986 Nov;407(5):561–563. doi: 10.1007/BF00657517. [DOI] [PubMed] [Google Scholar]
- Maruyama Y., Petersen O. H., Flanagan P., Pearson G. T. Quantification of Ca2+-activated K+ channels under hormonal control in pig pancreas acinar cells. Nature. 1983 Sep 15;305(5931):228–232. doi: 10.1038/305228a0. [DOI] [PubMed] [Google Scholar]
- Matteson D. R., Deutsch C. K channels in T lymphocytes: a patch clamp study using monoclonal antibody adhesion. Nature. 1984 Feb 2;307(5950):468–471. doi: 10.1038/307468a0. [DOI] [PubMed] [Google Scholar]
- Neher E., Marty A. Discrete changes of cell membrane capacitance observed under conditions of enhanced secretion in bovine adrenal chromaffin cells. Proc Natl Acad Sci U S A. 1982 Nov;79(21):6712–6716. doi: 10.1073/pnas.79.21.6712. [DOI] [PMC free article] [PubMed] [Google Scholar]
- Pipili E. Platelet membrane potential: simultaneous measurement of diSC3(5) fluorescence and optical density. Thromb Haemost. 1985 Oct 30;54(3):645–649. [PubMed] [Google Scholar]
- Rink T. J., Smith S. W., Tsien R. Y. Cytoplasmic free Ca2+ in human platelets: Ca2+ thresholds and Ca-independent activation for shape-change and secretion. FEBS Lett. 1982 Nov 1;148(1):21–26. doi: 10.1016/0014-5793(82)81234-9. [DOI] [PubMed] [Google Scholar]
- Sandler W. C., Le Breton G. C., Feinberg H. Movement of sodium into human platelets. Biochim Biophys Acta. 1980 Aug 4;600(2):448–455. doi: 10.1016/0005-2736(80)90447-2. [DOI] [PubMed] [Google Scholar]
- Schlichter L., Sidell N., Hagiwara S. Potassium channels mediate killing by human natural killer cells. Proc Natl Acad Sci U S A. 1986 Jan;83(2):451–455. doi: 10.1073/pnas.83.2.451. [DOI] [PMC free article] [PubMed] [Google Scholar]
- Weiss H. J. Platelet physiology and abnormalities of platelet function (first of two parts). N Engl J Med. 1975 Sep 11;293(11):531–541. doi: 10.1056/NEJM197509112931105. [DOI] [PubMed] [Google Scholar]
- Ypey D. L., Clapham D. E. Development of a delayed outward-rectifying K+ conductance in cultured mouse peritoneal macrophages. Proc Natl Acad Sci U S A. 1984 May;81(10):3083–3087. doi: 10.1073/pnas.81.10.3083. [DOI] [PMC free article] [PubMed] [Google Scholar]
- ZIEVE P. D., GAMBLE J. L., Jr, JACKSON D. P. EFFECTS OF THROMBIN ON THE POTASSIUM AND ATP CONTENT OF PLATELETS. J Clin Invest. 1964 Nov;43:2063–2069. doi: 10.1172/JCI105080. [DOI] [PMC free article] [PubMed] [Google Scholar]